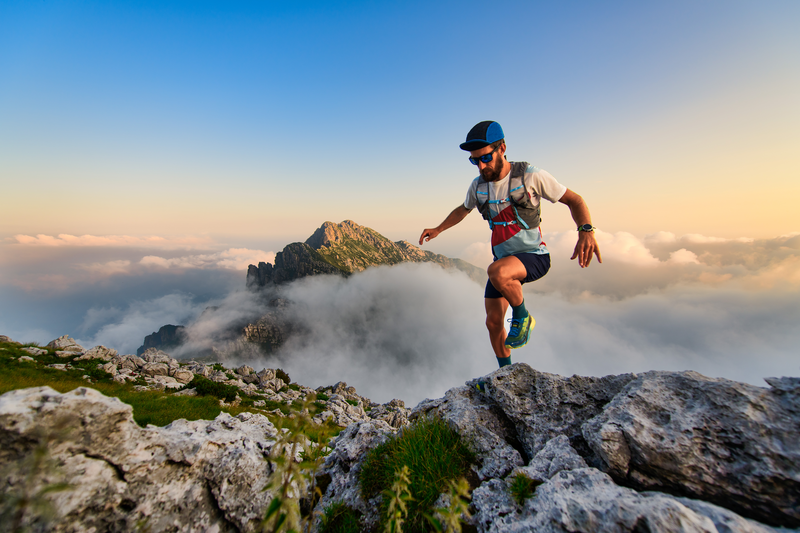
94% of researchers rate our articles as excellent or good
Learn more about the work of our research integrity team to safeguard the quality of each article we publish.
Find out more
ORIGINAL RESEARCH article
Front. Cardiovasc. Med. , 08 September 2022
Sec. Cardio-Oncology
Volume 9 - 2022 | https://doi.org/10.3389/fcvm.2022.930797
This article is part of the Research Topic Cardio-Oncology: Mechanisms and therapeutics View all 40 articles
A correction has been applied to this article in:
Corrigendum: Immune checkpoint inhibitor therapy increases systemic SDF-1, cardiac DAMPs Fibronectin-EDA, S100/Calgranulin, galectine-3, and NLRP3-MyD88-chemokine pathways
Background: Immune checkpoint inhibitors (ICIs) have significantly changed the oncology clinic in recent years, improving survival expectations in cancer patients. ICI therapy have a broad spectrum of side effects from endocrinopathies to cardiovascular diseases. In this study, pro-inflammatory and pro-fibrotic effects of short-term ICIs therapy in preclinical models were analyzed.
Methods: Firstly, in a human in vitro model, human cardiomyocytes co-cultured with hPBMC were exposed to ICIs (with CTLA-4 or PD-1 blocking agents, at 200 nM) for 72 h. After treatment, production of DAMPs and 12 cytokines were analyzed in the supernatant through colorimetric and enzymatic assays. C57/Bl6 mice were treated with CTLA-4 or PD-1 blocking agents (15 mg/kg) for 10 days. Before (T0), after three days (T3) and after treatments (T10), ejection fraction, fractional shortening, radial and longitudinal strain were calculated by using bidimensional echocardiography (Vevo 2100, Fujfilm). Fibrosis, necrosis, hypertrophy and vascular NF-kB expression were analyzed through Immunohistochemistry. Myocardial expression of DAMPs (S100- Calgranulin, Fibronectin and Galectine-3), MyD88, NLRP3 and twelve cytokines have been analyzed. Systemic levels of SDF-1, IL-1β, and IL-6 were analyzed before, during and after ICIs therapy.
Results: Radial and longitudinal strain were decreased after 10 days of ICIs therapy. Histological analysis of NF-kB expression shows that short-term anti-CTLA-4 or anti-PD-1 treatment increased vascular and myocardial inflammation. No myocardial hypertrophy was seen with the exception of the pembrolizumab group. Myocardial fibrosis and expression of galectin-3, pro-collagen 1-α and MMP-9 were increased after treatment with all ICIs. Both anti-CTLA-4 or anti-PD-1 treatments increased the expression of DAMPs, NLRP3 inflammasome and MyD88 and induced both in vitro and in vivo the secretion of IL-1β, TNF-α and IL-6. Systemic levels of SDF-1, IL-1β and IL-6 were increased during and after treatment with ICIs.
Conclusions: Short therapy with PD-1 and CTLA-4 blocking agents increases vascular expression of NF-kB, systemic SDF-1, IL-1β, IL-6 levels and myocardial NLRP3, MyD88 and DAMPs expression in preclinical models. A pro-inflammatory cytokine storm was induced in myocardial tissues and in cultured cardiac cells after ICIs therapy. The overall picture of the study suggests new putative biomarkers of ICIs-mediated systemic and myocardial damages potentially useful in clinical cardioncology.
Immune checkpoint inhibitors (ICIs) includes monoclonal antibodies that activate the host immune system for efficient killing of cancer cells through unspecific activation mechanisms (1). ICIs are directed against programmed cell death protein (PD-1), its associated ligand (PD-L1) or CTLA-4 (Cytotoxic T-Lymphocyte Antigen 4) leading to activation of lymphocytes and NK cell activity against cancer cells (2, 3). Clinical benefits were seen in melanoma, non-small cell lung cancer and metastatic breast cancer patients; association therapies with radiotherapy or chemotherapy or with other ICIs are still proposed worldwide (2). ICIs-mediated side effects involves T-lymphocyte-driven inflammation and direct cytotoxicity in many tissues, such as skin, intestine, lungs, liver, endocrine organs and cardiovascular system (4, 5). ICIs mediated cardiotoxicities are rare but can affect anticancer regimens and quality of life in cancer patients (6). Cardiovascular complications in cancer patients treated with ICIs include fatal myocarditis, vasculitis, arrhythmia, fibrosis and heart failure (7, 8). In different types of cancer, different ICIs may show different cardiotoxicity spectra (9). The incidence of ICIs-related cardiovascular events ranged from 0.15 to 10%. For example, in melanomas, PD-1/PD-L1 inhibitor use was closely related to high blood pressure and myocarditis (8, 9). Lung cancer patients, commonly experienced acute coronary syndrome, arrhythmia and heart failure (9). The most common cardiotoxic events after nivolumab and pembrolizumab therapy in lung cancer patients are arrhythmia, cardiac-related chest pain, cardiomyopathy, and myopericardial diseases. Moreover, renal cell carcinomas patients treated with nivolumab associated to ipilimumab frequently experienced hypertension (9); patients with urothelial carcinoma treated with atezolizumab had frequently hypertension and arrhythmia. Therefore, a complex interaction between cancer-related and immune-related factors plays a key role in pathogenesis of cardiovascular toxicities.
Known mechanisms of ICIs-induced cardiotoxicity involves immune-infiltration of CD3 +, CD4 + and CD8 + T lymphocytes in myocardial tissues that can attack cardiomyocytes or endothelial cells leading to metabolic failure and reduced cell viability (10, 11). Notably, ICIs can induce a pro-inflammatory phenotype in cardiac and vascular tissues therefore the identification of new players of cardiotoxicity mediated by short-term ICIs therapy still need further attention. Recent consensus statements highlights on the importance of new predictive biomarkers of ICIs-mediated cardiotoxicity (12, 13). Indeed, echocardiographic biomarkers such as changes in global longitudinal strain (GLS) (12), or myocardial work (MW) (13) or increases in plasma levels of galectin-3 and cytokines (14) are of great interest in clinical cardioncology. Considering that Pembrolizumab and Ipilimumab recognize both human and mice PD-1 and CTLA-4 epitopes (15, 16), we have highlighted on the vascular and myocardial inflammation in female mice through immunohistochemistry and ELISA methods shedding light on potential pathways involved in ICIs cardiotoxicity, including NLRP3, MyD88 and DAMPs.
Human cardiac cells (HFC cell line; Innoprot, Derio, Spain) were cultured following the manufacturer's instructions (17, 18). Culture medium was supplemented with Fetal Bovine Serum at 10% v/V (FBS, Sigma Aldrich, St. Louis MO, USA), Penicillin at 50 U/ml, Streptomycin at50 μg/mL and L-Glutammine at 1% v/V.
Considering that PD-1 and CTLA-4 are expressed in human cardiomyocytes, as recently analyzed by our group through cell ELISA assays on human fetal cardiomyocytes with anti-PD-1 and anti-CTLA-4 mAbs (19), Ipilimumab and Pembrolizumab were added to HFC cells co-cultured with human lymphocytes. In brief, cells were plated in 96-well flat-bottom plates (at 10,000 cells/well) for 16 h. Human Peripheral Blood Mononuclear Cells (hPBMCs) were added at effector: target ratio 5:1 in the absence or presence of the mAbs (200 nM), and incubated for 24 h at 37°C, as previously described (20). Cells un-incubated or incubated with aspecific antibodies IgG were defined as control. After treatment with antibodies, lymphocytes were removed and adherent cells were washed and counted through trypan blue method. Lymphocytes can be easily removed by collecting the supernatant of co-cultures and by washing the tumor adherent cells as the lymphocytes are non-adherent. Cell survival was expressed as percent of viable cells tested with drugs compared to the untreated ones, used as a negative control. Cardiac cell lysis was determined as described in other recent work (21, 22) through the quantification of released LDH (LDH detection kit, Thermo-Fisher Scientific, Meridian Rd., Rockoford, IL USA), following the manufacturer's instructions.
The Enzyme-linked immunosorbent assay (ELISA) was performed on mouse PBMCs and purified recombinant target proteins to test the human-mouse cross-reactivity of the antibodies. Mouse lymphocytes (4 × 105 cells/well) activated with anti-CD3/CD28 beads for 3 days were plated on round-bottom 96-well plates. The purified recombinant human or mouse CTLA-4/Fc or the Fc portion (used as a negative control) were coated on flat bottom plates and blocked with a buffer solution (PBS/milk 5% v/v) for 1 h. The plates were incubated with increasing concentrations of antibodies in a buffer solution (PBS/milk 2.5% v/v) for 2 h. After washing, plates were incubated with HRP-conjugated anti-human IgG (Fab')2 goat monoclonal antibody in a buffer solution (PBS/BSA 3% v/v) for 1 h. The following steps were performed as previously described (23). The Absorbance values at 450 nm were measured by an Envision plate reader (Perkin Elmer, 2102, San Diego, CA, USA).
Twenty four C57Bl/6 mice (female, 6 weeks/age) were purchased from Harlan, San Pietro al Natisone (Italy). As standard protocol, firstly mice were housed and maintained on a 12 h light-12 h dark cycle in a room with a fixed temperature of 22°C with the appropriate foods water. The experimental protocols were approved by the Ministry of Health with authorization number 1467/17-PR of 13-02-2017, and institutional ethics committees: Organismo preposto al benessere degli animali (OPBA) in accordance with EU Directive 2010/63/EU for animal experiments and Italian D.L.vo 26/2014 law. Briefly, animals were randomly divided into three groups (6 mice per group) as followings:
• Control group: mice daily received normal saline injection (by i.p) every three days for 10 days
• Ipilimumab group: mice received a short therapy with a CTLA-4 blocking agent (Bristol-Myers Squibb, Princeton, New Jersey, US) (15 mg/kg/day by i.p) every three days for 10 days;
• Pembrolizumab group: mice received a short therapy with a PD-1 blocking agent (Merck & Co., Inc., Kenilworth, NJ, USA) (15 mg/kg/day by i.p) every 3 days for 10 days.
Notably, every drug was used in the clinically available formulation. The injected dose of 15 mg/kg mean body-weight was comparable to the uses of ICIs in clinical oncology (16, 17, 24–26); moreover, the dose used is within the range of doses administered intraperitoneally (1–30 mg/kg) in preclinical models for pharmacokinetic studies and anticancer studies with ICIs (15, 27, 28).
To assess cardiac functions, the transthoracic echocardiography method was made in mice by using the Vevo 2100 (40-MHz transducer; Visual Sonics, Toronto, ON, Canada) which allows the determination of different cardiac function parameters in anesthetized mice (18, 29, 30). In brief, before (T0), after three days (T3) and at the end of treatments (T10) mice were prepared for cardiac function assessment by previous anesthesia through a solution composed by tiletamine zolazepam (both at 0,09 mg/g of weight) and atropine (at 0,04 mL/g of weight). The left ventricular echocardiography was performed in parasternal long-axis views (with a frame rate corresponding to 233 Hz). Firstly, in M-mode assessment, the left ventricular internal dimensions in diastole and a systole (LVID,d; LVID, s)were calculated from 3 to 5 beats. Fractional shortening and ejection fraction percentage (both in percentage) were determined as described in other recent work (15). Moreover, radial (RS, corresponding to the change in myocardial wall thickness) and longitudinal strain (LS, percent change in length of the ventricle) were calculated on long-axis views following the guide instructions of the Vevo 2100 software (15, 29).
After treatments, mice were anesthetized as described in section echocardiographic evaluation of ventricular functions and sacrificed via cervical dislocation. Hearts were excised, washed three times in physiological solution (to eliminate blood residues), weighed and divided into two parts through a proper longitudinal cut. One half was used for biochemical studies and the other part to histological analyzes. Firstly, hearts were lysed under ice in a lysis solution consisting of Triton × 100 1% V / v spiked with a protease inhibitor. To promote lysis, tissues were treated with ultrasounds for 5 min. After centrifugation at 4°C at 1,300 rpm for 10 min, the supernatant of the cardiac homogenates were used to quantitative analysis of six biomarkers of cardiac damages and inflammation, such as: myeloid differentiation primary response 88 (MYd-88) expression (through mouse MyD88 ELISA Kit (My Biosource, San Diego, CA, detection range of 78–5,000 pg/ml; sensitivity: 46.9 pg/ml); NOD-, LRR- and pyrin domain-containing protein 3 (NLRP-3) (through mouse NLRP3 ELISA Kit (OKEH05486, Aviva Systems Biology); Fibronectin-EDA, S100/Calgranulin and Galectine-3 [three DAMPs (quantified in cardiac tissues through selective quantitative assay); twelve cytokines and growth factors (IL-1α, IL-1β, IL-2, IL-4, IL-6, IL-10, IL-12, IL17-α, IFN-γ, TNF-α, G-CSF, GM-CSF) through a mouse cytokine Multiplex Assay kit (Qiagen, USA, pg/mg of heart tissue).
For systemic analysis, blood samples were collected in three points: before (T0), after three days (T3) and at the end of treatments (T10) in heparinised tubes and immediately centrifuged at 3,000 rpm for 10 min at 4°C in order to obtain plasma that was collected, frozen, and kept at −80°C until use. Circulating SDF-1 (CXCL-12), IL-1β, IL-6 were quantified in 0,1 mL of plasma through the use of mouse CXCL12/SDF-1 alpha Quantikine ELISA Kit (MCX120, R&D Systems, Minneapolis, MN, USA), mouse IL-1β ELISA kit (BMS6002, Thermo Fisher, Milan, Italy) and mouse IL-6 ELISA kit (KMC0061, Thermo Fisher, Milan, Italy).
Blinded histological examination of myocardial tissues were also performed. All selected samples were fixed in formalin and embedded in paraffin. Firstly, tissues were deparaffinized in a solution of xylene and rehydrated through graded alcohols. Antigen retrieval was performed with slides heated in 0.0.1 M edta buffer (pH 8.0.) for 10 min at 110 °C. Slides were rinsed with TBS and treated with a solution at 3 % v/V hydrogen peroxide. Another washing in BSA 5% v/V in PBS was performed as blocking step and an incubation for 12h with a primary antibody (diluted 1:100 in PBS) against mouse NF-kB (Abcam, Cambridge, UK) was performed. Sections were incubated with goat anti- anti-rabbit secondary IgG biotinylated secondary antibody for 0.5 h. Tissue reactivity was determined through the avidin–biotin–peroxydase method (Novocastra, Newcastle, UK) as described in other work (30). After, sections were counterstained with haematoxylin. To determine the structure of the heart, and to evaluate parameters such as hypertrophy, necrosis and fibrosis, the tissues of the heart were incubated with Mayer's hematoxylin for 30 s and washed properly with water (30). Antigen expression was evaluated by one experienced pathologist by using light microscopy. For NF-kB nuclear localization in vascular endothelium of the murine tissues under examination was considered. Immunostaining values were reported as percentage of positive cells in 10 non-overlapping fields by using magnification X400 (31).
Data are presented as means ± standard errors (SE). All data were tested for normality by Shapiro–Wilk. Normally distributed data in two groups were tested with Student's t-test, and non-normally distributed data in two groups by Wilcoxon–Mann–Whitney. Normally distributed data in multiple groups were tested by one-way analysis of variance (ANOVA) with Sidak correction. Non-normally distributed data were tested by ANOVA with Holm–Sidak post-testing. Paired data were tested using the paired versions of t-Student.
We first verified the immunoreactivity of Pembrolizumab and Ipilimumab compared to murine epitopes of PD-1 and CTLA-4, respectively, through ELISA binding assays. As specified in Figure 1, and in line with other recent research (15, 22), Pembrolizumab and Ipilimumab are both able to bind to the mouse targets even though the affinity is lower than that observed for their human counterparts. Cardiotoxicity of Pembrolizumab and Ipilimumab in human in vitro models of co-cultures of hPBMC and human cardiomyocytes. To confirm in a human-like environment the results obtained in mouse models on the cardiotoxic effects of the immunomodulatory mAbs, we tested their effects in in vitro human models based on co-cultures of human cardiomyocytes (HFC) and lymphocytes. As shown in Figure 1, three mAbs induced a significant cardiac cell lysis (up to 50% for Pembrolizumab), thus indicating that they can indeed activate immune responses against cardiac cells.
Figure 1. Binding assays of Pembrolizumab and Ipilimumab on mouse PBMCs and on human and mouse purified recombinant target proteins. ELISA assays were performed by using the indicated antibodies at increasing concentrations on mouse (black bars) CTLA-4/Fc or PD-1/Fc (A,B). The binding of antibodies was also tested on the Fc portion (empty bars), used as a negative control. Cell ELISA assays were performed by testing Pembrolizumab (C) (n = 3) or Ipilimumab (D) (n = 3) on mouse PBMCs untreated or activated with anti-CD3/CD28 beads. (E) LDH assay on the supernatant of co-cultures of HFC and hPBMCs treated as indicated (n = 3). Cell lysis was measured as described in the materials and methods section. Error bars depict means ± SD.
It was verified if ICIs could affect the production of DAMPs, pro-inflammatory cytokines, chemokines, and growth factors in co-cultures of human cardiomyocytes with human peripheral blood mononuclear cells (hPBMCs) by analyzing the supernatant by ELISA assays. Firstly, in a similar fashion to the in vivo findings, all ICIs increased the production of Fibronectin-EDA (Figure 2A), S199/Calgranulin (Figure 2B) and Galectine-3 (Figure 2C) compared to untreated cells. However, the analysis of cytokines secretion indicated that only IL-1α, IL-1β, IL-6, and TNF-α were significantly increased after incubation with ICIs (Figure 2D).
Figure 2. (A) DAMPs, including fibronectin, (B) S100 calgranulin, (C) galectin-3, and (D) cytokines and growth factors produced by cardiomyocytes in the supernatant of co-cultures of cardiomyocytes with hPBMC. The cytokines IL-1α, IL-1β, IL-2, IL-4, IL-6, IL-10, IL-12, IL17-α, IFN-γ, TNF-α, G-CSF, GM-CSF (n = 3) were quantified by ELISA assays. Error bars depict means ± SD.
We determined the cardiotoxic effects of PD-1 and CTLA-4 blocking agents in C57Bl/6 mice through the study of FS, EF, RS, and LS by using two-dimensional echocardiography (Vevo Strain 2100, Fujifilm). Analysis of EF and FS indicated that short term of ICIs therapies significantly reduces the cardiac function (Figure 3). No differences were seen between groups. Instead, more significant reductions were observed for radial and longitudinal strain in ICIs groups vs. control. Again, no differences between the ICIs were seen (Figure 3).
Figure 3. Cardiac function studies. Before (T0), after 3 days (T3) and at the end of treatments (T10) with saline or Ipilimumab or Pembrolizumab (15 mg/kg/die), cardiac functions studies were performed. Ejection fraction (%) (n = 6), fractional shortening (%) (n = 6), radial (n = 6) and longitudinal strain (%) (n = 6) were analyzed through VEVO 2100 echocardiography. Error bars depict means ± SD.
Analysis of vascular and myocardial NF-kB expression shows that short-term anti-CTLA-4 or anti-PD-1 treatment had differential effects (Figure 4). However, first, both ICIs increased significantly the vascular inflammation compared to untreated mice but the higher NF-kB expression was seen in Pembrolizumab compared to Ipilimumab group (Figure 4A). Histological characterization of the myocardial tissue phenotype confirms no detectable vascular NF-kB expression in untreated mice (Figure 4B) but a strong vascular expression was seen in ICIs groups (see arrows in Figures 2C,D).
Figure 4. Endothelial NF-kB expression in myocardial tissues of mice treated with ICIs (A) Quantitative analysis of NF-kB endothelial expression (%) in mice untreated or treated with ICIs (n = 6). Vascular expression of NF-kB in mice untreated (B) or treated with Ipilimumab (C) or Pembrolizumab (D).
Morphological characterization of the myocardial tissue phenotype in mice after short-term anti-CTLA-4 or anti-PD-1 treatment clearly indicates no cardiac hypertrophy with the exception of Pembrolizumab (Figure 5). Compared to control (sham), ematoxylin-eosin staining of longitudinal sections of mice treated with Ipilimumab did not show any significant hypertrophy; cardiomyocytes have a linear and homogeneous longitudinal aspect, the nucleus is not pycnotic and has a regular size and shape, and there are no cytoplasmic vacuoles. On the other hand, the cardiac longitudinal section of mice treated with Pembrolizumab shows a significant hypertrophy with evident increases in the cytoplasmic volume and an irregular course of the cardiomyocytes (Figure 5).
Figure 5. Hypertrophy analysis in myocardial tissues of mice treated with ICIs. (A) Hematoxylin-eosin staining of longitudinal sections with no hypertrophy in control untreated mice (x40); (B) hematoxylin-eosin staining of longitudinal sections with no hypertrophy in untreated mice and mice treated with Ipilimumab (×40); (C) hematoxylin-eosin staining of longitudinal sections highlights the development of marked hypertrophy in mice treated with Pembrolizumab (×40).
Histological characterization of the myocardial tissue phenotype in mice after short-term anti-CTLA-4 or anti-PD-1 treatment showed that ICIs increased cardiac fibrosis compared to untreated mice (Figures 6A–C). In control group (Figure 6A) hematoxylin-eosin staining of longitudinal sections evidenced the absence of fibrosis, whereas treatment with Ipilimumab or Pembrolizumab (Figures 6B,C) increased drastically the fibrotic phenotype. Myocardial expression of galectin-3 (Figure 6D), mouse pro-collagen 1-α (Figure 6E) and MMP-9 (Figure 6F), biomarkers of fibrosis, corroborates these findings: galectin-3 is almost undetectable in control group while it is drastically increased after treatment with all ICIs (1.7 ± 1.2 vs. 24.5 ± 4.2 vs. 32.3 ± 4.3 ng/mg of tissue for control, Ipilimumab and Pembrolizumab, respectively; p < 0.001 for control vs. ICIs). Similar results were seen for procollagen 1α1 (3.4 ±1.2 vs. 15.3 ± 3.1 vs. 16.8 ± 1.2 ng/mg of protein for control, Ipilimumab and Pembrolizumab, respectively; p < 0.005 for control vs. ICIs) and MMP-9 (407.8 ± 89.6 vs. 732.5 ± 102.2 vs. 895.5 ± 88.6 pg/mg of protein for control, Ipilimumab and Pembrolizumab, respectively; p < 0.05 for control vs. ICIs).
Figure 6. Histological analysis of the hearts of mice untreated or treated with ICIs. (A) Hematoxylin-eosin staining of longitudinal sections in control untreated mice (x40); (B) hematoxylin-eosin staining of longitudinal sections in mice treated with Ipilimumab (×40); (C) hematoxylin-eosin staining of longitudinal sections with fibrosis in mice treated with Pembrolizumab (×40). (D) Cardiac expression of galectin-3 (ng/mg of tissue), (E) mouse pro-collagen 1-α (ng/mg of total proten), (F) MMP-9 (pg/mg of protein), (G) Fibronectin EDA (fold of control), (H) S100 Calgranulin (ng/mg of protein in myocardial tissue lysate), (I) MyD-88 (pg/mg of protein in myocardial tissue lysate) and (J) NLRP-3 (pg/mg of protein in myocardial tissue lysate) after short-term treatment with ICIs. In (K), Twelve cytokines (IL-1α, IL-1β, IL-2, IL-4, IL-6, IL-10, IL-12, IL17-α, IFN-γ, TNF-α, G-CSF, GM-CSF) were analyzed in heart lysates and reported as pg of cytokine normalized for mg of tissue. Error bars depict means ± SD (n = 6).
We investigated on cardiac markers of inflammation and cell damages (DAMPs). Compared to untreated mice, ICIs increased significantly Fibronectin-EDA expression (Figure 6G) in heart lysates (2.3 ± 0.16 for Ipilimumab and 2.1 ± 0.25 for Pembrolizumab; p < 0.001 for control vs. ICIs). Similarly another DAMP, called S100 Calgranulin (Figure 6H) was significantly enhanced by ICIs treatment (1.86 ± 0.21 for Ipilimumab and 1.96 ± 0.24 ng/mg of protein for Pembrolizumab; p < 0.001 for control vs. ICIs); no significant differences between ICIs were seen. Inflammasome and myddosome complex stimulates DAMPs, therefore, myocardial expression of NLRP type 3 and MyD type 88 were analyzed. A drastic increase in MyD-88 expression (Figure 6I) (33.2 pg/mg of protein ± 15.6 for untreated mice:198.6 ± 18.3 for Ipilimumab; 217.5 ± 17.4 for Pembrolizumab; p < 0.005 for ICIs vs. control). The same behavior was seen for NLRP3 expression after treatment with all ICIs (47.3 pg/mg of protein ± 13.5 for untreated mice: 132.1 ±15.1 for Ipilimumab; 126.6 ± 11.2 for Pembrolizumab; p < 0.005 for ICIs vs. control) (Figure 6J).
Cytokines and chemokines are drivers of anticancer drug-induced cardiotoxicity, heart failure and myocarditis (32). Therefore, cytokines and chemokines in heart tissue of Ipilimumab or Pembrolizumab-treated female C57Bl6 mice were quantified (Figure 6K). Firstly, the family of IL-1 cytokines (IL-1α and IL-1β), increased in all ICIs treated group with respect to untreated mice (p < 0.001). IL-2 levels were also increased in ICIs group, highlighting immune-related reactions in myocardial tissue. Anti-inflammatory cytokines levels (IL-4 and IL-10) were reduced in ICIs group vs. untreated mice. Other pro-inflammatory cytokines (IL-6, IL17-α, IFN-γ, and TNF-α) were also increased in myocardial tissues of ICIs-treated mice vs. saline-treated groups. Levels of growth factors involved in heart failure and hypertrophy (G-CSF and GM-CSF) were also increased in ICIs groups (Figure 6K).
High plasma levels of Stromal Cell-Derived Factor 1 (SDF-1), IL-1β and IL-6 were associated to cardiac inflammation, heart failure and cardiovascular mortality (33). We analyzed if short term ICIs therapy could affect SDF-1, IL-1 β and IL-6 levels in plasma of C57/Bl6 mice (Figure 7). Before (T0), after three days (T3) and at the end of treatment (T10), systemic levels of all biomarkers were significantly increased with respect to control saline-treated mice (p < 0.05). For example, after 10 days of therapy, SDF-1 levels were 0.96 ng/ml ±0.24 for untreated mice; 2.75± 0.34 for Ipilimumab and 3.16 ± 0.29 for Pembrolizumab (p < 0.005 for ICIs vs. control). The same behavior was seen for IL-1 β and IL-6, indicating a systemic inflammation even after 3 days of treatment with ICIs (Figure 7).
Figure 7. Effects of ICI treatment on plasma levels of SDF-1 (CXCL-12), Interleukin 1-β and Interleukin-6. Female C57/Bl6 mice were treated daily with anti-CTLA-4 or anti-PD-1 antibodies (n = 6) or left untreated (n = 6) for 10 days. Before (T0), after three days (T3) and at the end of treatments (T10), SDF-1 (n = 6), Interleukin 1-β (n = 6) and Interleukin-6 (n = 6) were quantified in plasma. Levels were reported in ng/ml of plasma.
The current study aimed to evaluate the pro-inflammatory effects of short-term immune checkpoint inhibitors (ICIs) treatment in myocardial and vascular tissues in preclinical models (34, 35) (Figure 8). Notably, the use of ICIs in clinical oncology found significant clinical benefits in cancer patients, however, a wide spectrum of side effects are being seen (called irAEs): rash (maculopapular, lichenoid), diarrhea, colitis, mucositis, hypo or hyper thyroidism, hepatitis, inflammatory arthritis, myalgia (36). Endocrinopathies and inflammatory pathologies induced by PD-1 / PDL-1 or CTLA-4 blocking agents are frequently reported in both monotherapy and combinatorial therapies. Clinical studies report both short-term and long-term side effects in cancer patients and the mechanisms are not yet well-known (37). There are both immune-mediated and non-immune-mediated mechanisms involved in irAEs. A recent meta-analysis reports a high incidence of myocarditis (about 11 times higher than in other therapies) which reaches a mortality rate of about 50% in case of combination therapies (PD1 / PDL-1 associated to CTLA-4 blocking agents) (38, 39). Furthermore, ICIs have recently been shown to accelerate the process of atherosclerosis in both preclinical and clinical study models. Even just a short treatment with ICIs increases the inflammatory state in the vascular endothelium by accelerating the atherosclerotic process (39). Other recent studies show that short ICSi treatments can cause arrhythmias, Takotsubo syndrome and inflammatory vascular events (40, 41). Current data regarding ICIs- associated pericardial involvement are limited, but case-reports include pericarditis, pericardial effusion (42, 43). Notably, a deep knowledge of ICIs-induced myocardial injuries is needed. Immune cells uptake and infiltration in myocardial tissue were always seen in human histological studies (CD4, CD8 T cells and macrophages). Immune-related side effects involves several chemokines like CXCR 10, 9 and 3, high levels of granzyme B especially in myocardial tissue (44). Our data suggest that NLRP3 and MyD88 pathways could contribute to the increased vascular and myocardial inflammation of anti-CTLA-4/anti-PD-1 treatments. NLRP3 drives cytosolic damages, hypertrophy and inflammation through cytokines and overproduction of hs-CRP.
Figure 8. Short-term ICI Therapy increases DAMPs, NLRP3 and MyD-88 mediated fibrosis and vascular inflammation. Short-term treatment with immune checkpoint inhibitors (ICIs) induced significant increases in DAMPs, galectine-3, cytokines and chemokines through NLRP3 and MyD88 pathways inducing myocardial hypertrophy, fibrosis and strong vascular inflammation.
As described in Figure 6 and summarized in Figure 8, our data confirms that ICIs increases DAMPs in cardiomyocytes and myocardial tissue of mice models. It has been described that in patients with unstable angina or with AMI, endogenous DAMPs like Fibronectin-EDA, S100/Calgranulin, Galectine-3 are released from damaged cardiac cells and signal through TLR receptors. There is also emerging evidence for the involvement of Toll-like receptors type-9 in heart failure, which can be activated by endogenous DAMPs, including mitochondrial DNA, to modulate the progression of the disease (45, 46). The increases in DAMPs levels after short-term ICIs treatment in mice indicates myocardial injuries (46). Whether long-term ICI therapy affects myocardial stress and vascular inflammation is unknown. Nevertheless, our data suggest that even short-term ICIs therapy induces vascular inflammation, fibrosis and levels of myocardial NLRP3 and MyD88 (Figure 8). Whether ICIs- induced inflammation persist after cessation of the therapy and how they affect myocardial work in the long-term is not currently known (47).
This work has several limitations: firstly, the use of a tumor-free mice model. As cardiovascular diseases and cancer share many pathophysiological pathways, including inflammation, the use of a tumor-bearing model would have increased the translational potential of our study. Moreover, there are clinical evidences that combination ICIs-therapy exerts the most frequent and pro-inflammatory cardiac and endocrine side effects compared to monotherapies (48) therefore, further cardiotoxic studies in preclinical models will be performed after the combination of anti-CTLA4 and anti-PD-1/PD-L1.
Another limitation is based on the short period of treatment with ICIs without a longer follow up. In real world clinical experience, cancer patients experienced ICIs-mediated side effects also many months after therapy cessation (47, 49). These effects could be also partially related to endocrine changes due to PD-1/CTLA-4 blocking pathways that exerts a detrimental cardiotoxic effects in these patients. Therefore, further preclinical studies on long-term cardiovascular side effects after ICIs therapy will be performed.
Another methodological limitation of this work is the absence of a more proper control group in animal studies based on the administration of IgG control antibody, however, as reported in cellular experiments (Figure 8), any changes in pro-inflammatory and cell dead markers were seen after incubation with control IgG. Moreover, it is plausible that the intraperitoneal administration of nonspecific IgG as a control does not change neither cardiac functions nor cardiac and systemic inflammatory status in mouse models as confirmed by recent similar research papers (50, 51).
In conclusion, cardiotoxicity, although rare, is a clinically relevant problem in cancer patients undergoing ICIs. Long-term side effects of ICIs are reported, however, some biochemical changes may occur even a few days after treatment with ICIs, as specified in this work. The involvement of NLRP3 MyD-88 and some DAMPs in ICIs-associated cardiovascular disease was seen. Relevant histological effects such as cardiac endothelial inflammation and overexpression of pro-fibrotic and pro-inflammatory cytokines is a non-negligible fact that deserves further investigation. The increase in systemic levels of SDF-1, IL-1β and il-6 indicates systemic pro-inflammatory effects induced by ICIs that can directly and indirectly increase the risk of myocarditis, however more detailed studies on the mechanisms of systemic and direct cardiac toxicity will have to be carried out. Of note, considering that PD-1 and CTLA-4 blocking agents recognize the murine epitope with lower affinity than the human epitope, the effects observed in this work could also be underestimated (12) consequently, short and long term clinical studies during ICIs deserve urgent investigation. Moreover, the results of this study warrant further preclinical cardioprotective trials with anti-cytokine (52, 53), anti-NLRP3 (54–56) or anti-MyD88 (57, 58) therapies in primary or secondary prevention of ICIs-related cardiotoxicity.
This study suggests that short-term ICI therapy affects myocardial and vascular inflammation through DAMPs and cytokines through NLRP-3 and MyD-88 related pathways (Figure 8). It is plausible that ICIs exerts both systemic and cardiac toxicities through the activation of cytokines cascades that exacerbate the inflammatory damages in cardiomyocytes. These results are in line with another recent work (59) demonstrating pro-atherosclerotic effects of short-ICIs therapy in mice models. Clinical studies are required to elucidate the effects of ICIs on myocardial and vascular inflammation and confirms the role of NLRP3 and Myd88 in progression of ICIs-mediated cardiovascular diseases.
The data presented in the study are deposited in the Zenodo repository, accession number https://zenodo.org/record/7040431#.YyMLcYrP1D9.
The animal study was reviewed and approved by the experimental protocols, in accordance with EU Directive 2010/63/EU for animal experiments and Italian D.L.vo 26/2014 law, were approved by the Ministry of Health with authorization number 1467/17-PR of 13-02-2017, and institutional ethics committees: Organismo preposto al benessere degli animali (OPBA).
VQ, SB, MP, AD, CC, GG, AB, GP, and AL performed the experiments and analyzed the data. AP, IB, AI, and MC provided technical assistance. VQ, CD, and NM designed the study and drafted the manuscript. All authors read and approved the final manuscript.
This work was funded by a “Ricerca Corrente” grant from the Italian Ministry of Health titled “Cardiotossicità da chemioterapie, targeted therapies e immunoterapie, diagnosi precoce e cardioprotezione, Ricerca preclinica e clinica”.
Authors MP and CD were employed by Ceinge-Biotecnologie Avanzate.
The remaining authors declare that the research was conducted in the absence of any commercial or financial relationships that could be construed as a potential conflict of interest.
All claims expressed in this article are solely those of the authors and do not necessarily represent those of their affiliated organizations, or those of the publisher, the editors and the reviewers. Any product that may be evaluated in this article, or claim that may be made by its manufacturer, is not guaranteed or endorsed by the publisher.
1. Meric-Bernstam F, Larkin J, Tabernero J, Bonini C. Enhancing anti-tumour efficacy with immunotherapy combinations. Lancet. (2021) 397:1010–22. doi: 10.1016/S0140-6736(20)32598-8
2. Rotte A, Jin JY, Lemaire V. Mechanistic overview of immune checkpoints to support the rational design of their combinations in cancer immunotherapy. Ann Oncol. (2018) 29:71–83. doi: 10.1093/annonc/mdx686
3. Davis AA, Patel VG. The role of PD-L1 expression as a predictive biomarker: an analysis of all US Food and Drug Administration (FDA) approvals of immune checkpoint inhibitors. J Immunother Cancer. (2019) 7:278. doi: 10.1186/s40425-019-0768-9
4. Boutros C, Tarhini A, Routier E, Lambotte O, Ladurie FL, Carbonnel F, et al. Safety profiles of anti-CTLA-4 and anti-PD-1 antibodies alone and in combination. Nat Rev Clin Oncol. (2016) 13:473–86. doi: 10.1038/nrclinonc.2016.58
5. Yin G, Guo W, Huang Z, Chen X. Efficacy of radiotherapy combined with immune checkpoint inhibitors in patients with melanoma: a systemic review and meta-analysis. Melanoma Res. (2022) 32:71–8. doi: 10.1097/CMR.0000000000000800
6. Johnson DB, Nebhan CA, Moslehi JJ, Balko JM. Immune-checkpoint inhibitors: long-term implications of toxicity. Nat Rev Clin Oncol. (2022) 19:254–67. doi: 10.1038/s41571-022-00600-w
7. Aras MA, Power JR, Moslehi JJ. Inflammatory biomarkers to detect immune checkpoint inhibitor-associated cardiotoxicity in lung cancer patients: ready for prime time? JACC CardioOncol. (2020) 2:503–5. doi: 10.1016/j.jaccao.2020.08.001
8. Geraud A, Gougis P, Vozy A, Anquetil C, Allenbach Y, Romano E, et al. Clinical pharmacology and interplay of immune checkpoint agents: a yin-yang balance. Annu Rev Pharmacol Toxicol. (2021) 61:85–112. doi: 10.1146/annurev-pharmtox-022820-093805
9. Dong M, Yu T, Zhang Z, Zhang J, Wang R, Tse G, et al. ICIs-Related cardiotoxicity in different types of cancer. J Cardiovasc Dev Dis. (2022) 9:203. doi: 10.3390/jcdd9070203
10. Wei SC, Meijers WC, Axelrod ML, Anang NAS, Screever EM, Wescott EC, et al. A genetic mouse model recapitulates immune checkpoint inhibitor-associated myocarditis and supports a mechanism-based therapeutic intervention. Cancer Discov. (2021) 11:614–25. doi: 10.1158/2159-8290.CD-20-0856
11. Michel L, Helfrich I, Hendgen-Cotta UB, Mincu RI, Korste S, Mrotzek SM, et al. Targeting early stages of cardiotoxicity from anti-PD1 immune checkpoint inhibitor therapy. Eur Heart J. (2022) 43:316–29. doi: 10.1093/eurheartj/ehab430
12. Awadalla M, Mahmood SS, Groarke JD, Hassan MZO, Nohria A, Rokicki A, et al. Global longitudinal strain and cardiac events in patients with immune checkpoint inhibitor-related myocarditis. J Am Coll Cardiol. (2020) 75:467–78. doi: 10.1016/j.jacc.2019.11.049
13. Vaz Ferreira V, Mano TB, Cardoso I, Coutinho Cruz M, Moura Branco L, Almeida-Morais L, et al. Myocardial work brings new insights into left ventricular remodelling in cardio-oncology patients. Int J Environ Res Public Health. (2022) 19:2826. doi: 10.3390/ijerph19052826
14. Besler C, Lang D, Urban D, Rommel KP, von Roeder M, Fengler K, et al. Plasma and cardiac galectin-3 in patients with heart failure reflects both inflammation and fibrosis: implications for its use as a biomarker. Circ Heart Fail. (2017) 10:e003804. doi: 10.1161/CIRCHEARTFAILURE.116.003804
15. Quagliariello V, Passariello M, Rea D, Barbieri A, Iovine M, Bonelli A, et al. Evidences of CTLA-4 and PD-1 blocking agents-induced cardiotoxicity in cellular and preclinical models. J Pers Med. (2020) 10:179. doi: 10.3390/jpm10040179
16. Department of Health and Ageing Australian Government. Australian Public Assessment Report for IPILIMUMAB. Bristol-Myers Squibb Australia Pty Ltd.: Mulgrave, Australia (2011).
17. Lu L, Xu X, Zhang B, Zhang R, Ji H, Wang X, et al. Combined PD-1 blockade and GITR triggering induce a potent antitumor immunity in murine cancer models and synergizes with chemotherapeutic drugs. J Transl Med. (2014) 12:36. doi: 10.1186/1479-5876-12-36
18. Peng Y, Popovic ZB, Sopko N, Drinko J, Zhang Z, Thomas D, et al. Speckle tracking echocardiography in the assessment of mouse models of cardiac dysfunction. Am J Physiol Circ Physiol. (2009) 297:H811–20. doi: 10.1152/ajpheart.00385.2009
19. Vetrei C, Passariello M, Froechlich G, Rapuano Lembo R, Zambrano N, De Lorenzo C. Immunomodulatory mAbs as tools to investigate on Cis-Interaction of PD-1/PD-L1 on tumor cells and to set up methods for early screening of safe and potent combinatorial treatments. Cancers. (2021) 13:2858. doi: 10.3390/cancers13122858
20. Rea D, Coppola C, Barbieri A, Monti MG, Misso G, Palma G, et al. Strain analysis in the assessment of a mouse model of cardiotoxicity due to chemotherapy: sample for preclinical research. In Vivo. (2016) 30:279–90.
21. De Lorenzo C, Paciello R, Riccio G, Rea D, Barbieri A, Coppola C, et al. Cardiotoxic effects of the novel approved anti-ErbB2 agents and reverse cardioprotective effects of ranolazine. Onco Targets Ther. (2018) 11:2241–50. doi: 10.2147/OTT.S157294
22. Quagliariello V, Passariello M, Coppola C, Rea D, Barbieri A, Scherillo M, et al. Cardiotoxicity and pro-inflammatory effects of the immune checkpoint inhibitor Pembrolizumab associated to Trastuzumab. Int J Cardiol. (2019) 292:171–9. doi: 10.1016/j.ijcard.2019.05.028
23. Passariello M, Yoshioka A, Takahashi K, Hashimoto SI, Rapuano Lembo R, Manna L, et al. Novel bi-specific immuno-modulatory tribodies potentiate T cell activation and increase anti-tumor efficacy. Int J Mol Sci. (2022) 23:3466. doi: 10.3390/ijms23073466
24. Selby MJ, Engelhardt JJ, Johnston RJ, Lu L-S, Han M, Thudium K, et al. Preclinical development of ipilimumab and nivolumab combination immunotherapy: mouse tumor models, in vitro functional studies, and cynomolgus macaque toxicology. PLoS ONE. (2016) 11:e0161779. doi: 10.1371/journal.pone.0161779
25. Chang CY, Park H, Malone DC, Wang CY, Wilson DL, Yeh YM, et al. Immune checkpoint inhibitors and immune-related adverse events in patients with advanced melanoma: a systematic review and network meta-analysis. JAMA Netw Open. (2020) 3:e201611. doi: 10.1001/jamanetworkopen.2020.1611
26. Ji C, Roy MD, Golas J, Vitsky A, Ram S, Kumpf SW, et al. Myocarditis in cynomolgus monkeys following treatment with immune checkpoint inhibitors. Clin Cancer Res. (2019) 25:4735–48. doi: 10.1158/1078-0432.CCR-18-4083
27. Sehgal K, Costa DB, Rangachari D. Extended-interval dosing strategy of immune checkpoint inhibitors in lung cancer: will it outlast the COVID-19 pandemic? Front Oncol. (2020) 10:1193. doi: 10.3389/fonc.2020.01193
28. Patnaik A, Kang SP, Rasco D, Papadopoulos KP, Elassaiss-Schaap J, Beeram M, et al. Phase I study of pembrolizumab (MK-3475; anti-PD-1 monoclonal antibody) in patients with advanced solid tumors. Clin Cancer Res. (2015) 21:4286–93. doi: 10.1158/1078-0432.CCR-14-2607
29. Riccio G, Antonucci S, Coppola C, D'Avino C, Piscopo G, Fiore D, et al. Ranolazine attenuates Trastuzumab-induced heart dysfunction by modulating ROS production. Front Physiol. (2018) 9:38. doi: 10.3389/fphys.2018.00038
30. Quagliariello V, De Laurentiis M, Rea D, Barbieri A, Monti MG, Carbone A, et al. The SGLT-2 inhibitor empagliflozin improves myocardial strain, reduces cardiac fibrosis and pro-inflammatory cytokines in non-diabetic mice treated with doxorubicin. Cardiovasc Diabetol. (2021) 20:150. doi: 10.1186/s12933-021-01346-y
31. Saito T, Hasegawa Y, Ishigaki Y, Yamada T, Gao J, Imai J, et al. Importance of endothelial NF-κB signalling in vascular remodelling and aortic aneurysm formation. Cardiovasc Res. (2013) 97:106–14. doi: 10.1093/cvr/cvs298
32. Subramanian S, Liu C, Aviv A, Ho JE, Courchesne P, Muntendam P, et al. Stromal cell-derived factor 1 as a biomarker of heart failure and mortality risk. Arterioscler Thromb Vasc Biol. (2014) 34:2100–5. doi: 10.1161/ATVBAHA.114.303579
33. Tschöpe C, Ammirati E, Bozkurt B, Caforio ALP, Cooper LT, Felix SB, et al. Myocarditis and inflammatory cardiomyopathy: current evidence and future directions. Nat Rev Cardiol. (2021) 18:169–93. doi: 10.1038/s41569-020-00435-x
34. Wang DY, Okoye GD, Neilan TG, Johnson DB, Moslehi JJ. Cardiovascular toxicities associated with cancer immunotherapies. Curr Cardiol Rep. (2017) 19:21. doi: 10.1007/s11886-017-0835-0
35. Leach DR, Krummel MF, Allison JP. Enhancement of antitumor immunity by CTLA-4 blockade. Science. (1996) 271:1734–6. doi: 10.1126/science.271.5256.1734
36. llouchery M, Beuvon C, Pérault-Pochat MC, Roblot P, Puyade M, Martin M. Safety of immune checkpoint inhibitor resumption after interruption for immune-related adverse events, a narrative review. Cancers. (2022) 14:955. doi: 10.3390/cancers14040955
37. Patel RP, Parikh R, Gunturu KS, Tariq RZ, Dani SS, Ganatra S, et al. Cardiotoxicity of immune checkpoint inhibitors. Curr Oncol Rep. (2021) 23:79. doi: 10.1007/s11912-021-01070-6
38. Tocchetti CG, Galdiero MR, Varricchi G. Cardiac toxicity in patients treated with immune checkpoint inhibitors: it is now time for cardio-immuno-oncology. J Am Coll Cardiol. (2018) 71:1765–7. doi: 10.1016/j.jacc.2018.02.038
39. Mahmood SS, Fradley MG, Cohen JV, Nohria A, Reynolds KL, Heinzerling LM, et al. Myocarditis in patients treated with immune checkpoint inhibitors. J Am Coll Cardiol. (2018) 71:1755–64. doi: 10.1016/j.jacc.2018.02.037
40. Carbone A, Bottino R, Russo V, D'Andrea A, Liccardo B, Maurea N, et al. Takotsubo cardiomyopathy as epiphenomenon of cardiotoxicity in patients with cancer: a meta-summary of case reports. J Cardiovasc Pharmacol. (2021) 78:e20–9. doi: 10.1097/FJC.0000000000001026
41. Serzan M, Rapisuwon S, Krishnan J, Chang IC, Barac A. Takotsubo cardiomyopathy associated with checkpoint inhibitor therapy: endomyocardial biopsy provides pathological insights to dual diseases. JACC CardioOncol. (2021) 3:330–4. doi: 10.1016/j.jaccao.2021.02.005
42. Braden J, Lee JH. Immune checkpoint inhibitor induced pericarditis and encephalitis in a patient treated with Ipilimumab and Nivolumab for metastatic melanoma: a case report and review of the literature. Front Oncol. (2021) 11:749834. doi: 10.3389/fonc.2021.749834
43. Lal JC, Brown SA, Collier P, Cheng F. A retrospective analysis of cardiovascular adverse events associated with immune checkpoint inhibitors. Cardiooncology. (2021) 7:19. doi: 10.1186/s40959-021-00106-x
44. Johnson DB, Balko JM, Compton ML, Chalkias S, Gorham J, Xu Y, et al. Fulminant myocarditis with combination immune checkpoint blockade. N Engl J Med. (2016) 375:1749–55. doi: 10.1056/NEJMoa1609214
45. Yu L, Feng Z. The role of toll-like receptor signaling in the progression of heart failure. Mediators Inflamm. (2018) 2018:9874109. doi: 10.1155/2018/9874109
46. Christia P, Frangogiannis NG. Targeting inflammatory pathways in myocardial infarction. Eur J Clin Invest. (2013) 43:986–95. doi: 10.1111/eci.12118
47. Li C, Bhatti SA, Ying J. Immune checkpoint inhibitors-associated cardiotoxicity. Cancers. (2022) 14:1145. doi: 10.3390/cancers14051145
48. Agostinetto E, Eiger D, Lambertini M, Ceppi M, Bruzzone M, Pondé N, et al. Cardiotoxicity of immune checkpoint inhibitors: a systematic review and meta-analysis of randomised clinical trials. Eur J Cancer. (2021) 148:76–91. doi: 10.1016/j.ejca.2021.01.043
49. Zhang L, Reynolds KL, Lyon AR, Palaskas N, Neilan TG. The evolving immunotherapy landscape and the epidemiology, diagnosis, and management of cardiotoxicity: JACC: CardioOncology Primer. JACC CardioOncol. (2021) 3:35–47. doi: 10.1016/j.jaccao.2020.11.012
50. Giannoccaro MP, Menassa DA, Jacobson L, Coutinho E, Prota G, Lang B, et al. Behaviour and neuropathology in mice injected with human contactin-associated protein 2 antibodies. Brain. (2019) 142:2000–12. doi: 10.1093/brain/awz119
51. Kallikourdis M, Martini E, Carullo P, Sardi C, Roselli G, Greco CM, et al. T cell costimulation blockade blunts pressure overload-induced heart failure. Nat Commun. (2017) 8:14680. doi: 10.1038/ncomms14680
52. Ridker PM. Anticytokine agents: targeting interleukin signaling pathways for the treatment of atherothrombosis. Circ Res. (2019) 124:437–50. doi: 10.1161/CIRCRESAHA.118.313129
53. Quagliariello V, Paccone A, Iovine M, Cavalcanti E, Berretta M, Maurea C, et al. Interleukin-1 blocking agents as promising strategy for prevention of anticancer drug-induced cardiotoxicities: possible implications in cancer patients with COVID-19. Eur Rev Med Pharmacol Sci. (2021) 25:6797–812. doi: 10.26355/eurrev_202111_27124
54. Toldo S, Mauro AG, Cutter Z, Van Tassell BW, Mezzaroma E, Del Buono MG, et al. The NLRP3 inflammasome inhibitor, OLT1177 (Dapansutrile), reduces infarct size and preserves contractile function after ischemia reperfusion injury in the mouse. J Cardiovasc Pharmacol. (2019) 73:215–22. doi: 10.1097/FJC.0000000000000658
55. Murphy SP, Kakkar R, McCarthy CP, Januzzi JL Jr. Inflammation in heart failure: JACC state-of-the-art review. J Am Coll Cardiol. (2020) 75:1324–40. doi: 10.1016/j.jacc.2020.01.014
56. Quagliariello V, De Laurentiis M, Cocco S, Rea G, Bonelli A, Caronna A, et al. NLRP3 as putative marker of Ipilimumab-induced cardiotoxicity in the presence of hyperglycemia in estrogen-responsive and triple-negative breast cancer cells. Int J Mol Sci. (2020) 21:7802. doi: 10.3390/ijms21207802
57. Miao Y, Ding Z, Zou Z, Yang Y, Yang M, Zhang X, et al. Inhibition of MyD88 by a novel inhibitor reverses two-thirds of the infarct area in myocardial ischemia and reperfusion injury. Am J Transl Res. (2020) 12:5151–69.
58. Bayer AL, Alcaide P. MyD88: at the heart of inflammatory signaling and cardiovascular disease. J Mol Cell Cardiol. (2021) 161:75–85. doi: 10.1016/j.yjmcc.2021.08.001
Keywords: immunotherapy, biomarkers, preclinical study, mechanisms, inflammation, interleukin
Citation: Quagliariello V, Passariello M, Di Mauro A, Cipullo C, Paccone A, Barbieri A, Palma G, Luciano A, Buccolo S, Bisceglia I, Canale ML, Gallucci G, Inno A, De Lorenzo C and Maurea N (2022) Immune checkpoint inhibitor therapy increases systemic SDF-1, cardiac DAMPs Fibronectin-EDA, S100/Calgranulin, galectine-3, and NLRP3-MyD88-chemokine pathways. Front. Cardiovasc. Med. 9:930797. doi: 10.3389/fcvm.2022.930797
Received: 28 April 2022; Accepted: 03 August 2022;
Published: 08 September 2022.
Edited by:
Dong Han, People's Liberation Army General Hospital, ChinaReviewed by:
Cristina M. Failla, Institute of Immaculate Dermatology (IRCCS), ItalyCopyright © 2022 Quagliariello, Passariello, Di Mauro, Cipullo, Paccone, Barbieri, Palma, Luciano, Buccolo, Bisceglia, Canale, Gallucci, Inno, De Lorenzo and Maurea. This is an open-access article distributed under the terms of the Creative Commons Attribution License (CC BY). The use, distribution or reproduction in other forums is permitted, provided the original author(s) and the copyright owner(s) are credited and that the original publication in this journal is cited, in accordance with accepted academic practice. No use, distribution or reproduction is permitted which does not comply with these terms.
*Correspondence: Vincenzo Quagliariello, cXVhZ2xpYXJpZWxsby5lbnpvQGdtYWlsLmNvbQ==; Nicola Maurea, bi5tYXVyZWFAaXN0aXR1dG90dW1vcmkubmEuaXQ=
Disclaimer: All claims expressed in this article are solely those of the authors and do not necessarily represent those of their affiliated organizations, or those of the publisher, the editors and the reviewers. Any product that may be evaluated in this article or claim that may be made by its manufacturer is not guaranteed or endorsed by the publisher.
Research integrity at Frontiers
Learn more about the work of our research integrity team to safeguard the quality of each article we publish.