- 1Division of Preventive Medicine, Department of Medicine, Brigham and Women’s Hospital and Harvard Medical School, Boston, MA, United States
- 2Department of Epidemiology, Harvard T.H. Chan School of Public Health, Boston, MA, United States
- 3Department of Cardiology, Tokyo Medical and Dental University, Tokyo, Japan
- 4Department of Cardiology, Tsuchiura Kyodo General Hospital, Tsuchiura, Japan
Background: Coronary flow capacity (CFC) is a potentially important physiologic marker of ischemia for guiding percutaneous coronary intervention (PCI) indication, while the changes through PCI have not been investigated.
Objectives: To assess the determinants and prognostic implication of delta CFC, defined as the change in the CFC status following PCI.
Materials and Methods: From a single-center registry, a total of 450 patients with chronic coronary syndrome (CCS) who underwent fractional flow reserve (FFR)-guided PCI with pre-/post-PCI invasive coronary physiological assessments were included. Associations between PCI-related changes in thermodilution method-derived CFC categories and incident target vessel failure (TVF) were assessed.
Results: The mean (SD) age was 67.1 (10.0) years and there were 75 (16.7%) women. Compared with patients showing no change in CFC categories after PCI, patients with category worsened, +1, +2, and +3 category improved had the hazard ratio (95% CI) for incident TVF of 2.27 (0.95, 5.43), 0.85 (0.33, 2.22), 0.45 (0.12, 1.63), and 0.14 (0.016, 1.30), respectively (p for linear trends = 0.0051). After adjustment for confounders, one additional change in CFC status was associated with 0.61 (0.45, 0.83) times the hazard of TVF. CFC changes were largely predicted by the pre-PCI CFC status.
Conclusion: Coronary flow capacity changes following PCI, which was largely determined by the pre-PCI CFC status, were associated with the lower risk of incident TVF in patients with CCS who underwent PCI. The CFC changes provide a mechanistic explanation on potential favorable effect of PCI on reducing vessel-oriented outcome in lesions with reduced CFC and low FFR.
Introduction
Globally, clinical practice is getting toward choosing a deferral of percutaneous coronary intervention (PCI) in patients with chronic coronary syndrome (CCS) given comparative effectiveness of PCI against medical therapy (1–4) with respect to patient outcomes. PCI is a costly procedure with potential adverse effects (5), and hence the patient selection for the intervention should be very strict especially intending to reduce future adverse events. On the contrary, deferring all elective PCIs in patients with CCS might be too simplistic, given evidence showing the effects of fractional flow reserve (FFR)-guided PCI on reducing spontaneous myocardial infarction or future revascularization (6, 7), and the prognostic benefit of PCI differential according to several factors (1, 2, 8). Recently, PCI is principally guided by FFR or instantaneous wave-free ratio (iFR), whereas integrating complementary characteristics for the purpose is emergingly warranted to tailor the intervention and maximize the clinical benefit.
Coronary flow capacity (CFC) is a relatively new, theoretically grounded physiological index that represents ischemia due to coronary flow limitation (9–12). Reduced CFC is a condition with low coronary flow reserve [CFR; hyperemic coronary flow (hCF) divided by resting CF] combined with slow hyperemic CF rather than fast resting CF. CFC holds interesting prognostic information where severely reduced CFC does not necessarily implicate elevated risk for future cardiovascular events if treated by PCI, whereas low CFR does regardless of PCI treatment (12, 13). Thus, reduced CFC may highlight a reversible feature of ischemic burden through revascularization, and we have previously reported the potential utility of CFC in guiding PCI to improve the overall prognostic benefit (12, 13). However, the change in CFC status following PCI, which is an important measure of assessing the impact of PCI with respect to the flow restoration and the consequent impact on clinical courses, has not been investigated.
In the present study, to fill the knowledge gap, we aimed to assess the prognostic implication of delta CFC, defined as the changes of CFC status following PCI. We also evaluated the predictability of delta CFC. We hypothesized that delta CFC would be associated with vessel-related outcomes and it would be predominantly determined by the pre-PCI CFC status.
Materials and Methods
Population
From January 2011 to April 2019, patients with known CCS who underwent PCI with the measurements of both pre- and post-PCI comprehensive coronary physiological assessments at Tsuchiura Kyodo General Hospital were identified from the institutional database. We excluded patients with indications for revascularization of ≥2 vessels, angiographically significant left main disease, previous CABG, renal insufficiency with baseline creatinine > 2.0 mg/dl, decompensated heart failure, cardiogenic shock, acute myocardial infarction, atrial fibrillation, extremely tortuous, or calcified coronary arteries, vessels with visible collateral development or ostial stenosis, and unreliable physiological assessment including CFR < 0.5 or CFR > 7. We did not exclude on the basis of the extent of stenosis outside of the above criteria, while subtotal lesions in which invasive physiological assessment could not be conducted were not included. The institutional ethics committee approved the study protocol. All patients provided written informed consent for enrollment in the institutional database for potential future investigations. All patient data and procedural details were obtained from medical records. The study complies with the Declaration of Helsinki.
Percutaneous Coronary Intervention and Multivessel Disease
Percutaneous coronary intervention was indicated according to clinical practice guidelines at the time of the procedure with necessarily presence of ischemia evaluated by FFR, stress echocardiogram, cardiac magnetic resonance tomography, coronary computed tomography, single-photon emission computerized tomography, or the combinations, and agreement between ≥2 board-certified cardiologists. The diseased vessel was defined as main branches having ≥50% stenosis on visual assessment, and multi-vessel disease corresponded to coronary arteries with ≥2 angiographical diseased vessels.
Coronary Physiological Assessment
Coronary physiological assessment was performed using thermodilution methods by using PressureWire (Abbott Vascular, St Paul, MN, United States) before and after PCI. After intracoronary nitrate (100 or 200 μg) administration, resting and hyperemic thermodilution curves were obtained in triplicate using three injections (3–4 ml each) of room-temperature saline, and the inverse of the average basal (bTmn) and hyperemic mean transit times (hTmn) were calculated. Hyperemia was induced by intravenous infusion of adenosine 5′-triphosphate (140–160 mg/kg/min). In vessels with tandem lesions, we optimized the treatment strategy in a standard way and conducted physiological assessment as follows: place the wire at the most distal of a target vessel to assess FFR, treat lesions where greater FFR step-up was observed, assess post-PCI physiological indices, and add PCI to residual treatable lesions with apparent FFR step-up (and if so again assess post-PCI physiological indices).
Fractional flow reserve was calculated as the ratio of mean distal coronary pressure (Pd) to mean aortic pressure (Pa) during maximal hyperemia. Basal (bCF) and hyperemic coronary flow (hCF) were defined as the inverse of bTmn and hTmn, respectively (14). CFR was calculated as the ratio of hyperemic to basal coronary flow. IMR was defined as hyperemic Pd * hTmn or hyperemic Pa * hTmn * [(1.35 * ratio of mean distal-to-aortic coronary pressure)–0.32] as detailed in elsewhere (15).
Definition of Coronary Flow Capacity
Coronary flow capacity is a concept incorporating decreased CFR and reduced hyperemic coronary flow originally proposed in PET (9). Most previous studies characterized CFC status as severely reduced, moderately reduced, mildly reduced, and normal, linking them to definite, potential, unlikely, and no ischemia, respectively (10, 12, 16, 17). We defined the CFC status in line with previously published largest study using thermodilution technique (12); normal CFC as CFR ≥ 2.80 with hCF ≥ 3.70; mildly reduced CFC as CFR < 2.80 and ≥2.10, combined with hCF < 3.70 and ≥2.56; moderately reduced CFC as CFR < 2.10 and ≥1.70, and 1/Tmn < 2.56 and ≤2.00; and severely reduced CFC otherwise (CFR < 1.70 and hCF < 2.00). The same criteria were applied for the pre- and post-PCI physiological assessments. Figure 1 illustrates the changes of CFC status before/after PCI in two representative cases.
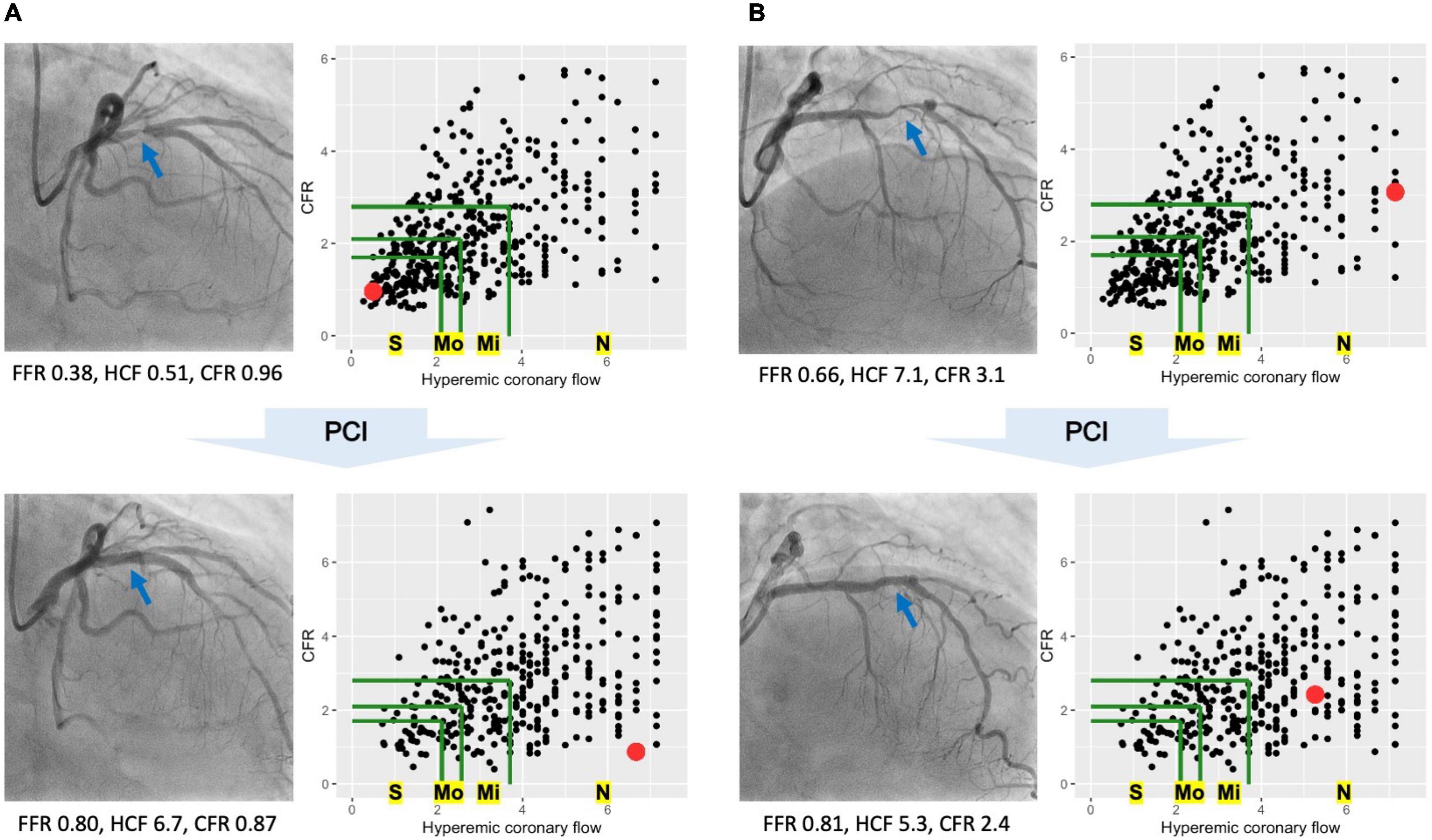
Figure 1. Coronary flow capacity (CFC) changes in two representative cases. Two representative cases showing distinct CFC changes following percutaneous coronary intervention (PCI). In each cine image, blue arrows indicate the culprit lesions in the left anterior descending arteries. Each scatter plot shows CFC map, where each dot representing one vessel is mapped according to the hyperemic coronary flow (hCF, x-axis) and coronary flow reserve (CFR, y-axis). Green lines are the boundaries of CFC categories; the bounded most inner to outer areas are corresponded to severely reduced (S), moderately reduced (Mo), mildly reduced (Mi), and normal CFC (N), respectively. Red dots in the CFC maps represent the cases of each cine image. (A) PCI increased the hCF from 0.51 to 6.7 with a little effect on CFR, leading to the improvement in CFC categories from severely reduced to normal ones. Benefit of PCI would be expected in such cases with greater CFC improvement (i.e., lower risk of target-vessel failure). (B) PCI did not let changes in CFC categories; pre-PCI normal to post-PCI normal CFC. In such cases with no CFC improvement following PCI, the improvement in fractional flow reserve (FFR) might indicate the modification in the epicardial lesions but not the coronary flow restoration, potentially highlighting the limited benefit of PCI.
Delta Coronary Flow Capacity
We ranked CFC categories as (1) for severely reduced, (2) for moderately reduced, (3) for mildly reduced, and (4) for normal. Delta CFC was defined as a numeric difference between post-PCI CFC minus pre-PCI CFC rank, ranging from -3 to +3; for example, +3 reflects the changes from severely reduced to normal CFC following PCI.
Clinical Follow-Up
Patients were followed-up by outpatient clinic visits or by telephone contact to ascertain the occurrence of target vessel failure (TVF), defined as a composite of cardiac death, acute MI not clearly attributable to a non-target vessel (target-vessel MI; TVMI), and clinically driven revascularization of the target (PCI-treated) vessel (target-vessel revascularization; TVR). All patient-reported adverse events were verified by evaluating hospital records or contacting the treating cardiologist or general practitioner. All events were checked at least twice by different experienced cardiologists.
Statistical Analysis
Continuous variables are presented as mean (SD) or median (Q1, Q3) and categorical variables are presented as counts (percentages). Missing values in covariates were imputed by classification and regression tree methods. Baseline characteristics according to the pre-PCI CFC status or CFC changes following PCI were compared based on the standardized mean differences (SMD).
The predictability of CFC changes was assessed for each pre-PCI characteristics, respectively, with use of area under the curve (AUC), sensitivity and specificity at the best cutoffs, and receiver operating characteristic (ROC) curves. The prediction for the continuous delta CFC was evaluated with the use of R-squared values.
Hazard ratios (HRs) of incident TVF were estimated by the COX proportional hazard models, either for categorical CFC changes [worsened, no change (reference), +1 to +3 categories improved] or of continuous delta CFC (per one category change). The p-values for linear trends were calculated from the COX models for continuous delta CFC. Models were adjusted for age (continuous), sex (men/women), diabetes (yes/no), vessel location (left anterior descending/left circumflex/right coronary artery), multivessel disease (yes/no), and FFR (continuous). Associations between pre- or post-PCI CFC categories and incident TVF were also assessed similarly. Relevant Kaplan–Meier curves were also computed.
The discrimination ability of incident TVF was assessed by various nested logistic regression models; Model 1 included age, sex, diabetes, vessel location, and multivessel disease; Model 2 was Model 1 plus pre-PCI FFR; Model 3 was Model 2 plus pre-PCI CFR (continuous); and Model 4 was Model 3 plus delta CFC (in ranks, ranging from -3 to +3). The improvements in the discrimination were assessed by net reclassification improvement (NRI) and integrated discrimination improvement (IDI).
The p-value for linear trend was calculated to estimate the statistical significance of the association of CFC in ranks (ranging from 1 to 4) or delta CFC (ranging from -3 to +3) and incident TVF in the COX proportional hazard models. Two-sided p values for linear trends < 0.05 were considered statistically significant. All analyses were conducted using R 4.0.3 (The R Foundation).
Results
Baseline Characteristics
A total of 450 patients with a clinical indication for revascularization and with 450 vessels with one coronary lesion indicated for and amenable to revascularization (1 vessel/subject) were included in the present analysis, and the mean (SD) age was 67.1 (10.0) years and there were 75 (16.7%) women. Median (Q1, Q3) FFR and CFR were 0.70 (0.63, 0.75) and 2.00 (1.33, 2.95), respectively. A total of 99, 63, 96, and 192 patients were classified as having severely reduced, moderately reduced, mildly reduced, and normal CFC status at baseline.
Table 1 summarizes the patient characteristics according to pre-PCI CFC status. The worse CFC status was associated with generally worse coronary physiologic profile. Medians (IQRs) FFR, CFR, and IMR were 0.62 (0.54, 0.69), 1.17 (1.03, 1.36), and 40.6 (35.0, 55.6) in patients with severely reduced CFC, and 0.73 (0.69, 0.78), 3.05 (2.28, 3.81), and 14.9 (11.0, 19.8) in those with normal CFC, respectively.
Coronary Flow Capacity Changes Following Percutaneous Coronary Intervention
Figure 2 illustrates the changes in CFC categories following PCI. In every pre-PCI CFC status, the majority were improved into normal CFC after PCI, leading to a total of 324 (80%) patients having post-PCI normal CFC status. Worse pre-PCI CFC status was associated with a higher probability of having worse post-PCI CFC status; for example, post-PCI moderately or severely reduced CFC was observed in 20 (24%), 11 (19%), 13 (14%), and 10 (6%) patients in pre-PCI severely reduced, moderately reduced, mildly reduced, and normal CFC status, respectively.
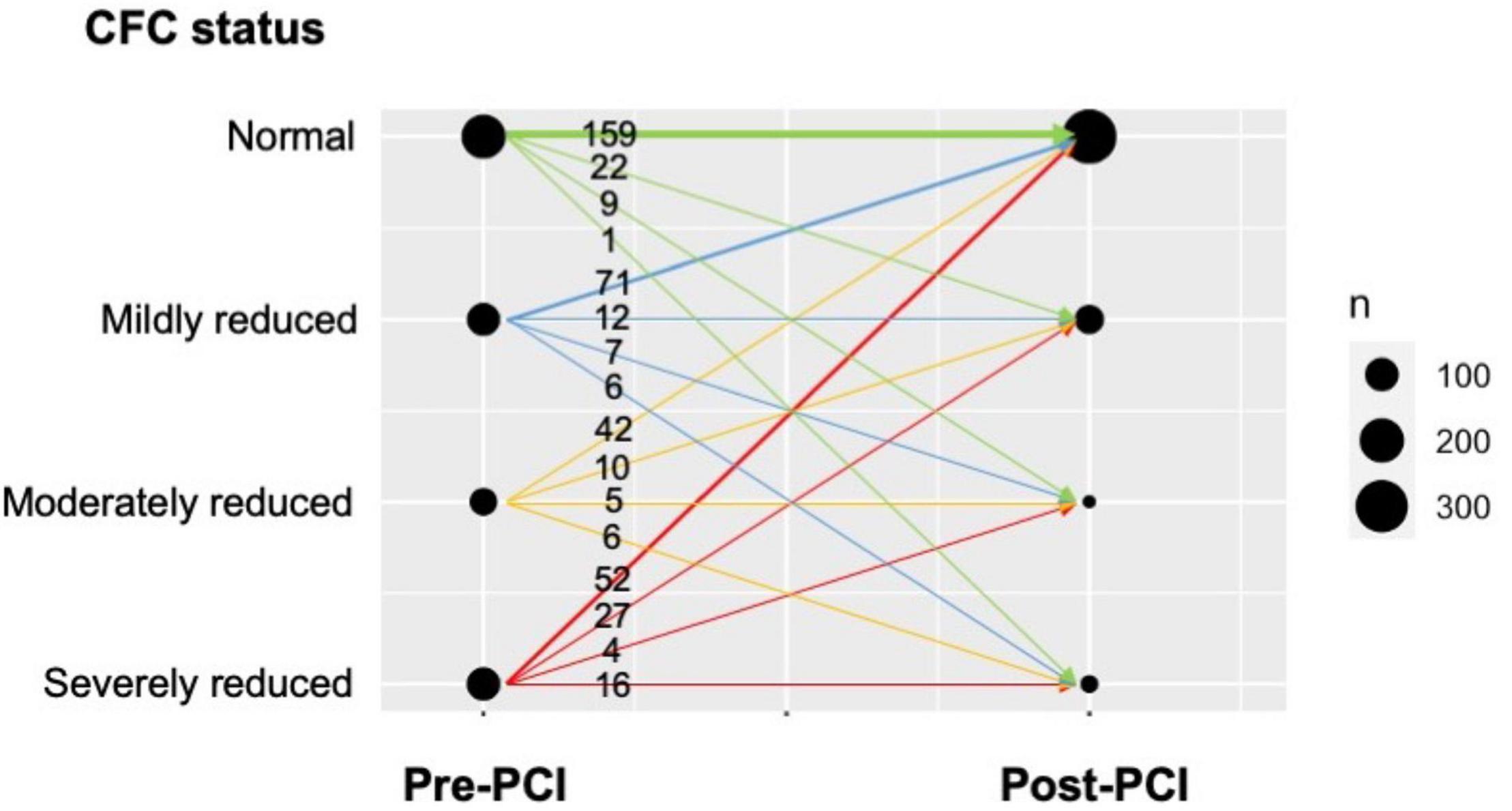
Figure 2. Changes in CFC following PCI in the present cohort. The plot visualizes the number of patients with respect to pre-PCI CFC, post-PCI CFC, and the change. Numbers indicate the number of patients corresponding to the CFC changes, illustrated as the arrows. Thickness of the arrows and circles reflect the number of patients in each CFC change and each pre-/post-CFC categories, respectively. The majority of the patients improved their CFC status into normal after PCI, while the proportion was smaller in those with pre-PCI reduced CFC.
Characteristics of each delta CFC category are summarized in Table 2. Worsening, no change, +1, +2, and +3 rank changes in CFC categories following PCI were observed in N = 52, 192, 85, 69, and 52 patients, respectively. There were no clear trends in demographics across the groups, while lower FFR, CFR and hyperemic coronary flow, higher IMR, and worse CFC profiles were associated with greater CFC improvement. Those with worsened CFC after PCI were characterized with a relatively higher proportion of LAD lesions, while no other clear differences were found comparing with the patients with no changes in CFC.
Prediction of Coronary Flow Capacity Changes
The pre-PCI CFC status, although it has only 4 categories, was highly predictive of the improvement in CFC status following PCI, with AUC (95% CI) of 0.95 (0.93, 0.97) for ≥2 categories improvement (Figure 3A). The sensitivity was 100% because such improvement can only be possible in vessels with pre-PCI moderately or severely reduced CFC. Additional consideration of FFR had little influence on the discrimination (AUC [95% CI]: 0.96 [0.94, 0.98], Figure 3B). Other non-physiological characteristics were not comparatively predictive (Table 3A). Notedly, 48.6% of the variability of continuous delta CFC was explained solely by pre-PCI CFC, while only 12.4% by FFR (Table 3B). Results on the predictions for ≥1 and ≥3 CFC categories improvement were summarized in Tables 3C,D, which is consistently supporting the critical role of pre-PCI CFC in the predictions.
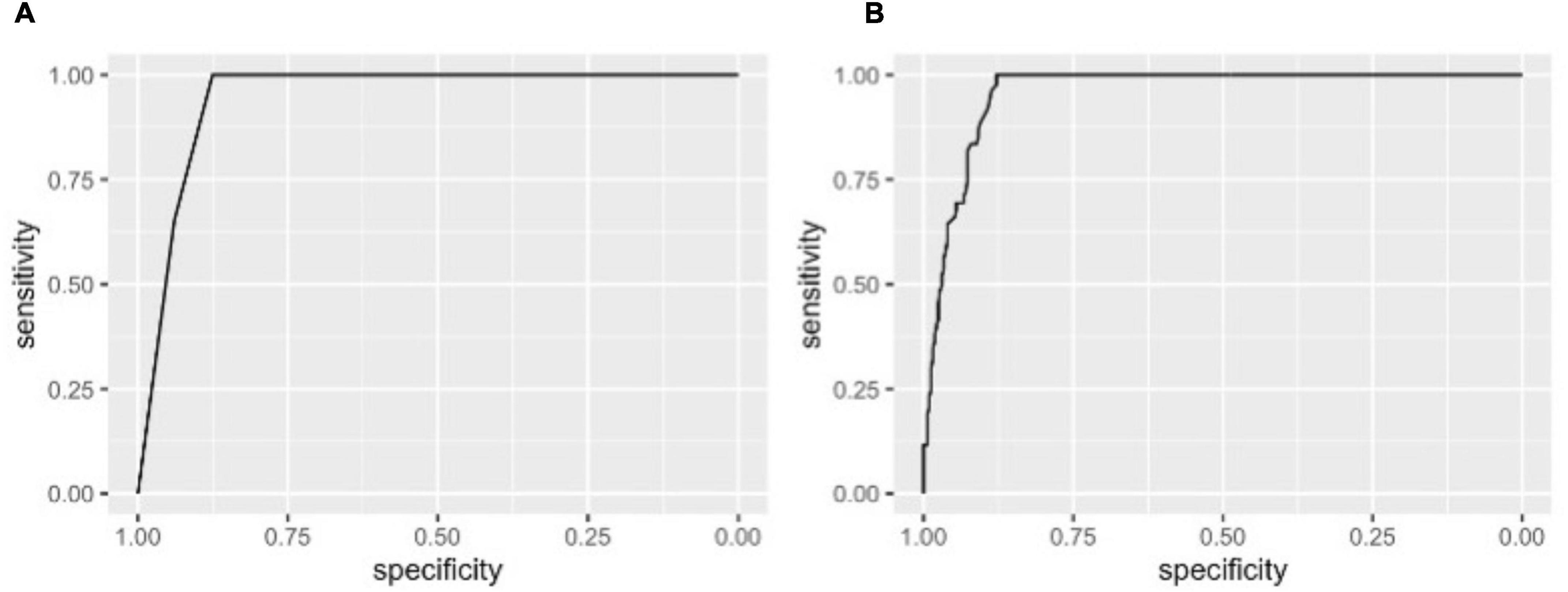
Figure 3. Receiver operating characteristic (ROC) curves for improvement in CFC by ≥2 categories following PCI. The ROC curves showing the discrimination of CFC improvement by ≥2 categories by pre-PCI CFC alone (A) and CFC plus FFR (B). Area under the curves (AUCs) (95% CI) were 0.95 (0.93, 0.97) and 0.96 (0.94, 0.98) in panels (A,B), respectively. Note, at the best cutoffs, the specificity was 100% because such improvement could only be observed in patients with pre-PCI severely or moderately reduced CFC.
Association Between Delta Coronary Flow Capacity and Incident Target Vessel Failure
During a median follow-up of 4.3 (IQR: 2.5, 6.9) years, a total of 36 events were confirmed. Associations between CFC changes and detailed outcomes are summarized in Table 4. Patients with worsened, unchanged, +1, +2, and +3 improved CFC categories had the TVF risk of 17.3, 8.3, 7.1, 5.8, and 1.9%, respectively. Approximately 10% of the patients with worsened CFC had cardiac death or TVMI, whereas only one TVR event was observed in the 52 patients with +3 CFC categories improvement. Compared with no change in CFC categories after PCI, patients with category worsened, +1, +2, and +3 category improved had the hazard ratio (HR) (95% CI) for incident TVF of 2.27 (0.95, 5.43), 0.85 (0.33, 2.22), 0.45 (0.12, 1.63), and 0.14 (0.016, 1.30), respectively (p for linear trends = 0.0051; Table 5). After adjustment for confounders, one additional improvement in CFC status was associated with 0.61 (0.45, 0.83) times the hazard of TVF (p for linear trends = 0.0017). Figure 4 depicts the relevant Kaplan–Meier curves.
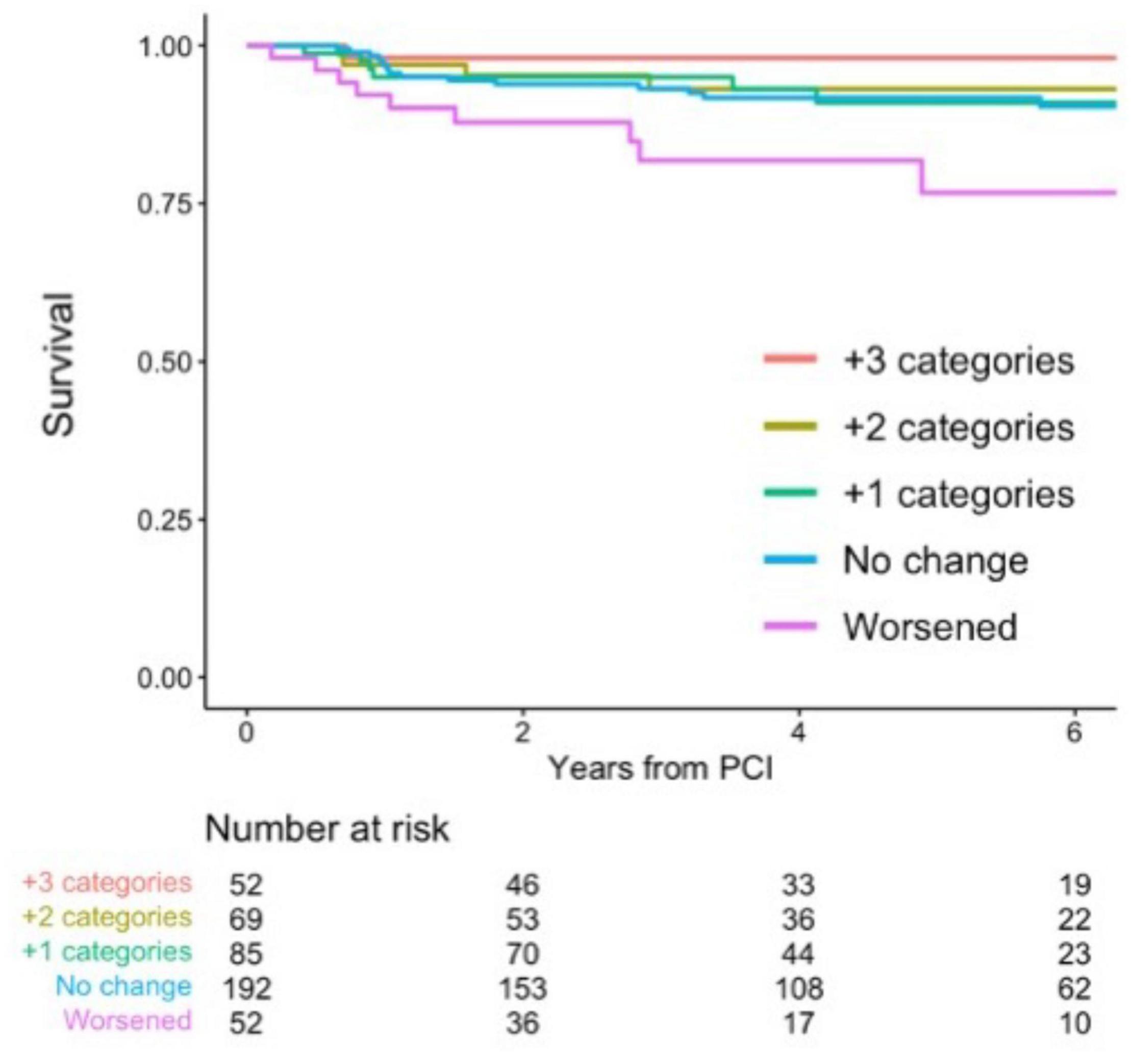
Figure 4. Survival from target-vessel failure (TVF) according to CFC changes. Kaplan–Meier curves showing survival from TVF according to the changes in CFC following PCI. CFC changes were categorized as worsened (-3 to -1 categories change), no change, +1, +2, or +3 categories improvement. Numbers indicate the number of patients at risk.
No survival differences were observed according to distinct pre-PCI CFC status with a multivariable-adjusted HR of 1.25 (0.88, 1.77) for one rank higher CFC category (Tables 6A,B and Figure 5A). There were significant associations between post-PCI CFC and incident TVF (Tables 6C,D and Figure 5B).
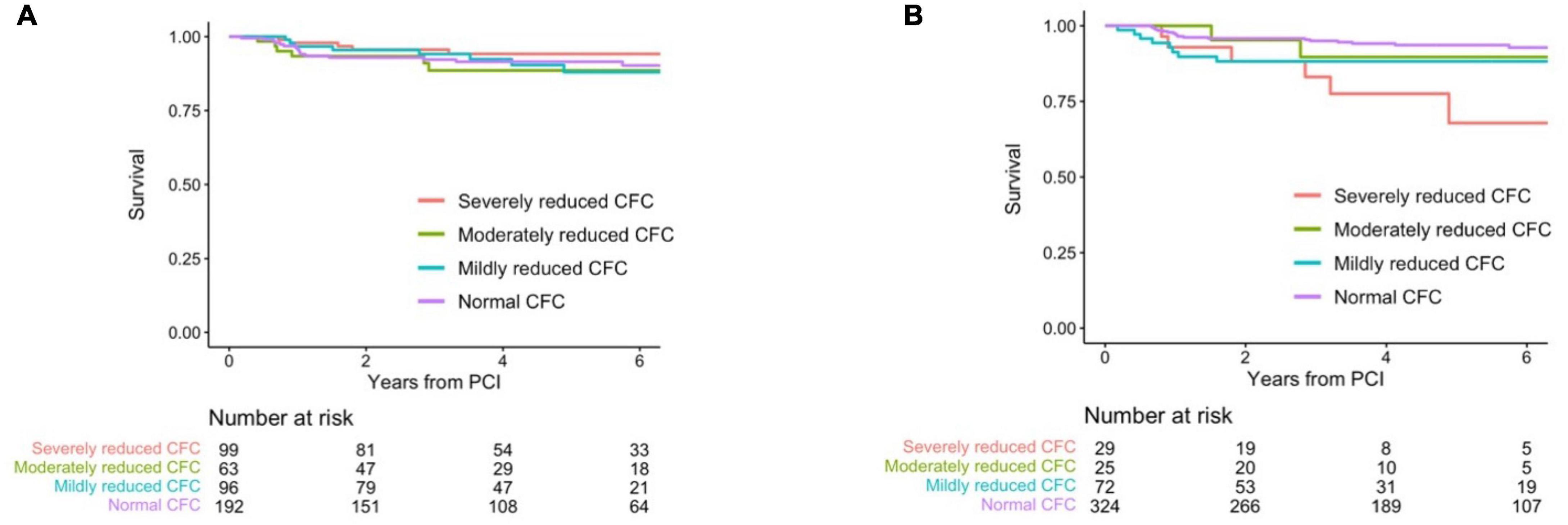
Figure 5. Kaplan–Meier curves showing survival from TVF according to the post-PCI CFC categories. Kaplan–Meier curves showing survival from TVF according to pre- (A) and post-CFC status (B). CFC status was categorized as severely reduced, moderately reduced, mildly reduced, and normal. Numbers indicate the number of patients at risk.
Table 7 shows the prediction of incident TVF by various nested models and the metrics for the improvement in the discrimination. Models comprised solely of demographics had AUC (95% CI) of 0.57 (0.47, 0.67) and the further consideration of pre-PCI FFR and pre-PCI CFR did not improve the discrimination. The model additionally including delta CFC had higher AUC (0.71 [95% CI: 0.62, 0.79]) and the discrimination was well improved compared with the model without delta CFC (NRI: 0.47 [95% CI: 0.14, 0.81] and IDI: 0.035 [95% CI: 0.011, 0.060]).
Discussion
In the present study, the changes in CFC status following PCI were robustly associated with incident TVF in patients with the CCS. The change was largely determined by the pre-PCI CFC status. Furthermore, no association between pre-PCI CFC and incident TVF was observed, suggesting prognostic benefits of PCI in patients with reduced CFC categories. This study provides a mechanistic explanation on potential favorable effects of PCI on reducing vessel-oriented outcomes in lesions with reduced CFC, supporting a use of CFC, in addition to FFR, in guiding PCI to maximize the benefit. A summary of the present study was illustrated in Figure 6.
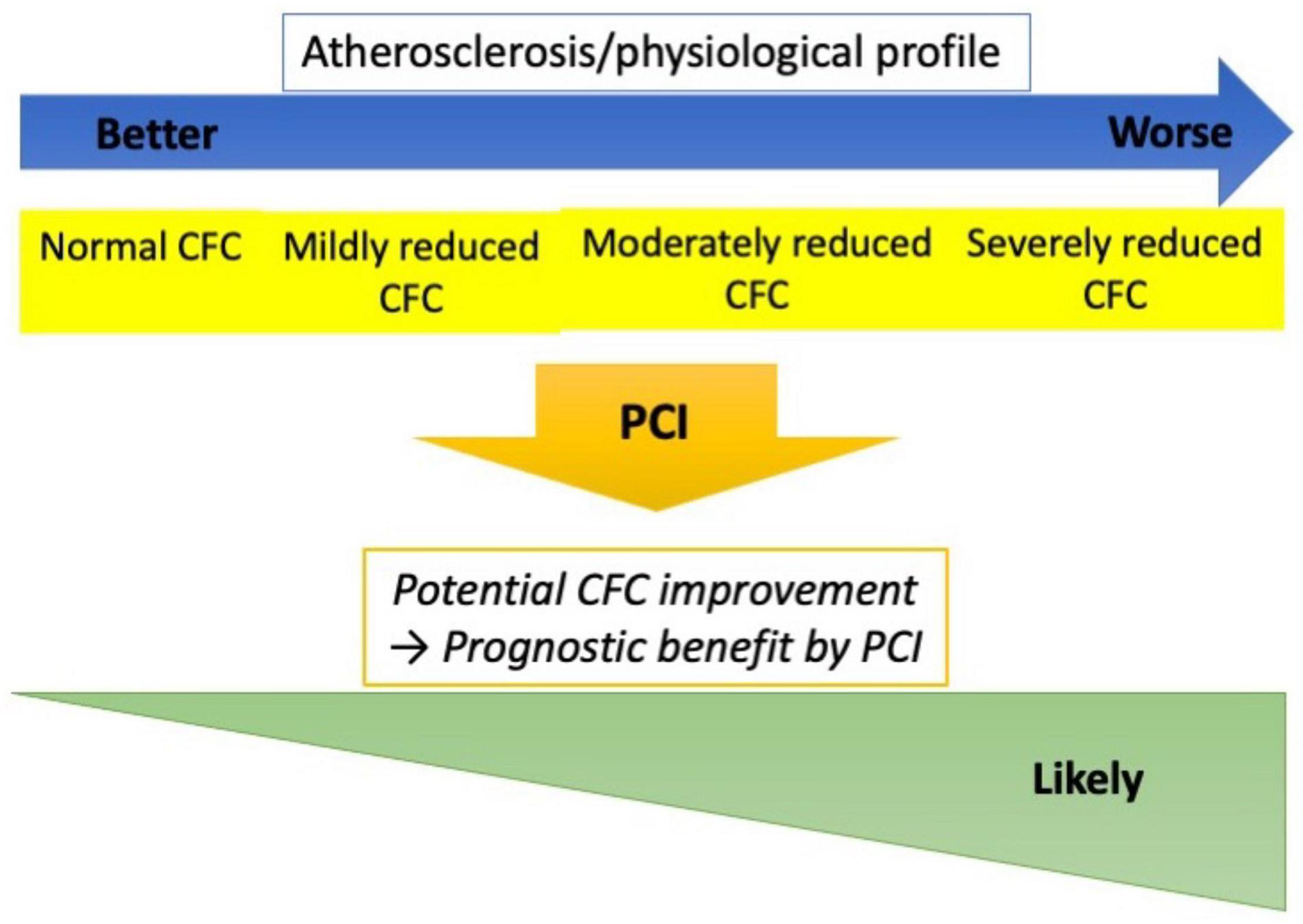
Figure 6. Study summary. Worse CFC status was associated with higher atherosclerotic risk and worse coronary physiological profile. However, the clinical courses were similar across the pre-PCI CFC if treated by elective PCI, while the changes in CFC following PCI were robustly associated with the risk of TVF. Furthermore, CFC changes are largely determined by the pre-PCI status. These observations implicate that lesions with reduced CFC could potentially attain greater CFC improvement by PCI, and consequently the lowered risk of vessel-oriented adverse events. CFC could thus serve as guidance for elective PCI indication, which needs to be evaluated in studies comparing PCI versus medical therapy in reduced CFC lesions.
Although FFR well captures the severity of epicardial atherosclerosis, the index does not directly incorporate the information on coronary flow and microvascular resistance. CFR has been attracted as a potential flow-related marker that could guide PCI, while a recent prospective study did not observe the role (18). This is partly because low CFR is a heterogeneous condition with varied resting and hyperemic coronary flow status (18). The physiological benefit of PCI primarily lies in modifying the hyperemic flow limitation (19–21). CFC is an integrated concept of CFR and hyperemic coronary flow, and thus low FFR combined with reduced CFC highlights hyperemic coronary flow limitation-based ischemia due to epicardial atherosclerosis, where PCI could maximally offer the physiological benefit. In accordance with the theoretical basis, we have previously showed a differential prognostic effect of PCI according to the CFC status in registry data (13). The present study further supports the role of CFC by highlighting the impact of changes in CFC status following PCI, a direct representation of the improvement in coronary flow and ischemic burden; notedly, TVF was observed in only 1 out of 52 patients whose CFC status changed from severely reduced to normal. The pre-PCI CFC status largely offers the prediction of the changes, supportive of the usage in guiding PCI.
From another aspect, current FFR-guidance might indicate too many stable vessels for revascularization in which the physiological benefit from PCI could not be expected. In particular, lesions with low FFR and normal CFC, comprising 43% of vessels in the present registry, hardly anticipates coronary flow restoration or reduction of ischemic burden, and thus these might better be treated medically with respect to prognostic advantage and possibly to symptomatic relief. Although there is a correlation between FFR and CFC, FFR only explains 12% of the variability of CFC changes, supportive of the merit of integrating CFC for guiding PCI indication in addition to FFR. Additionally, while this study demonstrated a clear prognostic contribution of the changes in the regional CFC following PCI, the impact of PCI on the global coronary flow property could be different as we previously described (19, 22, 23). Further consideration of global physiological indices might lead to better identification of patients with CCS who would likely benefit from the intervention.
The study has several limitations. The present analysis is based on a single-center registry and as such the generalizability is limited. The moderate sample size prevents rigorous adjustments of confounders. However, such adjustments could make the estimate further away from null, as higher CFC improvement can occur in patients with pre-PCI reduced CFC, which categories were generally associated with higher atherosclerotic risks and worse physiological profiles. Thermodilution methods could overestimate CFR compared with Doppler-technique (24). Finally, the present study does not directly indicate the usefulness of CFC in guiding PCI but offers an explanation on the potential mechanisms, i.e., improvement in CFC status. Another study is needed to demonstrate the prognostic impact of FFR plus CFC-guided compared with FFR only-guided PCI in a larger population.
Conclusion
Changes in CFC categories following PCI was associated with lower risk of incident TVF in patients with CCS who underwent PCI. The pre-PCI CFC status was a sole strong predictor for the CFC changes. This study provides a mechanistic explanation on a potential favorable effect of PCI on reducing vessel-oriented outcome in lesions with reduced CFC and low FFR.
Data Availability Statement
The raw data supporting the conclusions of this article will be made available by the authors, without undue reservation.
Ethics Statement
The studies involving human participants were reviewed and approved by Tsuchiura Kyodo General Hospital Ethics Committee. The patients/participants provided their written informed consent to participate in this study.
Author Contributions
RH: concept, design, drafting of the manuscript, and statistical analysis. All authors: acquisition, analysis, or interpretation of data. TK: critical revision of the manuscript for important intellectual content, administrative, technical, or material support, and supervision. All authors contributed to the article and approved the submitted version.
Conflict of Interest
The authors declare that the research was conducted in the absence of any commercial or financial relationships that could be construed as a potential conflict of interest.
Publisher’s Note
All claims expressed in this article are solely those of the authors and do not necessarily represent those of their affiliated organizations, or those of the publisher, the editors and the reviewers. Any product that may be evaluated in this article, or claim that may be made by its manufacturer, is not guaranteed or endorsed by the publisher.
Abbreviations
bCF, basal coronary flow; bTmn, basal mean transit time; CFC, coronary flow capacity; CFR, coronary flow reserve; FFR, fractional flow reserve; hCF, hyperemic coronary flow; hTmn, hyperemic mean transit time; PCI, percutaneous coronary intervention; TVF, target vessel failure.
References
1. Maron DJ, Hochman JS, Reynolds HR, Bangalore S, O’Brien SM, Boden WE, et al. Initial invasive or conservative strategy for stable coronary disease. N Engl J Med. (2020) 382:1395–407.
2. Bangalore S, Maron DJ, O’Brien SM, Fleg JL, Kretov EI, Briguori C, et al. Management of coronary disease in patients with advanced kidney disease. N Engl J Med. (2020) 382:1608–18.
3. Boden WE, O’Rourke RA, Teo KK, Hartigan PM, Maron DJ, Kostuk WJ, et al. Optimal medical therapy with or without PCI for stable coronary disease. N Engl J Med. (2007) 356:1503–16. doi: 10.1056/NEJMoa070829
4. Frye RL, August P, Brooks MM, Hardison RM, Kelsey SF, MacGregor JM, et al. A randomized trial of therapies for type 2 diabetes and coronary artery disease. N Engl J Med. (2009) 360:2503–15.
5. Ueki Y, Otsuka T, Bär S, Koskinas KC, Heg D, Häner J, et al. Frequency and outcomes of periprocedural MI in patients with chronic coronary syndromes undergoing PCI. J Am Coll Cardiol. (2022) 79:513–26. doi: 10.1016/j.jacc.2021.11.047
6. Zimmermann FM, Omerovic E, Fournier S, Kelbæk H, Johnson NP, Rothenbühler M, et al. Fractional flow reserve-guided percutaneous coronary intervention vs. medical therapy for patients with stable coronary lesions: meta-analysis of individual patient data. Eur Heart J. (2019) 40:180–6. doi: 10.1093/eurheartj/ehy812
7. Xaplanteris P, Fournier S, Pijls NHJ, Fearon WF, Barbato E, Tonino PAL, et al. Five-year outcomes with PCI guided by fractional flow reserve. N Engl J Med. (2018) 379:250–9. doi: 10.1056/NEJMoa1803538
8. De Bruyne B, Pijls NH, Kalesan B, Barbato E, Tonino PA, Piroth Z, et al. Fractional flow reserve-guided PCI versus medical therapy in stable coronary disease. N Engl J Med. (2012) 367:991–1001. doi: 10.1056/NEJMoa1205361
9. Johnson NP, Gould KL. Integrating noninvasive absolute flow, coronary flow reserve, and ischemic thresholds into a comprehensive map of physiological severity. JACC Cardiovasc Imaging. (2012) 5:430–40. doi: 10.1016/j.jcmg.2011.12.014
10. van de Hoef TP, Echavarría-Pinto M, van Lavieren MA, Meuwissen M, Serruys PW, Tijssen JG, et al. Diagnostic and prognostic implications of coronary flow capacity: a comprehensive cross-modality physiological concept in ischemic heart disease. JACC Cardiovasc Interv. (2015) 8:1670–80. doi: 10.1016/j.jcin.2015.05.032
11. Gould KL, Johnson NP. Coronary physiology beyond coronary flow reserve in microvascular angina: JACC state-of-the-art review. J Am Coll Cardiol. (2018) 72:2642–62. doi: 10.1016/j.jacc.2018.07.106
12. Hamaya R, Yonetsu T, Kanaji Y, Usui E, Hoshino M, Yamaguchi M, et al. Diagnostic and prognostic efficacy of coronary flow capacity obtained using pressure-temperature sensor-tipped wire-derived physiological indices. JACC Cardiovasc Interv. (2018) 11:728–37. doi: 10.1016/j.jcin.2018.01.249
13. Hamaya R, Lee JM, Hoshino M, Yonetsu T, Koo BK, Escaned J, et al. Clinical outcomes of fractional flow reserve-guided percutaneous coronary intervention by coronary flow capacity status in stable lesions. EuroIntervention. (2020) 17:e301–8. doi: 10.4244/EIJ-D-20-00401
14. Fearon WF, Balsam LB, Farouque HM, Caffarelli AD, Robbins RC, Fitzgerald PJ, et al. Novel index for invasively assessing the coronary microcirculation. Circulation. (2003) 107:3129–32. doi: 10.1161/01.CIR.0000080700.98607.D1
15. Yong AS, Layland J, Fearon WF, Ho M, Shah MG, Daniels D, et al. Calculation of the index of microcirculatory resistance without coronary wedge pressure measurement in the presence of epicardial stenosis. JACC Cardiovasc Interv. (2013) 6:53–8. doi: 10.1016/j.jcin.2012.08.019
16. de Winter RW, Jukema RA, van Diemen PA, Schumacher SP, Driessen RS, Stuijfzand WJ, et al. The impact of coronary revascularization on vessel-specific coronary flow capacity and long-term outcomes: a serial [15O]H2O positron emission tomography perfusion imaging study. Eur Heart J Cardiovasc Imaging. (2022) 23:743–52. doi: 10.1093/ehjci/jeab263
17. Murai T, Stegehuis VE, van de Hoef TP, Wijntjens GWM, Hoshino M, Kanaji Y, et al. Coronary flow capacity to identify stenosis associated with coronary flow improvement after revascularization: a combined analysis from DEFINE FLOW and ideal. J Am Heart Assoc. (2020) 9:e016130. doi: 10.1161/JAHA.120.016130
18. Johnson NP, Matsuo H, Nakayama M, Eftekhari A, Kakuta T, Tanaka N, et al. Combined pressure and flow measurements to guide treatment of coronary stenoses. JACC Cardiovasc Interv. (2021) 14:1904–13. doi: 10.1016/j.jcin.2021.07.041
19. Hamaya R, Sugano A, Kanaji Y, Fukuda T, Kanno Y, Yonetsu T, et al. Absolute myocardial blood flow after elective percutaneous coronary intervention evaluated on phase-contrast cine cardiovascular magnetic resonance imaging. Circ J. (2018) 82:1858–65. doi: 10.1253/circj.CJ-17-1449
20. Hamaya R, Kanaji Y, Usui E, Hoshino M, Murai T, Yonetsu T, et al. Improvement of fractional flow reserve after percutaneous coronary intervention does not necessarily indicate increased coronary flow. Eur Cardiol. (2019) 14:10–2. doi: 10.15420/ecr.2018.27.2
21. Kanaji Y, Murai T, Yonetsu T, Usui E, Araki M, Matsuda J, et al. Effect of elective percutaneous coronary intervention on hyperemic absolute coronary blood flow volume and microvascular resistance. Circ Cardiovasc Interv. (2017) 10:e005073. doi: 10.1161/CIRCINTERVENTIONS.117.005073
22. Hamaya R, Fukuda T, Sugano A, Kanaji Y, Hada M, Kanno Y, et al. Impact of regional functional ischemia on global coronary flow reserve in patients with stable coronary artery disease. J Cardiol. (2019) 73:263–70. doi: 10.1016/j.jjcc.2018.12.005
23. Kanaji Y, Yonetsu T, Hamaya R, Murai T, Usui E, Hoshino M, et al. Impact of elective percutaneous coronary intervention on global absolute coronary flow and flow reserve evaluated by phase-contrast cine-magnetic resonance imaging in relation to regional invasive physiological indices. Circ Cardiovasc Interv. (2018) 11:e006676. doi: 10.1161/CIRCINTERVENTIONS.118.006676
Keywords: coronary flow capacity, coronary flow reserve, percutaneous coronary intervention, fractional flow reserve, coronary artery disease
Citation: Hamaya R, Yonetsu T, Sayama K, Matsuda K, Ueno H, Nagamine T, Misawa T, Hada M, Hoshino M, Sugiyama T, Sasano T and Kakuta T (2022) Robust Association Between Changes in Coronary Flow Capacity Following Percutaneous Coronary Intervention and Vessel-Oriented Outcomes and the Implication for Clinical Practice. Front. Cardiovasc. Med. 9:901941. doi: 10.3389/fcvm.2022.901941
Received: 22 March 2022; Accepted: 18 May 2022;
Published: 15 June 2022.
Edited by:
Sara Seitun, Radiology Unit, San Martino Polyclinic Hospital (IRCCS), ItalyReviewed by:
Matteo Tebaldi, Azienda Ospedaliero Universitaria – Cardiology Unit, ItalySergio Berti, Toscana Gabriele Monasterio Foundation, Italy
Yuxiang Dai, Fudan University, China
Jan Piek, Amsterdam University Medical Center, Netherlands
Copyright © 2022 Hamaya, Yonetsu, Sayama, Matsuda, Ueno, Nagamine, Misawa, Hada, Hoshino, Sugiyama, Sasano and Kakuta. This is an open-access article distributed under the terms of the Creative Commons Attribution License (CC BY). The use, distribution or reproduction in other forums is permitted, provided the original author(s) and the copyright owner(s) are credited and that the original publication in this journal is cited, in accordance with accepted academic practice. No use, distribution or reproduction is permitted which does not comply with these terms.
*Correspondence: Rikuta Hamaya, cmt0cm9ja3lAZ21haWwuY29t; Tsunekazu Kakuta, a2F6QGpveS5lbWFpbC5uZS5qcA==