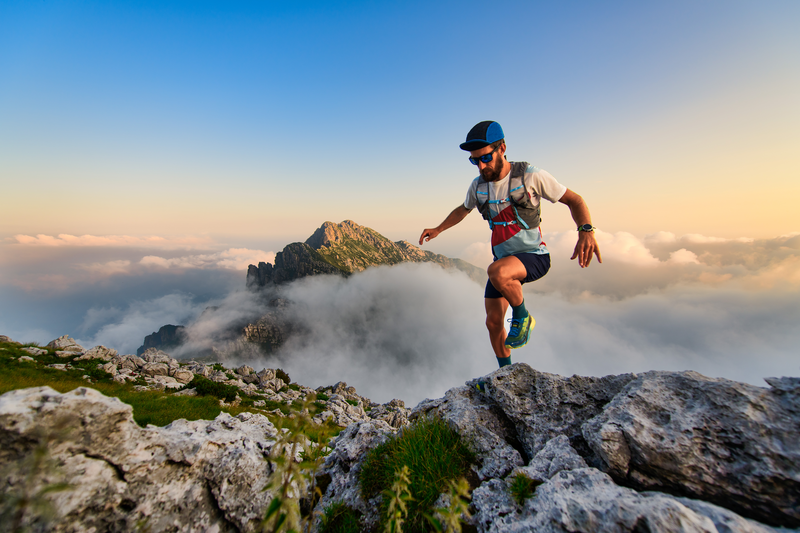
94% of researchers rate our articles as excellent or good
Learn more about the work of our research integrity team to safeguard the quality of each article we publish.
Find out more
REVIEW article
Front. Cardiovasc. Med. , 07 April 2022
Sec. General Cardiovascular Medicine
Volume 9 - 2022 | https://doi.org/10.3389/fcvm.2022.882027
This article is part of the Research Topic Myocarditis and Inflammatory Cardiomyopathies: Diagnosis, treatment and future directions View all 11 articles
In myocarditis caused by various etiologies, activated immune cells and the immune regulatory factors released by them play important roles. But in this complex microenvironment, non-immune cells and non-cardiomyocytes in the heart, such as cardiomyocytes (CMs), cardiac fibroblasts (CFs) and endothelial cells (ECs), play the role of “sentinel”, amplify inflammation, and interact with the cardiomyocytes. The complex interactions between them are rarely paid attention to. This review will re-examine the functions of CFs and ECs in the pathological conditions of myocarditis and their direct and indirect interactions with CMs, in order to have a more comprehensive understanding of the pathogenesis of myocarditis and better guide the drug development and clinical treatment of myocarditis.
The pathological standard of myocarditis refers to the infiltration of inflammatory cells, with or without myocardial necrosis in the staining of heart tissue sections according to the Dallas definition (1). A recent study estimates that the global prevalence of myocarditis is ~22 out of 100,000 patients per year (2). A variety of infectious pathogens, systemic diseases, drugs, and toxins can cause the disease (3). Myocarditis can appear with a variety of different characteristics, from mild symptoms of chest pain to ventricular arrhythmia or fatal cardiogenic shock (3). Inflammatory cells and inflammatory factors in innate immunity and adaptive immunity play important roles in the occurrence and development of myocarditis, and there has been review (4) clarifying the roles of various types of immune cells. However, the vast majority of the heart is non-inflammatory cell. As our understanding of the human immune response continues to increase, many cell types, such as CFs, ECs that were initially thought to be just bystanders have been proven to play vital roles in the inflammatory process and even determine the direction and intensity of the immune response. However, there is little research on the role of non-inflammatory cells in the process of myocarditis. Thus, in this review, we will briefly delineate the role of non-inflammatory cells, non-CMs including CFs and ECs, and focus on the everlasting cross-talk between CFs, ECs and CMs in the progression of cardiovascular diseases.
Fibroblasts in the adult mouse heart make up about 15% of the non-myocytes in the heart (5). The basic function of cardiac CFs is to synthesize a collagen-rich ECM network to provide structural integrity and biomechanical signals (6). They are dynamic participants in ventricular physiology and pathophysiology. CFs can be activated either by their infection or invasion (7, 8) or by heart stress. Activated CFs will secret inflammatory factors, chemokines, or other immunomodulatory substances and produce large amounts of ECM. In myocarditis, virus infection or cytokines induces fibroblast activation state and then CFs function through virus transmission, cell damage, chemokines, and inflammatory factors production, making heart inflammation to keep getting worse. At the same time, the extracellular matrix produced by CFs promotes myocarditis from acute inflammatory infiltration to the chronic phase (Figure 1).
Figure 1. Fibroblast and endothelial cell activation. Fibroblasts are activated in viral infection or in the environment containing cytokines. Then they produce pro-inflammatory factors and chemokines such as TNF-α, IL-6, MCP-1, IFN-β, CCL11, CCL12. They can also produce MMP family proteins to regulate cardiac interstitial fibrosis. Under the stimulation of these microenvironments, endothelial cells produce adhesion molecules to regulate the adhesion and infiltration of inflammatory cells. The participation of fibroblasts and endothelial cells promotes more severe cardiac inflammatory infiltration and more severe fibrosis.
CFs can directly serve as the host of the virus. Lindner et al. demonstrated that when both CMs and CFs are infected with coxsackie virus B3 (CVB3), the viral replication within CFs increases by 10-folds, indicating that they play a key role in promoting viral load in myocarditis (9). Within 9 h after infection, the CMs network loses its ability to contract spontaneously, and then decomposes and is replaced by overgrown CFs that survive from the infection (10).
Infected CFs can continue to damage the heart function at different stages of myocarditis. In the acute phase of viral myocarditis, after CFs are attacked and die, the virus in the cell continues to attack various types of cells, thereby making it a storage and transmission reservoir for the virus in the infection (11). While in the chronic phase, the virus that resides in CFs may be an important cause of persistent inflammation, heart dilation, and even heart failure. In addition, viral infection can cause damage to CFs and their release of damage-associated molecular patterns (DAMPs), such as HGMB1 (12).
Although CFs are not a component of the immune system, they play an important role in the occurrence and development of inflammation. The expression of chemokines has been found in a variety of disease processes related to tissue damage and leukocyte recruitment.
Eosinophils are multifunctional granulocytes that help trigger and regulate inflammation. It is reported that eosinophils aggravate the pathological severity and mortality of eosinophilic myocarditis (13). In general, the transport of eosinophils to the heart in eosinophilic myocarditis depends on the expression of the chemokine receptor CCR3 (14) and its ligands CCL11, CCL24, and CCL26 in the mouse model and the heart of patients with eosinophilic myocarditis. However, CFs produce CCL11 under the regulation of two cytokines IL-4 and IL-13 ADDIN EN.CITE during myocarditis (15).
IL-17 is involved in the pathogenesis of autoimmune myocarditis, and it has been shown that neutralizing IL-17 can reduce the severity of myocarditis (16). In vitro, the stimulation with TNF-α, IL-1β, and IL-17 can induce CFs to produce CCL20. CCL20 promotes adhesion of Th17 on endothelium and induces Th17 cell migration (17), thereby producing more IL-17 to form a vicious circle. What's more, IL-17A acts on the differentiation of Ly6C (low) monocytes into macrophages through CFs-derived GM-CSF in vitro, indicating that CFs promote monocyte differentiation and proliferation, and regulate monocytes and monocyte-derived macrophages phenotype and function (18).
The continuous expression of cytokines was observed in the mouse model of dilated cardiomyopathy (DCM) after myocarditis. Compared with other cytokines, the expression of the IL-1β gene in the chronic phase was relatively higher, and it was related to the ratio of heart weight/body weight and the degree of fibrotic lesions (19). IL-1β secreted by CFs initiates the inflammatory process and attracts proinflammatory immune cells. In addition, CFs-secreted IL-1β leads to the maintenance of inflammation in the later stage of wound healing (20).
Overall, in myocarditis caused by a variety of factors, CFs can participate in the entire inflammatory reaction process and even the chronic phase by secreting chemokines or inflammatory factors. Most of the inflammation involved in CFs is harmful, but there are also beneficial parts, so it is particularly important to find the reason for these differential effects and carry out corresponding interventions.
The ECM components in the interstitium of the heart form a complex network structure, which provides structural support for several different cell types, and integrates extracellular signals and cellular responses (21). The ECM is a highly dynamic structure that exists in all tissues and undergoes continuous controlled remodeling. In the body, CFs are considered to be a key regulator of ECM structure. CFs residing in the heart are not only the main producer of ECM components but also the main source of matrix metalloproteinases (MMPs) and tissue inhibitors of MMPs (TIMPs). In inflammatory heart disease, cytokines, growth factors can change ECM components and the production of MMPs and TIMPs by stimulating the migration and proliferation of CFs or mediating the interaction between CFs and other cell types (22).
For example, TNFα and IL-1β lead to increased expression of MMP-2, MMP-9, and MMP-13 in CFs (23) which cause ECM remodeling. On the one hand, prolonged activation time of CFs and progressive fibrosis can lead to further deformation of the tissue structure and deterioration of heart function. ECM remodeling during myocarditis can cause arrhythmia and even heart failure. On the other hand, ECM components may adsorb parasite antigens (24) and cytokines (25) that may contribute to the establishment and continuation of inflammation.
The regulation of matrix metalloproteinases (MMPs) in the acute and chronic phases of viral myocarditis and its role in myocardial interstitial remodeling have been reviewed (26) in detail. Therefore, adjusting the function of CFs to regulate cardiac inflammation and cardiac remodeling is gradually turning into a better solution.
ECs are arranged in the circulatory system and are essential for maintaining and regulating vascular homeostasis (27) by protecting material transport, controlling vascular permeability, and regulating vascular tension (28). They are the largest cell population in non-cardiomyocytes of the adult mouse heart, accounting for about 60% (5). Resting ECs isolate leukocyte-interacting proteins in specialized secretory vesicles (29), so they cannot interact with white blood cells. In addition, resting ECs also inhibit the transcription of other adhesion molecules, such as E-selectin, vascular cell adhesion molecule 1 (VCAM1), and to a large extent inhibit the transcription of intercellular adhesion molecule 1 (ICAM1) and pro-inflammatory cytokines (30).
However, under the influence of certain factors, ECs can be transformed into an activated state. In DCM developed from autoimmune myocardial inflammation, it is shown that endothelial activation in organ-specific diseases affects the function of blood vessels throughout the body. Vallbracht et al. proved this and found that patients with myocardial inflammation showed impaired endothelial function of radial artery, and these patients have ruled out the risk factors of classic arterial damage, such as coronary artery disease, severe left ventricular failure, diabetes or atherosclerosis (31). For myocarditis, myocardial inflammatory infiltration, endothelial activation, direct viral toxicity, and circulating cytokines cause endothelial dysfunction in patients with persistent myocardial viruses (32). The persistence of the myocardial virus can independently cause endothelial dysfunction. For patients with myocardial leukocyte infiltration, endothelial dysfunction is more obvious (32). Nearly half of patients with DCM have increased T lymphocyte density and increased immune activation of ECs and mesenchymal cells in cardiac biopsies (33).
ECs not only act as a transport device for immune cells in circulation, forming a mechanical barrier to resist invaders, but also produce chemokines, interleukins, interferons, and growth factors through paracrine after they are activated. In addition, they can induce the expression of adhesion molecules such as E-selectin, P-selectin, ICAM, or VCAM to attract and transfer immune cells to inflammatory sites (34) (Figure 1).
Many pathogenic microorganisms not only infect CMs and CFs, but also the ECs. Among T. cruzi and more than 20 viruses related to myocarditis, they are known to infect human and/or animal ECs (35). Parvovirus B19 is considered the pathogen in most cases of chronic myocarditis (36). And PVB19-related inflammatory cardiomyopathy is characterized by infection of the ECs of the heart in small arteries and veins (37).
Bültmann et al. reported (38) a patient with severe myocarditis, whose radioactive in situ hybridization detected the viral genome in ECs, but did not detect the viral genome in CMs or other tissue components. What's more, humans or mice infected with PVB19 show more particles related to endothelial cell damage in the blood circulation (39). Due to the high viral load in cardiac ECs, PVB19 infection of ECs is sufficient to induce coronary microcirculation damage and secondary CMs necrosis (40).
However, ECs, like a barrier that the virus needs to cross, can to a certain extent provide a buffer for the time when the heart damage occurs so that the immune system can have a longer time to exert a stronger immune effect before the virus invades the CMs (41).
Adhesion molecules (42) are a class of membrane receptors with various functions, including cell migration, cell-cell interaction, and cell-to-extracellular matrix adhesion. They are involved in various processes such as growth, differentiation, migration, and apoptosis. The adhesion molecules VCAM-1, ICAM-1, and E-selectin expressed on the vascular endothelium act as ligands for the counter-receptors of circulating inflammatory cells (43).
The expression of endothelial cell surface markers is dynamic and is affected by circulating cytokines and other stimuli (44). The inflammation state of endomyocardial biopsy in patients with dilated cardiomyopathy was evaluated by immunohistochemistry. It was found that 45.8% of patients had endothelial inflammation activation, which was manifested by increased expression of at least three adhesion molecules (Class I and Class II HLA, ICAM-1, VCAM-1) (45).
The increased expression of endothelial adhesion molecules in the myocardium of patients with infectious myocarditis may be related to pro-inflammatory cytokines. TNFα and IL-1β increase the expression of adhesion molecules ICAM-1, VCAM-1 and E-selectin on the ECs of the human coronary artery and cardiac microvascular system (46). Cytokines and chemokines driven by T. cruzi can also regulate adhesion molecules like VCAM-1 and ICAM-1 on the ECs of target tissues, and play a key role in cell recruitment (24).
The functions of various cells are not independent, they share the characteristics of repetitiveness and mutual reinforcement or containment. CMs are the most important cell type for the heart to perform biological functions. So we focus on the interaction between CMs and CFs, and CMs and ECs (Figure 2). On the one hand, these interactions promote the heart's adaptive response to internal and external stimuli and compensate or overcome these pressures. On the other hand, these interactions can also cause pathological remodeling related to heart disease, speed up the disease process, and lead to heart failure and cardiogenic death. Therefore, the study of the interaction between cells will help us to employ a synergistic protective effect between cells and avoid the expansion of malignant events.
Figure 2. Fibroblasts, endothelial cells and cardiomyocytes interaction diagram. Fibroblasts, endothelial cells can interact with cardiomyocytes through mechanical coupling and electrical coupling. They also act through paracrine effects and/or cell-secreted vesicles or exosomes. Cardiomyocytes secrete pro-inflammatory factors such as TNF-α, IL-1β, and IL-6 through paracrine effects to act on fibroblasts, and they can also deliver intracellular miRNAs through exosomes, such as miR92a, miR195, and miR378. These mediators can affect fibroblast proliferation, migration, differentiation and matrix production. Fibroblasts can also secrete factors such as TGF-β through paracrine action or deliver miRNAs into cardiomyocytes through exosomes, which affect cardiomyocyte contraction and cardiac hypertrophy. Similarly, endothelial cells can secrete effector molecules such as IL-8 through paracrine effects, and can also carry ROS through exosomes, thereby affecting various functions of cardiomyocytes. In contrast, cardiomyocytes can affect endothelial cell migration, proliferation, and angiogenesis by secreting exosomes carrying molecules such as miR-21.
There are mechanical coupling and electrical coupling (47) between CMs, CFs, and ECs. This kind of spatial connection enables the direct exchange of information between each other. Research shows blocking contact between CMs and CFs using antibodies against cardiac fibroblast plasma membrane protein or connexin will inhibit cardiomyocyte adhesion and reduce IL-6 production, but will not reduce TNFα production (48). However, the direct interaction is very dependent on the tight connection in space. Compared with the indirect action, its range of action is smaller. Therefore, we mainly discuss the indirect interaction between cells.
The interaction between CFs and CMs leads to paracrine of regulatory factors, which in turn affects cardiac conductivity, and hypertrophy, contraction (49), and apoptosis of CMs. While CMs change the proliferation, migration, differentiation, and matrix production capacity of CFs through paracrine (Table 1).
For example, TGF-β1 is the prototype of a large family of structurally related secreted dimeric proteins. It is pleiotropic and plays an important role in intercellular signal transduction (64). Disturbance of TGF-β action can lead to pathological conditions, including cardiovascular disease (65), fibrotic disease, and cancer (66). When subjected to mechanical stretching, TGF-β secreted by CMs and CFs induces the growth response of CMs and CFs in an autocrine/paracrine manner. In addition, the inflammatory factors released by immune cells will also promote the release of TGF-β from CFs. The increase of TGF-β will promote the protein synthesis rate of static CMs (67) and change the function of CMs.
In cardiac remodeling, myofibroblasts can induce CMs hypertrophy through cross-talk between CFs and CMs (68). IL-6 signaling in CMs co-cultured with CFs is activated to promote CMs hypertrophy (69). In addition, when CMs are co-cultured with cardiac CFs, the production of tumor necrosis factor (TNF)-α is also upregulated (48).
Similarly, CMs also have a paracrine effect on CFs. For example, the pro-inflammatory cytokine hypoxia-induced mitotic factor (HIMF) was overexpressed in CMs in vitro, and the cell supernatant was used as a conditioned medium and co-cultured with CFs. The results (52) show that CMs induce CFs migration, proliferation, and myofibroblast differentiation by paracrine IL-6.
Since ECs and CMs are relatively close in space, they can conduct direct cell signal transduction and two-way communication through paracrine. The interaction between CMs and ECs is essential for heart development, postnatal function, and heart repair (70). ECs influence cardiomyocyte morphology, contractility, apoptosis, oxidative stress, cell activity, and release of pro-inflammatory factors in a paracrine manner. At the same time, CMs can promote endothelial cell migration, proliferation, and blood vessel formation in the same way (Table 2).
For example, Endothelin-1 (ET-1) is described as a 21 amino acid peptide produced by ECs and is the most effective known endogenous vasoconstrictor. CMs mainly express ETA receptors. Under normal physiological conditions, ET-1 promotes the contraction of CMs. However, under pathological conditions, increased ET-1 can damage the contractile function of CMs through ETA receptors (81).
In addition to the direct effect of substances secreted by one type of cell on another type of cell, the substances secreted by cells can affect them through synergistic or antagonistic effects with other cells. Pummerer et al. showed that normal hearts are not easily affected by T cells that react with myosin (82). In myocarditis, the cardiac mesenchymal cells expressing MHC class II are significantly increased and the expression of endothelial ICAM-1 is strongly upregulated, making the heart vulnerable to passive metastasis and myosin responds to T cell attack.
In general, ECs and CMs can communicate extensively through secreted substances or “permissible action”, which is essential for the overall functioning of the heart.
The above-mentioned paracrine substances can play a corresponding role through the binding of receptors on the corresponding target cells. These substances are of fewer types and have a limited range of action. However, the production of EVs, as a new pathway of communication, enriches the medium of communication and expands the distance of interaction between cells.
EVs are cell-derived membrane structures that are secreted or shed from the plasma membrane after fusion of the endosome with the plasma membrane. Exosomes (83), as the current hottest research type in EVs, are membrane vesicles that carry biologically active molecules such as proteins, lipids, messenger RNA (mRNA), and micro RNA (miRNA) which mediate intercellular communication between different cell types within the body, thereby affecting normal and pathological states. And they have become a key medium for intercellular communication. Exosomes can be induced by many cell events including cell death, hypoxia, stress, oncogene expression, differentiation, and viral infection (84). They deliver downstream signals in the following three ways: cell internalization, direct fusion with the cell membrane, and receptor-ligand interaction (85). All major heart cell types including CMs, ECs, and CFs release exosomes to regulate cell function. What is special is that the protein content of cardiac exosomes is significantly different from other types of exosomes in the literature, which contain cytosolic, sarcomeric, and mitochondrial proteins (86).
For example, CFs-derived exosomes mediate crosstalk between CFs and CMs and lead to cardiomyocyte hypertrophy (60) via internal miRNA. Exosomes isolated from hypoxic CMs promote CFs apoptosis and inhibit their proliferation, migration, and invasion (87) via internal lncRNA.
In addition, exosomes can transfer genetic material between cells. CVB can cause a variety of life-threatening inflammatory diseases. It is a member of the picornaviridae family and is a non-enveloped single-stranded RNA virus related to human diseases including myocarditis (88) and pancreatitis. Although it is well-known that CVB spreads through cytolysis, recent reports reveal a second route in which CVB can be encapsulated in host membrane components and escape the cell in the form of exosome-like particles (89). Recent evidence suggests that CVB and other picornaviruses hijack the host membrane and obtain the envelope. Obtaining the envelope may provide unique benefits for CVB virus particles, such as resistance to neutralizing antibodies and effective non-lytic virus transmission (90). While EVs participate in the transmission of HSV-1, in which microvesicles (MVs) containing virus particles released by infected cells are endocytosed by immature cells, leading to productive infection (91).
As mentioned above, fibroblasts are activated under the influence of viruses or cytokines. Therefore, reducing the invasion of viruses in fibroblasts and reducing the stimulation of fibroblasts by other inflammatory factors are important means to prevent their activation. The study by Heim et al. showed that Ribavirin, the antiviral drug, has high cell-specific activity in fibroblasts, and it can reduce the viral load in fibroblasts (92). Moreover, Kania et al. demonstrated TGF-β-mediated myofibroblast differentiation and progression of myocardial fibrosis in human and mouse myocarditis (93). Anti-TGF-β treatment prevented myocardial fibrosis in mice with autoimmune myocarditis. In addition, IL-1 can prompt uninfected fibroblasts to enhance the induction of inflammation-related genes in the presence of infected fibroblasts. Blockade of IL-1 receptor signaling with an IL-1 receptor antagonist and knockdown of IL-1 receptor using siRNA abolished cytokines in human fibroblasts during CVB3 infection (94).
Fibroblasts can produce a variety of inflammatory factors and chemokines after activation. Among them, CCL20 is a chemokine that can effectively recruit Th17 cells. The neutralization of CCL20 can effectively reduce cardiac inflammation (17). In addition, blocking HMGB1 secreted by fibroblasts can effectively improve cardiac fibrosis in EAM mice (12). Studies have shown that fibroblasts in the heart are a heterogeneous cell population, in which Sca-1+ cardiac fibroblasts are not only efficient GM-CSF producers, but also have certain plasticity (95). They have a certain degree of plasticity to alter their own cytokine production under the influence of local microenvironment. Therefore, exploring the characteristics of different subpopulations of fibroblasts in myocarditis and finding targeted interventions based on their characteristics can better inform the treatment of patients with myocarditis.
After endothelial cells are infected by virus, the expressions of cell adhesion molecules such as ICAM-1, integrin αvβ3, P- and E-selectin are increased and the release of coagulation factor-von Willebrand factor (VWF) is also increased (96). The increase of adhesion molecules makes the circulating inflammatory cells more likely to adhere to blood vessels, while the increase of coagulation factors aggravates the retention of inflammatory cells and affects the execution of vascular function through the coagulation pathway. Telbivudine, an antiviral drug clinically used to treat hepatitis B, exerts endothelial protection in HPV B19 infected endothelial cells and can improve HPV B19-associated inflammatory cardiomyopathy (97). In addition, CVB3 virus can also infect endothelial cells through Coxsackie and adenovirus receptor (CAR). Bosentan and valsartan are angiotensin II receptor antagonists for the treatment of hypertension. In CVB3-induced myocarditis, bosentan and valsartan treatment can dose-dependently downregulate the levels of CAR mRNA and protein which is required for viral entry into cells. Reduction of CAR significantly reduced viral load in CVB3-infected Human Umbilical Vein Endothelial Cells (HUVECs) (98, 99). What's more, House Dust Mite Specific Antibodies, which can bind to cardiac vascular endothelial cells to weaken their barrier function, have been detected in the serum of patients with myocarditis. Since barrier dysfunction may lead to local tissue inflammation, removal of this antibody may be effective in relieving myocarditis (100). Moreover, compared with healthy rats, rats with autoimmune myocarditis exhibited marked mobilization of Endothelial Progenitor Cells (EPCs) from the bone marrow to the periphery, and their ability to adhere to fibronectin, mature endothelial cells, and cultured cardiomyocytes was significantly reduced. Metastasis of splenocyte-derived EPCs partially attenuates myocardial injury induced by experimental myocarditis. Of these, the transformation of EPCs into resident mature endothelial cells appears to be the most important reason (101). Therefore, augmentation of damaged endothelial cells by cell therapy could also serve as a promising therapeutic approach.
Among endothelial cell-derived proteins with altered secretion or expression in myocarditis, depletion of functional selectin ligand expression reduces the migration of pathogenic CD8+ T cells to the heart, which in turn reduces cardiac inflammatory infiltration and cardiomyocyte damage in myocarditis. In addition, the expression of MHC class II in myocarditis endothelial cells with dilated cardiomyopathy is increased (102). Studies have shown that loss of MHC class II expression on endothelial cells protects mice from experimental autoimmune myocarditis (103). Cyclosporine and statins reduce endothelial MHC class II levels and may prevent diffuse endothelial dysfunction in myocarditis (98). What's more, the antisense ICAM-1 inhibitor alicaforsen has been studied in four Phase 2 studies in ulcerative colitis (UC), and analysis suggests that alicaforsen enema may provide an effective and potential lasting response (104). However, it still needs more evidence to confirm whether these drugs can affect the expression of ICAM-1 in endothelial cells in myocarditis and exert their therapeutic effect on myocarditis.
In conclusion, the factors that cause the activation of fibroblasts and endothelial cells or the active substances produced by fibroblasts or endothelial cells that affect the occurrence and development of myocarditis can be used as potential targets for clinical treatment of myocarditis.
CFs and ECs can be infected by pathogens and release immunologically active substances to participate in the inflammatory response. In addition, CFs affect the fibrosis process and long-term prognosis of the heart through ECM changes. At the same time, through the interaction with the CMs, they may play a synergistic or antagonistic effect during the disease process.
However, there are still many problems. First of all, many of the interactions between cells are experiments conducted in vitro, such as the use of conditioned media or co-cultivation. It's difficult to perform in vivo experiments due to technical problems. However, the in vitro environment is quite different from the in vivo environment which has a more abundant neurohumoral regulation process.
In addition, a problem faced by cell experiments under in vitro conditions is that the conditioned medium may not be able to reproduce the observed effect. The composition and content of the conditioned medium will vary with the intervention environment, such as cell content, cell viability, cell intervention time, and the selection of cell lines, etc. That will make the results unstable.
Finally, the body is in a complex internal environment. The virus infection or the release of inflammatory factors both have beneficial and harmful aspects. This is closely related to the time and space relationship between cells. How to balance the pros and cons, and how to determine the correct intervention intensity, intervention time, and intervention methods is an important issue currently facing.
In general, a correct understanding of the function and mode of action of various cells, especially the non-inflammatory cells of the heart, such as CFs and ECs, will be more conducive to our understanding of diseases. And it contributes to the development of new myocarditis pathogenesis and corresponding treatment strategies.
DWW conceived this review, organized, and critically revised the manuscript. YX did major work of writing the manuscript. CC made contributions to editing the manuscript. ZW gave final approval of the version to be published. All authors were fully aware of and approved the submission of this manuscript.
This work was supported by grants from the National Natural Science Foundation of China (81630010 and 81790624 to DWW, 82070395 to ZW), the Natural Science Foundation of Hubei Province (2020CFA016 to CC), and the Tongji Hospital Clinical Research Flagship Program (2019CR207 to DWW). No funding bodies had any role in the program design, preparation of the manuscript, or decision to publish.
The authors declare that the research was conducted in the absence of any commercial or financial relationships that could be construed as a potential conflict of interest.
All claims expressed in this article are solely those of the authors and do not necessarily represent those of their affiliated organizations, or those of the publisher, the editors and the reviewers. Any product that may be evaluated in this article, or claim that may be made by its manufacturer, is not guaranteed or endorsed by the publisher.
1. Aretz HT, Billingham ME, Edwards WD, Factor SM, Fallon JT, Fenoglio JJ, et al. Myocarditis. A histopathologic definition and classification. Am J Cardiovasc Pathol. (1987) 1:3–14.
2. Vos T, Barber RM, Bell B, Bertozzi-Villa A, Biryukov S, Bolliger I, et al. Global, regional, and national incidence, prevalence, and years lived with disability for 301 acute and chronic diseases and injuries in 188 countries, 1990-2013: a systematic analysis for the Global Burden of Disease Study 2013. Lancet. (2015) 386:743–800. doi: 10.1016/S0140-6736(15)60692-4
3. Caforio AL, Pankuweit S, Arbustini E, Basso C, Gimeno-Blanes J, Felix SB, et al. Current state of knowledge on aetiology, diagnosis, management, and therapy of myocarditis: a position statement of the European Society of Cardiology Working Group on Myocardial and Pericardial Diseases. Eur Heart J. (2013) 34:2636–48:48a–d. doi: 10.1093/eurheartj/eht210
4. Hua X, Song J. Immune cell diversity contributes to the pathogenesis of myocarditis. Heart Fail Rev. (2019) 24:1019–30. doi: 10.1007/s10741-019-09799-w
5. Pinto AR, Ilinykh A, Ivey MJ, Kuwabara JT, D'Antoni ML, Debuque R, et al. Revisiting cardiac cellular composition. Circ Res. (2016) 118:400–9. doi: 10.1161/CIRCRESAHA.115.307778
6. Hynes RO. The extracellular matrix: not just pretty fibrils. Science. (2009) 326:1216–9. doi: 10.1126/science.1176009
7. Shen J, Zhai J, You Q, Zhang G, He M, Yao X, et al. Cancer-associated fibroblasts-derived VCAM1 induced by H. pylori infection facilitates tumor invasion in gastric cancer. Oncogene. (2020) 39:2961–74. doi: 10.1038/s41388-020-1197-4
8. Montes-Gómez AE, García-Cordero J, Marcial-Juárez E, Shrivastava G, Visoso-Carvajal G, Juárez-Delgado FJ, et al. Crosstalk between dermal fibroblasts and dendritic cells during dengue virus infection. Front Immunol. (2020) 11:538240. doi: 10.3389/fimmu.2020.538240
9. Lindner D, Li J, Savvatis K, Klingel K, Blankenberg S, Tschöpe C, et al. Cardiac fibroblasts aggravate viral myocarditis: cell specific coxsackievirus B3 replication. Mediators Inflamm. (2014) 2014:519528. doi: 10.1155/2014/519528
10. Kandolf R, Canu A, Hofschneider PH. Coxsackie B3 virus can replicate in cultured human foetal heart cells and is inhibited by interferon. J Mol Cell Cardiol. (1985) 17:167–81. doi: 10.1016/S0022-2828(85)80019-5
11. Werk D, Pinkert S, Heim A, Zeichhardt H, Grunert H-P, Poller W, et al. Combination of soluble coxsackievirus-adenovirus receptor and anti-coxsackievirus siRNAs exerts synergistic antiviral activity against coxsackievirus B3. Antiviral Res. (2009) 83:298–306. doi: 10.1016/j.antiviral.2009.07.002
12. Su Z, Yin J, Wang T, Sun Y, Ni P, Ma R, et al. Up-regulated HMGB1 in EAM directly led to collagen deposition by a PKCβ/Erk1/2-dependent pathway: cardiac fibroblast/myofibroblast might be another source of HMGB1. J Cell Mol Med. (2014) 18:1740–51. doi: 10.1111/jcmm.12324
13. Barin JG, Baldeviano GC, Talor MV, Wu L, Ong S, Fairweather D, et al. Fatal eosinophilic myocarditis develops in the absence of IFN-γ and IL-17A. J Immunol. (2013) 191:4038–47. doi: 10.4049/jimmunol.1301282
14. Hirasawa M, Ito Y, Shibata M-A, Otsuki Y. Mechanism of inflammation in murine eosinophilic myocarditis produced by adoptive transfer with ovalbumin challenge. Int Arch Allergy Immunol. (2007) 142:28–39. doi: 10.1159/000095996
15. Diny NL, Hou X, Barin JG, Chen G, Talor MV, Schaub J, et al. Macrophages and cardiac fibroblasts are the main producers of eotaxins and regulate eosinophil trafficking to the heart. Eur J Immunol. (2016) 46:2749–60. doi: 10.1002/eji.201646557
16. Sonderegger I, Röhn TA, Kurrer MO, Iezzi G, Zou Y, Kastelein RA, et al. Neutralization of IL-17 by active vaccination inhibits IL-23-dependent autoimmune myocarditis. Eur J Immunol. (2006) 36:2849–56. doi: 10.1002/eji.200636484
17. Yu M, Hu J, Zhu M-X, Zhao T, Liang W, Wen S, et al. Cardiac fibroblasts recruit Th17 cells infiltration into myocardium by secreting CCL20 in CVB3-induced acute viral myocarditis. Cell Physiol Biochem. (2013) 32:1437–50. doi: 10.1159/000356581
18. Hou X, Chen G, Bracamonte-Baran W, Choi HS, Diny NL, Sung J, et al. The cardiac microenvironment instructs divergent monocyte fates and functions in myocarditis. Cell Rep. (2019) 28:172–189.e7. doi: 10.1016/j.celrep.2019.06.007
19. Shioi T, Matsumori A, Sasayama S. Persistent expression of cytokine in the chronic stage of viral myocarditis in mice. Circulation. (1996) 94:2930–7. doi: 10.1161/01.CIR.94.11.2930 s
20. Boza P, Ayala P, Vivar R, Humeres C, Cáceres FT, Muñoz C, et al. Expression and function of toll-like receptor 4 and inflammasomes in cardiac fibroblasts and myofibroblasts: IL-1β synthesis, secretion, and degradation. Mol Immunol. (2016) 74:96–105. doi: 10.1016/j.molimm.2016.05.001
21. Valiente-Alandi I, Schafer AE, Blaxall BC. Extracellular matrix-mediated cellular communication in the heart. J Mol Cell Cardiol. (2016) 91:228–37. doi: 10.1016/j.yjmcc.2016.01.011
22. Kania G, Blyszczuk P, Eriksson U. Mechanisms of cardiac fibrosis in inflammatory heart disease. Trends Cardiovasc Med. (2009) 19:247–52. doi: 10.1016/j.tcm.2010.02.005
23. Siwik DA, Chang DL, Colucci WS. Interleukin-1beta and tumor necrosis factor-alpha decrease collagen synthesis and increase matrix metalloproteinase activity in cardiac fibroblasts in vitro. Circ Res. (2000) 86:1259–65. doi: 10.1161/01.RES.86.12.1259
24. Marino APMP, Silva AA, Pinho RT, Lannes-Vieira J. Trypanosoma cruzi infection: a continuous invader-host cell cross talk with participation of extracellular matrix and adhesion and chemoattractant molecules. Braz J Med Biol Res. (2003) 36:1121–33. doi: 10.1590/S0100-879X2003000800020
25. Westermann D, Savvatis K, Schultheiss H-P, Tschöpe C. Immunomodulation and matrix metalloproteinases in viral myocarditis. J Mol Cell Cardiol. (2010) 48:468–73. doi: 10.1016/j.yjmcc.2009.08.019
26. Hendry RG, Bilawchuk LM, Marchant DJ. Targeting matrix metalloproteinase activity and expression for the treatment of viral myocarditis. J Cardiovasc Transl Res. (2014) 7:212–25. doi: 10.1007/s12265-013-9528-2
27. Flammer AJ, Anderson T, Celermajer DS, Creager MA, Deanfield J, Ganz P, et al. The assessment of endothelial function: from research into clinical practice. Circulation. (2012) 126:753–67. doi: 10.1161/CIRCULATIONAHA.112.093245
28. Krüger-Genge A, Blocki A, Franke R-P, Jung F. Vascular endothelial cell biology: an update. Int J Mol Sci. (2019) 20:4411. doi: 10.3390/ijms20184411
29. Bonfanti R, Furie BC, Furie B, Wagner DD. PADGEM (GMP140) is a component of Weibel-Palade bodies of human endothelial cells. Blood. (1989) 73:1109–12. doi: 10.1182/blood.V73.5.1109.1109
30. De Caterina R, Libby P, Peng HB, Thannickal VJ, Rajavashisth TB, Gimbrone MA, et al. Nitric oxide decreases cytokine-induced endothelial activation. Nitric oxide selectively reduces endothelial expression of adhesion molecules and proinflammatory cytokines. J Clin Invest. (1995) 96:60–8. doi: 10.1172/JCI118074
31. Vallbracht KB, Schwimmbeck PL, Seeberg B, Kühl U, Schultheiss H-P. Endothelial dysfunction of peripheral arteries in patients with immunohistologically confirmed myocardial inflammation correlates with endothelial expression of human leukocyte antigens and adhesion molecules in myocardial biopsies. J Am Coll Cardiol. (2002) 40:515–20. doi: 10.1016/S0735-1097(02)01990-3
32. Vallbracht KB, Schwimmbeck PL, Kühl U, Rauch U, Seeberg B, Schultheiss H-P. Differential aspects of endothelial function of the coronary microcirculation considering myocardial virus persistence, endothelial activation, and myocardial leukocyte infiltrates. Circulation. (2005) 111:1784–91. doi: 10.1161/01.CIR.0000160863.30496.9B
33. Kühl U, Noutsias M, Seeberg B, Schultheiss HP. Immunohistological evidence for a chronic intramyocardial inflammatory process in dilated cardiomyopathy. Heart. (1996) 75:295–300. doi: 10.1136/hrt.75.3.295
34. Sturtzel C. Endothelial cells. Adv Exp Med Biol. (2017) 1003:71–91. doi: 10.1007/978-3-319-57613-8_4
35. Woudstra L, Juffermans LJM, van Rossum AC, Niessen HWM, Krijnen PAJ. Infectious myocarditis: the role of the cardiac vasculature. Heart Fail Rev. (2018) 23:583–95. doi: 10.1007/s10741-018-9688-x
36. Schmidt-Lucke C, Spillmann F, Bock T, Kühl U, Van Linthout S, Schultheiss H-P, et al. Interferon beta modulates endothelial damage in patients with cardiac persistence of human parvovirus b19 infection. J Infect Dis. (2010) 201:936–45. doi: 10.1086/650700
37. Verdonschot J, Hazebroek M, Merken J, Debing Y, Dennert R, Brunner-La Rocca H-P, et al. Relevance of cardiac parvovirus B19 in myocarditis and dilated cardiomyopathy: review of the literature. Eur J Heart Fail. (2016) 18:1430–41. doi: 10.1002/ejhf.665
38. Bültmann BD, Klingel K, Sotlar K, Bock CT, Baba HA, Sauter M, et al. Fatal parvovirus B19-associated myocarditis clinically mimicking ischemic heart disease: an endothelial cell-mediated disease. Hum Pathol. (2003) 34:92–5. doi: 10.1053/hupa.2003.48
39. Bachelier K, Biehl S, Schwarz V, Kindermann I, Kandolf R, Sauter M, et al. Parvovirus B19-induced vascular damage in the heart is associated with elevated circulating endothelial microparticles. PLoS ONE. (2017) 12:e0176311. doi: 10.1371/journal.pone.0176311
40. Devaux B, Scholz D, Hirche A, Klövekorn WP, Schaper J. Upregulation of cell adhesion molecules and the presence of low grade inflammation in human chronic heart failure. Eur Heart J. (1997) 18:470–9. doi: 10.1093/oxfordjournals.eurheartj.a015268
41. Park J-H, Shin H-H, Rhyu H-S, Kim S-H, Jeon E-S, Lim B-K. Vascular endothelial integrity affects the severity of enterovirus-mediated cardiomyopathy. Int J Mol Sci. (2021) 22:3053. doi: 10.3390/ijms22063053
42. Elangbam CS, Qualls CW Jr., Dahlgren RR. Cell adhesion molecules–update. Vet Pathol. (1997) 34:61–73. doi: 10.1177/030098589703400113
43. Springer TA. Traffic signals on endothelium for lymphocyte recirculation and leukocyte emigration. Annu Rev Physiol. (1995) 57:827–72. doi: 10.1146/annurev.ph.57.030195.004143
44. Mantovani A, Garlanda C, Introna M, Vecchi A. Regulation of endothelial cell function by pro- and anti-inflammatory cytokines. Transplant Proc. (1998) 30:4239–43. doi: 10.1016/S0041-1345(98)01402-X
45. Prochorec-Sobieszek M, Bilińska ZT, Grzybowski J, Mazurkiewicz L, Skwarek M, Walczak E, et al. Assessment of the inflammatory process by endomyocardial biopsy in patients with dilated cardiomyopathy based on pathological and immunohistochemical methods. Kardiol Pol. (2006) 64:479–87.
46. McDouall RM, Farrar MW, Khan S, Yacoub MH, Allen SP. Unique sensitivities to cytokine regulated expression of adhesion molecules in human heart-derived endothelial cells. Endothelium. (2001) 8:25–40. doi: 10.3109/10623320109063155
47. Kohl P, Camelliti P, Burton FL, Smith GL. Electrical coupling of fibroblasts and myocytes: relevance for cardiac propagation. J Electrocardiol. (2005) 38(4 Suppl.):45–50. doi: 10.1016/j.jelectrocard.2005.06.096
48. Bowers SLK, Borg TK, Baudino TA. The dynamics of fibroblast-myocyte-capillary interactions in the heart. Ann N Y Acad Sci. (2010) 1188:143–52. doi: 10.1111/j.1749-6632.2009.05094.x
49. Mayourian J, Ceholski DK, Gonzalez DM, Cashman TJ, Sahoo S, Hajjar RJ, et al. Physiologic, pathologic, and therapeutic paracrine modulation of cardiac excitation-contraction coupling. Circ Res. (2018) 122:167–83. doi: 10.1161/CIRCRESAHA.117.311589
50. Ribeiro da Silva A, Neri EA, Turaça LT, Dariolli R, Fonseca-Alaniz MH, Santos-Miranda A, et al. NOTCH1 is critical for fibroblast-mediated induction of cardiomyocyte specialization into ventricular conduction system-like cells in vitro. Sci Rep. (2020) 10:16163. doi: 10.1038/s41598-020-73159-0
51. Wang Y, Yao F, Wang L, Li Z, Ren Z, Li D, et al. Single-cell analysis of murine fibroblasts identifies neonatal to adult switching that regulates cardiomyocyte maturation. Nat Commun. (2020) 11:2585. doi: 10.1038/s41467-020-16204-w
52. Kumar S, Wang G, Zheng N, Cheng W, Ouyang K, Lin H, et al. HIMF (Hypoxia-induced mitogenic factor)-IL (interleukin)-6 signaling mediates cardiomyocyte-fibroblast crosstalk to promote cardiac hypertrophy and fibrosis. Hypertension. (2019) 73:1058–70. doi: 10.1161/HYPERTENSIONAHA.118.12267
53. Yuan J, Liu H, Gao W, Zhang L, Ye Y, Yuan L, et al. MicroRNA-378 suppresses myocardial fibrosis through a paracrine mechanism at the early stage of cardiac hypertrophy following mechanical stress. Theranostics. (2018) 8:2565–82. doi: 10.7150/thno.22878
54. Aránguiz P, Romero P, Vásquez F, Flores-Vergara R, Aravena D, Sánchez G, et al. Polycystin-1 mitigates damage and regulates CTGF expression through AKT activation during cardiac ischemia/reperfusion. Biochim Biophys Acta Mol Basis Dis. (2021) 1867:165986. doi: 10.1016/j.bbadis.2020.165986
55. Wang X, Morelli MB, Matarese A, Sardu C, Santulli G. Cardiomyocyte-derived exosomal microRNA-92a mediates post-ischemic myofibroblast activation both in vitro and ex vivo. ESC Heart Fail. (2020) 7:284–8. doi: 10.1002/ehf2.12584
56. Morelli MB, Shu J, Sardu C, Matarese A, Santulli G. Cardiosomal microRNAs are essential in post-infarction myofibroblast phenoconversion. Int J Mol Sci. (2019) 21:201. doi: 10.3390/ijms21010201
57. Cartledge JE, Kane C, Dias P, Tesfom M, Clarke L, McKee B, et al. Functional crosstalk between cardiac fibroblasts and adult cardiomyocytes by soluble mediators. Cardiovasc Res. (2015) 105:260–70. doi: 10.1093/cvr/cvu264
58. Koleini N, Santiago J-J, Nickel BE, Sequiera GL, Wang J, Fandrich RR, et al. Elimination or neutralization of endogenous high-molecular-weight FGF2 mitigates doxorubicin-induced cardiotoxicity. Am J Physiol Heart Circ Physiol. (2019) 316:H279–88. doi: 10.1152/ajpheart.00587.2018
59. Shi H, Zhang X, He Z, Wu Z, Rao L, Li Y. Metabolites of hypoxic cardiomyocytes induce the migration of cardiac fibroblasts. Cell Physiol Biochem. (2017) 41:413–21. doi: 10.1159/000456531
60. Bang C, Batkai S, Dangwal S, Gupta SK, Foinquinos A, Holzmann A, et al. Cardiac fibroblast-derived microRNA passenger strand-enriched exosomes mediate cardiomyocyte hypertrophy. J Clin Invest. (2014) 124:2136–46. doi: 10.1172/JCI70577
61. Abrial M, Da Silva CC, Pillot B, Augeul L, Ivanes F, Teixeira G, et al. Cardiac fibroblasts protect cardiomyocytes against lethal ischemia-reperfusion injury. J Mol Cell Cardiol. (2014) 68:56–65. doi: 10.1016/j.yjmcc.2014.01.005
62. Cosme J, Guo H, Hadipour-Lakmehsari S, Emili A, Gramolini AO. Hypoxia-induced changes in the fibroblast secretome, exosome, and whole-cell proteome using cultured, cardiac-derived cells isolated from neonatal mice. J Proteome Res. (2017) 16:2836–47. doi: 10.1021/acs.jproteome.7b00144
63. Luo H, Li X, Li T, Zhao L, He J, Zha L, et al. microRNA-423-3p exosomes derived from cardiac fibroblasts mediates the cardioprotective effects of ischaemic post-conditioning. Cardiovasc Res. (2019) 115:1189–204. doi: 10.1093/cvr/cvy231
64. Morikawa M, Derynck R, Miyazono K. TGF-β and the TGF-β family: context-dependent roles in cell and tissue physiology. Cold Spring Harb Perspect Biol. (2016) 8:a021873. doi: 10.1101/cshperspect.a021873
65. Goumans M-J, Ten Dijke P. TGF-β signaling in control of cardiovascular function. Cold Spring Harb Perspect Biol. (2018) 10:a022210. doi: 10.1101/cshperspect.a022210
66. Batlle E, Massagué J. Transforming growth factor-β signaling in immunity and cancer. Immunity. (2019) 50:924–40. doi: 10.1016/j.immuni.2019.03.024
67. Ruwhof C, van Wamel AE, Egas JM, van der Laarse A. Cyclic stretch induces the release of growth promoting factors from cultured neonatal cardiomyocytes and cardiac fibroblasts. Mol Cell Biochem. (2000) 208:89–98. doi: 10.1023/A:1007046105745
68. Fujiu K, Nagai R. Fibroblast-mediated pathways in cardiac hypertrophy. J Mol Cell Cardiol. (2014) 70:64–73. doi: 10.1016/j.yjmcc.2014.01.013
69. Fredj S, Bescond J, Louault C, Potreau D. Interactions between cardiac cells enhance cardiomyocyte hypertrophy and increase fibroblast proliferation. J Cell Physiol. (2005) 202:891–9. doi: 10.1002/jcp.20197
70. Hsieh PCH, Davis ME, Lisowski LK, Lee RT. Endothelial-cardiomyocyte interactions in cardiac development and repair. Annu Rev Physiol. (2006) 68:51–66. doi: 10.1146/annurev.physiol.68.040104.124629
71. Jiang F, Mohr F, Ullrich ND, Hecker M, Wagner AH. Endothelial cell modulation of cardiomyocyte gene expression. Exp Cell Res. (2019) 383:111565. doi: 10.1016/j.yexcr.2019.111565
72. Sniegon I, Prieß M, Heger J, Schulz R, Euler G. Endothelial mesenchymal transition in hypoxic microvascular endothelial cells and paracrine induction of cardiomyocyte apoptosis are mediated via TGFβ1/SMAD signaling. Int J Mol Sci. (2017) 18:2290. doi: 10.3390/ijms18112290
73. Kongpol K, Nernpermpisooth N, Prompunt E, Kumphune S. Endothelial-cell-derived human secretory leukocyte protease inhibitor (SLPI) protects cardiomyocytes against ischemia/reperfusion injury. Biomolecules. (2019) 9:678. doi: 10.3390/biom9110678
74. Zhang Q, Shang M, Zhang M, Wang Y, Chen Y, Wu Y, et al. Microvesicles derived from hypoxia/reoxygenation-treated human umbilical vein endothelial cells promote apoptosis and oxidative stress in H9c2 cardiomyocytes. BMC Cell Biol. (2016) 17:25. doi: 10.1186/s12860-016-0100-1
75. Chen C-H, Hsu S-Y, Chiu C-C, Leu S. MicroRNA-21 mediates the protective effect of cardiomyocyte-derived conditioned medium on ameliorating myocardial infarction in rats. Cells. (2019) 8:935. doi: 10.3390/cells8080935
76. Davidson SM, Riquelme JA, Zheng Y, Vicencio JM, Lavandero S, Yellon DM. Endothelial cells release cardioprotective exosomes that may contribute to ischaemic preconditioning. Sci Rep. (2018) 8:15885. doi: 10.1038/s41598-018-34357-z
77. Dougherty JA, Kumar N, Noor M, Angelos MG, Khan M, Chen C-A, et al. Extracellular vesicles released by human induced-pluripotent stem cell-derived cardiomyocytes promote angiogenesis. Front Physiol. (2018) 9:1794. doi: 10.3389/fphys.2018.01794
78. Cao Y, Wang Y, Xiao L, Xu J-Y, Liu Y, Jiang R, et al. Endothelial-derived exosomes induced by lipopolysaccharide alleviate rat cardiomyocytes injury and apoptosis. Am J Transl Res. (2021) 13:1432–44.
79. McCormick ME, Collins C, Makarewich CA, Chen Z, Rojas M, Willis MS, et al. Platelet endothelial cell adhesion molecule-1 mediates endothelial-cardiomyocyte communication and regulates cardiac function. J Am Heart Assoc. (2015) 4:e001210. doi: 10.1161/JAHA.114.001210
80. Lee A-S, Wang G-J, Chan H-C, Chen F-Y, Chang C-M, Yang C-Y, et al. Electronegative low-density lipoprotein induces cardiomyocyte apoptosis indirectly through endothelial cell-released chemokines. Apoptosis. (2012) 17:1009–18. doi: 10.1007/s10495-012-0726-1
81. Vignon-Zellweger N, Heiden S, Miyauchi T, Emoto N. Endothelin and endothelin receptors in the renal and cardiovascular systems. Life Sci. (2012) 91:490–500. doi: 10.1016/j.lfs.2012.03.026
82. Pummerer CL, Grässl G, Sailer M, Bachmaier KW, Penninger JM, Neu N. Cardiac myosin-induced myocarditis: target recognition by autoreactive T cells requires prior activation of cardiac interstitial cells. Lab Invest. (1996) 74:845–52.
83. van Niel G, D'Angelo G, Raposo G. Shedding light on the cell biology of extracellular vesicles. Nat Rev Mol Cell Biol. (2018) 19:213–28. doi: 10.1038/nrm.2017.125
84. Ratajczak J, Wysoczynski M, Hayek F, Janowska-Wieczorek A, Ratajczak MZ. Membrane-derived microvesicles: important and underappreciated mediators of cell-to-cell communication. Leukemia. (2006) 20:1487–95. doi: 10.1038/sj.leu.2404296
85. Colombo M, Raposo G, Théry C. Biogenesis, secretion, and intercellular interactions of exosomes and other extracellular vesicles. Annu Rev Cell Dev Biol. (2014) 30:255–89. doi: 10.1146/annurev-cellbio-101512-122326
86. Malik ZA, Kott KS, Poe AJ, Kuo T, Chen L, Ferrara KW, et al. Cardiac myocyte exosomes: stability, HSP60, and proteomics. Am J Physiol Heart Circ Physiol. (2013) 304:H954–65. doi: 10.1152/ajpheart.00835.2012
87. Wang L, Zhang J. Exosomal lncRNA AK139128 derived from hypoxic cardiomyocytes promotes apoptosis and inhibits cell proliferation in cardiac fibroblasts. Int J Nanomed. (2020) 15:3363–76. doi: 10.2147/IJN.S240660
88. Seong IW, Choe SC, Jeon ES. Fulminant coxsackieviral myocarditis. N Engl J Med. (2001) 345:379. doi: 10.1056/NEJM200108023450518
89. Sin J, McIntyre L, Stotland A, Feuer R, Gottlieb RA. Coxsackievirus B escapes the infected cell in ejected mitophagosomes. J Virol. (2017) 91:e01347–17. doi: 10.1128/JVI.01347-17
90. Feng Z, Hensley L, McKnight KL, Hu F, Madden V, Ping L, et al. A pathogenic picornavirus acquires an envelope by hijacking cellular membranes. Nature. (2013) 496:367–71. doi: 10.1038/nature12029
91. Bello-Morales R, López-Guerrero JA. Extracellular vesicles in herpes viral spread and immune evasion. Front Microbiol. (2018) 9:2572. doi: 10.3389/fmicb.2018.02572
92. Heim A, Grumbach I, Pring-Akerblom P, Stille-Siegener M, Müller G, Kandolf R, et al. Inhibition of coxsackievirus B3 carrier state infection of cultured human myocardial fibroblasts by ribavirin and human natural interferon-alpha. Antiviral Res. (1997) 34:101–11. doi: 10.1016/S0166-3542(97)01028-0
93. Kania G, Blyszczuk P, Stein S, Valaperti A, Germano D, Dirnhofer S, et al. Heart-infiltrating prominin-1+/CD133+ progenitor cells represent the cellular source of transforming growth factor beta-mediated cardiac fibrosis in experimental autoimmune myocarditis. Circ Res. (2009) 105:462–70. doi: 10.1161/CIRCRESAHA.109.196287
94. Rehren F, Ritter B, Dittrich-Breiholz O, Henke A, Lam E, Kati S, et al. Induction of a broad spectrum of inflammation-related genes by Coxsackievirus B3 requires interleukin-1 signaling. Med Microbiol Immunol. (2013) 202:11–23. doi: 10.1007/s00430-012-0245-2
95. Chen G, Bracamonte-Baran W, Diny NL, Hou X, Talor MV, Fu K, et al. Sca-1 cardiac fibroblasts promote development of heart failure. Eur J Immunol. (2018) 48:1522–38. doi: 10.1002/eji.201847583
96. Magadum A, Kishore R. Cardiovascular manifestations of COVID-19 infection. Cells. (2020) 9:2508. doi: 10.3390/cells9112508
97. Van Linthout S, Elsanhoury A, Klein O, Sosnowski M, Miteva K, Lassner D, et al. Telbivudine in chronic lymphocytic myocarditis and human parvovirus B19 transcriptional activity. ESC Heart Fail. (2018) 5:818–29. doi: 10.1002/ehf2.12341
98. Funke C, Farr M, Werner B, Dittmann S, Überla K, Piper C, et al. Antiviral effect of Bosentan and Valsartan during coxsackievirus B3 infection of human endothelial cells. J Gen Virol. (2010) 91(Pt 8):1959–70. doi: 10.1099/vir.0.020065-0
99. Werner B, Dittmann S, Funke C, Überla K, Piper C, Niehaus K, et al. Effect of lovastatin on coxsackievirus B3 infection in human endothelial cells. Inflamm Res. (2014) 63:267–76. doi: 10.1007/s00011-013-0695-z
100. Chen X, Zhang Y-Y, Ye D, Yang G, Song Y-N, Mo L-H, et al. House dust mite specific antibodies induce neutrophilic inflammation in the heart. Theranostics. (2020) 10:8807–17. doi: 10.7150/thno.47134
101. Werner L, Deutsch V, Barshack I, Miller H, Keren G, George J. Transfer of endothelial progenitor cells improves myocardial performance in rats with dilated cardiomyopathy induced following experimental myocarditis. J Mol Cell Cardiol. (2005) 39:691–7. doi: 10.1016/j.yjmcc.2005.06.015
102. Turesson C. Endothelial expression of MHC class II molecules in autoimmune disease. Curr Pharm Des. (2004) 10:129–43. doi: 10.2174/1381612043453414
103. Thelemann C, Haller S, Blyszczuk P, Kania G, Rosa M, Eriksson U, et al. Absence of nonhematopoietic MHC class II expression protects mice from experimental autoimmune myocarditis. Eur J Immunol. (2016) 46:656–64. doi: 10.1002/eji.201545945
Keywords: myocarditis, cardiac fibroblasts, endothelial cells, cross-talk, extracellular vesicles
Citation: Xuan Y, Chen C, Wen Z and Wang DW (2022) The Roles of Cardiac Fibroblasts and Endothelial Cells in Myocarditis. Front. Cardiovasc. Med. 9:882027. doi: 10.3389/fcvm.2022.882027
Received: 24 February 2022; Accepted: 16 March 2022;
Published: 07 April 2022.
Edited by:
Zhanpeng Huang, The First Affiliated Hospital of Sun Yat-sen University, ChinaReviewed by:
Pingzhu Zhou, Boston Children's Hospital and Harvard Medical School, United StatesCopyright © 2022 Xuan, Chen, Wen and Wang. This is an open-access article distributed under the terms of the Creative Commons Attribution License (CC BY). The use, distribution or reproduction in other forums is permitted, provided the original author(s) and the copyright owner(s) are credited and that the original publication in this journal is cited, in accordance with accepted academic practice. No use, distribution or reproduction is permitted which does not comply with these terms.
*Correspondence: Zheng Wen, d2VuemhlbmdAdGpoLnRqbXUuZWR1LmNu;
Dao Wen Wang, ZHd3YW5nQHRqaC50am11LmVkdS5jbg==
Disclaimer: All claims expressed in this article are solely those of the authors and do not necessarily represent those of their affiliated organizations, or those of the publisher, the editors and the reviewers. Any product that may be evaluated in this article or claim that may be made by its manufacturer is not guaranteed or endorsed by the publisher.
Research integrity at Frontiers
Learn more about the work of our research integrity team to safeguard the quality of each article we publish.