- 1Department of Laboratory Medicine, Peking Union Medical College Hospital, Peking Union Medical College and Chinese Academy of Medical Sciences, Beijing, China
- 2State Key Laboratory of Complex Severe and Rare Diseases, Peking Union Medical College Hospital, Peking Union Medical College and Chinese Academy of Medical Sciences, Beijing, China
Copeptin is the carboxyl-terminus of the arginine vasopressin (AVP) precursor peptide. The main physiological functions of AVP are fluid and osmotic balance, cardiovascular homeostasis, and regulation of endocrine stress response. Copeptin, which is released in an equimolar mode with AVP from the neurohypophysis, has emerged as a stable and simple-to-measure surrogate marker of AVP and has displayed enormous potential in clinical practice. Cardiovascular disease (CVD) is currently recognized as a primary threat to the health of the population worldwide, and thus, rapid and effective approaches to identify individuals that are at high risk of, or have already developed CVD are required. Copeptin is a diagnostic and prognostic biomarker in CVD, including the rapid rule-out of acute myocardial infarction (AMI), mortality prediction in heart failure (HF), and stroke. This review summarizes and discusses the value of copeptin in the diagnosis, discrimination, and prognosis of CVD (AMI, HF, and stroke), as well as the caveats and prospects for the application of this potential biomarker.
Introduction
Cardiovascular disease (CVD) is currently acknowledged as a primary threat to the health of the global population. According to the Global Burden of Diseases, Injuries, and Risk Factors Study (GBD) 2016, CVDs are attributed to the largest number of deaths among non-communicable diseases, with an estimated 17.8 million (95% uncertainty interval 17.5–18.0 million) deaths, accounting for approximately one-third of all deaths globally (1). Driven by the growth and increasing age of the population, the total global deaths from CVD increased by 21.1% between 2007 and 2017 (1, 2). Multiple complicated factors have been found to contribute to the development and progression of CVDs, including individual-level risk factors [smoking (3), elevated blood pressure (4, 5), and cholesterol (6)], as well as societal-level health determinants (2). Deaths in patients with CVD mainly result from ischemic heart disease, stroke, hypertensive heart disease, cardiomyopathy, rheumatic heart disease, and atrial fibrillation (2). Mortality and morbidity caused by CVDs can be devastating for families and cause an enormous economic burden to society. Therefore, early identification and accurate diagnosis are crucial for adequate intervention and personalized treatment of relevant patients.
Arginine vasopressin (AVP) is a crucial hormone that regulates fluid homeostasis, vasoconstriction, and endocrine stress response. As the C-terminal fragment of the pro-AVP precursor, copeptin is released in an equimolar amount with AVP in response to osmotic, hemodynamic, and stress stimuli (7, 8). Copeptin has additional advantages compared to AVP (9–11) and has proven to be a potential biomarker and has been widely evaluated for its diagnostic value in clinical practice. The physiological function of endogenous AVP and potential clinical use of copeptin are depicted in Figure 1. Increased copeptin concentrations have also been described in acute disorders, including sepsis, stroke, and acute myocardial infarction (AMI) (12–14). Among these diseases, diagnosis, and management of CVDs could benefit the most from the introduction of copeptin measurement (15). Copeptin plays a variety of roles in the differential diagnosis, risk stratification, and prognostic prediction of patients with CVD, and it exerts additional value in the rapid rule-out of AMI and outcome prediction of heart failure (HF) when used in combination with other conventional cardiac biomarkers. Although copeptin has received increasing attention from medical practitioners, further investigation and evidence are required to provide better identification and differentiation of certain diseases to be accepted as a routine clinical measurement in the future. Here, we discuss the clinical efficacy of copeptin in CVDs, as well as the limitations and prospects of the clinical utility of copeptin as a routine biomarker.
Biology of Copeptin and Vasopressin
Copeptin, a leucine-rich glycopeptide (molecular mass ~4 kDa), was first discovered by Holwerda from the posterior pituitary of pigs (7), and its 39 amino acid sequence was determined in 1981 (human: ASDRSNATQLDGPAGALLLRLVQLAGAPEPFEPAQPDAY) (16). Copeptin is derived from the precursor peptide pre-pro-vasopressin (164 amino acids), which is formed from the AVP peptide, neurophysin II, and copeptin (17, 18). AVP, also known as antidiuretic hormone (ADH), is a 9 amino acids peptide [CYFQNCPRG (disulfide bond cys1-cys6)]. The gene that encodes this precursor is located on the short arm of chromosome 20 (20p13), with three exons and two introns (19, 20).
Pre-pro-vasopressin is synthesized in the magnocellular neurons of the supraoptic nucleus (SON) and paraventricular nucleus (PVN) of the hypothalamus. The axons of these neurons constitute the hypothalamic-pituitary tract, which terminates in the posterior pituitary. During transport along the tract, the precursor is proteolytically cleaved into AVP, neurophysin II, and copeptin, and the products are stored in the posterior pituitary (21–23). The complete process of synthesis, transport, and storage takes ~1–2 h (24). Elevated osmotic pressure or decreased volume of circulating fluids can stimulate osmoreceptors in the hypothalamus, resulting in the stoichiometric release of AVP and copeptin into nearby capillaries (25). AVP and copeptin secretions are also regulated by other neuroendocrine mechanisms. The parvocellular neurons of the PVN can produce the precursor, which is transported along the portal vessel to the anterior pituitary. In this pathway, AVP can interact with corticotrophin-releasing hormone (CRH) to stimulate the release of adrenocorticotropic hormone (ACTH) from endocrine cells in the anterior pituitary, reflecting somatic stress levels (26).
After release into the bloodstream, AVP activates intracellular effectors by binding to three receptor subtypes [V1a, V1b (V3), and V2] (27, 28). V1a receptor (V1aR) is located mainly in smooth muscle vascular cells (also in the liver, brain, and platelets) and controls cell contraction. V1bR is located in the anterior pituitary (and other areas in the brain, such as the hypothalamus) and regulates the release of ACTH, which stimulates the release of cortisol in the adrenal cortex [known as the hypothalamic-pituitary adrenal (HPA) axis] (29–31). V2R exists mainly in the basolateral membrane of collecting tubules in the kidney and mediates the aquaporin-2 water channel insertion into the apical membrane of collecting duct cells, causing water reabsorption, and maintaining osmotic homeostasis (32, 33). The neurophysin II gene with a missense mutation was found to be associated with human autosomal dominant neurohypophyseal diabetes insipidus (34). However, the physiological function of copeptin is unclear, and the specific receptor for copeptin has not yet been identified. A previous study proposed that copeptin could stimulate prolactin release from cultured pituitary cells, which was not supported by another study reporting that copeptin could not serve as a prolactin-releasing factor (35, 36). Another hypothesis indicated that copeptin assisted the refolding of the precursor by facilitating the interaction of misfolded monomers with the calnexin/calreticulin system (37). However, these hypotheses have not been fully validated. Copeptin is regarded as a surrogate marker of AVP.
The release of copeptin is regulated by osmotic pressure changes (38, 39). Fenske et al. (11) showed that median osmotic pressure (289–311 mOsm/kg H2O) caused similar release kinetics of plasma copeptin (from 4 to 29.3 pmol/L) and AVP (from 1 to 10.3 pg/ml). They also reported that copeptin and AVP concentrations were highly correlated, with a Spearman rank correlation coefficient of 0.94. Non-specific somatic stress is another factor that regulates copeptin. Copeptin levels are significantly higher in patients with acute diseases, such as sepsis (40), AMI, stroke (41), and preeclampsia (42), and can serve as a marker of endogenous stress levels (43). However, the clearance of copeptin in vivo has not yet been clearly investigated. Roussel et al. (44) found that copeptin levels were inversely correlated with decreasing glomerular filtration rate in patients with chronic kidney disease, indicating an association between decreased copeptin clearance and impaired renal function.
The normal range of plasma copeptin level is relatively low, which was determined to be 4.2 pmol/L (range 1–13.8 pmol/L) in a healthy cohort of 359 individuals (9). The distribution of copeptin concentration in the healthy population is skewed, and the 95, 97.5, and 99 percentiles are 9.8, 13, and 18.9 pmol/L, respectively (45). Median copeptin levels were significantly higher in men than in women but were not correlated with age (9, 46). The circadian rhythm and nutrient intake were found to slightly influence copeptin levels (47, 48), suggesting that copeptin is a robust and reliable marker in healthy individuals. Copeptin appeared to be increased by exercise but returned to normal levels after 1 h (9). In healthy participants who received an infusion of 3% hypertonic saline, copeptin was significantly higher in those who experienced nausea and/or vomiting (median 39.0 vs. 20.0 pmol/L) (49). Increased blood pressure was reported to correlate with plasma copeptin in a large population-based study of young and healthy adults (50). Confounding factors must be identified before interpreting the results based on the copeptin levels.
AVP has several detection limitations, including a short half-life, instability in circulation, and association with platelets, and the radioimmunoassay is not readily available in routine check-ups (10, 12, 51). Copeptin, in contrast, has a longer half-life (26 vs. 12 min of AVP) and is more stable; thus, it is simpler to measure using two commercially available approaches: the Brahms original sandwich immunoluminometric assay and the immunofluorescent assay on the KRYPTOR platform (9, 11). The turnaround time is 0.5–2.5 h, with a detection limit of 0.4 and 0.69 pmol/L, respectively. There are also some ELISA assays available but only used by research, and they lack approval and clinical validation. Plasma and serum samples are suggested to be used to measure copeptin concentration, while other sample types like cerebrospinal fluid need more validation before application (52). In all, copeptin is regarded as a potential surrogate marker for AVP.
Copeptin in AMI
As a common cardiac emergency, AMI caused by myocardial ischemia and necrosis has a high risk of morbidity and mortality if not treated timeously (53). Therefore, patients with the suspected acute coronary syndrome (ACS) should be referred immediately to the emergency department (ED) for evaluation (54). However, only ~10% of patients with chest pain in the ED were diagnosed with AMI (55), and a delay in the rapid rule-out of AMI can preclude the detection of the underlying disease, require prolonged monitoring, and serial blood sampling of patients, and waste medical resources. Besides, the “gray zone” for troponin elevation makes the sensitivity and negative predictive value (NPV) unsatisfactory. Therefore, rapid rule-out of AMI to avoid deleterious consequences for patients and elevated medical costs remains a major challenge. In addition, an effective procedure for risk stratification, as well as prompt identification and assessment of patients with AMI who are at risk of adverse outcomes, is essential for optimized care and resource allocation to preclude serious disability or sequelae. Physical examinations, such as 12-lead electrocardiography (ECG), are necessary for patients with symptoms suggestive of AMI, but the approaches can be confusing and unclear when the results are negative. Therefore, biomarkers that can rapidly and definitively reflect cardiac abnormalities are required.
Cardiac troponins (cTn) are a group of cardiac structural proteins that regulate the calcium-mediated interaction of actin and myosin (56). When a sufficient number of myocytes die due to necrosis or apoptosis, cTn can be detected in the blood (57). Two specific cardiac isoforms of troponin are cTnI and cTnT which are almost exclusively in the heart (58), but cTnT is found to express in skeletal muscles to a minor extent, which means some elevations of cTnT might be due to skeletal muscle abnormality (59). In patients with AMI, cTnI and cTnT start to appear in the circulation early after the AMI onset. The biomarkers reach a peak after 14–16 h and return to a normal level for 4–10 days (60, 61). According to the Fourth Universal Definition of Myocardial Infarction (62), AMI is defined as when there is an acute myocardial injury with clinical evidence of acute myocardial ischemia and with detection of a rise and/or fall of cTn values with at least one value above the 99th percentile URL. In 2007–2010, high-sensitivity assays started to be used to improve cTn assessment, and assays for high-sensitivity cTn (hs-cTn) with a coefficient of variance of <10% at the 99th percentile of the reference population have been developed and widely used (63). Compared with conventional cTn assays, hs-cTn assays demonstrate several advantages, including a higher NPV for AMI and reduction of the “troponin-blind” interval (to detect cTn increase earlier) (60, 64). Therefore, hs-cTn became the preferably recommended laboratory test to diagnose AMI in clinical practice (65). However, since cTn is an organ-specific instead of disease-specific biomarker, multiple factors must be considered (including age, sex, renal dysfunction, and time from chest pain onset) when interpreting the results. Besides, cTn cannot be used alone but in conjunction with careful assessment of chest pain characteristics and ECG.
Unlike cTn and other cardiac biomarkers, copeptin, which non-specifically reflects the endogenous stress level at the onset of AMI, has been advanced and widely validated for its clinical value in AMI.
The Release Pattern of Copeptin and cTn in Patients with AMI
As a marker of endogenous stress, copeptin levels rise sharply after myocardial injury following a swift decline (45, 66). A meta-analysis, including 14 studies (9,244 patients), showed that patients with AMI had higher copeptin levels than those without AMI (22.8 vs. 8.3 pmol/L, respectively) at presentation (14). Patients with non-ST-elevation myocardial infarction (NSTEMI) had a significantly lower level of copeptin than those with ST-elevation myocardial infarction (STEMI) but a higher level than those with unstable angina, indicating that copeptin levels were strongly associated with the extent of myocardial necrosis (67).
The release pattern of copeptin in 145 patients undergoing percutaneous coronary intervention (PCI) for a first STEMI was reported by Gu et al. (61), who reported that copeptin levels increased immediately after symptom onset to a peak of 249 pmol/L and normalized within 10 h. Similarly, Slagman et al. (68) reported that copeptin levels were already increased (median 94.0 pmol/L) at the time of first medical contact in the ambulance and decreased significantly over time in patients with NSTEMI. The diagnostic performance of copeptin was very high in early presenters [area under the curve (AUC) 0.96, 95% confidence interval (CI) 0.90–1.0; NPV 100% in the ambulance], but generally decreased over time (AUC 0.75, 95% CI 0.59–0.92 at 2 h), indicating that the particular time of blood collection can affect the amount of information copeptin provided. In contrast, conventional cardiac biomarkers, including creatine kinase isoenzyme (CK-MB), cTn, and hs-cTn increased to their maximum levels at >10 h (61, 67). A comparison between the release patterns of copeptin and other markers after AMI symptom onset is shown in Table 1. Interestingly, in 34 participants who underwent experimental balloon-induced ischemia, cTnI and cTnT levels increased after 30 s of ischemia, whereas copeptin levels did not change significantly (69). The reason for this inconsistency might be that the short duration of the induced reversible ischemia failed to affect the hemodynamic vascular system sufficiently to induce vasopressin release from the hypothalamus.
Copeptin to Rapidly Rule-Out AMI
For patients with AMI, the somatic stress burden is high, and the vasopressin system is activated (70). Copeptin as a surrogate marker of AVP and can indicate endogenous stress. However, as a single non-specific biomarker, copeptin showed modest diagnostic accuracy in the early rule-out of AMI [pooled sensitivity 0.67 (95% CI 0.60–0.73), pooled specificity 0.63 (95% CI 0.57–0.69)] according to a meta-analysis, including 15 studies (8,740 patients) (71), and copeptin alone should not be used as a single diagnostic marker in patients with suspected AMI, especially in late presenters (72). However, when used in combination with other classic biomarkers, copeptin evaluation has emerged as a complementary method.
The Dual-Marker Strategy Involving Copeptin and Cardiac Troponin
Given the different temporal release patterns of copeptin, when it is used in combination with the time-dependent cardiac marker cTn (dual-marker strategy), copeptin has the potential to allow for rapid and accurate rule-out of AMI. This hypothesis was first proposed by Reichlin et al. (67) in 2009 and was confirmed in the following trials (45, 73–77) and meta-analysis (14, 71), that the combination of copeptin and cTn significantly improved the specificity and NPV compared with cTn alone [increased sensitivity from 0.686 (95% CI 0.661–0.710) to 0.905 (95% CI 0.888–0.921), NPV from 0.93 (95% CI 0.924–0.936) to 0.97 (95% CI 0.964–0.975)] (14); thus, a higher proportion of patient discharges was achieved, preventing serial blood drawing and testing, prolonged hospital stay and surplus medical costs (64, 78). In Table 2, we summarize the published research on the diagnostic performance to rapidly rule out AMI when using cTn in combination with copeptin, suggesting that copeptin has the potential to improve the sensitivity and NPV of cTn, especially in patients presented early after chest pain onset, with a pre-specified cutoff value. The routine use of copeptin as an additional biomarker with conventional cTn assays for the early rule-out of MI is recommended by the 2020 ESC Guidelines (65).
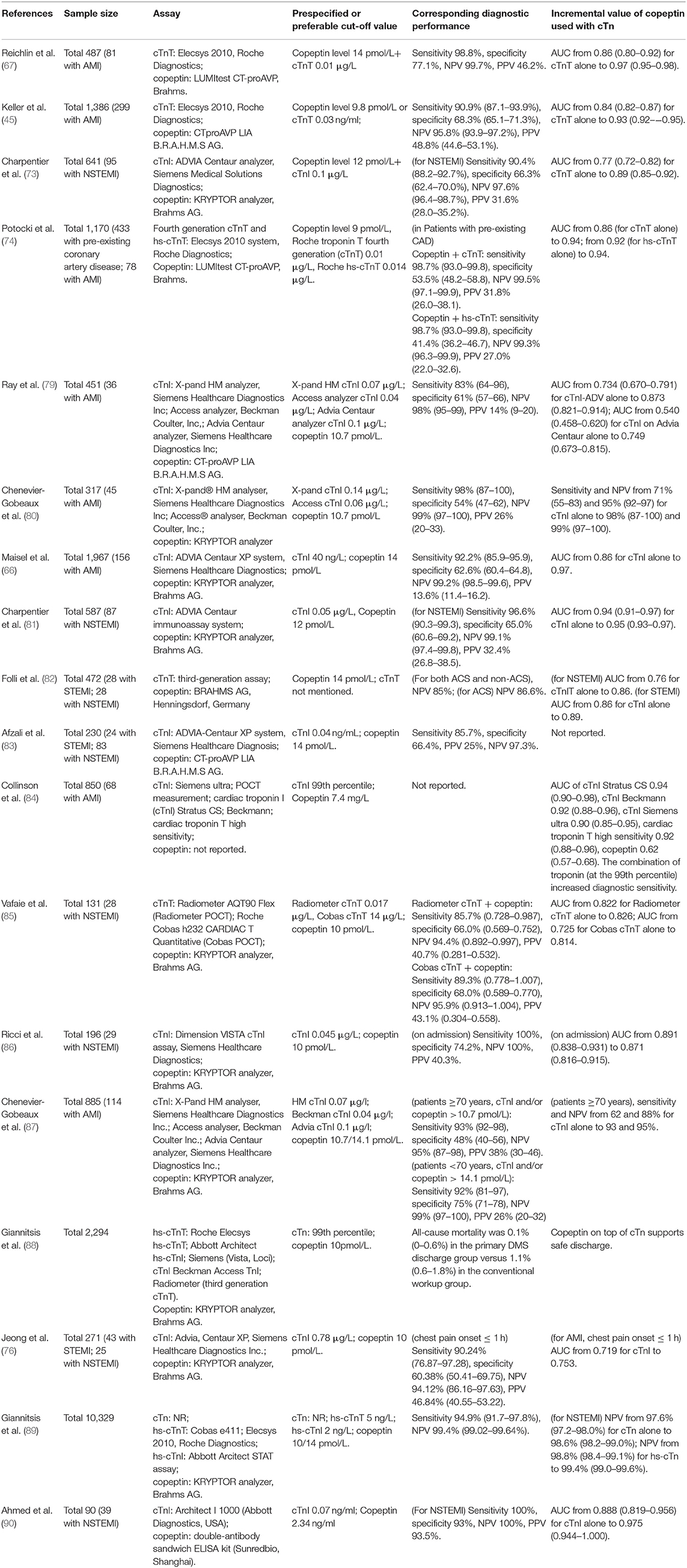
Table 2. Articles about the diagnostic performance of cTn (with or without hs-cTn) combined with copeptin measurement in the rule-out of AMI.
The Incremental Value of Copeptin Combined with hs-cTn
Although the combination of copeptin with conventional cTn provided significant benefits, the incremental value of copeptin when used with hs-cTn compared with hs-cTn alone remains unclear and inconsistent for an instant the rule-out of NSTEMI (75, 91–93). Several studies evaluated whether adding copeptin (with a pre-specified cut-off value) could increase the rule-out performance of hs-cTn (with a cut-off of 99th percentile of the healthy population) on admission with only single blood collection, and reported only numerically small or no incremental value (94–98). Therefore, it is suggested that copeptin could be effective only used with less sensitive conventional cTn assays, but not with hs-cTn (64, 65).
When the hs-cTn assay is available and well validated, patients with “very low” or limit of detection (LOD) level of hs-cTn (Roche hs-cTnT < 5 ng/L; Abbott hs-cTnI < 4 ng/L) on admission (0 h) could be eligible for “rule-out” and may be discharged if the ECG and/or clinical symptoms suggest a low risk of ACS (65). In order to compared the “very low” algorithms with combination of copeptin level assessment on admission, Restan et al. (99) recently reported that adding copeptin < 9 pmol/L did not increase the sensitivity of the rule-out provided by hs-cTnT < 5 ng/L or hs-cTnI < 4 ng/L in patients presenting > 3 h after chest pain onset (sensitivity 98.9% for hs-cTnT < 5 ng/L vs. 98.9% for hs-cTnT < 7 ng/L and copeptin < 9 pmol/L, NPV 99.6 vs. 99.7%; sensitivity 97.8% for hs-cTnI < 4 ng/L vs. 97.8% for hs-cTnI < 7 ng/L and copeptin < 9 pmol/L, NPV 99.5 vs. 99.5%). Interestingly, when analyzing patients presenting early (<3 h) from chest pain onset, higher sensitivity for the dual marker strategy was observed in one cohort. This is consistent with the guidelines in which the use of a 0 h hs-cTn-only approach is not recommended (65). The reason why the incremental value of copeptin is smaller when used with hs-cTn than with cTn may be that the highly sensitive assay has, to a large extent, overcome the sensitivity deficit of conventional cTn in clinical practice, recognizing the elevation of cTn in AMI patients earlier. Therefore, the added value brought by combining copeptin into hs-cTn is much less obvious.
A secondary analysis (100) from a multicenter study, including 1,439 patients, investigated whether a second copeptin measurement (at 1 h) could improve the rule-out and rule-in algorithm for patients with initially negative hs-cTn and copeptin results. It was found that 1 h-copeptin did not significantly increase the NPV [98.6% (95% CI 97.4–99.3%) vs. 98.6% (95% CI 97.3–99.3%)], but 1 h-hs-cTnT did [NPV 99.6% (95% CI 98.7–99.9%)]. Besides, in the intermediate-risk group (negative hs-cTnT but increased copeptin on admission), a similar finding was observed. These results extended and corroborated previous findings that the concentration of copeptin had already declined, whereas that of cTn kept rising in a large proportion of AMI patients presented to the ED. In addition, it is also suggested that if the purpose is to investigate the diagnostic performance of copeptin in early rule-out AMI at admission, blood samples must be drawn as early as possible after the onset of symptoms. Blood samples were drawn several hours after admission and after treatment initiation was unqualified for this purpose (101).
However, some studies have concluded that this dual marker strategy (combination of copeptin and hs-cTn) might provide higher eligibility or efficacy (quantified by the percentage of the overall cohort assigned to the rule-out zone) to rule out NSTEMI (89, 102, 103). Giannitsis et al. evaluated pooled data of 10,329 patients and concluded that the NPV for ruling out NSTEMI was high for the dual marker strategy (copeptin < 10 pmol/L and/or hs-cTn ≤ 14 ng/L) and single marker strategy (hs-cTnT < 5 ng/L) to be 99.0 and 99.2%, respectively, but the former had a 2.4-fold higher eligibility [64.6% (63.0–66.2%) vs. 27.9% (26.2–29.7%)]. Another prospective study enrolling 1,920 patients found that the NPV (reflecting safety) was very high and comparable in the LOD algorithm (hs-cTnT < 5 ng/L) and the dual marker strategy (copeptin < 9 pmol/L and/or hs-cTn ≤ 14 ng/L) [NPV 99.6% (98.6–99.9%) vs. 98.8% (97.9–99.4%)]. For efficacy, the dual marker strategy performed better than the LOD algorithm (allowed rule-out in 47.6% of patients vs. 25.8% of patients). Using the hs-cTnI assay had similar results (102). These studies corroborated the safety and effectiveness of the dual marker strategy using hs-cTn and copeptin. However, given that more non-MI patients might be ruled in on admission and the cost of running the copeptin measurement platform 24/7, health economic analysis using the dual marker strategy vs. the single marker strategy in patients with suspected AMI in ED should be conducted, and detailed practical guidelines are desirable. Table 3 shows articles on the diagnostic performance of hs-cTn combined with copeptin measurement in the rule-out of AMI.
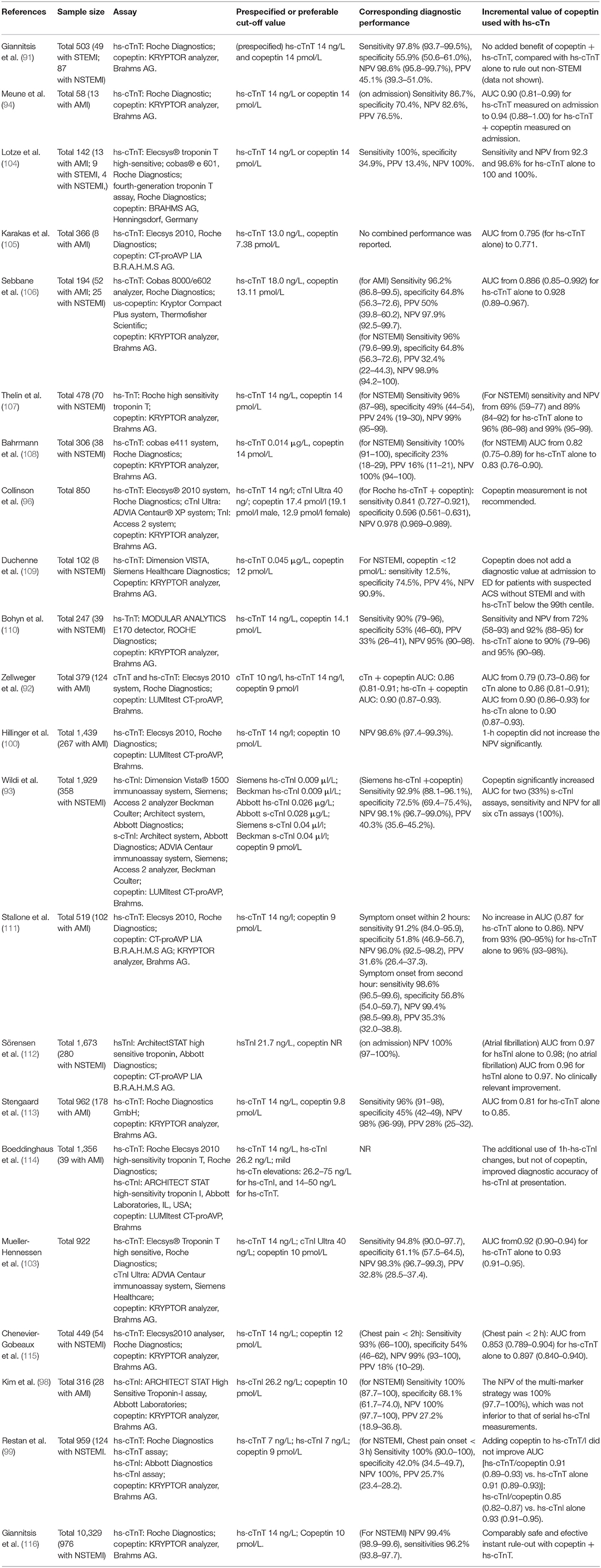
Table 3. Articles about the diagnostic performance of hs-cTn combined with copeptin measurement in the rule-out of AMI.
The Diagnostic Performance of Copeptin in Different Subgroups
The diagnostic performance of copeptin in different subgroups of the population was investigated further. A study on sex-based differences of copeptin combined with cTn for early rule-out of NSTEMI reported that men had a similar NPV (100%) as women [99.6% (95% CI 98.8–100%)] (145). Another study also concluded that the diagnostic performance (assessed by the AUC) of cTnT/hs-cTnT and copeptin performed, as well was in women as in men (75). Identifying NSTEMI in elderly patients is also a diagnostic challenge. A study has shown that an optimal copeptin cut-off value was 8.1 pmol/L in patients < 70 years and 10.7 pmol/L in patients > 70 years, and copeptin in combination with cTnI could also improve sensitivity and NPV for the diagnosis of NSTEMI in older patients (87). As for hs-cTn, the additional use of copeptin (>14 pmol/L) might help reliably rule-out NSTEMI (NPV 100%) in older populations presenting to the ED (108). Between different races (black population vs. Caucasian population), copeptin at a cut-off value of 14 pmol/L was reported to demonstrate an unequal diagnostic performance in ruling out AMI [NPV 98.0% (95% CI 96.2–99.1%) in the black population; 94.1% (95% CI 92.1–95.7%) in Caucasian population], which might provide more precise patient management (146).
Copeptin Increases the Performance of POCT Assays for cTn
Point-of-care testing (POCT) assays for cTn are important detection tools when commercial cTn or hs-cTn assays in laboratory platforms are unavailable, but the analytical sensitivity of POCT assays cannot fulfill this requirement. Vafaie et al. (85) found that the addition of copeptin increased the sensitivity of POCT assays (from 67.9 to 89.3% on Cobas and from 71.4 to 85.7% on Radiometer), indicating that copeptin could improve the diagnostic performance (sensitivity) of POCT assays, and suggested that copeptin combined with POCT for cTn might achieve a performance comparable to that of hs-cTn at admission. However, studies on the diagnostic value improvement of copeptin with POCT assays for cTn is still lacking and require further exploration and validation.
Caveats and Caution
Of note, there are several caveats and cautions when using copeptin to rapidly identify AMI. First, as a relatively novel biomarker, the optimal cut-off value of copeptin should be carefully investigated to reach a consensus, as assays for both copeptin and cTn have been improved to an increased sensitivity level. Many studies adopted a copeptin cut-off of 14 pmol/L (66, 67, 83, 95), mainly because it was used in the first publication (67). Keller et al. (45) compared the 95, 97.5, and 99 percentiles of the general population as the copeptin cut-off to evaluate the NPV and found the highest NPV was determined at a cut-off value of 9.8 pmol/L (95th percentile). Therefore, a lower cut-off value of 10 pmol/L should be considered to improve the NPV for the diagnosis of AMI, as recommended by the 2015 ESC guidelines (64, 77, 147).
Second, it is essential to realize that negative copeptin results do not indicate the absence of coronary artery disease, because the extent of copeptin release triggered by ischemia (such as unstable angina) was weaker than other acute stimuli, such as AMI (45, 67), and additional evidence, such as coronary angiography, is required for diagnosis (148). In addition, the copeptin/troponin ratio cannot predict the final infarct size or myocardial salvage index in patients with STEMI (149). Other available clinical check-ups and evidence, such as chest pain characteristics and ECGs, are indispensable for ruling out AMI, together with this dual marker strategy (62, 148, 150).
Third, as a non-specific biomarker, copeptin can be significantly elevated in many fluid disorders and stress-associated diseases (151–153). Elevated copeptin levels are present in approximately one in five patients with non-cardiac chest pain and are associated with aging, cardiac and non-cardiac comorbidities, and mortality (154). Therefore, in patients with suspected non-cardiac causes of acute chest pain (such as musculoskeletal pain, anxiety disorder, and gastroesophageal reflux), the increasing level of copeptin does not warrant any specific evidence of cardiac disorders and should be meticulously checked with further specific evaluations (148).
Copeptin to Discriminate Between Type 1 and 2 Myocardial Infarction
There are different subtypes of myocardial infarction (MI), the most frequent are type 1 MI (T1MI) (caused by atherothrombotic coronary artery disease) and type 2 MI (T2MI) (associated with a mismatch between oxygen supply and demand) (62). The differentiation between T1MI and T2MI is based on the results of angiography indicating plaque disruption, and on the causes of symptoms, such as arrhythmias and severe hypertension (155). Identification and discrimination between the two subtypes may aid in proper and specific treatment, but it remains an unmet need and challenge (65). A clinical trial conducted in 2017 developed a diagnostic model (including female sex, not having radiating chest pain, and a baseline hs-cTnI ≤ 40.8 ng/L) to predict T2MI, and only achieved an AUC of 0.71 (patients with the highest score of 3 had a 72% probability of T2MI, while 5% probability with a score of 0) (156).
In 2020, a retrospective study (157) analyzed 2,071 patients in the Copeptin Helps in the Early Detection of Patients with Acute Myocardial Infarction (CHOPIN) study and investigated the discrimination ability of several biomarkers, including cTnI, copeptin, and mid-regional pro-atrial natriuretic peptide (MR-pro-ANP). They found that patients with T1MI had higher levels of cTnI at presentation and with subsequent measurements, while copeptin levels were higher in T2MI at the fourth and fifth follow-up time points but not at earlier measurements. Combining all biomarkers (including copeptin) resulted in a similar accuracy to a model using clinical variables (including sex, race, atrial fibrillation, warfarin, and location of pain) and cTnI (0.854 vs. 0.884), while adding all biomarkers to the clinical model yielded the highest AUC (0.917). Although copeptin's ability to predict T2MI was weak, its addition to the model improved discrimination by both net reclassification improvement (NRI) [0.687 (95% CI 0.434–0.940)] and integrated discrimination improvement (IDI) [0.066 (95% CI 0.031–0.101)]. Another recent study (158), however, argued discriminating between T2MI and T1MIs could be directly improved by copeptin [AUC of hs-cTnI alone 0.74 vs. 0.81 after addition of copeptin evaluation, odds ratio (OR) of copeptin in multivariable logistic regression 1.97, p = 0.0016]. The difference between the two studies may be attributed to the adjudication of the final diagnosis: the former is based on cTnI and the latter is based on hs-cTnI, and a higher percentage of minor T2MIs might be identified with hs-cTnI.
Copeptin to Predicting Mortality and Adverse Outcome in AMI
Prompt assessment and identification of patients with AMI who are at risk of adverse outcomes are necessary for optimized care and resource allocation. Therefore, prognostic markers for predicting the mortality rate and adverse outcomes of MI are extensively favored. Nowadays, serial measurement of hs-cTn, as well as B-type natriuretic peptide (BNP) or N-terminal pro-B-type natriuretic peptide (NT-proBNP) concentration have been recommended for the assessment of prognosis (159, 160). Elevated hs-cTn levels were reported to predict a higher risk of death. However, the role of copeptin in the prognosis is less validated and has not been recommended.
Several studies have indicated that copeptin is a useful prognostic predictor for adverse outcomes in patients with ACS (83, 161–164), and the predictive performance for 180-day mortality was significantly better in combination with copeptin and cTnI than with cTnI alone (75). However, some controversial results have argued that there is no significant predictive ability of copeptin (alone or with cTn) for ACS outcomes (108, 165, 166). To obviate the influence of different study designs and limited sample sizes, a meta-analysis (167) was conducted to evaluate the prognostic value of copeptin in predicting mortality in ACS and found that the pooled sensitivity and specificity were 0.77 (95% CI 0.59–0.89) and 0.60 (95% CI 0.47–0.71), respectively, and the AUC was 0.73 (95% CI 0.69–0.77), indicating an acceptable performance for predicting mortality in ACS. Previous studies (166, 168, 169) have shown that a multiple-marker approach seems to provide more prognostic value for adverse outcomes in ACS. While there is a lack of evidence regarding to the incremental value of copeptin added to other multi-markers in ACS, further studies are needed to provide insight into copeptin as a prognostic marker.
For AMI patients who successfully underwent PCI, the prognostic value of copeptin was also studied, and it was reported that the increased copeptin levels were correlated with increased incidence of major adverse cardiac events (MACE) during long-term follow-up (median 30.1 months) in the participants (OR = 1.6) (170). For patients undergoing non-cardiac surgery, several studies have investigated the incremental value of copeptin in predicting MACE when added to established preoperative risk indices. Jarai et al. reported that a copeptin level >14 pmol/L was a significant independent predictor of perioperative and postoperative outcomes in patients undergoing vascular surgery (hazard ratio, HR = 2.842, p = 0.002) (171). Another study found that copeptin ≥ 9.6 pmol/L was associated with significantly higher rates of myocardial injury and improved risk stratification. However, current data are insufficient to provide results on the added predictive performance of copeptin for any of the outcomes, so further research is still required to improve the prognostic strategies in this group of patients (172).
Copeptin in Heart Failure
Heart failure (HF), resulting from an abnormality in cardiac structure or function, is one of the most common causes of hospitalization and mortality worldwide (173). HF is defined as “a complex clinical syndrome that results from any structural or functional impairment of ventricular filling or ejection of blood” according to the 2013 ACCF/AHA guideline (174). A wide range of etiologies can result in HF conditions, primarily including four underlying conditions: ischemic heart disease (IHD), chronic obstructive pulmonary disease, hypertensive heart disease, and rheumatic heart disease (175). IHD was the leading cause of death globally, accounting for 8.9 million all-age deaths in 2017 (1). IHD refers to an imbalance between myocardial energy state and coronary blood flow, which can occur under the following conditions: atherosclerosis, coronary microvascular dysfunction, inflammation, and vasospasm (176). Hypertensive heart disease is ascribed to chronic blood pressure overload, which exposes cardiac myocytes to higher hemodynamic stress and neurohormones, leading to left ventricular hypertrophy. Progressive hypertrophy and fibrotic changes ultimately result in diastolic heart failure (177).
HF can be classified into two categories based on systolic function: heart failure with reduced ejection fraction (HFrEF) and heart failure with preserved ejection fraction (HFpEF). HFrEF is defined as a clinical diagnosis of HF and left ventricular ejection fraction (LVEF) < 40%, whereas HFpEF refers to those with LVEF ≥ 50% (178). Patients with LVEF in the range of 40–49% represent a “gray area”, which is defined as HF with mid-range EF (HFmrEF). Compared with HFrEF, HFpEF patients are often older women with a history of hypertension and atrial fibrillation (179). Of note, the diagnosis of HFpEF is more challenging than that of HFrEF, because HFpEF generally do not have a dilated left ventricular, making additional examination and serum biomarker tests necessary.
The clinical syndromes and signs of HF are non-specific; therefore, careful history taking and physical examination are pivotal in the assessment of HF (180, 181). Currently, BNP, NT-proBNP, and MR-pro-ANP have been recommended and extensively accepted as biomarkers for the evaluation and differentiation of patients presenting in the ED or CCU/ICU with symptoms suggestive of HF (178, 182, 183). Patients with BNP < 100 pg/ml, NT-proBNP <300 pg/ml, and MR-proANP < 120 pg/ml can be excluded from HF diagnosis (178). The diagnosis of HFpEF is more difficult because other potential non-cardiac causes of symptoms suggestive of HF are supposed to be excluded. A recent meta-analysis evaluated the diagnostic accuracy of currently available biomarkers for chronic HFpEF and showed that the pooled sensitivities and specificities of BNP and NT-proBNP were 0.787 (95% CI 0.719–0.842) and 0.796 (95% CI 0.672–0.882) for BNP, and 0.696 (95% CI 0.599–0.779) and 0.882 (95% CI 0.778–0.941) for NT-proBNP, respectively (184). Although BNP and NT-proBNP remain the most reliable biomarkers for HFpEF diagnosis, it is worth noting that elevated levels of natriuretic peptides can be non-specific and caused by a series of cardiac and non-cardiac factors, including pulmonary embolism, myocarditis, and renal dysfunction (185, 186). Therefore, additional biomarkers and improved treatment strategies are required.
Vasopressin System in HF
Owing to a relatively well-established knowledge, the current treatment of HF is mainly targeted at the neurohormonal systems with a predominance of a blockade of adrenergic receptors and the renin-angiotensin-aldosterone system, such as angiotensin-converting enzyme inhibitors, beta-blockers, and angiotensin-II receptor antagonists (178). Overactivation of the vasopressin system in patients with HF has been largely neglected (187).
Patients with HF suffer greatly from water retention and volume overload, which is assumed to be attributed to the inappropriate activation of the vasopressin system. In addition, a non-osmotic pathway is also believed to regulate AVP release through intra-cardiac pressures, intra-arterial pressures, angiotensin II, pain, and adrenergic central nervous stimuli (25, 188). Significantly increased AVP levels have been reported in patients with HF (acute HF with hyponatremia and chronic HF with or without hyponatremia) and LV dysfunction (25, 189–194). Regarding the pharmacological value, vasopressin receptor antagonists can block the V2R in renal tubules to promote aquaresis or can block the V1aR in smooth blood vessels to inhibit vasoconstriction and cardiac remodeling (195). Tolvaptan, a highly selective vasopressin V2 receptor antagonist, may be used to treat patients with volume overload and resistant hyponatremia; however, it was found to have no effect on long-term mortality or HF-related morbidity in patients hospitalized with HF (196).
Clinical Value of Copeptin in HF
The performance of copeptin as a surrogate marker of AVP in risk stratification of patients with HF has been proposed and widely evaluated. Xu et al. found (197) that copeptin and NT-proBNP levels increased as NYHA grade increased in patients with HFrEF, but not in patients with HFpEF. In addition, increased copeptin levels in patients with advanced HF have also been reported to be associated with a reduced cardiac index (138). These results, together with other evaluations (119, 198), suggest the clinical value of risk stratification and disease severity of copeptin in patients with HF. Furthermore, in a prospective cohort study (143), individuals in the top quartile of copeptin had a significantly increased risk of developing HF (HR = 1.63, 95% CI 1.20–2.21) compared to the reference first quartile after multivariate adjustment for conventional risk factors, indicating that elevated copeptin was a predictor of HF development in older adults.
Currently, copeptin has also been demonstrated to be a relatively good predictor of mortality in patients presenting to the ED with acute dyspnea (187, 199). A cohort study (122) that enrolled 287 patients with acute dyspnea showed that the AUC of copeptin, NT-proBNP, and BNP to predict 30-day mortality was 0.83 (95% CI 0.76–0.90), 0.76 (95% CI 0.67–0.84) and 0.63 (95% CI−0.530.74), respectively. After adjusting for common cardiovascular risk factors and NT-proBNP levels, copeptin was the strongest independent predictor of short-term mortality [HR 3.88 (95% CI 1.94–7.77)]. For those with acute decompensated heart failure (ADHF), copeptin had an HR of 5.99 (95% CI 2.55–14.07) to independently predict short-term mortality.
In addition, for patients with HF, copeptin has been proposed as a potential predictor of outcome or mortality. Copeptin was reported to be associated with left ventricular dysfunction, volumes, remodeling, and clinical HF post-AMI, indicating that copeptin measurement might add up prognostic information (200). The first study of the prognostic performance of copeptin in HF was performed in 2006 by Stoiser et al., who investigated the ability of copeptin and BNP to predict death and re-hospitalization and found that copeptin served as an independent predictor that was superior to BNP in patients with advanced HF (multivariate analysis of combined endpoint: copeptin [chi(2) = 20] vs. BNP [chi(2) = 4.9)] (117). Similar conclusions have been drawn and the prognostic value of copeptin in patients with HF has been widely discussed and confirmed (125, 141, 201–203).
For patients with acute HF, a multicenter trial reported significantly increased the 90-day mortality, readmissions, and ED visits in patients with elevated copeptin levels, especially in those with hyponatremia (HR = 7.36) (125). Another study found that increased copeptin concentrations predicted mortality in acute HF (HR = 1.61) and acute exacerbation of chronic obstructive pulmonary disease (AECOPD) (HR = 1.72), and copeptin reclassified a significant proportion of patients into a more accurate risk stratification in acute HF [0.39 (95% CI 0.06–0.71)] and AECOPD [NRI 0.60 (95% CI 0.19–1.02)] (135). These findings indicated the reliable value of copeptin in the prediction and prognosis of patients with acute HF. For patients with chronic HF, a long-term observational study found that increased copeptin levels correlated with excess mortality irrespective of the clinical signs of disease severity (119). Copeptin has also been demonstrated to be a predictor of long-term mortality in patients with chronic HFrEF (132).
Furthermore, the combination of copeptin with other biomarkers (such as hs-cTnT and NT-proBNP) might improve the prognostic performance of patients with HF compared to a single marker (124, 127). In a prospective study (137), the capacity of mid-regional pro-adrenomedullin (MR-proADM), copeptin, and interleukin-6 were combined with conventional clinical markers to predict the 30-day mortality of patients with acute HF was investigated. The AUC of the clinical model plus copeptin and NT-proBNP was 0.75 (95% CI 0.67–0.83), which was better than the clinical model alone [AUC 0.67 (95% CI 0.58–0.76)]. In summary, copeptin, in combination with other biomarkers, might benefit the monitoring of disease severity and predict the prognosis of HF. Table 4 shows articles on the prognostic value of copeptin in prediction of the outcome of HF.
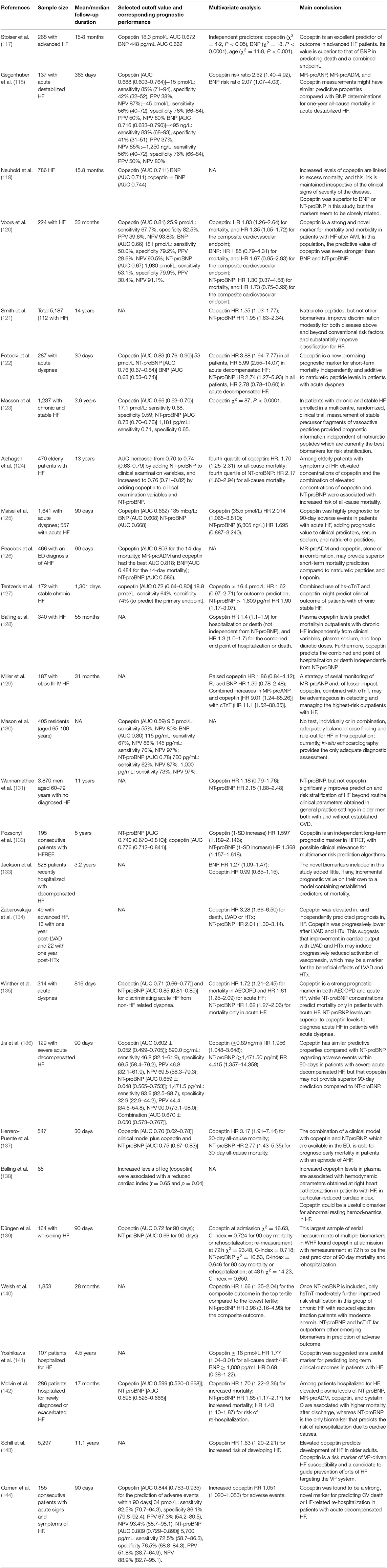
Table 4. Articles about the prognostic value of copeptin (alone or with BNP/NT-proBNP) in predicting the outcome of HF.
However, contradictory results that copeptin alone or in combination with other conventional biomarkers has limited clinical value cannot be ignored (130, 204). For instance, a randomized controlled trial found that the HR for the composite outcome for copeptin was 1.66 (95% CI 1.35–2.04), but when copeptin was included in a clinical prediction model (including NT-proBNP), there was no additional improvement for risk stratification in this group of patients with chronic HFrEF (140). The ineffectiveness of copeptin might be attributed to heterogeneity in study design, regional and individual differences, variation in biomarker detection, and cut-off values used.
In addition, it remains unclear whether copeptin can be used to monitor and guide medical therapy for patients with HF, and whether there exists a single cut-off level of copeptin for physicians to decide healthcare allocation in HF. Studies have investigated whether NT-proBNP and copeptin levels have the ability to optimize beta-blocker (BB) up-titration in patients with HF, but they came to inconsistent conclusions in terms of the performance of copeptin (205, 206), indicating the need for further well-designed studies with longer follow-up periods to elucidate the role of copeptin in guiding BB therapy. In terms of the therapeutic means targeting the vasopressin system, a prospective study compared a tolvaptan-based vs. furosemide-based diuretic regimen on short-term clinical responses in hyponatremic acute HF. Plasma copeptin levels increased in the tolvaptan group. The reason for this increase might be that tolvaptan increases serum sodium levels and urine output in HF (196). This phenomenon was consistent with another study (207), which reported that copeptin increased from baseline to week 3 (6.3 vs. 21.9 pmol/L) in tolvaptan-treated patients with autosomal dominant polycystic kidney disease. In addition, patients with higher baseline copeptin levels had a larger tolvaptan treatment effect, and those with larger changes in copeptin after 3 weeks had a better disease outcome (less kidney growth and eGFR decline). However, whether pre-treatment copeptin and treatment-induced change can predict the treatment efficacy of tolvaptan in patients with HF still lacks evidence and requires further investigation. In addition, the treatment effect of V1a antagonists or a combined V1a/V2-receptor blockade, as well as the role of copeptin as a therapy-guiding indicator in patients with HF should be explored in future clinical trials.
Copeptin in Stroke
Stroke is the second leading cause of CVD burden, resulting in over 6 million deaths in 2013 (2, 208). Stroke can be classified into two types, ischemic stroke (IS) and brain hemorrhage, including intracerebral hemorrhage (ICH) and subarachnoid hemorrhage (SAH) (209, 210). Early identification and prediction of adverse outcomes are crucial for optimized treatment and improved prognosis in patients with stroke.
Stroke conditions serve as acute stressors, activating the HPA axis, which regulates the release of CRH from the hypothalamus. CRH stimulates the release of ACTH from the anterior pituitary. Another stimulated hypothalamic hormone is AVP, which can interact with CRH and lead to the secretion of ACTH (211). It has been shown that cerebral ischemia may increase AVP levels in the plasma of patients with stroke (212, 213) and that V1R, but not V2R, is involved in the pathophysiology of secondary brain damage after focal cerebral ischemia (214). A meta-analysis revealed mean copeptin levels in different groups: stroke and non-stroke groups (19.8 ± 17.4 vs. 9.7 ± 6.6 pmol/L, respectively), good vs. poor outcome groups (12.0 ± 3.6 vs. 29.4 ± 14.5 pmol/L, respectively), and survive vs. non-survive stroke patients (13.4 ± 3.2 vs. 33.0 ± 12.3, respectively) (215), suggesting copeptin may guide the management of stroke patients. Interestingly, no significant difference was observed between the stroke group and stroke mimics (diseases with symptoms frequently seen in patients with stroke but caused by non-cerebrovascular pathogeneses) groups (216), indicating that copeptin could not discriminate between stroke and stroke mimics. The team also found no correlation between copeptin levels and the time from symptom onset, although copeptin levels quickly increased within the first minute after the event. Nonetheless, copeptin was independently associated with an increased risk of incident stroke in older men with diabetes [HR = 2.34 (95% CI 1.04–5.27)] (217), indicating that the vasopressin system might be a therapeutic target with effects on stroke risk in this population. Copeptin has been widely proposed as a prognostic marker for predicting the outcomes of patients with stroke.
Copeptin in Ischemic Stroke
IS is associated with detrimental and fatal conditions and contributed to 62.4% of all stroke incident cases in 2019 (210). Early risk assessments of disease severity and the prognosis are of great importance for optimized treatment and allocation of medical resources. The National Institutes of Health Stroke Scale (NIHSS) score, which ranges from 0 to 42, is used to predict mortality and functional outcomes in patients with stroke (218). In a prospective observational study, copeptin was assessed for the first time in patients with stroke for the prognostic value (219). It was demonstrated that those with unfavorable outcomes and non-survival had significantly higher copeptin levels on admission, and copeptin seemed to be an independent predictor of functional outcomes and mortality. In addition, adding copeptin to the NIHSS improved the prognostic accuracy for the functional outcome (AUC 0.75–0.79) and mortality (AUC 0.85–0.89). Other studies and meta-analyses also demonstrated that copeptin had a high prognostic performance in IS to predict adverse outcomes and mortality (41, 153, 220–222). Furthermore, in a prospective cohort study, copeptin was reported to improve the prognostic ability of the ABCD2 score for the prediction of IS in 31.2% of patients (223), requiring further studies to validate whether the addition of copeptin to the ABCD2 score can help refine the management of patients with the transient ischemic attack (TIA) and reduce healthcare costs. In 2019, De Marchis et al. (224) proposed a copeptin-based prognostic score (CoRisk score) encompassing copeptin levels, age, NIHSS score, and recanalization therapy. This CoRisk score correctly classified 75% of the patients, with an NRI between the calibrated CoRisk scores with and without copeptin of 46%. Further studies are needed to assess the prognostic accuracy of copeptin in combination with other biomarkers.
Recurrent vascular events after TIA and IS should be carefully monitored and predicted. Greisenegger et al. (225) investigated the value of copeptin in the prediction of long-term risk of vascular events after TIA and IS and reported that copeptin could predict recurrent vascular events (adjusted HR = 1.47), vascular-related death (HR = 0.85), all-cause mortality (HR = 1.75), and recurrent IS (HR = 1.22), particularly in patients with cardioembolic stroke (HR = 1.84). However, the lack of adjustment for additional cardiac indices largely limited the interpretation of the results. Therefore, whether copeptin can serve as an independent predictor of vascular events in patients with stroke requires further evaluation in a large population-based cohort.
Copeptin in Intracerebral Hemorrhage
ICH is the second most common cause of stroke after IS, but ICH accounts for more disability and mortality and a greater economic burden worldwide (226, 227). In patients with SAH, AVP is elevated in the plasma and cerebrospinal fluid, and V1aR is overexpressed in an experimental model of traumatic brain injury (213, 228, 229). Inhibition of V1aR has been reported to reduce the severity of SAH and prevent rebleeding by blunting the post-hemorrhagic hypertonic response in a rat model (230), indicating a potential approach to treat SAH.
Copeptin was elevated in patients with cerebral infarction (CI), ICH, and SAH compared to healthy controls, but it could not discriminate CI, ICH, and SAH from each other (231). In addition, a meta-analysis (232) in 2017 revealed significantly higher copeptin levels in ICH patients with poor prognosis than in survivors and that high copeptin levels were independently associated with a higher risk of mortality in patients with ICH. The World Federation of Neurological Surgeons subarachnoid hemorrhage scale (WFNS) is an accurate staging system for prognostic prediction after aneurysmal subarachnoid hemorrhage (aSAH) (233). The addition of plasma copeptin concentration significantly improved the predictive performance of WFNS scores for symptomatic cerebral vasospasm (AUC 0.848–0.921) and 6-month poor outcome after aSAH (AUC 0.867–0.940) (234). However, copeptin lacks specificity and can be easily influenced by other stressors, so it is suggested to investigate if it is an independent indicator of the prognosis of ICH and whether it can be used in combination with other biomarkers to achieve an optimal prognostic value in stroke and other life-threatening acute conditions.
Discussion
Provided that copeptin is secreted in equimolar amounts with AVP and correlates well with AVP release, it can serve as a promising and reliable surrogate of AVP, which is difficult to measure. Copeptin measurements have been shown to play essential roles in harmful CVDs. Copeptin has been reported to be an excellent tool for AMI rapid rule-out when combined with cTn evaluation in patients with potential AMI, as well as for risk stratification and outcome prediction in patients with AMI, HF, and stroke. However, additional larger, well-designed trials are still required to assess the incremental value of copeptin when added to conventional diagnostic or prognostic models of CVD and to evaluate the clinical benefits and applicability of copeptin measurement in routine practice and patient care. Whether copeptin can serve as a treatment indicator in therapies targeting the vasopressin system should be further explored and validated.
Author Contributions
DM wrote the first draft of the manuscript and prepared the figures. JC prepared the tables. XC and LQ conducted review of the manuscript. All authors contributed to the final revision of the manuscript and approved the submitted version.
Conflict of Interest
The authors declare that the research was conducted in the absence of any commercial or financial relationships that could be construed as a potential conflict of interest.
Publisher's Note
All claims expressed in this article are solely those of the authors and do not necessarily represent those of their affiliated organizations, or those of the publisher, the editors and the reviewers. Any product that may be evaluated in this article, or claim that may be made by its manufacturer, is not guaranteed or endorsed by the publisher.
References
1. Global, regional, and national age-sex-specific mortality for 282 causes of death in 195 countries and territories, 1980-2017: 1980-2017: a systematic analysis for the Global Burden of Disease Study 2017. Lancet. (2018) 392:1736–88. doi: 10.1016/s0140-6736(18)32203-7
2. Joseph P, Leong D, McKee M, Anand SS, Schwalm JD, Teo K, et al. Reducing the global burden of cardiovascular disease, part 1: the epidemiology and risk factors. Circ Res. (2017) 121:677–94. doi: 10.1161/CIRCRESAHA.117.308903
3. Teo KK, Ounpuu S, Hawken S, Pandey MR, Valentin V, Hunt D, et al. Tobacco use and risk of myocardial infarction in 52 countries in the INTERHEART study: a case-control study. Lancet. (2006) 368:647–58. doi: 10.1016/S0140-6736(06)69249-0
4. Lewington S, Clarke R, Qizilbash N, Peto R, Collins R. Age-specific relevance of usual blood pressure to vascular mortality: a meta-analysis of individual data for one million adults in 61 prospective studies. Lancet. (2002) 360:1903–13. doi: 10.1016/S0140-6736(02)11911-8
5. Wright JT, Jr., Williamson JD, Whelton PK, Snyder JK, Sink KM, et al. A randomized trial of intensive versus standard blood-pressure control. N Engl J Med. (2015) 373:2103–16. doi: 10.1056/NEJMoa1511939
6. McQueen MJ, Hawken S, Wang X, Ounpuu S, Sniderman A, Probstfield J, et al. Lipids, lipoproteins, and apolipoproteins as risk markers of myocardial infarction in 52 countries (the INTERHEART study): a case-control study. Lancet. (2008) 372:224–33. doi: 10.1016/S0140-6736(08)61076-4
7. Holwerda DA. A glycopeptide from the posterior lobe of pig pituitaries. I. Isolation and characterization. Eur J Biochem. (1972) 28:334–9. doi: 10.1111/j.1432-1033.1972.tb01918.x
8. Mu D, Ma C, Cheng J, Zou Y, Qiu L, Cheng X. Copeptin in fluid disorders and stress. Clin Chim Acta. (2022) 529:46–60. doi: 10.1016/j.cca.2022.02.002
9. Morgenthaler NG, Struck J, Alonso C, Bergmann A. Assay for the measurement of copeptin, a stable peptide derived from the precursor of vasopressin. Clin Chem. (2006) 52:112–9. doi: 10.1373/clinchem.2005.060038
10. Robertson GL, Mahr EA, Athar S, Sinha T. Development and clinical application of a new method for the radioimmunoassay of arginine vasopressin in human plasma. J Clin Invest. (1973) 52:2340–52. doi: 10.1172/JCI107423
11. Fenske WK, Schnyder I, Koch G, Walti C, Pfister M, Kopp P, et al. Release and decay kinetics of copeptin vs AVP in response to osmotic alterations in healthy volunteers. J Clin Endocrinol Metab. (2018) 103:505–13. doi: 10.1210/jc.2017-01891
12. Jochberger S, Morgenthaler NG, Mayr VD, Luckner G, Wenzel V, Ulmer H, et al. Copeptin and arginine vasopressin concentrations in critically ill patients. J Clin Endocrinol Metab. (2006) 91:4381–6. doi: 10.1210/jc.2005-2830
13. Baranowska B, Kochanowski J. Copeptin - a new diagnostic and prognostic biomarker in neurological and cardiovascular diseases. Neuro Endocrinol Lett. (2019) 40:207–14. Available online at: https://pubmed.ncbi.nlm.nih.gov/32112544/
14. Lipinski MJ, Escarcega RO, D'Ascenzo F, Magalhaes MA, Baker NC, Torguson R, et al. A systematic review and collaborative meta-analysis to determine the incremental value of copeptin for rapid rule-out of acute myocardial infarction. Am J Cardiol. (2014) 113:1581–91. doi: 10.1016/j.amjcard.2014.01.436
15. Morawiec B, Kawecki D. Copeptin: a new marker in cardiology. J Cardiovasc Med. (2013) 14:19–25. doi: 10.2459/JCM.0b013e3283590d59
16. Seidah NG, Benjannet S, Chrétien M. The complete sequence of a novel human pituitary glycopeptide homologous to pig posterior pituitary glycopeptide. Biochem Biophys Res Commun. (1981) 100:901–7. doi: 10.1016/S0006-291X(81)80258-6
17. Land H, Schütz G, Schmale H, Richter D. Nucleotide sequence of cloned cDNA encoding bovine arginine vasopressin-neurophysin II precursor. Nature. (1982) 295:299–303. doi: 10.1038/295299a0
18. Levy B, Chauvet MT, Chauvet J, Acher R. Ontogeny of bovine neurohypophysial hormone precursors. II. Foetal copeptin, the third domain of the vasopressin precursor. Int J Peptide Prot Res. (1986) 27:320–4. doi: 10.1111/j.1399-3011.1986.tb01827.x
19. Land H, Grez M, Ruppert S, Schmale H, Rehbein M, Richter D, et al. Deduced amino acid sequence from the bovine oxytocin-neurophysin I precursor cDNA. Nature. (1983) 302:342–4. doi: 10.1038/302342a0
20. Riddell DC, Mallonee R, Phillips JA, Parks JS, Sexton LA, Hamerton JL. Chromosomal assignment of human sequences encoding arginine vasopressin-neurophysin II and growth hormone releasing factor. Somatic Cell Mol Genet. (1985) 11:189–95. doi: 10.1007/BF01534707
22. Sachs H, Takabatake Y. Evidence for a precursor in vasopressin biosynthesis. Endocrinology. (1964) 75:943–8. doi: 10.1210/endo-75-6-943
23. Vandesande F, Dierickx K. Identification of the vasopressin producing and of the oxytocin producing neurons in the hypothalamic magnocellular neurosecretroy system of the rat. Cell Tissue Res. (1975) 164:153–62. doi: 10.1007/BF00218970
24. Sklar AH, Schrier RW. Central nervous system mediators of vasopressin release. Physiol Rev. (1983) 63:1243–80. doi: 10.1152/physrev.1983.63.4.1243
25. Schrier RW, Berl T, Anderson RJ. Osmotic and nonosmotic control of vasopressin release. Am J Physiol. (1979) 236:F321–F32. doi: 10.1152/ajprenal.1979.236.4.F321
26. Acher R, Chauvet J, Rouille Y. Dynamic processing of neuropeptides: sequential conformation shaping of neurohypophysial preprohormones during intraneuronal secretory transport. J Mol Neurosci. (2002) 18:223–8. doi: 10.1385/JMN:18:3:223
27. Birnbaumer M. Vasopressin receptors. Trends Endocrinol Metab. (2000) 11:406–10. doi: 10.1016/S1043-2760(00)00304-0
28. Jard S. Vasopressin: mechanisms of receptor activation. Prog Brain Res. (1983) 60:383–94. doi: 10.1016/S0079-6123(08)64405-2
29. Ostrowski NL, Young WS, 3rd, Knepper MA, Lolait SJ. Expression of vasopressin V1a and V2 receptor messenger ribonucleic acid in the liver and kidney of embryonic, developing, and adult rats. Endocrinology. (1993) 133:1849–59. doi: 10.1210/endo.133.4.8404628
30. Natochin YV, Golosova DV. Vasopressin receptor subtypes and renal sodium transport. Vitam Horm. (2020) 113:239–58. doi: 10.1016/bs.vh.2019.08.013
31. Tagawa T, Imaizumi T, Endo T, Shiramoto M, Hirooka Y, Ando S, et al. Vasodilatory effect of arginine vasopressin is mediated by nitric oxide in human forearm vessels. J Clin Invest. (1993) 92:1483–90. doi: 10.1172/JCI116726
32. Deen PM, Verdijk MA, Knoers NV, Wieringa B, Monnens LA, van Os CH, et al. Requirement of human renal water channel aquaporin-2 for vasopressin-dependent concentration of urine. Science. (1994) 264:92–5. doi: 10.1126/science.8140421
33. Moeller HB, Fuglsang CH, Fenton RA. Renal aquaporins and water balance disorders. Best Pract Res Clin Endocrinol Metab. (2016) 30:277–88. doi: 10.1016/j.beem.2016.02.012
34. Bahnsen U, Oosting P, Swaab DF, Nahke P, Richter D, Schmale H. A missense mutation in the vasopressin-neurophysin precursor gene cosegregates with human autosomal dominant neurohypophyseal diabetes insipidus. EMBO J. (1992) 11:19–23. doi: 10.1002/j.1460-2075.1992.tb05022.x
35. Nagy G, Mulchahey JJ, Smyth DG, Neill JD. The glycopeptide moiety of vasopressin-neurophysin precursor is neurohypophysial prolactin releasing factor. Biochem Biophys Res Commun. (1988) 151:524–9. doi: 10.1016/0006-291X(88)90625-0
36. Hyde JF, Ben-Jonathan N, North WG. The vasopressin-associated glycopeptide is not a prolactin-releasing factor: studies with lactating brattleboro rats. Endocrinology. (1989) 125:35–40. doi: 10.1210/endo-125-1-35
37. Barat C, Simpson L, Breslow E. Properties of human vasopressin precursor constructs: inefficient monomer folding in the absence of copeptin as a potential contributor to diabetes insipidus. Biochemistry. (2004) 43:8191–203. doi: 10.1021/bi0400094
38. Szinnai G, Morgenthaler NG, Berneis K, Struck J, Müller B, Keller U, et al. Changes in plasma copeptin, the c-terminal portion of arginine vasopressin during water deprivation and excess in healthy subjects. J Clin Endocrinol Metab. (2007) 92:3973–8. doi: 10.1210/jc.2007-0232
39. Balanescu S, Kopp P, Gaskill MB, Morgenthaler NG, Schindler C, Rutishauser J. Correlation of plasma copeptin and vasopressin concentrations in hypo-, iso-, and hyperosmolar States. J Clin Endocrinol Metab. (2011) 96:1046–52. doi: 10.1210/jc.2010-2499
40. Morgenthaler NG, Müller B, Struck J, Bergmann A, Redl H, Christ-Crain M. Copeptin, a stable peptide of the arginine vasopressin precursor, is elevated in hemorrhagic and septic shock. Shock. (2007) 28:219–26. doi: 10.1097/SHK.0b013e318033e5da
41. Choi KS, Kim HJ, Chun HJ, Kim JM, Yi HJ, Cheong JH, et al. Prognostic role of copeptin after stroke: a systematic review and meta-analysis of observational studies. Sci Rep. (2015) 5:11665. doi: 10.1038/srep11665
42. Bellos I, Pergialiotis V, Papapanagiotou A, Loutradis D, Daskalakis G. Association between serum copeptin levels and preeclampsia risk: A meta-analysis. Eur J Obstet Gynecol Reprod Biol. (2020) 250:66–73. doi: 10.1016/j.ejogrb.2020.04.051
43. Katan M, Morgenthaler N, Widmer I, Puder JJ, König C, Müller B, et al. Copeptin, a stable peptide derived from the vasopressin precursor, correlates with the individual stress level. Neuro Endocrinol Lett. (2008) 29:341–6. Available online at: https://pubmed.ncbi.nlm.nih.gov/18580851/
44. Roussel R, Fezeu L, Marre M, Velho G, Fumeron F, Jungers P, et al. Comparison between copeptin and vasopressin in a population from the community and in people with chronic kidney disease. J Clin Endocrinol Metab. (2014) 99:4656–63. doi: 10.1210/jc.2014-2295
45. Keller T, Tzikas S, Zeller T, Czyz E, Lillpopp L, Ojeda FM, et al. Copeptin improves early diagnosis of acute myocardial infarction. J Am College Cardiol. (2010) 55:2096–106. doi: 10.1016/j.jacc.2010.01.029
46. Bhandari SS, Loke I, Davies JE, Squire IB, Struck J, Ng LL. Gender and renal function influence plasma levels of copeptin in healthy individuals. Clin Sci. (2009) 116:257–63. doi: 10.1042/CS20080140
47. Beglinger S, Drewe J, Christ-Crain M. The circadian rhythm of copeptin, the C-terminal portion of arginine vasopressin. J Biomark. (2017) 2017:4737082. doi: 10.1155/2017/4737082
48. Walti C, Siegenthaler J, Christ-Crain M. Copeptin levels are independent of ingested nutrient type after standardised meal administration–the CoMEAL study. Biomarkers. (2014) 19:557–62. doi: 10.3109/1354750X.2014.940504
49. Brooks E, Bachmeier C, Vorster J, Sorbello J, Peer F, Chikani V, et al. Copeptin is increased by nausea and vomiting during hypertonic saline infusion in healthy individuals. Clin Endocrinol. (2021) 94:820–6. doi: 10.1111/cen.14417
50. Schoen T, Hohmann EM, Van Der Lely S, Aeschbacher S, Reusser A, Risch M, et al. Plasma copeptin levels and ambulatory blood pressure characteristics in healthy adults. J Hypertens. (2015) 33:1571–9. doi: 10.1097/HJH.0000000000000610
51. Preibisz JJ, Sealey JE, Laragh JH, Cody RJ, Weksler BB. Plasma and platelet vasopressin in essential hypertension and congestive heart failure. Hypertension. (1983) 5:I129–38. doi: 10.1161/01.HYP.5.2_Pt_2.I129
52. Mindt S, Andrade-Barazarte H, Tokhi U, Ludtka C, Neumaier M, Hänggi D. Immunoluminometric assay for copeptin measurement in cerebrospinal fluid: technical aspects and pilot study. Clin Chim Acta. (2019) 490:181–5. doi: 10.1016/j.cca.2018.09.005
53. Anderson JL, Morrow DA. Acute myocardial infarction. New Engl J Med. (2017) 376:2053–64. doi: 10.1056/NEJMra1606915
54. Amsterdam EA, Wenger NK, Brindis RG, Casey DE, Ganiats TG, Holmes DR, et al. 2014 AHA/ACC Guideline for the Management of Patients with Non-ST-Elevation Acute Coronary Syndromes: a report of the American College of Cardiology/American Heart Association Task Force on Practice Guidelines. J Am College Cardiol. (2014) 64:e139-e228. doi: 10.1016/j.jacc.2014.09.016
55. Mockel M, Searle J, Muller R, Slagman A, Storchmann H, Oestereich P, et al. Chief complaints in medical emergencies: do they relate to underlying disease and outcome? The Charité Emergency Medicine Study (CHARITEM). Eur J Emerg Med. (2013) 20:103–8. doi: 10.1097/MEJ.0b013e328351e609
56. Adams JE, 3rd, Abendschein DR, Jaffe AS. Biochemical markers of myocardial injury. Is MB creatine kinase the choice for the 1990s? Circulation. (1993) 88:750–63. doi: 10.1161/01.CIR.88.2.750
57. Weil BR, Young RF, Shen X, Suzuki G, Qu J, Malhotra S, et al. Brief myocardial ischemia produces cardiac troponin I release and focal myocyte apoptosis in the absence of pathological infarction in swine. JACC Basic Transl Sci. (2017) 2:105–14. doi: 10.1016/j.jacbts.2017.01.006
58. Thygesen K, Mair J, Giannitsis E, Mueller C, Lindahl B, Blankenberg S, et al. How to use high-sensitivity cardiac troponins in acute cardiac care. Eur Heart J. (2012) 33:2252–7. doi: 10.1093/eurheartj/ehs154
59. du Fay de Lavallaz J, Prepoudis A, Wendebourg MJ, Kesenheimer E, Kyburz D, Daikeler T, et al. Skeletal muscle disorders: a non-cardiac source of cardiac troponin T. Circulation. (2022) doi: 10.1161/CIRCULATIONAHA.121.058489
60. Lazar DR, Lazar FL, Homorodean C, Cainap C, Focsan M, Cainap S, et al. High-sensitivity troponin: a review on characteristics, assessment, and clinical implications. Dis Markers. (2022) 2022:9713326. doi: 10.1155/2022/9713326
61. Gu YL, Voors AA, Zijlstra F, Hillege HL, Struck J, Masson S, et al. Comparison of the temporal release pattern of copeptin with conventional biomarkers in acute myocardial infarction. Clin Res Cardiol. (2011) 100:1069–76. doi: 10.1007/s00392-011-0343-y
62. Thygesen K, Alpert JS, Jaffe AS, Chaitman BR, Bax JJ, Morrow DA, et al. Fourth universal definition of myocardial infarction (2018). Circulation. (2018) 138:e618–51. doi: 10.1161/CIR.0000000000000617
63. Apple FS, Collinson PO. Analytical characteristics of high-sensitivity cardiac troponin assays. Clin Chem. (2012) 58:54–61. doi: 10.1373/clinchem.2011.165795
64. Roffi M, Patrono C, Collet JP, Mueller C, Valgimigli M, Andreotti F, et al. 2015 ESC guidelines for the management of acute coronary syndromes in patients presenting without persistent ST-segment elevation: Task Force for the Management of Acute Coronary Syndromes in Patients Presenting without Persistent ST-Segment Elevation of the European Society of Cardiology (ESC). Eur Heart J. (2016) 37:267–315. doi: 10.1093/eurheartj/ehv320
65. Collet J-P, Thiele H, Barbato E, Barthélémy O, Bauersachs J, Bhatt DL, et al. 2020 ESC guidelines for the management of acute coronary syndromes in patients presenting without persistent ST-segment elevation. Eur Heart J. (2021) 42:1289–367. doi: 10.15829/1560-4071-2021-4418
66. Maisel A, Mueller C, Neath SX, Christenson RH, Morgenthaler NG, McCord J, et al. Copeptin helps in the early detection of patients with acute myocardial infarction: primary results of the CHOPIN trial (Copeptin Helps in the early detection Of Patients with acute myocardial INfarction). J Am Coll Cardiol. (2013) 62:150–60. doi: 10.1016/j.jacc.2013.04.011
67. Reichlin T, Hochholzer W, Stelzig C, Laule K, Freidank H, Morgenthaler NG, et al. Incremental value of copeptin for rapid rule out of acute myocardial infarction. J Am Coll Cardiol. (2009) 54:60–8. doi: 10.1016/j.jacc.2009.01.076
68. Slagman A, Searle J, Müller C, Möckel M. Temporal release pattern of copeptin and troponin T in patients with suspected acute coronary syndrome and spontaneous acute myocardial infarction. Clin Chem. (2015) 61:1273–82. doi: 10.1373/clinchem.2015.240580
69. Arnadottir A, Pedersen S, Bo Hasselbalch R, Goetze JP, Friis-Hansen LJ, Bloch-Munster AM, et al. Temporal release of high-sensitivity cardiac troponin T and I and copeptin after brief induced coronary artery balloon occlusion in humans. Circulation. (2021) 143:1095–104. doi: 10.1161/CIRCULATIONAHA.120.046574
70. Christ-Crain M. Vasopressin and copeptin in health and disease. Rev Endocr Metab Disord. (2019) 20:283–94. doi: 10.1007/s11154-019-09509-9
71. Raskovalova T, Twerenbold R, Collinson PO, Keller T, Bouvaist H, Folli C, et al. Diagnostic accuracy of combined cardiac troponin and copeptin assessment for early rule-out of myocardial infarction: a systematic review and meta-analysis. Eur Heart J Acute Cardiovasc Care. (2014) 3:18–27. doi: 10.1177/2048872613514015
72. Ay MO, Erenler AK, Dogan T, Yetim M. Diagnostic value of copeptin in acute myocardial infarction. Eur Rev Med Pharmacol Sci. (2017) 21:1576–82. Available online at: https://www.europeanreview.org/article/12526
73. Charpentier S, Maupas-Schwalm F, Cournot M, Elbaz M, Botella JM, Lauque D. Combination of copeptin and troponin assays to rapidly rule out non-ST elevation myocardial infarction in the emergency department. Acad Emerg Med. (2012) 19:517–24. doi: 10.1111/j.1553-2712.2012.01350.x
74. Potocki M, Reichlin T, Thalmann S, Zellweger C, Twerenbold R, Reiter M, et al. Diagnostic and prognostic impact of copeptin and high-sensitivity cardiac troponin T in patients with pre-existing coronary artery disease and suspected acute myocardial infarction. Heart. (2012) 98:558–65. doi: 10.1136/heartjnl-2011-301269
75. Balmelli C, Meune C, Twerenbold R, Reichlin T, Rieder S, Drexler B, et al. Comparison of the performances of cardiac troponins, including sensitive assays, and copeptin in the diagnostic of acute myocardial infarction and long-term prognosis between women and men. Am Heart J. (2013) 166:30–7. doi: 10.1016/j.ahj.2013.03.014
76. Jeong JH, Seo YH, Ahn JY, Kim KH, Seo JY, Chun KY, et al. Performance of copeptin for early diagnosis of acute myocardial infarction in an emergency department setting. Ann Lab Med. (2020) 40:7–14. doi: 10.3343/alm.2020.40.1.7
77. Mockel M, Searle J, Hamm C, Slagman A, Blankenberg S, Huber K, et al. Early discharge using single cardiac troponin and copeptin testing in patients with suspected acute coronary syndrome (ACS): a randomized, controlled clinical process study. Eur Heart J. (2015) 36:369–76. doi: 10.1093/eurheartj/ehu178
78. Reinhold T, Giannitsis E, Mockel M, Frankenstein L, Vafaie M, Vollert JO, et al. Cost analysis of early discharge using combined copeptin/cardiac troponin testing versus serial cardiac troponin testing in patients with suspected acute coronary syndrome. PLoS ONE. (2018) 13:e0202133. doi: 10.1371/journal.pone.0202133
79. Ray P, Charpentier S, Chenevier-Gobeaux C, Reichlin T, Twerenbold R, Claessens YE, et al. Combined copeptin and troponin to rule out myocardial infarction in patients with chest pain and a history of coronary artery disease. Am J Emerg Med. (2012) 30:440–8. doi: 10.1016/j.ajem.2011.12.008
80. Chenevier-Gobeaux C, Freund Y, Claessens YE, Guérin S, Bonnet P, Doumenc B, et al. Copeptin for rapid rule out of acute myocardial infarction in emergency department. Int J Cardiol. (2013) 166:198–204. doi: 10.1016/j.ijcard.2011.10.098
81. Charpentier S, Lepage B, Maupas-Schwalm F, Cinq-Frais C, Bichard-Bréaud M, Botella JM, et al. Copeptin improves the diagnostic performance of sensitive troponin I-Ultra but cannot rapidly rule out non-ST-elevation myocardial infarction at presentation to an emergency department. Ann Emerg Med. (2013) 61:549–58.e1. doi: 10.1016/j.annemergmed.2012.12.018
82. Folli C, Consonni D, Spessot M, Salvini L, Velati M, Ranzani G, et al. Diagnostic role of copeptin in patients presenting with chest pain in the emergency room. Eur J Intern Med. (2013) 24:189–93. doi: 10.1016/j.ejim.2012.09.006
83. Afzali D, Erren M, Pavenstädt H-J, Vollert JO, Hertel S, Waltenberger J, et al. Impact of copeptin on diagnosis, risk stratification, and intermediate-term prognosis of acute coronary syndromes. Clin Res Cardiol. (2013) 102:755–63. doi: 10.1007/s00392-013-0583-0
84. Collinson P, Gaze D, Goodacre S. Comparison of contemporary troponin assays with the novel biomarkers, heart fatty acid binding protein and copeptin, for the early confirmation or exclusion of myocardial infarction in patients presenting to the emergency department with chest pain. Heart. (2014) 100:140–5. doi: 10.1136/heartjnl-2013-304716
85. Vafaie M, Biener M, Mueller M, Abu Sharar H, Hartmann O, Hertel S, et al. Addition of copeptin improves diagnostic performance of point-of-care testing (POCT) for cardiac troponin T in early rule-out of myocardial infarction - A pilot study. Int J Cardiol. (2015) 198:26–30. doi: 10.1016/j.ijcard.2015.06.122
86. Ricci F, Di Scala R, Massacesi C, Di Nicola M, Cremonese G, De Pace D, et al. Ultra-sensitive copeptin and cardiac troponin in diagnosing non-ST-segment elevation acute coronary syndromes–the COPACS study. Am J Med. (2016) 129:105–14. doi: 10.1016/j.amjmed.2015.06.033
87. Chenevier-Gobeaux C, Charpentier S, Meune C, Claessens YE, Lavieuville M, Ray P. Copeptin improves the sensitivity of cardiac troponin in patients 70 years or older, but not enough to rule out myocardial infarction at emergency department presentation. Eur J Emerg Med. (2017) 24:142–8. doi: 10.1097/MEJ.0000000000000316
88. Giannitsis E, Clifford P, Slagman A, Ruedelstein R, Liebetrau C, Hamm C, et al. Multicentre cross-sectional observational registry to monitor the safety of early discharge after rule-out of acute myocardial infarction by copeptin and troponin: the pro-core registry. BMJ Open. (2019) 9:e028311. doi: 10.1136/bmjopen-2018-028311
89. Giannitsis E, Slagman A, Hamm CW, Gehrig S, Vollert JO, Huber K. Copeptin combined with either non-high sensitivity or high sensitivity cardiac troponin for instant rule-out of suspected non-ST segment elevation myocardial infarction. Biomarkers. (2020) 25:649–58. doi: 10.1080/1354750X.2020.1833084
90. Ahmed TAN, Johny JS, Abdel-Malek MY, Fouad DA. The additive value of copeptin for early diagnosis and prognosis of acute coronary syndromes. Am J Emerg Med. (2021) 50:413–21. doi: 10.1016/j.ajem.2021.08.069
91. Giannitsis E, Kehayova T, Vafaie M, Katus HA. Combined testing of high-sensitivity troponin T and copeptin on presentation at prespecified cutoffs improves rapid rule-out of non-ST-segment elevation myocardial infarction. Clin Chem. (2011) 57:1452–1455. doi: 10.1373/clinchem.2010.161265
92. Zellweger C, Wildi K, Twerenbold R, Reichlin T, Naduvilekoot A, Neuhaus JD, et al. Use of copeptin and high-sensitive cardiac troponin T for diagnosis and prognosis in patients with diabetes mellitus and suspected acute myocardial infarction. Int J Cardiol. (2015) 190:190–7. doi: 10.1016/j.ijcard.2015.04.134
93. Wildi K, Zellweger C, Twerenbold R, Jaeger C, Reichlin T, Haaf P, et al. Incremental value of copeptin to highly sensitive cardiac troponin I for rapid rule-out of myocardial infarction. Int J Cardiol. (2015) 190:170–6. doi: 10.1016/j.ijcard.2015.04.133
94. Meune C, Zuily S, Wahbi K, Claessens YE, Weber S, Chenevier-Gobeaux C. Combination of copeptin and high-sensitivity cardiac troponin T assay in unstable angina and non-ST-segment elevation myocardial infarction: a pilot study. Arch Cardiovasc Dis. (2011) 104:4–10. doi: 10.1016/j.acvd.2010.11.002
95. Eggers KM, Venge P, Lindahl B. High-sensitive cardiac troponin T outperforms novel diagnostic biomarkers in patients with acute chest pain. Clin Chim Acta. (2012) 413:1135–40. doi: 10.1016/j.cca.2012.03.011
96. Collinson PO, Gaze DC, Thokala P, Goodacre S. Randomised assessment of treatment using panel assay of cardiac markers–contemporary biomarker evaluation (RATPAC CBE). Health Technol Assess. (2013) 17:v–vi, 1–122. doi: 10.3310/hta17150
97. Alquézar A, Santaló M, Rizzi M, Gich I, Grau M, Sionis A, et al. Combined high-sensitivity copeptin and troponin T evaluation for the diagnosis of non-ST elevation acute coronary syndrome in the emergency department. Emergencias. (2017) 29:237–44. Available online at: http://emergencias.portalsemes.org/descargar/evaluacinde-una-estrategia-diagnstica-combinada-con-copeptinaytroponina-t-ultrasensibles-en-el-infarto-de-miocardio-sinelevacin-delsegmento-st-en-los-servicios-de-urgencias/
98. Kim KS, Suh GJ, Song SH, Jung YS, Kim T, Shin SM, et al. Copeptin with high-sensitivity troponin at presentation is not inferior to serial troponin measurements for ruling out acute myocardial infarction. Clin Exp Emerg Med. (2020) 7:35–42. doi: 10.15441/ceem.19.013
99. Restan IZ, Sanchez AY, Steiro OT, Lopez-Ayala P, Tjora HL, Langørgen J, et al. Adding stress biomarkers to high-sensitivity cardiac troponin for rapid non-ST-elevation myocardial infarction rule-out protocols. Eur Heart J Acute Cardiovasc Care. (2022) 11:201–12. doi: 10.1093/ehjacc/zuab124
100. Hillinger P, Twerenbold R, Jaeger C, Wildi K, Reichlin T, Rubini Gimenez M, et al. Optimizing early rule-out strategies for acute myocardial infarction: utility of 1-hour copeptin. Clin Chem. (2015) 61:1466–74. doi: 10.1373/clinchem.2015.242743
101. Möckel M. Copeptin adds to high-sensitivity troponin T in rapid rule out of acute myocardial infarction. Clin Chem. (2012) 58:306–7. doi: 10.1373/clinchem.2011.171058
102. Wildi KS, Twerenbold R, Rubini Gimenez M, Reichlin T, Puelacher C, Hillinger P, et al. Direct comparison of the safety and efficacy of two rule-out strategies for acute myocardial infarction: undetectable levels of hs-troponin versus copeptin in combination with troponin [Conference Abstract]. Eur Heart J. (2015) 36:173–4. doi: 10.1093/eurheartj/ehv399
103. Mueller-Hennessen M, Lindahl B, Giannitsis E, Vafaie M, Biener M, Haushofer AC, et al. Combined testing of copeptin and high-sensitivity cardiac troponin T at presentation in comparison to other algorithms for rapid rule-out of acute myocardial infarction. Int J Cardiol. (2019) 276:261–7. doi: 10.1016/j.ijcard.2018.10.084
104. Lotze U, Lemm H, Heyer A, Müller K. Combined determination of highly sensitive troponin T and copeptin for early exclusion of acute myocardial infarction: first experience in an emergency department of a general hospital. Vasc Health Risk Manag. (2011) 7:509–15. doi: 10.2147/VHRM.S21753
105. Karakas M, Januzzi JL Jr, Meyer J, Lee H, Schlett CL, Truong QA, et al. Copeptin does not add diagnostic information to high-sensitivity troponin T in low- to intermediate-risk patients with acute chest pain: results from the rule out myocardial infarction by computed tomography (ROMICAT) study. Clin Chem. (2011) 57:1137–45. doi: 10.1373/clinchem.2010.160192
106. Sebbane M, Lefebvre S, Kuster N, Jreige R, Jacques E, Badiou S, et al. Early rule out of acute myocardial infarction in ED patients: value of combined high-sensitivity cardiac troponin T and ultrasensitive copeptin assays at admission. Am J Emerg Med. (2013) 31:1302–8. doi: 10.1016/j.ajem.2013.04.033
107. Thelin J, Borna C, Erlinge D, Öhlin B. The combination of high sensitivity troponin T and copeptin facilitates early rule-out of ACS: a prospective observational study. BMC Cardiovasc Disord. (2013) 13:42. doi: 10.1186/1471-2261-13-42
108. Bahrmann P, Bahrmann A, Breithardt O-A, Daniel WG, Christ M, Sieber CC, et al. Additional diagnostic and prognostic value of copeptin ultra-sensitive for diagnosis of non-ST-elevation myocardial infarction in older patients presenting to the emergency department. Clin Chem Lab Med. (2013) 51:1307–19. doi: 10.1515/cclm-2012-0401
109. Duchenne J, Mestres S, Dublanchet N, Combaret N, Marceau G, Caumon L, et al. Diagnostic accuracy of copeptin sensitivity and specificity in patients with suspected non-ST-elevation myocardial infarction with troponin I below the 99th centile at presentation. BMJ Open. (2014) 4:e004449. doi: 10.1136/bmjopen-2013-004449
110. Bohyn E, Dubie E, Lebrun C, Jund J, Beaune G, Lesage P, et al. Expeditious exclusion of acute coronary syndrome diagnosis by combined measurements of copeptin, high-sensitivity troponin, and GRACE score. Am J Emerg Med. (2014) 32:293–6. doi: 10.1016/j.ajem.2013.11.043
111. Stallone F, Schoenenberger AW, Puelacher C, Rubini Gimenez M, Walz B, Naduvilekoot Devasia A, et al. Incremental value of copeptin in suspected acute myocardial infarction very early after symptom onset. Eur Heart J Acute Cardiovasc Care. (2016) 5:407–15. doi: 10.1177/2048872616641289
112. Sörensen NA, Shah AS, Ojeda FM, Peitsmeyer P, Zeller T, Keller T, et al. High-sensitivity troponin and novel biomarkers for the early diagnosis of non-ST-segment elevation myocardial infarction in patients with atrial fibrillation. Eur Heart J Acute Cardiovasc Care. (2016) 5:419–27. doi: 10.1177/2048872615611108
113. Stengaard C, Sørensen JT, Ladefoged SA, Lassen JF, Rasmussen MB, Pedersen CK, et al. The potential of optimizing prehospital triage of patients with suspected acute myocardial infarction using high-sensitivity cardiac troponin T and copeptin. Biomarkers. (2017) 22:351–60. doi: 10.1080/1354750X.2016.1265008
114. Boeddinghaus J, Reichlin T, Nestelberger T, Twerenbold R, Meili Y, Wildi K, et al. Early diagnosis of acute myocardial infarction in patients with mild elevations of cardiac troponin. Clin Res Cardiol. (2017) 106:457–67. doi: 10.1007/s00392-016-1075-9
115. Chenevier-Gobeaux C, Sebbane M, Meune C, Lefebvre S, Dupuy AM, Lefèvre G, et al. Is high-sensitivity troponin, alone or in combination with copeptin, sensitive enough for ruling out NSTEMI in very early presenters at admission? A post hoc analysis performed in emergency departments. BMJ Open. (2019) 9:e023994. doi: 10.1136/bmjopen-2018-023994
116. Giannitsis E, Huber K, Hamm CW, Möckel M. Instant rule-out of suspected non-ST-segment elevation myocardial infarction using high-sensitivity cardiac troponin T with copeptin versus a single low high-sensitivity cardiac troponin T: findings from a large pooled individual data analysis on 10,329 patients. Clin Res Cardiol. (2021) 110:194–9. doi: 10.1007/s00392-020-01712-y
117. Stoiser B, Mörtl D, Hülsmann M, Berger R, Struck J, Morgenthaler NG, et al. Copeptin, a fragment of the vasopressin precursor, as a novel predictor of outcome in heart failure. Eur J Clin Investig. (2006) 36:771–8. doi: 10.1111/j.1365-2362.2006.01724.x
118. Gegenhuber A, Struck J, Dieplinger B, Poelz W, Pacher R, Morgenthaler NG, et al. Comparative evaluation of B-type natriuretic peptide, mid-regional pro-A-type natriuretic peptide, mid-regional pro-adrenomedullin, and Copeptin to predict 1-year mortality in patients with acute destabilized heart failure. J Card Fail. (2007) 13:42–9. doi: 10.1016/j.cardfail.2006.09.004
119. Neuhold S, Huelsmann M, Strunk G, Stoiser B, Struck J, Morgenthaler NG, et al. Comparison of copeptin, B-type natriuretic peptide, and amino-terminal pro-B-type natriuretic peptide in patients with chronic heart failure: prediction of death at different stages of the disease. J Am Coll Cardiol. (2008) 52:266–72. doi: 10.1016/j.jacc.2008.03.050
120. Voors AA, von Haehling S, Anker SD, Hillege HL, Struck J, Hartmann O, et al. C-terminal provasopressin (copeptin) is a strong prognostic marker in patients with heart failure after an acute myocardial infarction: results from the OPTIMAAL study. Eur Heart J. (2009) 30:1187–94. doi: 10.1093/eurheartj/ehp098
121. Smith JG, Newton-Cheh C, Almgren P, Struck J, Morgenthaler NG, Bergmann A, et al. Assessment of conventional cardiovascular risk factors and multiple biomarkers for the prediction of incident heart failure and atrial fibrillation. J Am Coll Cardiol. (2010) 56:1712–9. doi: 10.1016/j.jacc.2010.05.049
122. Potocki M, Breidthardt T, Mueller A, Reichlin T, Socrates T, Arenja N, et al. Copeptin and risk stratification in patients with acute dyspnea. Crit Care. (2010) 14:R213. doi: 10.1186/cc9336
123. Masson S, Latini R, Carbonieri E, Moretti L, Rossi MG, Ciricugno S, et al. The predictive value of stable precursor fragments of vasoactive peptides in patients with chronic heart failure: data from the GISSI-heart failure (GISSI-HF) trial. Eur J Heart Fail. (2010) 12:338–47. doi: 10.1093/eurjhf/hfp206
124. Alehagen U, Dahlström U, Rehfeld JF, Goetze JP. Association of copeptin and N-terminal proBNP concentrations with risk of cardiovascular death in older patients with symptoms of heart failure. JAMA. (2011) 305:2088–95. doi: 10.1001/jama.2011.666
125. Maisel A, Xue Y, Shah K, Mueller C, Nowak R, Peacock WF, et al. Increased 90-day mortality in patients with acute heart failure with elevated copeptin: secondary results from the biomarkers in acute heart failure (BACH) study. Circ Heart Fail. (2011) 4:613–20. doi: 10.1161/CIRCHEARTFAILURE.110.960096
126. Peacock WF, Nowak R, Christenson R, DiSomma S, Neath SX, Hartmann O, et al. Short-term mortality risk in emergency department acute heart failure. Acad Emerg Med. (2011) 18:947–58. doi: 10.1111/j.1553-2712.2011.01150.x
127. Tentzeris I, Jarai R, Farhan S, Perkmann T, Schwarz MA, Jakl G, et al. Complementary role of copeptin and high-sensitivity troponin in predicting outcome in patients with stable chronic heart failure. Eur J Heart Fail. (2011) 13:726–33. doi: 10.1093/eurjhf/hfr049
128. Balling L, Kistorp C, Schou M, Egstrup M, Gustafsson I, Goetze JP, et al. Plasma copeptin levels and prediction of outcome in heart failure outpatients: relation to hyponatremia and loop diuretic doses. J Card Fail. (2012) 18:351–8. doi: 10.1016/j.cardfail.2012.01.019
129. Miller WL, Hartman KA, Grill DE, Struck J, Bergmann A, Jaffe AS. Serial measurements of midregion proANP and copeptin in ambulatory patients with heart failure: incremental prognostic value of novel biomarkers in heart failure. Heart. (2012) 98:389–94. doi: 10.1136/heartjnl-2011-300823
130. Mason JM, Hancock HC, Close H, Murphy JJ, Fuat A, de Belder M, et al. Utility of biomarkers in the differential diagnosis of heart failure in older people: findings from the heart failure in care homes (HFinCH) diagnostic accuracy study. PLoS ONE. (2013) 8:e53560. doi: 10.1371/journal.pone.0053560
131. Wannamethee SG, Welsh P, Whincup PH, Lennon L, Papacosta O, Sattar N. N-terminal pro brain natriuretic peptide but not copeptin improves prediction of heart failure over other routine clinical risk parameters in older men with and without cardiovascular disease: population-based study. Eur J Heart Fail. (2014) 16:25–32. doi: 10.1093/eurjhf/hft124
132. Pozsonyi Z, Förhécz Z, Gombos T, Karádi I, Jánoskuti L, Prohászka Z. Copeptin (C-terminal pro arginine-vasopressin) is an independent long-term prognostic marker in heart failure with reduced ejection fraction. Heart Lung Circ. (2015) 24:359–67. doi: 10.1016/j.hlc.2014.10.008
133. Jackson CE, Haig C, Welsh P, Dalzell JR, Tsorlalis IK, McConnachie A, et al. The incremental prognostic and clinical value of multiple novel biomarkers in heart failure. Eur J Heart Fail. (2016) 18:1491–8. doi: 10.1002/ejhf.543
134. Zabarovskaja S, Hage C, Gabrielsen A, Mellbin L, Lund LH. Copeptin in heart failure, post-left ventricular assist device and post-heart transplantation. Heart Lung Circ. (2017) 26:143–9. doi: 10.1016/j.hlc.2016.05.119
135. Winther JA, Brynildsen J, Høiseth AD, Strand H, Følling I, Christensen G, et al. Prognostic and diagnostic significance of copeptin in acute exacerbation of chronic obstructive pulmonary disease and acute heart failure: data from the ACE 2 study. Respir Res. (2017) 18:184. doi: 10.1186/s12931-017-0665-z
136. Jia J, Chang GL, Qin S, Chen J, He WY, Lu K, et al. Comparative evaluation of copeptin and NT-proBNP in patients with severe acute decompensated heart failure, and prediction of adverse events in a 90-day follow-up period: a prospective clinical observation trial. Exp Ther Med. (2017) 13:1554–60. doi: 10.3892/etm.2017.4111
137. Herrero-Puente P, Prieto-García B, García-García M, Jacob J, Martín-Sánchez FJ, Pascual-Figal D, et al. Predictive capacity of a multimarker strategy to determine short-term mortality in patients attending a hospital emergency Department for acute heart failure. BIO-EAHFE study. Clin Chim Acta. (2017) 466:22–30. doi: 10.1016/j.cca.2017.01.003
138. Balling L, Goetze JP, Jung MH, Rossing K, Boesgaard S, Gustafsson F. Copeptin levels and invasive hemodynamics in patients with advanced heart failure. Biomark Med. (2018) 12:861–70. doi: 10.2217/bmm-2017-0439
139. Düngen HD, Tscholl V, Obradovic D, Radenovic S, Matic D, Musial Bright L, et al. Prognostic performance of serial in-hospital measurements of copeptin and multiple novel biomarkers among patients with worsening heart failure: results from the MOLITOR study. ESC Heart Fail. (2018) 5:288–96. doi: 10.1002/ehf2.12231
140. Welsh P, Kou L, Yu C, Anand I, van Veldhuisen DJ, Maggioni AP, et al. Prognostic importance of emerging cardiac, inflammatory, and renal biomarkers in chronic heart failure patients with reduced ejection fraction and anaemia: RED-HF study. Eur J Heart Fail. (2018) 20:268–277. doi: 10.1002/ejhf.988
141. Yoshikawa Y, Shiomi H, Kuwahara K, Sowa N, Yaku H, Yamashita Y, et al. Utility of copeptin for predicting long-term clinical outcomes in patients with heart failure. J Cardiol. (2019) 73:379–85. doi: 10.1016/j.jjcc.2018.11.008
142. Molvin J, Jujic A, Bachus E, Gallo W, Tasevska-Dinevska G, Holm H, et al. Cardiovascular biomarkers predict post-discharge re-hospitalization risk and mortality among Swedish heart failure patients. ESC Heart Fail. (2019) 6:992–9. doi: 10.1002/ehf2.12486
143. Schill F, Timpka S, Nilsson PM, Melander O, Enhörning S. Copeptin as a predictive marker of incident heart failure. ESC Heart Fail. (2021) 8:3180–8. doi: 10.1002/ehf2.13439
144. Ozmen C, Deveci OS, Tepe O, Yesildas C, Ünal I, Yildiz I, et al. Prognostic performance of copeptin among patients with acute decompensated heart failure. Acta Cardiol. (2021) 76:842–51. doi: 10.1080/00015385.2020.1786624
145. Vargas KG, Tajsic M, Latsuzbaia A, Bastian S, Andric T, Kassem M, et al. Gender-based differences of copeptin alone or combined with troponin for early rule-out of non-ST-elevation myocardial infarction. Am J Emerg Med. (2021) 45:248–53. doi: 10.1016/j.ajem.2020.08.053
146. Beri N, Daniels LB, Jaffe A, Mueller C, Anand I, Peacock WF, et al. Copeptin to rule out myocardial infarction in Blacks versus Caucasians. Eur Heart J Acute Cardiovasc Care. (2019) 8:395–403. doi: 10.1177/2048872618772500
147. Möckel M, Searle J. Copeptin-marker of acute myocardial infarction. Curr Atherosc Rep. (2014) 16:421. doi: 10.1007/s11883-014-0421-5
148. Mueller C, Möckel M, Giannitsis E, Huber K, Mair J, Plebani M, et al. Use of copeptin for rapid rule-out of acute myocardial infarction. Eur Heart J Acute Cardiovasc Care. (2018) 7:570–576. doi: 10.1177/2048872617710791
149. Árnadóttir Á, Schoos M, Lønborg J, Ahtarovski K, Kelbæk H, Helqvist S, et al. Can copeptin and troponin T ratio predict final infarct size and myocardial salvage index in patients with ST-elevation myocardial infarction: a sub-study of the DANAMI-3 trial. Clin Biochem. (2018) 59:37–42. doi: 10.1016/j.clinbiochem.2018.06.012
150. Rubini Gimenez M, Reiter M, Twerenbold R, Reichlin T, Wildi K, Haaf P, et al. Sex-specific chest pain characteristics in the early diagnosis of acute myocardial infarction. JAMA Intern Med. (2014) 174:241–9. doi: 10.1001/jamainternmed.2013.12199
151. Fenske W, Stork S, Blechschmidt A, Maier SG, Morgenthaler NG, Allolio B. Copeptin in the differential diagnosis of hyponatremia. J Clin Endocrinol Metab. (2009) 94:123–9. doi: 10.1210/jc.2008-1426
152. Struck J, Morgenthaler NG, Bergmann A. Copeptin, a stable peptide derived from the vasopressin precursor, is elevated in serum of sepsis patients. Peptides. (2005) 26:2500–4. doi: 10.1016/j.peptides.2005.04.019
153. Xu Q, Tian Y, Peng H, Li H. Copeptin as a biomarker for prediction of prognosis of acute ischemic stroke and transient ischemic attack: a meta-analysis. Hypertens Res. (2017) 40:465–71. doi: 10.1038/hr.2016.165
154. Stallone F, Twerenbold R, Wildi K, Reichlin T, Rubini Gimenez M, Haaf P, et al. Prevalence, characteristics and outcome of non-cardiac chest pain and elevated copeptin levels. Heart. (2014) 100:1708–14. doi: 10.1136/heartjnl-2014-305583
155. Thygesen K, Alpert JS, Jaffe AS, Simoons ML, Chaitman BR, White HD, et al. Third universal definition of myocardial infarction. Eur Heart J. (2012) 33:2551–67. doi: 10.1093/eurheartj/ehs184
156. Neumann JT, Sörensen NA, Rübsamen N, Ojeda F, Renné T, Qaderi V, et al. Discrimination of patients with type 2 myocardial infarction. Eur Heart J. (2017) 38:3514–20. doi: 10.1093/eurheartj/ehx457
157. Horiuchi Y, Wettersten N, Patel MP, Mueller C, Neath SX, Christenson RH, et al., Anand I, Wu AHB, Ebmeyer S, Jaffe AS, Peacock WF, Maisel A. Biomarkers enhance discrimination and prognosis of type 2 myocardial infarction. Circulation. (2020) 142:1532–44. doi: 10.1161/CIRCULATIONAHA.120.046682
158. Neumann JT, Weimann J, Sorensen NA, Hartikainen TS, Haller PM, Lehmacher J, et al. A biomarker model to distinguish types of myocardial infarction and injury. J Am Coll Cardiol. (2021) 78:781–90. doi: 10.1016/j.jacc.2021.06.027
159. Mueller C. Biomarkers and acute coronary syndromes: an update. Eur Heart J. (2014) 35:552–6. doi: 10.1093/eurheartj/eht530
160. Thygesen K, Mair J, Mueller C, Huber K, Weber M, Plebani M, et al. Recommendations for the use of natriuretic peptides in acute cardiac care: a position statement from the study group on biomarkers in cardiology of the ESC working group on acute cardiac care. Eur Heart J. (2012) 33:2001–6. doi: 10.1093/eurheartj/ehq509
161. O'Malley RG, Bonaca MP, Scirica BM, Murphy SA, Jarolim P, Sabatine MS, et al. Prognostic performance of multiple biomarkers in patients with non-ST-segment elevation acute coronary syndrome: analysis from the MERLIN-TIMI 36 trial (metabolic efficiency with ranolazine for less ischemia in non-ST-elevation acute coronary syndromes-thrombolysis in myocardial infarction 36). J Am Coll Cardiol. (2014) 63:1644–53. doi: 10.1016/j.jacc.2013.12.034
162. Khan SQ, Dhillon OS, O'Brien RJ, Struck J, Quinn PA, Morgenthaler NG, et al. C-terminal provasopressin (copeptin) as a novel and prognostic marker in acute myocardial infarction: leicester acute myocardial infarction peptide (LAMP) study. Circulation. (2007) 115:2103–10. doi: 10.1161/CIRCULATIONAHA.106.685503
163. Morawiec B, Kawecki D, Przywara-Chowaniec B, Opara M, Muzyk P, Ho L, et al. Copeptin as a prognostic marker in acute chest pain and suspected acute coronary syndrome. Dis Mark. (2018) 2018:6597387. doi: 10.1155/2018/6597387
164. Waldsperger H, Biener M, Stoyanov KM, Vafaie M, Katus HA, Giannitsis E, et al. Prognostic value of elevated copeptin and high-sensitivity cardiac troponin t in patients with and without acute coronary syndrome: the ConTrACS study. J Clin Med. (2020) 9:3627. doi: 10.3390/jcm9113627
165. Llorens P, Sánchez M, Herrero P, Martín-Sánchez FJ, Piñera P, Miró O. The utility of copeptin in the emergency department for non-ST-elevation myocardial infarction rapid rule out: COPED-MIRRO study. Eur J Emerg Med. (2014) 21:220–9. doi: 10.1097/MEJ.0b013e3283632f8b
166. O'Donoghue ML, Morrow DA, Cannon CP, Jarolim P, Desai NR, Sherwood MW, et al. Multimarker risk stratification in patients with acute myocardial infarction. J Am Heart Assoc. (2016) 5:e002586. doi: 10.1161/JAHA.115.002586
167. Lu J, Wang S, He G, Wang Y. Prognostic value of copeptin in patients with acute coronary syndrome: a systematic review and meta-analysis. PLoS ONE. (2020) 15:e0238288. doi: 10.1371/journal.pone.0238288
168. Sabatine MS, Morrow DA, de Lemos JA, Gibson CM, Murphy SA, Rifai N, et al. Multimarker approach to risk stratification in non-ST elevation acute coronary syndromes: simultaneous assessment of troponin I, C-reactive protein, and B-type natriuretic peptide. Circulation. (2002) 105:1760–1763. doi: 10.1161/01.CIR.0000015464.18023.0A
169. Tello-Montoliu A, Marín F, Roldán V, Mainar L, López MT, Sogorb F, et al. A multimarker risk stratification approach to non-ST elevation acute coronary syndrome: implications of troponin T, CRP, NT pro-BNP and fibrin D-dimer levels. J Intern Med. (2007) 262:651–8. doi: 10.1111/j.1365-2796.2007.01871.x
170. Choi HJ, Kim MC, Sim DS, Hong YJ, Kim JH, Jeong MH, et al. Serum copeptin levels predict clinical outcomes after successful percutaneous coronary intervention in patients with acute myocardial infarction. Ann Lab Med. (2018) 38:538–44. doi: 10.3343/alm.2018.38.6.538
171. Jarai R, Mahla E, Perkmann T, Jarai R, Archan S, Tentzeris I, et al. Usefulness of pre-operative copeptin concentrations to predict post-operative outcome after major vascular surgery. Am J Cardiol. (2011) 108:1188–95. doi: 10.1016/j.amjcard.2011.06.024
172. Vernooij LM, van Klei WA, Moons KG, Takada T, van Waes J, Damen JA. The comparative and added prognostic value of biomarkers to the revised cardiac risk index for preoperative prediction of major adverse cardiac events and all-cause mortality in patients who undergo noncardiac surgery. Cochrane Database Syst Rev. (2021) 12:Cd013139. doi: 10.1002/14651858.CD013139.pub2
173. Groenewegen A, Rutten FH, Mosterd A, Hoes AW. Epidemiology of heart failure. Eur J Heart Fail. (2020) 22:1342–56. doi: 10.1002/ejhf.1858
174. Yancy CW, Jessup M, Bozkurt B, Butler J, Casey DE Jr, Drazner MH, et al. 2013 ACCF/AHA guideline for the management of heart failure: a report of the American College of Cardiology Foundation/American Heart Association Task Force on Practice Guidelines. J Am Coll Cardiol. (2013) 62:e147–239. doi: 10.1161/CIR.0b013e31829e8776
175. Ziaeian B, Fonarow GC. Epidemiology and aetiology of heart failure. Nat Rev Cardiol. (2016) 13:368–78. doi: 10.1038/nrcardio.2016.25
176. Severino P, D'Amato A, Pucci M, Infusino F, Adamo F, Birtolo LI, et al. Ischemic heart disease pathophysiology paradigms overview: from plaque activation to microvascular dysfunction. Int J Mol Sci. (2020) 21:8118. doi: 10.3390/ijms21218118
177. Slivnick J, Lampert BC. Hypertension and heart failure. Heart Fail Clin. (2019) 15:531–541. doi: 10.1016/j.hfc.2019.06.007
178. Ponikowski P, Voors AA, Anker SD, Bueno H, Cleland JG, Coats AJ, et al. 2016 ESC guidelines for the diagnosis treatment of acute chronic heart failure: the task force for the diagnosis treatment of acute chronic heart failure of the European Society of Cardiology (ESC). Developed with the special contribution of the Heart Failure Association (HFA) of the ESC. Eur J Heart Fail. (2016) 18:891–975. doi: 10.1002/ejhf.592
179. Owan TE, Hodge DO, Herges RM, Jacobsen SJ, Roger VL, Redfield MM. Trends in prevalence and outcome of heart failure with preserved ejection fraction. N Engl J Med. (2006) 355:251–9. doi: 10.1056/NEJMoa052256
180. McMurray JJV, Adamopoulos S, Anker SD, Auricchio A, Böhm M, Dickstein K, et al. ESC guidelines for the diagnosis and treatment of acute and chronic heart failure 2012: the task force for the diagnosis and treatment of acute and chronic heart failure 2012 of the European Society of Cardiology. Developed in collaboration with the Heart Failure Association (HFA) of the ESC. Eur J Heart Fail. (2012) 14:803–69. doi: 10.1093/eurjhf/hfs105
181. Katz AM, Rolett EL. Heart failure: when form fails to follow function. Eur Heart J. (2016) 37:449–54. doi: 10.1093/eurheartj/ehv548
182. Tsutamoto T, Wada A, Maeda K, Hisanaga T, Mabuchi N, Hayashi M, et al. Plasma brain natriuretic peptide level as a biochemical marker of morbidity and mortality in patients with asymptomatic or minimally symptomatic left ventricular dysfunction. Comparison with plasma angiotensin II and endothelin-1. Eur Heart J. (1999) 20:1799–807. doi: 10.1053/euhj.1999.1746
183. Hunt SA, Baker DW, Chin MH, Cinquegrani MP, Feldman AM, Francis GS, et al. ACC/AHA guidelines for the evaluation and management of chronic heart failure in the adult: executive summary a report of the American College of Cardiology/American Heart Association Task Force on practice guidelines (committee to revise the 1995 guidelines for the evaluation and management of heart failure): developed in collaboration with the international society for heart and lung transplantation; endorsed by the Heart Failure Society of America. Circulation. (2001) 104:2996–3007. doi: 10.1161/hc4901.102568
184. Chen H, Chhor M, Rayner BS, McGrath K, McClements L. Evaluation of the diagnostic accuracy of current biomarkers in heart failure with preserved ejection fraction: a systematic review and meta-analysis. Arch Cardiovasc Dis. (2021) 114:793–804. doi: 10.1016/j.acvd.2021.10.007
185. Zois NE, Bartels ED, Hunter I, Kousholt BS, Olsen LH, Goetze JP. Natriuretic peptides in cardiometabolic regulation and disease. Nat Rev Cardiol. (2014) 11:403–12. doi: 10.1038/nrcardio.2014.64
186. Gupta DK, Wang TJ. Natriuretic peptides and cardiometabolic health. Circ J. (2015) 79:1647–55. doi: 10.1253/circj.CJ-15-0589
187. Balling L, Gustafsson F. Copeptin in heart failure. Adv Clin Chem. (2016) 73:29–64. doi: 10.1016/bs.acc.2015.10.006
188. Finley JJt, Konstam MA, Udelson JE. Arginine vasopressin antagonists for the treatment of heart failure and hyponatremia. Circulation. (2008) 118:410–21. doi: 10.1161/CIRCULATIONAHA.108.765289
189. Udelson JE, Smith WB, Hendrix GH, Painchaud CA, Ghazzi M, Thomas I, et al. Acute hemodynamic effects of conivaptan, a dual V(1A) and V vasopressin receptor antagonist, in patients with advanced heart failure. Circulation. (2001) 104:2417–23. doi: 10.1161/hc4501.099313
190. Goldsmith SR, Francis GS, Cowley AW, Levine TB, Cohn JN. Increased plasma arginine vasopressin levels in patients with congestive heart failure. J Am Coll Cardiol. (1983) 1:1385–90. doi: 10.1016/S0735-1097(83)80040-0
191. Creager MA, Faxon DP, Cutler SS, Kohlmann O, Ryan TJ, Gavras H. Contribution of vasopressin to vasoconstriction in patients with congestive heart failure: comparison with the renin-angiotensin system and the sympathetic nervous system. J Am Coll Cardiol. (1986) 7:758–65. doi: 10.1016/S0735-1097(86)80333-3
192. Riegger GA, Kochsiek K. Vasopressin, renin and norepinephrine levels before and after captopril administration in patients with congestive heart failure due to idiopathic dilated cardiomyopathy. Am J Cardiol. (1986) 58:300–3. doi: 10.1016/0002-9149(86)90066-4
193. Francis GS, Benedict C, Johnstone DE, Kirlin PC, Nicklas J, Liang CS, et al. Comparison of neuroendocrine activation in patients with left ventricular dysfunction with and without congestive heart failure. A substudy of the Studies of Left Ventricular Dysfunction (SOLVD). Circulation. (1990) 82:1724–9. doi: 10.1161/01.CIR.82.5.1724
194. Szatalowicz VL, Arnold PE, Chaimovitz C, Bichet D, Berl T, Schrier RW. Radioimmunoassay of plasma arginine vasopressin in hyponatremic patients with congestive heart failure. N Engl J Med. (1981) 305:263–6. doi: 10.1056/NEJM198107303050506
195. Vishram-Nielsen JK, Gustafsson F. Vasopressin and vasopressin antagonists in heart failure. Handb Exp Pharmacol. (2017) 243:307–28. doi: 10.1007/164_2017_28
196. Konstam MA, Gheorghiade M, Burnett JC, Jr., Grinfeld L, Maggioni AP, et al. Effects of oral tolvaptan in patients hospitalized for worsening heart failure: the EVEREST outcome trial. JAMA. (2007) 297:1319–31. doi: 10.1001/jama.297.12.1319
197. Xu L, Liu X, Wu S, Gai L. The clinical application value of the plasma copeptin level in the assessment of heart failure with reduced left ventricular ejection fraction: a cross-sectional study. Medicine. (2018) 97:e12610. doi: 10.1097/MD.0000000000012610
198. Kilicgedik A, Eroglu E, Kahveci G, Isgandarov K, Acar E, Ozturk S, et al. Severe mitral regurgitation is associated with increased copeptin levels in heart failure with reduced ejection fraction. Kardiologia Polska. (2017) 75:1307–14. doi: 10.5603/KP.a2017.0140
199. Huang Z, Zhong J, Ling Y, Zhang Y, Lin W, Tang L, et al. Diagnostic value of novel biomarkers for heart failure: a meta-analysis. Herz. (2020) 45:65–78. doi: 10.1007/s00059-018-4702-6
200. Kelly D, Squire IB, Khan SQ, Quinn P, Struck J, Morgenthaler NG, et al. C-terminal provasopressin (copeptin) is associated with left ventricular dysfunction, remodeling, and clinical heart failure in survivors of myocardial infarction. J Card Fail. (2008) 14:739–45. doi: 10.1016/j.cardfail.2008.07.231
201. Zhang P, Wu X, Li G, Sun H, Shi J. Prognostic role of copeptin with all-cause mortality after heart failure: a systematic review and meta-analysis. Ther Clin Risk Manage. (2017) 13:49–58. doi: 10.2147/TCRM.S124689
202. Yan J-J, Lu Y, Kuai Z-P, Yong Y-H. Predictive value of plasma copeptin level for the risk and mortality of heart failure: a meta-analysis. J Cell Mol Med. (2017) 21:1815–25. doi: 10.1111/jcmm.13102
203. Smaradottir MI, Andersen K, Gudnason V, Näsman P, Rydén L, Mellbin LG. Copeptin is associated with mortality in elderly people. Eur J Clin Invest. (2021) 51:e13516. doi: 10.1111/eci.13516
204. Iano? RD, Pop C, Iancu M, Rahaian R, Cozma A, Procopciuc LM. Diagnostic performance of serum biomarkers fibroblast growth factor 21, galectin-3 and copeptin for heart failure with preserved ejection fraction in a sample of patients with type 2 diabetes mellitus. Diagnostics. (2021) 11:1577. doi: 10.3390/diagnostics11091577
205. Loncar G, von Haehling S, Tahirovic E, Inkrot S, Mende M, Sekularac N, et al. Effect of beta blockade on natriuretic peptides and copeptin in elderly patients with heart failure and preserved or reduced ejection fraction: results from the CIBIS-ELD trial. Clin Biochem. (2012) 45:117–22. doi: 10.1016/j.clinbiochem.2011.11.010
206. Cvetinovic N, Sekularac N, Haehling SV, Tahirovic E, Inkrot S, Lainscak M, et al. The β-blocker uptitration in elderly with heart failure regarding biomarker levels: CIBIS-ELD substudy. Biomark Med. (2018) 12:1261–70. doi: 10.2217/bmm-2018-0136
207. Gansevoort RT, van Gastel MDA, Chapman AB, Blais JD, Czerwiec FS, Higashihara E, et al. Plasma copeptin levels predict disease progression and tolvaptan efficacy in autosomal dominant polycystic kidney disease. Kidney Int. (2019) 96:159–69. doi: 10.1016/j.kint.2018.11.044
208. Roth GA, Forouzanfar MH, Moran AE, Barber R, Nguyen G, Feigin VL, et al. Demographic and epidemiologic drivers of global cardiovascular mortality. N Engl J Med. (2015) 372:1333–41. doi: 10.1056/NEJMoa1406656
209. Caplan LR. Intracranial branch atheromatous disease: a neglected, understudied, and underused concept. Neurology. (1989) 39:1246–50. doi: 10.1212/WNL.39.9.1246
210. Global, regional, and national burden of stroke and its risk factors, 1990-2019: 1990-2019: a systematic analysis for the Global Burden of Disease Study 2019. Lancet Neurol. (2021) 20:795–820. doi: 10.1016/s1474-4422(21)00252-0
211. Volpi S, Rabadan-Diehl C, Aguilera G. Vasopressinergic regulation of the hypothalamic pituitary adrenal axis and stress adaptation. Stress. (2004) 7:75–83. doi: 10.1080/10253890410001733535
212. Barreca T, Gandolfo C, Corsini G, Del Sette M, Cataldi A, Rolandi E, et al. Evaluation of the secretory pattern of plasma arginine vasopressin in stroke patients. Cerebrovasc Dis. (2001) 11:113–8. doi: 10.1159/000047622
213. Joynt RJ, Feibel JH, Sladek CM. Antidiuretic hormone levels in stroke patients. Ann Neurol. (1981) 9:182–4. doi: 10.1002/ana.410090212
214. Vakili A, Kataoka H, Plesnila N. Role of arginine vasopressin V1 and V2 receptors for brain damage after transient focal cerebral ischemia. J Cereb Blood Flow Metab. (2005) 25:1012–9. doi: 10.1038/sj.jcbfm.9600097
215. Blek N, Szwed P, Putowska P, Nowicka A, Drela WL, Gasecka A, et al. The diagnostic and prognostic value of copeptin in patients with acute ischemic stroke and transient ischemic attack: a systematic review and meta-analysis. Cardiol J. (2022) doi: 10.5603/CJ.a2022.0045. [Epub ahead of print].
216. Wendt M, Ebinger M, Kunz A, Rozanski M, Waldschmidt C, Weber JE, et al. Copeptin levels in patients with acute ischemic stroke and stroke mimics. Stroke. (2015) 46:2426–31. doi: 10.1161/STROKEAHA.115.009877
217. Wannamethee SG, Welsh P, Lennon L, Papacosta O, Whincup PH, Sattar N. Copeptin and the risk of incident stroke, CHD and cardiovascular mortality in older men with and without diabetes: the British Regional Heart Study. Diabetologia. (2016) 59:1904–12. doi: 10.1007/s00125-016-4011-7
218. Brott T, Adams HP, Jr., Olinger CP, Marler JR, Barsan WG, et al. Measurements of acute cerebral infarction: a clinical examination scale. Stroke. (1989) 20:864–70. doi: 10.1161/01.STR.20.7.864
219. Katan M, Fluri F, Morgenthaler NG, Schuetz P, Zweifel C, Bingisser R, et al. Copeptin: a novel, independent prognostic marker in patients with ischemic stroke. Ann Neurol. (2009) 66:799–808. doi: 10.1002/ana.21783
220. Soldozy S, Yagmurlu K, Norat P, Elsarrag M, Costello J, Farzad F, et al. Biomarkers predictive of long-term outcome after ischemic stroke: a meta-analysis. World Neurosurg. (2021) 163:e1–42. doi: 10.1016/j.wneu.2021.10.157
221. De Marchis GM, Katan M, Weck A, Fluri F, Foerch C, Findling O, et al. Copeptin adds prognostic information after ischemic stroke: results from the CoRisk study. Neurology. (2013) 80:1278–86. doi: 10.1212/WNL.0b013e3182887944
222. Urwyler SA, Schuetz P, Fluri F, Morgenthaler NG, Zweifel C, Bergmann A, et al. Prognostic value of copeptin: one-year outcome in patients with acute stroke. Stroke. (2010) 41:1564–7. doi: 10.1161/STROKEAHA.110.584649
223. De Marchis GM, Weck A, Audebert H, Benik S, Foerch C, Buhl D, et al. Copeptin for the prediction of recurrent cerebrovascular events after transient ischemic attack: results from the CoRisk study. Stroke. (2014) 45:2918–23. doi: 10.1161/STROKEAHA.114.005584
224. De Marchis GM, Dankowski T, König IR, Fladt J, Fluri F, Gensicke H, et al. A novel biomarker-based prognostic score in acute ischemic stroke: the CoRisk score. Neurology. (2019) 92:e1517–25. doi: 10.1212/WNL.0000000000007177
225. Greisenegger S, Segal HC, Burgess AI, Poole DL, Mehta Z, Rothwell PM. Copeptin and long-term risk of recurrent vascular events after transient ischemic attack and ischemic stroke: population-based study. Stroke. (2015) 46:3117–23. doi: 10.1161/STROKEAHA.115.011021
226. Cordonnier C, Demchuk A, Ziai W, Anderson CS. Intracerebral haemorrhage: current approaches to acute management. Lancet. (2018) 392:1257–68. doi: 10.1016/S0140-6736(18)31878-6
227. Ikram MA, Wieberdink RG, Koudstaal PJ. International epidemiology of intracerebral hemorrhage. Curr Atheroscler Rep. (2012) 14:300–6. doi: 10.1007/s11883-012-0252-1
228. Mather HM, Ang V, Jenkins JS. Vasopressin in plasma and CSF of patients with subarachnoid haemorrhage. J Neurol Neurosurg Psychiatry. (1981) 44:216–9. doi: 10.1136/jnnp.44.3.216
229. Szmydynger-Chodobska J, Chung I, Kozniewska E, Tran B, Harrington FJ, Duncan JA, et al. Increased expression of vasopressin v1a receptors after traumatic brain injury. J Neurotrauma. (2004) 21:1090–102. doi: 10.1089/0897715041651033
230. Hockel K, Schöller K, Trabold R, Nussberger J, Plesnila N. Vasopressin V(1a) receptors mediate posthemorrhagic systemic hypertension thereby determining rebleeding rate and outcome after experimental subarachnoid hemorrhage. Stroke. (2012) 43:227–32. doi: 10.1161/STROKEAHA.111.626168
231. Aksu F, Gurger M, Yilmaz M, Atescelik M, Yildiz M, Ilhan N, et al. Copeptin levels in cerebral infarction, intracranial hemorrhage and subarachnoid hemorrhage. Clin Lab. (2016) 62:2387–93. doi: 10.7754/Clin.Lab.2016.160532
232. Zhang R, Liu J, Zhang Y, Liu Q, Li T, Cheng L. Association between circulating copeptin level and mortality risk in patients with intracerebral hemorrhage: a systemic review and meta-analysis. Mol Neurobiol. (2017) 54:169–74. doi: 10.1007/s12035-015-9626-z
233. Report of World Federation of Neurological Surgeons Committee on a universal subarachnoid hemorrhage grading scale. J Neurosurg. (1988) 68:985–6.
Keywords: copeptin, cardiovascular diseases, acute myocardial infarction, heart failure, biomarker
Citation: Mu D, Cheng J, Qiu L and Cheng X (2022) Copeptin as a Diagnostic and Prognostic Biomarker in Cardiovascular Diseases. Front. Cardiovasc. Med. 9:901990. doi: 10.3389/fcvm.2022.901990
Received: 22 March 2022; Accepted: 07 June 2022;
Published: 04 July 2022.
Edited by:
Chia-chi Liu, The University of Sydney, AustraliaReviewed by:
Lana McClements, University of Technology Sydney, AustraliaYunjia Zhang, University of New South Wales, Australia
Copyright © 2022 Mu, Cheng, Qiu and Cheng. This is an open-access article distributed under the terms of the Creative Commons Attribution License (CC BY). The use, distribution or reproduction in other forums is permitted, provided the original author(s) and the copyright owner(s) are credited and that the original publication in this journal is cited, in accordance with accepted academic practice. No use, distribution or reproduction is permitted which does not comply with these terms.
*Correspondence: Ling Qiu, lingqiubj@163.com; Xinqi Cheng, chengxq@pumch.cn