- 1School of Rehabilitation Medicine, Weifang Medical University, Weifang, China
- 2Department of Rehabilitation Medicine, Affiliated Hospital, Weifang Medical University, Weifang, China
- 3The Second Affiliated Hospital of Shandong University of Traditional Chinese Medicine, Jinan, China
- 4School of Basic Medicine, Weifang Medical University, Weifang, China
Normal endothelial function plays a pivotal role in maintaining cardiovascular homeostasis, while endothelial dysfunction causes the occurrence and development of cardiovascular diseases. Yes-associated protein (YAP) and its homolog transcriptional co-activator with PDZ-binding motif (TAZ) serve as crucial nuclear effectors in the Hippo signaling pathway, which are regulated by mechanical stress, extracellular matrix stiffness, drugs, and other factors. Increasing evidence supports that YAP/TAZ play an important role in the regulation of endothelial-related functions, including oxidative stress, inflammation, and angiogenesis. Herein, we systematically review the factors affecting YAP/TAZ, downstream target genes regulated by YAP/TAZ and the roles of YAP/TAZ in regulating endothelial functions, in order to provide novel potential targets and effective approaches to prevent and treat cardiovascular diseases.
Introduction
The vascular endothelium is a cell layer lining the internal surface of the vascular lumen (1). Endothelial cells can sense factors acting on the vascular inner wall, such as fluid shear stress, stretch stress, and extracellular matrix (ECM) hardness, and then release nitric oxide, prostacyclin, reactive oxygen species (ROS), and other vasoactive substances to maintain the normal function of blood vessels (2, 3). Yes-associated protein (YAP) and transcriptional co-activator with PDZ-binding motif (TAZ), two closely related transcriptional regulators in the classical Hippo signaling pathway, play a crucial role in organ growth, tissue regeneration, and tumor development through the regulation of diverse transcriptional factors (4–6). Recently, researchers have found that YAP/TAZ also play an indispensable role in regulating endothelial biological functions, including inflammation, oxidative stress, and angiogenesis (7–12). In the present review, we aim to describe the YAP/TAZ structural characteristics, summarize the factors regulating YAP/TAZ, and elucidate the downstream target genes regulated by YAP/TAZ and the effects of YAP/TAZ on vascular endothelial functions.
Structural characteristics of yes-associated protein/transcriptional co-activator with PDZ-binding motif
In 1994, Sudol identified and cloned the cDNA of a new protein that binds to the SRC homology 3 (SH3) domain of Yes proto-oncogene product through an anti-idiotypic antibody (13), and since then YAP has been discovered. Transcriptional regulators YAP and TAZ have quickly attracted the attention of researchers due to their important roles in cell growth and differentiation, tissue regeneration and repair, cancer, and cardiovascular diseases. YAP is mapped at chromosome 11q22 with a molecular weight of 65 kDa (14, 15). The N-terminal of YAP is connected to the proline-rich ligand. Because YAP lacks a DNA binding domain, it can only act as a transcriptional regulator via interacting with the TEAD binding domain or other transcription factors (16, 17). TEAD is a pivotal DNA binding platform of YAP and includes the 14-3-3 binding domain. The phosphorylation site of YAP at serine 127 (S127) was found to interact with 14-3-3 protein, resulting in the accumulation of YAP in the cytoplasm (18). YAP, also known as YAP1, contains eight splice isomers, and YAP1-1 and YAP1-2 are the two main isomers (15, 19, 20). The difference is that YAP1-1 has only one WW domain, while YAP1-2 contains two WW domains. The WW domain can identify the PPxY motif (proline/proline/any amino acid/tyrosine), which is present in a series of proteins known to be YAP/TAZ interactors (19). In addition, YAP harbors an SH3 binding domain that is located between the WW domain and coiled-coil domain (17). TAD is the transcriptional activation domain, and the PDZ binding domain is the C-terminal domain (Figure 1A).
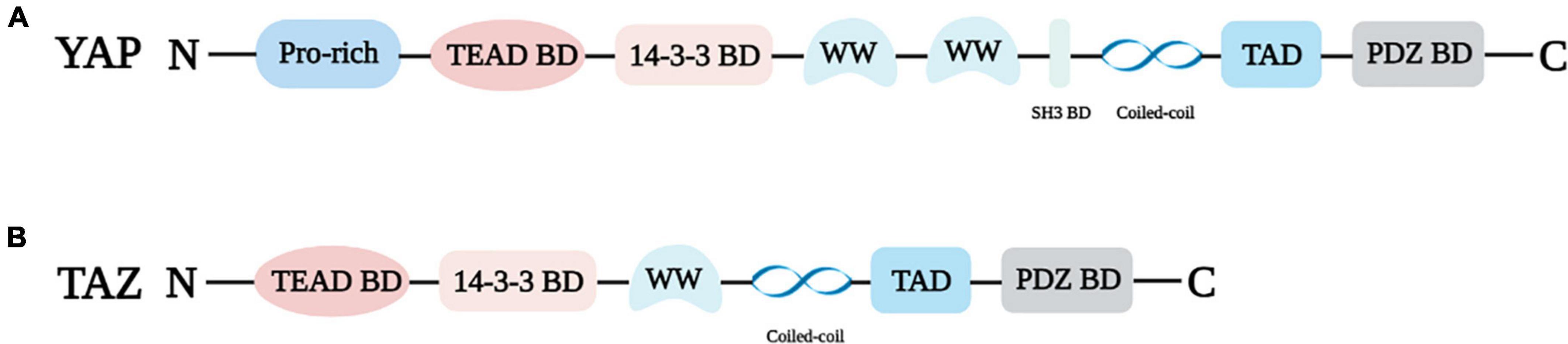
Figure 1. Structural properties of YAP (A) and TAZ (B). Pro-rich: Proline-rich; TEAD BD: TEAD binding domain; 14-3-3 BD: 14-3-3 binding domain, WW: WW domain; SH3 BD: SH3 binding domain; PDZ BD: PDZ binding domain.
TAZ, the paralog of YAP, was isolated as 14-3-3 binding protein, and it is located on chromosome 3q23-3q24 (14, 21). YAP and TAZ share several similar structures, but also possess distinctive structural features (Figure 1). The important shared structural features are the TEAD binding domain, WW domain, SH3 binding domain, Coiled-coil domain, and C-terminal transactivation domain. The main structurally distinctive feature is a proline-rich motif at the N-terminal end of YAP that is not conserved in TAZ (19) (Figure 1B).
YAP and TAZ are located both in the cytoplasm and nucleus. Phosphorylation of YAP/TAZ on multiple serine residues by LATS and other kinases, such as AKT and JNK contributes to YAP/TAZ inactivation and cytoplasmic accumulation (22, 23). Nevertheless, phosphorylation by c-Abl on YAPY357 results in YAP/TAZ activation and the sequestration of YAP/TAZ into the nucleus (24, 25). The nuclear localization of YAP/TAZ plays a vital role in determining cell behaviors, including proliferation, differentiation, and migration.
Regulatory factors of yes-associated protein/transcriptional co-activator with PDZ-binding motif
Effect of mechanical stress on yes-associated protein/transcriptional co-activator with PDZ-binding motif
Studies have shown that YAP and TAZ are not only nuclear sensors in the Hippo pathway, but also signal carriers and amplifiers of mechanical stress in the extracellular microenvironment (26). YAP/TAZ can sense and distinguish diverse mechanical stress and trigger different biomechanical responses. Zhong et al. used a microfluidic perfusion device to demonstrate for the first time that YAP could respond to different magnitudes of shear stress (27). Subsequent studies found that the activity of YAP/TAZ could be regulated by different forms of shear stress, including laminar shear stress (LSS) and oscillatory shear stress (OSS) (28–30). On the one hand, by promoting the LATS1/2-dependent phosphorylation of YAPS127 in the Hippo pathway, LSS inhibited the activation of YAP to resist inflammation and maintain the stability in normal endothelial cells (28, 31). On the other hand, LSS could also down-regulate YAP activation through autophagy-dependent pathway to decrease the expression of pro-inflammatory genes and interrupt the formation of atherosclerosis plaque (32). For damaged vascular endothelium, LSS ameliorated endothelial functions by activating YAP/TAZ. For example, in the injury model of cardiac microvascular endothelial cells (CMECs), LSS increased the expression of platelet-endothelial cell adhesion molecule-1 (PECAM1) and phosphorylated endothelial nitric oxide synthase (p-eNOS). The increased PECAM1 and p-eNOS subsequently activated YAP to protect CMECs from ischemia reperfusion injury (33). However, when human umbilical vein endothelial cells (HUVECs) were exposed to OSS, it was observed that YAP/TAZ was activated and translocated from vascular actin to the nucleus. Meantime, the expression of pro-inflammatory factors, including intercellular adhesion molecule-1 (ICAM-1), vascular cell adhesion molecule-1 (VCAM-1), and interleukin-6 (IL-6) were increased (29, 34, 35), which led to vascular endothelial injury and atherosclerosis. A recent study also revealed that HUVECs in the microfluidic chip showed obvious nuclear translocation after being stimulated by OSS for 6 h. Moreover, the activation of YAP/TAZ in HUVECs induced a significant increase in the expression of molecules related to the occurrence and development of atherosclerosis, such as ICAM-1 and von Willebrand factor (36). In addition, when human adipose-derived microvascular endothelial cells were stimulated with 20% stretch stress based on a normal physiological condition, the nuclear to cytoplasmic ratio of YAP in the experimental group cells was significantly higher than that in the control group (37), which indicated that physiological stretch stress could also promote the activation of YAP. The above evidence shows that mechanical stress, including LSS, WSS and physiological stretch stress, could modulate the activity of YAP/TAZ. Specifically, LSS can promote YAPS127 phosphorylation and inactivate YAP, while OSS and physiological stretch stress are able to activate YAP. Exercise is an effective method to improve endothelial functions and maintain vascular homeostasis. Our previous study showed that pulsatile flow shear stress induced by moderate intensity exercise was a key factor in mediating vascular endothelial functions improvement (38). However, the underlying mechanism is not entirely understood. Therefore, whether exercise-induced blood flow shear stress can regulate arterial endothelial functions via modulating YAP/TAZ deserves further study.
Effect of extracellular matrix hardness on yes-associated protein/transcriptional co-activator with PDZ-binding motif
Extracellular matrix (ECM) hardness is one of the important factors that determine cell adhesion and diffusion processes, which also has significant impacts on cell growth, migration, and differentiation. The activation of YAP/TAZ could be modulated by the hardness of ECM (39–41). In the early report, YAP activation was observed in breast epithelial cells under the hard matrix. Dupont et al. found that the hard substrate promoted the translocation of YAP/TAZ into the nucleus, whereas the soft substrate remained YAP/TAZ in the cytoplasm (26). Afterward, Shin and Mooney compared the content of YAP in human K-562 cells under different ECM hardness and observed that the expression level of YAP in cells under the hard matrix was higher than that under the soft matrix (42), which again confirmed the previous research results. Relevant studies also pointed out that the process of ECM hardness regulating YAP not only depended on Rho GTPase activity and actin cytoskeleton tension, but also relied on Src family kinases (39, 43, 44). Recently, Deng’s team found that the stiff substrate promoted the expression of focal adhesion kinase (FAK) and p-Paxillin, and then elevated the level of Rac1 in cells, contributing to the increase in cytoskeleton tissue stiffness. Subsequently, YAP was transferred to the nucleus, and the expression of target genes was up-regulated to promote the formation of endothelial tip cells (45). Similarly, Matsuo et al. confirmed that YAP activation was decreased in endothelial cells on soft substrate compared to cells on stiff substrate, and the YAP-Dll4-Notch signaling pathway was involved in modulating the effect of substrate stiffness on endothelial cell functions (46). Other studies have pointed out that the change in YAP activity induced by ECM hardness also plays a crucial role in cardiomyocyte regeneration. In addition, in the mouse myocardial infarction model, ECM protein Agrin activated YAP by up-regulating FAK and LRP4-MuSK and then promoted cardiomyocyte proliferation (47–49).
Effect of drugs on yes-associated protein/transcriptional co-activator with PDZ-binding motif
A series of drugs, including anti-atherosclerotic and anti-neoplastic drugs, have previously been found to downregulate YAP/TAZ activity in endothelial cells (25, 29, 31, 50). Anti-atheroslerotic drugs, such as rosuvastatin, simvastatin, and lovastatin, can inhibit YAP/TAZ activation to ameliorate the occurrence and development of cardiovascular diseases (29, 31, 50, 51). For example, rosuvastatin markedly attenuated YAP expression on TNF-α treatment and then decreased ICAM1 and VCAM1 expression in HUVECs, playing anti-inflammatory and atheroprotective roles (50). Simvastatin treatment significantly suppressed YAP/TAZ activation to attenuate the disturbed flow-induced proliferation and inflammation (29, 31). Lovastatin decreased YAP/TAZ activation and diminished angiotensin II-induced cardiovascular fibrosis (52). Additionally, methotrexate, an anti-neoplastic drug, also markedly inhibited disturbed flow shear stress induced YAP/TAZ activation in an AMPK-dependent manner, and further reduced pro-inflammatory factor secretion and monocyte adhesion in HUVECs (35). Thus, inhibition of YAP/TAZ activation via drugs is a promising endothelial protection and athero-protective therapeutic strategy.
Bosutinib, a tyrosine kinase inhibitor, significantly decreased the level of the phosphorylation of YAP at tyrosine 357 (Y357) and YAP activation to alleviate endothelium injury and the development of atherosclerosis (25). Likewise, salvianolic acid B, harmine and tetramethylpyrazine, the extracts from the traditional medicinal plants, inhibited YAP nuclear translocation and activation, and thus played a potent atheroprotective role (8, 9, 53). These inhibitor and extracts from the traditional medicinal plants might serve as potential therapeutical candidates for improving endothelial function and cardiovascular diseases via regulating the YAP/TAZ pathway.
Other factors regulating yes-associated protein/transcriptional co-activator with PDZ-binding motif
In addition to the above factors, YAP and TAZ are also regulated by glucose metabolism, hypoxia, and osmotic stress (41, 54–62). Under normal physiological conditions, YAP promoted glucose metabolism by up-regulating glucose transporter 3. Phosphorylation of YAPS127 increased when glucose metabolism was insufficient. On the contrary, in response to high glucose stimulation, YAP was activated and unregulated, and YAP activation led to vascular endothelial inflammation and increased monocyte adhesion (41, 54–58). In myocardial fibroblasts, high glucose promoted the increase of YAP expression in the nucleus by down-regulating p-MST1 and p-LATS1, resulting in inflammation, cell proliferation, and invasion (59). High expression of YAP/TAZ and VCAM-1 and vascular intima thickening were also observed in diabetic mice (54, 60). Besides, when the cells were under hypoxia, the production of 3-hydroxymethylglutaryl CoA reductase (HMGCR) increased. The up-regulated HMGCR suppressed the activation of LATS1/2 in the Hippo signaling pathway, and further promoted YAP nuclear accumulation and induced the increase in cysteine-rich angiogenic inducer 61 (CYR61) and connective tissue growth factor (CTGF) (61, 62). In addition, osmotic stress-induced the increase in phosphorylation of YAPS128 through NLK kinase localized YAP/TAZ in the nucleus (63).
Taken together, LSS, soft matrix, and abovementioned drugs and potential drugs promote YAP/TAZ inactivation and cytoplasm accumulation to resist inflammation and maintain vascular homeostasis (8, 25, 27, 28, 31, 46). Whereas, OSS, physiological stretch stress, hard matrix, glucose metabolism, hypoxia, and osmotic stress lead to YAP/TAZ activation and nuclear translocation, and further stimulate the expression of their downstream target genes to cause vascular endothelial injury and atherosclerosis (26, 29, 34, 35, 54–60).
Regulation of yes-associated protein/transcriptional co-activator with PDZ-binding motif on downstream target genes
Accumulating evidence has shown that YAP/TAZ induce the expression of downstream target genes after binding with the transcription factors of the TEAD binding domain and then plays an vital role in angiogenesis, ECM remodeling and atherosclerosis by regulating cell proliferation and migration (7, 29, 39, 47, 64–68) (Table 1). In a study of ApoE–/– mice fed with high-fat diets, an abnormal increase in YAP/TAZ expression was found in endothelial cells, as well as the augmentation of CYR61, CTGF and ankyrin repeat domain 1 (ANKRD1) contents. Moreover, YAP/TAZ activation induced the up-regulation of abovementioned genes and promoted the proliferation and migration of ECs, contributing to the thickening of common carotid artery wall and narrowing of vascular cavity in mice clearly observed by HE staining. In addition, in in vitro cell studies, overexpression of YAP/TAZ also increased the expression levels of the abovementioned genes. Therefore, these results confirmed that CYR61, CTGF, and ANKRD1 were upregulated by YAP/TAZ activation (25, 28, 29, 31, 35). It was also observed that the expression of angiopoietin-2 (Ang-2), a regulator of angiogenesis, decreased accordingly after the targeted knockdown of YAP, revealing that Ang-2 was also unregulated by YAP activation (7, 69, 70). Likewise, target genes such as hear shock protein A12B (HSPA12B), deleted-in-liver-cancer 1 (DLC1), microfibrillar-associated protein 5 (MFAP5), cell division cycle 42 (CDC42), and delta-like ligand 4 (DLL4) were modulated by YAP to further promote vascular germination or the formation of vascular reticular structure (64, 65, 71). In addition, YAP inactivation led to the decrease in expression levels of downstream genes, such as insulin-like growth factor binding protein 3 (IGFBP3) and diaphanous homology 3 (DIAPH3), and the down-regulated IGFBP3 and DIAPH3 destroyed ECM remodeling by inhibiting the increase in ECM hardness (39, 47). In addition, YAP activation reduced the expression of tumor necrosis factor superfamily member 10 (TNFSF10) to cause cells apoptosis (71, 72). In brief, YAP/TAZ activation up-regulate the abovementioned downstream target genes, including CYR61, CTGF, ANKRD1, to affect cells biological functions via regulating cells proliferation, migration and apoptosis.
Effect of yes-associated protein/transcriptional co-activator with PDZ-binding motif on the biological functions of the vascular endothelium
Yes-associated protein/transcriptional co-activator with PDZ-binding motif and inflammation
Atherosclerosis is the main inducer of cardiovascular diseases. Studies have confirmed that the activation of YAP/TAZ in endothelial cells plays an important role in the occurrence and development of atherosclerosis by promoting an inflammatory response (12, 67, 73, 74). Overexpression of YAP in an ApoE–/– mouse model upregulated the inflammatory related factors in arterial endothelial cells, such as IL-6, VCAM-1 and IL-8, and thus increased the atherosclerotic plaque and lesion range in aortic arch (8, 33). Moreover, it was found that after activating tumor necrosis factor-α (TNF-α) in HUVECs, YAP/TAZ expression was increased and transferred into the nucleus. The up-regulation of YAP/TAZ further promoted the increase in VCAM-1 and ICAM-1 in HUVECs, leading to inflammation (75). In addition, YAP/TAZ also increased monocyte adhesion by stimulating the JNK signaling pathway, triggering an inflammatory response in HUVECs (31). Yang et al. down-regulated YAP/TAZ in endothelial cells through the application of salvianolic acid B and found that inflammatory related factors, such as IL-6, IL-1β, and TNF-α, were decreased significantly, which confirmed that inhibiting YAP/TAZ reduced the expression of inflammatory factors (9). YAP/TAZ also acted as important regulators of macrophage intervention in the pro-inflammatory response and participated in the development of atherosclerosis through macrophages (73). Many researchers believe that the decrease of YAP/TAZ in cells inhibits the expression of inflammatory factors. However, Lv et al. found that the expression of E-selectin and ICAM-1 in mouse lung endothelial cells and the number of adherent neutrophils in postcapillary venules increased in mice with endothelial-specific deletion of YAP (76). This study suggested that YAP knockout did not suppress the expression of inflammatory factors, but promoted the endothelial cells activation and inflammatory response in pulmonary endothelial cells, which was inconsistent with the aforementioned studies (76). The reason of difference results might due to the cells observed in Lv’s research was pulmonary endothelial cells, which was different from HUVECs or other types of endothelial cells used in other investigations.
Yes-associated protein/transcriptional co-activator with PDZ-binding motif and oxidative stress
The dynamic balance between the oxidation and antioxidant system plays an important role in maintaining the homeostasis of the body. Under normal circumstances, the human body has a natural antioxidant system to fight against the oxidation system. When the production of superoxide anion, hydrogen peroxide, hydroxyl radical and other oxides in the body exceeds that of superoxide dismutase (SOD), glutathione, and other antioxidants, oxidative stress occurs (77). Oxidative stress is involved in the pathogenesis of atherosclerosis. Studies have shown that YAP participated in the regulation of oxidative stress, leading to the occurrence of atherosclerosis (78–80). In human aortic endothelial cells induced by ox-LDL, after down-regulating YAP, the expression of ROS was generally reduced. The decrease in ROS alleviated the endothelial injury caused by oxidative stress (11). Other findings showed that knockdown of YAP increased the expression of Rac1 in cells, and the up-regulation of Rac1 further caused excessive production of ROS, which eventually led to cell death related to autophagy (79, 81). Consistent with these, in in vivo animal experiments, under the conditions of YAP inhibitors or RNAi silencing, SOD expression content in rats was significantly decreased compared to the control group (58). Additionally, a study found that the activity of YAP was affected by ROS in breast cancer cells. When ROS production was reduced, intracellular YAP and JNK activation was attenuated accordingly, leading to mitochondrial dysfunction and apoptosis (10). The results of abovementioned investigations might suggest that YAP and ROS could promote each other’s activation or expression (10, 11, 76–78).
Yes-associated protein/transcriptional co-activator with PDZ-binding motif and angiogenesis
Angiogenesis is a process of forming new capillaries from pre-existing blood vessels, which involves a series of events, including endothelial cells germination, branching, lumen formation, and remodeling into a functional perfusion vascular network (82, 83). The significant role of YAP in angiogenesis has been repeatedly reported. It was found that the expression of YAP was increased in the process of differentiation from endothelial progenitor cells to endothelial cells. YAP nuclear localization further activated the vascular endothelium and promoted neovascularization, indicating that YAP is closely related to angiogenesis (7, 40). Conversely, YAP deletion seriously hindered the formation of the vascular network structure in endothelial cells (84, 85). In the mouse model of myocardial infarction, YAP overexpression reduced myocardial injury by promoting angiogenesis, improving cardiac function, and elevating the survival rate (86, 87). However, in mice, endothelial-specific deletion of YAP/TAZ induced the reduction and deformity of filopodia at the vascular front and the decrease and disarranged distribution of tight and adherent junction proteins, leading to destruction of vascular barrier network (88). Thus, YAP was shown to be an essential factor in promoting angiogenesis and treating ischemic cardiovascular diseases. YAP binded to signal transducer and activator of transcription 3 (STAT3), resulting in the phosphorylation of STAT3 and the increase of STAT3 expression in the nucleus, which further activated downstream Ang-2 and accelerated angiogenesis (70, 85, 89). Similarly, the miR-205/YAP pathway depended on STAT3 to promote vascular germination and angiogenesis in HUVECs (90, 91). Other studies have found that the DLL4-Notch1 signaling pathway was closely related to angiogenesis. Inhibiting the DLL4-Notch1 signaling pathway promoted the expression of vascular endothelial growth factor receptor 2 (VEGFR2), and VEGFR2 regulated downstream Ang-2 by activating YAP to repair damaged vascular endothelium (46, 64, 92). The abovementioned evidence shows that YAP/TAZ play an important role in angiogenesis and are expected to become potential targets for the clinical treatment of pathological angiogenesis-related diseases.
Conclusion and perspectives
Endothelial dysfunction is one of triggers for the development of cardiovascular diseases (93, 94). YAP and TAZ are important downstream regulators of the Hippo pathway, which are involved in the regulation of vascular endothelial functions and play a prominent role in the development of cardiovascular diseases. Current studies have suggested that LSS, some drugs, and soft matrix induce YAP/TAZ inactivation and cytoplasm accumulation, resulting in the attenuating of inflammation and oxidative stress and the improvement of endothelial functions (25–29, 31). However, OSS, physiological stretch stress, and hard matrix cause YAP/TAZ activation and nuclear translocation, leading to vascular endothelial injury (34, 35, 41, 45) (Figure 2). Thus, modulating mechanical stress and matrix stiffness and using drugs might be served as treatment strategies for ameliorating endothelial functions. Our previous study showed that exercise-induced shear stress is a key factor to regulate endothelial function (38). However, the underlying mechanism is not entirely understood. Therefore, whether exercise-induced shear stress can improve arterial endothelial functions via modulating YAP/TAZ deserves further clarification. Furthermore, if YAP and TAZ mediate endothelial functions improvement under exercise-induced shear stress, whether the combined effects of exercise-induced shear stress and drugs would be achieve better synergistic effect in improving endothelial functions via YAP/TAZ regulation also need further study. In addition, glucose metabolism, hypoxia and osmotic stress can regulate YAP/TAZ and affect endothelial-related biological functions. However, how the abovementioned factors regulate YAP/TAZ and whether there are other downstream target genes modulated by YAP/TAZ are not well clarified. Accumulating evidence has indicated that YAP/TAZ play a central role in modulating biological functions of the vascular endothelium, including inflammation, oxidative stress, and angiogenesis (12, 67, 73–87). Whether there are other biological functions of the vascular endothelium affected by YAP/TAZ are also worthy to be further studied. Further studies of YAP/TAZ related biological functions of vascular endothelium and signal pathways will provide novel targets for the prevention and treatment of endothelial cells functions related cardiovascular diseases.
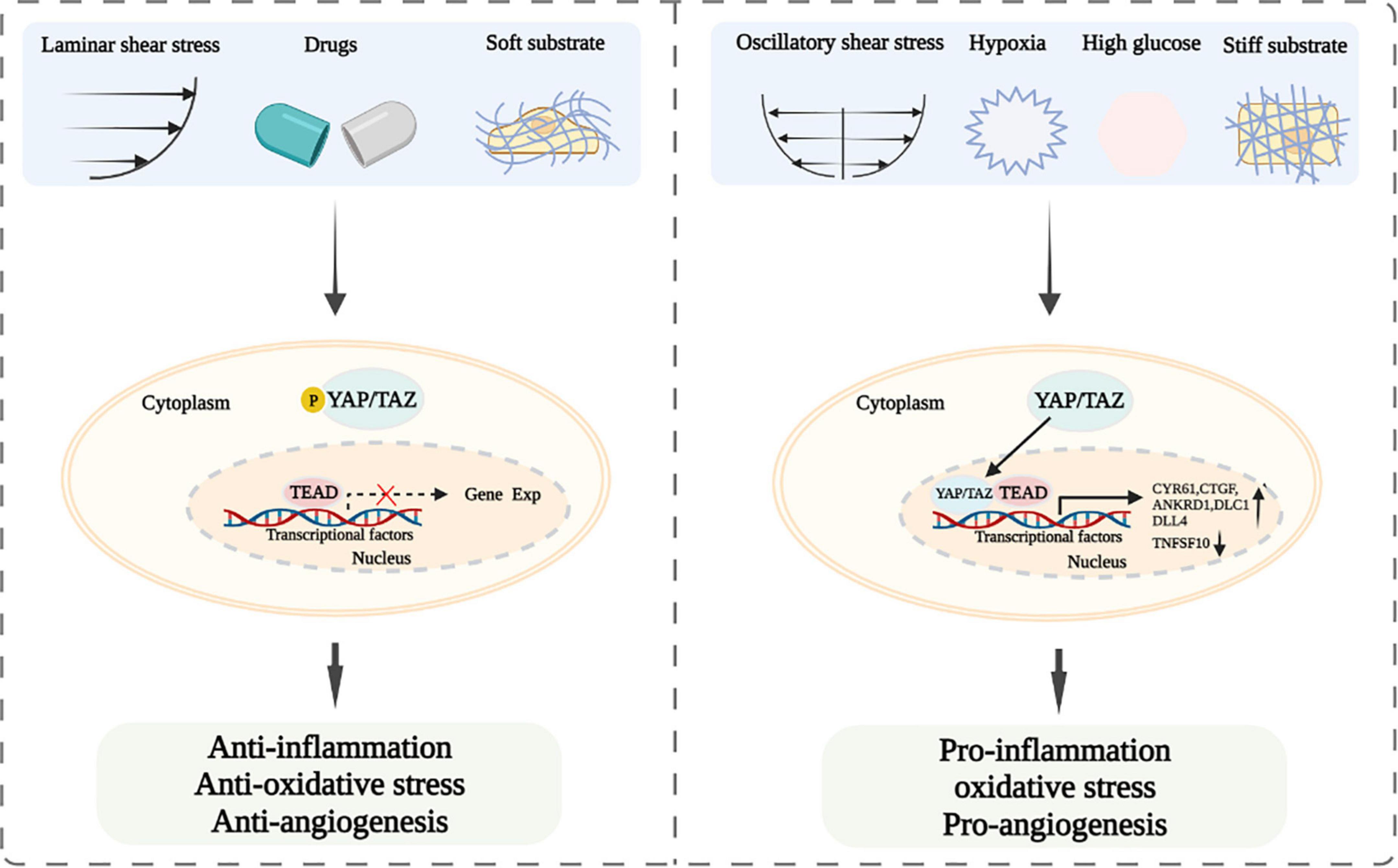
Figure 2. Schematic diagram of YAP/TAZ signaling and their modulation of endothelial functions after exposing to laminar and oscillatory shear stress, different drugs, soft and hard substrate, hypoxia or high glucose.
Author contributions
Y-XW and MC designed the work. WZ, Y-XW, and Q-QL drafted the manuscript. H-YG, Y-CW, and MC revised the manuscript. All authors contributed to the article and approved the submitted version.
Funding
This work was supported by the National Natural Science Foundation of China (Grant Nos. 32000927 and 81870237) and Shandong Province Natural Science Foundation (Grant No. ZR2020QC092).
Conflict of interest
The authors declare that the research was conducted in the absence of any commercial or financial relationships that could be construed as a potential conflict of interest.
Publisher’s note
All claims expressed in this article are solely those of the authors and do not necessarily represent those of their affiliated organizations, or those of the publisher, the editors and the reviewers. Any product that may be evaluated in this article, or claim that may be made by its manufacturer, is not guaranteed or endorsed by the publisher.
References
1. Rajendran P, Rengarajan T, Thangavel J, Nishigaki Y, Sakthisekaran D, Sethi G, et al. The vascular endothelium and human diseases. Int J Biol Sci. (2013) 9:1057–69. doi: 10.7150/ijbs.7502
2. Hsieh HJ, Liu CA, Huang B, Tseng AH, Wang DL. Shear-Induced endothelial mechanotransduction: the interplay between reactive oxygen species (Ros) and nitric oxide (No) and the pathophysiological implications. J Biomed Sci. (2014) 21:3. doi: 10.1186/1423-0127-21-3
3. Russell-Puleri S, Ebong EE, Tarbell JM. “Mechanisms of flow-dependent endothelial Cox-2 and Pgi2 expression,”in Proceedings of the 2014 40th Annual Northeast Bioengineering Conference (NEBEC), (Piscataway, NJ: IEEE) (2014).
4. Moya IM, Halder G. Hippo–Yap/taz signalling in organ regeneration and regenerative medicine. Nat Rev Mol Cell Biol. (2018) 20:211–26. doi: 10.1038/s41580-018-0086-y
5. Pan D. The hippo signaling pathway in development and cancer. Dev Cell. (2010) 19:491–505. doi: 10.1016/j.devcel.2010.09.011
6. Klaihmon P, Lorthongpanich C, Kheolamai P, Luanpitpong S, Issaragrisil S. Distinctive roles of yap and taz in human endothelial progenitor cells growth and functions. Biomedicines. (2022) 10:147. doi: 10.3390/biomedicines10010147
7. Choi HJ, Zhang H, Park H, Choi KS, Lee HW, Agrawal V, et al. Yes-Associated protein regulates endothelial cell contact-mediated expression of angiopoietin-2. Nat Commun. (2015) 6:6943. doi: 10.1038/ncomms7943
8. Yang Y, Ma Q, Li Z, Wang H, Zhang C, Liu Y, et al. Harmine alleviates atherogenesis by inhibiting disturbed flow-mediated endothelial activation via protein tyrosine phosphatase ptpn14 and yap. Br J Pharmacol. (2021) 178:1524–40. doi: 10.1111/bph.15378
9. Yang Y, Pei K, Zhang Q, Wang D, Feng H, Du Z, et al. Salvianolic acid B ameliorates atherosclerosis via inhibiting Yap/Taz/Jnk signaling pathway in endothelial cells and pericytes. Biochim Biophys Acta Mol Cell Biol Lipids. (2020) 1865:158779. doi: 10.1016/j.bbalip.2020.158779
10. Wang L, Wang C, Tao Z, Zhao L, Zhu Z, Wu W, et al. Curcumin derivative Wz35 inhibits tumor cell growth via ros-yap-jnk signaling pathway in breast cancer. J Exp Clin Cancer Res. (2019) 38:460. doi: 10.1186/s13046-019-1424-4
11. Xie T, Wang C, Jin Y, Meng Q, Liu Q, Wu J, et al. Coenzymeq10-Induced activation of ampk-yap-opa1 pathway alleviates atherosclerosis by improving mitochondrial function. Inhibiting oxidative stress and promoting energy metabolism. Front Pharmacol. (2020) 11:1034. doi: 10.3389/fphar.2020.01034
12. Xu K, Zhao H, Qiu X, Liu X, Zhao F, Zhao Y. Vgll4 protects against oxidized-ldl-induced endothelial cell dysfunction and inflammation by activating hippo-Yap/Tead1 signaling pathway. Mediators Inflamm. (2020) 2020:8292173. doi: 10.1155/2020/8292173
13. Sudol M. Yes-Associated protein (Yap65) is a proline-rich phosphoprotein that binds to the sh3 domain of the yes proto-oncogene product. Oncogene. (1994) 9:2145–52.
14. Chen YA, Lu CY, Cheng TY, Pan SH, Chen HF, Chang NS. WW domain-containing proteins yap and taz in the hippo pathway as key regulators in stemness maintenance, tissue homeostasis, and tumorigenesis. Front Oncol. (2019) 9:60. doi: 10.3389/fonc.2019.00060
15. Sudol M, Bork P, Einbond A, Kastury K, Druck T, Negrini M, et al. Characterization of the mammalian yap (yes-associated protein) gene and its role in defining a novel protein module, the Ww domain. J Biol Chem. (1995) 270:14733–41. doi: 10.1074/jbc.270.24.14733
16. Zhao B, Lei QY, Guan KL. The hippo-yap pathway: new connections between regulation of organ size and cancer. Curr Opin Cell Biol. (2008) 20:638–46. doi: 10.1016/j.ceb.2008.10.001
17. Reggiani F, Gobbi G, Ciarrocchi A, Sancisi V. Yap and Taz are not identical twins. Trends Biochem Sci. (2020) 46:154–68. doi: 10.1016/j.tibs.2020.08.012
18. Yu Y, Su X, Qin Q, Hou Y, Zhang X, Zhang H, et al. Yes-Associated protein and transcriptional coactivator with pdz-binding motif as new targets in cardiovascular diseases. Pharmacol Res. (2020) 159:105009. doi: 10.1016/j.phrs.2020.105009
19. Sudol M. Yap1 oncogene and its eight isoforms. Oncogene. (2013) 32:3922. doi: 10.1038/onc.2012.520
20. Komuro A, Nagai M, Navin NE, Sudol M. Ww domain-containing protein yap associates with Erbb-4 and acts as a co-transcriptional activator for the carboxyl-terminal fragment of Erbb-4 that translocates to the nucleus. J Biol Chem. (2003) 278:33334–41. doi: 10.1074/jbc.M305597200
21. Kanai F, Marignani PA, Sarbassova D, Yagi R, Hall RA, Donowitz M, et al. Taz: a novel transcriptional co-activator regulated by interactions with 14-3-3 and pdz domain proteins. Embo J. (2000) 19:6778–91. doi: 10.1093/emboj/19.24.6778
22. Piccolo S, Dupont S, Cordenonsi M. The biology of Yap/Taz: hippo signaling and beyond. Physiol Rev. (2014) 94:1287–312. doi: 10.1152/physrev.00005.2014
23. Zhao B, Li L, Tumaneng K, Wang CY, Guan KLA. Coordinated phosphorylation by lats and ck1 regulates yap stability through scf(Beta-Trcp). Genes Dev. (2010) 24:72–85. doi: 10.1101/gad.1843810
24. Levy D, Adamovich Y, Reuven N, Shaul Y. Yap1 Phosphorylation by C-Abl Is a Critical Step in Selective Activation of Proapoptotic Genes in Response to DNA Damage. Mol Cell. (2008) 29:350–61. doi: 10.1016/j.molcel.2007.12.022
25. Li B, He J, Lv H, Liu Y, Lv X, Zhang C, et al. C-Abl regulates yapy357 phosphorylation to activate endothelial atherogenic responses to disturbed flow. J Clin Invest. (2019) 129:1167–79. doi: 10.1172/JCI122440
26. Dupont S, Morsut L, Aragona M, Enzo E, Giulitti S, Cordenonsi M, et al. Role of Yap/Taz in Mechanotransduction. Nature. (2011) 474:179–83. doi: 10.1038/nature10137
27. Zhong W, Tian K, Zheng X, Li L, Zhang W, Wang S, et al. Mesenchymal stem cell and chondrocyte fates in a multishear microdevice are regulated by yes-associated protein. Stem Cells Dev. (2013) 22:2083–93. doi: 10.1089/scd.2012.0685
28. Xu S, Koroleva M, Yin M, Jin ZG. Atheroprotective laminar flow inhibits hippo pathway effector yap in endothelial cells. Transl Res. (2016) 176:18–28.e2. doi: 10.1016/j.trsl.2016.05.003
29. Wang KC, Yeh YT, Nguyen P, Limqueco E, Lopez J, Thorossian S, et al. Flow-dependent Yap/Taz activities regulate endothelial phenotypes and atherosclerosis. Proc Natl Acad Sci U.S.A. (2016) 113:11525–30. doi: 10.1073/pnas.1613121113
30. Chitragari G, Shalaby SY, Sumpio BJ, Kurita J, Sumpio BE. Regulation of yes-associated protein by laminar flow. Ann Vasc Surg. (2018) 52:183–91. doi: 10.1016/j.avsg.2018.03.002
31. Wang L, Luo JY, Li B, Tian XY, Chen LJ, Huang Y, et al. Integrin-YAP/TAZ-JNK cascade mediates atheroprotective effect of unidirectional shear flow. Nature. (2016) 540:579–82. doi: 10.1038/nature20602
32. Yuan P, Hu Q, He X, Long Y, Song X, Wu F, et al. Laminar flow inhibits the hippo/yap pathway via autophagy and sirt1-mediated deacetylation against atherosclerosis. Cell Death Dis. (2020) 11:141. doi: 10.1038/s41419-020-2343-1
33. Zhang Q, Cao Y, Liu Y, Huang W, Ren J, Wang P, et al. Shear stress inhibits cardiac microvascular endothelial cells apoptosis to protect against myocardial ischemia reperfusion injury via Yap/Mir-206/Pdcd4 signaling pathway. Biochem Pharmacol. (2021) 186:114466. doi: 10.1016/j.bcp.2021.114466
34. Nakajima H, Yamamoto K, Agarwala S, Terai K, Fukui H, Fukuhara S, et al. Flow-dependent endothelial yap regulation contributes to vessel maintenance. Dev Cell. (2017) 40:523–36.e6. doi: 10.1016/j.devcel.2017.02.019
35. Liu D, Lv H, Liu Q, Sun Y, Hou S, Zhang L, et al. Atheroprotective effects of methotrexate via the inhibition of Yap/Taz under disturbed flow. J Transl Med. (2019) 17:378. doi: 10.1186/s12967-019-02135-8
36. Walther BK, Rajeeva Pandian NK, Gold KA, Kiliç ES, Sama V, Gu J, et al. Mechanotransduction-on-Chip: vessel-chip model of endothelial yap mechanobiology reveals matrix stiffness impedes shear response. Lab Chip. (2021) 21:1738–51. doi: 10.1039/d0lc01283a
37. Landau S, Ben-Shaul S, Levenberg S. Oscillatory strain promotes vessel stabilization and alignment through fibroblast yap-mediated mechanosensitivity. Adv Sci. (2018) 5:1800506. doi: 10.1002/advs.201800506
38. Wang YX, Liu HB, Li PS, Yuan WX, Liu B, Liu ST, et al. Ros and no dynamics in endothelial cells exposed to exercise-induced wall shear stress. Cell Mol Bioeng. (2019) 12:107–20. doi: 10.1007/s12195-018-00557-w
39. Calvo F, Ege N, Grande-Garcia A, Hooper S, Jenkins RP, Chaudhry SI, et al. Mechanotransduction and Yap-dependent matrix remodelling is required for the generation and maintenance of cancer-associated fibroblasts. Nat Cell Biol. (2013) 15:637–46. doi: 10.1038/ncb2756
40. Jiang X, Hu J, Wu Z, Cafarello ST, Di Matteo M, Shen Y, et al. Protein phosphatase 2a mediates Yap activation in endothelial cells upon vegf stimulation and matrix stiffness. Front Cell Dev Biol. (2021) 9:675562. doi: 10.3389/fcell.2021.675562
41. Liu Y, Li M, Lv X, Bao K, Yu Tian X, He L, et al. Yes-associated protein targets the transforming growth factor β pathway to mediate high-fat/high-sucrose diet-induced arterial stiffness. Circ Res. (2022) 130:851–67. doi: 10.1161/circresaha.121.320464
42. Shin JW, Mooney DJ. Extracellular matrix stiffness causes systematic variations in proliferation and chemosensitivity in myeloid leukemias. Proc Natl Acad Sci U.S.A. (2016) 113:12126–31. doi: 10.1073/pnas.1611338113
43. Yamashiro Y, Thang BQ, Ramirez K, Shin SJ, Kohata T, Ohata S, et al. Matrix mechanotransduction mediated by Thrombospondin-1/Integrin/Yap in the vascular remodeling. Proc Natl Acad Sci U.S.A. (2020) 117:9896–905. doi: 10.1073/pnas.1919702117
44. Ma H, Wang J, Zhao X, Wu T, Huang Z, Chen D, et al. Periostin promotes colorectal tumorigenesis through integrin-fak-src pathway-mediated Yap/Taz activation. Cell Rep. (2020) 30:793–806. doi: 10.1016/j.celrep.2019.12.075
45. Guo Y, Mei F, Huang Y, Ma S, Wei Y, Zhang X, et al. Matrix stiffness modulates tip cell formation through the P-Pxn-Rac1-Yap signaling axis. Bioact Mater. (2021) 7:364–76. doi: 10.1016/j.bioactmat.2021.05.033
46. Matsuo E, Okamoto T, Ito A, Kawamoto E, Asanuma K, Wada K, et al. Substrate stiffness modulates endothelial cell function via the Yap-Dll4-Notch1 pathway. Exp Cell Res. (2021) 408:112835. doi: 10.1016/j.yexcr.2021.112835
47. Chakraborty S, Njah K, Pobbati AV, Lim YB, Raju A, Lakshmanan M, et al. Agrin as a mechanotransduction signal regulating Yap through the hippo pathway. Cell Rep. (2017) 18:2464–79. doi: 10.1016/j.celrep.2017.02.041
48. Wang X, Senapati S, Akinbote A, Gnanasambandam B, Park PS, Senyo SE. Microenvironment stiffness requires decellularized cardiac extracellular matrix to promote heart regeneration in the neonatal mouse heart. Acta Biomater. (2020) 113:380–92. doi: 10.1016/j.actbio.2020.06.032
49. Bassat E, Mutlak YE, Genzelinakh A, Shadrin IY, Baruch Umansky K, Yifa O, et al. The extracellular matrix protein agrin promotes heart regeneration in mice. Nature. (2017) 547:179–84. doi: 10.1038/nature22978
50. Jia M, Li Q, Guo J, Shi W, Zhu L, Huang Y, et al. Deletion of bach1 attenuates atherosclerosis by reducing endothelial inflammation. Circ Res. (2022) 130:1038–55. doi: 10.1161/circresaha.121.319540
51. Sorrentino G, Ruggeri N, Specchia V, Cordenonsi M, Mano M, Dupont S, et al. Metabolic control of Yap and Taz by the mevalonate pathway. Nat Cell Biol. (2014) 16:357–66. doi: 10.1038/ncb2936
52. Wu P, Liu Z, Zhao T, Xia F, Gong L, Zheng Z, et al. Lovastatin attenuates angiotensin ii induced cardiovascular fibrosis through the suppression of Yap/Taz signaling. Biochem Biophys Res Commun. (2019) 512:736–41. doi: 10.1016/j.bbrc.2019.03.158
53. Zhu X, Shan Y, Yu M, Shi J, Tang L, Cao H, et al. Tetramethylpyrazine ameliorates peritoneal angiogenesis by regulating Vegf/Hippo/Yap signaling. Front Pharmacol. (2021) 12:649581. doi: 10.3389/fphar.2021.649581
54. Ortillon J, Le Bail JC, Villard E, Léger B, Poirier B, Girardot C, et al. High glucose activates Yap signaling to promote vascular inflammation. Front Physiol. (2021) 12:665994. doi: 10.3389/fphys.2021.665994
55. Wang W, Xiao ZD, Li X, Aziz KE, Gan B, Johnson RL, et al. Ampk modulates hippo pathway activity to regulate energy homeostasis. Nat Cell Biol. (2015) 17:490–9. doi: 10.1038/ncb3113
56. Mo JS, Meng Z, Kim YC, Park HW, Hansen CG, Kim S, et al. Cellular energy stress induces ampk-mediated regulation of Yap and the hippo pathway. Nat Cell Biol. (2015) 17:500–10. doi: 10.1038/ncb3111
57. Wei F, Wang A, Wang Q, Han W, Rong R, Wang L, et al. Plasma endothelial cells-derived extracellular vesicles promote wound healing in diabetes through Yap and the Pi3k/Akt/Mtor pathway. Aging. (2020) 12:12002–18. doi: 10.18632/aging.103366
58. Yu S, Dong X, Yang M, Yu Q, Xiong J, Chen J, et al. (Pro)Renin receptor involves in myocardial fibrosis and oxidative stress in diabetic cardiomyopathy via the Prr-Yap pathway. Sci Rep. (2021) 11:3259. doi: 10.1038/s41598-021-82776-2
59. Liu J, Xu L, Zhan X. Lncrna malat1 regulates diabetic cardiac fibroblasts through the hippo-Yap signaling pathway. Biochem Cell Biol. (2020) 98:537–47. doi: 10.1139/bcb-2019-0434
60. Chao ML, Luo S, Zhang C, Zhou X, Zhou M, Wang J, et al. S-Nitrosylation-mediated coupling of G-protein alpha-2 with Cxcr5 induces hippo/Yap-dependent diabetes-accelerated atherosclerosis. Nat Commun. (2021) 12:4452. doi: 10.1038/s41467-021-24736-y
61. Zhao C, Zeng C, Ye S, Dai X, He Q, Yang B, et al. Yes-Associated protein (Yap) and transcriptional coactivator with a Pdz-binding motif (Taz): a nexus between hypoxia and cancer. Acta Pharm Sin B. (2020) 10:947–60. doi: 10.1016/j.apsb.2019.12.010
62. Zhang X, Li Y, Ma Y, Yang L, Wang T, Meng X, et al. Yes-Associated protein (Yap) binds to hif-1α and sustains hif-1α protein stability to promote hepatocellular carcinoma cell glycolysis under hypoxic stress. J Exp Clin Cancer Res. (2018) 37:216. doi: 10.1186/s13046-018-0892-2
63. Hong AW, Meng Z, Yuan HX, Plouffe SW, Moon S, Kim W, et al. Osmotic stress-induced phosphorylation by Nlk at ser128 activates Yap. EMBO Rep. (2017) 18:72–86. doi: 10.15252/embr.201642681
64. Yasuda D, Kobayashi D, Akahoshi N, Ohto-Nakanishi T, Yoshioka K, Takuwa Y, et al. Lysophosphatidic acid-induced Yap/Taz activation promotes developmental angiogenesis by repressing notch ligand Dll4. J Clin Invest. (2019) 129:4332–49. doi: 10.1172/jci121955
65. van der Stoel M, Schimmel L, Nawaz K, van Stalborch AM, de Haan A, Klaus-Bergmann A, et al. Dlc1 is a direct target of activated Yap/Taz that drives collective migration and sprouting angiogenesis. J Cell Sci. (2020) 133:jcs239947. doi: 10.1242/jcs.239947
66. Shen Z, Stanger BZ. Yap regulates S-phase entry in endothelial cells. PLoS One. (2015) 10:e0117522. doi: 10.1371/journal.pone.0117522
67. Coleman PR, Lay AJ, Ting KK, Zhao Y, Li J, Jarrah S, et al. Yap and the rhoc regulator arhgap18, are required to mediate flow-dependent endothelial cell alignment. Cell Commun Signal. (2020) 18:18. doi: 10.1186/s12964-020-0511-7
68. Rausch V, Bostrom JR, Park J, Bravo IR, Feng Y, Hay DC, et al. The hippo pathway regulates caveolae expression and mediates flow response via caveolae. Curr Biol. (2019) 29:242–55. doi: 10.1016/j.cub.2018.11.066
69. Huang Y, Pan M, Shu H, He B, Zhang F, Sun L. Vascular endothelial growth factor enhances tendon-bone healing by activating yes-associated protein for angiogenesis induction and rotator cuff reconstruction in rats. J Cell Biochem. (2020) 121:2343–53. doi: 10.1002/jcb.29457
70. He J, Bao Q, Zhang Y, Liu M, Lv H, Liu Y, et al. Yes-associated protein promotes angiogenesis via signal transducer and activator of transcription 3 in endothelial cells. Circ Res. (2018) 122:591–605. doi: 10.1161/circresaha.117.311950
71. Marti P, Stein C, Blumer T, Abraham Y, Dill MT, Pikiolek M, et al. Yap promotes proliferation, chemoresistance, and angiogenesis in human cholangiocarcinoma through tead transcription factors. Hepatology. (2015) 62:1497–510. doi: 10.1002/hep.27992
72. Wang S, El-Deiry WS. Trail and apoptosis induction by tnf-family death receptors. Oncogene. (2003) 22:8628–33. doi: 10.1038/sj.onc.1207232
73. Mia MM, Cibi DM, Abdul Ghani SAB, Song W, Tee N, Ghosh S, et al. Yap/Taz deficiency reprograms macrophage phenotype and improves infarct healing and cardiac function after myocardial infarction. PLoS Biol. (2020) 18:e3000941. doi: 10.1371/journal.pbio.3000941
74. Xu Q, Zhuo K, Cai R, Su X, Zhang L, Liu Y, et al. Activation of yes-associated protein/Pdz-binding motif pathway contributes to endothelial dysfunction and vascular inflammation in angiotensinii hypertension. Front Physiol. (2021) 12:732084. doi: 10.3389/fphys.2021.732084
75. Choi HJ, Kim NE, Kim BM, Seo M, Heo JH. TNF-α-induced Yap/Taz activity mediates leukocyte-endothelial adhesion by regulating vcam1 expression in endothelial cells. Int J Mol Sci. (2018) 19:3428. doi: 10.3390/ijms19113428
76. Lv Y, Kim K, Sheng Y, Cho J, Qian Z, Zhao YY, et al. Yap controls endothelial activation and vascular inflammation through Traf6. Circ Res. (2018) 123:43–56. doi: 10.1161/circresaha.118.313143
77. Kattoor AJ, Pothineni NVK, Palagiri D, Mehta JL. Oxidative stress in atherosclerosis. Curr Atheroscler Rep. (2017) 19:42. doi: 10.1007/s11883-017-0678-6
78. Shao D, Zhai P, Del Re DP, Sciarretta S, Yabuta N, Nojima H, et al. A functional interaction between hippo-Yap signalling and foxo1 mediates the oxidative stress response. Nat Commun. (2014) 5:3315. doi: 10.1038/ncomms4315
79. Zhou Y, Wang Y, Zhou W, Chen T, Wu Q, Chutturghoon VK, et al. Yap promotes multi-drug resistance and inhibits autophagy-related cell death in hepatocellular carcinoma via the Rac1-Ros-Mtor pathway. Cancer Cell Int. (2019) 19:179. doi: 10.1186/s12935-019-0898-7
80. Marchio P, Guerra-Ojeda S, Vila JM, Aldasoro M, Victor VM, Mauricio MD. Targeting Early atherosclerosis: a focus on oxidative stress and inflammation. Oxid Med Cell Longev. (2019) 2019:8563845. doi: 10.1155/2019/8563845
81. Patel S, Tang J, Overstreet JM, Anorga S, Lian F, Arnouk A, et al. Rac-Gtpase promotes fibrotic Tgf-B 1 signaling and chronic kidney disease via egfr. P53, and Hippo/Yap/Taz pathways. Faseb J. (2019) 33:9797–810. doi: 10.1096/fj.201802489RR
82. Potente M, Gerhardt H, Carmeliet P. Basic and therapeutic aspects of angiogenesis. Cell. (2011) 146:873–87. doi: 10.1016/j.cell.2011.08.039
83. Chung AS, Ferrara N. Developmental and pathological angiogenesis. Annu Rev Cell Dev Biol. (2011) 27:563–84. doi: 10.1146/annurev-cellbio-092910-154002
84. Yan Z, Shi H, Zhu R, Li L, Qin B, Kang L, et al. Inhibition of Yap ameliorates choroidal neovascularization via inhibiting endothelial cell proliferation. Mol Vis. (2018) 24:83–93.
85. Zhu M, Liu X, Wang Y, Chen L, Wang L, Qin X, et al. Yap via interacting with stat3 regulates vegf-induced angiogenesis in human retinal microvascular endothelial cells. Exp Cell Res. (2018) 373:155–63. doi: 10.1016/j.yexcr.2018.10.007
86. Fan M, Yang K, Wang X, Wang Y, Tu F, Ha T, et al. Endothelial cell Hspa12b and yes-associated protein cooperatively regulate angiogenesis following myocardial infarction. JCI Insight. (2020) 5:e139640. doi: 10.1172/jci.insight.139640
87. Lin Z, von Gise A, Zhou P, Gu F, Ma Q, Jiang J, et al. Cardiac-specific yap activation improves cardiac function and survival in an experimental murine mi model. Circ Res. (2014) 115:354–63. doi: 10.1161/circresaha.115.303632
88. Kim J, Kim YH, Kim J, Park DY, Bae H, Lee DH, et al. Yap/Taz regulates sprouting angiogenesis and vascular barrier maturation. J Clin Invest. (2017) 127:3441–61. doi: 10.1172/jci93825
89. Fan X, Shan X, Jiang S, Wang S, Zhang F, Tian Q, et al. Yap promotes endothelial barrier repair by repressing Stat3/Vegf signaling. Life Sci. (2020) 256:117884. doi: 10.1016/j.lfs.2020.117884
90. Du YE, Tu G, Yang G, Li G, Yang D, Lang L, et al. Mir-205/Yap1 in activated fibroblasts of breast tumor promotes vegf-independent angiogenesis through stat3 signaling. Theranostics. (2017) 7:3972–88. doi: 10.7150/thno.18990
91. Shen Y, Wang X, Liu Y, Singhal M, Gürkaşlar C, Valls AF, et al. Stat3-Yap/Taz signaling in endothelial cells promotes tumor angiogenesis. Sci Signal. (2021) 14:eabj8393. doi: 10.1126/scisignal.abj8393
92. Hultgren NW, Fang JS, Ziegler ME, Ramirez RN, Phan DTT, Hatch MMS, et al. Slug regulates the Dll4-Notch-Vegfr2 axis to control endothelial cell activation and angiogenesis. Nat Commun. (2020) 11:5400. doi: 10.1038/s41467-020-18633-z
93. Gimbrone MA Jr, García-Cardeña G Endothelial cell dysfunction and the pathobiology of atherosclerosis. Circ Res. (2016) 118:620–36. doi: 10.1161/circresaha.115.306301
94. Bai T, Li M, Liu Y, Qiao Z, Wang Z. Inhibition of ferroptosis alleviates atherosclerosis through attenuating lipid peroxidation and endothelial dysfunction in mouse aortic endothelial cell. Free Radic Biol Med. (2020) 160:92–102. doi: 10.1016/j.freeradbiomed.2020.07.026
Keywords: YAP/TAZ, endothelial cells, oxidative stress, inflammation, angiogenesis
Citation: Zhang W, Li Q-q, Gao H-y, Wang Y-c, Cheng M and Wang Y-X (2022) The regulation of yes-associated protein/transcriptional coactivator with PDZ-binding motif and their roles in vascular endothelium. Front. Cardiovasc. Med. 9:925254. doi: 10.3389/fcvm.2022.925254
Received: 21 April 2022; Accepted: 04 July 2022;
Published: 22 July 2022.
Edited by:
Wen-Jun Tu, Chinese Academy of Medical Sciences and Peking Union Medical College, ChinaReviewed by:
Sang Joon Ahn, University of Illinois at Chicago, United StatesMonica Y. Lee, University of Illinois at Chicago, United States
Copyright © 2022 Zhang, Li, Gao, Wang, Cheng and Wang. This is an open-access article distributed under the terms of the Creative Commons Attribution License (CC BY). The use, distribution or reproduction in other forums is permitted, provided the original author(s) and the copyright owner(s) are credited and that the original publication in this journal is cited, in accordance with accepted academic practice. No use, distribution or reproduction is permitted which does not comply with these terms.
*Correspondence: Min Cheng, mincheng@wfmc.edu.cn; Yan-Xia Wang, wangyanxia6666@wfmc.edu.cn