- 1Cardiology Department, Hunan Provincial People's Hospital, Changsha, China
- 2Clinical Research Center for Heart Failure in Hunan Province, Changsha, China
- 3Institute of Cardiovascular Epidemiology, Hunan Provincial People's Hospital, Changsha, China
- 4Department of Cardiology, The Central Hospital of ShaoYang, Shaoyang, China
Background: Upstream transcription factor 1 (USF1) single-nucleotide polymorphisms (SNPs) are significantly associated with serum lipid levels in several different ethnic groups or populations, but their association with lipid levels and the risk of early-onset coronary artery disease (EOCAD) has not been reported in Han populations of southern China.
Methods: Six USF1 SNPs (rs3737787, rs2774276, rs2516839, rs2516838, rs1556259, and rs2516837) were genotyped by next-generation sequencing (NGS) techniques in 686 control subjects and 728 patients with EOCAD.
Results: The genotypic and allelic frequencies of the USF1 rs3737787 SNP were significantly different between the control and EOCAD groups. The subgroup analysis identified that the rs3737787T allele was related to a decreased risk of EOCAD, whereas the rs3737787C–rs2774276G–rs2516839A and rs3737787C–rs2774276G–rs2516839G haplotypes were related to an increased risk of EOCAD in men, and the rs3737787C–rs2774276G–rs2516839A and rs3737787T–rs2774276C–rs2516839A haplotypes were correlated with an increased risk of EOCAD in women (p <0.05–0.01). Male rs3737787T allele carriers had lower low-density lipoprotein cholesterol (LDL-C), total cholesterol (TC), and triglyceride (TG) concentrations than the rs3737787T allele non-carriers (p < 0.01). The interactions of rs3737787 with alcohol consumption and rs2516839 with smoking affected serum TC and LDL-C levels in men, whereas the interaction of rs3737787 with alcohol consumption affected serum high-density lipoprotein cholesterol (HDL-C) levels and the rs2516839-smoking interaction affected serum TC levels in women (pI < 0.001). The expression levels of the USF1 mRNA, interleukin 1β (IL-1β), tumor necrosis factor-α (TNF-α), and interleukin 6 (IL-6) were significantly lower in controls than in patients with EOCAD, and rs3737787T allele carriers displayed lower IL-1β, TNF-α, IL-6, and USF1 mRNA expression levels than the rs3737787T allele non-carriers. In addition, IL-1β, TNF-α, and IL-6 expression levels were significantly positively correlated with USF1 mRNA levels (p < 0.01).
Conclusion: Sex-specific correlations were identified between the USF1 rs3737787T allele with blood lipid levels and the risk of EOCAD. The USF1 rs3737787T allele affects the risk of EOCAD by modulating serum lipid levels and the expression of inflammatory factors, including IL-1β, TNF-α, and IL-6.
Introduction
Coronary artery disease (CAD) is the single most important cause of death and disability worldwide. It causes millions of deaths and hundreds of millions of disabilities every year and places a heavy economic burden on the families of patients and society as a whole (1). In the past, CAD mainly affected elderly individuals; however, with changes in lifestyle, the prevalence of CAD in young people has increased rapidly in recent years. CAD occurring in individuals under the age of 50 years is defined as early-onset coronary artery disease (EOCAD) (2). Recent studies have shown that CAD is a complex and multifactorial disorder caused by a combination of factors, including genomic background, changes in blood lipid levels, environmental factors, and unhealthy lifestyles, as well as interactions between these factors (3, 4). Hyperlipidemia is considered one of the most important risk factors for CAD and its complications. As early as 2013, the guidelines on blood cholesterol treatment issued by The American College of Cardiology (ACC)/American Heart Association (AHA) emphasized that lipid-lowering therapy should not only focus on reducing the low-density lipoprotein cholesterol (LDL-C) levels but also recommended that patients with CAD, especially patients suffering from acute coronary syndrome (ACS), should be treated with comprehensive lipid-lowering therapy to further reduce the cardiovascular risk caused by atherosclerosis in adults (5). Furthermore, several recent research findings suggested that comprehensive lipid-lowering therapy, including reducing the levels of LDL-C (6), total cholesterol (TC) (7), and total triglyceride (TG) (7), will further reduce the risk of cardiovascular events compared with simply reducing LDL-C levels (8). Currently, the 6% efficacy of statins is a common problem in clinical practice, and doubling the dose of statins only reduces the plasma LDL-C level by 6.4%. Therefore, the lipid-lowering regimen of a proprotein convertase subtilisin/kexin type 9 (PCSK9) inhibitor combined with statins is recommended for patients with ACS to further reduce the risk of cardiovascular events (9).
Hyperlipidemia is a highly hereditary disease, and accumulating evidence from the studies of families (10, 11) and twins (12) revealed that single-nucleotide polymorphisms (SNPs) account for 10–50% of the variation in blood lipid levels. Familial combined hyperlipidemia (FCHL) represents the most common metabolic and genetic form of dyslipidemia, with an incidence of 1–3% in the general population and an incidence of 20–38% in those with a prior history of myocardial infarction (MI) (13). FCHL is closely related to EOCAD, and up to 10–14% of patients with EOCAD are complicated by FCHL (14). The serum lipid profile of patients with FCHL is characterized by elevated TG and/or TC levels, and some patients also have increased levels of very LDL-C (VLDL-C), apolipoprotein (Apo) B, and LDL-C, and decreased levels of high-density lipoprotein cholesterol (HDL-C) (15).
The pathogenesis of FCHL is very complex. Although the role of environmental factors is very important, the effect of genetic factors on the pathogenesis of FCHL cannot be ignored (16, 17). The upstream transcription factor 1 (USF1) gene (also named UEF, FCHL, MLTF, FCHL1, MLTFI, HYPLIP1, and bHLHb11, gene ID: 7391, OMIM: 191523, HGNC: 12593) is located on chromosome 1q23.3 (exon count: 12) and encodes a protein belonging to the basic helix–loop–helix leucine zipper family that functions as a key transcription factor to regulate the expression levels of genes such as FASN, ACC, ACLY, and SREBP-1c related to glucose and lipid metabolism (18, 19). Previously, USF1 was identified as the first major gene related to FCHL in the Finnish population, and the variation in this gene significantly affects the risk of cardiovascular disease (20). Coon et al. noticed that rs3737787, the SNP that maintained the strongest relation to FCHL, was located on the 3'UTR of USF1 and correlated significantly with serum LDL-C and TG levels in the Utah population (21). Holzapfel et al. found that the USF1 rs3737787T allele was significantly related to a reduced risk of type 2 diabetes mellitus (T2DM), and other intron variations, including rs2774276C and rs1556259C alleles, were significantly correlated with reduced LDL-C levels in Caucasian women (22). Reiner et al. indicated that the rs2516837T allele, a genetic variation in the 5'UTR of USF1, was significantly correlated with elevated serum LDL-C levels in European Americans (23). The rs2516838 and rs2516839 SNPs are located in the intron and 5'UTR of USF1, respectively, and Zeggini et al. found that the rs2516838G and rs2516839C alleles are significantly associated with reduced serum TC levels in the Utah population (24). In addition, Laurila et al. found that the USF1 rs2516839T and rs1556259T risk alleles are significantly related to increased serum TC and decreased serum HDL-C levels in Australians (25). Based on this evidence, the USF1 gene and its genetic variants are significantly correlated with serum lipid levels, and this correlation is specific to different ethnic groups. Nevertheless, the association between USF1 rs3737787, rs2516837, rs2774276, rs1556259, rs2516838, and rs2516839 SNPs and the risk of EOCAD remains unclear and has not been reported in the Han populations of southern China. Therefore, this study was designed to investigate the correlations between six selected SNPs and serum lipid levels and the risk of EOCAD in the Han populations of southern China.
Materials and Methods
Sample Size Analysis
The QUANTO program (version 1.2.3) (26) was used to calculate the sample size. The minimum total sample sizes that can achieve statistical efficiency and that can be used to calculate the genotypic frequencies of six SNPs and explore the interaction between SNP–SNP/smoking/alcohol consumption are 506, 1,097, 1,198, and 1,113, respectively.
EOCAD Group
A total of 728 patients with EOCAD were recruited from the Cardiovascular Department of Hunan Provincial People's Hospital. Their ages ranged from 18 to 49 years, and the average age was 39.25 ± 5.15 years. The diagnosis of EOCAD was based on the findings of electrocardiography, cardiac biomarkers, clinical manifestations, and coronary angiography. Inclusion criteria for patients with EOCAD were as follows: EOCAD was defined as significant coronary artery stenosis (≥50%) in at least one of the three major coronary arteries and/or their major branches (branch diameter ≥ 2 mm) detected by using coronary angiography (27). Subjects with a history of neoplastic, type 1 diabetes (T1DM), thyroid, hematologic, autoimmune, liver, and/or renal diseases were excluded. Study protocols were developed based on the guidelines from the Ethics Committee of Hunan Provincial People's Hospital and the 2008 revision of the Declaration of Helsinki of 1975 (http://www.wma.net/en/30publications/10policies/b3/). All subjects provided written and informed consent.
Control Group
A total of 686 age- and sex-matched healthy subjects were recruited from the Physical Examination Center of Hunan Provincial People's Hospital. Their ages ranged from 18 to 49 years, and the average age was 39.46 ± 5.18 years. None of these participants suffered from CAD at the time of clinical, biochemical, electrocardiogram, medical history, or imaging examinations, such as 64-slice computed tomography coronary angiography. Meanwhile, subjects with a history of cardiomyopathy, valvular disease, congenital heart disease, other systemic diseases, and/or those who took some medications, including β-adrenergic-blocking agents, hypoglycemic agents, lipid-lowering agents, hormones, or thiazide diuretics, were excluded.
Blood Sample Collection and Blood Lipid Level Detection
About 12 ml of blood were collected from each subject after fasting for more than 12 h, divided into four equal parts (3 ml for each part), and temporarily stored at −20°C until further analysis. Two parts of the sample were collected and placed in yellow glass tubes for blood biochemical tests or enzyme-linked immunosorbent assays (ELISAs). The third part of the sample was collected in an anticoagulant tube containing ethylenediamine tetraacetic acid (EDTA) for RNA extraction. The fourth part of the sample was collected in tubes containing anticoagulants (14.70 g/L glucose, 4.80 g/L citric acid, and 13.20 g/L trisodium citrate) and was utilized to extract DNA. Serum samples were obtained from blood samples after centrifugation at 3,000 rpm for 10 min, and serum LDL-C, ApoB, ApoA1, HDL-C, TG, and TC levels were measured using an autoanalyzer (Type 7170A; Hitachi Ltd., Tokyo, Japan) at the Clinical Laboratory of Hunan Provincial People's Hospital.
Epidemiological Analysis
Universally standardized protocols and methods were utilized to perform the epidemiological investigations (28). A standard set of questionnaires was used to collect a detailed past medical history, family history, medication, smoking and alcohol consumption, and demographic characteristics. Information on alcohol included questions about the number of liangs (~50 ml) of rum, corn wine, beer, rice wine, or liquor consumed during the preceding 12 months. Total alcohol consumption for each participant was calculated by summing the contributions of rum, corn wine, beer, rice wine, and liquor. Drinking more than once a month was defined as alcohol consumption while drinking less than once a month was defined as non-alcohol consumption (29). Subjects who had smoked more than 100 cigarettes during their lifetime, even if they were not currently smoking, and who currently smoked more than one cigarette per day were classified as smokers, and other subjects were classified as non-smokers (30). Body mass index (BMI), height, waist circumference, weight, and blood pressure were measured as previously described (31).
SNP Selection and Genotyping
The USF1 6 SNPs were selected based on the following criteria: (1) tagging SNPs were identified using Haploview (Broad Institute of MIT and Harvard, USA, version 4.2), and the latest version of the 1,000 Genome Project Database was utilized to predict the functional SNPs that might be associated with blood lipid parameters. (2) More details on SNPs were obtained from NCBI dbSNP Build 132 (http://www.Ncbi.nlm.nih.gov/SNP/). (3) The minor allele frequency (MAF) of the six selected SNPs was >5%. (4) Six USF1 SNPs, namely, rs3737787, rs2774276, rs2516839, rs2516838, rs1556259, and rs2516837, were chosen using the block-based method. This step is achieved by marking the degree of linkage imbalance (LD) between SNPs using Haploview (r2 > 0.8). (5) The six selected SNPs have been reported to be correlated with serum lipid parameters or atherosclerosis in different ethnic groups or populations (21–25, 32). The six SNPs were genotyped using next-generation sequencing (NGS) technology at the Center for Human Genetics Research, Shanghai Genesky Bio-Tech Co. Ltd., China (33). The specific steps for multiplex polymerase chain reaction (PCR) and high-throughput sequencing are described in our previous studies (34).
Diagnostic Criteria
The normal reference ranges of blood lipid parameters were TG (0.56–1.70 mmol/L), ApoB (0.80–1.05 g/L), ApoA1/ApoB ratio (1.00–2.50), HDL-C (1.16–1.42 mmol/L), ApoA1 (1.20–1.60 g/L), LDL-C (2.70–3.10 mmol/L), and TC (3.10–5.17 mmol/L). Diagnostic criteria for diabetes (35), hypertension (36), normal weight, overweight, and obesity (37) were described in previous studies.
Definition of Environmental Factors
Sex, age, smoking, alcohol consumption, hypertension, diabetes, and BMI may affect lipid levels. Thus, referring to previous studies (38), we selected these factors as environmental factors to further explore the effect of SNP–environmental factor interactions on serum lipid levels.
Real-Time Quantitative Reverse Transcription PCR
A total of 90 blood samples from patients with EOCAD and 90 samples from normal controls were randomly selected from the total samples of individuals with different rs3737787 genotypes in both the EOCAD and control groups (30 individuals each with the CC, CT, and TT genotypes in both groups). Total RNA was extracted from isolated whole blood samples (3 ml) according to the instructions provided with the TRIzol kit (Invitrogen, USA). Then, the RNA was reverse transcribed into cDNAs using the PrimeScript RT kit (Takara Bio, Japan). Real-Time Quantitative reverse transcription PCR (qRT–PCR) was performed using cDNAs as templates and glyceraldehyde 3-phosphate dehydrogenase (GAPDH) as an internal reference. qRT–PCR was performed using the ABI Prism 7500 sequence-detection system (Applied Biosystems, USA) and the Taq PCR Master Mix kit (Takara). As shown in Supplementary Figure 1, the products of RT-qPCR had a single melting curve indicating the breakdown of only one PCR. The qRT–PCR data were standardized with the 2−ΔΔCt method. qRT–PCR was performed three times for each sample.
ELISA
Blood samples (3 ml) from the same batch of subjects detected using qRT–PCR were centrifuged at 3,000 rpm at room temperature for 10 min to collect serum samples. The levels of interleukin 1β (IL-1β), tumor necrosis factor-α (TNF-α), and interleukin 6 (IL-6) in human serum were determined according to the instructions of the ELISA kits (IL-1β, ab46052; TNF-α, ab181421; and IL-6, ab178013). The standard curve of human TNF-α, IL-6, and IL-1β is shown in Supplementary Figure 2.
Statistical Analyses
SPSS software (Version 22.0) was utilized to perform the statistical analyses. SHEsis software (39) was utilized to calculate the pairwise linkage disequilibrium (LD) and the frequency of haplotypes among the six selected SNPs. The student's unpaired t-test was utilized to evaluate the normally distributed quantitative data [means ± standard deviation (SD)]. Since TG levels were not normally distributed, they are presented as median values and quartile ranges, and differences were calculated using the Wilcoxon–Mann–Whitney test. Qualitative parameters, including the numbers of drinkers and smokers, genotype distribution, and sex ratio, were analyzed using the chi-squared test. A standard goodness-of-fit test was utilized to calculate the Hardy–Weinberg equilibrium (HWE). The correlation between genotypes and serum lipid levels was calculated using analysis of covariance (ANCOVA), and a corrected p-value was adopted after the Bonferroni correction. Several confounding parameters, including sex, alcohol consumption, diabetes, age, smoking, hypertension, and BMI, were adjusted for the statistical analysis. The 95% confidence intervals (CI) and odds ratios (OR) obtained after adjustment for potential confounders were determined using unconditional logistic regression analysis. The effects of the interactions between the six selected SNPs and alcohol consumption, BMI, and cigarette smoking on serum lipid levels were assessed using factorial regression analysis after controlling for several potential confounders. Pearson's correlation analysis was utilized to determine the interactions between USF1 mRNA expression and TNF-α, IL-1β, and IL-6 levels.
Results
Common and Biochemical Characteristics
No significant differences in age structure, diastolic blood pressure, the proportion of smokers, height, or sex ratio were observed between the control and EOCAD groups. The pulse pressure, weight, systolic blood pressure, glucose, and BMI were significantly higher and the proportion of subjects who consumed alcohol was significantly lower in the EOCAD group than in the control group (Table 1).
Serum Lipid Levels in the Control Group
As shown in Table 2, no significant differences in serum ApoB levels were not observed between the control and EOCAD groups. The serum HDL-C, ApoA1, TC, LDL-C, and TG levels, and the ApoA1/ApoB ratio were significantly lower in the EOCAD group than in the control group.
Genotypic and Allelic Frequencies
As depicted in Table 3, the genotype distribution of six selected SNPs was consistent with HWE in both the control and EOCAD groups (p > 0.05). The genotypic (CC, 50.7%; CT, 39.4%; TT, 9.9% vs. CC, 63.5%; CT, 31.9%; TT, 4.6%; p = 3.17E-10) and allelic (C, 70%; T, 30% vs. C, 80.1%; and T, 19.9%; p = 9.69E-11) frequencies of the rs3737787 SNP were significantly different between the control and EOCAD groups. Significant differences in the genotypic and allelic frequencies of the other five SNPs, rs2774276, rs2516839, rs2516838, rs1556259, and rs2516837, were not observed between the control and EOCAD groups. In addition, detailed and specific genotype frequency distributions of the six detected SNPs in the control and EOCAD groups are also depicted in Supplementary File 1.
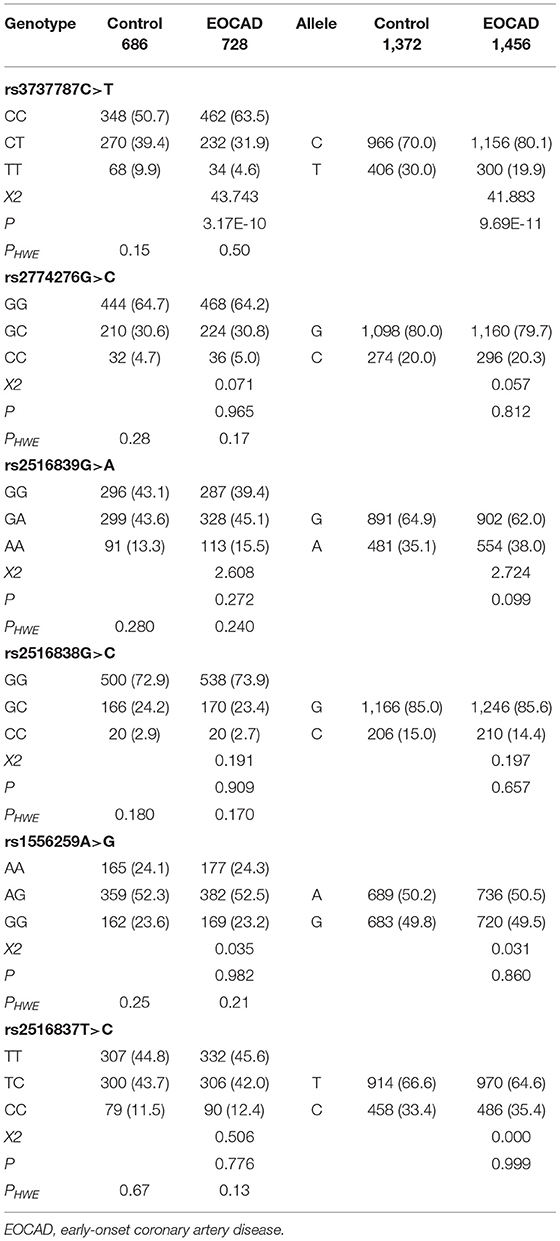
Table 3. Genotype and allele frequencies of the six upstream transcription factor 1 (USF1) single-nucleotide polymorphisms (SNPs) in control and EOCAD groups [n (%)].
Genotypes and the Risk of Diseases
As presented in Table 4, after the Bonferroni correction, only the rs3737787 SNP was correlated with the risk of EOCAD (p < 0.008, where a value of 0.05 after adjustment for six variables was considered statistically significant). The codominant model (TT vs. CC, OR = 0.36, 95% CI = 0.23–0.56, p = 9.62E-6), dominant model (CT/TT vs. CC, OR = 0.59, 95% CI = 0.48–0.73, p = 1.28E-6), recessive model (TT vs. CC/CT, OR = 0.42, 95% CI = 0.27–0.66, p = 2.11E-6), overdominant model (TT vs. CC/CT, OR = 0.72, 95% CI = 0.57–0.91, p = 0.0022), and log-additive model (T vs. C, OR = 0.62, 95% CI = 0.52–0.74, p = 7.62E-5) were considered statistically significant. In addition, a subgroup analysis based on sex revealed that male rs3737787T allele carriers maintained a decreased risk of EOCAD compared with rs3737787T allele non-carriers (CT/TT vs. CC, OR = 0.42, 95% CI = 0.31–0.58, pI = 0.0055); however, this phenomenon was not observed in women (Table 5).
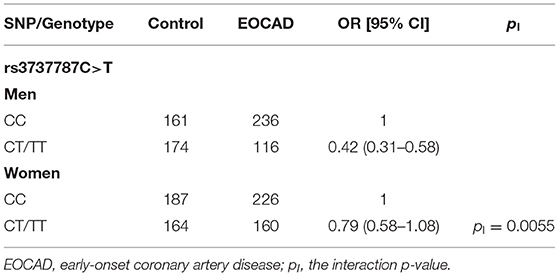
Table 5. Associations between the dominant model of rs3737787 and the risk of EOCAD in men and women populations.
Relationship Between Genotypes and Serum Lipid Parameters
Figure 1 shows that rs3737787T allele carriers maintained lower levels of TC, TG, and LDL-C levels than non-carriers in men but not in women (p < 0.008, corresponding to p < 0.05 after adjusting for six independent tests using the Bonferroni correction). In addition, no significant correlation was observed between the other five SNPs and serum lipid levels (p ≥ 0.008 for all).
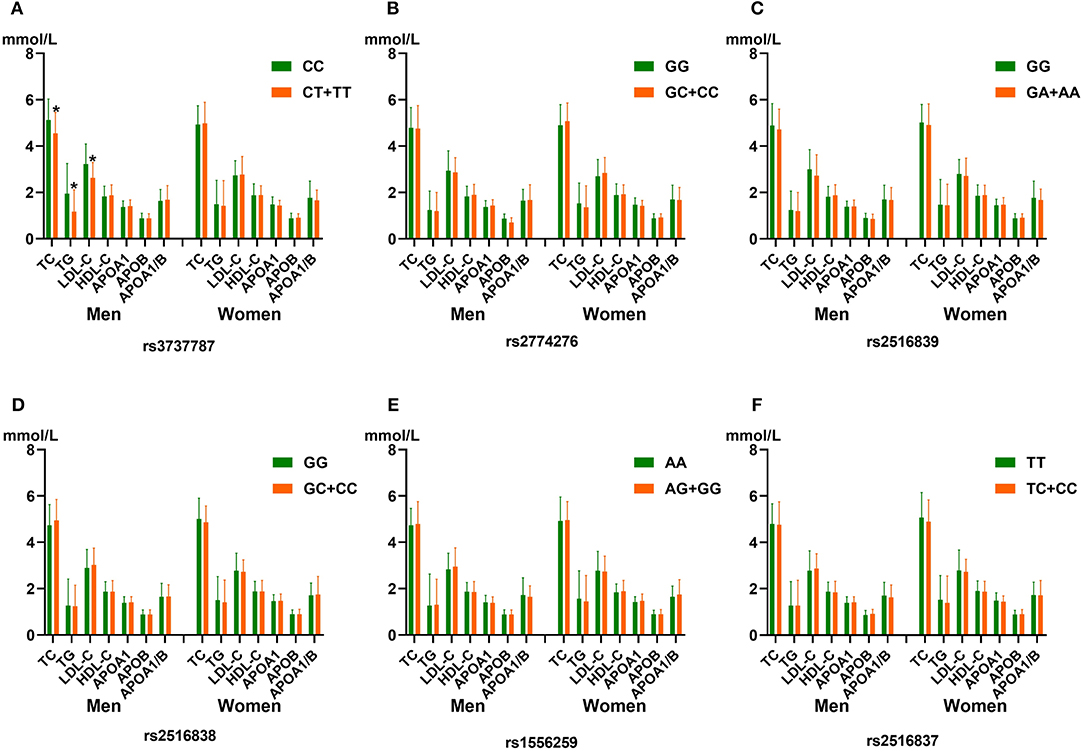
Figure 1. The association between the genotypes of six upstream transcription factor 1 (USF1) single-nucleotide polymorphisms (SNPs) and blood lipid levels in men and women from the control group. (A) rs3737787C>T, (B) rs2774276G>C, (C) rs2516839G>A, (D) rs2516838G>C, (E) rs1556259A>G, and (F) rs2516837T>C SNPs. HDL-C, high-density lipoprotein cholesterol; TC, total cholesterol; ApoA1, apolipoprotein A1; TG, triglyceride; ApoB, apolipoprotein B; LDL-C, low-density lipoprotein cholesterol; ApoA1/ApoB, the ratio of apolipoprotein A1 to apolipoprotein B. *p < 0.008 (after adjusting for six independent tests using the Bonferroni correction) was considered to be statistically significant.
Haplotype Frequencies and the Risk of EOCAD
As shown in Figure 2, moderate LD was noted among the rs3737787, rs2774276, and rs2516839 SNPs in men (Figures 2A,C) and women (Figures 2B,D) (D', 0.75–0.99 and r2, 0.22–0.58). Haplotype analyses were performed among the aforementioned three SNPs. As shown in Table 6, the major haplotype is rs3737787C–rs2774276G–rs2516839G. The rs3737787C–rs2774276G–rs2516839A (adjusted OR = 1.967, 95% CI = 1.422–2.720, p = 3.52E-005) and rs3737787C–rs2774276G–rs2516839G (adjusted OR = 1.619, 95% CI = 1.298–2.019, p = 1.89E-005) haplotypes were correlated with an increased risk of EOCAD in men, and the rs3737787C–rs2774276G–rs2516839A (adjusted OR = 2.951, 95% CI = 1.996–4.362, p = 1.86E-008) and rs3737787T–rs2774276C–rs2516839A (adjusted OR = 1.515, 95% CI = 1.158–1.983, p = 0.002374) haplotypes correlated with an increased risk of EOCAD in women.
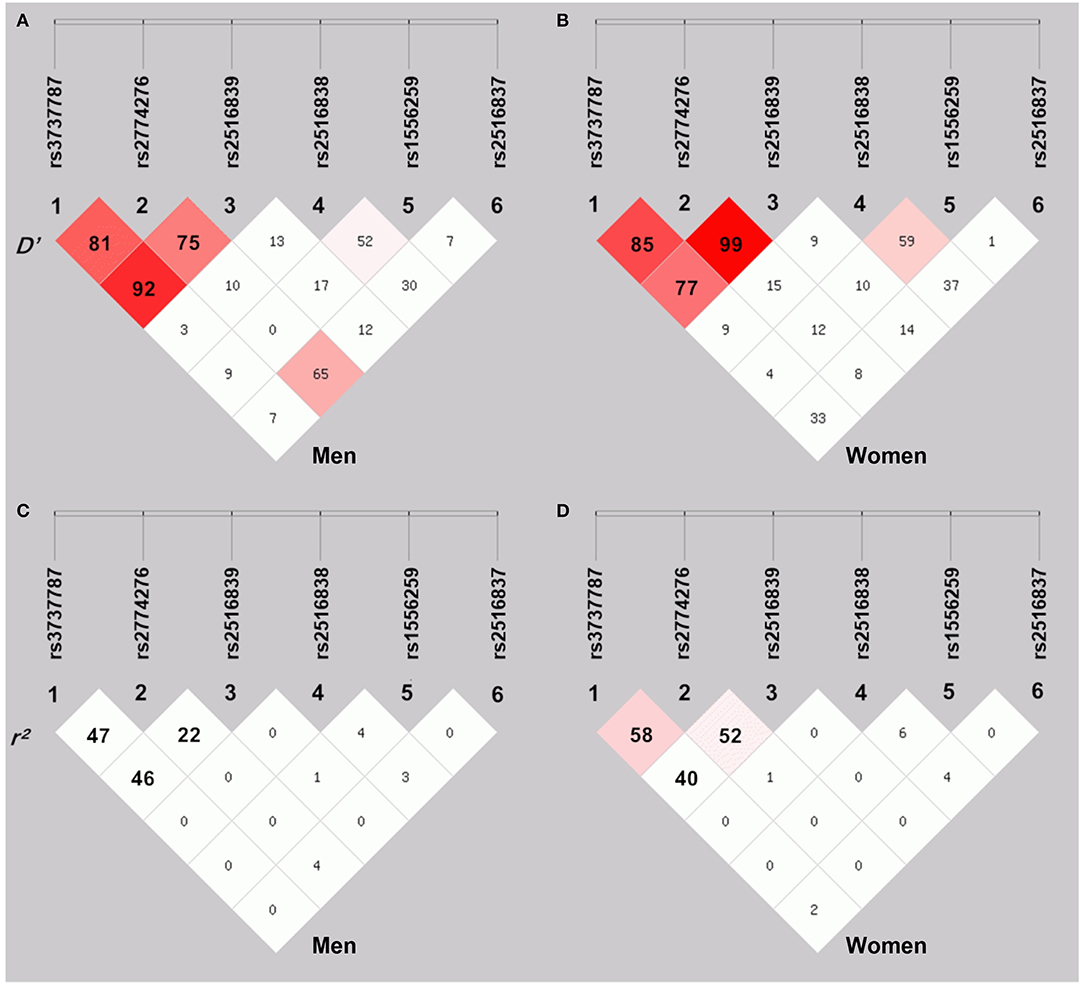
Figure 2. The linkage disequilibrium (LD) represents pairwise D' × 100 (A,B) and r2 × 100 (C,D) in men and women.
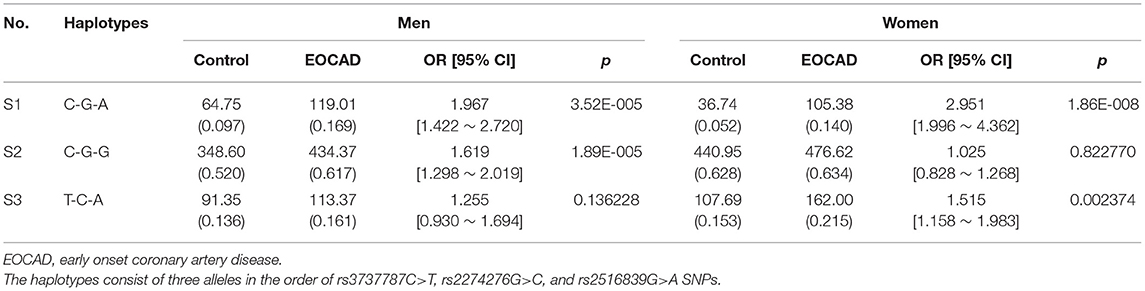
Table 6. Haplotype frequencies and the associations between haplotypes and the risk of EOCAD in men and women populations.
Effects of SNP–Smoking/Alcohol Consumption/BMI Interactions on Serum Lipid Levels
Table 7 depicts the p-values for the interactions (pI) of SNP–alcohol consumption/smoking/BMI on blood lipid parameters in the control group. The rs3737787 and rs2516839 SNPs interacted with smoking or alcohol consumption to affect serum LDL-C and TC levels in men (pI < 0.001, respectively; pI < 0.003 was considered statistically significant after the Bonferroni correction: six SNPs × three risk factors). The rs3737787 SNP interacted with alcohol consumption to affect the levels of HDL-C, and the rs2516839 SNP interacted with smoking to affect the levels of TC in women (pI < 0.001). As shown in Figure 3, the interaction between rs3737787 CT/TT and alcohol consumption decreased TC and LDL-C levels and smoking increased TC and LDL-C levels in men. In addition, the interaction between rs3737787 CT/TT and alcohol consumption increased HDL-C levels; the interaction between rs2516839 GA/AA and smoking increased TC levels in women.
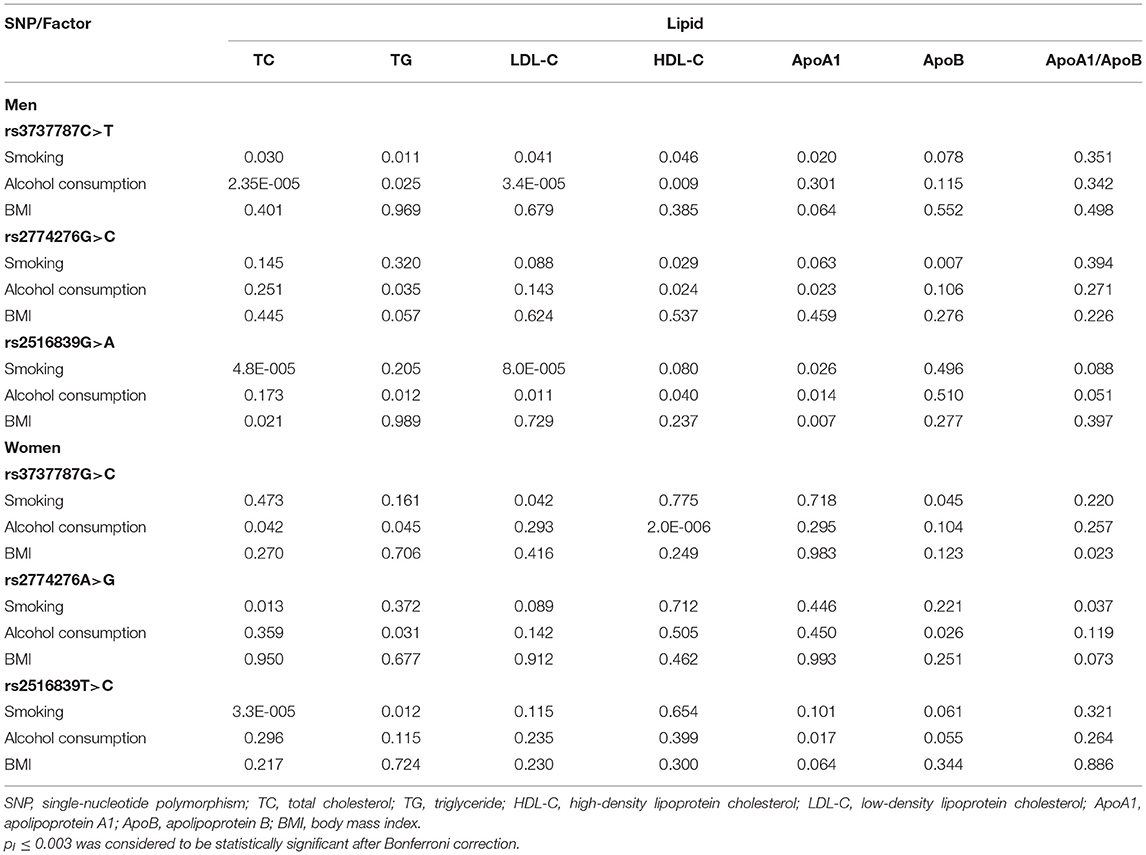
Table 7. The pI values for the interactions of genotypes and alcohol consumption, smoking, and BMI on serum lipid levels.
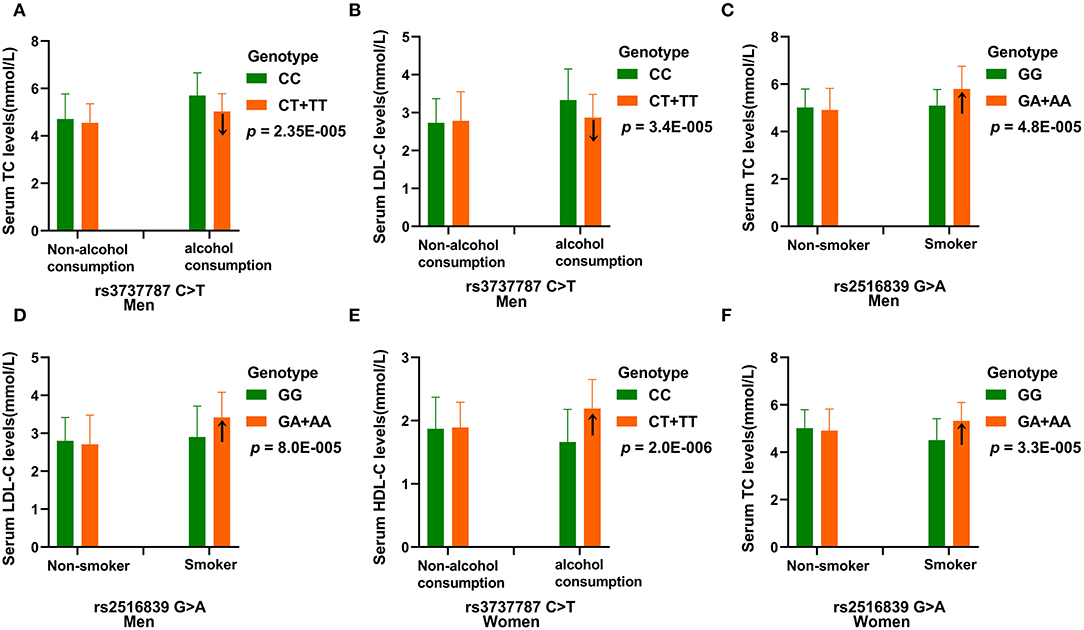
Figure 3. The effect of rs3737787-alcohol consumption and rs2516839-smoking interactions on serum lipid levels. (A) Effect of the rs3737787-alcohol consumption interaction on TC levels in men. (B) Effect of the rs3737787-alcohol consumption interaction on LDL-C levels in men. (C) Effect of the rs2516839-smoking interaction on TC levels in men. (D) Effect of the rs2516839-smoking interaction on LDL-C levels in men. (E) Effect of the rs3737787-alcohol consumption interaction on HDL-C levels in women. (F) Effect of the rs2516839-smoking interaction on TC levels in women. TC, total cholesterol; LDL-C, low-density lipoprotein cholesterol, HDL-C, high-density lipoprotein cholesterol. pI < 0.003 was considered statistically significant after the Bonferroni correction (five SNPs × three risk factors).
Effect of SNP–SNP Interactions on the Risk of EOCAD
As presented in Table 8, subjects with the rs3737787 TT and rs2516839 GA/AA genotypes; the rs3737787 CT/CC and rs2516839 GG genotypes; the rs3737787 CT/CC and rs2516839 GA/AA genotypes maintained a higher risk of EOCAD than those with the rs3737787 TT and rs2516839 GG genotypes in men (adjusted OR = 1.76, 95% CI = 1.13–2.75, p = 0.012; adjusted OR = 2.04, 95% CI = 1.41–2.95, p = 1.71E-4; adjusted OR = 2.74, 95% CI = 1.89–3.98, p = 1.13E-6) and women (adjusted OR = 1.85, 95% CI = 1.16–2.95, p = 0.009; adjusted OR = 2.35, 95% CI = 1.52–3.62, p = 1.21E-4; adjusted OR = 3.16, 95% CI = 1.92–5.19, p = 5.55E-6).
Effects of SNP–Smoking/Alcohol Consumption Interactions on the Risk of EOCAD
As presented in Table 9, the rs3737787 CT/TT–alcohol consumption interaction decreased the risk of EOCAD in both men (adjusted OR = 0.47, 95% CI = 0.29–0.76, pI = 0.0024) and women (adjusted OR = 0.70, 95% CI = 0.52–0.93, pI = 0.011). The rs2516839 GA/AA–smoking interaction increased the risk of EOCAD in both men (adjusted OR = 2.28, 95% CI = 1.39–3.74, pI = 0.00042) and women (adjusted OR = 1.93, 95% CI = 1.14–3.24, pI = 0.009).
Effect of Haplotype–Smoking Interactions on the Risk of EOCAD
As presented in Table 10, the interactions between the rs3737787C–rs2774276G–rs2516839A haplotype and smoking increased the risk of EOCAD in men (adjusted OR = 2.91, 95% CI = 2.15–3.94, p = 4.56E-12) and women (adjusted OR = 4.01, 95% CI = 2.90–5.54, p = 3.89E-17). The rs3737787C–rs2774276G–rs2516839G-smoking interactions (adjusted OR = 2.57, 95% CI = 1.90–3.47, p = 2.51E-12) increased the risk of EOCAD in men, and rs3737787T–rs2774276C–rs2516839A-smoking interactions (adjusted OR = 2.79, 95% CI = 2.08–3.74, p = 6.22E-12) increased the risk of EOCAD in women. However, the effects of the haplotype-BMI/alcohol consumption interactions on the risk of EOCAD were not detected in the current study.
USF1 mRNA Expression Levels
As shown in Figure 4, the qRT–PCR results revealed a markedly increase in USF1 mRNA expressionin patients suffering from EOCAD compared with controls (Figure 4A). In addition, we also noticed that carriers of the rs3737787T allele maintained a lower USF1 mRNA level than rs3737787T non-carriers (Figure 4B).
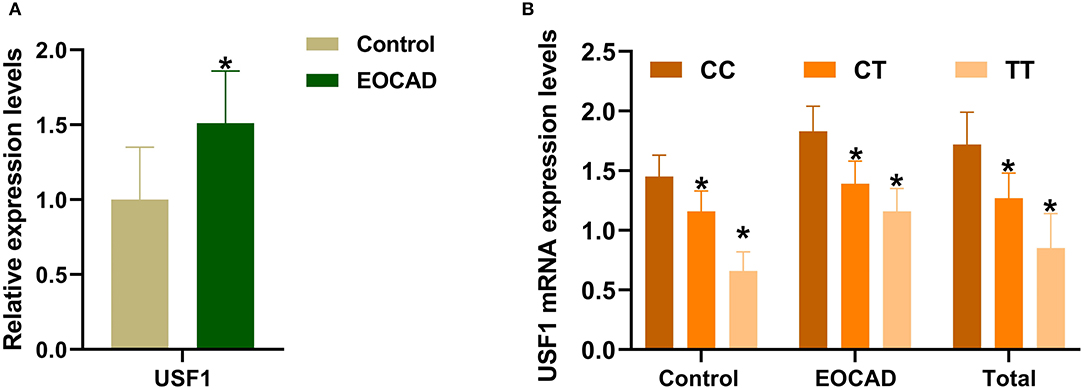
Figure 4. The expression levels of the USF1 mRNA in the control and EOCAD groups. (A) USF1 expression levels were significantly higher in the EOCAD group than in the control group. (B) The rs3737787T allele carriers maintained lower expression levels of the USF1 mRNA than T non-carriers. USF1, upstream transcription factor 1; EOCAD, early-onset coronary artery disease. *p < 0.01.
TNF-α, IL-1β, and IL-6 Expression Levels
Expression levels of IL-1β, IL-6, and TNF-α were markedly increased in patients with EOCAD compared with control subjects (Figure 5A). Carriers of the rs3737787T allele maintained lower levels of IL-1β, IL-6, and TNF-α than rs3737787T non-carriers (Figure 5B). In addition, Pearson's correlation analysis suggested that the levels of TNF-α (Figure 6A), IL-1β (Figure 6B), and IL-6 (Figure 6C) were positively correlated with USF1 mRNA expression levels.
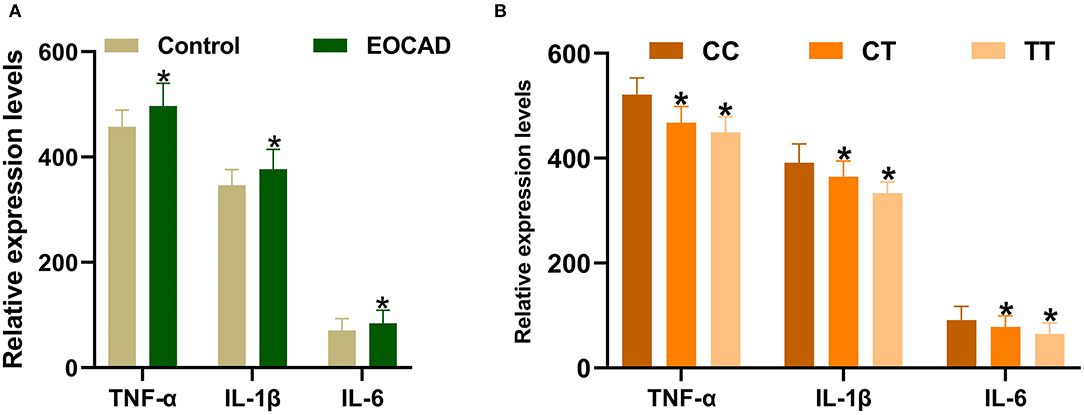
Figure 5. Expression levels of TNF-α, IL-1β, and IL-6 in the control and EOCAD groups. (A) Significantly higher expression levels of TNF-α, IL-1β, and IL-6 were detected in the EOCAD group than in the control group. (B) The rs3737787T allele carriers maintained lower levels of TNF-α, IL-1β, and IL-6 than T non-carriers. USF1, upstream transcription factor 1; EOCAD, early-onset coronary artery disease; TNF-α, tumor necrosis factor-α; IL-1β, interleukin 1β; IL-6, interleukin 6. *p < 0.01.
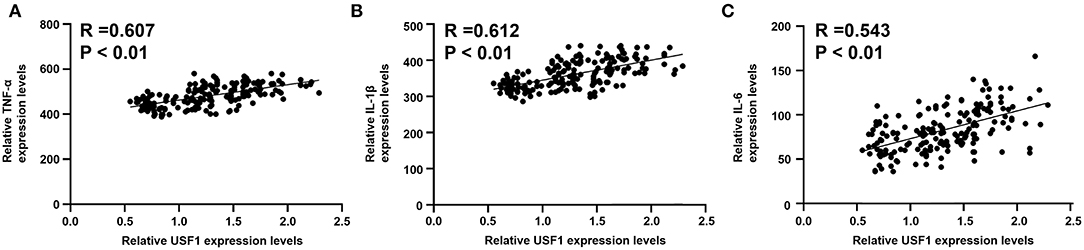
Figure 6. Correlations between USF1 mRNA expression and serum TNF-α, IL-1β, and IL-6 levels. (A) TNF-α levels were positively correlated with the USF1 mRNA expression levels. (B) IL-1β levels were positively correlated with the USF1 mRNA expression levels. (C) IL-6 levels were positively correlated with the USF1 mRNA expression levels. USF1, upstream transcription factor 1; TNF-α, tumor necrosis factor-α; IL-1β, interleukin 1β; IL-6, interleukin 6.
Discussion
As the initial stage of CAD (40), atherosclerosis results from the combination of a chronic inflammatory response (41) and abnormal lipid levels, including reduced levels of Apo A1 (42) and HDL-C (43) along with increased levels of ApoB (42), LDL-C (44), TG (45), and TC (46) in serum. Previous studies have shown that hyperlipidemia, the most common form of dyslipidemia, is essentially a highly heritable disease, and SNPs potentially explain 10–50% of the changes in blood lipid levels (10–12). Meanwhile, a large number of genes and SNPs related to lipid metabolism were also identified as significantly correlated with the risk of CAD (47, 48). The identification of genetic variations related to blood lipid levels and CAD will help to clarify the genetic mechanisms of hyperlipidemia and CAD and will be very important for the prevention and treatment of CAD. Thus, in our current research, we aimed to explore the effects of 6 USF1 SNPs and their interactions with environmental factors on serum lipid levels and the risk of EOCAD. We noticed that the frequencies of the USF1 rs3737787 CT and TT genotypes and T allele were significantly lower in patients with EOCAD than in control subjects, and the USF1 rs3737787T allele was correlated with decreased levels of TC, LDL-C, and TG, as well as a decreased risk of EOCAD in men but not in women. Meanwhile, the rs3737787-alcohol consumption interaction altered serum TC and LDL-C levels in men, serum HDL-C and TC levels in women, and the risk of EOCAD in both men and women. In addition, several haplotypes and interactions of the haplotypes with smoking were associated with different levels of EOCAD risk in men and women. Based on these results, the difference in genotype frequency of the rs3737787 SNP may be an important genetic factor contributing to a significant difference in the susceptibility to EOCAD between men and women.
The allele and genotypic frequencies of the USF1 rs3737787 SNP in different ethnic/racial groups are not completely clear. By searching the International 1,000 Genomes database (https://www.ncbi.nlm.nih.gov/variation/tools/1000~genomes/), we noticed that the frequencies of the rs3737787T allele and the CT, TT genotypes were 28.57, 44.90, and 6.10% in individuals of Mexican ancestry in Los Angeles, California (MEX); 20.93, 39.53, and 1.16% in Japanese individuals; 12.24, 20.28, and 2.10% in the Maasai in Kinyawa, Kenya (MKK); 26.99, 39.82, and 7.08% in Europeans; 3.10, 6.19, and 0.00% in Sub-Saharan Africans; 6.11, 12.22, and 0.00% in the Luhya in Webuye, Kenya (LWK); 10.20, 20.41, and 0.00% in Americans of African Ancestry in the Southwestern USA (ASW); 23.17, 31.71, and 7.30% in Han Chinese individuals in Beijing (CHB); 12.50, 25.00, and 0.00% in Gujarati Indians in Houston (GIH); and 28.98, 37.50, and 10.23% in the Maasai in Toscans in Italy (TSI). In the present study, the allelic and genotypic frequencies of the USF1 rs3737787 SNP were significantly different between the control and EOCAD groups. The frequencies of the CT and TT genotypes and T allele in the current study populations were 30.00, 39.40, and 9.90% in controls and 19.90, 31.90, and 4.70% in patients with EOCAD. These findings indicate that the frequency distribution of the USF1 rs3737787 SNP may display ethnic/racial and population-specific patterns. However, these findings still require further confirmation in other populations or ethnic groups with larger sample sizes.
The correlations between the rs3737787 SNP and serum lipid parameters have been reported in several different ethnic groups. Coon et al. found that USF1 rs3737787, the most significant genetic variant associated with FCHL, is significantly correlated with serum TG and LDL-C levels in the Utah population (21). Lee et al. found that the USF1 rs3737787C risk allele is significantly correlated with TG, TC, and ApoB levels, as well as FCHL in Dutch males, and with BMI and serum TG levels in American white males (32). Reiner et al. found that the USF1 rs3737787T allele correlates with decreased serum TC and LDL-C levels (23). Song et al. found that the USF1 rs3737787 SNP is associated with TG and Apo E levels in families with T2DM in northern China (49). However, the potential associations between the rs3737787 SNP with serum lipid levels and the risk of EOCAD among the Han population in southern China remain unclear. In the present study, we found that the rs3737787 SNP was significantly correlated with serum LDL-C, TG, and TC levels; male rs3737787T allele carriers maintained lower TC, LDL-C, and TG levels than non-carriers; and the dominant model of the rs3737787 SNP decreased the morbidity of EOCAD in men but not in women. When further exploring the effects of the interactions of SNP–environment factors on serum lipid levels and the risk of EOCAD, we found that the rs3737787-alcohol consumption interaction decreased serum TC and LDL-C levels in men, increased serum HDL-C levels in women, and decreased the risk of EOCAD in both men and women. Thus, the effects of the rs3737787 SNP and the rs3737787-alcohol consumption interaction on serum lipid levels and the risk of EOCAD are specific to ethnicity and sex.
When further exploring the LD among the six SNPs, moderate LD was noticed among the rs3737787, rs2774276, and rs2516839 SNPs. The haplotype analysis revealed that rs3737787C-rs2774276G-rs2516839G is the dominant haplotype, and it correlates with an increased risk of EOCAD in men but not in women. Previous studies suggested that the rs3737787C allele was correlated with an increased risk of early-onset coronary atherosclerosis in young adults (23). According to Laurila et al. the rs2516839A allele is significantly correlated with advanced atherosclerosis of the coronary artery and abdominal aorta (25). Similarly, in the present study, the rs3737787C allele represented a risk allele that was significantly associated with EOCAD in men; however, no significant correlation between the rs2516839 SNP and the risk of EOCAD was observed. Interestingly, the rs3737787C–rs2774276G–rs2516839A haplotype and the interaction between rs3737787C and rs2516839A alleles increased the risk of EOCAD in both men and women. Based on these results, rs2516839A may function as a risk allele synergistically with the rs3737787C allele to increase the risk of EOCAD in individuals carrying both alleles. In addition, we also noticed that the rs3737787T–rs2774276C–rs2516839A haplotype correlated with an increased risk of EOCAD in women. Furthermore, the rs3737787C–rs2774276G–rs2516839A-, rs3737787C–rs2774276G–rs2516839G-, and rs3737787T–rs2774276C–rs2516839A-smoking interactions increased the risk of EOCAD in men and/or women. These results suggested that smoking represents a risk factor that functions synergistically with several risk haplotypes to increase the risk of EOCAD, and the effect of haplotype–environment interactions on the risk of EOCAD is also sex-specific. Thus, in addition to the role of genetic factors, environmental factors such as smoking and the interactions between haplotype and smoking also significantly affect the risk of EOCAD. However, more basic research is still needed to clarify the molecular mechanism underlying the effects of these interactions on the risk of EOCAD.
In recent years, with more in-depth research, researchers have gradually realized that arteriosclerosis is actually a chronic inflammatory process characterized by strong immune activity (41). Previous studies have reported that USF1, a transcription factor, not only regulates glucose and lipid metabolism but also regulates immune and stress responses (50) and increases the expression of inflammatory factors by activating the nuclear factor kB (NF-kB) signaling pathway, ultimately leading to the occurrence of inflammatory diseases (51). As shown in the study by Ruuth et al. the inactivation of USF1 effectively promotes the cholesterol efflux from macrophages and reduces the accumulation of cholesterol in macrophages induced by inflammation to alleviate the progression of atherosclerotic lesions (52). Laurila et al. found that USF1 knockout effectively ameliorates insulin resistance, dyslipidemia, hepatic steatosis, obesity, and atherosclerosis induced by a high-fat diet in a mouse model (53). Furthermore, Colombo et al. noticed a significant increase in the expression of vascular endothelial growth factor (VEGF) in patients with systemic lupus erythematosus (SLE), and it correlates with accelerated atherosclerosis by functioning as a potent angiogenic and vasoactive molecule (54). Silencing of USF1 expression increases the expression of VEGF (55). In addition, Li et al. proved that inhibiting the expression of USF1 significantly reduces the expression of inflammatory factors such as IL-1β, TNF-α, and IL-6, thereby alleviating atherosclerotic inflammatory responses (56). Therefore, USF1 may be involved in atherosclerosis by regulating the expression of IL-1β, VEGF, TNF-α, and IL-6.
By querying the miRdSNP (57) and SNPinfo (58) databases, we found that the rs3737787 SNP is located in the binding site of miRNAs, including miR-148a-3p, miR-148b-3p, and miR-152-3p, in the 3′UTR of USF1. Bu et al. suggested that the rs1056628 SNP in the 3'UTR of the matrix metallopeptidase 9 (MMP9) gene alters the expression levels of the MMP9 mRNA and protein by mediating the binding of miR-491-5p to MMP9 and subsequently affecting the susceptibility of Chinese populations to idiopathic calcium kidney stones (59). Zhou et al. proved that SNPs in the 3′UTR of amyloid precursor protein (APP) alter the regulation of APP expression by miRNAs, including miR-144-3p, miR-101-3p, miR-383-5p, and miR-153-3p, and subsequently modulate the occurrence of Alzheimer's disease (60). However, researchers have not clearly determined whether the rs3737787 SNP alters USF1 expression levels. In the present study, significantly higher expression of the USF1 mRNA, TNF-α, IL-1β, and IL-6 was detected in patients with EOCAD than in control subjects; the expression levels of TNF-α, IL-1β, and IL-6 were positively correlated with the USF1 mRNA expression levels, and rs3737787T carriers maintained lower USF1 mRNA and IL-1β, TNF-α, and IL-6 expression levels than rs3737787T carriers. These results suggested that the rs3737787 SNP may affect the expression of inflammatory factors such as IL-1β, TNF-α, and IL-6 by mediating the expression of USF1, ultimately affecting the risk of EOCAD. The potential underlying molecular mechanism is that the rs3737787T allele enhances the binding of miR-148a-3p, miR-148b-3p, and miR-152-3p to USF1, thereby decreasing the expression of USF1. However, more in vivo and in vitro studies are needed to confirm these findings.
The current research may have several limitations. First, compared with some previous genetic studies analyzing large samples, the numbers of controls and patients with EOCAD were relatively small. Second, the vast majority of patients with EOCAD were taking some secondary prevention drugs for CAD that may alter blood lipid levels; thus, a calculation of the correlations between the rs3737787 SNP and serum lipid levels in the EOCAD group is inappropriate. Third, although fasting venous blood collected after a 12 h fast was used to detect serum lipid levels, subjects' long-term dietary habits might affect blood lipid levels and EOCAD risk, and the differences among the subjects' dietary habits were not considered in this study. Finally, the VEGF expression level was not detected in this study, and we have not yet clearly determined whether USF1 is involved in CAD by regulating VEGF expression.
Conclusion
The current study revealed that the USF1 rs3737787 SNP is one of the important genetic factors affecting susceptibility to EOCAD, and the potential mechanism may be that the USF1 rs3737787T allele alters the binding of miRNAs to the target gene USF1 to reduce USF1 mRNA expression, thus affecting blood lipid levels and the expression of inflammatory factors, including IL-1β, TNF-α, and IL-6, and ultimately altering the risk of EOCAD. The USF1 gene is expected to become a potential therapeutic target for the prevention and treatment of EOCAD.
Data Availability Statement
The datasets presented in this study can be found in online repositories. The names of the repository/repositories and accession number(s) can be found in the article/Supplementary Material.
Ethics Statement
The study design was approved by the Ethics Committee of Hunan Provincial People's Hospital (No: LL-20210615-144). The patients/participants provided their written informed consent to participate in this study.
Author Contributions
P-FZ conceived the study, participated in the design, performed the statistical analyses, and drafted the manuscript. Z-FZ conceived the study, participated in the design, and helped draft the manuscript. H-WP performed the epidemiological survey and collected the samples. L-ZC and PL performed the statistical analyses. PL helped to revise the manuscript. All authors read and approved the final manuscript.
Funding
This study was supported by the Key Research and Development Program of the Hunan Provincial Science and Technology Department (Grant No. 2019SK2021). The funding agency had no role in the design of the study and collection, analysis, and interpretation of data or in writing the manuscript.
Conflict of Interest
The authors declare that the research was conducted in the absence of any commercial or financial relationships that could be construed as a potential conflict of interest.
Publisher's Note
All claims expressed in this article are solely those of the authors and do not necessarily represent those of their affiliated organizations, or those of the publisher, the editors and the reviewers. Any product that may be evaluated in this article, or claim that may be made by its manufacturer, is not guaranteed or endorsed by the publisher.
Supplementary Material
The Supplementary Material for this article can be found online at: https://www.frontiersin.org/articles/10.3389/fcvm.2022.882728/full#supplementary-material
References
1. Ralapanawa U, Sivakanesan R. Epidemiology and the magnitude of coronary artery disease and acute coronary syndrome: a narrative review. J Epidemiol Glob Health. (2021) 11:169–77. doi: 10.2991/jegh.k.201217.001
2. Xuan C, Tian QW, Li H, Guo JJ, He GW, Lun LM. Serum fatty acids profile and association with early-onset coronary artery disease. Ther Adv Chronic Dis. (2021) 12:20406223211033102–02. doi: 10.1177/20406223211033102
3. Zhang QH, Yin RX, Chen WX, Cao XL, Wu JZ. TRIB1 and TRPS1 variants, G × G and G × E interactions on serum lipid levels, the risk of coronary heart disease and ischemic stroke. Sci Rep. (2019) 9:2376–76. doi: 10.1038/s41598-019-38765-7
4. Li KG, Yin RX, Huang F, Chen WX, Wu JZ, Cao XL. XKR6 rs7014968 SNP increases serum total cholesterol levels and the risk of coronary heart disease and ischemic stroke. Clin Appl Thromb Hemost. (2020) 26:1076029620902844. doi: 10.1177/1076029620902844
5. Ray KK, Kastelein JJ, Boekholdt SM, Nicholls SJ, Khaw KT, Ballantyne CM, et al. The ACC/AHA 2013 guideline on the treatment of blood cholesterol to reduce atherosclerotic cardiovascular disease risk in adults: the good the bad and the uncertain: a comparison with ESC/EAS guidelines for the management of dyslipidaemias 2011. Eur Heart J. (2014) 35:960–8. doi: 10.1093/eurheartj/ehu107
6. Can M, Acikgoz S, Mungan G, Ugurbas E, Ankarali H, Sumbuloglu V, et al. Is direct method of low density lipoprotein cholesterol measurement appropriate for targeting lipid lowering therapy? Int J Cardiol. (2010) 142:105–7. doi: 10.1016/j.ijcard.2008.11.141
7. Chapman MJ, Ginsberg HN, Amarenco P, Andreotti F, Borén J, Catapano AL, et al. Triglyceride-rich lipoproteins and high-density lipoprotein cholesterol in patients at high risk of cardiovascular disease: evidence and guidance for management. Eur Heart J. (2011) 32:1345–61. doi: 10.1016/S1567-5688(11)70033-2
8. Ferrieres J, Amber V, Crisan O, Chazelle F, Junger C, Wood D. Total lipid management and cardiovascular disease in the dyslipidemia international study. Cardiology. (2013) 125:154–63. doi: 10.1159/000348859
9. Gencer B, Koskinas KC, Räber L, Karagiannis A, Nanchen D, Auer R, et al. Eligibility for PCSK9 inhibitors according to American college of cardiology (ACC) and European society of cardiology/European atherosclerosis society (ESC/EAS) guidelines after acute coronary syndromes. J Am Heart Assoc. (2017) 6:e006537. doi: 10.1161/JAHA.117.006537
10. Namboodiri KK, Kaplan EB, Heuch I, Elston RC, Green PP, Rao DC, et al. The collaborative lipid research clinics family study: biological and cultural determinants of familial resemblance for plasma lipids and lipoproteins. Genet Epidemiol. (1985) 2:227–54. doi: 10.1002/gepi.1370020302
11. Spielmann N, Leon AS, Rao DC, Rice T, Skinner JS, Bouchard C, et al. CETP genotypes and HDL-cholesterol phenotypes in the HERITAGE family study. Physiol Genomics. (2007) 31:25–31. doi: 10.1152/physiolgenomics.00281.2006
12. Heller DA, de Faire U, Pedersen NL, Dahlén G, McClearn GE. Genetic and environmental influences on serum lipid levels in twins. N Engl J Med. (1993) 328:1150–6. doi: 10.1056/NEJM199304223281603
13. Brouwers MC, van Greevenbroek MM, Stehouwer CD, de Graaf J, Stalenhoef AF. The genetics of familial combined hyperlipidaemia. Nat Rev Endocrinol. (2012) 8:352–62. doi: 10.1038/nrendo.2012.15
14. Minicocci I, Prisco C, Montali A, Di Costanzo A, Ceci F, Pigna G, et al. Contribution of mutations in low density lipoprotein receptor (LDLR) and lipoprotein lipase (LPL) genes to familial combined hyperlipidemia (FCHL): a reappraisal by using a resequencing approach. Atherosclerosis. (2015) 242:618–24. doi: 10.1016/j.atherosclerosis.2015.06.036
15. Taghizadeh E, Mirzaei F, Jalilian N, Ghayour Mobarhan M, Ferns GA, Pasdar A. A novel mutation in USF1 gene is associated with familial combined hyperlipidemia. IUBMB Life. (2020) 72:616–23. doi: 10.1002/iub.2186
16. Taghizadeh E, Esfehani RJ, Sahebkar A, Parizadeh SM, Rostami D, Mirinezhad M, et al. Familial combined hyperlipidemia: an overview of the underlying molecular mechanisms and therapeutic strategies. IUBMB Life. (2019) 71:1221–29. doi: 10.1002/iub.2073
17. Eurlings PM, van der Kallen CJ, Geurts JM, van Greevenbroek MM, de Bruin TW. Genetic dissection of familial combined hyperlipidemia. Mol Genet Metab. (2001) 74:98–104. doi: 10.1006/mgme.2001.3232
18. Viscarra J, Sul HS. Epigenetic regulation of hepatic lipogenesis: role in hepatosteatosis and diabetes. Diabetes. (2020) 69:525–31. doi: 10.2337/dbi18-0032
19. Song Y, Li N, He L, Xun T, Chen D, Hu Y. A common polymorphism of upstream transcription factor 1 gene is associated with lipid profile: a study in Chinese type 2 Diabetes Families. Int J Biomed Sci. (2009) 5:305–12.
20. Pajukanta P, Lilja HE, Sinsheimer JS, Cantor RM, Lusis AJ, Gentile M, et al. Familial combined hyperlipidemia is associated with upstream transcription factor 1 (USF1). Nat Genet. (2004) 36:371–6. doi: 10.1038/ng1320
21. Coon H, Xin Y, Hopkins PN, Cawthon RM, Hasstedt SJ, Hunt SC. Upstream stimulatory factor 1 associated with familial combined hyperlipidemia, LDL cholesterol, and triglycerides. Hum Genet. (2005) 117:444–51. doi: 10.1007/s00439-005-1340-x
22. Holzapfel C, Baumert J, Grallert H, Müller AM, Thorand B, Khuseyinova N, et al. Genetic variants in the USF1 gene are associated with low-density lipoprotein cholesterol levels and incident type 2 diabetes mellitus in women: results from the MONICA/KORA augsburg case-cohort study, 1984-2002. Eur J Endocrinol. (2008) 159:407–16. doi: 10.1530/EJE-08-0356
23. Reiner AP, Carlson CS, Jenny NS, Durda JP, Siscovick DS, Nickerson DA, et al. USF1 gene variants, cardiovascular risk, and mortality in European Americans: analysis of two US cohort studies. Arterioscler Thromb Vasc Biol. (2007) 27:2736–42. doi: 10.1161/ATVBAHA.107.154559
24. Zeggini E, Damcott CM, Hanson RL, Karim MA, Rayner NW, Groves CJ, et al. Variation within the gene encoding the upstream stimulatory factor 1 does not influence susceptibility to type 2 diabetes in samples from populations with replicated evidence of linkage to chromosome 1q. Diabetes. (2006) 55:2541–8. doi: 10.2337/db06-0088
25. Laurila PP, Naukkarinen J, Kristiansson K, Ripatti S, Kauttu T, Silander K, et al. Genetic association and interaction analysis of USF1 and APOA5 on lipid levels and atherosclerosis. Arterioscler Thromb Vasc Biol. (2010) 30:346–52. doi: 10.1161/ATVBAHA.109.188912
26. Neri A, Scalzotto E, Corradi V, Caprara C, Salin A, Cannone M, et al. Acute rejection in kidney transplantation and the evaluation of associated polymorphisms (SNPs): the importance of sample size. Diagnosis. (2019) 6:287–95. doi: 10.1515/dx-2018-0110
27. Franceschini N, Carty C, Buzkova P, Reiner AP, Garrett T, Lin Y, et al. Association of genetic variants and incident coronary heart disease in multiethnic cohorts: the PAGE study. Circ Cardiovasc Genet. (2011) 4:661–72. doi: 10.1161/CIRCGENETICS.111.960096
28. An epidemiological study of cardiovascular cardiopulmonary disease risk factors in four populations in the People's Republic of China. Baseline report from the P.R.C.-U.S.A. collaborative study. People's republic of China–United States cardiovascular and cardiopulmonary epidemiology research group. Circulation. (1992) 85:1083–96. doi: 10.1161/01.CIR.85.3.1083
29. Yin RX, Aung LH, Long XJ, Yan TT, Cao XL, Huang F, et al. Interactions of several genetic polymorphisms and alcohol consumption on blood pressure levels. Biofactors. (2015) 41:339–51. doi: 10.1002/biof.1234
30. Yin RX, Wu DF, Wu JZ, Cao XL, Aung LHH, Miao L, et al. Interactions of several lipid-related gene polymorphisms and cigarette smoking on blood pressure levels. Int J Biol Sci. (2012) 8:685–96. doi: 10.7150/ijbs.4401
31. Guo T, Yin RX, Li H, Wang YM, Wu JZ, Yang DZ. Association of the Trp316Ser variant (rs1801690) near the apolipoprotein H (beta2-glycoprotein-I) gene and serum lipid levels. Int J Clin Exp Pathol. (2015) 8:7291–304.
32. Lee JC, Weissglas-Volkov D, Kyttälä M, Sinsheimer JS, Jokiaho A, de Bruin TW, et al. USF1 contributes to high serum lipid levels in Dutch FCHL families and U.S. whites with coronary artery disease. Arterioscler Thromb Vasc Biol. (2007) 27:2222–7. doi: 10.1161/ATVBAHA.107.151530
33. Onda Y, Takahagi K, Shimizu M, Inoue K, Mochida K. Multiplex PCR targeted amplicon sequencing (MTA-Seq): simple, flexible, and versatile SNP genotyping by highly multiplexed PCR amplicon sequencing. Front Plant Sci. (2018) 9:201. doi: 10.3389/fpls.2018.00201
34. Zheng PF, Yin RX, Wei BL, Liu CX, Deng GX, Guan YZ. Associations of PRKN-PACRG SNPs and G × G and G × E interactions with the risk of hyperlipidaemia. Sci Rep. (2020) 10:13010. doi: 10.1038/s41598-020-68826-1
35. Alberti KG, Zimmet PZ. Definition, diagnosis and classification of diabetes mellitus and its complications. Part 1: diagnosis and classification of diabetes mellitus provisional report of a WHO consultation. Diabet Med. (1998) 15:539–53. doi: 10.1002/(SICI)1096-9136(199807)15:7<539::AID-DIA668>3.0.CO;2-S
36. Whitworth JA. 2003 World Health Organization (WHO)/international society of hypertension (ISH) statement on management of hypertension. J Hypertens. (2003) 21:1983–92. doi: 10.1097/00004872-200311000-00002
37. Khounphinith E, Yin RX, Cao XL, Huang F, Wu JZ, Li H. TIMD4 rs6882076 SNP is associated with decreased levels of triglycerides and the risk of coronary heart disease and ischemic stroke. Int J Med Sci. (2019) 16:864–71. doi: 10.7150/ijms.31729
38. Liu CX, Yin RX, Shi ZH, Zheng PF, Deng GX, Guan YZ, et al. Associations between TUBB-WWOX SNPs, their haplotypes, gene-gene, and gene-environment interactions and dyslipidemia. Aging. (2021) 13:5906–27. doi: 10.18632/aging.202514
39. Shi YY, He L. SHEsis, a powerful software platform for analyses of linkage disequilibrium, haplotype construction, and genetic association at polymorphism loci. Cell Res. (2005) 15:97–8. doi: 10.1038/sj.cr.7290272
40. Libby P, Theroux P. Pathophysiology of coronary artery disease. Circulation. (2005) 111:3481–8. doi: 10.1161/CIRCULATIONAHA.105.537878
41. Li B, Li W, Li X, Zhou H. Inflammation: a novel therapeutic target/direction in atherosclerosis. Curr Pharm Des. (2017) 23:1216–27. doi: 10.2174/1381612822666161230142931
42. Kwiterovich PO Jr, Coresh J, Smith HH, Bachorik PS, Derby CA, Pearson TA. Comparison of the plasma levels of apolipoproteins B and A-1, and other risk factors in men and women with premature coronary artery disease. Am J Cardiol. (1992) 69:1015–21. doi: 10.1016/0002-9149(92)90856-T
43. Silbernagel G, Schottker B, Appelbaum S, Scharnagl H, Kleber ME, Grammer TB, et al. High-density lipoprotein cholesterol, coronary artery disease, and cardiovascular mortality. Eur Heart J. (2013) 34:3563–71. doi: 10.1093/eurheartj/eht343
44. Crouse JR, Parks JS, Schey HM, Kahl FR. Studies of low density lipoprotein molecular weight in human beings with coronary artery disease. J Lipid Res. (1985) 26:566–74. doi: 10.1016/S0022-2275(20)34343-1
45. Criqui MH, Heiss G, Cohn R, Cowan LD, Suchindran CM, Bangdiwala S, et al. Plasma triglyceride level and mortality from coronary heart disease. N Engl J Med. (1993) 328:1220–5. doi: 10.1056/NEJM199304293281702
46. Shekelle RB, Shryock AM, Paul O, Lepper M, Stamler J, Liu S, et al. Diet, serum cholesterol, and death from coronary heart disease. The western electric study. N Engl J Med. (1981) 304:65–70. doi: 10.1056/NEJM198101083040201
47. Zheng PF, Yin RX, Cao XL, Chen WX, Wu JZ, Huang F. Effect of SYTL3-SLC22A3 variants, their haplotypes, and g × e interactions on serum lipid levels and the risk of coronary artery disease and ischaemic stroke. Front Cardiovasc Med. (2021) 8:713068. doi: 10.3389/fcvm.2021.713068
48. Zheng PF, Yin RX, Deng GX, Guan YZ, Wei BL, Liu CX. Association between the XKR6 rs7819412 SNP and serum lipid levels and the risk of coronary artery disease and ischemic stroke. BMC Cardiovasc Disord. (2019) 19:202. doi: 10.1186/s12872-019-1179-z
49. Song Y, Li N, He L, Xun T, Chen D, Hu Y. A common polymorphism of upstream transcription factor 1 gene is associated with lipid profile: a study in Chinese type 2 diabetes families. Int J Biomed Sci. (2009) 5:305–12.
50. Corre S, Galibert MD. Upstream stimulating factors: highly versatile stress-responsive transcription factors. Pigment Cell Res. (2005) 18:337–48. doi: 10.1111/j.1600-0749.2005.00262.x
51. Song X, Zhu M, Li H, Liu B, Yan Z, Wang W, et al. USF1 promotes the development of knee osteoarthritis by activating the NF-κB signaling pathway. Exp Ther Med. (2018) 16:3518–24. doi: 10.3892/etm.2018.6608
52. Ruuth M, Soronen J, Kaiharju E, Merikanto K, Perttilä J, Metso J, et al. USF1 deficiency alleviates inflammation, enhances cholesterol efflux and prevents cholesterol accumulation in macrophages. Lipids Health Dis. (2018) 17:285–85. doi: 10.1186/s12944-018-0930-2
53. Laurila PP, Soronen J, Kooijman S, Forsström S, Boon MR, Surakka I, et al. USF1 deficiency activates brown adipose tissue and improves cardiometabolic health. Sci Transl Med. (2016) 8:323ra13. doi: 10.1126/scitranslmed.aad0015
54. Colombo BM, Cacciapaglia F, Puntoni M, Murdaca G, Rossi E, Rodriguez G, et al. Traditional and non traditional risk factors in accelerated atherosclerosis in systemic lupus erythematosus: role of vascular endothelial growth factor (VEGATS study). Autoimmun Rev. (2009) 8:309–15. doi: 10.1016/j.autrev.2008.10.002
55. Kim KC, Yun J, Son DJ, Kim JY, Jung J-K, Choi JS, et al. Suppression of metastasis through inhibition of chitinase 3-like 1 expression by miR-125a-3p-mediated up-regulation of USF1. Theranostics. (2018) 8:4409–28. doi: 10.7150/thno.26467
56. Li P, Xing J, Zhang J, Jiang J, Liu X, Zhao D, et al. Inhibition of long noncoding RNA HIF1A-AS2 confers protection against atherosclerosis via ATF2 downregulation. J Adv Res. (2020) 26:123–35. doi: 10.1016/j.jare.2020.07.015
57. Bruno AE, Li L, Kalabus JL, Pan Y, Yu A, Hu Z. miRdSNP: a database of disease-associated SNPs and microRNA target sites on 3'UTRs of human genes. BMC Genomics. (2012) 13:44. doi: 10.1186/1471-2164-13-44
58. Xu Z, Taylor JA. SNPinfo: integrating GWAS and candidate gene information into functional SNP selection for genetic association studies. Nucleic Acids Res. (2009) 37:W600–5. doi: 10.1093/nar/gkp290
59. Bu Q, Zhu Y, Chen QY, Li H, Pan Y. A polymorphism in the 3'-untranslated region of the matrix metallopeptidase 9 gene is associated with susceptibility to idiopathic calcium nephrolithiasis in the Chinese population. J Int Med Res. (2020) 48:300060520980211. doi: 10.1177/0300060520980211
Keywords: early-onset coronary artery disease, USF1, single-nucleotide polymorphism, lipids, inflammatory factors
Citation: Zheng P-F, Chen L-Z, Pan H-W, Liu P and Zheng Z-F (2022) Effects of USF1 SNPs and SNP–Environment Interactions on Serum Lipid Profiles and the Risk of Early-Onset Coronary Artery Disease in the Chinese Population. Front. Cardiovasc. Med. 9:882728. doi: 10.3389/fcvm.2022.882728
Received: 25 February 2022; Accepted: 16 May 2022;
Published: 15 June 2022.
Edited by:
Chayakrit Krittanawong, New York University, United StatesReviewed by:
Sabba Mehmood, National University of Medical Sciences (NUMS), PakistanEray Metin Guler, University of Health Sciences, Turkey
Alexander Lykov, Novosibirsk Research Tuberculosis Institute MH RF, Russia
Mitesh Dwivedi, Uka Tarsadia University, India
Fahimeh Kazemi, Alzahra University, Iran
Giuseppe Murdaca, University of Genoa, Italy
Willians Fernando Vieira, Massachusetts General Hospital and Harvard Medical School, United States
Thiago Britto-Borges, Heidelberg University Hospital, Germany
Isela Juarez-Rojop, Universidad Juárez Autónoma de Tabasco, Mexico
Copyright © 2022 Zheng, Chen, Pan, Liu and Zheng. This is an open-access article distributed under the terms of the Creative Commons Attribution License (CC BY). The use, distribution or reproduction in other forums is permitted, provided the original author(s) and the copyright owner(s) are credited and that the original publication in this journal is cited, in accordance with accepted academic practice. No use, distribution or reproduction is permitted which does not comply with these terms.
*Correspondence: Peng Liu, ying_lpxm@163.com; Zhao-Fen Zheng, zhaofenz@foxmai.com