- 1Division of Cardiovascular Medicine, Radcliffe Department of Medicine, University of Oxford, John Radcliffe Hospital, Oxford, United Kingdom
- 2Aristotle University of Thessaloniki, Thessaloniki, Greece
- 3Division of Cardiology, Columbia University Irving Medical Center, New York, NY, United States
Cardiac computed tomography (CCT) is now considered a first-line diagnostic test for suspected coronary artery disease (CAD) providing a non-invasive, qualitative, and quantitative assessment of the coronary arteries and pericoronary regions. CCT assesses vascular calcification and coronary lumen narrowing, measures total plaque burden, identifies plaque composition and high-risk plaque features and can even assist with hemodynamic evaluation of coronary lesions. Recent research focuses on computing coronary endothelial shear stress, a potent modulator in the development and progression of atherosclerosis, as well as differentiating an inflammatory from a non-inflammatory pericoronary artery environment using the simple measurement of pericoronary fat attenuation index. In the present review, we discuss the role of the above in the diagnosis of coronary atherosclerosis and the prediction of adverse cardiovascular events. Additionally, we review the current limitations of cardiac computed tomography as an imaging modality and highlight how rapid technological advancements can boost its capacity in predicting cardiovascular risk and guiding clinical decision-making.
Introduction
Cardiac computed tomography (CCT) has emerged in the last decade as an important non-invasive modality for the evaluation of coronary artery disease (CAD) with actively expanding indications. Initial research led to the establishment of a coronary artery calcium score (CACS) for improved risk stratification of asymptomatic patients with intermittent probability for adverse atherosclerotic events; a CACS of zero is associated with low rates of future adverse events (1). Similarly, CCT angiography (CCTA) was shown to be a reliable modality for ruling out CAD in low-risk patients who present to the emergency room with chest pain, leading additionally to decreased length of stay (2). Further studies examined the role of cardiac CCTA in the evaluation of suspected CAD in patients with stable angina. The non-invasive anatomic assessment was compared to standard of care non-invasive functional assessment as an additional or standalone modality (3, 4). The addition of CCTA resulted in a lower risk for long-term coronary death or myocardial infarction, as the increased sensitivity of CCTA for the detection of coronary atherosclerosis led to higher rates of guideline-directed preventive therapy initiation. The use of CCTA as a standalone modality resulted in similar outcomes compared to stress testing or invasive coronary angiography (5). These results highlighted that CCTA is an excellent non-invasive modality for the evaluation of suspected CAD in symptomatic patients; in fact, the latest European Society of Cardiology (ESC) guidelines give a class I recommendation for cardiac CCTA for the evaluation of stable CAD (6). Additionally, however, these results underline the importance of complementary functional assessment that so far had been obtained by stress testing and invasive indices. The latter represents an active area of research in CCTA that could improve its prognostic value for coronary events in patients where coronary atherosclerosis is diagnosed (7, 8). Parallel to the above, a significant number of studies focused on the potential role of CCTA for the identification of “vulnerable plaques”, i.e., coronary atherosclerosis sites that would be associated with a much higher chance of plaque rupture and thrombotic events (9). Although today, the focus has switched to the “vulnerable patient” (10), assessment of high-risk plaques remains important. Finally, CCTA has recently been pivotal in understanding the role of perivascular adipose tissue in atherosclerosis (11) and how associated pericoronary inflammation can be used as a novel prognostic index of adverse coronary outcomes (12) (Figure 1).
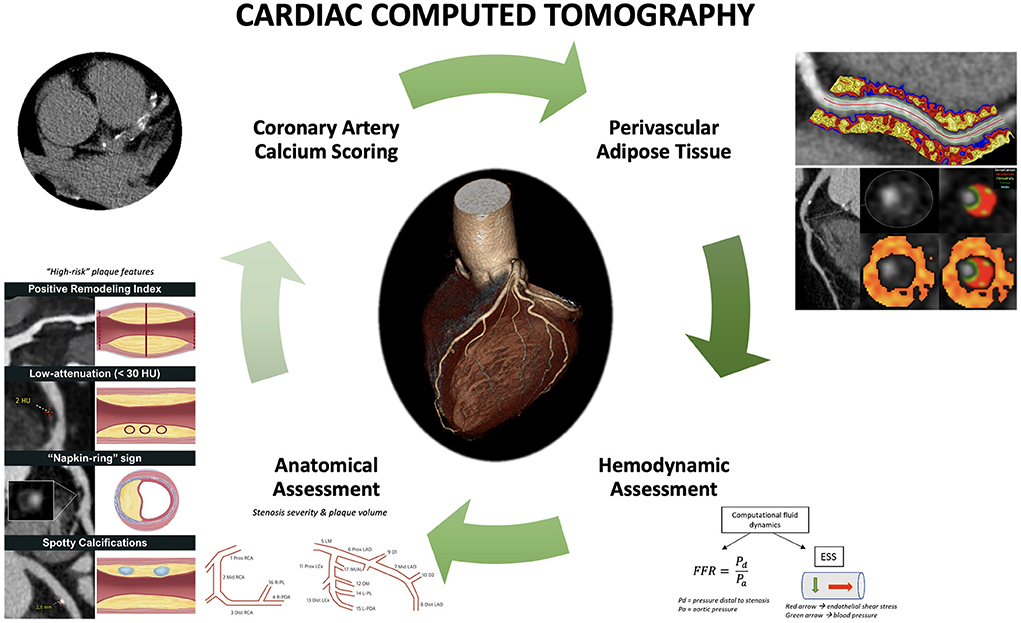
Figure 1. Current and emerging roles of cardiac computed tomography in predicting adverse coronary events. Comprehensive assessment of coronary artery disease with cardiac computed tomography (CCT) includes coronary artery calcium score, anatomic assessment to identify stenosis, plaque volume, and high-risk plaque features, hemodynamic assessment using computational fluid dynamics to compute fractional flow reserve (FFR) and endothelial shear stress (ESS), as well as derivation of perivascular fat attenuation index. Perivascular fat attenuation index figure is owned by: Oxford Academic Cardiovascular CT Core Lab and Lab of Inflammation and Cardiometabolic Diseases at NHLBI, published under Attribution-NonCommercial 2.0 Generic (CC BY-NC 2.0). Link to license: https://creativecommons.org/licenses/by-nc/2.0/.
In the current review, we aim to delineate the contribution of CCT in predicting future adverse cardiovascular outcomes across different clinical scenarios. First, we discuss how CCT can quantitatively assess coronary calcium, the degree of luminal stenosis and total plaque burden, and how these are related to adverse events. We then discuss the role of CCTA based-plaque characterization and its clinical role. Furthermore, we summarize the current state of functional and hemodynamic assessment via CCTA including endothelial shear stress. We elaborate on the role of perivascular fat inflammation in relation to CCTA. Finally, we appraise the limitations of CCT and discuss how technological advances can enhance the capability of CCT in cardiovascular risk prediction in the near future.
Coronary artery calcium score
CCT allows quantification of plaque calcium burden by measuring the Agatston score (13). CACS is based on a low radiation dose, non-contrast CT (14) and represents a simple, quick, inexpensive, and reproducible test. CACS has shown to correlate well with long-term risk of cardiac events when used as a binary or categorical number (1, 15–18). For example, the 10-year risk for adverse atherosclerotic events of a 65-year-old male with hyperlipidemia and medically treated hypertension is over 10%. If the same individual has a CACS of zero, then the risk becomes 3.5%. Importantly, the predictive value of CACS is incremental to that of traditional risk factors and risk calculators that have included CACS have outperformed established risk scores, such as the Framingham score and the 2013 American College of Cardiology (ACC)/American Heart Association (AHA) risk estimator (19). Today, CACS is an established way to better assess the Atherosclerotic Cardiovascular Disease (ASCVD) risk of asymptomatic individuals that otherwise fall into intermediate risk and start appropriate risk factor modification therapy (class IIb recommendation in European Society of Cardiology guidelines and IIa in ACC/AHA). The serial use of CACS is less clear, particularly in patients who are on statin therapy (20).
Nevertheless, the clinical application of CACS must take into account the pre-test probability of CAD, even when CACS is zero, as plaque can be non-calcified, particularly in younger, high-risk patients. Along these lines, clinical decisions for symptomatic patients should not be based solely on CACS, and in fact, CACS is not recommended in that scenario, as shown in the CORE64 trial where >10% of symptomatic patients with CACS of zero had obstructive CAD (21).
Anatomic assessment
Stenosis and plaque volume
Established applications of anatomic assessment via CCTA include the evaluation of symptomatic patients with suspected CAD. The degree of luminal stenosis as well as the presence of obstructive disease on CCTA, defined as >70% stenosis, correlate very well with mortality risk (22, 23). CCTA exhibits moderate to high sensitivity and specificity in discriminating lesion severity (24), with diagnostic accuracy curbed mainly by technical artifacts and limitations. Nevertheless, anatomic assessment with CCTA in the ISCHEMIA trial effectively ruled out left main disease, while clinical and stress testing were weak predictors (25). On the other hand, the advantage of CCTA compared to invasive angiography is the more precise evaluation of the presence and extent of non-obstructive lesions. This is clinically important since it has been shown that recurrent adverse events, specifically cardiovascular death, cardiac arrest, myocardial infarction, or unstable angina, arise from equally from culprit and non-culprit lesions during an index hospitalization (26). In fact, non-culprit lesions frequently cause mild stenoses. In contrast, severe plaque burden has been associated with adverse outcomes regardless of the degree of luminal stenosis (27). Of note, total plaque burden in asymptomatic individuals with type-2 diabetes has demonstrated additional prognostic value for cardiac events compared to clinical risk assessment and CACS (28). Coronary plaque burden on CCTA has also been shown to correlate with levels of high-sensitivity cardiac troponin T (hs-cTnT) in patients that were ruled out for myocardial infarction or even in asymptomatic patients. Even mild CAD has been associated with quantifiable circulating levels of hs-cTnT (29). As evidenced by in vitro studies, these elevated levels of hs-cTnT are not necessarily corresponding to myocardial necrosis, but they suggest that there is sufficient ischemia to result in cell stress, activation of caspase-3, cleavage, and release of cTnT (30). Possible mechanisms of ischemia are physical exercise, emotional stress, dislodgement of thrombi in coronary microvasculature and plaque erosion (31). CCTA-derived parameters can also be directly used for non-invasive evaluation of atherosclerotic features. For instance, positive remodeling of a plaque as well as non-calcified plaque volume have both been associated with increased risk for acute coronary syndromes (ACS) in a meta-analysis of 18 CCTA studies (32). Additional prognostic information could be obtained by serial examinations, as plaque progression has been associated with increased risk for ACS. However, serial CCTAs result in cumulative radiation exposure and are currently not recommended as standard of care. Studies are attempting to identify predictors of coronary plaque progression based on features of a baseline CCTA (33).
High-risk plaques: Characteristics and prognostic value
The concept of high-risk or “vulnerable” plaques is that certain anatomic plaque characteristics can predict plaque rupture, erosion, and thus future thrombotic events. The concept originated from thin cap fibroatheromas, i.e., plaques with a necrotic or lipid core and a thin layer of overlying epithelial cells, which were associated with higher risk of adverse events (34). High-risk characteristics have been identified using CCTA include positive remodeling, low attenuation, spotty calcification, and the “napkin-ring” sign (35). Interestingly, these features respond well to statin use and may regress in serial scans (36). The greatest challenge in the application of these high-risk features is the complex natural history of coronary atherosclerosis itself; most plaque ruptures or erosions are now thought to be asymptomatic events that lead to plaque growth (37). In addition, it has been shown that coronary plaques have dynamic morphology and thin cap fibroatheromas may involve into thick cap fibroatheromas, i.e., lower risk plaques, at follow up (38). Equally importantly, symptomatic plaque rupture or erosion is determined by additional hemodynamic local factors such as endothelial shear stress, as well as systemic factors that determine the individual's thrombophilic state (38); thus prediction of clinically meaningful events is extremely complex and “vulnerable” plaques cannot be easily targeted for revascularization (10). Nevertheless, it is worth discussing the CCTA-derived characteristics of “vulnerable” plaques and their association with coronary events.
Positive remodeling is defined as the compensatory enlargement of the outer vessel wall at the site of an atherosclerotic lesion as the plaque burden increases (39). From a histopathological perspective, this enlargement results from macrophage infiltration and a large amount of necrotic core. CCTA can reliably measure and quantify positive remodeling using an index defined as the ratio of the vessel cross-sectional area at the site of maximal stenosis and the average of proximal and distal reference cross-sectional areas. A threshold of 1.1 is typically preferred in the assessment of CCTA images (40). Several studies have shown that a higher positive remodeling index can identify thin cap fibroatheroma (41, 42), as well as culprit lesions in ACS, but not in stable angina (43). In a prospective study including 74 participants with ACS or stable angina undergoing CCTA, positive remodeling was found in 96% of patients with ACS and ruptured fibrous caps, but only in 20% of those with ACS and intact fibrous caps and in 14% of individuals with stable angina (p <0.001) (44).
Low attenuation (<30 Hounsfield units) is typically used to easily describe plaques with a large lipid-rich necrotic core, as lipids typically have the lowest CCTA attenuation value. Ruptured plaques tend to have lower attenuation compared to stable lesions (44), while a lower plaque attenuation has been found in patients with ACS compared to those with stable angina (45). In a prospective study by Ozaki et al. including patients with ACS or stable angina, low attenuation plaques were more frequently observed in individuals with ACS and ruptured fibrous caps than in those with ACS and intact fibrous cap and those with stable angina (88, 40, and 18%, respectively; p = 0.001) (44). However, it is important to note that the CT attenuation value of a plaque may be influenced by various factors, including the concentration of the contrast agent, plaque burden, slice thickness, image noise, and tube voltage (46). Moreover, although fibrous tissue typically has higher attenuation values compared to lipids, there is a substantial overlap of densities often rendering their distinction from a CCTA impossible (47). Therefore, a reliable differentiation of lipid-rich and fibrous-rich plaques based on CCTA-attenuation remains challenging.
“Napkin-ring” sign. Refers to a specific plaque attenuation pattern with is a central area of low CCTA attenuation in contact with the arterial lumen surrounded by a ring-like higher attenuation plaque tissue (48). The central low attenuation corresponds to a large necrotic core, while the surrounding are of higher CCTA attenuation corresponds to fibrous plaque tissue, both of which are important predictors of plaque rupture (49). Napkin-ring sign is more frequent in thin cap fibroatheromas identified by optic coherence tomography (OCT) (41), and is strongly associated with future adverse cardiac events, independently from other high-risk plaque features (50). In a prospective study of 895 patients, the hazard ratio (HR) of ACS in patients with napkin-ring sign was 5.55 (p < 0.001) indicating a strong and statistically significant association (50). Although napkin ring signs seems to be a specific feature of rupture-prone plaques, its sensitivity remains relatively modest and thus, further in-depth analysis of plaque attenuation patterns is warranted.
Spotty calcifications are defined as <3 mm calcifications in plaques with a density of more than 130 Hounsfield units (51). Calcification marks local inflammation, which can exert mechanical effects on the plaque that make it susceptible to rupture (52). Spotty calcification has been associated with accelerated atherosclerosis progression in individuals with stable angina pectoris (53), while it has also been associated to culprit plaques in patients with ACS (54). In a study including 38 patients with ACS and 33 with stable angina pectoris, spotty calcification was significantly more frequent in ACS lesions (63 vs. 21%, p < 0.001), while large calcification was observed less frequently in ACS lesions than in stable angina (22 vs. 55%; p < 0.05) (43). An important limitation is that CCTA cannot visualize micro-calcifications that are smaller than 0.5 mm in diameter which are thought to be a common feature of unstable coronary lesions (55, 56).
Hemodynamic assessment
Fractional flow reserve
CCTA cannot reliably say whether a lesion is mild, moderate, or severe and thus, current research is focused on achieving a complementary functional assessment of coronary plaques that so far has been obtained by stress testing and invasive indices. If successful, it would tremendously improve the prognostic value of CCTA. Functional flow reserve (FFR) is defined as the ratio between the maximum achievable blood flow in the presence of coronary stenosis and the theoretical maximum flow if the stenosis was not present (57). FFR is measured invasively using pressure wires in the coronary arteries. Invasive FFR >0.75–0.8 indicates hemodynamically non-significant stenosis for which percutaneous coronary intervention can be deferred (58). Recent advantages have allowed a non-invasive calculation of FFR using CCTA and computational fluid dynamics. A negative CT-FFR, i.e., a value above a specific cut-off, carries the promise of safely deferring invasive angiography in patients with stable angina (59). However, CT-FFR has its limitations and introduces an error of its own, while an evidence-based cut-off value for non-invasive FFR is yet to be defined. In a small study of almost 190 patients with suspected CAD and intermediate coronary lesions, CT-FFR >0.8 was used to defer invasive angiography; patients had no adverse events occurring during a median follow-up of 12 months (60). In another study of around 250 patients that presented with acute chest pain and no known CAD, revascularization was deferred when CT-FFR was >0.8 with no difference in occurrence of major adverse cardiac events (61). Current evidence suggests that a dichotomous decision can be made for CT-FFR higher than 0.80 as well as for values equal or lower than 0.70, whereas for the range between 0.71 and 0.80 remains a “gray zone” and referral to invasive angiography should be considered individually (62).
Myocardial perfusion
Another technique that can add functional information in CCT is myocardial computed tomography perfusion (CTP) (63). Moreover, dynamic CTP offers absolute quantification of myocardial blood flow, as in positron-emission tomography (PET) (64). Multicenter studies have demonstrated that myocardial CTP can provide incremental diagnostic value over CCTA alone for the identification of hemodynamically significant coronary artery disease (63, 65), similar to that of magnetic resonance perfusion (65). Additionally, dynamic CTP has been shown to be superior to machine learning empowered CT-FFR for identifying obstructive lesions (66). Dynamic myocardial CTP also has incremental predictive value over CCTA or clinical risk factors for the prediction of future major adverse cardiac events, allowing for improved risk stratification (67, 68). In one study, myocardial blood flow derived from dynamic CTP was the strongest predictor for major adverse cardiovascular events outperforming high risk plaque features and CT-FFR (69).
Endothelial shear stress
Endothelial shear stress (ESS) is the frictional force produced when blood flows through an artery and has proven to trigger biological signaling in the endothelium (70). Physiologic ESS, typically found in straight vascular regions, upregulates anti-inflammatory genes and is atheroprotective. Low ESS, often found at branch points, bifurcations, and regions of high curvature, initiates cellular pathways that promote inflammation and are considered to be atherogenic (71). Specifically in native arteries, low ESS has been associated with the initiation and progression of atherosclerosis, development of high-risk plaque characteristics, need for revascularization, and major adverse cardiovascular events (26, 72). In stented arteries, low ESS has been correlated with neo-intima hyperplasia and neo-atherosclerosis, which can ultimately lead to further adverse cardiovascular events, including stent restenosis (73, 74). ESS is a promising hemodynamic index that can be evaluated using CCTA and computational fluid dynamics. As the lower imaging resolution of CCTA can have an impact on the accuracy of the estimated ESS, higher-resolution models reconstructed from a fusion of CCTA with intravascular imaging techniques, such as intravascular ultrasound (IVUS) and OCT, have been developed (75). Studies using CCTA-based models have shown that ESS assessment can provide incremental value in discriminating coronary segments more likely to exhibit atherosclerotic disease progression (76). In the Exploring the Mechanism of Plaque Rupture in Acute Coronary Syndrome Using Coronary CT Angiography and Computational Fluid Dynamics (EMERALD) study including 72 patients with ACS that had previously underwent CCTA, hemodynamic assessment, including ESS evaluation, provided additional value in identification of high-risk plaques that had ultimately caused ACS (72). However, further information on the clinical utility of CCTA-ESS remains to be seen.
Perivascular adipose tissue
Recent evidence suggests that perivascular adipose tissue (PVAT) is linked to atherosclerosis as a key regulator and sensor of coronary inflammation (77, 78). PVAT lies in proximity with the vascular wall and plays an important role in the pathogenic process of atherosclerosis by regulating the local microenvironment through the release of a variety of substances, such as bioactive adipokines, cytokines, and chemokines (77, 79). Pericoronary fat attenuation index (FAI) is a novel CCTA-derived biomarker based on the concept that spatial changes in composition induced by inflammation cause a shift in CT attenuation toward more negative HU values (80). FAI is increased in patients with CAD compared to healthy individuals and is particularly increased around culprit lesions of patients presenting with ACS (80). In the Cardiovascular Risk Prediction using Computed Tomography (CRISP-CT) study, two independent cohorts with a total of 3,912 participants undergoing CCT were used to derive and validate the prognostic value of perivascular fat attenuation mapping (12). Based on the results, higher FAI values around proximal right coronary artery and left anterior descending artery were also associated with a higher risk for all-cause death and cardiac death (12). Finally, although FAI has been shown to be modifiable, as it decreased significantly when measured 5 weeks after an index event (80), whether risk-reduction therapies, such as statins, can reverse FAI is yet to be investigated. Future incorporation of CCT derived indices describing perivascular adipose tissue inflammation may improve risk assessment in patients with CAD (Table 1).
Current limitations
Despite its many strengths, CCT for the evaluation of CAD has a few limitations. First, a variety of factors can introduce noise, therefore limiting image quality. Examples include increased heart rate, arrhythmias, high-density materials (e.g., calcium, stents), high body mass index, and poor patient cooperation (e.g., movement, inappropriate breath control). It is typical for calcifications to appear falsely enlarged due to blooming or partial volume artifacts and thus, result in overestimation of the extent of CAD (81). Studies have shown that CCT may provide conflicting results with overestimation or underestimation of the lumen area when compared to IVUS (82, 83). For these reasons, invasive coronary angiography remains the gold standard for coronary lesions and in many cases, CCT can only be used as a gatekeeper to more invasive testing. Additionally, different scanners, protocols, and technical parameters can lead to a variation of results and false interpretations (84). Regarding the use of CCT, another important concern is exposure to radiation and the risk of cancer (85). Furthermore, the contrast required for CCTA carries the risk of contrast-induced nephropathy (86). Finally, as CCT and CCTA are primarily anatomic modalities that assess coronary stenosis, plaque burden and characteristics, the addition of a functional test to increase diagnostic accuracy is often clinically useful (87, 88). In other words, a multimodality or hybrid imaging approach to CAD can be pursued in the appropriate clinical setting.
Cardiac computed tomography and “omics”: Radiomics, proteomics, and lipidomics
The development of radiomics, as well as the integration of proteomics and lipidomics with CCT images, show promise for increased diagnostic and prognostic performance of CCT images, yielding potentially important clinical benefits. In fact, a radiomic-based machine learning model to identify advanced atheromatous lesions was found to be superior when compared to visual assessment (89). Similarly, in the CRISP-CT study, radiomic mapping of the perivascular fat was shown to offer incremental value for predicting adverse cardiac events compared to traditional risk profile assessment or presence of high-risk plaque features (90). Additionally, several studies have screened the proteome aiming to investigate whether specific proteins can relate to the presence or extent of coronary atherosclerosis. To illustrate, in a CCTA-based cohort, four proteins involved in vascular processes were found to be specifically associated with either low or high CAD burden (91). Similarly, other studies have assessed the association between lipid profiles with atherosclerotic plaque findings (92). In general, the new era of proteomics and lipidomics could provide a deeper insight to the atherosclerotic process beyond traditional risk factors as well as identify potential and novel treatment targets promising a more individualized patient care in the future.
Dual-energy and photon-counting computed tomography
In the past few years, new CCT techniques such as dual-energy CCT and photon-counting CCT have aimed at improving the spatial resolution and the contrast-to-noise ratio of CT scanners (93). Dual-energy CCT uses two CCT datasets acquired with different photon spectra and tube potentials. With regards to CAD, it can enhance luminal assessment, evaluation of atherosclerotic plaque composition and evaluation of myocardial perfusion (94). Future studies are still needed to ensure wide external validation and existence of incremental clinical value when compared to already established technology. Photon-counting CCT allow for direct detection of incident X-ray photons, in contrast to energy-integrating scanners (i.e., those currently used in clinical practice), which are based on light photon detectors that are converted to electric signals at scintillation layer (95). This allows for increased signal to noise ratio, which in turn allows for better image quality, decreased radiation doses and elimination of beam hardening artifacts. In addition, photon-counting CCT could enhance the ability of the CCT scanner to detect different combinations of contrast agents, such as atherosclerotic plaque-specific nanoparticles (96). Although photon-counting CCT holds a great promise for the future, its access is currently limited to a few centers, and many technical challenges still need to be overcome prior to wide implementation.
Conclusion and future perspectives
CCT has become more widely available and is currently viewed as a first-line diagnostic test CAD. In certain asymptomatic patients, it also provides improved risk stratification, guiding prevention of future adverse events. Further research on image processing could bridge the diagnostic accuracy gap between invasive and non-invasive coronary angiography in regards to grading of calcified plaques and hemodynamic evaluation of lesions. Evaluation of pericoronary fat inflammation could also be broadly adopted for the prediction of future adverse events. In addition, technologic developments aiming at reducing radiation (90) could result in the expansion of CCTA and help clarify the role of serial scanning. New techniques, such as dual-energy CCT and photon-counting CCT also hold promise at achieving higher spatial resolution and improving contrast-to-noise ratio. Finally, incorporating CCT and CCTA with machine learning and computational fluid dynamics could lead to more precise and individualized risk assessment.
Author contributions
All authors contributed to the writing and revision of the manuscript and approved the final manuscript.
Funding
MINOCA-GR study (Role of CCT in the diagnostic evaluation and risk stratification of patients with myocardial infarction and non-obstructive coronary arteries), an investigator-initiated study, supported by Menarini Hellas S.A (Ref. No. 27.11.2020/72059).
Conflict of interest
The authors declare that the research was conducted in the absence of any commercial or financial relationships that could be construed as a potential conflict of interest.
Publisher's note
All claims expressed in this article are solely those of the authors and do not necessarily represent those of their affiliated organizations, or those of the publisher, the editors and the reviewers. Any product that may be evaluated in this article, or claim that may be made by its manufacturer, is not guaranteed or endorsed by the publisher.
References
1. Budoff MJ, Young R, Lopez VA, Kronmal RA, Nasir K, Blumenthal RS, et al. Progression of coronary calcium and incident coronary heart disease events: MESA (multi-ethnic study of atherosclerosis). J Am Coll Cardiol. (2013) 61:1231–9. doi: 10.1016/j.jacc.2012.12.035
2. Hoffmann U, Truong QA, Schoenfeld DA, Chou ET, Woodard PK, Nagurney JT, et al. Coronary CT angiography versus standard evaluation in acute chest pain. N Engl J Med. (2012) 367:299–308. doi: 10.1056/NEJMoa1201161
3. Investigators SCOT-HEART, Newby DE., Adamson PD, Berry C, Boon NA, Dweck MR, et al. Coronary CT angiography and 5-year risk of myocardial infarction. N Engl J Med. (2018) 379:924–33. doi: 10.1056/NEJMoa1805971
4. Douglas PS, Hoffmann U, Patel MR, Mark DB, Al-Khalidi HR, Cavanaugh B, et al. Outcomes of anatomical versus functional testing for coronary artery disease. N Engl J Med. (2015) 372:1291–300. doi: 10.1056/NEJMoa1415516
5. Group DT, Maurovich-Horvat P, Bosserdt M, Kofoed KF, Rieckmann N, Benedek T, et al. CT or invasive coronary angiography in stable chest pain. N Engl J Med. (2022). 386:1591–602. doi: 10.1056/NEJMoa2200963
6. Knuuti J, Wijns W, Saraste A, Capodanno D, Barbato E, Funck-Brentano C, et al. 2019 ESC Guidelines for the diagnosis and management of chronic coronary syndromes. Eur Heart J. (2020) 41:407–77. doi: 10.1093/eurheartj/ehz425
7. Min JK, Leipsic J, Pencina MJ, Berman DS, Koo BK, van Mieghem C, et al. Diagnostic accuracy of fractional flow reserve from anatomic CT angiography. JAMA. (2012) 308:1237–45. doi: 10.1001/2012.jama.11274
8. Marwan M. Computational fluid dynamics: can computed tomography imaging compete with cath-lab physiology? Cardiovasc Res. (2019) 115:e41–3. doi: 10.1093/cvr/cvz059
9. Lu G, Ye W, Ou J, Li X, Tan Z, Li T, et al. Coronary computed tomography angiography assessment of high-risk plaques in predicting acute coronary syndrome. Front Cardiovasc Med. (2021) 8:743538. doi: 10.3389/fcvm.2021.743538
10. Arbab-Zadeh A, Fuster V. From detecting the vulnerable plaque to managing the vulnerable patient: JACC state-of-the-art review. J Am Coll Cardiol. (2019) 74:1582–93. doi: 10.1016/j.jacc.2019.07.062
11. Mancio J, Oikonomou EK, Antoniades C. Perivascular adipose tissue and coronary atherosclerosis. Heart. (2018) 104:1654–62. doi: 10.1136/heartjnl-2017-312324
12. Oikonomou EK, Marwan M, Desai MY, Mancio J, Alashi A, Hutt Centeno E, et al. Non-invasive detection of coronary inflammation using computed tomography and prediction of residual cardiovascular risk (the CRISP CT study): a post-hoc analysis of prospective outcome data. Lancet. (2018) 392:929–39. doi: 10.1016/S0140-6736(18)31114-0
13. Agatston AS, Janowitz WR, Hildner FJ, Zusmer NR, Viamonte M Jr., Detrano R. Quantification of coronary artery calcium using ultrafast computed tomography. J Am Coll Cardiol. (1990) 15:827–32. doi: 10.1016/0735-1097(90)90282-T
14. Blankstein R, Gupta A, Rana JS, Nasir K. The implication of coronary artery calcium testing for cardiovascular disease prevention and diabetes. Endocrinol Metab. (2017) 32:47–57. doi: 10.3803/EnM.2017.32.1.47
15. Obaid DR, Calvert PA, Gopalan D, Parker RA, Hoole SP, West NE, et al. Atherosclerotic plaque composition and classification identified by coronary computed tomography: assessment of computed tomography-generated plaque maps compared with virtual histology intravascular ultrasound and histology. Circ Cardiovasc Imaging. (2013) 6:655–64. doi: 10.1161/CIRCIMAGING.112.000250
16. Sangiorgi G, Rumberger JA, Severson A, Edwards WD, Gregoire J, Fitzpatrick LA, et al. Arterial calcification and not lumen stenosis is highly correlated with atherosclerotic plaque burden in humans: a histologic study of 723 coronary artery segments using nondecalcifying methodology. J Am Coll Cardiol. (1998) 31:126–33. doi: 10.1016/S0735-1097(97)00443-9
17. Elias-Smale SE, Proenca RV, Koller MT, Kavousi M, van Rooij FJ, Hunink MG, et al. Coronary calcium score improves classification of coronary heart disease risk in the elderly: the Rotterdam study. J Am Coll Cardiol. (2010) 56:1407–14. doi: 10.1016/j.jacc.2010.06.029
18. Razavi A, Iftekhar Uddin S, Dardari Z, Berman D, Budoff M, Miedema M, et al. Coronary artery calcium for risk stratification of sudden cardiac death: the coronary artery calcium consortium. JACC Cardiovasc Imaging. (2022). doi: 10.1016/j.jcmg.2022.02.011
19. McClelland RL, Jorgensen NW, Budoff M, Blaha MJ, Post WS, Kronmal RA, et al. 10-year coronary heart disease risk prediction using coronary artery calcium and traditional risk factors: derivation in the MESA (multi-ethnic study of atherosclerosis) with validation in the HNR (Heinz Nixdorf Recall) Study and the DHS (Dallas Heart Study). J Am Coll Cardiol. (2015) 66:1643–53. doi: 10.1016/j.jacc.2015.08.035
20. Nakazato R, Gransar H, Berman DS, Cheng VY, Lin FY, Achenbach S, et al. Statins use and coronary artery plaque composition: results from the International Multicenter CONFIRM Registry. Atherosclerosis. (2012) 225:148–53. doi: 10.1016/j.atherosclerosis.2012.08.002
21. Gottlieb I, Miller JM, Arbab-Zadeh A, Dewey M, Clouse ME, Sara L, et al. The absence of coronary calcification does not exclude obstructive coronary artery disease or the need for revascularization in patients referred for conventional coronary angiography. J Am Coll Cardiol. (2010) 55:627–34. doi: 10.1016/j.jacc.2009.07.072
22. Min JK, Shaw LJ, Devereux RB, Okin PM, Weinsaft JW, Russo DJ, et al. Prognostic value of multidetector coronary computed tomographic angiography for prediction of all-cause mortality. J Am Coll Cardiol. (2007) 50:1161–70. doi: 10.1016/j.jacc.2007.03.067
23. Min JK, Dunning A, Lin FY, Achenbach S, Al-Mallah M, Budoff MJ, et al. Age- and sex-related differences in all-cause mortality risk based on coronary computed tomography angiography findings results from the International Multicenter CONFIRM (Coronary CT Angiography Evaluation for Clinical Outcomes: An International Multicenter Registry) of 23,854 patients without known coronary artery disease. J Am Coll Cardiol. (2011) 58:849–60. doi: 10.1016/j.jacc.2011.02.074
24. Miller JM, Rochitte CE, Dewey M, Arbab-Zadeh A, Niinuma H, Gottlieb I, et al. Diagnostic performance of coronary angiography by 64-row CT. N Engl J Med. (2008) 359:2324–36. doi: 10.1056/NEJMoa0806576
25. Senior R, Reynolds HR, Min JK, Berman DS, Picard MH, Chaitman BR, et al. Predictors of left main coronary artery disease in the ISCHEMIA trial. J Am Coll Cardiol. (2022) 79:651–61. doi: 10.1016/j.jacc.2021.11.052
26. Stone PH, Maehara A, Coskun AU, Maynard CC, Zaromytidou M, Siasos G, et al. Role of low endothelial shear stress and plaque characteristics in the prediction of nonculprit major adverse cardiac events: the PROSPECT Study. JACC Cardiovasc Imaging. (2018) 11:462–71. doi: 10.1016/j.jcmg.2017.01.031
27. Lee SE, Sung JM, Rizvi A, Lin FY, Kumar A, Hadamitzky M, et al. Quantification of coronary atherosclerosis in the assessment of coronary artery disease. Circ Cardiovasc Imaging. (2018) 11:e007562. doi: 10.1161/CIRCIMAGING.117.007562
28. Halon DA, Azencot M, Rubinshtein R, Zafrir B, Flugelman MY, Lewis BS. Coronary computed tomography (CT) angiography as a predictor of cardiac and noncardiac vascular events in asymptomatic type 2 diabetics: a 7-year population-based cohort study. J Am Heart Assoc. (2016) 5:e003226. doi: 10.1161/JAHA.116.003226
29. Laufer EM, Mingels AM, Winkens MH, Joosen IA, Schellings MW, Leiner T, et al. The extent of coronary atherosclerosis is associated with increasing circulating levels of high sensitive cardiac troponin T. Arterioscler Thromb Vasc Biol. (2010) 30:1269–75. doi: 10.1161/ATVBAHA.109.200394
30. Feng YJ, Chen C, Fallon JT, Lai T, Chen L, Knibbs DR, et al. Comparison of cardiac troponin I, creatine kinase-MB, and myoglobin for detection of acute ischemic myocardial injury in a swine model. Am J Clin Pathol. (1998) 110:70–7. doi: 10.1093/ajcp/110.1.70
31. Lee KK, Bularga A, O'Brien R, Ferry AV, Doudesis D, Fujisawa T, et al. Troponin-guided coronary computed tomographic angiography after exclusion of myocardial infarction. J Am Coll Cardiol. (2021) 78:1407–17. doi: 10.1016/j.jacc.2021.07.055
32. Thomsen C, Abdulla J. Characteristics of high-risk coronary plaques identified by computed tomographic angiography and associated prognosis: a systematic review and meta-analysis. Eur Heart J Cardiovasc Imaging. (2016) 17:120–9. doi: 10.1093/ehjci/jev325
33. Stone PH, Saito S, Takahashi S, Makita Y, Nakamura S, Kawasaki T, et al. Prediction of progression of coronary artery disease and clinical outcomes using vascular profiling of endothelial shear stress and arterial plaque characteristics: the PREDICTION Study. Circulation. (2012) 126:172–81. doi: 10.1161/CIRCULATIONAHA.112.096438
34. Arbab-Zadeh A, Nakano M, Virmani R, Fuster V. Acute coronary events. Circulation. (2012) 125:1147–56. doi: 10.1161/CIRCULATIONAHA.111.047431
35. Kim U, Leipsic JA, Sellers SL, Shao M, Blanke P, Hadamitzky M, et al. Natural history of diabetic coronary atherosclerosis by quantitative measurement of serial coronary computed tomographic angiography: results of the PARADIGM Study. JACC Cardiovasc Imaging. (2018) 11:1461–71. doi: 10.1016/j.jcmg.2018.04.009
36. Lee SE, Chang HJ, Sung JM, Park HB, Heo R, Rizvi A, et al. Effects of statins on coronary atherosclerotic plaques: the PARADIGM Study. JACC Cardiovasc Imaging. (2018) 11:1475–84. doi: 10.1016/j.jcmg.2018.04.015
37. Davies MJ. Acute coronary thrombosis–the role of plaque disruption and its initiation and prevention. Eur Heart J. (1995) 16(Suppl. L):3–7. doi: 10.1093/eurheartj/16.suppl_L.3
38. Arbab-Zadeh A, Fuster V. The myth of the “vulnerable plaque”: transitioning from a focus on individual lesions to atherosclerotic disease burden for coronary artery disease risk assessment. J Am Coll Cardiol. (2015) 65:846–55. doi: 10.1016/j.jacc.2014.11.041
39. Nakamura M, Nishikawa H, Mukai S, Setsuda M, Nakajima K, Tamada H, et al. Impact of coronary artery remodeling on clinical presentation of coronary artery disease: an intravascular ultrasound study. J Am Coll Cardiol. (2001) 37:63–9. doi: 10.1016/S0735-1097(00)01097-4
40. Achenbach S, Ropers D, Hoffmann U, MacNeill B, Baum U, Pohle K, et al. Assessment of coronary remodeling in stenotic and nonstenotic coronary atherosclerotic lesions by multidetector spiral computed tomography. J Am Coll Cardiol. (2004) 43:842–7. doi: 10.1016/j.jacc.2003.09.053
41. Kashiwagi M, Tanaka A, Kitabata H, Tsujioka H, Kataiwa H, Komukai K, et al. Feasibility of noninvasive assessment of thin-cap fibroatheroma by multidetector computed tomography. JACC Cardiovasc Imaging. (2009) 2:1412–9. doi: 10.1016/j.jcmg.2009.09.012
42. Ito T, Terashima M, Kaneda H, Nasu K, Matsuo H, Ehara M, et al. Comparison of in vivo assessment of vulnerable plaque by 64-slice multislice computed tomography versus optical coherence tomography. Am J Cardiol. (2011) 107:1270–7. doi: 10.1016/j.amjcard.2010.12.036
43. Motoyama S, Kondo T, Sarai M, Sugiura A, Harigaya H, Sato T, et al. Multislice computed tomographic characteristics of coronary lesions in acute coronary syndromes. J Am Coll Cardiol. (2007) 50:319–26. doi: 10.1016/j.jacc.2007.03.044
44. Ozaki Y, Okumura M, Ismail TF, Motoyama S, Naruse H, Hattori K, et al. Coronary CT angiographic characteristics of culprit lesions in acute coronary syndromes not related to plaque rupture as defined by optical coherence tomography and angioscopy. Eur Heart J. (2011) 32:2814–23. doi: 10.1093/eurheartj/ehr189
45. Kim SY, Kim KS, Seung MJ, Chung JW, Kim JH, Mun SH, et al. The culprit lesion score on multi-detector computed tomography can detect vulnerable coronary artery plaque. Int J Cardiovasc Imaging. (2010) 26:245–52. doi: 10.1007/s10554-010-9712-2
46. Cademartiri F, Mollet NR, Runza G, Bruining N, Hamers R, Somers P, et al. Influence of intracoronary attenuation on coronary plaque measurements using multislice computed tomography: observations in an ex vivo model of coronary computed tomography angiography. Eur Radiol. (2005) 15:1426–31. doi: 10.1007/s00330-005-2697-x
47. Voros S, Rinehart S, Qian Z, Vazquez G, Anderson H, Murrieta L, et al. Prospective validation of standardized, 3-dimensional, quantitative coronary computed tomographic plaque measurements using radiofrequency backscatter intravascular ultrasound as reference standard in intermediate coronary arterial lesions: results from the ATLANTA (assessment of tissue characteristics, lesion morphology, and hemodynamics by angiography with fractional flow reserve, intravascular ultrasound and virtual histology, and noninvasive computed tomography in atherosclerotic plaques) I study. JACC Cardiovasc Interv. (2011) 4:198–208. doi: 10.1016/j.jcin.2010.10.008
48. Maurovich-Horvat P, Hoffmann U, Vorpahl M, Nakano M, Virmani R, Alkadhi H. The napkin-ring sign: CT signature of high-risk coronary plaques? JACC Cardiovasc Imaging. (2010) 3:440–4. doi: 10.1016/j.jcmg.2010.02.003
49. Finn AV, Nakano M, Narula J, Kolodgie FD, Virmani R. Concept of vulnerable/unstable plaque. Arterioscler Thromb Vasc Biol. (2010) 30:1282–92. doi: 10.1161/ATVBAHA.108.179739
50. Otsuka K, Fukuda S, Tanaka A, Nakanishi K, Taguchi H, Yoshikawa J, et al. Napkin-ring sign on coronary CT angiography for the prediction of acute coronary syndrome. JACC Cardiovasc Imaging. (2013) 6:448–57. doi: 10.1016/j.jcmg.2012.09.016
51. Ferencik M, Schlett CL, Ghoshhajra BB, Kriegel MF, Joshi SB, Maurovich-Horvat P, et al. A computed tomography-based coronary lesion score to predict acute coronary syndrome among patients with acute chest pain and significant coronary stenosis on coronary computed tomographic angiogram. Am J Cardiol. (2012) 110:183–9. doi: 10.1016/j.amjcard.2012.02.066
52. Mori H, Torii S, Kutyna M, Sakamoto A, Finn AV, Virmani R. Coronary artery calcification and its progression: what does it really mean? JACC Cardiovasc Imaging. (2018) 11:127–42. doi: 10.1016/j.jcmg.2017.10.012
53. Kataoka Y, Wolski K, Uno K, Puri R, Tuzcu EM, Nissen SE, et al. Spotty calcification as a marker of accelerated progression of coronary atherosclerosis: insights from serial intravascular ultrasound. J Am Coll Cardiol. (2012) 59:1592–7. doi: 10.1016/j.jacc.2012.03.012
54. Ehara S, Kobayashi Y, Yoshiyama M, Shimada K, Shimada Y, Fukuda D, et al. Spotty calcification typifies the culprit plaque in patients with acute myocardial infarction: an intravascular ultrasound study. Circulation. (2004) 110:3424–9. doi: 10.1161/01.CIR.0000148131.41425.E9
55. Burke AP, Weber DK, Kolodgie FD, Farb A, Taylor AJ, Virmani R. Pathophysiology of calcium deposition in coronary arteries. Herz. (2001) 26:239–44. doi: 10.1007/PL00002026
56. Joshi NV, Vesey AT, Williams MC, Shah AS, Calvert PA, Craighead FH, et al. 18F-fluoride positron emission tomography for identification of ruptured and high-risk coronary atherosclerotic plaques: a prospective clinical trial. Lancet. (2014) 383:705–13. doi: 10.1016/S0140-6736(13)61754-7
57. Pijls NH, Van Gelder B, Van der Voort P, Peels K, Bracke FA, Bonnier HJ, et al. Fractional flow reserve. A useful index to evaluate the influence of an epicardial coronary stenosis on myocardial blood flow. Circulation. (1995) 92:3183–93. doi: 10.1161/01.CIR.92.11.3183
58. Pijls NH, De Bruyne B, Peels K, Van Der Voort PH, Bonnier HJ, Bartunek JKJJ, et al. Measurement of fractional flow reserve to assess the functional severity of coronary-artery stenoses. N Engl J Med. (1996) 334:1703–8. doi: 10.1056/NEJM199606273342604
59. Jensen JM, Botker HE, Mathiassen ON, Grove EL, Ovrehus KA, Pedersen KB, et al. Computed tomography derived fractional flow reserve testing in stable patients with typical angina pectoris: influence on downstream rate of invasive coronary angiography. Eur Heart J Cardiovasc Imaging. (2018) 19:405–14. doi: 10.1093/ehjci/jex068
60. Norgaard BL, Hjort J, Gaur S, Hansson N, Botker HE, Leipsic J, et al. Clinical use of coronary CTA-derived FFR for decision-making in stable CAD. JACC Cardiovasc Imaging. (2017) 10:541–50. doi: 10.1016/j.jcmg.2015.11.025
61. Chinnaiyan KM, Safian RD, Gallagher ML, George J, Dixon SR, Bilolikar AN, et al. Clinical use of CT-derived fractional flow reserve in the emergency department. JACC Cardiovasc Imaging. (2020) 13:452–61. doi: 10.1016/j.jcmg.2019.05.025
62. Matsumura-Nakano Y, Kawaji T, Shiomi H, Kawai-Miyake K, Kataoka M, Koizumi K, et al. Optimal cutoff value of fractional flow reserve derived from coronary computed tomography angiography for predicting hemodynamically significant coronary artery disease. Circ Cardiovasc Imaging. (2019) 12:e008905. doi: 10.1161/CIRCIMAGING.119.008905
63. Nous FMA, Geisler T, Kruk MBP, Alkadhi H, Kitagawa K, Vliegenthart R, et al. Dynamic myocardial perfusion CT for the detection of hemodynamically significant coronary artery disease. JACC Cardiovasc Imaging. (2022) 15:75–87. doi: 10.1016/j.jcmg.2021.07.021
64. Branch KR, Haley RD, Bittencourt MS, Patel AR, Hulten E, Blankstein R. Myocardial computed tomography perfusion. Cardiovasc Diagn Ther. (2017) 7:452–62. doi: 10.21037/cdt.2017.06.11
65. Rief M, Chen MY, Vavere AL, Kendziora B, Miller JM, Bandettini WP, et al. Coronary artery disease: analysis of diagnostic performance of CT perfusion and MR perfusion imaging in comparison with quantitative coronary angiography and SPECT-multicenter prospective trial. Radiology. (2018) 286:461–70. doi: 10.1148/radiol.2017162447
66. Li Y, Yu M, Dai X, Lu Z, Shen C, Wang Y, et al. Detection of hemodynamically significant coronary stenosis: CT myocardial perfusion versus machine learning CT fractional flow reserve. Radiology. (2019) 293:305–14. doi: 10.1148/radiol.2019190098
67. Nakamura S, Kitagawa K, Goto Y, Omori T, Kurita T, Yamada A, et al. Incremental prognostic value of myocardial blood flow quantified with stress dynamic computed tomography perfusion imaging. JACC Cardiovasc Imaging. (2019) 12:1379–87. doi: 10.1016/j.jcmg.2018.05.021
68. van Assen M, De Cecco CN, Eid M, von Knebel Doeberitz P, Scarabello M, Lavra F, et al. Prognostic value of CT myocardial perfusion imaging and CT-derived fractional flow reserve for major adverse cardiac events in patients with coronary artery disease. J Cardiovasc Comput Tomogr. (2019) 13:26–33. doi: 10.1016/j.jcct.2019.02.005
69. Yu L, Lu Z, Dai X, Shen C, Zhang L, Zhang J. Prognostic value of CT-derived myocardial blood flow, CT fractional flow reserve and high-risk plaque features for predicting major adverse cardiac events. Cardiovasc Diagn Ther. (2021) 11:956–66. doi: 10.21037/cdt-21-219
70. Gijsen F, Katagiri Y, Barlis P, Bourantas C, Collet C, Coskun U, et al. Expert recommendations on the assessment of wall shear stress in human coronary arteries: existing methodologies, technical considerations, and clinical applications. Eur Heart J. (2019) 40:3421–33. doi: 10.1093/eurheartj/ehz551
71. Davies PF. Hemodynamic shear stress and the endothelium in cardiovascular pathophysiology. Nat Clin Pract Cardiovasc Med. (2009) 6:16–26. doi: 10.1038/ncpcardio1397
72. Lee JM, Choi G, Koo BK, Hwang D, Park J, Zhang J, et al. Identification of high-risk plaques destined to cause acute coronary syndrome using coronary computed tomographic angiography and computational fluid dynamics. JACC Cardiovasc Imaging. (2019) 12:1032–43. doi: 10.1016/j.jcmg.2018.01.023
73. Torii R, Stettler R, Raber L, Zhang YJ, Karanasos A, Dijkstra J, et al. Implications of the local hemodynamic forces on the formation and destabilization of neoatherosclerotic lesions. Int J Cardiol. (2018) 272:7–12. doi: 10.1016/j.ijcard.2018.06.065
74. Papafaklis MI, Bourantas CV, Theodorakis PE, Katsouras CS, Naka KK, Fotiadis DI, et al. The effect of shear stress on neointimal response following sirolimus- and paclitaxel-eluting stent implantation compared with bare-metal stents in humans. JACC Cardiovasc Interv. (2010) 3:1181–9. doi: 10.1016/j.jcin.2010.08.018
75. van der Giessen AG, Schaap M, Gijsen FJ, Groen HC, van Walsum T, Mollet NR, et al. 3D fusion of intravascular ultrasound and coronary computed tomography for in-vivo wall shear stress analysis: a feasibility study. Int J Cardiovasc Imaging. (2010) 26:781–96. doi: 10.1007/s10554-009-9546-y
76. Park JB, Choi G, Chun EJ, Kim HJ, Park J, Jung JH, et al. Computational fluid dynamic measures of wall shear stress are related to coronary lesion characteristics. Heart. (2016) 102:1655–61. doi: 10.1136/heartjnl-2016-309299
77. Oikonomou EK, West HW, Antoniades C. Cardiac computed tomography: assessment of coronary inflammation and other plaque features. Arterioscler Thromb Vasc Biol. (2019) 39:2207–19. doi: 10.1161/ATVBAHA.119.312899
78. Liu Y, Sun Y, Hu C, Liu J, Gao A, Han H, et al. Perivascular adipose tissue as an indication, contributor to, and therapeutic target for atherosclerosis. Front Physiol. (2020) 11:615503. doi: 10.3389/fphys.2020.615503
79. Antonopoulos AS, Margaritis M, Coutinho P, Shirodaria C, Psarros C, Herdman L, et al. Adiponectin as a link between type 2 diabetes and vascular NADPH oxidase activity in the human arterial wall: the regulatory role of perivascular adipose tissue. Diabetes. (2015) 64:2207–19. doi: 10.2337/db14-1011
80. Antonopoulos AS, Sanna F, Sabharwal N, Thomas S, Oikonomou EK, Herdman L, et al. Detecting human coronary inflammation by imaging perivascular fat. Sci Transl Med. (2017) 9:aal2658. doi: 10.1126/scitranslmed.aal2658
81. Li P, Xu L, Yang L, Wang R, Hsieh J, Sun Z, et al. Blooming artifact reduction in coronary artery calcification by a new de-blooming algorithm: initial study. Sci Rep. (2018) 8:6945. doi: 10.1038/s41598-018-25352-5
82. Fujimoto S, Kondo T, Kodama T, Fujisawa Y, Groarke J, Kumamaru KK, et al. A novel method for non-invasive plaque morphology analysis by coronary computed tomography angiography. Int J Cardiovasc Imaging. (2014) 30:1373–82. doi: 10.1007/s10554-014-0461-5
83. Conte E, Mushtaq S, Pontone G, Li Piani L, Ravagnani P, Galli S, et al. Plaque quantification by coronary computed tomography angiography using intravascular ultrasound as a reference standard: a comparison between standard and last generation computed tomography scanners. Eur Heart J Cardiovasc Imaging. (2020) 21:191–201. doi: 10.1093/ehjci/jez089
84. Ghekiere O, Salgado R, Buls N, Leiner T, Mancini I, Vanhoenacker P, et al. Image quality in coronary CT angiography: challenges and technical solutions. Br J Radiol. (2017) 90:20160567. doi: 10.1259/bjr.20160567
85. Einstein AJ, Henzlova MJ, Rajagopalan S. Estimating risk of cancer associated with radiation exposure from 64-slice computed tomography coronary angiography. JAMA. (2007) 298:317–23. doi: 10.1001/jama.298.3.317
86. Maaniitty T, Stenstrom I, Uusitalo V, Ukkonen H, Kajander S, Bax JJ, et al. Incidence of persistent renal dysfunction after contrast enhanced coronary CT angiography in patients with suspected coronary artery disease. Int J Cardiovasc Imaging. (2016) 32:1567–75. doi: 10.1007/s10554-016-0935-8
87. Schuijf JD, Wijns W, Jukema JW, Atsma DE, de Roos A, Lamb HJ, et al. Relationship between noninvasive coronary angiography with multi-slice computed tomography and myocardial perfusion imaging. J Am Coll Cardiol. (2006) 48:2508–14. doi: 10.1016/j.jacc.2006.05.080
88. Gaemperli O, Schepis T, Valenta I, Koepfli P, Husmann L, Scheffel H, et al. Functionally relevant coronary artery disease: comparison of 64-section CT angiography with myocardial perfusion SPECT. Radiology. (2008) 248:414–23. doi: 10.1148/radiol.2482071307
89. Kolossvary M, Karady J, Kikuchi Y, Ivanov A, Schlett CL, Lu MT, et al. Radiomics versus visual and histogram-based assessment to identify atheromatous lesions at coronary CT angiography: an ex vivo study. Radiology. (2019) 293:89–96. doi: 10.1148/radiol.2019190407
90. Oikonomou EK, Siddique M, Antoniades C. Artificial intelligence in medical imaging: a radiomic guide to precision phenotyping of cardiovascular disease. Cardiovasc Res. (2020) 116:2040–54. doi: 10.1093/cvr/cvaa021
91. Ferrannini E, Manca ML, Ferrannini G, Andreotti F, Andreini D, Latini R, et al. Differential proteomics of cardiovascular risk and coronary artery disease in humans. Front Cardiovasc Med. (2021) 8:790289. doi: 10.3389/fcvm.2021.790289
92. Nakazato R, Gransar H, Berman DS, Cheng VY, Lin FY, Achenbach S, et al. Relationship of low- and high-density lipoproteins to coronary artery plaque composition by CT angiography. J Cardiovasc Comput Tomogr. (2013) 7:83–90. doi: 10.1016/j.jcct.2013.01.008
93. Danad I, Fayad ZA, Willemink MJ, Min JK. New applications of cardiac computed tomography: dual-energy, spectral, and molecular CT imaging. JACC Cardiovasc Imaging. (2015) 8:710–23. doi: 10.1016/j.jcmg.2015.03.005
94. Si-Mohamed SA, Congi A, Ziegler A, Tomasevic D, Tatard-Leitman V, Broussaud T, et al. Early prediction of cardiac complications in acute myocarditis by means of extracellular volume quantification with the use of dual-energy computed tomography. JACC Cardiovasc Imaging. (2021) 14:2041–2. doi: 10.1016/j.jcmg.2021.04.008
95. Sandfort V, Persson M, Pourmorteza A, Noel PB, Fleischmann D, Willemink MJ. Spectral photon-counting CT in cardiovascular imaging. J Cardiovasc Comput Tomogr. (2021) 15:218–25. doi: 10.1016/j.jcct.2020.12.005
Keywords: cardiac computed tomography, coronary artery disease, coronary artery calcium score, perivascular fat, computational fluid dynamics, adverse coronary events
Citation: Emfietzoglou M, Mavrogiannis MC, Samaras A, Rampidis GP, Giannakoulas G and Kampaktsis PN (2022) The role of cardiac computed tomography in predicting adverse coronary events. Front. Cardiovasc. Med. 9:920119. doi: 10.3389/fcvm.2022.920119
Received: 14 April 2022; Accepted: 28 June 2022;
Published: 15 July 2022.
Edited by:
Sara Seitun, San Martino Polyclinic Hospital IRCCS, ItalyReviewed by:
Chiara De Biase, Centro Sanitario Locale Napoli 1 Centro, ItalyAlberto Clemente, Gabriele Monasterio Tuscany Foundation (CNR), Italy
Cesare Mantini, University of Studies G. d'Annunzio Chieti and Pescara, Italy
Copyright © 2022 Emfietzoglou, Mavrogiannis, Samaras, Rampidis, Giannakoulas and Kampaktsis. This is an open-access article distributed under the terms of the Creative Commons Attribution License (CC BY). The use, distribution or reproduction in other forums is permitted, provided the original author(s) and the copyright owner(s) are credited and that the original publication in this journal is cited, in accordance with accepted academic practice. No use, distribution or reproduction is permitted which does not comply with these terms.
*Correspondence: Polydoros N. Kampaktsis, pkampaktsis@yahoo.com