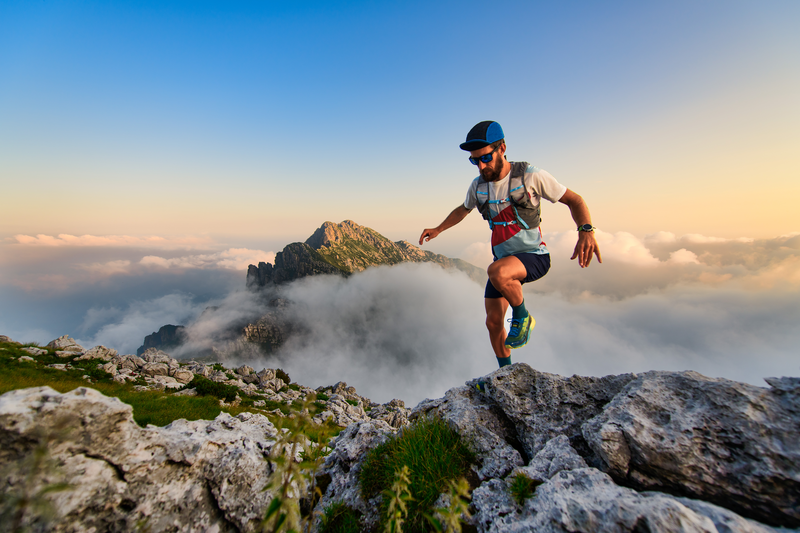
94% of researchers rate our articles as excellent or good
Learn more about the work of our research integrity team to safeguard the quality of each article we publish.
Find out more
MINI REVIEW article
Front. Cardiovasc. Med. , 25 April 2022
Sec. General Cardiovascular Medicine
Volume 9 - 2022 | https://doi.org/10.3389/fcvm.2022.883450
This article is part of the Research Topic Population Data Science in Cardiovascular Medicine View all 13 articles
Heart failure (HF) is a challenging situation in healthcare worldwide. Secondary mitral regurgitation (SMR) is a common condition in HF patients with reduced ejection fraction (HFrEF) and tends to be increasingly associated with unfavorable clinical outcomes as the severity of SMR increases. It is worth noting that SMR can deteriorate dynamically under stress. Over the past three decades, the characteristics of dynamic SMR have been studied. Dynamic SMR contributes to the reduction in exercise capacity and adverse clinical outcomes. Current guidelines refer to the indication of transcatheter edge-to-edge repair (TEER) for significant SMR based on data from the Cardiovascular Outcomes Assessment of the MitraClip Percutaneous Therapy for Heart Failure Patients with Functional Mitral Regurgitation (COAPT) trial if symptomatic despite optimal guideline-directed medical therapy (GDMT) and cardiac resynchronization therapy (CRT), but nonpharmacological treatment for dynamic SMR remains challenging. In HFrEF patients with LV dyssynchrony and dynamic SMR, CRT can improve LV dyssynchrony and subsequently attenuate SMR at rest and during exercise. Also, a recent study suggests that TEER with GDMT and CRT is more effective in symptomatic patients with HFrEF and dynamic SMR than GDMT and CRT alone. Further studies are needed to evaluate the safety and efficacy of nonpharmacological treatments for dynamic SMR. In this review, current evidence and challenges for the future of dynamic SMR are discussed.
Heart failure (HF) is a challenging situation in healthcare worldwide (1–3). HF with reduced ejection fraction (HFrEF) is seen in approximately half of the patients with HF (4). Secondary mitral regurgitation (SMR) with structurally normal leaflets is a common disease in patients with HFrEF (5, 6). Moreover, as the severity of SMR increases, the condition significantly tends to be incrementally associated with unfavorable clinical outcomes (6). As for the treatments for HFrEF with severe SMR, maximally tolerated guideline-directed medical therapy (GDMT) s is recommended (7–10). In patients with an ischemic etiology of the condition, the revascularization of significant coronary artery disease is recommended if applicable. Also, cardiac resynchronization therapy (CRT) for left ventricular (LV) dyssynchrony should be considered when the condition is refractory to the treatments above. Moreover, current guidelines recommend transcatheter edge-to-edge repair (TEER) if feasible when patients with HFrEF and severe SMR have symptoms despite optimal GDMT and CRT.
It is worth noting that SMR can deteriorate dynamically according to changes in hemodynamics (Figure 1). The characteristics of dynamic SMR have been investigated during the past three decades (11–13). Dynamic SMR contributes to reductions in exercise capacity and adverse clinical outcomes (14–19). Although there are evidence-based nonpharmacological approaches for symptomatic SMR, the optimal treatment of dynamic SMR remains a matter of debate. In HFrEF patients with LV dyssynchrony and dynamic SMR, CRT can ameliorate LV dyssynchrony and subsequently attenuate dynamic SMR during exercise (20–22). Also, a recent study has demonstrated that TEER may be effective for dynamic severe SMR (23). Therefore, it is time to renew our knowledge of dynamic SMR and reconsider the optimal therapy of symptomatic dynamic SMR.
Figure 1. Dynamic changes in SMR during transesophageal echocardiography in a 66-year-old male patient who had an anterior old myocardial infarction and heart failure with reduced ejection fraction. Mild SMR (EROA 0.10 cm2) under sedation using midazolam under a systolic blood pressure of approximately 70 mm Hg. (A) Two-dimensional B-mode and color Doppler images from the intercommissural view and (B) a three-dimensional color Doppler image from the en-face view. Dynamic severe SMR (EROA 0.51 cm2) under an elevated systolic blood pressure of approximately 110 mm Hg using norepinephrine. (C) Two-dimensional B-mode and color Doppler images from the intercommissural view and (D) a three-dimensional color Doppler image from the en-face view. SMR, secondary mitral regurgitation; EROA, effective regurgitant orifice area.
This review summarizes current evidence and challenges for the future of dynamic SMR in light of the mechanisms of dynamic SMR, its prognostic value, and its potentially effective treatment options.
The mitral valve apparatus is intricately comprised of several components, including the mitral annulus, anterior and posterior mitral leaflets, chordae tendinae, anterolateral and posteromedial papillary muscles, and adjacent LV wall. MR can be regulated based on an exquisite balance among these components during systole. In SMR, tethering and closing forces of the mitral valve during systole are essential to understand the intricate mechanism. The tethering force is affected by LV dilatation, LV sphericity, LV regional wall motion abnormalities, papillary muscle displacement, papillary muscle dyssynchrony, papillary muscle asymmetry, annular dilatation, and annular flattening. The closing force is decreased due to LV contractility impairments, LV dyssynchrony, increased left atrial (LA) pressure, and decreased mitral annular contraction.
Over the past three decades, the characteristics of dynamic SMR have been studied (11–13). Previous reports have elucidated that changes in LV dyssynchrony, LV sphericity, LV regional wall motion abnormality, increased mitral valve coaptation depth and tenting area, and mitral annular dilatation during exercise are crucial in light of the mechanism of dynamic SMR (24–32). It is worth noting that when comparing ischemic cardiomyopathy with apical and inferobasal scars, the coaptation depth is important in the case of an anterior myocardial infarction while the tenting area and LV regional wall motion abnormality are crucial in the case of an inferior myocardial infarction (24). There is a paucity of data on resting factors associated with dynamic SMR although only LV dyssynchrony at rest is suggested to be related to dynamic SMR (31). It may be because mitral valve tethering and closing forces change based on complicated combinations of dynamic changes of LV and LA geometry and MV apparatus during exercise.
SMR can deteriorate dynamically during exercise (Figure 2). Dynamic SMR is expected to affect a patient's exercise tolerance due to the abruptly deteriorated severity, leading to a reduction in the forward stroke volume and an increase in the overload on the LA and pulmonary circulation during exercise. Izumo et al. elucidated that changes in the effective regurgitant orifice area (EROA) of SMR during exercise stress echocardiography (ESE) are significantly associated with the peak VO2 and VE/VCO2 slope and that the rate of exercise termination is higher in patients with dynamic SMR (ΔEROA ≥0.13 cm2 during exercise) than in those without dynamic SMR (18). Also, Bandera et al. investigated the exercise capacity of patients with HFrEF via cardiopulmonary exercise testing combined with ESE and reported that the exercise tolerance of patients with dynamic severe SMR (EROA ≥0.20 cm2 during exercise) was less than that of patients without dynamic severe SMR (19).
Figure 2. Dynamic changes in SMR during exercise stress echocardiography in an 85-year-old male patient who had an anterior old myocardial infarction and heart failure with reduced ejection fraction. Moderate SMR (EROA 0.22 cm2) at rest. (A,B) Two-dimensional color Doppler images from three- and two-chamber views. Dynamic severe SMR (EROA 0.46 cm2) under stress. (C,D) Two-dimensional color Doppler images from three-chamber and two-chamber views. SMR, secondary mitral regurgitation; EROA, effective regurgitant orifice area.
Lancellotti et al. initially reported that dynamic SMR with changes in the EROA ≥0.13 cm2 during exercise was independently associated with adverse clinical outcomes at mid-term (14, 15). Moreover, long-term clinical outcomes in patients with dynamic severe SMR (EROA ≥0.20 cm2 during exercise) were shown to be unfavorable by Suzuki et al. (16). Also, Piérard et al. (33) investigated the association of dynamic SMR with acute pulmonary edema in patients who recently suffered from acute pulmonary edema and underwent ESE after the improvement of pulmonary edema. Then, changes in the EROA on exercise were reported to be significantly associated with recent acute pulmonary edema. Furthermore, in patients that require hospitalization for acute decompensated HF, dynamic severe SMR on hospitalization is expected to be similar to persistent severe SMR in light of favorable outcomes (17).
The four classes of drugs that constitute GDMT in HFrEF are angiotensin-converting enzyme inhibitors (ACEi)/angiotensin II receptor blockers (ARB)/angiotensin receptor-neprilysin inhibitors (ARNI), beta-blockers (BB), mineralocorticoid receptor agonists (MRA), and sodium-glucose cotransporter 2 inhibitors (SGLT2i); these drugs should be titrated to the maximum tolerated doses in all patients with HFrEF regardless of the presence of SMR (7–10). The optimization of GDMT using ACEi/ARB, BB, and MRA in HFrEF patients is expected to reduce the severity of SMR (34, 35). Also, ARNI has recently received attention as an effective basic HF drug and is recommended prior to ACEi/ARB in patients with HFrEF if applicable according to current guidelines (7–10). ARNIs are effective for LV and LA reverse remodeling (36–39). Moreover, ARNIs are reported to reduce the SMR (40). Also, according to data from a previous meta-analysis, the new “golden triangle” consisting of ARNIs, BBs, and MRAs is the most effective remedy for LV reverse remodeling among several combinations using some GDMT drugs (ACEi, ARB, ARNI, BB, and MRA) (41), which may be expected to bring about further improvements in the dynamic SMR.
A meta-analysis of three cardiac magnetic resonance imaging trials reported that SGLT2i therapy in patients with HFrEF was not significantly associated with reverse cardiac remodeling, including left ventricular ejection fraction, end-systolic volume, and end-diastolic volume; however, there was a tendency toward the improvement of these parameters (42).
In HFrEF patients with persistent severe SMR, GDMT should be optimized as much as possible; however, such patients often suffer from either residual or worsening HF symptoms or undergo repeat HF hospitalization. Thus, when these patients have symptoms despite optimal GDMT, current guidelines recommend nonpharmacological treatment, including CRT and TEER (if applicable), after appropriate revascularization for significant coronary artery disease. However, there remains a matter for consideration in terms of nonpharmacological treatment for dynamic SMR in patients with HFrEF. Then, the nonpharmacological treatment of dynamic SMR is discussed below with a focus on CRT and TEER.
In HFrEF patients with LV dyssynchrony, CRT can suppress LV dyssynchrony and subsequently improve LV hemodynamics while attenuating MR at rest and during exercise (Figure 3) (20, 22). Madaric et al. elucidated the time course of changes in LV dyssynchrony, LV contractility, and SMR at rest and during exercise following CRT (21). Approximately 1 week after CRT, LV dyssynchrony and SMR during exercise did not adequately improve despite ameliorations in LV dyssynchrony and SMR at rest. However, approximately 3 months after CRT, LV dyssynchrony and dynamic SMR were controlled even during exercise with resting SMR and in LV volumes progressively reduced despite there being no additional improvement in the resting LV dyssynchrony. Moreover, the cardiopulmonary performance after CRT improved at late follow-up in HFrEF patients with dynamic SMR although no reports showed a prognostic value of CRT.
Figure 3. Dynamic changes of SMR during exercise stress echocardiography before CRT and controlled SMR following CRT during exercise stress echocardiography in a 71-year-old male patient who had an anterior old myocardial infarction and heart failure with reduced ejection fraction. (A) Complete left bundle branch block with a QRS duration of 154 ms in the electrocardiogram before CRT. (B,C) Mild SMR (EROA 0.14 cm2) at rest and dynamic moderate-to-severe SMR (EROA 0.32 cm2) under stress in two-dimensional color Doppler images from the three-chamber view before CRT. (D) Non-left bundle branch block with a QRS duration of 128 ms in the electrocardiogram after CRT. (E,F) Trivial SMR at rest and under stress 1 year after the CRT in two-dimensional color Doppler images from the three-chamber view after CRT. SMR, secondary mitral regurgitation; CRT, cardiac resynchronization therapy, EROA, effective regurgitant orifice area.
Also, it was (reportedly) possible to induce a left bundle branch block (LBBB) during exercise by increasing the heart rate of HFrEF patients with non-LBBB at rest (43). Rate-related LBBB may be accompanied by LV dyssynchrony and dynamic SMR, subsequently leading to deteriorated hemodynamics and worsening HF symptoms. In the case report, the patient underwent CRT to correct rate-related LBBB, LV dyssynchrony, and dynamic SMR and had favorable clinical outcomes during follow-up (43). Thus, dynamic SMR with rate-related LBBB may be assessed using ESE in unexplained symptomatic patients with HFrEF despite resting non-LBBB, no dyssynchrony, and non-significant SMR.
In patients with HFrEF and dynamic SMR, it may be possible that HF symptoms remain or recur with dynamic SMR refractory to optimal GDMT and CRT. In such patients, TEER can control not only the SMR at rest but also the dynamic change during exercise (Figure 4). Previously, Lancellotti et al. suggested that dynamic SMR should be considered in HFrEF patients with moderate SMR at rest and unexplained dyspnea under optimal GDMT and nonpharmacological treatments, including CRT and revascularization, if indicated, and TEER might be indicated when dynamic SMR was performed during exercise (44). Recently, Izumo et al. reported that TEER is suggested to be safe and effective in light of HF symptoms and clinical outcomes during follow-up in symptomatic HFrEF patients with dynamic SMR (ΔEROA ≥0.13 cm2 during exercise) refractory to treatment with optimized GDMT and CRT if applicable (23). Of note, the patients in the non-TEER group, who had no significant SMR (the EROA of 0.20 ± 0.08 cm2) at rest but significant SMR (the EROA of 0.38 ± 0.10 cm2) during exercise, had a significantly higher rate of HF-related hospitalization and all-cause mortality than those in the TEER group. This suggested that resting SMR in patients with HFrEF is potentially underestimated unless ESE is performed and, consequently, even a non-significant resting SMR can bring about adverse clinical events if medically treated. In addition, this study addressed the association of EROA of SMR with left ventricular end-diastolic volume at rest and during exercise. In the study patients, EROA and left ventricular end-diastolic volume increased significantly during exercise, and as a result, the relationship between EROA in the study and left ventricular end-diastolic volume during exercise was similar to that in the COAPT study. Thus, ESE may be useful to figure out symptomatic patients with HFrEF who have potentially disproportionate dynamic SMR and are expected to receive adequate benefit from TEER.
Figure 4. Dynamic changes in SMR during exercise stress echocardiography before TEER and controlled SMR following TEER during exercise stress echocardiography in an 83-year-old male patient who had an anterior and inferior old myocardial infarction and heart failure with reduced ejection fraction after CRT. (A,B) Trivial SMR at rest in two-dimensional color Doppler images from four-chamber and two-chamber views. (C,D) Dynamic severe SMR (EROA 0.52 cm2) under stress in two-dimensional color Doppler images from four-chamber and two-chamber views. (E,F) Mild MR under stress 6 months after the TEER in two-dimensional color Doppler images from four-chamber and two-chamber views. SMR, secondary mitral regurgitation; TEER, transcatheter edge-to-edge repair; CRT, cardiac resynchronization therapy; EROA, effective regurgitant orifice area; MR, mitral regurgitation.
TEER can reduce the intensity of the symptoms of SMR and its prevalence because of the acute changes in mitral valve geometry as follows; improved coaptation area and mitral valve tethering, decreased anteroposterior diameter and area of the mitral annulus, and increased sphericity of the mitral annulus (45–50), all of which lead to a persistent reduction of the SMR and improvement of the functional status (45, 46). Such acute changes following TEER seem to resist dynamic SMR derived from changes in the mitral valve geometry during exercise. Therefore, TEER could be a reasonable treatment for dynamic SMR in patients with HFrEF. Further studies are required to evaluate the safety and efficacy of TEER for dynamic SMR.
Optimal GDMT reduces the severity of SMR in patients with HFrEF (34, 35, 40). Current guidelines recommend TEER for SMR in patients with HFrEF if they had HF symptoms despite the uptitration of HF drugs as long as tolerated (7, 8). However, a previous study reported that optimal GDMT before TEER was not necessarily achieved (51). Less than 50% of the overall population received >50% of the target dose of ACEi/ARB/ARNI and BB, which suggests the difficulty in the maximal optimization of GDMT in clinical practice. This might be because of hypotension, worsening HF, drug intolerance, and worsening kidney function.
The study also reported that 67% of the patients who underwent TEER had either unchanged or uptitrated GDMT (51). Such patients showed a lower rate of recurrent MR ≥3+, more reduced LV end-systolic volumes, and lower NYHA classes during follow-up than those with downtitrated GDMT. Moreover, unchanged or uptitrated GDMT following TEER was associated with favorable clinical outcomes, which was defined as freedom from death and heart transplantation.
TEER for dynamic SMR may be expected to improve hemodynamics, mitral valve geometry, and HF symptoms, subsequently enabling patients to avoid downtitrated GDMT and gain clinical benefits as with TEER for persistent severe SMR. Thus, optimal GDMT even after TEER for dynamic SMR is also considered the crucial cornerstone of HF management considering its effect in further cardiac reverse remodeling and SMR reduction (45–53). Further studies are needed in light of the importance of optimal GDMT after TEER as well as the safety and efficacy of TEER in patients with HFrEF and dynamic SMR.
Also, there are issues with ESE in patients with HFrEF and SMR; such patients can not exercise long enough to reach peak stress. Therefore, it may be difficult to compare dynamic SMRs among different patients based on certain stress criteria. Then, low-load ESE, which can be performed for a shorter time and under lower stress than conventional ESE, may be reasonable to evaluate the dynamic changes of SMR under specific stress in HFrEF patients. In such patients, it is expected that the usefulness of low load ESE will be examined in future.
Dynamic SMR is associated with exercise performance impairments and adverse clinical outcomes in patients with HFrEF. In such patients, optimal GDMT and CRT are expected to ameliorate the deteriorated mitral valve apparatus, which subsequently leads to improvements in the dynamic SMR. Dynamic SMR can be residual or recurrent even after administering the appropriate treatments above. In such cases, other invasive treatment options, including TEER, may be indicated considering the effectiveness of TEER for dynamic SMR. CRT and TEER, along with GDMT, can improve deteriorated mitral valves and LVs, left ventricular dynamics, HF symptoms, exercise tolerance, and clinical outcomes. Even after obtaining such clinical benefits from CRT and TEER, GDMT regimens should be re-evaluated and reinforced as long as patients are tolerated to aim at further cardiac reverse remodeling and the reduction and prevention of dynamic SMR, subsequently leading to the amelioration of exercise tolerance and clinical outcomes.
HO and MI drafted the manuscript and prepared the figures. TN, SN, and YA revised the manuscript. All authors read and approved the final manuscript.
The authors declare that the research was conducted in the absence of any commercial or financial relationships that could be construed as a potential conflict of interest.
All claims expressed in this article are solely those of the authors and do not necessarily represent those of their affiliated organizations, or those of the publisher, the editors and the reviewers. Any product that may be evaluated in this article, or claim that may be made by its manufacturer, is not guaranteed or endorsed by the publisher.
The authors thank Motoki Miyauchi, Hidekazu Kikuchi, Ayako Shinoda, Maki Suzuki, Toshio Shimamura, Naoki Tanaka, Akino Yoshimura, Yuya Takahashi, and Mayu Kubota for their support in echocardiographic data acquisition.
1. Shimokawa H, Miura M, Nochioka K, Sakata Y. Heart failure as a general pandemic in Asia. Eur J Heart Fail. (2015) 17:884–92. doi: 10.1002/ejhf.319
2. Vos T, Flaxman AD, Naghavi M, Lozano R, Michaud C, Ezzati M, et al. Years lived with disability (YLDs) for 1160 sequelae of 289 diseases and injuries 1990-2010: a systematic analysis for the global burden of disease study 2010. Lancet. (2012) 380:2163–96. doi: 10.1016/S0140-6736(12)61729-2
3. Bui AL, Horwich TB, Fonarow GC. Epidemiology and risk profile of heart failure. Nat Rev Cardiol. (2011) 8:30–41. doi: 10.1038/nrcardio.2010.165
4. Shah KS, Xu H, Matsouaka RA, Bhatt DL, Heidenreich PA, Hernandez AF, et al. Heart failure with preserved, borderline, and reduced ejection fraction: 5-year outcomes. J Am Coll Cardiol. (2017) 70:2476–86. doi: 10.1016/j.jacc.2017.08.074
5. Dziadzko V, Dziadzko M, Medina-Inojosa JR, Benfari G, Michelena HI, Crestanello JA, et al. Causes and mechanisms of isolated mitral regurgitation in the community: clinical context and outcome. Eur Heart J. (2019) 40:2194–202. doi: 10.1093/eurheartj/ehz314
6. Benfari G, Antoine C, Essayagh B, Batista R, Maalouf J, Rossi A, et al. Functional mitral regurgitation outcome and grading in heart failure with reduced ejection fraction. JACC Cardiovasc Imaging. (2021) 14:2303–15. doi: 10.1016/j.jcmg.2021.05.017
7. Otto CM, Nishimura RA, Bonow RO, Carabello BA, Erwin III JP, Gentile F, et al. 2020 ACC/AHA guideline for the management of patients with valvular heart disease: a report of the American college of cardiology/american heart association joint committee on clinical practice guidelines. J Am Coll Cardiol. (2021) 77:e25–e197. doi: 10.1016/j.jacc.2020.11.018
8. Vahanian A, Beyersdorf F, Praz F, Milojevic M, Baldus S, Bauersachs J, et al. 2021 ESC/EACTS guidelines for the management of valvular heart disease. Eur Heart J. (2022) 43:561–632. doi: 10.1093/eurheartj/ehab395
9. Maddox TM, Januzzi JL Jr, Allen LA, Breathett K, Butler J, Davis LL, et al. 2021 Update to the 2017 ACC expert consensus decision pathway for optimization of heart failure treatment: answers to 10 pivotal issues about heart failure with reduced ejection fraction: a report of the American college of cardiology solution set oversight committee. J Am Coll Cardiol. (2021) 77:772–810. doi: 10.1016/j.jacc.2020.11.022
10. McDonagh TA, Metra M, Adamo M, Gardner RS, Baumbach A, Bohm M, et al. 2021 ESC Guidelines for the diagnosis and treatment of acute and chronic heart failure. Eur Heart J. (2021) 42:3599–3726. doi: 10.1093/eurheartj/ehab368
11. Stevenson LW, Bellil D, Grover-McKay M, Brunken RC, Schwaiger M, Tillisch JH, et al. Effects of afterload reduction (diuretics and vasodilators) on left ventricular volume and mitral regurgitation in severe congestive heart failure secondary to ischemic or idiopathic dilated cardiomyopathy. Am J Cardiol. (1987) 60:654–8. doi: 10.1016/0002-9149(87)90376-6
12. Stevenson LW, Brunken RC, Belil D, Grover-McKay M, Schwaiger M, Schelbert HR, et al. Afterload reduction with vasodilators and diuretics decreases mitral regurgitation during upright exercise in advanced heart failure. J Am Coll Cardiol. (1990) 15:174–80. doi: 10.1016/0735-1097(90)90196-v
13. Keren G, Katz S, Strom J, Sonnenblick EH, LeJemtel TH. Dynamic mitral regurgitation. An important determinant of the hemodynamic response to load alterations and inotropic therapy in severe heart failure. Circulation. (1989) 80:306–13. doi: 10.1161/01.cir.80.2.306
14. Lancellotti P, Troisfontaines P, Toussaint AC, Pierard LA. Prognostic importance of exercise-induced changes in mitral regurgitation in patients with chronic ischemic left ventricular dysfunction. Circulation. (2003) 108:1713–7. doi: 10.1161/01.CIR.0000087599.49332.05
15. Lancellotti P, Gerard PL, Pierard LA. Long-term outcome of patients with heart failure and dynamic functional mitral regurgitation. Eur Heart J. (2005) 26:1528–32. doi: 10.1093/eurheartj/ehi189
16. Suzuki T, Izumo M, Suzuki K, Koto D, Tsukahara M, Teramoto K, et al. Prognostic value of exercise stress echocardiography in patients with secondary mitral regurgitation: a long-term follow-up study. J Echocardiogr. (2019) 17:147–56. doi: 10.1007/s12574-018-0404-6
17. Kubo S, Kawase Y, Hata R, Maruo T, Tada T, Kadota K. Dynamic severe mitral regurgitation on hospital arrival as prognostic predictor in patients hospitalized for acute decompensated heart failure. Int J Cardiol. (2018) 273:177–82. doi: 10.1016/j.ijcard.2018.09.093
18. Izumo M, Suzuki K, Moonen M, Kou S, Shimozato T, Hayashi A, et al. Changes in mitral regurgitation and left ventricular geometry during exercise affect exercise capacity in patients with systolic heart failure. Eur J Echocardiogr. (2011) 12:54–60. doi: 10.1093/ejechocard/jeq105
19. Bandera F, Generati G, Pellegrino M, Garatti A, Labate V, Alfonzetti E, et al. Mitral regurgitation in heart failure: insights from CPET combined with exercise echocardiography. Eur Heart J Cardiovasc Imaging. (2017) 18:296–303. doi: 10.1093/ehjci/jew096
20. Ennezat PV, Gal B, Kouakam C, Marquie C, LeTourneau T, Klug D, et al. Cardiac resynchronisation therapy reduces functional mitral regurgitation during dynamic exercise in patients with chronic heart failure: an acute echocardiographic study. Heart. (2006) 92:1091–5. doi: 10.1136/hrt.2005.071654
21. Madaric J, Vanderheyden M, Van Laethem C, Verhamme K, Feys A, Goethals M, et al. Early and late effects of cardiac resynchronization therapy on exercise-induced mitral regurgitation: relationship with left ventricular dyssynchrony, remodelling and cardiopulmonary performance. Eur Heart J. (2007) 28:2134–41. doi: 10.1093/eurheartj/ehm126
22. Witte KK, Sasson Z, Persaud JA, Jolliffe R, Wald RW, Parker JD. Biventricular pacing: impact on exercise-induced increases in mitral insufficiency in patients with chronic heart failure. Can J Cardiol. (2008) 24:379–84. doi: 10.1016/s0828-282x(08)70601-9
23. Izumo M, Kuwata S, Ishibashi Y, Suzuki T, Ohara H, Watanabe M, et al. Prognostic impact of transcatheter mitral valve repair in patients with exercise-induced secondary mitral regurgitation. Eur Heart J Cardiovasc Imaging. (2021) 22:530–8. doi: 10.1093/ehjci/jeaa200
24. Lancellotti P, Lebrun F, Pierard LA. Determinants of exercise-induced changes in mitral regurgitation in patients with coronary artery disease and left ventricular dysfunction. J Am Coll Cardiol. (2003) 42:1921–8. doi: 10.1016/j.jacc.2003.04.002
25. Giga V, Ostojic M, Vujisic-Tesic B, Djordjevic-Dikic A, Stepanovic J, Beleslin B, et al. Exercise-induced changes in mitral regurgitation in patients with prior myocardial infarction and left ventricular dysfunction: relation to mitral deformation and left ventricular function and shape. Eur Heart J. (2005) 26:1860–5. doi: 10.1093/eurheartj/ehi431
26. Lancellotti P, Stainier PY, Lebois F, Pierard LA. Effect of dynamic left ventricular dyssynchrony on dynamic mitral regurgitation in patients with heart failure due to coronary artery disease. Am J Cardiol. (2005) 96:1304–7. doi: 10.1016/j.amjcard.2005.06.077
27. Ennezat PV, Marechaux S, Le Tourneau T, Lamblin N, Bauters C, Van Belle E, et al. Myocardial asynchronism is a determinant of changes in functional mitral regurgitation severity during dynamic exercise in patients with chronic heart failure due to severe left ventricular systolic dysfunction. Eur Heart J. (2006) 27:679–83. doi: 10.1093/eurheartj/ehi682
28. D'Andrea A, Caso P, Cuomo S, Scarafile R, Salerno G, Limongelli G, et al. Effect of dynamic myocardial dyssynchrony on mitral regurgitation during supine bicycle exercise stress echocardiography in patients with idiopathic dilated cardiomyopathy and 'narrow' QRS. Eur Heart J. (2007) 28:1004–11. doi: 10.1093/eurheartj/ehm021
29. Yamano T, Nakatani S, Kanzaki H, Toh N, Amaki M, Tanaka J, et al. Exercise-induced changes of functional mitral regurgitation in asymptomatic or mildly symptomatic patients with idiopathic dilated cardiomyopathy. Am J Cardiol. (2008) 102:481–5. doi: 10.1016/j.amjcard.2008.03.086
30. Kang SJ, Lim HS, Hwang J, Choi JH, Seo KW, Choi BJ, et al. Impact of changes in myocardial velocity assessed by tissue Doppler imaging during exercise on dynamic mitral regurgitation in patients with nonischemic cardiomyopathy. Echocardiography. (2008) 25:394–400. doi: 10.1111/j.1540-8175.2007.00621.x
31. Izumo M, Lancellotti P, Suzuki K, Kou S, Shimozato T, Hayashi A, et al. Three-dimensional echocardiographic assessments of exercise-induced changes in left ventricular shape and dyssynchrony in patients with dynamic functional mitral regurgitation. Eur J Echocardiogr. (2009) 10:961–7. doi: 10.1093/ejechocard/jep114
32. Pecini R, Hammer-Hansen S, Dalsgaard M, Gotze JP, Hassager C, Kober L. Determinants of exercise-induced increase of mitral regurgitation in patients with acute coronary syndromes. Echocardiography. (2010) 27:567–74. doi: 10.1111/j.1540-8175.2009.01077.x
33. Piérard LA, Lancellotti P. The role of ischemic mitral regurgitation in the pathogenesis of acute pulmonary edema. N Engl J Med. (2004) 351:1627–34. doi: 10.1056/NEJMoa040532
34. Stolfo D, Merlo M, Pinamonti B, Poli S, Gigli M, Barbati G, et al. Early improvement of functional mitral regurgitation in patients with idiopathic dilated cardiomyopathy. Am J Cardiol. (2015) 115:1137–43. doi: 10.1016/j.amjcard.2015.01.549
35. Nasser R, Van Assche L, Vorlat A, Vermeulen T, Van Craenenbroeck E, Conraads V, et al. Evolution of functional mitral regurgitation and prognosis in medically managed heart failure patients with reduced ejection fraction. JACC Heart Fail. (2017) 5:652–9. doi: 10.1016/j.jchf.2017.06.015
36. Moon MG, Hwang IC, Choi W, Cho GY, Yoon YE, Park JB, et al. Reverse remodelling by sacubitril/valsartan predicts the prognosis in heart failure with reduced ejection fraction. ESC Heart Fail. (2021) 8:2058–69. doi: 10.1002/ehf2.13285
37. Paolini C, Mugnai G, Dalla Valle C, Volpiana A, Ferraglia A, Frigo AC, et al. Effects and clinical implications of sacubitril/valsartan on left ventricular reverse remodeling in patients affected by chronic heart failure: a 24-month follow-up. Int J Cardiol Heart Vasc. (2021) 35:100821. doi: 10.1016/j.ijcha.2021.100821
38. Wang Y, Zhou R, Lu C, Chen Q, Xu T, Li D. Effects of the angiotensin-receptor neprilysin inhibitor on cardiac reverse remodeling: meta-analysis. J Am Heart Assoc. (2019) 8:e012272. doi: 10.1161/JAHA.119.012272
39. Pascual-Figal D, Bayes-Genis A, Beltran-Troncoso P, Caravaca-Perez P, Conde-Martel A, Crespo-Leiro MG, et al. Sacubitril-Valsartan, clinical benefits and related mechanisms of action in heart failure with reduced ejection fraction. A review. Front Cardiovasc Med. (2021) 8:754499. doi: 10.3389/fcvm.2021.754499
40. Kang DH, Park SJ, Shin SH, Hong GR, Lee S, Kim MS, et al. Angiotensin receptor neprilysin inhibitor for functional mitral regurgitation. Circulation. (2019) 139:1354–65. doi: 10.1161/CIRCULATIONAHA.118.037077
41. Bao J, Kan R, Chen J, Xuan H, Wang C, Li D, et al. Combination pharmacotherapies for cardiac reverse remodeling in heart failure patients with reduced ejection fraction: a systematic review and network meta-analysis of randomized clinical trials. Pharmacol Res. (2021) 169:105573. doi: 10.1016/j.phrs.2021.105573
42. Dhingra NK, Mistry N, Puar P, Verma R, Anker S, Mazer CD, et al. SGLT2 inhibitors and cardiac remodelling: a systematic review and meta-analysis of randomized cardiac magnetic resonance imaging trials. ESC Heart Fail. (2021) 8:4693–700. doi: 10.1002/ehf2.13645
43. Ingraham BS, Chareonthaitawee P, Reddy YNV. Exercise-Induced left bundle branch block resulting in severe mitral regurgitation. JACC Case Rep. (2021) 3:1287–90. doi: 10.1016/j.jaccas.2021.06.029
44. Lancellotti P, Fattouch K, La Canna G. Therapeutic decision-making for patients with fluctuating mitral regurgitation. Nat Rev Cardiol. (2015) 12:212–9. doi: 10.1038/nrcardio.2015.16
45. Schueler R, Momcilovic D, Weber M, Welz A, Werner N, Mueller C, et al. Acute changes of mitral valve geometry during interventional edge-to-edge repair with the MitraClip system are associated with midterm outcomes in patients with functional valve disease: preliminary results from a prospective single-center study. Circ Cardiovasc Interv. (2014) 7:390–9. doi: 10.1161/CIRCINTERVENTIONS.113.001098
46. Schueler R, Kaplan S, Melzer C, Ozturk C, Weber M, Sinning JM, et al. Impact of interventional edge-to-edge repair on mitral valve geometry. Int J Cardiol. (2017) 230:468–75. doi: 10.1016/j.ijcard.2016.12.081
47. Al Amri I, Debonnaire P, van der Kley F, Schalij MJ, Bax JJ, Marsan NA, et al. Acute effect of MitraClip implantation on mitral valve geometry in patients with functional mitral regurgitation: insights from three-dimensional transoesophageal echocardiography. EuroIntervention. (2016) 11:1554–61. doi: 10.4244/EIJY15M09_09
48. Utsunomiya H, Itabashi Y, Kobayashi S, Yoshida J, Ikenaga H, Rader F, et al. Comparison of mitral valve geometrical effect of percutaneous edge-to-edge repair between central and eccentric functional mitral regurgitation: clinical implications. Eur Heart J Cardiovasc Imaging. (2019) 20:455–66. doi: 10.1093/ehjci/jey117
49. Trasca L, Sanchis L, Regueiro A, Freixa X, Vinereanu D, Sitges M. Changes in mitral valve geometry after percutaneous valve repair with the MitraClip® System. Int J Cardiovasc Imaging. (2021) 37:1577–85. doi: 10.1007/s10554-020-02137-4
50. Tusa MB, Barletta M, Popolo Rubbio A, Travaglio N, Saffioti S, Granata G, et al. Acute changes in mitral valve geometry after percutaneous valve repair with MitraClip XTR by three-dimensional echocardiography. Echocardiography. (2021) 38:1913–23. doi: 10.1111/echo.15238
51. Stolfo D, Castrichini M, Biagini E, Compagnone M, De Luca A, Caiffa T, et al. Modifications of medical treatment and outcome after percutaneous correction of secondary mitral regurgitation. ESC Heart Fail. (2020) 7:1753–63. doi: 10.1002/ehf2.12737
52. Stone GW, Lindenfeld J, Abraham WT, Kar S, Lim DS, Mishell JM, et al. Transcatheter mitral-valve repair in patients with heart failure. N Engl J Med. (2018) 379:2307–18. doi: 10.1056/NEJMoa1806640
Keywords: dynamic secondary mitral regurgitation, heart failure with reduced left ventricular ejection fraction, guideline-directed medical therapy, cardiac resynchronization therapy, transcatheter edge-to-edge repair
Citation: Onishi H, Izumo M, Naganuma T, Nakamura S and Akashi YJ (2022) Dynamic Secondary Mitral Regurgitation: Current Evidence and Challenges for the Future. Front. Cardiovasc. Med. 9:883450. doi: 10.3389/fcvm.2022.883450
Received: 25 February 2022; Accepted: 28 March 2022;
Published: 25 April 2022.
Edited by:
Victor Chien-Chia Wu, Chang Gung Memorial Hospital, TaiwanReviewed by:
Ajay Vallakati, The Ohio State University, United StatesCopyright © 2022 Onishi, Izumo, Naganuma, Nakamura and Akashi. This is an open-access article distributed under the terms of the Creative Commons Attribution License (CC BY). The use, distribution or reproduction in other forums is permitted, provided the original author(s) and the copyright owner(s) are credited and that the original publication in this journal is cited, in accordance with accepted academic practice. No use, distribution or reproduction is permitted which does not comply with these terms.
*Correspondence: Masaki Izumo, aGVhcnRpenVtb0B5YWhvby5jby5qcA==
Disclaimer: All claims expressed in this article are solely those of the authors and do not necessarily represent those of their affiliated organizations, or those of the publisher, the editors and the reviewers. Any product that may be evaluated in this article or claim that may be made by its manufacturer is not guaranteed or endorsed by the publisher.
Research integrity at Frontiers
Learn more about the work of our research integrity team to safeguard the quality of each article we publish.