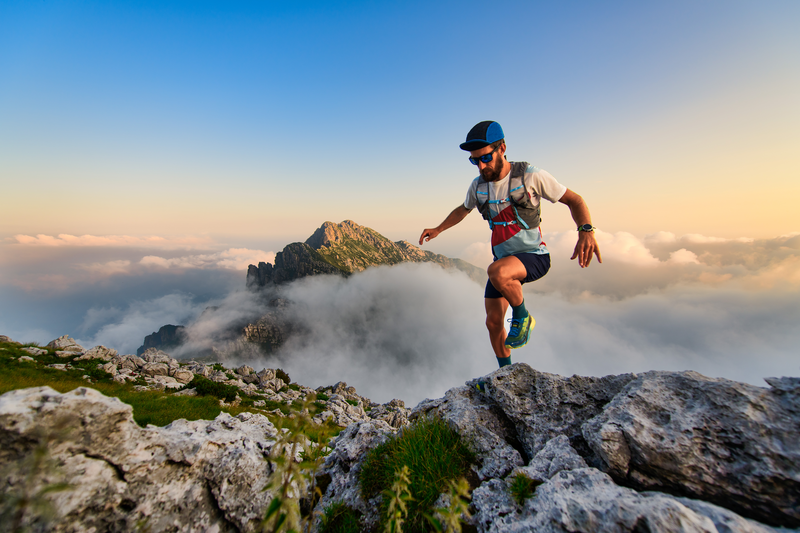
94% of researchers rate our articles as excellent or good
Learn more about the work of our research integrity team to safeguard the quality of each article we publish.
Find out more
REVIEW article
Front. Cardiovasc. Med. , 22 July 2022
Sec. General Cardiovascular Medicine
Volume 9 - 2022 | https://doi.org/10.3389/fcvm.2022.916770
This article is part of the Research Topic Health Technology Assessment in Cardiovascular Diseases View all 17 articles
Neurally mediated syncope (NMS) is a common type of syncope in children in clinical practice, among which vasovagal syncope (VVS) is the most frequent. In recent years, more and more studies have been carried out to assess the diagnosis and prognosis of VVS. The electrocardiographic indicators such as heart rate variability (HRV), QT dispersion (QTd), P-wave dispersion (Pd), ventricular late potentials (VLP), deceleration ability of heart rate (DC), etc., are easy to obtain and inexpensive. With the help of electrocardiographic indicators, the diagnostic procedure and individualized treatment strategies of pediatric VVS can be optimized. This article reviews the value of electrocardiographic indicators in the diagnosis and prognosis of children with VVS.
Syncope is a transient loss of consciousness (TLOC) and the inability to maintain the posture due to transient global cerebral hypoperfusion, and its clinical characteristics are sudden occurrence, transient, and completely spontaneous recovery (1). Hu et al. (2) reported that the incidence of syncope in children and adolescents aged 2–18 years in Changsha was 17.37%, with a peak age of 16 years. The incidence of syncope in girls was higher than that of boys [31.69% (315/994 cases) vs. 26.28% (288/1096 cases), χ2 = 7.44, P < 0.05], and was higher in adolescence than the school-age children [28.85% (603/2090 cases) vs. 8.32% (136/1635 cases), χ2 = 243.21, P < 0.01] and the preschool-age children [28.85% (603/2090) vs. 2.71% (17/627), χ2 = 187.13, P < 0.01]. Neurally mediated syncope (NMS) is the most common cause of syncope, accounting for about 67% of syncope in children, and vasovagal syncope (VVS) is the most common hemodynamic type (3). According to the hemodynamic changes of the head-up tilt test (HUTT), VVS can be divided into three subtypes: vasoinhibitory vasovagal syncope (VVS-VI), cardioinhibitory vasovagal syncope (VVS-CI), and mixed vasovagal syncope (VVS-M) (4). Some children with recurrent syncope are accompanied by cardiac arrest lasting more than 3 s or severe hypotension and bradycardia, which are called malignant VVS (5, 6). The pathogenesis of VVS is still unclear. Hypovolemia, autonomic dysfunction, vasomotor dysfunction, baroreceptor reflex abnormalities, endothelial dysfunction, serotonin surges, and gut microbiota are involved in the underlying mechanism of VVS (7). At present, autonomic dysfunction is a focus of attention. It is believed that the transient abnormality of nerve reflex regulation causes decreased sympathetic tone and/or increased vagal tone, resulting in vasodilation, decreased blood pressure, and slowed heart rate, which contributes to an insufficient blood supply to the brain, causing syncope or pre-syncope to occur (8). Cardiac arrest in malignant VVS is caused by transient sinus node depression, which is caused by transiently intense vagal activity (6). For the judgment of autonomic nerve function, there are many studies on cardiac electrophysiological parameters, such as heart rate variability (HRV), QT dispersion (QTd), P-wave dispersion (Pd), ventricular late potentials (VLP), deceleration ability of heart rate (DC), etc. (7). The treatments for children with VVS mainly include non-pharmacological therapy, pharmacological therapy, and pacemaker therapy. Among these non-pharmacological therapy is the most important method, including health education, autonomic nerve function exercise, and increasing the intake of water and salt. The pharmacological therapy includes midodrine hydrochloride and metoprolol. The pacemaker therapy may be effective for children with VVS with cardiac arrest (4, 6, 9). Although VVS is a self-limiting disease and has a good prognosis, recurrent syncope attacks can seriously affect children’s physical and mental health and quality of life (10). The current accepted criteria to diagnose VVS in children comprise a combination of clinical data and clinical symptoms observed during HUTT. However, the HUTT may cause episodes of syncope or asystole, usually leading to discomfort among children and adding to their psychological loads, and its widespread clinical application is thus restricted (11, 12). Therefore, novel, acceptable, safe, and simple approaches are required to optimize diagnostic procedures and individualized treatment strategies for VVS in children. Based on the electrophysiological parameters, this article reviews the prediction of different electrocardiographic indicators on the diagnosis, treatment effects, and the recurrence of VVS.
The variation over time of the period between consecutive heartbeats, HRV, reflects the regulation of the cardiovascular system by neurohumoral factors. Increased sympathetic nerve activity or decreased vagal nerve activity leads to an increase in the heart rate and a decrease in the HRV. On the contrary, decreased sympathetic nerve activity or increased vagal nerve activity leads to a decrease in the heart rate and an increase in the HRV (13). Therefore, HRV is often used clinically to evaluate the changes in the autonomic nervous system function. HRV includes time-domain indicators and frequency-domain indicators. In the time-domain indicators, the standard deviation of all normal-to-normal intervals (SDNN) reflects the overall tension of the autonomic nerve, the standard deviation of the average normal-to-normal intervals (SDANN), and the standard deviation of all normal-to-normal intervals index (SDNN index) reflect the sympathetic nerve activity, which is related to the slow change components of heart rate and appears to decrease with increased sympathetic nerve activity. The root mean square of differences between successive RR intervals (RMSSD) and the number of successive difference of intervals, that differs by more than 50 ms and is expressed as a percentage of the total number of electrocardiogram (ECG) cycles analyzed (pNN50), reflect the vagal nerve activity, which is related to the rapid change components of heart rate and appears to decrease with decreased vagal nerve activity. In the frequency domain indicators, total power (TP) reflects the overall tension of the autonomic nerves, ultra-low frequency (ULF), very low-frequency (VLF), low-frequency (LF), and normalized LF (nLF) reflect sympathetic nerve activity, and high-frequency (HF) and normalized HF (nHF) reflect vagal nerve activity, and the LF/HF ratio reflects the balance between autonomic nerves (14, 15).
The HUTT is often used to diagnose unexplained syncope and clarify the hemodynamic classification, mainly to simulate orthostatic stress state in the laboratory (16). Alehan et al. (17) reported that 49 children diagnosed with VVS had differences in HRV indicators during HUTT. The nLF and LF/HF in the positive HUTT group were higher than those in the negative HUTT group in the upright tilt state [nLF: (67.2 ± 17.5) nU vs. (56.4 ± 12.3) nU, P = 0.01; LF/HF: (3.7 ± 2.9) vs. (1.7 ± 1.0), P < 0.01], whereas nHF was lower [(26.6 ± 13.4) nU vs. (39.0 ± 12.3) nU, P < 0.01]. The nLF and LF/HF of children with positive HUTT responses were significantly increased in the first 5 min after tilting compared with those in the supine position [nLF: (67.2 ± 17.5) nU vs. (43.9 ± 14.3) nU, P < 0.01; LF/HF: (3.7 ± 2.9) vs. (1.1 ± 0.8), P < 0.01], while nHF significantly decreased [(26.6 ± 13.4) nU vs. (48.5 ± 14.6) nU, P < 0.01]. Children with negative responses to HUTT also had the same changes in the first 5 min of tilting. The nLF and LF/HF were significantly increased compared to the supine position [nLF: (56.4 ± 12.3) nU vs. (39.9 ± 14.6) nU, P < 0.01; LF/HF:(1.7 ± 1.0) vs. (1.0 ± 0.7), P < 0.01] and nHF decreased significantly [(39.0 ± 12.3) nU vs. (50.4 ± 15.5) nU, P < 0.01]. These differences suggest that children with VVS mainly show increased sympathetic nerve activity in the supine position, and the increase in sympathetic nerve activity is more pronounced in children with positive HUTT responses than children with negative responses within the first 5 min after changing body positions, such as leaning upright. It was recommended that LF/HF > 2.7 within the first 5 min after changing body positions can be used as an indicator to distinguish positive HUTT from negative HUTT in children with VVS. The sensitivity, specificity, positive predictive value, and negative predictive value were 52%, 93%, 85%, and 41%, respectively. Evrengul et al. (18) also reported the same results that LF and LF/HF within 5 min before tilting in children with unexplained syncope were significantly higher than those of healthy children [LF:(61.1 ± 6.4) ms2 vs. (52.8 ± 6.0) ms2, P < 0.01; LF/HF:(1.88 ± 0.2) vs. (1.42 ± 0.1), P < 0.05], while HF was significantly lower [(36.1 ± 7.2) ms2 vs. (44.8 ± 6.4) ms2, P < 0.05]. On the other hand, LF and LF/HF were decreased significantly during syncope attacks [LF: (37.6 ± 4.8) ms2 vs. (58.7 ± 8.9) ms2, P < 0.01; LF/HF: (0.65 ± 0.2) vs. (1.63 ± 0.4), P < 0.05], while HF was significantly increased [(58.9 ± 6.2) ms2 vs. (50.5 ± 2.0) ms2, P < 0.05], and all of these indicated that the basic sympathetic nerve activity in children with unknown syncope was decreased before the syncope attack, but the vagal nerve activity was increased during the syncope attack. However, further statistical differences in these indicators were not observed in different hemodynamic types of VVS. Ciliberti et al. (19) observed that there was a significant statistical difference in VLF between the syncope and non-syncope groups in adults in the supine position (2421.09 ms2 vs. 895.49 ms2, P < 0.01). According to the receiver operating characteristic (ROC) curve (AUC = 0.889), VLF > 2,048 ms2 was recommended as the best cutoff value for predicting syncope during HUTT, and its sensitivity, specificity, positive predictive value, and negative predictive value were 87.5%, 72.2%, 75%, and 89%, respectively. However, it remains controversial whether VLF is also statistically different in children. Chen et al. (20) found that VLF had a difference between NMS children and healthy children [(34668.56 ± 17039.12) Hz vs. (25391.12 ± 11040.34) Hz, t = 2.025, P < 0.05]. However, Alehan et al. (17) and Shim et al. (21) did not find a statistically significant difference in VLF between VVS children and healthy children.
Different hemodynamic types of VVS were also associated with HRV. Zygmunt et al. (22) observed that the SDNN of VVS-CI was significantly lower than that of VVS-M children (114 ms vs. 164 ms, P < 0.05), suggesting that different hemodynamics may have different autonomic nerve function sensitivities. Guzmán et al. (23) found that the HF of VVS-CI and VVS-M in the supine position were significantly higher than those of VVS-VI children [(10.52 ± 0.48) ln(ms2) vs. (7.56 ± 1.3) ln(ms2), (11.09 ± 1.9) ln(ms2) vs. (7.56 ± 1.3) ln(ms2), P < 0.01, respectively]. Also, during the entire tilting process, the HF in the VVS-CI and VVS-M groups showed a downward trend until the syncope occurred 1 min later, suggesting that the pathogenesis of VVS-CI and VVS-M may be due to the increase in the vagal nerve activity. While the RMSSD and pNN50 of the VVS-VI children were significantly decreased [RMSSD: (18 ± 7) ms vs. (52 ± 17) ms, (18 ± 7) ms vs. (81 ± 9) ms, P < 0.01, respectively; pNN50: (2 ± 2)% vs. (20 ± 10)%, (2 ± 2)% vs. (25 ± 14)%, P < 0.01, respectively], suggesting that the pathogenesis of VVS-VI is related to the predominance of sympathetic nerve activity. Wang et al. (24) observed 85 syncope children aged 7–16 years and found that the daytime ULF (dULF), nocturnal ULF (nULF), daytime VLF (dVLF), and nocturnal VLF (nVLF) of children with VVS were significantly higher than those children with postural tachycardia syndrome (POTS). The dULF cut-off value is 36.2 ms2 (AUC = 0.826), with a sensitivity of 73.3% and a specificity of 72.5% in distinguishing VVS from POTS.
The above HRV indicators in children with VVS have certain statistical differences in different hemodynamic types during HUTT. VLF > 993.52 ms2 (AUC = 0.645) and dULF>36.2 ms2 can be recommended for the initial diagnosis of patients with VVS. For the diagnosis of different hemodynamic types, further research is needed to explore the relationship between the HRV and children’s diagnosis and the prediction of efficacy.
The QT interval on the ECG represents the time from the beginning of ventricular depolarization (beginning of the QRS complex) to the end of ventricular repolarization (end of the T wave). It is affected by the heart rate and the autonomic nerve, and is shortened by increased sympathetic nerve activity and prolonged by increased vagal never activity. Prolongation of the QT interval has been associated with arrhythmias and sudden cardiac death in several conditions, but particularly the inherited long QT syndrome (LQTS) (25–27). QTd refers to the difference between the maximum QT interval (QTmax) and the minimum QT interval (QTmin) in the ECG, which mainly reflects the inhomogeneity of ventricular muscle repolarization. It increased with the imbalance between the sympathetic nerve and the vagal nerve. QTd can be used as an indicator to predict malignant ventricular arrhythmias, sudden cardiac death, or syncope, and is often associated with arrhythmic events (such as LQTS, heart failure, coronary artery disease, post-myocardial infarction, or hypertrophic cardiomyopathy) (26, 27). Since the QT interval is affected by heart rate, corrected QT interval (QTc interval), and corrected QTd (QTcd) are often used (28). For children with VVS who have QT interval changes, it is necessary to be alert to the occurrence of arrhythmia events and the risk of sudden death. Kula et al. (29) observed syncopal children aged 8–18 and found that there was a difference in QTcd circadian rhythms between children with positive responses to HUTT, negative responses to HUTT, and healthy children. Compared with the negative HUTT group and healthy children, the QTcd in the positive HUTT group was significantly higher in the morning and night. [07:00–09:00: (76.92 ± 4.31) ms vs. (63.51 ± 4.76) ms, (76.92 ± 4.31) ms vs. (53.21 ± 4.29) ms, P < 0.05, respectively; 23:00–01:00: (81.84 ± 1.51) ms vs. (60.03 ± 4.91) ms, (81.84 ± 1.51) ms vs. (57.79 ± 7.0) ms, P < 0.05, respectively]. These results are consistent with the epidemiology that VVS mostly occurs in the morning. KarataS̨ et al. (30) reported 152 children aged 4–18 with a history of syncope and 67 healthy children and found that the QTd and QTcd of the positive HUTT group were significantly higher than those of the negative HUTT group [QTd: (34.9 ± 1.4) ms vs. (27.9 ± 1.3) ms, P < 0.01; QTcd: (58.5 ± 2.1) ms vs. (42.5 ± 1.7) ms, P < 0.01], and QTd and QTcd in positive HUTT group were significantly higher than those of healthy children [QTd: (34.9 ± 1.4) ms vs. (25.8 ± 1.0) ms, P < 0.01; QTcd: (58.5 ± 2.1) ms vs. (37.2 ± 1.4) ms, P < 0.01]. Also, it was recommended to use QTcd > 50 ms as an indicator for predicting positive responses to HUTT, with a specificity and sensitivity of 76.5% and 59.5%, respectively. In this experiment, the positive HUTT group included two subtypes, VVS and POTS, and no statistical difference was found in QTcd between the two subtypes. Khalilian et al. (31) also found that the QTd in the positive HUTT group was significantly higher than that of the negative HUTT group [(42.37 ± 9.52) ms vs. (23.85 ± 4.79) ms, P < 0.01], and compared with VVS-VI, the QTd of VVS-M group was significantly increased [(46.74 ± 9.64) ms vs. (38.00 ± 7.29) ms, P < 0.05]. According to ROC, QTd > 32 ms (ACU = 0.944) and QTd > 40 ms (AUC = 0.784) were recommended as indicators for predicting positive HUTT results and VVS-M type, respectively. The sensitivity and specificity were 92% and 98% and 84%, and 63%, respectively. It suggested that VVS-CI may be more sensitive to QTd changes than VVS-VI, but the disadvantage is that the experiment did not set up a control comparison of QTd between the VVS-CI group and other subtypes. Liu et al. (32) found that the related indicators of QT interval in the VVS-CI group were longer than those in healthy children [QTmax: (414 ± 18) ms vs. (386 ± 15) ms, t = –10.44, P < 0.01; QTmin: (379 ± 17) ms vs. (364 ± 16) ms, t = -5.892, P < 0.01; QTd: (34 ± 6) ms vs. (22 ± 6) ms, t = –12.504, P < 0.01; QTcmax: (464 ± 19) ms vs. (443 ± 19) ms, t = –7.086, P < 0.01; QTcd: (38 ± 6) ms vs. (26 ± 7) ms, t = –11.499, P < 0.01], and the follow-up after non-pharmacological therapy showed that indicators of QT interval in VVS-CI children were longer in the non-response group than in the response group [QTmax: (418 ± 13) ms vs. (402 ± 16) ms, t = 2.713, P < 0.05; QTd: (37 ± 4) ms vs. (29 ± 5) ms, t = 4.222, P < 0.01; QTcmax: (477 ± 14) ms vs. (455 ± 14) ms, t = 3.767, P < 0.01; QTcmin: (435 ± 13) ms vs. (422 ± 14) ms, t = 2.455, P < 0.05; QTcd: (42 ± 4) ms vs. (33 ± 7) ms, t = 3.745, P < 0.01]. According to ROC, QTd > 28.50 ms (AUC = 0.914) and QTd < 34.50 ms (AUC = 0.906) were recommended as indicators for the differential diagnosing of VVS-CI and estimating its prognosis, respectively. The sensitivity and specificity were 86.30% and 84.95% and 90.00% and 82.35%. Wang et al. (33) observed and followed up on 40 cases of children with POTS and found that the symptoms of those with QTcd ≥ 43.0 ms were improved after physical treatment [(69.2 ± 31.2) ms vs. (43.5 ± 25.9) ms, t/Z = 2.58, P < 0.05], indicating that QTcd ≥ 43.0 ms (AUC = 0.73) can be used as a predictor of physical treatment with a sensitivity of 90% and a specificity of 60%. Subsequently, Wang et al. (34) observed and followed up on 50 children with POTS and found that the symptoms of those with QTcd ≥ 47.9 ms were improved after metoprolol treatment [(66.3 ± 20.3) ms vs. (45.7 ± 19.9) ms, Z = -3.339, P < 0.01], indicating that QTcd ≥ 47.9 ms (AUC = 0.822) can be used as a predictor of the efficacy of metoprolol in the treatment of POTS, with a sensitivity of 78.9% and a specificity of 83.3%. Similar to POTS, some children with VVS can also choose metoprolol therapy or physical treatment, both of which have autonomic dysfunction or high catecholamine status. Therefore, when the QTcd ≥ 43.0 ms, physical treatment can be selected. When QTcd ≥ 47.9 ms, metoprolol treatment can be recommended. In conclusion, children with VVS have abnormal QT intervals, and there are statistical differences in QT intervals between different hemodynamic types. According to ROC, it was recommended that QTd > 40 ms (AUC = 0.784) and QTd > 28.50 ms (AUC = 0.914) can be used as indicators to predict VVS-M and VVS-CI, and QTd < 34.50 ms (AUC = 0.906) can predict that treatment of VVS-CI has a good prognosis.
The P wave is the potential change that occurs when the left and right atria are depolarized. Autonomic dysfunction can cause changes in the amplitude, duration, and morphology of the P-wave. When the sympathetic nerve activity increases, the P-wave amplitude increases, forming a “pulmonary-type P-wave,” and the maximum P-wave duration (Pmax) and Pd are significantly increased (35). Prolonged P-wave duration (Pwd) (Pwd > 120 ms), a marker of left atrial abnormality, has been linked with electromechanical dysfunction and poor left atrial contractility, which has been associated with myocardial fibrosis, heart failure, atrial fibrillation, and sudden cardiac death (36, 37). Pd refers to the difference between Pmax and the minimum P-wave duration (Pmin) on the surface ECG. It is a sign of inhomogeneous electrical activity in the atria and an important predictor of cardiac arrhythmias, especially atrial fibrillation (35), and is associated with sudden cardiac death and severe ventricular arrhythmias (36, 38, 39). Kose et al. (40) reported that 100 cases of VVS children had significantly prolonged Pd compared with healthy children [HUTT positive: (50.2 ± 18.5) ms vs. (32.0 ± 11.2) ms, P < 0.05; HUTT negative: (39.6 ± 14.2) ms vs. (32.0 ± 11.2) ms, P < 0.05], the Pd in the positive HUTT group was also significantly longer than that of the negative HUTT group [(50.2 ± 18.5) ms vs. (39.6 ± 14.2) ms, P < 0.05]. In the positive HUTT group, there were also significant differences in Pd among different VVS subtypes, and the Pd in the VVS-CI group was significantly longer than that in VVS-VI and VVS-M [(51.1 ± 23.6) ms vs. (46.9 ± 21.7) ms, (51.1 ± 23.6) ms vs. (44.4 ± 15.8) ms, both P < 0.05, respectively]. It verified the existence of autonomic dysfunction in children with VVS, suggesting that Pd can be used as an ECG indicator for early clinical prediction of VVS, and may be more sensitive in VVS-CI type. Wang et al. (41) reported that the P-wave related parameters of 43 cases of children with VVS-CI aged 5∼17, such as Pd, Pmax, Pwd, corrected the maximum P-wave duration (Pcmax), corrected P-wave dispersion (Pcd), were significantly longer than those of healthy children [Pd: (36 ± 7) ms vs. (26 ± 4) ms, t/Z = 8.270, P < 0.01; Pmax: (97 ± 7) ms vs. (88 ± 4) ms, t/Z = 7.128, P < 0.01; Pwd: (79 ± 7) ms vs. (75 ± 4) ms, t/Z = 3.639, P < 0.01; Pcmax: (120 ± 12) ms vs. (112 ± 7) ms, t/Z = 3.390, P < 0.01; Pcd: (44 ± 8) ms vs. (33 ± 5) ms, t/Z = 7.043, P < 0.01]. It is further verified that children with VVS-CI have autonomic dysfunction and abnormal atrial electrical activity. Pd ≥ 27.42 ms (AUC = 0.908) was recommended as an ECG indicator for early clinical prediction of VVS-CI, with the sensitivity and specificity of 95.35% and 69.77%, respectively. In addition, de Gregorio et al. (42) observed 55 syncopal patients aged 14–75 years old and found that 75% of patients with P-wave peaking (PWP, percent increase in PWP from rest to both 15-min and peak-HR) ≤50% at peak heart rate had positive responses to HUTT, while only 5% of patients with PWP ≥ 100% had positive responses to HUTT, suggesting a potential relationship between HUTT positivity and low or no PWP. However, the relevant data on children have not been retrieved yet, so the PWP indicator deserves further study. Therefore, for P-wave related indicators, Pd = 27.42 ms (AUC = 0.908) is currently recommended as an ECG indicator for early clinical prediction of VVS-CI.
T wave refers to the ventricular repolarization process on the synchronous 12-lead ECG. Since most of the repolarization potentials cancel each other during the ventricular repolarization process, the T wave actually reflects the potential difference of ventricular repolarization that has not been canceled out. Autonomic dysfunction can cause T wave changes, with an incidence of 20–40%. For example, when sympathetic nerve activity increases, T waves can appear bimodal, flat, or inverted, and the Niagara waterfall-like T wave (giant T wave inversion) may even occur when the activity of the sympathetic nerve increases excessively. When vagal nerve activity increases, the T wave height tip may appear, and T wave changes can be used as a predictor of sudden cardiac death and malignant arrhythmia (43, 44).
VVS is related to T wave amplitude and morphology. Mayuga et al. (45) found that T wave changes in leads aVF, V5, and V6 were correlated with VVS (P < 0.05). Kolarczyk et al. (46) observed T wave changes in 30 children with VVS, and 19 patients had T wave morphological changes after HUTT, mainly in leads V4, V5, and V6, and during the HUTT in these 19 children, the QTc and T wave peak-to-end interval (Tp-Te interval) in lead V5 were significantly longer than the syncope children without T wave morphological changes [QTc: (451.3 ± 13.4) ms vs. (434.4 ± 15.4) ms, P < 0.01; Tp-Te: (100.0 ± 3.3) ms vs. (88.2 ± 4.0) ms, P < 0.01]. Markiewicz-Łoskot et al. (47) also found that 23 of 40 children with negative HUTT VVS had T wave morphological changes in lead V5 during HUTT, and the QTc and Tp-Te intervals were significantly longer than those of VVS children without T wave changes, and there is a risk of arrhythmia. Xue et al. (48) reported that children with unexplained syncope had lower T wave amplitudes in lead V3–V6 during HUTT than healthy children, and the positive HUTT group had lower T wave amplitudes in some leads (II, III, aVR, aVL, and aVF) during HUTT than negative HUTT group (all P < 0.05). Wu et al. (49) reported that the morphological changes of T wave were correlated with VVS, and the incidence of syncope can be reduced after oral β-blockers in VVS children with orthostatic T wave changes (28.6% vs. 72.7%, P < 0.01), suggesting that treatment of β-blocker can be recommended to VVS children with orthostatic T wave changes. Wu et al. (50) reported that the T wave amplitude in leads II, aVR, and aVF during syncope had a certain predictive value for short-term non-pharmacological therapy in children with VVS.
The Tp-Te interval refers to the total time from the peak of the T wave to the end of the T wave on the synchronized 12-lead ECG, which represents the difference between the epicardial repolarization time and the M-cell repolarization time during the cardiac repolarization process, and is an indicator of transmural dispersion of repolarization (TDR). The prolongation of Tp-Te interval has predictive values for clinical malignant ventricular arrhythmias (51–53). Tp-Te/QT reflects the proportion of Tp-Te interval in the process of repolarization. As a more sensitive indicator for judging arrhythmia, it can eliminate the influence of heart rate and individual QT interval variability on Tp-Te (54). Markiewicz-Łoskot et al. (47) found that the Tp-Te interval in lead V5 was consistently prolonged during HUTT in 40 children with VVS compared to healthy children [in supine position: 89 ms vs. 80 ms, P < 0.01; syncope occurred: 100 ms vs. 60 ms, P < 0.01; after syncope: 90 ms vs. 80 ms, P < 0.01]. According to ROC, Tp-Te interval > 70 ms (AUC = 1) was recommended for predicted VVS with good sensitivity and specificity (both 100%).
The above studies show that β-blockers or non-pharmacological therapy is recommended for VVS children with T-wave morphology and T-wave amplitude changes, and the diagnosis of VVS can be preliminarily predicted when the Tp-Te interval >70 ms.
DC is an indicator to evaluate the function of the autonomic nervous system. Through the analysis of the RR interval in the Holter, the cardiac vagal nerve activity is quantitatively measured, that is, the decrease of DC reflects the decrease of the cardiac vagal nerve activity, and confirms that the DC damage is a strong predictor of mortality after myocardial infarction (55). Tong et al. (56) analyzed 90 cases of children’s Holter ECG and found that children with VVS had abnormal autonomic nerve function during the asymptomatic period, and it was related to age [school-age group vs. adolescence group: (7.94 ± 0.62) ms vs. (8.59 ± 1.15) ms, t = 2.49, P < 0.05]. It was recommended that the DC of school-age (7–10 years old) is 7.72 ms (AUC = 0.717), and the DC of adolescence (11–18 years old) is 8.36 ms (AUC = 0.692) as the cutoff values have a good predictive value for the initial diagnosis of VVS, and its sensitivity and specificity were 68.8%, 68.7% and 65.5%, 62.1%, respectively.
Immediate heart rate change refers to the change of the patient’s heart rate from supine to an upright position, including acceleration index (AI) and 30/15 ratio. AI reflects sympathetic nervous system function, and the 30/15 ratio reflects vagal nerve system function (57, 58). Tao et al. (59, 60) observed differences in AI and 30/15 ratios between children with VVS and POTS. Compared with the POTS group, the AI of the VVS group was significantly decreased, and the 30/15 ratio increased [(23.440 ± 8.693) vs. (33.495 ± 8.472), t/Z = -4.724, P < 0.01; (1.025 ± 0.084) vs. (0.962 ± 0.067), t/Z = 3.187, P < 0.01], suggesting that the pathogenesis of VVS and POTS may be different. Also, it was found that AI and 30/15 ratio can be used as indicators to distinguish POTS and VVS, with AI = 28.180 (AUC = 0.801) or 30/15 = 1.025 (AUC = 0.738) as the cutoff value, the sensitivity and specificity of differentiating POTS from VVS were 79.2%, 73.1% or 87.5%, 61.5%, respectively. After the upright training intervention, the average acceleration index of VVS children with the improved condition was lower than those without improvement [(21.10 ± 6.61) vs. (31.36 ± 9.00), P < 0.01], and the predictive ability was the best when AI < 26.7 (AUC = 0.827) with sensitivity and specificity of 85.0% and 69.2%. Similarly, Zhang et al. (61) found that the heart rate before a positive response to HUTT was significantly higher in the effective treatment group with metoprolol than that of the ineffective treatment group [(123 ± 15) bpm vs. (96 ± 17) bpm, P < 0.01], and the heart rate increment before the positive response to HUTT showed a significant difference between the two groups [(42 ± 16) bpm vs. (18 ± 13) bpm, P < 0.01]. Compared with the baseline value, if an increase of 30 bpm in heart rate before a positive response to HUTT was taken as a cut-off value, with respect to predicting the metoprolol efficacy in the treatment of VVS, the sensitivity was 81% and the specificity was 80%, suggesting that β-blocker therapy is recommended for those with markedly increased heart rate before a positive response to HUTT.
VLP is a high-frequency, low-amplitude fragmented electrical activity characterized by multi-shaped sharp waves caused by local delayed depolarization of the myocardium. It is caused by slow and irregular reentry activity in the ischemic myocardium, and it is an electrophysiological indicator reflecting the instability of myocardial electrical activity (62). The main detection indicators are: (1) total QRS time (TQRS, ms), which refers to the time from the start of QRS to the end of VLP on the filtered leads X, Y, and Z; (2) root mean square value (RMS40, μV), which is the voltage at the end of the QRS wave at 40 ms; and (3) the high frequency and low amplitude time limit (LAS40, ms), the time limit when the terminal voltage of the QRS after filtering is lower than 40 μV (63). Previous studies have shown that there are circadian rhythm changes in VLP associated with autonomic nerves (64, 65), and VLP is associated with the induction of malignant arrhythmias, the prognosis of patients with myocardial infarction, and sudden cardiac death (66–68). Zhang et al. (63) found that the LAS40 of VVS children was significantly prolonged compared with healthy children [(29.04 ± 6.59) μV vs. (26.15 ± 5.82) μV, t = 2.204, P < 0.05], suggesting that VVS children have abnormal myocardial electrical activity and the risk of cardiac events, and such children should be alert to the possibility of malignant arrhythmia. Zou et al. (69) reported that the TQRS, RMS40, and LAS40 of 184 cases of VVS-VI children were longer than those of healthy children [TQRS: (84.89 ± 12.05) ms vs. (81.21 ± 8.23) ms, P < 0.01; RMS40: (28.73 ± 7.23) μV vs. (26.89 ± 7.36) μV, P < 0.05; LAS40: (62.43 ± 19.17) ms vs. (56.79 ± 18.75) ms, P < 0.05], and the VVS-VI group had more abnormally prolonged LAS40 (94.57% vs. 83.80%, P < 0.01), suggesting that monitoring VLP in children with VVS-VI can help predict the possibility of malignant arrhythmia.
For the clinical prediction of VVS diagnosis (Table 1), LF/HF > 2.7, dULF>36.2 ms2, QTcd > 50 ms, QTd > 32 ms, Tp-Te > 70 ms, school-age DC > 7.72 ms, adolescent DC > 8.36 ms, AI < 28.18, and 30/15 > 1.025 were used as indicators to distinguish positive and negative HUTT in children, according to the maximum AUC (1.000), Tp-Te > 70 ms is recommended as a rapid identification of VVS in ECG indicator. For different hemodynamic types (Table 2), VVS-CI can be predicted with QTd > 28.5 ms and Pd ≥ 27.42 ms. According to the maximum AUC (0.914), QTd > 28.5 ms is recommended as the best ECG indicator for predicting VVS- CI, when QTd > 40 ms can be used to predict VVS-M. For the selection of VVS treatment measures and the prediction of efficacy (Table 3), QTd < 34.5 ms, AI < 26.7, heart rate before positive HUTT reaction increased by 30 bpm from basal heart rate were used as predictors for the effectiveness of non-pharmacological therapy, upright training, and metoprolol intervention.
VVS is a self-limiting disease caused by the disturbance of autonomic regulation, which usually occurs in early adolescence. ECG indicators such as HRV, Pd, QTd, Tp-Te interval, Tp-Te/QT, T-wave amplitude and shape, immediate heart rate change, DC and VLP are easily available, non-invasive, and inexpensive. However, there is still a lack of systematic large-scale, multi-center, long-term follow-up studies, and the value of some indicators needs to be further confirmed. With the help of relevant ECG indicators, individual treatment plans can be selected for patients with different hemodynamic types to improve the long-term prognosis of children and adolescents with VVS.
TZ conceptualized, prepared, and wrote the manuscript and made the tables. SW, MW, HC, YW, YX, and RZ participated in providing documentation. RZ and CW reviewed, edited, and revised the manuscript. All authors have read and approved the final manuscript and assume full responsibility for its contents.
This work was supported by grants from the Hunan Province Clinical Medical Technology Innovation Guidance Project (2020SK53405) and the Health and Family Planning Commission of Hunan Province in China (20201217).
The authors declare that the research was conducted in the absence of any commercial or financial relationships that could be construed as a potential conflict of interest.
All claims expressed in this article are solely those of the authors and do not necessarily represent those of their affiliated organizations, or those of the publisher, the editors and the reviewers. Any product that may be evaluated in this article, or claim that may be made by its manufacturer, is not guaranteed or endorsed by the publisher.
1. Moya A, Sutton R, Ammirati F, Blanc JJ, Brignole M, Dahm JB, et al. Guidelines for the diagnosis and management of syncope (version 2009). Eur Heart J. (2009) 30:2631–71. doi: 10.1093/eurheartj/ehp298
2. Hu E, Liu X, Chen Q, Wang C. Investigation on the incidence of syncope in children and adolescents aged 2-18 years in Changsha. Front Pediatr. (2021) 9:638394. doi: 10.3389/fped.2021.638394
3. Chen L, Wang C, Wang HW, Tian H, Tang CS, Jin HF, et al. Underlying diseases in syncope of children in China. Med Sci Monit. (2011) 17:H49–53. doi: 10.12659/msm.881795
4. Wang C, Li Y, Liao Y, Tian H, Huang M, Dong X, et al. 2018 Chinese pediatric cardiology society (CPCS) guideline for diagnosis and treatment of syncope in children and adolescents. Sci Bull. (2018) 63:1558–64. doi: 10.1016/j.scib.2018.09.019
5. Pentousis D, Cooper JP, Cobbe SM. Prolonged asystole induced by head up tilt test. Report of four cases and brief review of the prognostic significance and medical management. Heart. (1997) 77:273–5. doi: 10.1136/hrt.77.3.273
6. Xu WR, Jin HF, Du JB. Diagnosis and treatment of malignant vasovagal syncope inchildren. Chin J Pediatr. (2022) 60:64–6. doi: 10.3760/cma.j.cn112140-20211018-00883
7. Li HX, Gao L, Yuan Y. Advance in the understanding of vasovagal syncope in children and adolescents. World J Pediatr. (2021) 17:58–62. doi: 10.1007/s12519-020-00367-z
8. Xiao Y, Zhang XH, Wei HF, Dong XY. Research progress on the pathogenesis of orthostatic intolerance in children. Lanzhou Da Xue Xue Bao Yi Xue Ban. (2021) 47:82–8. doi: 10.13885/j.issn.1000-2812.2021.06.013
9. Xu WR, Du JB, Jin HF. Can pediatric vasovagal syncope be individually managed? World J Pediatr. (2022) 18:4–6. doi: 10.1007/s12519-021-00495-0
10. Anderson JB, Czosek RJ, Knilans TK, Marino BS. The effect of paediatric syncope on health-related quality of life. Cardiol Young. (2012) 22:583–8. doi: 10.1017/S1047951112000133
11. Chu W, Wang C, Lin P, Li F, Wu L, Xie Z. Transient aphasia: a rare complication of head-up tilt test. Neurol Sci. (2014) 35:1127–32. doi: 10.1007/s10072-014-1664-1
12. Kim PH, Ahn SJ, Kim JS. Frequency of arrhythmic events during head-up tilt testing in patients with suspected neurocardiogenic syncope or presyncope. Am J Cardiol. (2004) 94:1491–5. doi: 10.1016/j.amjcard.2004.08.025
13. Rajendra Acharya U, Paul Joseph K, Kannathal N, Lim CM, Suri JS. Heart rate variability: a review. Med Biol Eng Comput. (2006) 44:1031–51. doi: 10.1007/s11517-006-0119-0
14. Zhang J, Zheng T, Lin Y, Liu Y, Shi L. Changes in heart rate deceleration capacity and variability in resting children with vasovagal syncope. Chin J Appl Clin Pediatr. (2019) 34:986–9. doi: 10.3760/cma.j.issn.2095-428X.2019.13.005
15. Malik M, Bigger JT, Camm AJ, Kleiger RE, Malliani A, Moss AJ, et al. Heart rate variability: standards of measurement, physiological interpretation, and clinical use. Eur Heart J. (1996) 17:354–81. doi: 10.1093/oxfordjournals.eurheartj.a014868
16. Alehan D, Celiker A, Ozme S. Head-up tilt test: a highly sensitive, specific test for children with unexplained syncope. Pediatr Cardiol. (1996) 17:86–90. doi: 10.1007/BF02505089
17. Alehan D, Ayabakan C, Ozer S. Heart rate variability and autonomic nervous system changes in children with vasovagal syncope. Pacing Clin Electrophysiol. (2002) 25:1331–8. doi: 10.1046/j.1460-9592.2002.01331.x
18. Evrengul H, Tavli V, Evrengul H, Tavli T, Dursunoglu D. Spectral and time-domain analyses of heart-rate variability during head-upright tilt-table testing in children with neurally mediated syncope. Pediatr Cardiol. (2006) 27:670–8. doi: 10.1007/s00246-003-0598-9
19. Ciliberti MAP, Santoro F, Di Martino LFM, Rinaldi AC, Salvemini G, Cipriani F, et al. Predictive value of very low frequency at spectral analysis among patients with unexplained syncope assessed by head-up tilt testing. Arch Cardiovasc Dis. (2018) 111:95–100. doi: 10.1016/j.acvd.2017.04.006
20. Chen L, Zhang CY, Du JB. Diagnostic values of heart rate variability on unexplained syncope in children. Beijing Da Xue Xue Bao Yi Xue Ban. (2013) 45:761–5. doi: 10.3969/j.issn.1671-167X.2013.05.021
21. Shim SH, Park SY, Moon SN, Oh JH, Lee JY, Kim HH, et al. Baseline heart rate variability in children and adolescents with vasovagal syncope. Korean J Pediatr. (2014) 57:193–8. doi: 10.3345/kjp.2014.57.4.193
22. Zygmunt A, Stanczyk J. Heart rate variability in children with neurocardiogenic syncope. Clin Auton Res. (2004) 14:99–106. doi: 10.1007/s10286-004-0168-0
23. Guzmán CE, Sánchez GM, Márquez MF, Hermosillo AG, Cárdenas M. Differences in heart rate variability between cardioinhibitory and vasodepressor responses to head-up tilt table testing. Arch Med Res. (1999) 30:203–11. doi: 10.1016/s0188-0128(99)00022-6
24. Wang Y, Zhang C, Chen S, Li X, Jin H, Du J. Frequency domain indices of heart rate variability are useful for differentiating vasovagal syncope and postural tachycardia syndrome in children. J Pediatr. (2019) 207:59–63. doi: 10.1016/j.jpeds.2018.11.054
25. Nielsen JB, Graff C, Rasmussen PV, Pietersen A, Lind B, Olesen MS, et al. Risk prediction of cardiovascular death based on the QTc interval: evaluating age and gender differences in a large primary care population. Eur Heart J. (2014) 35:1335–44. doi: 10.1093/eurheartj/ehu081
26. Liu YS, Li YG. Autonomic nerves and the QT interval. J Clin Electrocardiol. (2006) 15:9–10. doi: 10.3969/j.issn.1005-0272.2006.01.008
27. Statters DJ, Malik M, Ward DE, Camm AJ. QT dispersion: problems of methodology and clinical significance. J Cardiovasc Electrophysiol. (1994) 5:672–85. doi: 10.1111/j.1540-8167.1994.tb01190.x
28. Indik JH, Pearson EC, Fried K, Woosley RL. Bazett and Fridericia QT correction formulas interfere with measurement of drug-induced changes in QT interval. Heart Rhythm. (2006) 3:1003–7. doi: 10.1016/j.hrthm.2006.05.023
29. Kula S, Olgunturk R, Tunaoglu FS, Canter B. Circadian variation of QTc dispersion in children with vasovagal syncope. Int J Cardiol. (2004) 97:407–10. doi: 10.1016/j.ijcard.2003.10.024
30. KarataS̨ Z, Alp H, Sap F, Altın H, Baysal T, Karaarslan S. Usability of QTc dispersion for the prediction of orthostatic intolerance syndromes. Eur J Paediatr Neurol. (2012) 16:469–74. doi: 10.1016/j.ejpn.2011.12.009
31. Khalilian MR, Ghasemi A, Khazaei N, Khoshkhou S, Mahmoudi E. Repolarization disparity as a predictor of response to Head up tlt-table test in pediatric syncope. Pacing Clin Electrophysiol. (2021) 44:1397–403. doi: 10.1111/pace.14305
32. Liu JT, Wang YW, Li F, Lin P, Cai H, Zou RM, et al. Diagnostic efficacy and prognostic evaluation value of QT interval dispersion in children and adolescents with cardioinhibitory vasovagal syncope. Chin Pediatr Emerg Med. (2021) 28:192–7. doi: 10.3760/cma.j.issn.1673-4912.2021.03.007
33. Lu W, Yan H, Wu S, Chen S, Xu W, Jin H, et al. Electrocardiography-derived predictors for therapeutic response to treatment in children with postural tachycardia syndrome. J Pediatr. (2016) 176:128–33. doi: 10.1016/j.jpeds.2016.05.030
34. Wang Y, Sun Y, Zhang Q, Zhang C, Liu P, Wang Y, et al. Baseline corrected QT interval dispersion is useful to predict effectiveness of metoprolol on pediatric postural tachycardia syndrome. Front Cardiovasc Med. (2022) 8:808512. doi: 10.3389/fcvm.2021.808512
35. Qu XF. Influence of autonomic nerves on P wave amplitude and duration. J Clin Electrocardiol. (2006) 15:5–6. doi: 10.3969/j.issn.1005-0272.2006.01.005
36. Van Beeumen K, Duytschaever M, Tavernier R, Van de Veire N, De Sutter J. Intra-and interatrial asynchrony in patients with heart failure. Am J Cardiol. (2007) 99:79–83. doi: 10.1016/j.amjcard.2006.07.066
37. Maheshwari A, Norby FL, Soliman EZ, Alraies MC, Adabag S, O’Neal WT, et al. Relation of prolonged P-wave duration to risk of sudden cardiac death in the general population (from the atherosclerosis risk in communities study). Am J Cardiol. (2017) 119:1302–6. doi: 10.1016/j.amjcard.2017.01.012
38. Badran HM, Faheem N, Wassely KW, Yacoub M. Relationship of left atrial mechanics to electrical activity on surface electrocardiography in idiopathic dilated cardiomyopathy. Glob Cardiol Sci Pract. (2019) 2019:7. doi: 10.21542/gcsp.2019.7
39. Türe M, Balık H, Akın A, Bilici M, Nergiz A. The relationship between electrocardiographic data and mortality in children diagnosed with dilated cardiomyopathy. Eur J Pediatr. (2020) 179:813–9. doi: 10.1007/s00431-020-03569-9
40. Köse MD, Bağ Ö, Güven B, Mes̨e T, Öztürk A, Tavlı V. P-wave dispersion: an indicator of cardiac autonomic dysfunction in children with neurocardiogenic syncope. Pediatr Cardiol. (2014) 35:596–600. doi: 10.1007/s00246-013-0825-y
41. Wang SS, Yi XY, Ji Q, Wang YW, Wang C. Change in P wave on electrocardiogram and its diagnostic value in children and adolescents with cardioinhibitory vasovagal syncope. Chin J Contemp Pediatr. (2019) 21:1084–8. doi: 10.7499/j.issn.1008-8830.2019.11.006
42. de Gregorio C, Lentini C, Grimaldi P, Zagari D, Andò G, Di Bella G, et al. P-wave voltage and peaking on electrocardiogram in patients undergoing head-up tilt testing for history of syncope. Eur J Intern Med. (2014) 25:383–7. doi: 10.1016/j.ejim.2014.03.007
43. Rosenbaum DS, Jackson LE, Smith JM, Garan H, Ruskin JN, Cohen RJ. Electrical alternans and vulnerability to ventricular arrhythmias. N Engl J Med. (1994) 330:235–41. doi: 10.1056/NEJM199401273300402
44. Liu DP. Autonomic nerves and T wave changes. J Clin Electrocardiol. (2006) 15:8–9. doi: 10.3969/j.issn.1005-0272.2006.01.007
45. Mayuga KA, Fouad-Tarazi F. Dynamic changes in T-wave amplitude during tilt table testing: correlation with outcomes. Ann Noninvasive Electrocardiol. (2007) 12:246–50. doi: 10.1111/j.1542-474X.2007.00168.x
46. Kolarczyk E, Markiewicz-Łoskot G, Szydłowski L. The repolarization period during the head-up tilt test in children with vasovagal syncope. Int J Environ Res Public Health. (2020) 17:1908. doi: 10.3390/ijerph17061908
47. Markiewicz-Łoskot G, Kolarczyk E, Mazurek B, Łoskot M, Szydłowski L. Prolongation of electrocardiographic T wave parameters recorded during the head-up tilt table test as independent markers of syncope severity in children. Int J Environ Res Public Health. (2020) 17:6441. doi: 10.3390/ijerph17186441
48. Xue XH, Wang C, Lin P, Cao MJ, Li MX, Ding YY, et al. Dynamic changes of synchronous 12-lead electrocardiogram P wave and ST segment and T wave amplitude in vasovagal syncope children during head-up tilt test. Chin J Crit Car Med. (2010) 30:689–93. doi: 10.3969/j.issn.1002-1949.2010.08.005
49. Wu YF, Xu JY, Sun RP, Wang LY. The relation between body posture T wave inversions and vasovagal syncope. Chin Pract Med. (2007) 2:17–20. doi: 10.3969/j.issn.1673-7555.2007.15.007
50. Wu LJ, Wang C, Hu CY, Kumar P, Xu Y, Lin P, et al. Predictive value of T wave amplitude in short-term curative effect of nonpharmacological therapy of vasovagal syncope (WS) children during head-up tilt test. Chin J Crit Car Med. (2010) 30:126–30. doi: 10.3969/j.issn.1002-1949.2010.02.010
51. Zhang P, Zou CL, Huang WJ. Value of Tp-Te interval and QTd in predicting malignant arrhythmia in coronary heart disease. J Med Res. (2013) 42:94–7. doi: 10.3969/j.issn.1673-548X.2013.03.029
52. Antzelevitch C. Heterogeneity and cardiac arrhythmias: an overview. Heart Rhythm. (2007) 4:964–72. doi: 10.1016/j.hrthm.2007.03.036
53. Zhao DH, Liang B, Peng J, Tang LY, Su RB, Luo LL, et al. Tp-e and (Tp-e)/QT ratio as a non-invasive risk factors for malignant ventricular arrhythmia in patients with idiopathic ventricular premature complexes. J Clin Lab Anal. (2021) 35:e23636. doi: 10.1002/jcla.23636
54. Wang HY. Tp-Te interval. J Clin Electrocardiol. (2013) 22:175–7. doi: 10.3969/j.issn.1005-0272.2013.03.009
55. Bauer A, Kantelhardt JW, Barthel P, Schneider R, Mäkikallio T, Ulm K, et al. Deceleration capacity of heart rate as a predictor of mortality after myocardial infarction: cohort study. Lancet. (2006) 367:1674–81. doi: 10.1016/S0140-6736(06)68735-7
56. Tong K, He S, Ming L, Zhu L, Yu GS. Analysis of autonomic nervous function in asymptomatic vasovagal syncope in children. J Clin Pediatr. (2020) 38:665–70. doi: 10.3969/j.issn.1000-3606.2020.09.007
57. Sundkvist G, Lilja B, Manhem P, Almér LO. Responses of plasma catecholamines to tilt in patients with diabetes mellitus. Acta Med Scand. (1984) 216:223–7. doi: 10.1111/j.0954-6820.1984.tb03796.x
58. Ewing DJ, Campbell IW, Murray A, Neilson JM, Clarke BF. Immediate heart-rate response tostanding: simple test for autonomic neuropathy in diabetes. Br Med J. (1978) 1:145–7. doi: 10.1136/bmj.1.6106.145
59. Tao CY, Chen S, Li HX, Wang YY, Wang YL, Liu P, et al. Value of immediate heart rate alteration from supine to upright in differential diagnosis between vasovagal syncope and postural tachycardia syndrome in children. Front Pediatr. (2018) 6:343. doi: 10.3389/fped.2018.00343
60. Tao CY, Li XY, Tang CS, Jin HF, Du JB. Acceleration index predicts efficacy of orthostatic training on vasovagal syncope in children. J Pediatr. (2019) 207:54–8. doi: 10.1016/j.jpeds.2018.10.063
61. Zhang QY, Du JB, Zhen JL, Li WZ, Wang YL. Hemodynamic changes during head-up tilttest and predictive value thereof in predicting the efficacy of metoprolol therapy in children with vasovagal syncope. Chin Med J. (2007) 87:1260–2. doi: 10.3760/j:issn:0376-2491.2007.18.011
62. el-Sherif N. Electrophysiologic basis of ventricular late potentials. Prog Cardiovasc Dis. (1993) 35:417–27. doi: 10.1016/0033-0620(93)90026-a
63. Zhang YR, Xu Y, Wang C, Zheng HF, Cao MJ, Xue XH, et al. Changes of ventricular late potential in the children with vasovagal syncope. Chin J Crit Car Med. (2007) 27:688–90. doi: 10.3969/j.issn.1002-1949.2007.08.006
64. Qu XF, Li JJ, Huang YL, Gao GY, Ji SY, Du XD. The influcence of autonomic nerve on long-term ventricular late potential. Chin J Cardiac Pacing Electrophysiol. (2002) 16:274–5. doi: 10.3969/j.issn.1007-2659.2002.04.012
65. Nakagawa M, Iwao T, Ishida S, Yonemochi H, Fujino T, Saikawa T, et al. Circadian rhythm of the signal averaged electrocardiogram and its relation to heart rate variability in healthy subjects. Heart. (1998) 79:493–6. doi: 10.1136/hrt.79.5.493
66. Amino M, Yoshioka K, Ichikawa T, Watanabe E, Kiyono K, Nakamura M, et al. The presence of late potentials after percutaneous coronary intervention for the treatment of acute coronary syndrome as a predictor for future significant cardiac events resulting in re- hospitalization. J Electrocardiol. (2019) 53:71–8. doi: 10.1016/j.jelectrocard.2019.01.003
67. Yodogawa K, Seino Y, Ohara T, Iwasaki YK, Hayashi M, Miyauchi Y, et al. Prognostic significance of ventricular late potentials in patients with pulmonary sarcoidosis. Heart Rhythm. (2018) 15:798–802. doi: 10.1016/j.hrthm.2018.03.013
68. Gatzoulis KA, Arsenos P, Trachanas K, Dilaveris P, Antoniou C, Tsiachris D, et al. Signal– averaged electrocardiography: past, present, and future. J Arrhythm. (2018) 34:222–9. doi: 10.1002/joa3.12062
Keywords: vasovagal syncope, diagnosis, prognosis, electrocardiography, children
Citation: Zhao T, Wang S, Wang M, Cai H, Wang Y, Xu Y, Zou R and Wang C (2022) Research progress on the predictive value of electrocardiographic indicators in the diagnosis and prognosis of children with vasovagal syncope. Front. Cardiovasc. Med. 9:916770. doi: 10.3389/fcvm.2022.916770
Received: 10 April 2022; Accepted: 28 June 2022;
Published: 22 July 2022.
Edited by:
Deepak Saxena, Indian Institute of Public Health Gandhinagar (IIPHG), IndiaReviewed by:
Oksana Boyarchuk, Ternopil State Medical University, UkraineCopyright © 2022 Zhao, Wang, Wang, Cai, Wang, Xu, Zou and Wang. This is an open-access article distributed under the terms of the Creative Commons Attribution License (CC BY). The use, distribution or reproduction in other forums is permitted, provided the original author(s) and the copyright owner(s) are credited and that the original publication in this journal is cited, in accordance with accepted academic practice. No use, distribution or reproduction is permitted which does not comply with these terms.
*Correspondence: Cheng Wang, d2FuZ2NoZW5nMm5kQGNzdS5lZHUuY24=
†ORCID: Cheng Wang, orcid.org/0000-0002-7120-0654
Disclaimer: All claims expressed in this article are solely those of the authors and do not necessarily represent those of their affiliated organizations, or those of the publisher, the editors and the reviewers. Any product that may be evaluated in this article or claim that may be made by its manufacturer is not guaranteed or endorsed by the publisher.
Research integrity at Frontiers
Learn more about the work of our research integrity team to safeguard the quality of each article we publish.