- 1Institute of Geriatric Cardiovascular Disease, Chengdu Medical College, Chengdu, China
- 2State Key Laboratory of Biocontrol, School of Life Sciences, Sun Yat-sen University, Guangzhou, China
- 3School of Acupuncture-Moxibustion and Tuina, Chengdu University of Traditional Chinese Medicine, Chengdu, China
- 4Department of Cardiology, Sichuan Academy of Medical Sciences and Sichuan Provincial People’s Hospital, University of Electronic Science and Technology of China, Chengdu, China
- 5The Sichuan Provincial Key Laboratory of Human Disease Study, Institute of Laboratory Medicine, Sichuan Provincial People’s Hospital, University of Electronic Science and Technology of China, Chengdu, China
- 6The Center for Heart Development, Hunan Normal University, Changsha, China
- 7Guangdong Provincial Key Laboratory of Pathogenesis, Targeted Prevention and Treatment of Heart Disease, Guangzhou, China
Background: Atrioventricular nodal reentrant tachycardia (AVNRT) is a common arrhythmia. Growing evidence suggests that family aggregation and genetic factors are involved in AVNRT. However, in families with a history of AVNRT, disease-causing genes have not been reported.
Objective: To investigate the genetic contribution of familial AVNRT using a whole-exome sequencing (WES) approach.
Methods: Blood samples were collected from 20 patients from nine families with a history of AVNRT and 100 control participants, and we systematically analyzed mutation profiles using WES. Gene-based burden analysis, integration of previous sporadic AVNRT data, pedigree-based co-segregation, protein-protein interaction network analysis, single-cell RNA sequencing, and confirmation of animal phenotype were performed.
Results: Among 95 related reference genes, seven candidate pathogenic genes have been identified both in sporadic and familial AVNRT, including CASQ2, AGXT, ANK2, SYNE2, ZFHX3, GJD3, and SCN4A. Among the 37 reference genes from sporadic AVNRT, five candidate pathogenic genes were identified in patients with both familial and sporadic AVNRT: LAMC1, ryanodine receptor 2 (RYR2), COL4A3, NOS1, and ATP2C2. To identify the common pathogenic mechanisms in all AVNRT cases, five pathogenic genes were identified in patients with both familial and sporadic AVNRT: LAMC1, RYR2, COL4A3, NOS1, and ATP2C2. Considering the unique internal candidate pathogenic gene within pedigrees, three genes, TRDN, CASQ2, and WNK1, were likely to be the pathogenic genes in familial AVNRT. Notably, the core calcium-signaling pathway may be closely associated with the occurrence of AVNRT, including CASQ2, RYR2, TRDN, NOS1, ANK2, and ATP2C2.
Conclusion: Our pedigree-based studies demonstrate that RYR2 and related calcium signaling pathway play a critical role in the pathogenesis of familial AVNRT using the WES approach.
Introduction
Atrioventricular nodal reentrant tachycardia (AVNRT) is a relatively common arrhythmia, accounting for approximately 45–65% of paroxysmal supraventricular tachycardia (PSVT; 1). The heart rate of a normal adult is typically 60 to 100 beats per minute, whereas the heart rate of patients with AVNRT exceeds 150 beats per minute (2–4). This continuous re-excitement of the myocardium can induce arrhythmias, syncope, and even sudden death.
Slow and fast atrioventricular nodal pathways are currently recognized as the pathobiological mechanism for AVNRT, wherein the calcium-signaling pathway may be a crucial regulator (5). Calmodulin-dependent protein kinase II (CaMKII) can directly phosphorylate L-type voltage-gated calcium channels (Cav1.2) to increase Ca2+ influx in cardiomyocytes (6), inducing early depolarization and causing arrhythmia (7). In addition, CaMKII can phosphorylate the ryanodine receptor 2 (RYR2) on the sarcoplasmic reticulum (SR) to release a large amount of Ca2+ into the cytoplasm from SR (8). Excessive Ca2+ activates the Na+/Ca2+ exchanger (NCX), resulting in spontaneous myocyte depolarization and abnormal rhythm (8). Furthermore, the inhibition of NO synthase 1 (NOS1) in SR decreased RYR2 activity because of reducing Ca2+ sparks and shortened action potential causing arrhythmia susceptibility (9). Although radiofrequency ablation for the treatment of AVNRT has shown good results, its precise reentry path and its molecular mechanism remain to be explained.
Atrioventricular nodal reentrant tachycardia was considered a sporadic disease in the past, with a prevalence of 22.5 cases per 10,000 persons (10). Nevertheless, several studies have reported that AVNRT occurred in twins and the same family member (10–13), indicating the phenomenon of family clustering of AVNRT. To date, few studies of AVNRT pedigrees have been available (10, 12, 14, 15), as this is relatively a rare phenomenon. Familial AVNRT pedigree was reported for the first time in 2004 (12). Subsequently, the European clinical study reported 24 AVNRT pedigrees in 2017 (10). Recently, we described the clinical reports of eight families with a history of AVNRT in China in 2021 (15).
The familial AVNRT phenomenon indicates that genetic factors play a crucial role in AVNRT pathogenesis; however, investigations at a molecular level are currently lacking. No report is available on the pathogenic genes of AVNRT. In addition, only two studies have explored the screening of pathogenic genes of AVNRT (5, 16). In 2018, Andreasen et al. first sequenced 67 known pathogenic genes associated with arrhythmia in 298 patients with AVNRT and reported mutations in genes encoding various Na+ and Ca2+ channels (16), suggesting that AVNRT is associated with various ion channels. Recently, we found that AVNRT is closely associated with the neuronal system or ion channels, and 10 potential candidate pathogenic genes were screened out in 82 patients with sporadic AVNRT using whole-exome sequencing (WES; 5). Although variants of genes were identified in patients with sporadic AVNRT, it is difficult to identify the disease phenotype and genotype. Fortunately, the emergence of pedigree-based studies addressed this issue (17). The pedigree-based study had several advantages for a rare variant: reduced genetic heterogeneity, enriched rare alleles, and co-segregated with the disease phenotype and genotype (18). Therefore, we hypothesized that the application of a more integrated approach might help elucidate the genetic etiology of AVNRT disease.
To the best of our knowledge, this is the first study that primarily aimed to investigate the genetic contribution of familial AVNRT using a WES approach. In this study, we used WES to identify potential key genes on the basis of gene-based burden, pedigree-based co-segregation, protein-protein interaction (PPI) analyses, single-cell RNA sequencing, and confirmation of phenotype for AVNRT disease.
Materials and Methods
Collection of Peripheral Blood Samples
Patients with AVNRT were enrolled in the Sichuan Provincial People’s Hospital in China from 2013 to 2020. Familial AVNRT defined that two or more AVNRT patients in a family, or 1 or more clinically diagnosed PSVT patients in a family of AVNRT proband patient. In addition, 100 unrelated ethnically matched healthy participants were recruited from the Sichuan Provincial People’s Hospital. All probands were diagnosed with AVNRT using intracardiac electrophysiological examination during treatment with radiofrequency catheter ablation, and family members diagnosed with AVNRT underwent intracardiac electrophysiological or transesophageal atrial pacing examination. Healthy participants did not have a history of cardiovascular diseases, arrhythmia, systemic immune diseases, cancers, or any other diseases known to cause arrhythmias. Whole blood samples from 20 patients with AVNRT and 100 normal control participants were collected in heparinized vacutainer tubes. Patients had signed an informed consent form before enrollment. This study was approved by the ethics committee of the Sichuan Academy of Medical Sciences and the Sichuan Provincial People’s Hospital.
Intracardiac Electrophysiological Study
Intracardiac electrophysiology recordings included atrial stimulation (burst or additional stimulation pacing) and ventricular stimulation in patients. AVNRT diagnosis is established on the basis of published standards and applicable pacing operations. The physiology of dual atrioventricular node is defined as the atrial-His (AH) interval increase of ≥50 ms after a decreasing interval of 10 ms during the additional stimulation of the single atrium or the AH interval increase of ≥50 ms after the pacing cycle length is shortened by 10 ms. If continuous AVNRT is not induced (lasting more than 30 s), the same pacing procedure was repeated with isoproterenol administration as described above.
Whole-Exome Sequencing, Variant Selection, and Annotation
In brief, we purified DNA from the peripheral blood using the QIAamp DNA Blood Mini Kit (Qiagen, Hilden, Germany). Whole-exome enrichment was performed using the SureSelect Human All Exon kit V6 (Agilent Technologies, Santa Clara, CA, United States). The genomic DNA library was sequenced using the HiSeq X and NovaSeq systems (Illumina, San Diego, CA, United States).
The sequenced DNA fragments were aligned with Human Reference Genome (National Center for Biotechnology Information Build 37) on the basis of the Burrows–Wheeler transform. The removal of duplication, realignment, and recalibration were performed with Picard tools1 and GATK2.
The single-nucleotide polymorphisms and insertion-deletion polymorphisms (indels) were performed using GATK3.7 software. The high-confidence variants were annotated with snpEff (Version 4.2)3. In addition, the annotations of all variants were further performed using 1000 Genomes Project data (2014 Oct release)4, the Exome Aggregation Consortium5, EVS6, the ClinVar7 database, and Online Mendelian Inheritance in Man8.
Rare Variants of the Pathogenic Reference Genes
In total, 95 related reference genes as arrhythmia were selected for the analysis of rare variants in patients with AVNRT and control participants (5). These genes were considered reference genes according to our previous study (5). To increase reliability and generalizability of reference genes, data integration was used to select the genes following our previous sporadic AVNRT study. Therefore, the reference genes were identified in patients with both the sporadic and familial AVNRT and were assessed for segregation within families.
Biological process (BP) of Gene Ontology (GO) was performed by database for annotation, visualization and integrated discovery (DAVID) bioinformatics resources according to previous study (19). PPI network of candidate genes were obtained from the STRING database9. The images of single-cell sequencing data from healthy human cardiac tissue were obtained from the Human Protein Atlas10. The mouse phenotypes associated with pathogenic reference genes were extracted from the Mouse Genome Informatics (MGI) database11.
Rare Variants of Common Pathogenic Genes in Sporadic and Familial Atrioventricular Nodal Reentrant Tachycardia
To identify the common pathogenic mechanisms in all AVNRT cases, the 37 most likely pathogenic genes from our previously sporadic AVNRT study (5) were considered the intersection of both sporadic and familial AVNRT, and then candidate pathogenic genes were identified in patients with both familial and sporadic AVNRT, and were assessed for segregation within families.
Biological process analysis was performed by DAVID bioinformatics resources (19). The PPI network of candidate genes was obtained from the STRING database (see text footnote 9). The images of single-cell sequencing data from 24 healthy human tissues are obtained from the Human Protein Atlas (see text footnote 10). There are 51 cell types of human tissues. The mouse phenotypes associated with pathogenic reference genes were extracted from the MGI database (see text footnote 11).
Rare Variants of Potential Pathogenic Genes in Familial Atrioventricular Nodal Reentrant Tachycardia
To analyze the aggregate association of rare variants at the gene level, we performed gene-based burden analysis to obtain gene-level significant associations of familial AVNRT patients (n = 20) and control subjects (n = 100). Rare variants were defined as “deleterious variants” according to 1000 Genomes Project data and ExAC with MAF < 0.001, MAF < 0.01, or MAF < 0.05. Fisher’s exact test was used to evaluate gene-based burden analysis. The gene level across the genome was used to identify risk genes across different allele frequency spectrums.
The significant genes were submitted to the KOBAS3.0 web server12 to obtain the functional gene set Reactome Pathway enrichment. The PPI network of candidate genes was obtained from the STRING database (see text footnote 9). The images of single-cell sequencing data from healthy human cardiac tissue were obtained from the Human Protein Atlas (see text footnote 10). The mouse phenotypes associated with pathogenic reference genes were extracted from the MGI database (see text footnote 11).
Protein–Protein Interactions Network of Potential Pathogenic Genes
Protein–protein interactions network of candidate genes were obtained from the STRING database (see text footnote 9). The relationships among the screened genes were predicted by STRING database and visualized with Cytoscape v2.3 software.
Results
Clinical Data of the Patients
In this study, a total of 20 patients and 100 control participants were included to perform WES. These 20 patients were assessed in nine families, including a total of 93 members (Figure 1). Among 20 patients enrolled in this study, the male to female ratio was 1.86, the mean age at onset was approximately 47.5 years, the heart rate at onset was approximately 176.9 beats per minute, and all the patients were free from structural heart disease (Table 1). All the patients showed typical slow-fast AVNRT, and 60.0% of the patients were successfully treated by radiofrequency ablation during the operation.
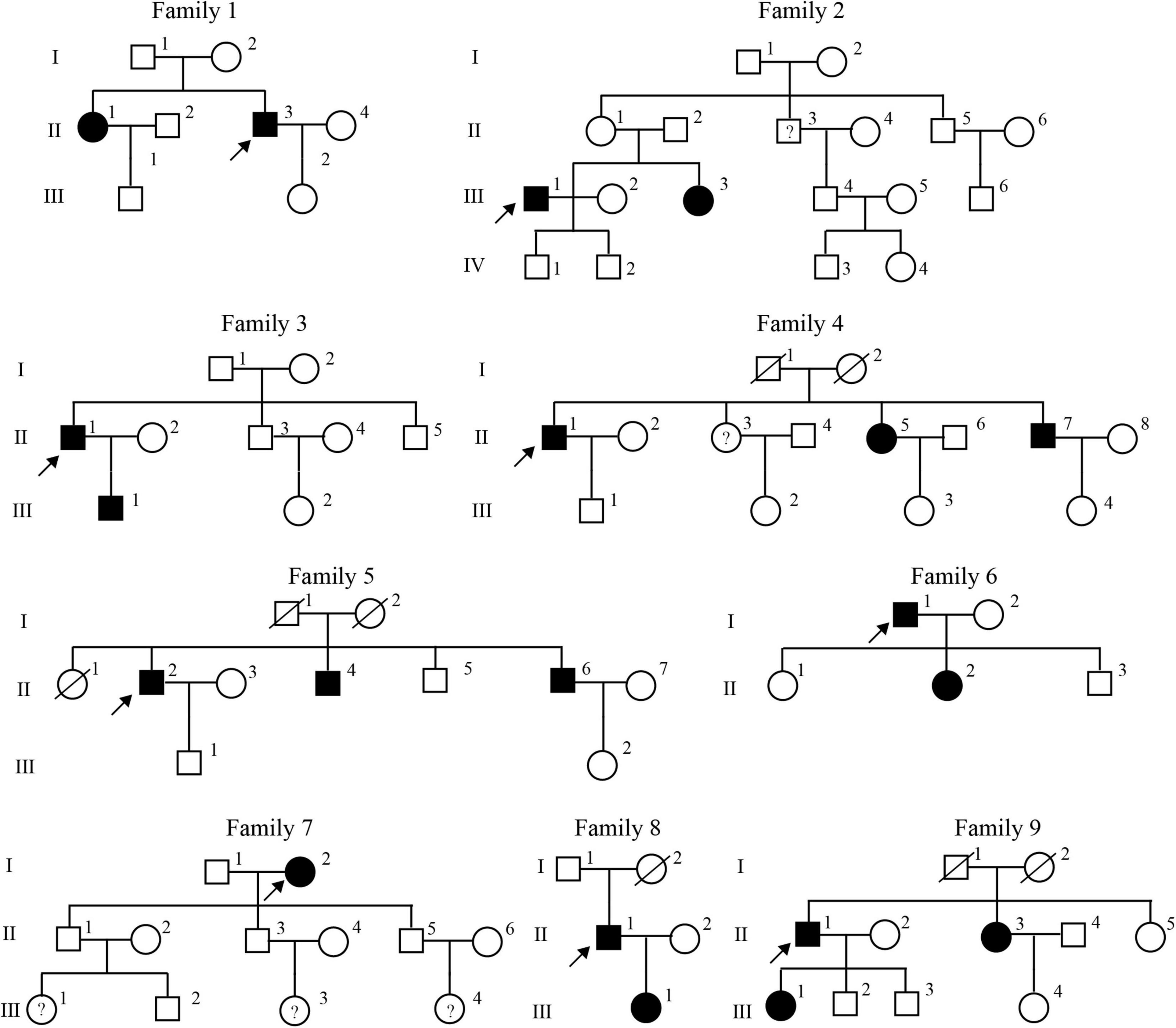
Figure 1. Pedigrees of the nine families with familial atrioventricular nodal reentrant tachycardia. The open squares and circles indicate the normal male and female members, respectively. The filled squares and circles indicate the affected male and female members, respectively. Arrow denotes a proband, and WES investigated the affected individuals. Question mark (?) denotes clinically diagnosed PSVT.
Rare Variants of the Pathogenic Reference Genes
To increase reliability and generalizability of related reference genes, data integration was used to confirm the genes following our previous sporadic AVNRT study (5). Therefore, patients with both sporadic and familial AVNRT were enrolled in this study. Among the 95 related reference genes, seven candidate pathogenic genes have been identified in patients with both familial and sporadic AVNRT: CASQ2, AGXT, ANK2, SYNE2, ZFHX3, GJD3, and SCN4A (Supplementary Table 1). We found CASQ2 have distinct feature between familial and sporadic AVNRT (1 rare variant, 6/20 patients, familial AVNRT; 1 rare variant, 1/82 patient, sporadic AVNRT). Furthermore, rare variants of AGXT, ANK2, SYNE2, GJD3, and SCN4A co-segregated within one pedigree and those of CASQ2 and ZFHX3 within two and three pedigrees, respectively (Supplementary Table 1).
The bubble plot of GO-BP analysis showed that the functions of these genes were mainly associated with cardiac conduction, muscle contraction, and the release of sequestered calcium ions (Supplementary Table 1 and Figure 2A). Furthermore, PPI networks of these genes indicated that CASQ2, ANK2, and SCN4A constituted the network, and ZFHX3 interacted with SYNE2 (Figure 2B). In addition, the results of single-cell sequencing showed that the relative expression of CASQ2, ANK2, and SYNE2 was higher in cardiomyocytes than others (Figure 2C and Supplementary Figure 1), whereas the expression of SCN4A, ZFHX3, AGXT, and GJD3 was relatively lower or not expressed (Figure 2C and Supplementary Figure 1).
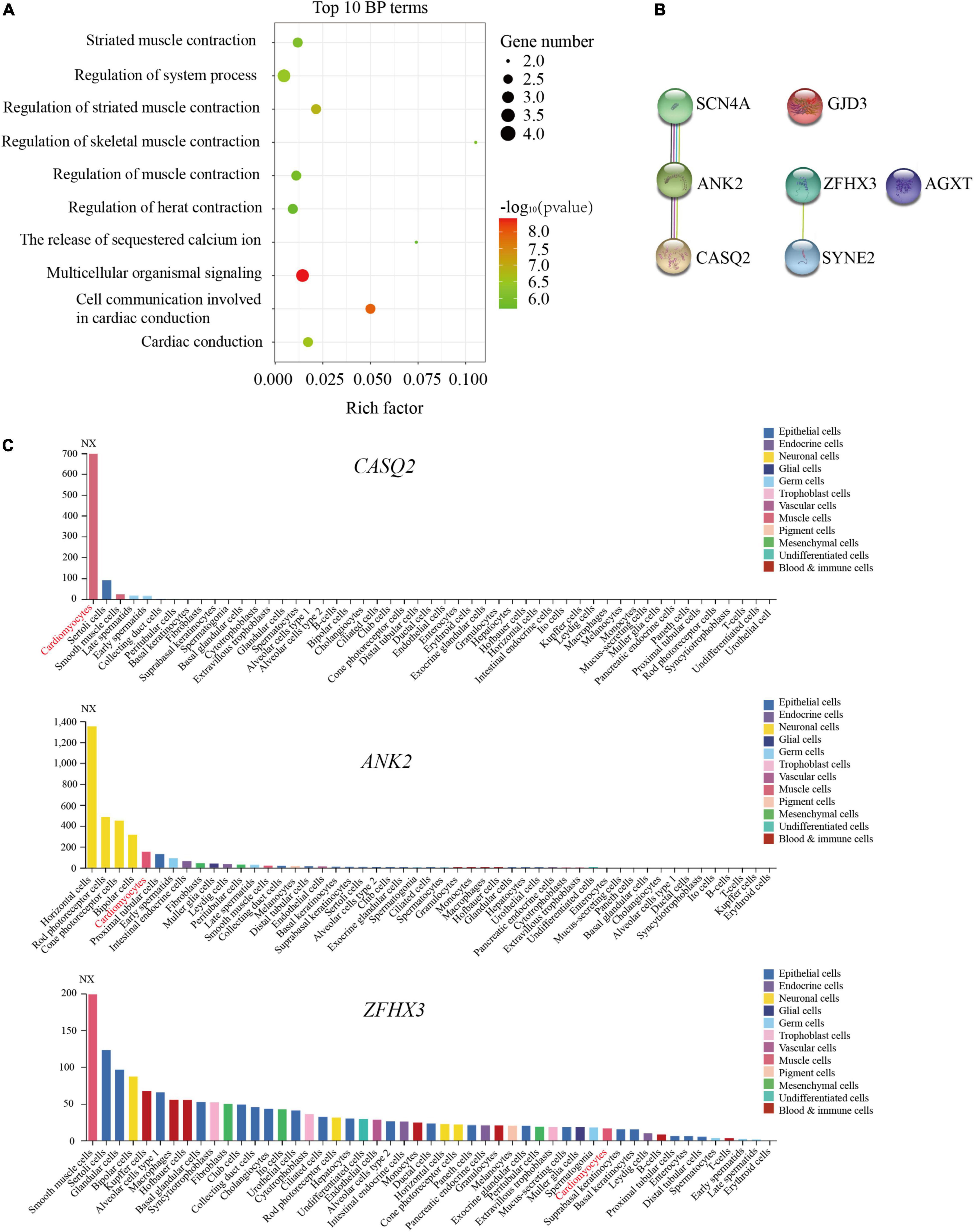
Figure 2. Identification of pathogenic reference genes in familial atrioventricular nodal reentrant tachycardia. (A) The top 10 biological process terms of seven pathogenic genes are depicted using enrichment analysis (P < 0.05). (B) The protein–protein interactions analysis of seven pathogenic genes. (C) The expression of CASQ2, ANK2, and ZFHX3 was shown in different cell types by the single-cell sequencing data.
To further verify these gene functions, the MGI database was used to confirm their phenotype. The disruption of CASQ2, essential for Ca2+ storage, led to ventricular tachycardia in both mice and humans (Table 2). Moreover, the abnormal function of ankyrin-2 (ANK2) may lead to sinoatrial node disease and ankyrin-B-related cardiac arrhythmia in humans (Table 2). In addition, its abnormality increased heart rate variability and caused the abnormal sinoatrial node conduction in the mouse (Table 2). ZFHX3 was identified as a crucial risk factor for atrial fibrillation (20), SYNE2 contributed to cardiac arrhythmia (Table 2), and GJD3 caused abnormal atrioventricular node conduction (Table 2).
Based on MGI database and previous study (20), we suggested that CASQ2, ANK2, SYNE2, GJD3, and ZFHX3 were the most likely pathogenic genes for AVNRT.
Rare Variants of Common Pathogenic Genes in Sporadic and Familial Atrioventricular Nodal Reentrant Tachycardia
To identify the common pathogenic mechanisms in all AVNRT cases, the 37 most likely pathogenic genes from our previous sporadic AVNRT study (5) were considered the intersection of both sporadic and familial AVNRT. Among these genes, five pathogenic genes were identified in patients with both familial and sporadic AVNRT: LAMC1, RYR2, COL4A3, NOS1, and ATP2C2 (Supplementary Table 2). We identified only one gene NOS1 that was totally shared the same two rare variants both in six familial (6/20 patients) and 33 sporadic (33/82 patients) AVNRT patients (Supplementary Table 2). Other genes have distinct features between familial and sporadic AVNRT: LAMC1 (1 rare variant, 2/20 patients, familial AVNRT; 4 rare variants, 7/82 patients, sporadic AVNRT), RYR2 (1 rare variant, 1/20 patient, familial AVNRT; 8 rare variants, 8/82 patients, sporadic AVNRT), COL4A3 (1 rare variant, 2/20 patients, familial AVNRT; 5 rare variants, 5/82 patients, sporadic AVNRT); ATP2C2 (2 rare variants, 2/20 patients, familial AVNRT; 5 rare variants, 5/82 patients, sporadic AVNRT; Supplementary Table 2). Furthermore, the rare variants of LAMC1, COL4A3, NOS1, and ATP2C2 co-segregated within one pedigree apart from RYR2 (Supplementary Table 2).
The BP enrichment analysis suggested that the functions of these genes were mainly associated with heart contraction and the regulation of calcium ion (Figure 3A and Supplementary Table 2). In addition, the PPI networks showed that RYR2, NOS1, and ATP2C2 constituted the network, and COL4A3 interacted with LAMC1 (Figure 3B). Moreover, the results of single-cell sequencing data showed that the relative expression of RYR2 and LAMC1 was higher in cardiomyocytes than others (Figure 3C and Supplementary Figure 2), whereas the expression of COL4A3, NOS1, and ATP2C2 was relatively lower or not expressed (Figure 3C and Supplementary Figure 2).
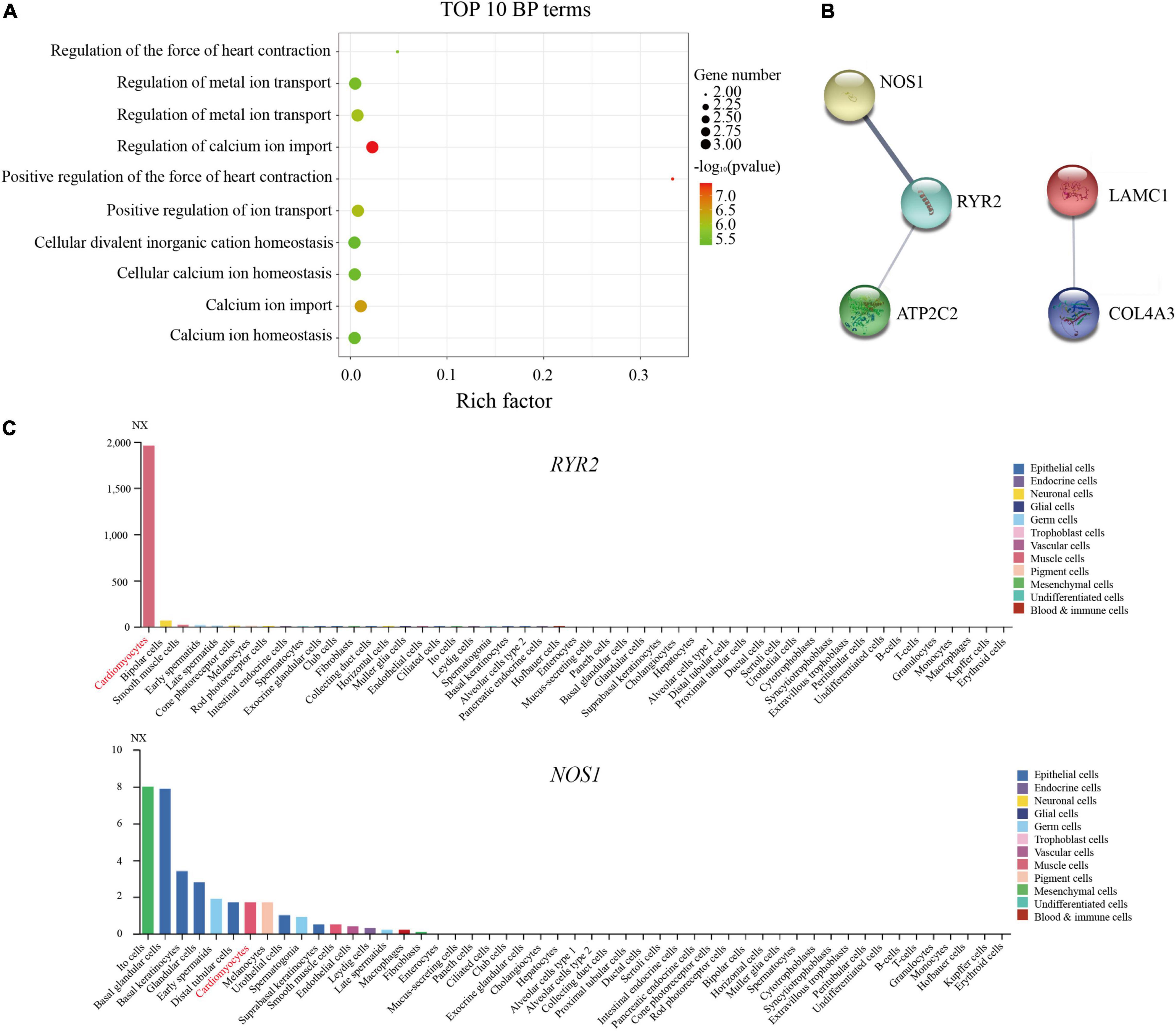
Figure 3. Identification of common pathogenic genes in sporadic and familial atrioventricular nodal reentrant tachycardia. (A) The top 10 biological process terms of five pathogenic genes are depicted using enrichment analysis (P < 0.05). (B) The protein–protein interactions analysis of five pathogenic genes. (C) The expression of RYR2 and NOS1 was shown in different cell types using the single-cell sequencing data.
The biological function and phenotype of these genes were further explored using the MGI database. Homozygous mutation in the NOS1 gene led to abnormal cardiac muscle relaxation and increased heart rate in the mouse (Table 2). The disruption of RYR2 was associated with ventricular dysplasia and ventricular tachycardia in humans, whereas it is mainly associated with an increased heart rate and ventricular tachycardia in the mouse (Table 2). However, cardiac diseases were independent of the functions of LAMC1, COL4A3, and ATP2C2 (Table 2).
Considering their functions and previous study (5), RYR2 and NOS1 were likely to be causal genes for AVNRT.
Rare Variants of Pathogenic Genes in Familial Atrioventricular Nodal Reentrant Tachycardia
In search of the underlying pathogenic mechanisms within AVNRT pedigrees, we imposed more restrictive criteria: more than two mutations and one homozygous mutation in one gene segregated at least two pedigrees. A total of 299 genes with 452 rare variants were identified (Supplementary Table 3).
As shown in Supplementary Table 3, the three AVNRT-related traits among the pathways in the Reactome databases were as follows: (1) stimuli-sensing channels, (2) RYR tetramers transport Ca2+ from the SR lumen to the cytosol, and (3) ion channel transport. In addition, seven pathogenic genes were identified, including TRDN, ANO6, SLC9C1, CASQ2, ATP6V0A4, SGK2, and WNK1. Remarkably, CASQ2 has been involved in AVNRT as reference genes.
Mouse genome informatics database and previous studies was further used to confirm the phenotype of these genes. The disruption of TRDN contributed to ventricular tachycardia in humans (Table 2; 21). Mice lacking ANO6 developed shortened PQ intervals (Table 2). The aberration of WNK1 led to hereditary sensory and autonomic neuropathy in humans (22). Considering their functions and previous study (21, 22), TRDN, CASQ2, and WNK1 were likely to be the common pathogenic genes in familial AVNRT.
The Calcium-Signaling Pathway of Atrioventricular Nodal Reentrant Tachycardia
To explore the internal relationship of the candidate pathogenic genes in this study, PPI network analysis was further constructed. Among these 14 candidate pathogenic genes, three networks were established; these genes were CASQ2, AGXT, ANK2, SYNE2, ZFHX3, GJD3, SCN4A, LAMC1, RYR2, COL4A3, NOS1, ATP2C2, TRDN, and WNK1 (Figure 4A). PPI networks indicated that the genes constituted network 1 (CASQ2, ANK2, SCN4A, RYR2, NOS1, ATP2C2, and TRDN), network 2 (SYNE2 and ZFHX3), and network 3 (LAMC1 and COL4A3).
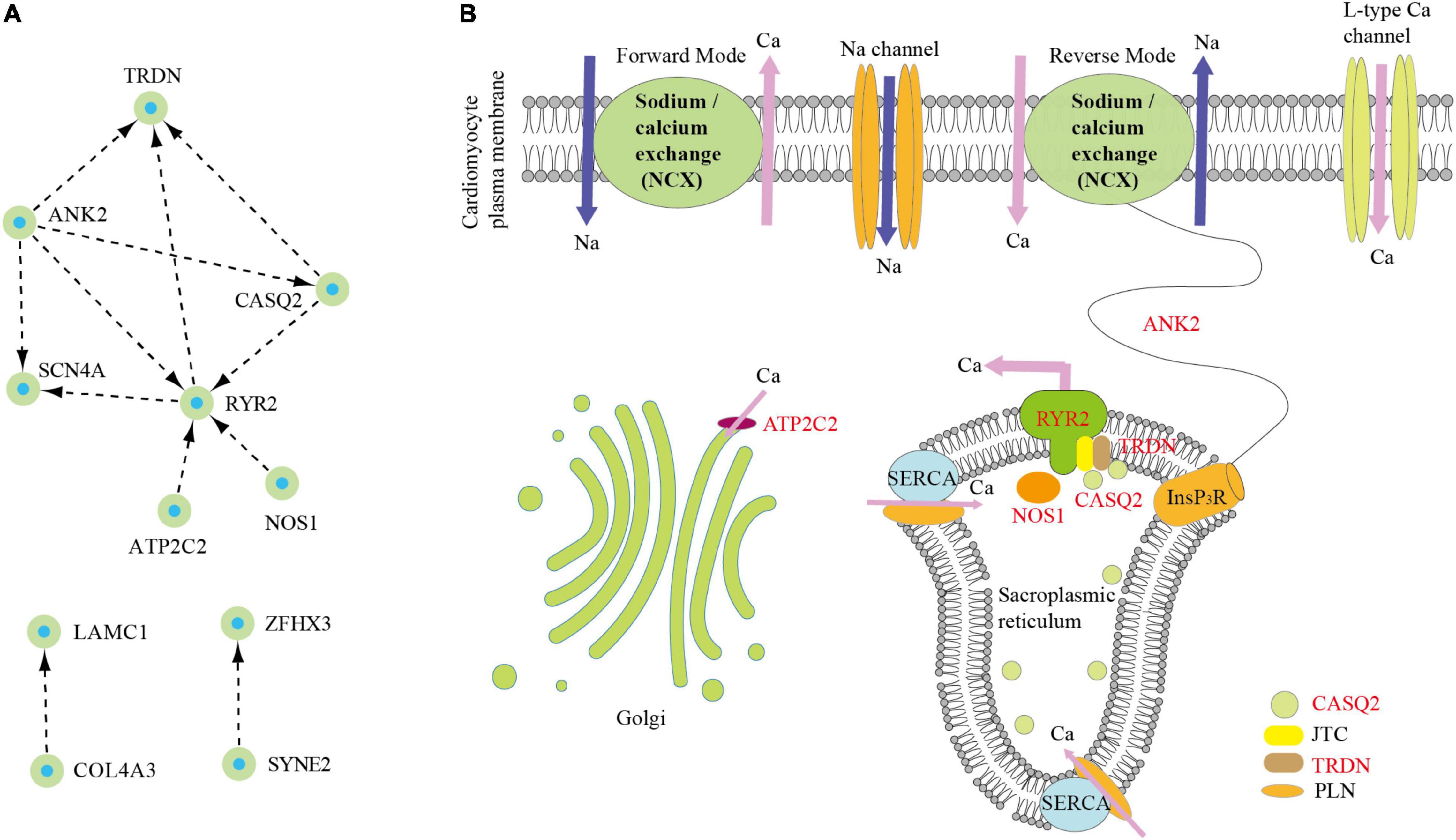
Figure 4. Identification of core-signaling pathway in atrioventricular nodal reentrant tachycardia (AVNRT). (A) The protein–protein interactions analysis of 14 pathogenic genes. (B) Schematic representation of core-signaling pathway in AVNRT.
The maximum network 1 was mainly associated with the calcium-signaling pathway using Kyoto Encyclopedia of Genes and Genomes enrichment analysis (Figure 4B). Among them, RYR2 acted as a calcium channel that released calcium ions into the cytoplasm from the SR and thus regulated cardiac muscle contraction (23). The RYR forms a complex with TRDN, junction (JTC), and CASQ instead of acting independently (24). Moreover, the mutations of RYR2 or CASQ lead to Ca2+ leak in ventricular tachycardia and thus contribute to Ca2+ waves in arrhythmogenic as a result of the increasing Ca2+ spark frequency and rising flux (24, 25). Particularly, CASQ2 and TRDN have also been identified in this study. Furthermore, another RYR2-related gene is neuronal NOS1, which is located in the cardiac SR and enhances contraction through NO production (26). The present studies have shown that the inhibition of NOS1 decreased RYR2 activity because of reducing Ca2+ sparks and shortened action potential causing arrhythmia susceptibility (9, 26). In addition, ANK2 from the SR promotes the flow of calcium ions into the plasma membrane through the inositol triphosphate receptor and NCX (27). ATP2C2 encodes calcium-transporting ATPase, removing calcium from the cytosol into the Golgi body (28). Therefore, the calcium-signaling pathway may be closely associated with the occurrence of AVNRT.
Discussion
Although significant inroads have been achieved in elucidating the pathogenesis of AVNRT (1, 5), the molecular mechanisms associated with this disease remain in its early stages. The sporadic studies contributed to the discovery of a large number of candidate pathogenic genes (5); however, it is difficult to effectively rule out unrelated genes. Unlike the sporadic studies, the pedigree-based linkage study directly observes the segregation of variants with disease phenotype (17). The integrated analysis of sporadic and familiar studies may provide novel strategies for exploring the more prevalent pathogenesis. Phenotypes associated with pathogenic genes were further confirmed using the MGI database. Thus, we took advantage of phenotype analysis and integrated sporadic and pedigree analyses to reveal the novel genetic associations with AVNRT. In this study, genes such as CASQ2, ANK2, ZFHX3, RYR2, NOS1, TRDN, and WNK1 were likely pathogenic.
Recently, accumulating studies have revealed that genetic factors may contribute to the pathogenesis of AVNRT (10–13). However, little is known about the genetic role of AVNRT. In 298 patients with AVNRT, the disease was observed to be associated with Na+ and Ca2+ channels detected using next-generation sequencing (16). Recently, we, for the first time, found that AVNRT was closely associated with the neuronal system or ion channels, and 10 potential candidate pathogenic genes were screened out in 82 patients with sporadic AVNRT using WES (5). Among the pathogenic reference genes, multiple variants in ion channel genes (CASQ2, ANK2, and SCN4A) were further confirmed both in previous sporadic (5) and these familial studies. The gene CASQ2, encoding the calcium-binding protein, played a crucial role in excitation-contraction coupling, regulated the heart rate, and was associated with ventricular tachycardia (29–31). Moreover, another calcium ion transport-related gene ANK2 may lead to cardiac arrhythmia (32). ZFHX3 was identified as a crucial risk factor for atrial fibrillation (33), and SYNE2 contributed to atrial fibrillation (34). These results suggested calcium handling might have played a crucial role in the pathogenesis of AVNRT.
Slow and fast atrioventricular nodal pathways are currently recognized as the mainly pathobiological mechanism for AVNRT, wherein the calcium-signaling pathway may be a crucial regulator (5). CaMKII can directly phosphorylate L-type voltage-gated calcium channels (Cav1.2) to increase Ca2+ influx in cardiomyocytes, inducing early depolarization and causing an arrhythmia (6). Moreover, CaMKII can phosphorylate the RYR2 on the SR to release a large amount of Ca2+ into the cytoplasm from the SR, and excessive Ca2+ activates the NCX, resulting in spontaneous myocyte depolarization and abnormal rhythm (8). Furthermore, the inhibition of NOS1 in the SR decreased RYR2 activity because of reducing Ca2+ sparks and shortened action potential causing arrhythmia susceptibility (9).
In both sporadic and familial AVNRT, there were six calcium channel-associated genes, including RYR2, NOS1, TRDN, CASQ2, ANK2, and ATP2C2. RYR2 acted as a calcium channel that released calcium ions into the cytoplasm from the SR and thus regulated cardiac muscle contraction (23). The RYR forms a complex with TRDN, JTC, and CASQ instead of acting independently (24). Moreover, the mutations of RYR2 or CASQ lead to Ca2+ leak in ventricular tachycardia, thus contributing to Ca2+ waves in arrhythmogenic as a result of the increasing Ca2+ spark frequency and rising flux (24, 25). Particularly, CASQ2 and TRDN have also been identified in this study. Furthermore, another RYR2-related gene was neuronal NOS1. NOS1 is located in the cardiac SR and enhances contraction through NO production (26). The studies showed that the inhibition of NOS1 decreased RYR2 activity because of reducing Ca2+ sparks and shortened action potential causing arrhythmia susceptibility (9, 26). From these findings, RYR2 as the core-signaling pathway may be closely associated with the occurrence of AVNRT. The functions of these calcium channel-associated genes are currently being explored in functional experiments.
To the best of our knowledge, this is the first study primarily aimed to investigate the genetic contribution of familial AVNRT using a WES approach. The calcium-signaling pathway should be considered seriously for AVNRT.
Data Availability Statement
The data presented in this study are deposited in the CNGB Sequence Archive (CNSA) of China National GeneBank DataBase (CNGBdb) repository, accession number CNP0003176.
Ethics Statement
This study was approved by the Ethics Committee of the Sichuan Academy of Medical Sciences and the Sichuan Provincial People’s Hospital. The patients/participants provided their written informed consent to participate in this study.
Author Contributions
JH, RL, CZ, ZY, XW, and XL: conceptualization. JH, RL, and XL: methodology. JH and CZ: software. JH, RL, XC, and YZ: validation. YZ, TH, ML, and XL: investigation. JH and XL: writing—original draft preparation. JH, CZ, XW, and XL: writing—review and editing. ZY, XW, and XL: resources. XL: supervision and project administration. JH, RL, XW, and XL: funding acquisition. All authors have read and agreed to the published version of the manuscript.
Funding
This work was supported by grants from Chinese National Natural Science Foundation (Nos. 81770379, 32171182, and 81670290) and the Foundation of Chengdu Medical College (CYZZD21-04 and 2021LHPJ-02).
Conflict of Interest
The authors declare that the research was conducted in the absence of any commercial or financial relationships that could be construed as a potential conflict of interest.
Publisher’s Note
All claims expressed in this article are solely those of the authors and do not necessarily represent those of their affiliated organizations, or those of the publisher, the editors and the reviewers. Any product that may be evaluated in this article, or claim that may be made by its manufacturer, is not guaranteed or endorsed by the publisher.
Supplementary Material
The Supplementary Material for this article can be found online at: https://www.frontiersin.org/articles/10.3389/fcvm.2022.910826/full#supplementary-material
Supplementary Figure 1 | The expression of pathogenic reference genes in familial AVNRT. The expression of SCN4A, SYNE2, AGXT, and GJD3 was shown in different cell types by the single-cell sequencing data.
Supplementary Figure 2 | The expression of common pathogenic genes in sporadic and familial AVNRT. The expression of LAMC1, COL4A3, and ATP2C2 was shown in different cell types by the single-cell sequencing data.
Footnotes
- ^ http://picard.sourceforge.net/
- ^ http://www.broadinstitute.org/gsa/wiki/index.php/Home_Page
- ^ http://snpeff.sourceforge.net/
- ^ http://www.1000genomes.org
- ^ http://exac.broadinstitute.org
- ^ http://evs.gs.washington.edu/EVS
- ^ http://www.ncbi.nlm.nih.gov/clinvar
- ^ http://www.omim.org
- ^ https://cn.string-db.org
- ^ http://www.proteinatlas.org
- ^ http://www.informatics.jax.org/
- ^ http://kobas.cbi.pku.edu.cn/kobas3
References
1. Akhtar M, Jazayeri MR, Sra J, Blanck Z, Deshpande S, Dhala A. Atrioventricular nodal reentry: clinical, electrophysiological, and therapeutic considerations. Circulation. (1993) 88:282–95.
2. Porter MJ, Morton JB, Denman R, Lin AC, Tierney S, Santucci PA, et al. Influence of age and gender on the mechanism of supraventricular tachycardia. Heart Rhythm. (2004) 1:393–6.
3. Gonzalez-Torrecilla E, Almendral J, Arenal A, Atienza F, Atea LF, del Castillo S, et al. Combined evaluation of bedside clinical variables and the electrocardiogram for the differential diagnosis of paroxysmal atrioventricular reciprocating tachycardias in patients without pre-excitation. J Am Coll Cardiol. (2009) 53:2353–8. doi: 10.1016/j.jacc.2009.02.059
4. Liuba I, Jonsson A, Safstrom K, Walfridsson H. Gender-related differences in patients with atrioventricular nodal reentry tachycardia. Am J Cardiol. (2006) 97:384–8.
5. Luo R, Zheng C, Yang H, Chen X, Jiang P, Wu X, et al. Identification of potential candidate genes and pathways in atrioventricular nodal reentry tachycardia by whole-exome sequencing. Clin Transl Med. (2020) 10:238–57. doi: 10.1002/ctm2.25
6. Cheng J, Xu L, Lai D, Guilbert A, Lim HJ, Keskanokwong T, et al. CaMKII inhibition in heart failure, beneficial, harmful, or both. Am J Physiol Heart Circ Physiol. (2012) 302:H1454–65.
7. Weiss JN, Garfinkel A, Karagueuzian HS, Chen PS, Qu Z. Early afterdepolarizations and cardiac arrhythmias. Heart Rhythm. (2010) 7:1891–9.
8. Maier LS, Zhang T, Chen L, DeSantiago J, Brown JH, Bers DM. Transgenic CaMKIIdeltaC overexpression uniquely alters cardiac myocyte Ca2+ handling: reduced SR Ca2+ load and activated SR Ca2+ release. Circ Res. (2003) 92:904–11. doi: 10.1161/01.RES.0000069685.20258.F1
9. Wang H, Viatchenko-Karpinski S, Sun J, Gyorke I, Benkusky NA, Kohr MJ, et al. Regulation of myocyte contraction via neuronal nitric oxide synthase: role of ryanodine receptor S-nitrosylation. J Physiol. (2010) 588:2905–17. doi: 10.1113/jphysiol.2010.192617
10. Michowitz Y, Anis-Heusler A, Reinstein E, Tovia-Brodie O, Glick A, Belhassen B. Familial occurrence of atrioventricular nodal reentrant tachycardia. Circ Arrhythm Electrophysiol. (2017) 10:e004680.
11. Lu CW, Wu MH, Chu SH. Paroxysmal supraventricular tachycardia in identical twins with the same left lateral accessory pathways and innocent dual atrioventricular pathways. Pacing Clin Electrophysiol. (2000) 23:1564–6. doi: 10.1046/j.1460-9592.2000.01564.x
12. Hayes JJ, Sharma PP, Smith PN, Vidaillet HJ. Familial atrioventricular nodal reentry tachycardia. Pacing Clin Electrophysiol. (2004) 27:73–6.
13. Namgung J, Kwak JJ, Choe H, Kwon SU, Doh JH, Lee SY, et al. Familial occurrence of atrioventricular nodal reentrant tachycardia in a mother and her son. Korean Circ J. (2012) 42:718–21. doi: 10.4070/kcj.2012.42.10.718
14. Stec S, Deutsch K, Zienciuk-Krajka A. The world’s largest family with familial atrio-ventricular nodal reentry tachycardia. Kardiol Pol. (2015) 73:1339. doi: 10.5603/KP.2015.0249
15. Chen XP, Yan C, Luo R, Zhu Y, Qian M, Liu X, et al. Clinical report of 8 families with atrioventricular nodal reentrant tachycardia from China. Kardiol Pol. (2021) 79:185–7. doi: 10.33963/KP.15739
16. Andreasen L, Ahlberg G, Tang C, Andreasen C, Hartmann JP, Tfelt-Hansen J, et al. Next-generation sequencing of AV nodal reentrant tachycardia patients identifies broad spectrum of variants in ion channel genes. Eur J Hum Genet. (2018) 26:660–8. doi: 10.1038/s41431-017-0092-0
17. Wolf JB, Lindell J, Backstrom N. Speciation genetics: current status and evolving approaches. Philos Trans R Soc Lond B Biol Sci. (2010) 365:1717–33. doi: 10.1098/rstb.2010.0023
18. Zhang Z, Fye S, Borecki IB, Rader JS. Polymorphisms in immune mediators associate with risk of cervical cancer. Gynecol Oncol. (2014) 135:69–73.
19. Dennis G Jr., Sherman BT, Hosack DA, Yang J, Gao W, Lane HC, et al. DAVID: database for annotation, visualization, and integrated discovery. Genome Biol. (2003) 4:3.
20. Tomomori S, Nakano Y, Ochi H, Onohara Y, Sairaku A, Tokuyama T, et al. Maintenance of low inflammation level by the ZFHX3 SNP rs2106261 minor allele contributes to reduced atrial fibrillation recurrence after pulmonary vein isolation. PLoS One. (2018) 13:e0203281. doi: 10.1371/journal.pone.0203281
21. Rabbani B, Khorgami M, Dalili M, Zamani N, Mahdieh N, Gollob MH. Novel cases of pediatric sudden cardiac death secondary to TRDN mutations presenting as long QT syndrome at rest and catecholaminergic polymorphic ventricular tachycardia during exercise: the TRDN arrhythmia syndrome. Am J Med Genet. (2021) 185:3433–45. doi: 10.1002/ajmg.a.62464
22. Loggia ML, Bushnell MC, Tetreault M, Thiffault I, Bherer C, Mohammed NK, et al. Carriers of recessive WNK1/HSN2 mutations for hereditary sensory and autonomic neuropathy type 2 (HSAN2) are more sensitive to thermal stimuli. J Neurosci. (2009) 29:2162–6. doi: 10.1523/JNEUROSCI.4633-08.2009
23. Gillespie D, Fill M. Pernicious attrition and inter-RyR2 CICR current control in cardiac muscle. J Mol Cell Cardiol. (2013) 58:53–8. doi: 10.1016/j.yjmcc.2013.01.011
24. Eisner DA, Caldwell JL, Kistamas K, Trafford AW. Calcium and Excitation-Contraction Coupling in the Heart. Circ Res. (2017) 121:181–95.
25. Song L, Alcalai R, Arad M, Wolf CM, Toka O, Conner DA, et al. Calsequestrin 2 (CASQ2) mutations increase expression of calreticulin and ryanodine receptors, causing catecholaminergic polymorphic ventricular tachycardia. J Clin Invest. (2007) 117:1814–23. doi: 10.1172/JCI31080
26. Nikolaienko R, Bovo E, Zima AV. Redox dependent modifications of ryanodine receptor: basic mechanisms and implications in heart diseases. Front Physiol. (2018) 9:1775. doi: 10.3389/fphys.2018.01775
27. Chagula DB, Rechcinski T, Rudnicka K, Chmiela M. Ankyrins in human health and disease - an update of recent experimental findings. Arch Med Sci. (2020) 16:715–26. doi: 10.5114/aoms.2019.89836
28. Missiaen L, Dode L, Vanoevelen J, Raeymaekers L, Wuytack F. Calcium in the Golgi apparatus. Cell Calcium. (2007) 41:405–16.
29. Knollmann BC, Chopra N, Hlaing T, Akin B, Yang T, Ettensohn K, et al. Casq2 deletion causes sarcoplasmic reticulum volume increase, premature Ca2+ release, and catecholaminergic polymorphic ventricular tachycardia. J Clin Invest. (2006) 116:2510–20. doi: 10.1172/JCI29128
30. Denegri M, Bongianino R, Lodola F, Boncompagni S, De Giusti VC, Avelino-Cruz JE, et al. Single delivery of an adeno-associated viral construct to transfer the CASQ2 gene to knock-in mice affected by catecholaminergic polymorphic ventricular tachycardia is able to cure the disease from birth to advanced age. Circulation. (2014) 129:2673–81. doi: 10.1161/CIRCULATIONAHA.113.006901
31. Hwang HS, Hasdemir C, Laver D, Mehra D, Turhan K, Faggioni M, et al. Inhibition of cardiac Ca2+ release channels (RyR2) determines efficacy of class I antiarrhythmic drugs in catecholaminergic polymorphic ventricular tachycardia. Circ Arrhythm Electrophysiol. (2011) 4:128–35. doi: 10.1161/CIRCEP.110.959916
32. Koenig SN, Mohler PJ. The evolving role of ankyrin-B in cardiovascular disease. Heart Rhythm. (2017) 14:1884–9. doi: 10.1016/j.hrthm.2017.07.032
33. Zhai C, Cong H, Liu Y, Zhang Y, Liu X, Zhang H, et al. Rs7193343 polymorphism in zinc finger homeobox 3 (ZFHX3) gene and atrial fibrillation: an updated meta-analysis of 10 case-control comparisons. BMC Cardiovasc Disord. (2015) 15:58. doi: 10.1186/s12872-015-0044-y
Keywords: familial AVNRT, arrhythmia, whole-exome sequencing, pathogenic genes, calcium-signaling pathway
Citation: Huang J, Luo R, Zheng C, Cao X, Zhu Y, He T, Liu M, Yang Z, Wu X and Li X (2022) Integrative Analyses Identify Potential Key Genes and Calcium-Signaling Pathway in Familial Atrioventricular Nodal Reentrant Tachycardia Using Whole-Exome Sequencing. Front. Cardiovasc. Med. 9:910826. doi: 10.3389/fcvm.2022.910826
Received: 01 April 2022; Accepted: 20 June 2022;
Published: 18 July 2022.
Edited by:
Zhihua Wang, Chinese Academy of Medical Sciences and Peking Union Medical College, ChinaReviewed by:
Xujie Liu, Chinese Academy of Medical Sciences and Peking Union Medical College, ChinaQingqing Yan, Chinese Academy of Medical Sciences and Peking Union Medical College, China
Copyright © 2022 Huang, Luo, Zheng, Cao, Zhu, He, Liu, Yang, Wu and Li. This is an open-access article distributed under the terms of the Creative Commons Attribution License (CC BY). The use, distribution or reproduction in other forums is permitted, provided the original author(s) and the copyright owner(s) are credited and that the original publication in this journal is cited, in accordance with accepted academic practice. No use, distribution or reproduction is permitted which does not comply with these terms.
*Correspondence: Xiaoping Li, lixiaoping0119@163.com
†These authors have contributed equally to this work