- 1Division of Cardiology, Department of Internal Medicine, Chonnam National University Hospital, Chonnam National University Medical School, Gwangju, South Korea
- 2Division of Cardiology, Department of Internal Medicine, Heart Vascular Stroke Institute, Samsung Medical Center, Sungkyunkwan University School of Medicine, Seoul, South Korea
- 3Department of Cardiology, Shanghai Institute of Cardiovascular Diseases, Zhongshan Hospital, Fudan University, Shanghai, China
- 4Division of Cardiovascular Medicine, Department of Internal Medicine, University of Iowa Carver College of Medicine, Iowa City, IA, United States
- 5Department of Radiology, Cardiovascular Imaging Center, Heart Vascular Stroke Institute, Samsung Medical Center, Sungkyunkwan University School of Medicine, Seoul, South Korea
- 6Department of Internal Medicine and Cardiovascular Center, Chosun University Hospital, University of Chosun College of Medicine, Gwangju, South Korea
- 7Division of Cardiovascular Medicine, Department of Internal Medicine, Seoul National University Bundang Hospital, Seongnam, South Korea
- 8Division of Cardiology, Department of Internal Medicine, Gangneung Asan Hospital, University of Ulsan College of Medicine, Gangneung, South Korea
- 9Cardiovascular Center and Cardiology Division, Seoul St. Mary's Hospital, The Catholic University of Korea, Seoul, South Korea
Objectives: While coronary computed tomography angiography (CCTA) enables the evaluation of anatomic and hemodynamic plaque characteristics of coronary artery disease (CAD), the clinical roles of these characteristics are not clear. We sought to evaluate the prognostic implications of CCTA-derived anatomic and hemodynamic plaque characteristics in the prediction of subsequent coronary events.
Methods: The study cohort consisted of 158 patients who underwent CCTA with suspected CAD within 6–36 months before percutaneous coronary intervention (PCI) for acute myocardial infarction (MI) or unstable angina and age-/sex-matched 62 patients without PCI as the control group. Preexisting high-risk plaque characteristics (HRPCs: low attenuation plaque, positive remodeling, napkin-ring sign, spotty calcification, minimal luminal area <4 mm2, or plaque burden ≥70%) and hemodynamic parameters (per-vessel fractional flow reserve [FFRCT], per-lesion ΔFFRCT, and percent ischemic myocardial mass) were analyzed from prior CCTA. The primary outcome was a subsequent coronary event, which was defined as a composite of vessel-specific MI or revascularization for unstable angina. The prognostic impact of clinical risk factors, HRPCs, and hemodynamic parameters were compared between vessels with (160 vessels) and without subsequent coronary events (329 vessels).
Results: Vessels with a subsequent coronary event had higher number of HRPCs (2.6 ± 1.4 vs. 2.3 ± 1.4, P = 0.012), lower FFRCT (0.76 ± 0.13 vs. 0.82 ± 0.11, P < 0.001), higher ΔFFRCT (0.14 ± 0.12 vs. 0.09 ± 0.08, P < 0.001), and higher percent ischemic myocardial mass (29.0 ± 18.5 vs. 26.0 ± 18.4, P = 0.022) than those without a subsequent coronary event. Compared with clinical risk factors, HRPCs and hemodynamic parameters showed higher discriminant abilities for subsequent coronary events with ΔFFRCT being the most powerful predictor. HRPCs showed additive discriminant ability to clinical risk factors (c-index 0.620 vs. 0.558, P = 0.027), and hemodynamic parameters further increased discriminant ability (c-index 0.698 vs. 0.620, P = 0.001) and reclassification abilities (NRI 0.460, IDI 0.061, P < 0.001 for all) for subsequent coronary events. Among vessels with negative FFRCT (>0.80), adding HRPCs into clinical risk factors significantly increased discriminant and reclassification abilities for subsequent coronary events (c-index 0.687 vs. 0.576, P = 0.005; NRI 0.412, P = 0.002; IDI 0.064, P = 0.001) but not for vessels with positive FFRCT (≤0.80).
Conclusion: In predicting subsequent coronary events, both HRPCs and hemodynamic parameters by CCTA allow better prediction of subsequent coronary events than clinical risk factors. HRPCs provide more incremental predictability than clinical risk factors alone among vessels with negative FFRCT but not among vessels with positive FFRCT.
Clinical Trial Registration: PreDiction and Validation of Clinical CoursE of Coronary Artery DiSease With CT-Derived Non-INvasive HemodYnamic Phenotyping and Plaque Characterization (DESTINY Study), NCT04794868.
Introduction
Identification of patients with coronary atherosclerotic disease (CAD) at high risk of acute coronary syndrome (ACS) and who may benefit from intensified preventive measures has been of major interest (1). Since postmortem studies provided insights into plaque vulnerability and rupture as the major causes of ACS and sudden cardiac death, various imaging modalities have been used to identify characteristics of vulnerable plaques, which are prone to rupture (high-risk plaque characteristics [HRPCs]) (2–6).
Nevertheless, given the limited predictive value of HRPCs alone in the prediction of subsequent coronary events, (7) contemporary practice has been guided by the hemodynamic significance of CAD determined by invasive physiologic indexes, such as fractional flow reserve (FFR) (8), but not by HRPCs. Recent studies have suggested the importance of both hemodynamic significance and plaque vulnerability in CAD and their complementary roles in the progression of the disease and the development of ACS (9). Comprehensive assessment of CAD has become possible in clinical practice with recent advances in coronary computed tomography angiography (CCTA) and computational fluid dynamics (CFD), which allow simultaneous noninvasive assessment of anatomic plaque characteristics (4–6) and hemodynamic significance of CAD (10).
However, studies on the clinical role of the comprehensive evaluation of these features are limited, and it is still unclear whether integrating various aspects of the pathophysiology of CAD would increase the predictability of subsequent coronary events (9). Furthermore, it would be important to better understand the prognostic implications and the potential role of utilizing HRPCs in contemporary CAD management. In this regard, this study sought to evaluate (1) prognostic implications of combined analysis of CCTA-derived HRPCs and hemodynamic parameters in the prediction of subsequent coronary events and (2) differential prognostic implications of CCTA-derived HRPCs according to the hemodynamic significance of CAD.
Materials and Methods
Study Design and Population
To evaluate the prognostic impact of anatomic and hemodynamic plaque characteristics on subsequent coronary events, this study enrolled two separate patient populations (the ACS cohort and the negative control cohort) (Figure 1). The ACS cohort included consecutive patients who underwent CCTA within 6–36 months before percutaneous coronary intervention (PCI) for acute myocardial infarction (MI) or unstable angina admitted to Samsung Medical Center between 2003 and 2019. The negative control cohort included age-/sex-matched patients who underwent CCTA within 6–36 months before invasive coronary angiography for suspected stable angina but did not undergo PCI because there was no significant lesion at the time of angiography. In both cohorts, CCTA was performed under the judgment of the respective physicians as a routine clinical evaluation for suspected CAD. In addition, the operators were blinded to the detailed core laboratory analyses of anatomic and hemodynamic plaque characteristics at the time of invasive angiography.
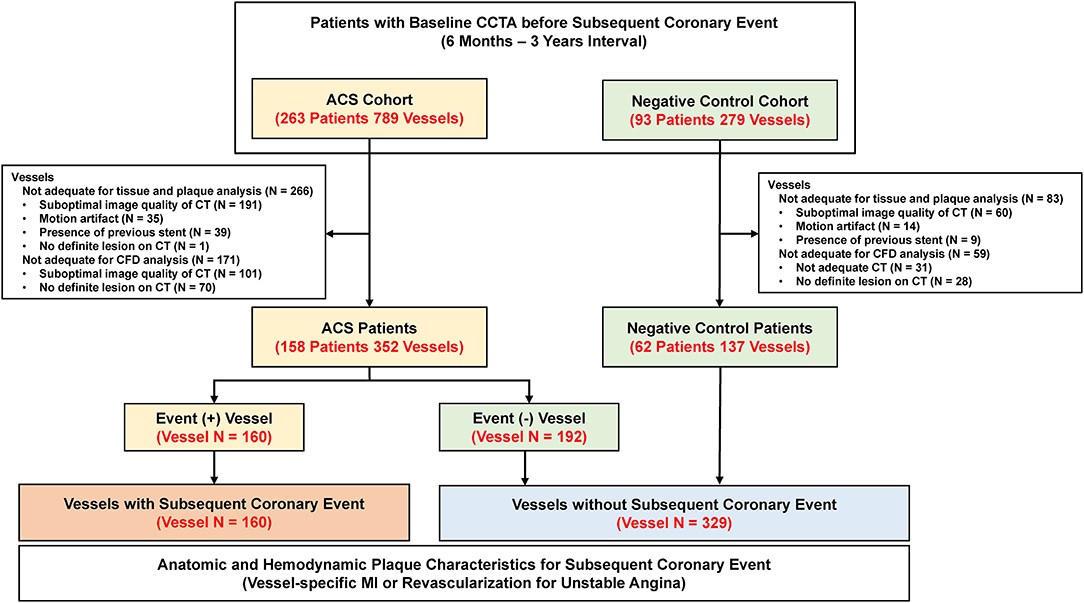
Figure 1. Study flow. This study enrolled two separate patient populations (ACS and negative control cohort). The ACS cohort included consecutive patients who underwent CCTA within 6–36 months before PCI for acute myocardial infarction or unstable angina. The negative control cohort included age-/sex-matched patients who underwent CCTA within 6–36 months before invasive coronary angiography for suspected stable angina but did not undergo PCI because there was no significant lesion at the time of angiography. A total of 489 vessels (160 vessels with subsequent coronary event and 329 vessels without subsequent coronary event) were finally included in the study. ACS, acute coronary syndrome; CCTA, coronary computed tomography angiography; CFD, computational fluid dynamics; CT, computed tomography; PCI, percutaneous coronary intervention.
In the ACS cohort, patients without clear culprit lesions in invasive angiography, intravascular ultrasound, or optical coherence tomography were excluded. Additional exclusion criteria were patients with ACS caused by in-stent restenosis, vessels with stents before CCTA, previous history of coronary artery bypass grafting, and type 2 myocardial infarction due to other general medical conditions. In both ACS and negative control cohorts, patients with unavailable CCTA images or suboptimal image quality for the analysis of plaque characteristics or CFD were excluded by the CCTA core laboratory (Elucid Bioimaging, Inc., Boston, MA, USA) or the CFD core laboratory (Shanghai Institute of Cardiovascular Diseases, Shanghai, China), respectively. The study protocol was approved by the institutional review board of Samsung Medical Center. This study was conducted in accordance with the Declaration of Helsinki and registered on clinicaltrials.gov (PreDiction and Validation of Clinical CoursE of Coronary Artery DiSease With CT-Derived Non-INvasive HemodYnamic Phenotyping and Plaque Characterization [DESTINY Study], NCT02374775).
Analysis of Anatomic Plaque Characteristics in CCTA
Coronary computed tomography angiography images were obtained in accordance with the Society of Cardiovascular Computed Tomography Guidelines on Performance of CCTA, with 64-channel scanner (GE Healthcare, Milwaukee, WI, USA) or 128-channel dual-source scanner platforms (Siemens Medical System, Forchheim, Germany) with electrocardiographic gating (11). A standardized protocol for heart rate control with beta-blockers and sublingual nitroglycerin was administered. All CCTA images were analyzed regarding anatomic plaque characteristics in a blinded fashion using histologically validated plaque quantification software (vascuCAP, clinical edition) at a core laboratory (Elucid Bioimaging, Inc., Boston, MA, USA) (12).
For anatomic severity, diameter stenosis, area stenosis, minimum lumen area (MLA), and lesion length were measured. Whole vessel and plaque tissue characterization were performed by defining the vessel wall into different components: calcified tissue, intra-plaque hemorrhage, lipid-rich necrotic core, matrix, or perivascular adipose tissue (13). Plaque burden was defined as the ratio of wall area divided by the overall vessel area (12, 13). Any lesions with diameter stenosis >30% were selected for plaque tissue characterization. In cases of multiple lesions in the same target vessel, the lesion with the greatest diameter stenosis was selected as the representative lesion. The presence of the following HRPCs was analyzed according to the definitions from previous studies: (1) low attenuation plaque (average density ≤ 30 Hounsfield units [HU].); (2) positive remodeling (lesion diameter/reference diameter ≥1.1); (3) napkin-ring sign (ring-like attenuation pattern with peripheral high attenuation tissue surrounding a central lower attenuation portion); and (4) spotty calcification (average density >130 HU and diameter <3 mm) (4, 5). In this analysis, HRPCs were defined by combining both qualitative and quantitative parameters based on previous studies with the presence of any of the following features: low attenuation plaque, positive remodeling, napkin-ring sign, spotty calcification, MLA <4 mm2, or plaque burden ≥70% (2–5).
Analysis of Hemodynamic Plaque Characteristics in CCTA
Hemodynamic parameters derived from CCTA were analyzed in a blinded fashion at a core laboratory (Zhongshan Hospital, Shanghai, China) using a commercialized offline software system (RuiXin-FFR, version 1.0, Raysight Medical, Shenzhen, China). First, three-dimensional anatomical computational models of the coronary tree were reconstructed from CCTA images. Second, patient-specific boundary conditions were obtained from the CCTA images. Third, hemodynamics parameters were solved by CFD-based FFRCT calculation. Detailed methods of three-dimensional model reconstruction and CFD-based FFRCT calculation are described in the Supplementary Appendix. Briefly, coronary models were constructed using segmentation algorithms that extracted the luminal surface of the epicardial coronary arteries and branches. Coronary flow and pressure were computed by solving the Navier–Stokes equations, assuming that blood is approximated as a Newtonian fluid. Boundary conditions for hyperemia were derived from myocardial mass, vessel sizes at each outlet, and the response of the microcirculation to adenosine. As with plaque tissue characterization, only lesions with a diameter stenosis of >30% were selected for the computation of hemodynamic parameters. For this study, three hemodynamic parameters were used, namely, per-vessel FFRCT, per-lesion delta FFRCT (ΔFFRCT), and per-vessel percent ischemic myocardial mass. First, FFRCT was defined as the ratio of mean downstream coronary pressure (Pd) and the aortic pressure (Pa) derived from the CFD analysis under a simulated hyperemic condition. Second, ΔFFRCT was defined by computing the difference in FFRCT values at the proximal and distal sites of each lesion. Third, the percent ischemic myocardial mass of each vessel segment was defined as the ratio between the myocardial mass subtended beyond the point at which the vessel's FFRCT is ≤0.80 and the entire vessel segment (14). Myocardial mass was computed using a stem-and-crown model (15, 16), which is based on allometric scaling between the length of the coronary arterial tree and myocardial mass (15, 16).
Data Collection and Clinical Outcomes
Clinical data were collected by reviewing electronic medical records. All angiograms were analyzed, and culprit lesions were determined in a blinded fashion at core laboratories (Samsung Medical Center, Seoul, Korea). The primary outcome was a subsequent coronary event, which was defined as a composite of vessel-specific MI or revascularization for unstable angina. The definition of clinical outcomes was in accordance with the Academic Research Consortium. Acute MI was defined according to the universal definition of MI (17).
Statistical Analysis
Data were analyzed on a per-patient basis for clinical characteristics and on a per-vessel basis for anatomic and hemodynamic plaque characteristics and vessel-specific clinical outcomes. For per-patient analyses, the Student's t-test and the chi-square test were used to compare continuous and categorical variables, respectively. For per-vessel analyses, a generalized estimating equation was used to adjust for intra-subject variability among vessels from the same patient. An analysis of variance test was used to compare differences in the number of clinical risk factors and hemodynamic parameters according to the classification by the number of HRPCs.
The discriminant function of clinical characteristics and anatomic and hemodynamic plaque characteristics for the primary outcome were evaluated using the c-index and 95% confidence interval (CI) in receiver operating curve analysis. Optimal cutoff values for HRPCs and hemodynamic parameters were determined based on receiver operating curve analysis and results of previous studies (5, 8, 9). Diagnostic performance was presented as sensitivity, specificity, positive predictive value, negative predictive value, and diagnostic accuracy. Incremental predictability of HRPCs and hemodynamic parameters for the primary outcome was compared using a global chi-square estimated by the likelihood ratio test. The cumulative incidence of the primary outcome was presented as Kaplan–Meier estimates and compared using a log-rank test. To adjust for the interrogated vessels within the same patient, multivariable marginal Cox proportional hazards regression was used to calculate the adjusted hazard ratio (HR) and 95% CI. Adjusted covariables were age, sex, hypertension, diabetes mellitus, dyslipidemia, chronic kidney disease, and current smoker. The assumption of proportionality was assessed graphically using a log minus log plot, and all Cox proportional hazard models satisfied the proportional hazards assumption.
Three prediction models were constructed to assess the incremental prognostic value of HRPCs and hemodynamic parameters: (1) model 1: clinical risk factors; (2) model 2: model 1 + individual components of HRPCs; and (3) model 3: model 2 + hemodynamic parameters. Clinical risk factors included age, sex, hypertension, diabetes mellitus, dyslipidemia, chronic kidney disease, and current smoker. Hemodynamic parameters included FFRCT, ΔFFRCT, and percent ischemic myocardial mass. Discriminant ability was compared using the c-index, and reclassification performance was compared using the relative integrated discrimination improvement (IDI) and category-free net reclassification index (NRI). Subgroup analysis was performed to assess the differential prognostic implications of HRPCs according to hemodynamic significance. Vessels were divided into subgroups according to optimal cutoff values of FFRCT and ΔFFRCT, and the incremental prognostic value for the primary outcome of HRPCs was evaluated in each subgroup.
All analyses were two-sided, and P-values <0.05 were considered statistically significant. Statistical analyses were performed using R version 4.0.3 (R Foundation for Statistical Computing, Vienna, Austria).
Results
Characteristics of Patients
A total of 220 patients with 489 vessels were selected for the current analyses (Figure 1). Among them, 158 patients (71.8%) were from the ACS cohort and 62 (28.2%) were from the negative control cohort. In the ACS cohort, 17.1 and 82.9% of patients presented with acute MI and unstable angina, respectively. Among the ACS cohort, 160 vessels had subsequent coronary events and 192 vessels were non-culprit vessels. With 137 vessels from the negative control cohort, a total of 329 vessels were not related to subsequent coronary events. The mean interval between CCTA and invasive coronary angiography was 554.3 ± 268.2 days. In the comparison of the clinical characteristics of patients, there was no significant difference in demographics, cardiovascular comorbidities, or profiles of medical treatment after CCTA (Table 1).
Anatomic and Hemodynamic Plaque Characteristics
Table 2 shows the comparison of anatomic and hemodynamic plaque characteristics between 160 vessels with subsequent coronary events and 329 vessels without subsequent coronary events. In addition, Supplementary Table 1 presents the anatomical and hemodynamic plaque characteristics of 137 vessels from the negative control cohort. Vessels with subsequent coronary events showed significantly lower MLA and higher plaque burden than vessels without events. Regarding anatomic plaque characteristics, vessels with subsequent coronary events showed a significantly higher proportion of low attenuation plaque, MLA <4 mm2, and plaque burden at lumen area ≥70% than vessels without events. As a result, vessels with subsequent coronary events had a higher number of HRPCs (2.6 ± 1.4 vs. 2.3 ± 1.4, P = 0.009) than vessels without events. There was no significant difference in other individual components of the whole vessel and plaque tissue characterization between the two groups.
In terms of hemodynamic plaque characteristics, vessels with subsequent coronary events had lower FFRCT (0.76 ± 0.13 vs. 0.82 ± 0.11, P < 0.001), higher ΔFFRCT (0.14 ± 0.12 vs. 0.09 ± 0.08, P < 0.001), and higher percent ischemic myocardial mass (29.0 ± 18.5 vs. 26.0 ± 18.4, P = 0.022) than vessels without events. There was a significant association between the number of HRPCs and the number of clinical risk factors, FFRCT, ΔFFRCT, and percent ischemic myocardial mass. With an increased number of HRPCs, there was a significant increase in the number of clinical risk factors, ΔFFRCT, and percent ischemic myocardial mass and a significant decrease in FFRCT (overall P < 0.001 for all comparisons) (Supplementary Figure 1).
Prognostic Implications of Individual Anatomic and Hemodynamic Plaque Characteristics
Compared with clinical risk factors, individual components of HRPCs and hemodynamic parameters showed higher discriminant abilities for subsequent coronary events. Among anatomic and hemodynamic parameters, ΔFFRCT showed the highest c-index to predict subsequent coronary events (c-index 0.661, 95% CI: 0.609–0.712) (Figure 2).
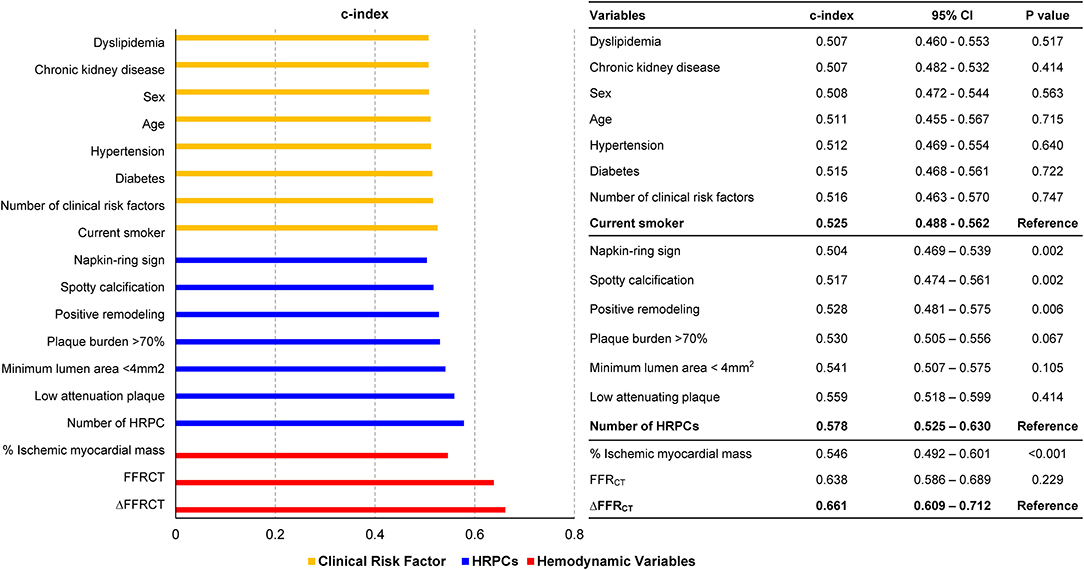
Figure 2. Comparison of discrimination abilities of clinical risk factors, HRPCs, and hemodynamic parameters for subsequent coronary events. Discrimination abilities of clinical risk factors (green bar), HRPCs (blue bar), and hemodynamic parameters (red bar) for subsequent coronary events are presented as c-index and 95% CI. For various variables in each group, the c-index comparison was performed with the variable with the highest c-index in each group. CI, confidence interval; FFRCT, fractional flow reserve by coronary computed tomography angiography; HRPCs, high-risk plaque characteristics.
Optimal cutoff values of hemodynamic parameters and the number of HRPCs for predicting subsequent coronary events were FFRCT ≤ 0.80, ΔFFRCT ≥ 0.06, percent ischemic myocardial mass ≥ 40%, and the number of HRPCs ≥ 3 (Supplementary Table 2). When the risk of subsequent coronary events was compared according to optimal cutoff values of hemodynamic parameters and the number of HRPCs, vessels with FFRCT ≤ 0.80, ΔFFRCT ≥ 0.06, percent ischemic myocardial mass ≥40%, and the number of HRPCs ≥3 were independently associated with an increased risk of subsequent coronary events than vessels with FFRCT > 0.80, ΔFFRCT < 0.06, percent ischemic myocardial mass <40%, and the number of HRPCs <3, respectively (Figure 3 and Table 3).
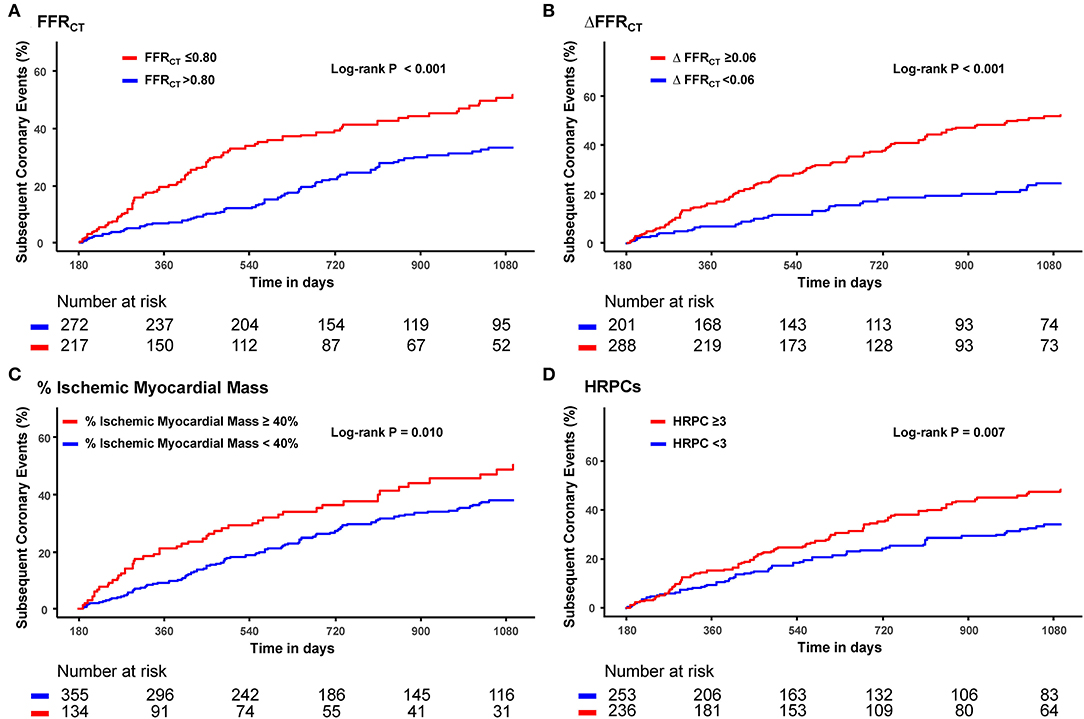
Figure 3. Cumulative incidence of subsequent coronary events according to FFRCT, ΔFFRCT, % ischemic myocardial mass, and HRPCs. Cumulative incidence of subsequent coronary events according to optimal cutoff values of (A) FFRCT, (B) ΔFFRCT, (C) % ischemic myocardial mass, and (D) HRPCs. FFRCT, fractional flow reserve by coronary computed tomography angiography; HRPCs, high-risk plaque characteristics.
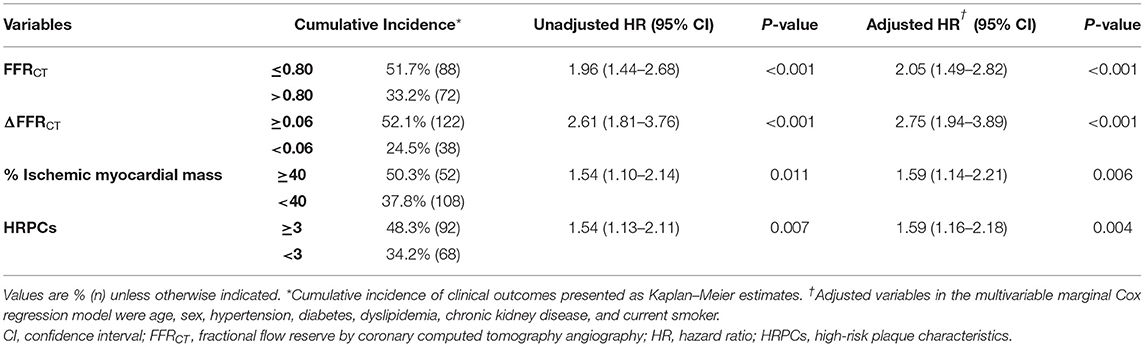
Table 3. Cumulative incidence of subsequent coronary events according to hemodynamic parameters and HRPCs.
However, the positive predictive value and diagnostic accuracy of each hemodynamic parameter and the number of HRPCs were modest to predict subsequent coronary events as individual parameters (Supplementary Table 2). Nevertheless, the addition of percent ischemic myocardial mass, HRPCs, FFRCT, and ΔFFRCT into clinical risk factors showed a stepwise increase in predictability for subsequent coronary events (Figure 4). Among anatomic and hemodynamic parameters, ΔFFRCT showed the highest incremental predictability of the other variables (P < 0.001 for comparisons with the others).
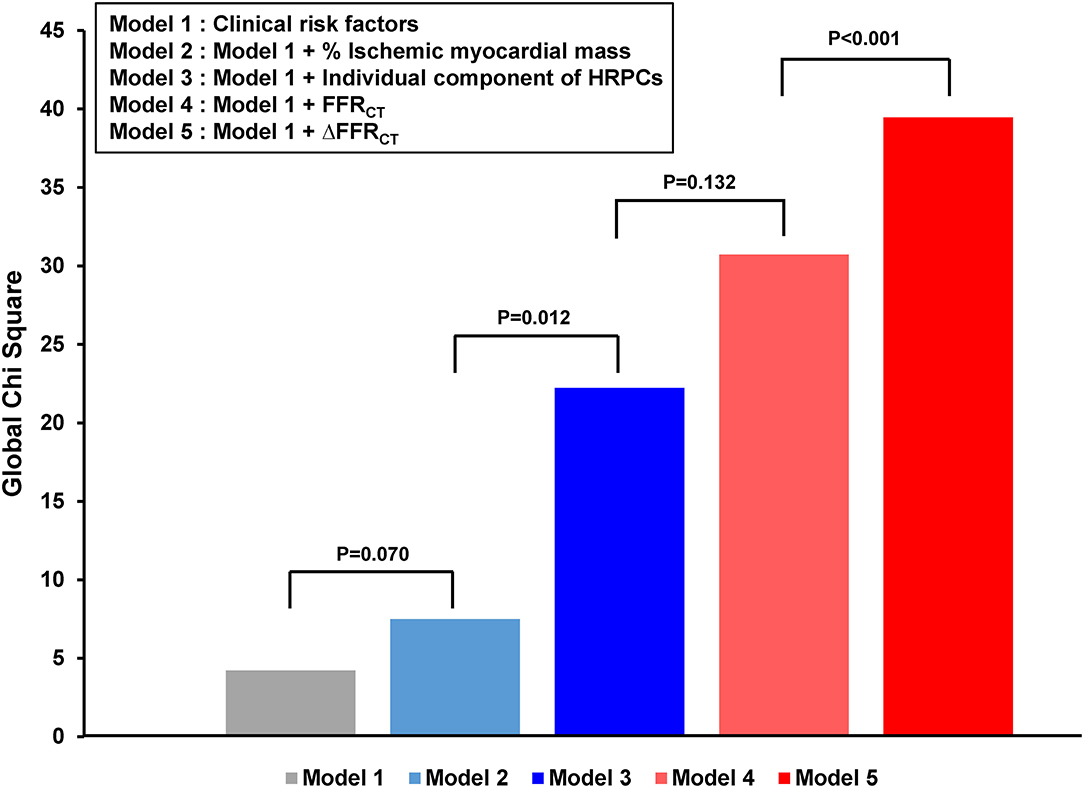
Figure 4. Incremental prognostic impact of HRPCs and hemodynamic parameters for subsequent coronary events. The addition of percent ischemic myocardial mass, HRPCs, ΔFFRCT, and ΔFFRCT into clinical risk factors showed increased predictability for subsequent coronary events. Clinical risk factors included age, sex, hypertension, diabetes mellitus, dyslipidemia, chronic kidney disease, and current smoker. HRPCs included low attenuation plaque, positive remodeling, napkin-ring sign, spotty calcification, minimum luminal area <4 mm2, or plaque burden ≥70%. FFRCT, fractional flow reserve by coronary computed tomography angiography; HRPCs, high-risk plaque characteristics.
Prediction Models for Subsequent Coronary Events
Table 4 and Supplementary Figure 2 show the comparison of discriminant and reclassification abilities of three models for the prediction of subsequent coronary events. Compared with model 1 with clinical risk factors, additional integration of HRPCs into model 1 (model 2) showed higher discriminant ability (c-index 0.620 vs. 0.558, P = 0.027) and higher reclassification ability (NRI 0.269, P = 0.004; IDI 0.037, P < 0.001). Model 3, which included additional integration of hemodynamic parameters in model 2, further improved model 2 in terms of discriminant ability (c-index 0.698 vs. 0.620, P = 0.001) and reclassification ability (NRI 0.460, P < 0.001; IDI 0.061, P < 0.001) (Table 4 and Supplementary Figure 2). Furthermore, a simplified model (c-index 0.680, 95% CI: 0.630–0.731) was constructed by selecting only the variables with the best discriminant abilities among HRPCs (low attenuating plaque) and hemodynamic variables (ΔFFRCT), respectively, and adding them to clinical risk factors showed similar discriminant ability to model 3 (c-index 0.680 vs. 0.698, P = 0.234).
Differential Prognostic Implication of HRPCs According to Hemodynamic Significance
To evaluate the differential prognostic implications of HRPCs according to hemodynamic significance, target vessels were stratified according to their hemodynamic significance determined by the optimal cutoff value of FFRCT ≤ 0.80 vs. >0.80 or ΔFFRCT ≥ 0.06 vs. <0.06. In vessels with FFRCT ≤ 0.80, there was no significant difference in the distribution of HRPCs among vessels with or without subsequent coronary events. Conversely, in vessels with FFRCT > 0.80, the vessels with subsequent coronary events showed a significantly higher proportion of low attenuating plaque and plaque burden ≥70% and showed a higher number of HRPCs (Supplementary Table 3). Stratified analysis by ΔFFRCT showed similar results, and the number of HRPCs was significantly higher in vessels with subsequent coronary events only among vessels with ΔFFRCT <0.06. Conversely, there was no significant difference in the number of HRPCs among vessels with ΔFFRCT ≥ 0.06 (Supplementary Table 4).
When the risk of subsequent coronary events was compared according to the number of HRPCs, a significantly higher risk of subsequent coronary events in vessels with HRPCs ≥ 3 than in vessels with HRPCs < 3 was observed only among vessels with negative FFRCT or ΔFFRCT (Figure 5). In addition, integration of HRPCs significantly improved discriminant and reclassification abilities for subsequent coronary events only in vessels with negative hemodynamic significance (FFRCT > 0.80; 0.687 vs. 0.576, P = 0.005; NRI 0.412, P = 0.002; IDI 0.064, P = 0.001; ΔFFRCT < 0.06; 0.733 vs. 0.623, P = 0.034; NRI 0.620, P < 0.001; IDI 0.075, P = 0.001) (Figure 6).
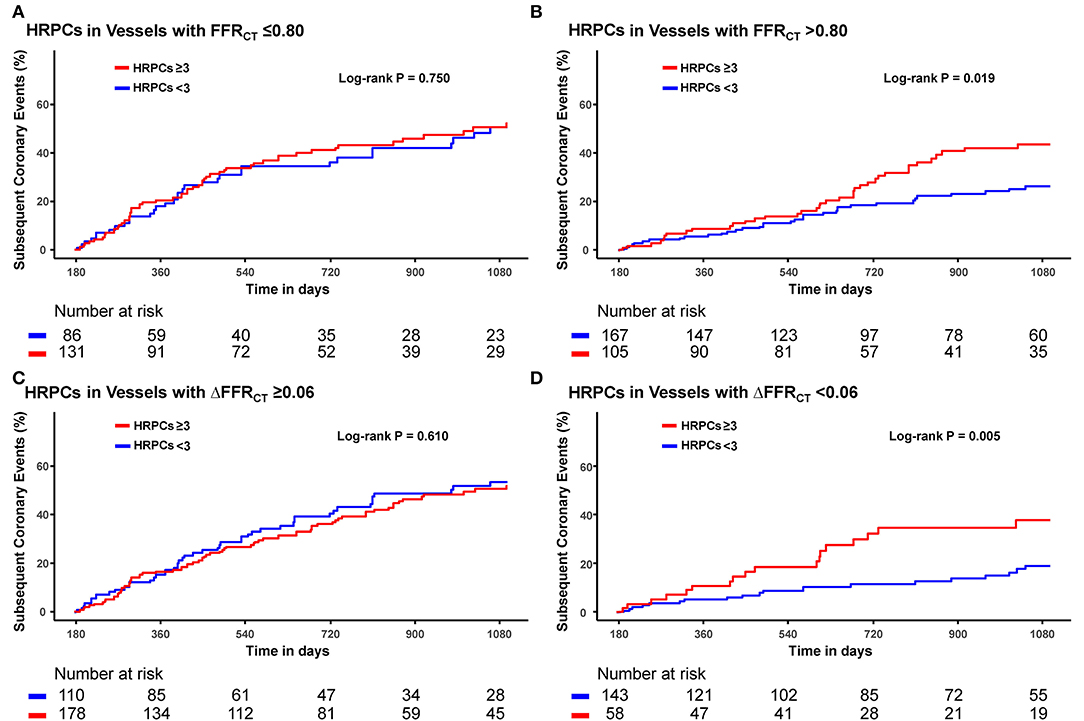
Figure 5. Differential prognostic impact of HRPCs for subsequent coronary events according to hemodynamic parameters. Differential prognostic impact of number of HRPCs of ≥3 is evaluated in vessels with (A) FFRCT ≤0.80, (B) FFRCT >0.80 (C) ΔFFRCT ≥0.06, and (D) ΔFFRCT <0.06. Abbreviations: FFRCT, fractional flow reserve by coronary computed tomography angiography; HRPCs, high-risk plaque characteristics.
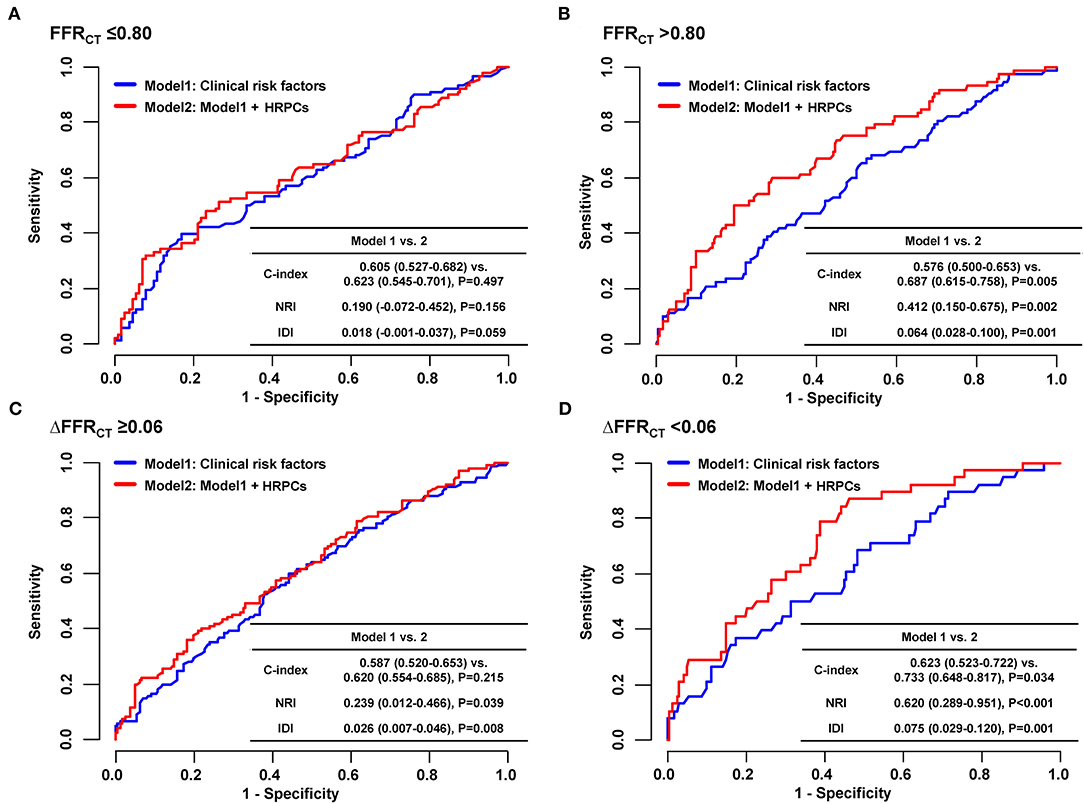
Figure 6. Incremental prognostic value of HRPCs for subsequent coronary events according to hemodynamic parameters. To assess incremental discriminatory and reclassification abilities of HRPCs in addition to clinical risk factors for subsequent coronary events, two models were constructed as follows: model 1: clinical risk factors (age, sex, hypertension, diabetes mellitus, dyslipidemia, chronic kidney disease, and current smoker) and model 2: clinical risk factors + HRPCs (low attenuation plaque, positive remodeling, napkin-ring sign, spotty calcification, minimal luminal area <4 mm2, or plaque burden ≥70%). Incremental discriminatory and reclassification abilities of HRPCs were evaluated in vessels with (A) FFRCT ≤ 0.80, (B) FFRCT > 0.80, (C) ΔFFRCT ≥ 0.06, and (D) ΔFFRCT < 0.06. FFRCT, fractional flow reserve by coronary computed tomography angiography; HRPCs, high-risk plaque characteristics; IDI, relative integrated discrimination improvement; NRI, category-free net reclassification index.
Discussion
This study evaluated (1) the prognostic implications of CCTA-derived anatomic and hemodynamic plaque characteristics to predict subsequent coronary events, and (2) the differential prognostic implications of anatomic plaque characteristics according to the hemodynamic significance of CAD. The main findings are as follows: First, HRPCs and hemodynamic parameters showed higher discriminant abilities for subsequent coronary events than clinical risk factors, with ΔFFRCT being the most powerful predictor. Second, HRPCs showed additive discriminant and reclassification abilities to clinical risk factors in the prediction of subsequent coronary events, which were further increased by adding hemodynamic parameters. Third, the prognostic impact of HRPCs was significant among vessels with negative hemodynamic significance (FFRCT >0.80 or ΔFFRCT <0.06) but not among those with positive hemodynamic significance (FFRCT ≤ 0.80 or ΔFFRCT ≥ 0.06).
Risk Stratification for Subsequent Coronary Events Using CCTA
Identification of patients at increased risk of ACS who may benefit from intensified preventive measures has been a major challenge, and previous studies have consistently shown that prediction based on clinical risk factors is insufficient for adequate individual risk assessment (1). In contemporary practice, patients with suspected ischemic heart disease are commonly evaluated by noninvasive stress testing, which determines the need for invasive coronary angiography (8). However, the revascularization is not indicated in two-third of the cases sent for invasive coronary angiography due to anatomically or hemodynamically nonobstructive stenosis in epicardial coronary arteries (18). Previous studies demonstrated that those nonobstructive CAD could be accompanied by major adverse cardiovascular events including ACS (19), and the treatment strategy based on noninvasive stress testing did not significantly reduce the risk of ACS or ACS-related mortality compared with medical treatment alone (20, 21).
Conversely, recent trials showed that CCTA-based evaluation of CAD provided better risk stratification of high-risk patients and improved prognosis compared with standard care (22). Furthermore, multiple studies showed that CCTA-derived anatomic plaque characteristics (MLA, plaque burden, positive remodeling, low-attenuation plaque, napkin-ring sign, and spotty calcification) could provide additional information on the risk of ACS (4, 5). In addition, other studies presented the prognostic implications of CCTA-derived hemodynamic parameters. The prognostic value of FFRCT has been confirmed for up to 5 years in several studies (10, 23, 24). Of note, in the ADVANCE registry, with the largest sample size (N = 5083), patients with negative FFRCT > 0.80 showed a significantly lower risk of cardiac death or MI than those with FFRCT ≤ 0.80 at 1 year (0.2% vs. 0.8%, P = 0.01) (10). The EMERALD study retrospectively evaluated 72 patients who had undergone CCTA before ACS events and compared CCTA-derived anatomic and hemodynamic plaque characteristics (FFRCT, ΔFFRCT, wall shear stress, and axial plaque stress) between the culprit and non-culprit vessels. In this study, plaques with adverse anatomic and hemodynamic characteristics had a significantly higher risk of ACS than those without (9). Despite the lack of a negative control group not presenting with ACS being a major limitation of the EMERALD study, the results supported the potential role of CCTA-based anatomic and hemodynamic plaque characteristics for better identification of high-risk patients. However, studies on the clinical role of the comprehensive evaluation of these features over clinical risk factors have been limited. Furthermore, there has been limited study, which evaluated the differential prognostic implications of CCTA-derived HRPCs according to the hemodynamic significance of CAD.
Increased Predictability by CCTA-Derived Anatomic and Hemodynamic Plaque Evaluation
As with previous studies (1), discriminant ability of clinical risk factors to predict subsequent coronary events was limited, and the c-index of clinical risk factors was lower than that of most CCTA-derived anatomic plaque characteristics. Among the CCTA-derived anatomic plaque characteristics, plaque burden ≥70%, MLA <4 mm2, and low attenuation plaque showed higher discrimination abilities for subsequent coronary events than any individual clinical risk factor or other parameters of HRPCs. As with previous results from the 3V-FFR-FRIENDS study (5), the number of HRPCs showed a higher discrimination ability than either individual parameters of HRPCs or clinical risk factors.
More importantly, CFD-derived hemodynamic parameters were more predictive for subsequent coronary events than clinical risk factors or HRPCs. The three CFD-derived hemodynamic parameters evaluated in this study, namely, FFRCT, ΔFFRCT, and percent ischemic myocardial mass, represent different aspects of hemodynamic significance in the target vessel territory. The FFRCT represents the severity of myocardial ischemia, whereas the percent ischemic myocardial mass represents the extent of myocardial ischemia. Furthermore, FFRCT reflects cumulative hemodynamic deprivation of the entire target vessel, representing vessel-level significance, whereas ΔFFRCT reflects the severity of local stenosis within the target vessel, representing lesion-level significance. Among these hemodynamic parameters, the discrimination abilities of FFRCT and ΔFFRCT were significantly higher than those of any clinical risk factors, individual HRPCs, or the number of HRPCs. These results support the contemporary practice guidelines that recommend treatment decisions based on the hemodynamic significance of the target lesion (8). Of note, ΔFFRCT showed the highest discriminant ability for subsequent coronary events, suggesting that lesion-level hemodynamic significance may be the most important determinant of subsequent coronary events among other anatomic and hemodynamic parameters, including vessel-level FFRCT.
In the prediction of subsequent coronary events, CCTA-derived HRPCs and CFD-derived hemodynamic parameters showed incremental predictability when added to clinical risk factors. The final model with clinical risk factors, HRPCs, and hemodynamic parameters showed significantly increased discrimination and reclassification abilities. Considering that CCTA enables simultaneous assessment of both HRPCs and hemodynamic parameters without additional scans or invasive procedures, radiation exposure, or use of hyperemic agents, it would be a practical diagnostic and prognostic stratification tool for patients with suspected CAD who may need intensive medical treatment to prevent plaque progression and rupture. Further study is warranted to incorporate this concept into daily practice.
Differential Prognostic Implications of HRPCs According to Hemodynamic Significance
Previous studies showed that there were associations among lesion severity, anatomic plaque characteristics, and hemodynamic lesion severity (2, 3, 5, 25). Similarly, we found that HRPCs were significantly associated with hemodynamic parameters, namely, FFRCT, ΔFFRCT, and percent ischemic myocardial mass. These associations can differ in each patient/lesion due to numerous patient- or lesion-specific parameters such as plaque content, presence of positive or negative remodeling, lesion location, or variation in coronary flow and microvascular function. Nevertheless, since current guidelines recommend treatment decisions based on hemodynamic significance but not the anatomic plaque characteristics, there has been an ongoing debate regarding the prognostic significance of HRPCs in lesions with negative hemodynamic significance. A recent 3V-FFR-FRIENDS study demonstrated that, among deferred vessels with FFR >0.80, those with ≥3 HRPCs showed a significantly higher risk of vessel-specific MI, revascularization, or cardiac death at 5 years compared with those with <3 HRPCs (5).
In this study, there was no additional role for HRPCs in predicting subsequent coronary events in vessels with positive hemodynamic significance (FFRCT ≤ 0.80 or ΔFFRCT < 0.06). Conversely, in vessels with negative hemodynamic significance, the presence of ≥3 HRPCs was independently associated with a higher risk of subsequent coronary events, and HRPCs showed an incremental discrimination ability when added to clinical risk factors. These results imply that additional evaluation of CCTA-based anatomic plaque characteristics in hemodynamically insignificant lesions might improve risk stratification in patients with CAD. Since ischemia-based imaging assessments with noninvasive stress tests cannot detect CAD without hemodynamic significance, CCTA-based anatomic plaque assessment would be helpful to select individuals at elevated risk for subsequent coronary events who could have been underdiagnosed by the stress tests. Furthermore, considering the differential prognostic implications of HRPCs according to hemodynamic significance, it should be further evaluated whether intensive medical therapy and/or preemptive PCI in hemodynamically insignificant lesions with HRPCs can induce stabilization of plaque characteristics (26) and eventually improve patient prognosis. Current ongoing trials (PREVENT [NCT02316886] and PROSPECT II [NCT02171065]) will help to clarify this issue.
Limitations
Some limitations should be acknowledged. First, this study has limitations related to the observational design of the study. Consequently, confounding bias may occur due to measured and unmeasured variables. Second, only patients who underwent invasive coronary angiography 6–36 months after CCTA were included in the study, which may have caused selection bias. Therefore, the current results may not be generalized to an overall population who underwent CCTA. Further external validation is needed in future studies. Third, vessels that were not suitable for anatomic and hemodynamic plaque characteristics analyses were excluded. This may also have caused selection bias. In particular, vessels with severe calcification were excluded from the analysis as they were considered suboptimal for plaque characterization. Fourth, the decision to perform CCTA and PCI was left to the operator's discretion. Fifth, neither group received adequate preventive medication according to the current consensus. However, this might reflect real-world practice and show the natural course of the patients. Sixth, it should be noted that the overall accuracy of models to predict future ACS occurrences was not very high. This might reflect the complex nature of the underlying mechanism of ACS.
Conclusion
In predicting subsequent coronary events, both HRPCs and hemodynamic parameters by CCTA allow for better prediction of subsequent coronary events than clinical risk factors alone. HRPCs provide incremental predictability than clinical risk factors among vessels with negative FFRCT but not among vessels with positive FFRCT (Figure 7).
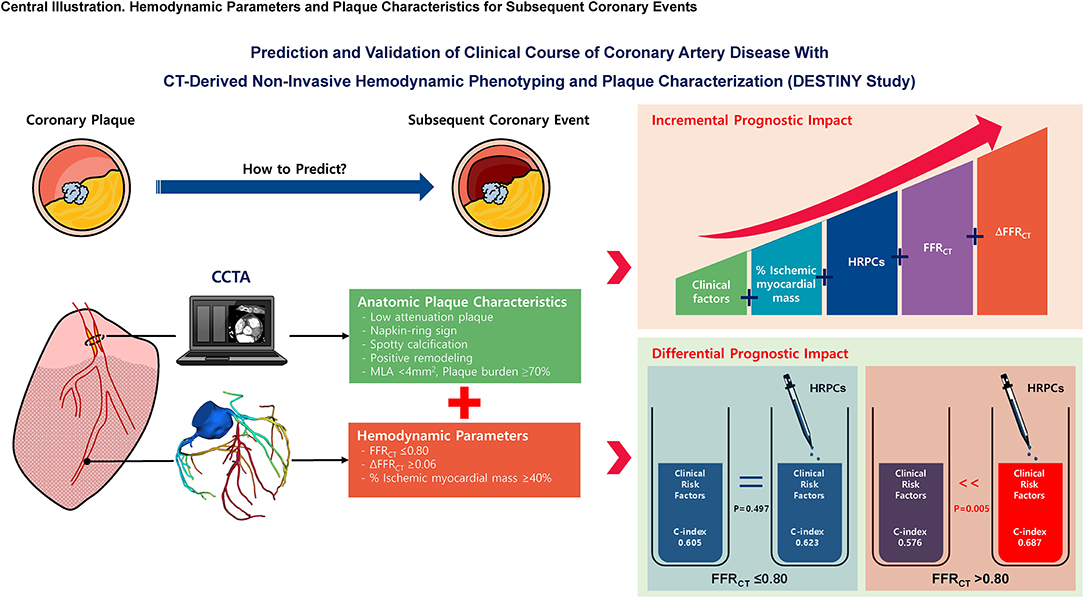
Figure 7. Hemodynamic parameters and plaque characteristics for subsequent coronary events. This study evaluated the prognostic implications of CCTA-derived HRPCs and hemodynamic parameters in the prediction of subsequent coronary events. Compared with clinical risk factors, HRPCs and hemodynamic parameters showed higher discriminant abilities for subsequent coronary events. HRPCs showed an additive discriminant ability to clinical risk factors, and hemodynamic parameters further increased discriminant ability for subsequent coronary events. Among vessels with negative FFRCT (>0.80), adding HRPCs into clinical risk factors significantly increased discriminant and reclassification abilities for subsequent coronary events but not for vessels with positive FFRCT (≤0.80). These results imply that additional evaluation of CCTA-based anatomic plaque characteristics in hemodynamically insignificant lesions might improve risk stratification in patients with CAD. FFRCT, fractional flow reserve by coronary computed tomography angiography; HRPCs, high-risk plaque characteristics; MLA, minimum lumen area.
Data Availability Statement
The datasets presented in this article are not readily available because data cannot be shared publicly due to the privacy of individuals that participated in the study. The data will be shared on reasonable request to the corresponding author. Requests to access the datasets should be directed to JL, ZHJvbmU4MEBoYW5tYWlsLm5ldA==.
Ethics Statement
The studies involving human participants were reviewed and approved by Samsung Medical Center. The patients/participants provided their written informed consent to participate in this study.
Author Contributions
SL, DH, and JL: conception, design, analysis, interpretation of data, drafting and revising of the manuscript, and final approval of the manuscript submitted. ND, DS, KC, SK, HK, K-HJ, SH, KL, TP, JY, YS, S-HC, YC, H-CG, and JG: interpretation of data, revising of the manuscript, and final approval of the manuscript submitted. All authors contributed to the article and approved the submitted version.
Conflict of Interest
JL received a Research Grant from St. Jude Medical (Abbott Vascular) and Philips Volcano.
The remaining authors declare that the research was conducted in the absence of any commercial or financial relationships that could be construed as a potential conflict of interest.
Publisher's Note
All claims expressed in this article are solely those of the authors and do not necessarily represent those of their affiliated organizations, or those of the publisher, the editors and the reviewers. Any product that may be evaluated in this article, or claim that may be made by its manufacturer, is not guaranteed or endorsed by the publisher.
Supplementary Material
The Supplementary Material for this article can be found online at: https://www.frontiersin.org/articles/10.3389/fcvm.2022.871450/full#supplementary-material
Abbreviations
ACS, acute coronary syndrome; CAD, coronary atherosclerotic disease; CCTA, coronary computed tomography angiography; CFD, computational fluid dynamics; FFRCT, fractional flow reserve by coronary computed tomography angiography; HR, hazard ratio; HRPCs, high-risk plaque characteristics; MI, myocardial infarction; PCI, percutaneous coronary intervention.
References
1. D'Agostino RB, Vasan RS, Pencina MJ, Wolf PA, Cobain M, Massaro JM, et al. General cardiovascular risk profile for use in primary care: the framingham heart study. Circulation. (2008) 117:743–53. doi: 10.1161/CIRCULATIONAHA.107.699579
2. Stone GW, Maehara A, Lansky AJ, de Bruyne B, Cristea E, Mintz GS, et al. A prospective natural-history study of coronary atherosclerosis. N Engl J Med. (2011) 364:226–35. doi: 10.1056/NEJMoa1002358
3. Cheng JM, Garcia-Garcia HM, de Boer SP, Kardys I, Heo JH, Akkerhuis KM, et al. In vivo detection of high-risk coronary plaques by radiofrequency intravascular ultrasound and cardiovascular outcome: results of the atheroremo-ivus study. Eur Heart J. (2014) 35:639–47. doi: 10.1093/eurheartj/eht484
4. Motoyama S, Ito H, Sarai M, Kondo T, Kawai H, Nagahara Y, et al. Plaque Characterization by coronary computed tomography angiography and the likelihood of acute coronary events in mid-term follow-up. J Am Coll Cardiol. (2015) 66:337–46. doi: 10.1016/j.jacc.2015.05.069
5. Lee JM, Choi KH, Koo BK, Park J, Kim J, Hwang D, et al. Prognostic implications of plaque characteristics and stenosis severity in patients with coronary artery disease. J Am Coll Cardiol. (2019) 73:2413–24. doi: 10.1016/j.jacc.2019.02.060
6. Chang HJ, Lin FY, Lee SE, Andreini D, Bax J, Cademartiri F, et al. Coronary Atherosclerotic Precursors of Acute Coronary Syndromes. J Am Coll Cardiol. (2018) 71:2511–22. doi: 10.1016/j.jacc.2018.02.079
7. Koskinas KC, Ughi GJ, Windecker S, Tearney GJ, Raber L. Intracoronary imaging of coronary atherosclerosis: validation for diagnosis, prognosis and treatment. Eur Heart J. (2016) 37:524–35a-c. doi: 10.1093/eurheartj/ehv642
8. Knuuti J, Wijns W, Saraste A, Capodanno D, Barbato E, Funck-Brentano C, et al. 2019 Esc guidelines for the diagnosis and management of chronic coronary syndromes. Eur Heart J. (2020) 41:407–77. doi: 10.1093/eurheartj/ehz425
9. Lee JM, Choi G, Koo BK, Hwang D, Park J, Zhang J, et al. Identification of high-risk plaques destined to cause acute coronary syndrome using coronary computed tomographic angiography and computational fluid dynamics. JACC Cardiovasc Imaging. (2019) 12:1032–43. doi: 10.1016/j.jcmg.2018.01.023
10. Patel MR, Norgaard BL, Fairbairn TA, Nieman K, Akasaka T, Berman DS, et al. 1-year impact on medical practice and clinical outcomes of ffrct: the advance registry. JACC Cardiovasc Imaging. (2020) 13:97–105. doi: 10.1016/j.jcmg.2019.03.003
11. Taylor AJ, Cerqueira M, Hodgson JM, Mark D, Min J, O'Gara P, et al. Accf/Scct/Acr/Aha/Ase/Asnc/Nasci/Scai/Scmr 2010 appropriate use criteria for cardiac computed tomography. a report of the American College of Cardiology Foundation Appropriate Use Criteria Task Force, the Society of Cardiovascular Computed Tomography, the American College of Radiology, the American Heart Association, the American Society of Echocardiography, the American Society of Nuclear Cardiology, the North American Society for Cardiovascular Imaging, the Society for Cardiovascular Angiography and Interventions, and the Society for Cardiovascular Magnetic Resonance. J Cardiovasc Comput Tomogr. (2010) 4:407 e1-33. doi: 10.1016/j.jcct.2010.11.001
12. van Assen M, Varga-Szemes A, Schoepf UJ, Duguay TM, Hudson HT, Egorova S, et al. Automated plaque analysis for the prognostication of major adverse cardiac events. Eur J Radiol. (2019) 116:76–83. doi: 10.1016/j.ejrad.2019.04.013
13. Buckler AJ, Karlöf E, Lengquist M, Gasser TC, Maegdefessel L, Perisic Matic L, et al. Virtual transcriptomics: noninvasive phenotyping of atherosclerosis by decoding plaque biology from computed tomography angiography imaging. Arterioscler Thromb Vasc Biol. (2021) 41:1738–50. doi: 10.1161/ATVBAHA.121.315969
14. Ihdayhid AR, Norgaard BL, Achenbach S, Khav N, Gaur S, Leipsic J, et al. Ischemic myocardial burden subtended by computed tomography-derived fractional flow reserve (Approach(Ffrct)): an exploratory analysis on diagnostic performance. JACC Cardiovasc Imaging. (2020) 13:2264–7. doi: 10.1016/j.jcmg.2020.05.008
15. West GB, Brown JH, Enquist BJ. A General model for the origin of allometric scaling laws in biology. Science. (1997) 276:122–6. doi: 10.1126/science.276.5309.122
16. Kim HY, Doh JH, Lim HS, Nam CW, Shin ES, Koo BK, et al. Identification of coronary artery side branch supplying myocardial mass that may benefit from revascularization. JACC Cardiovasc Interv. (2017) 10:571–81. doi: 10.1016/j.jcin.2016.11.033
17. Thygesen K, Alpert JS, Jaffe AS, Chaitman BR, Bax JJ, Morrow DA, et al. Fourth universal definition of myocardial infarction (2018). J Am Coll Cardiol. (2018) 72:2231–64. doi: 10.1016/j.jacc.2018.08.1038
18. Patel MR, Peterson ED Dai D, Brennan JM, Redberg RF, Anderson HV, et al. Low diagnostic yield of elective coronary angiography. N Engl J Med. (2010) 362:886–95. doi: 10.1056/NEJMoa0907272
19. Fishbein MC, Siegel RJ. How big are coronary atherosclerotic plaques that rupture? Circulation. (1996) 94:2662–6. doi: 10.1161/01.CIR.94.10.2662
20. Sedlis SP, Hartigan PM, Teo KK, Maron DJ, Spertus JA, Mancini GB, et al. Effect of Pci on long-term survival in patients with stable ischemic heart disease. N Engl J Med. (2015) 373:1937–46. doi: 10.1056/NEJMoa1505532
21. Maron DJ, Hochman JS, Reynolds HR, Bangalore S, O'Brien SM, Boden WE, et al. Initial invasive or conservative strategy for stable coronary disease. N Engl J Med. (2020) 382:1395–407. doi: 10.1056/NEJMoa1915922
22. Investigators S-H, Newby DE, Adamson PD, Berry C, Boon NA, Dweck MR, et al. Coronary Ct angiography and 5-year risk of myocardial infarction. N Engl J Med. (2018) 379:924–33. doi: 10.1056/NEJMoa1805971
23. Douglas PS, De Bruyne B, Pontone G, Patel MR, Norgaard BL, Byrne RA, et al. 1-Year outcomes of Ffrct-guided care in patients with suspected coronary disease: the platform study. J Am Coll Cardiol. (2016) 68:435–45. doi: 10.1016/j.jacc.2016.05.057
24. Norgaard BL, Terkelsen CJ, Mathiassen ON, Grove EL, Botker HE, Parner E, et al. Coronary Ct angiographic and flow reserve-guided management of patients with stable ischemic heart disease. J Am Coll Cardiol. (2018) 72:2123–34. doi: 10.1016/j.jacc.2018.07.043
25. Driessen RS, Stuijfzand WJ, Raijmakers PG, Danad I, Min JK, Leipsic JA, et al. Effect of plaque burden and morphology on myocardial blood flow and fractional flow reserve. J Am Coll Cardiol. (2018) 71:499–509. doi: 10.1016/j.jacc.2017.11.054
Keywords: coronary artery disease, coronary CT angiography, myocardial ischemia, vulnerable plaques, prognosis
Citation: Lee SH, Hong D, Dai N, Shin D, Choi KH, Kim SM, Kim HK, Jeon K-H, Ha SJ, Lee KY, Park TK, Yang JH, Song YB, Hahn J-Y, Choi S-H, Choe YH, Gwon H-C, Ge J and Lee JM (2022) Anatomic and Hemodynamic Plaque Characteristics for Subsequent Coronary Events. Front. Cardiovasc. Med. 9:871450. doi: 10.3389/fcvm.2022.871450
Received: 08 February 2022; Accepted: 19 April 2022;
Published: 23 May 2022.
Edited by:
Sara Seitun, San Martino Polyclinic Hospital IRCCS, ItalyReviewed by:
Leandro Slipczuk, Montefiore Health System, United StatesAndreas Mitsis, Medical School, University of Cyprus, Cyprus
Stefano Benenati, San Martino Hospital (IRCCS), Italy
Copyright © 2022 Lee, Hong, Dai, Shin, Choi, Kim, Kim, Jeon, Ha, Lee, Park, Yang, Song, Hahn, Choi, Choe, Gwon, Ge and Lee. This is an open-access article distributed under the terms of the Creative Commons Attribution License (CC BY). The use, distribution or reproduction in other forums is permitted, provided the original author(s) and the copyright owner(s) are credited and that the original publication in this journal is cited, in accordance with accepted academic practice. No use, distribution or reproduction is permitted which does not comply with these terms.
*Correspondence: Joo Myung Lee, ZHJvbmU4MEBoYW5tYWlsLm5ldA==; am9vbXl1bmcubGVlQHNhbXN1bmcuY29t
†These authors have contributed equally to this work