- 1Wallace H. Coulter Department of Biomedical Engineering at the Georgia Institute of Technology and Emory University, Atlanta, GA, United States
- 2Department of Biomedical Engineering, University of Massachusetts Lowell, Lowell, MA, United States
- 3Department of Radiology, St. Paul’s Hospital, The University of British Columbia, Vancouver, BC, Canada
- 4Structural Heart and Valve Center, Emory University Hospital, Atlanta, GA, United States
- 5Cardiovascular Branch, Division of Intramural Research, National Heart Lung and Blood Institute, National Institutes of Health, Bethesda, MD, United States
- 6Department of Radiology and Imaging Science, Emory University School of Medicine, Atlanta, GA, United States
Background: A clinical study comparing the hemodynamic outcomes of transcatheter mitral valve replacement (TMVR) with vs. without Laceration of the Anterior Mitral leaflet to Prevent Outflow Obstruction (LAMPOON) has never been designed nor conducted.
Aims: To quantify the hemodynamic impact of LAMPOON in TMVR using patient-specific computational (in silico) models.
Materials: Eight subjects from the LAMPOON investigational device exemption trial were included who had acceptable computed tomography (CT) data for analysis. All subjects were anticipated to be at prohibitive risk of left ventricular outflow tract (LVOT) obstruction from TMVR, and underwent successful LAMPOON immediately followed by TMVR. Using post-procedure CT scans, two 3D anatomical models were created for each subject: (1) TMVR with LAMPOON (performed procedure), and (2) TMVR without LAMPOON (virtual control). A validated computational fluid dynamics (CFD) paradigm was then used to simulate the hemodynamic outcomes for each condition.
Results: LAMPOON exposed on average 2 ± 0.6 transcatheter valve cells (70 ± 20 mm2 total increase in outflow area) which provided an additional pathway for flow into the LVOT. As compared to TMVR without LAMPOON, TMVR with LAMPOON resulted in lower peak LVOT velocity, lower peak LVOT gradient, and higher peak LVOT effective orifice area by 0.4 ± 0.3 m/s (14 ± 7% improvement, p = 0.006), 7.6 ± 10.9 mmHg (31 ± 17% improvement, p = 0.01), and 0.2 ± 0.1 cm2 (17 ± 9% improvement, p = 0.002), respectively.
Conclusion: This was the first study to permit a quantitative, patient-specific comparison of LVOT hemodynamics following TMVR with and without LAMPOON. The LAMPOON procedure achieved a critical increment in outflow area which was effective for improving LVOT hemodynamics, particularly for subjects with a small neo-left ventricular outflow tract (neo-LVOT).
Introduction
Left ventricular outflow tract (LVOT) obstruction is a prevalent and potentially fatal complication of transcatheter mitral valve replacement (TMVR) caused by displacement of the anterior leaflet toward the ventricular septum (1). Laceration of the Anterior Mitral leaflet to Prevent Outflow Obstruction (LAMPOON) is a catheter-based technique designed to alleviate the risk of obstruction by mimicking surgical anterior leaflet resection. The optimal result of the LAMPOON procedure is a complete midline laceration of the anterior leaflet which, following TMVR, exposes open cells of the transcatheter valve. These exposed cells are thought to permit additional blood flow through the LVOT and decrease the risk of obstruction (2). LAMPOON is considered for patients who have a predicted residual LVOT neo-left ventricular outflow tract (neo-LVOT) area <200 mm2 and are therefore anticipated to have significant LVOT obstruction from TMVR (3).
Despite the growing clinical experience with this technique, a controlled clinical trial comparing the outcomes of patients undergoing TMVR with vs. without LAMPOON has never been designed nor conducted. It would be impossible to conduct such a trial in which the control intervention (i.e., TMVR without LAMPOON) would be anticipated to cause immediate LVOT obstruction following valve implantation. Thus, while the LAMPOON technique has been shown to be safe and feasible, its impact on left ventricular outflow hemodynamics has never been investigated.
For this purpose, we conducted a computational (in silico) controlled study of TMVR with LAMPOON vs. TMVR without LAMPOON. We used a validated in silico approach using post-procedure computed tomography (CT) scans from subjects at prohibitive risk of LVOT obstruction who successfully underwent TMVR with LAMPOON (4). 3-D anatomical and flow information were extracted from the CT datasets and used as inputs into personalized computational fluid dynamics (CFD) models. For each subject, hemodynamics of TMVR with and without LAMPOON were simulated and compared. We hypothesized that LAMPOON improves flow dynamics through the LVOT in patients with a small neo-LVOT.
Materials and Methods
LAMPOON Trial Dataset
De-identified subject data from the LAMPOON investigational device exemption trial (NCT03015194) were retrospectively evaluated under an Institutional Review Board approved protocol (4). All subjects in that trial (N = 30) were considered at prohibitive risk for TMVR-related LVOT obstruction based on a predicted end-systolic neo-LVOT area of <200 mm2. Subjects underwent a valve-in-ring or valve-in-mitral annular calcification procedure with the SAPIEN 3 valve (Edwards Lifesciences, Irvine, CA, United States) with retrograde LAMPOON prior to valve implantation. The LAMPOON technique and 30-day clinical outcomes have been previously reported (2, 4). Briefly, this technique involves three main steps: (1) anterior leaflet traversal with a guidewire, followed by (2) leaflet laceration by electrifying and pulling the guidewire from base to tip, immediately followed by (3) TMVR. Complete details on the utilized retrograde technique and newer LAMPOON technique iterations were recently described (5).
Selection of Study Cohort
Inclusion criteria for this study were: (1) the availability of a post-procedure, multi-phase CT scan with contrast-enhancement, and (2) a splayed anterior leaflet that was visible on post-procedure CT and exposed cells of the implanted transcatheter valve. Of the thirty subjects in the LAMPOON investigational device exemption trial, sixteen subjects did not have adequate visualization of the anterior mitral leaflet on the post-procedure CT scan due to image quality, and two subjects did not have an available post-procedure CT due to patient death. Of the remaining twelve subjects, four had CTs in which we could not visualize exposed cells of the transcatheter valve, despite a successful anterior leaflet laceration. Thus, eight subjects were selected for the present study who had a splayed anterior leaflet which exposed open cells of the implanted transcatheter valve, as verified on post-procedure CT. These eight subjects were at risk of a fixed LVOT obstruction from TMVR due to a small predicted neo-LVOT, and not a dynamic obstruction due to a long anterior leaflet.
Post-procedure Computed Tomography Datasets
Contrast-enhanced, multi-phase CT scans were acquired after the procedure, either pre-discharge or within 30-days of follow-up. CT images were reconstructed in 10% intervals over the cardiac cycle within <1.0-mm slice thickness. Mimics 20.0 (Materialize, Leuven, Belgium) image post-processing software was used to generate 3-D models of the left ventricle and aorta using previously described segmentation techniques (6). The left ventricular volume was segmented from the CT images for all phases across the cardiac cycle, and the time rate of change in volume was calculated to provide time-varying flow rate. This method for deriving flow rate from left ventricular volumes has been described previously (7).
3D Models Created for Each Subject
Two different 3D models were created for each subject using the post-procedure CT datasets. The first model represented “TMVR with LAMPOON.” In this model, the actual implanted transcatheter valve and splayed anterior leaflet were reconstructed in 3D from the post-procedure CT (Figure 1, left). These reconstructions of the implanted valve therefore incorporated the actual valve deployment depth, angulation, and expansion characteristics. The positions of transcatheter valve cells exposed by LAMPOON were identified visually on the post-procedure CT, and these exposed cells were intentionally left patent in this model.
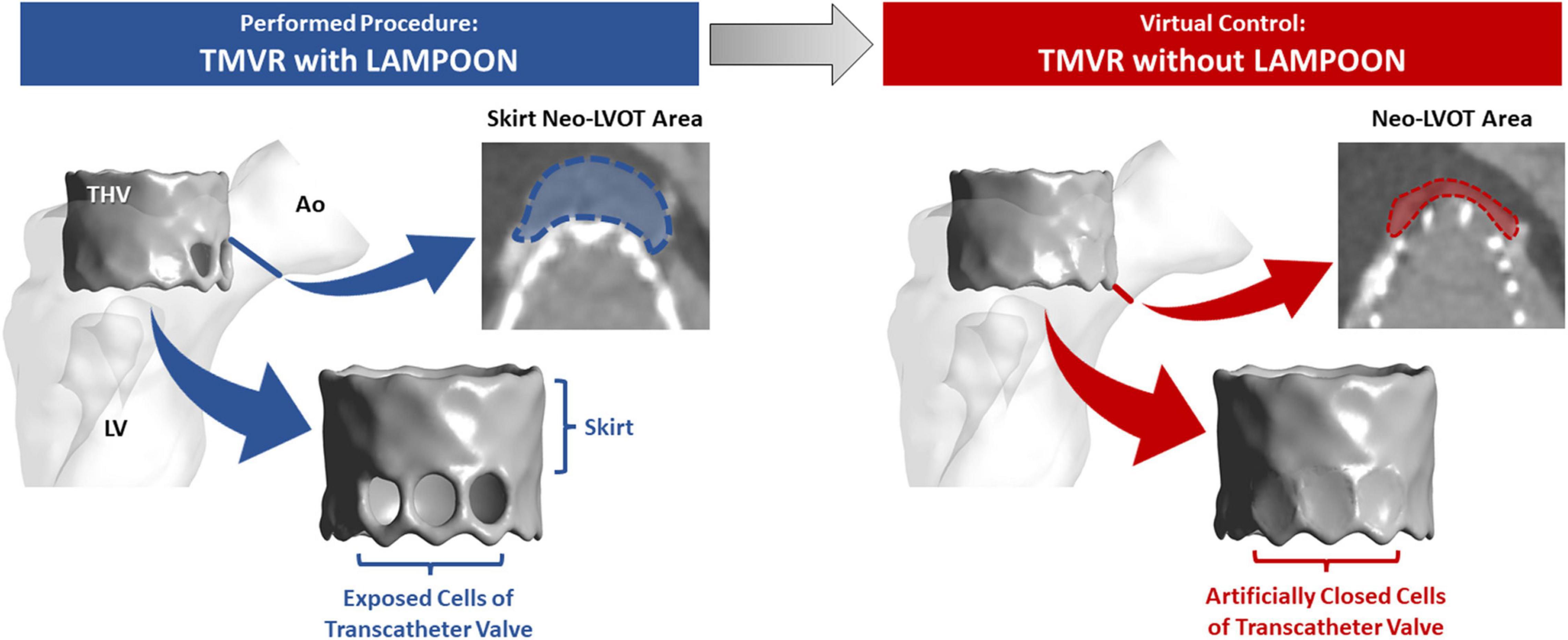
Figure 1. 3D anatomical models created for each subject. The left model represents the actual performed procedure (TMVR with LAMPOON). In this model, the left ventricular anatomy and implanted transcatheter valve geometry were segmented directly from the post-procedure CT dataset. The number and location of transcatheter valve cells exposed by LAMPOON were modeled based on visualization of the anterior leaflet splay in the post-procedure CT dataset. To simulate a “virtual control” procedure in which LAMPOON was not performed (TMVR without LAMPOON), we artificially closed the exposed cells of the transcatheter valve as shown in the right model. Ao, aorta; LV, left ventricle; THV, transcatheter heart valve.
The second model represented “TMVR without LAMPOON.” In this model, the exposed cells of the transcatheter valve were artificially closed, mimicking a completely intact anterior leaflet geometry (Figure 1, right). Since the model of “TMVR with LAMPOON” mimicked the performed interventional procedure, the model of “TMVR without LAMPOON” was considered to mimic a control “virtual procedure.” Both models were designed to be anatomically identical other than the presence or absence of the splayed anterior leaflet.
Measurement of Neo-Left Ventricular Outflow Tract and Skirt Neo-Left Ventricular Outflow Tract Area
Computed tomography datasets were utilized to measure the neo-LVOT and skirt neo-LVOT area. The neo-LVOT area was measured in a plane perpendicular to the LVOT and located at the narrowest point along the residual LVOT after TMVR (Figure 1, right). Following LAMPOON, the transcatheter valve skirt still protrudes into the LVOT, creating a narrowing at the transcatheter valve skirt called the “skirt” neo-LVOT (8). Figure 1 illustrates differences between the neo-LVOT and skirt neo-LVOT. The skirt neo-LVOT area was measured in a plane perpendicular to the LVOT and located at the level of the transcatheter valve skirt (Figure 1, left). Both neo-LVOT and skirt neo-LVOT area measurements were first predicted on baseline CT, and then measured on post-procedure CT.
Computational Fluid Dynamics Simulations
Computational fluid dynamics (CFD) is a method used to simulate fluid flow with computer modeling. In this study, we utilized a validated CFD workflow for simulating patient-specific LVOT hemodynamics based on cardiac CT data (7). Patient-specific CFD simulations were performed for both modeled conditions (TMVR with and without LAMPOON) for each subject. The output of these simulations provided spatially varying velocity and pressure data across the entire left ventricle and aorta. The workflow used for performing the CFD simulations has been previously described and validated against clinical measurements from Doppler echocardiography (7). The average difference between CFD-derived and echocardiography-derived peak LVOT velocity measurements was <10%, indicating a good agreement between the CFD simulations and clinical measurements. This CFD workflow is briefly summarized below.
All CFD simulations were performed for the peak-systolic phase of the cardiac cycle. The cardiac phase of the post-procedure CT scan which most closely coincided with the peak systolic flow rate was selected for creating a 3D model of the left heart anatomy and implanted transcatheter valve. For each 3D model, an inlet was placed at the left ventricular apex, and an outlet was placed at the sino-tubular junction. 3-Matic 12.0 (Materialize, Leuven, Belgium) computer aided design software was used to place a virtual wall at the level of the bioprosthetic valve leaflets to prevent regurgitant flow. A volumetric mesh was then generated using ANSYS Fluent Meshing (Ansys, Inc. Canonsburg, PA, United States). This mesh included polyhedral elements with an edge length of 0.5 mm, and a prismatic boundary layer mesh (10 layers with geometric growth of 1.05) which was applied on the left ventricular wall. The total number of mesh elements per patient model was on average ∼1,800,000 elements. Transition-to-turbulence characteristics in the flow field were accounted for using the scale-adaptive simulation turbulence model. Turbulence boundary conditions included (1) hydraulic diameter (which is the inlet diameter, DH) and (2) turbulence intensity (approximated using 0.16 × ReD–0.125, where ReD is the Reynolds number based on the patient-specific flow rate and the hydraulic diameter).
The time-varying left ventricular volume curve (obtained from the post-procedure CT scan) was used to calculate a peak systolic flow rate, which was then prescribed as the inlet boundary condition. The outlet boundary condition was set to a zero-reference pressure. All structures were assumed to be rigid and non-permeable, and a no-slip boundary condition was applied at the walls. Blood was modeled as a single-phase Newtonian fluid with a density of 1060 kg/m3 and dynamic viscosity of 0.0034 poise. The 3-D Navier-Stokes equations were solved in ANSYS Fluent 19.0 using the SIMPLE scheme for pressure-velocity coupling. A bounded second-order implicit transient formulation was utilized with a time step of 0.001 s. The solution was considered converged when residuals in momentum and turbulence variables declined below 10–4. Three-thousand time steps were simulated, with the last time step being used for data analysis.
Obtaining Simulated Hemodynamics
Hemodynamic metrics were extracted from each CFD simulation, including the peak LVOT velocity (Vpeak), peak LVOT pressure gradient (ΔPpeak), and peak LVOT effective orifice area (EOApeak). To calculate ΔPpeak, the ventricular pressure was derived from an average pressure across the model inlet (LV apical plane), and the aortic pressure was derived from an average pressure across the model outlet (sino-tubular junction plane). ΔPpeak was then calculated as the difference between the ventricular and aortic pressures. EOApeak was calculated as the ratio of the peak volumetric flow rate (Qpeak) to the peak outflow velocity (Vpeak) (9).
Data Analysis
All data analyses were performed with the MedCalc statistical software package (MedCalc, Ostend, Belgium). Simulated hemodynamics for each subject were compared between the two modeled conditions (TMVR with and without LAMPOON) using the paired samples t-test or Wilcoxon test for parametric and non-parametric data, respectively. Statistical significance was defined as p < 0.05.
Results
Subject Characteristics
Baseline, procedural and post-procedural characteristics are shown in Table 1. All subjects were considered to be at prohibitive risk of LVOT obstruction based on small ventricular anatomy and small LVOT clearance (end-systolic neo-LVOT area <200 mm2 as predicted on baseline CT), and therefore were considered candidates for the LAMPOON procedure. All subjects included were female, likely due to the selection of patients with small ventricular anatomy for LAMPOON. The indication for mitral valve replacement was mitral regurgitation in three subjects, and mitral stenosis in five subjects.
All subjects underwent successful midline anterior leaflet laceration (LAMPOON procedure) immediately followed by investigational use of the SAPIEN 3 transcatheter heart valve in the mitral position. The setting of valve replacement was valve-in-ring for two subjects, and valve-in-mitral annular calcification for six subjects. Following valve implantation, all subjects had trace or no para-valvular regurgitation. The number of transcatheter valve cells exposed by the splayed anterior mitral leaflet ranged from 1 to 3 cells. The combined area of the exposed cells ranged from 41 to 88 mm2, and the area of a single exposed cell was on average 36 ± 7 mm2. Valve deployment depth (percent deployed in left ventricle) ranged from 39 to 85%. At follow up, the peak systolic flow rate (calculated from the post-procedure CT-derived LV volumes) ranged from 13 to 38 L/min.
Effect of LAMPOON on Simulated Left Ventricular Flow Patterns
Simulations of left ventricular flow patterns are depicted by color maps (red = highest velocity, blue = lowest velocity) with streamlines for a representative subject (Subject 5) (Figure 2). For the condition of the virtual control procedure (TMVR without LAMPOON), flow acceleration occurred at the level of the neo-LVOT, resulting in a narrowed outflow jet (Figure 2, left panel). In this condition, the neo-LVOT was shown to be the predominant geometric narrowing for systolic flow. All forward flow was directed through the neo-LVOT, causing an increase in velocity at this location due to the reduced flow area. Additionally, there was a region of reduced flow adjacent to the transcatheter valve due to the narrowed outflow jet, as noted in the neo-LVOT short-axis view (Figure 2, upper left image).
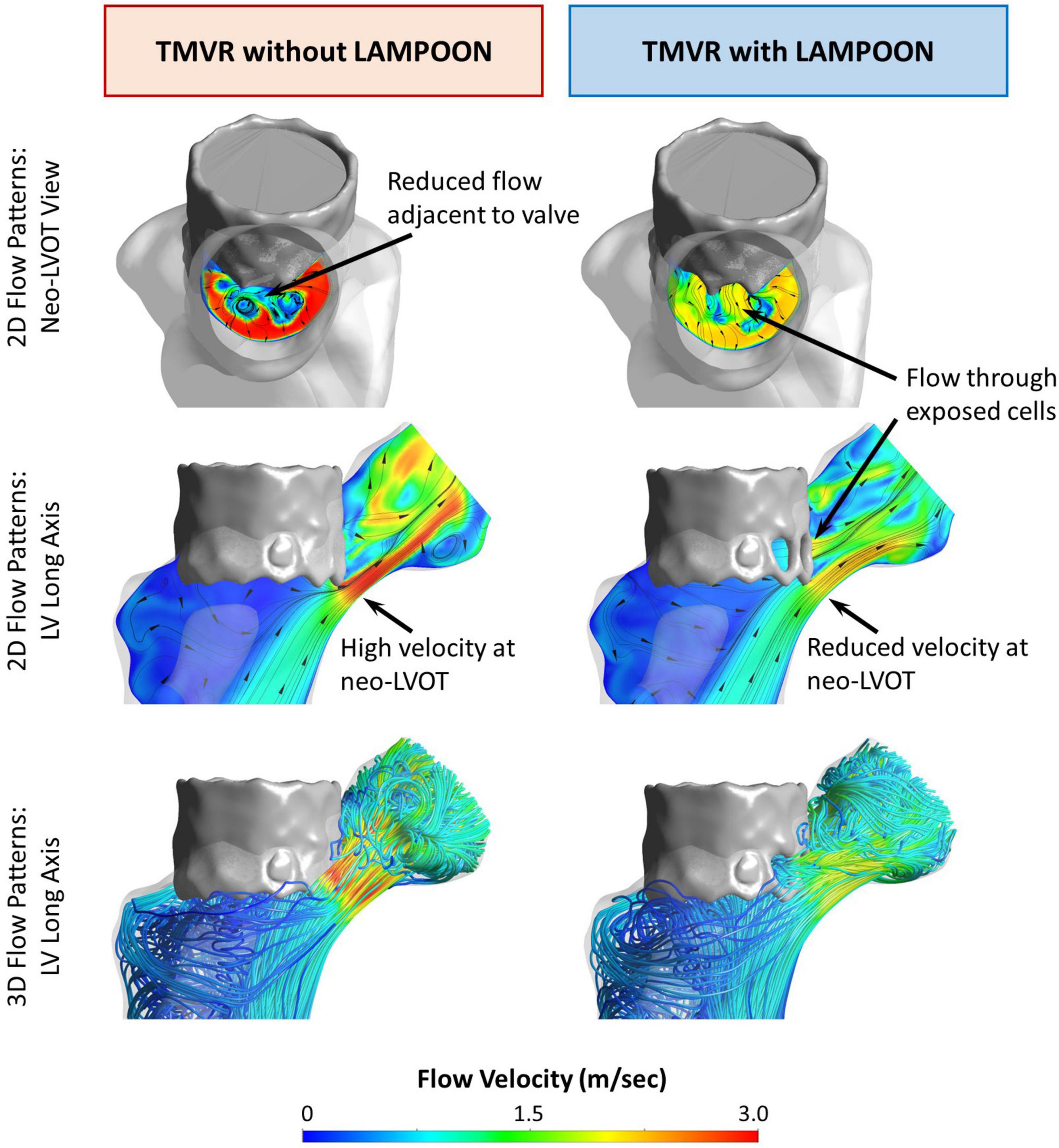
Figure 2. Effect of LAMPOON on left ventricular flow patterns. Simulated flow patterns for a representative subject (Subject 5) are shown in color maps (red = highest velocity, blue = lowest velocity) with streamlines. The left column shows the flow simulation for the virtual control procedure (TMVR without LAMPOON). For this condition, all flow is directed through the narrowed residual LVOT (neo-LVOT), resulting in a narrowed and high-velocity outflow jet. Additionally, there is a region of reduced flow in the immediate vicinity of the valve (upper left image). The right column shows the flow simulation for the actual performed procedure (TMVR with LAMPOON). For this condition, additional flow occurs through exposed cells of the transcatheter valve due to LAMPOON. Following LAMPOON, the velocity of flow through the neo-LVOT is reduced, and there is improved flow in the immediate vicinity of the valve (upper right image). Ao, aorta; LV, left ventricle; neo-LVOT, neo-left ventricular outflow tract; THV, transcatheter heart valve.
For the condition of the performed interventional procedure (TMVR with LAMPOON), flow occurred both through the neo-LVOT and through exposed cells of the transcatheter valve (Figure 2, right panel). LAMPOON provided an additional source of flow into the LVOT, leading to a reduction in outflow velocity due to the increased flow area. The region of reduced flow adjacent to the valve in the case of TMVR without LAMPOON was decreased in the case of TMVR with LAMPOON, thus reflecting flow closer to normal flow physiology (Figure 2, upper right image). Following TMVR with LAMPOON, the main impediment to forward flow was observed to be the transcatheter valve skirt which still projected into the native LVOT.
Effect of LAMPOON on Simulated Left Ventricular Pressure Fields
Simulations of left ventricular pressure fields are depicted by color maps (red = highest pressure, blue = lowest pressure) for a representative subject (Subject 5) (Figure 3). For the condition of the virtual control procedure (TMVR without LAMPOON), there was a substantial pressure drop which occurred at the location of the neo-LVOT, corresponding to the location of geometric narrowing. For the condition of the performed interventional procedure (TMVR with LAMPOON), the simulated pressure drop was reduced at the neo-LVOT due to the increase in flow area from the splayed anterior leaflet. In this subject, the splayed anterior leaflet from LAMPOON exposed three cells of the transcatheter valve, corresponding to a total increase in flow area of 87 mm2.
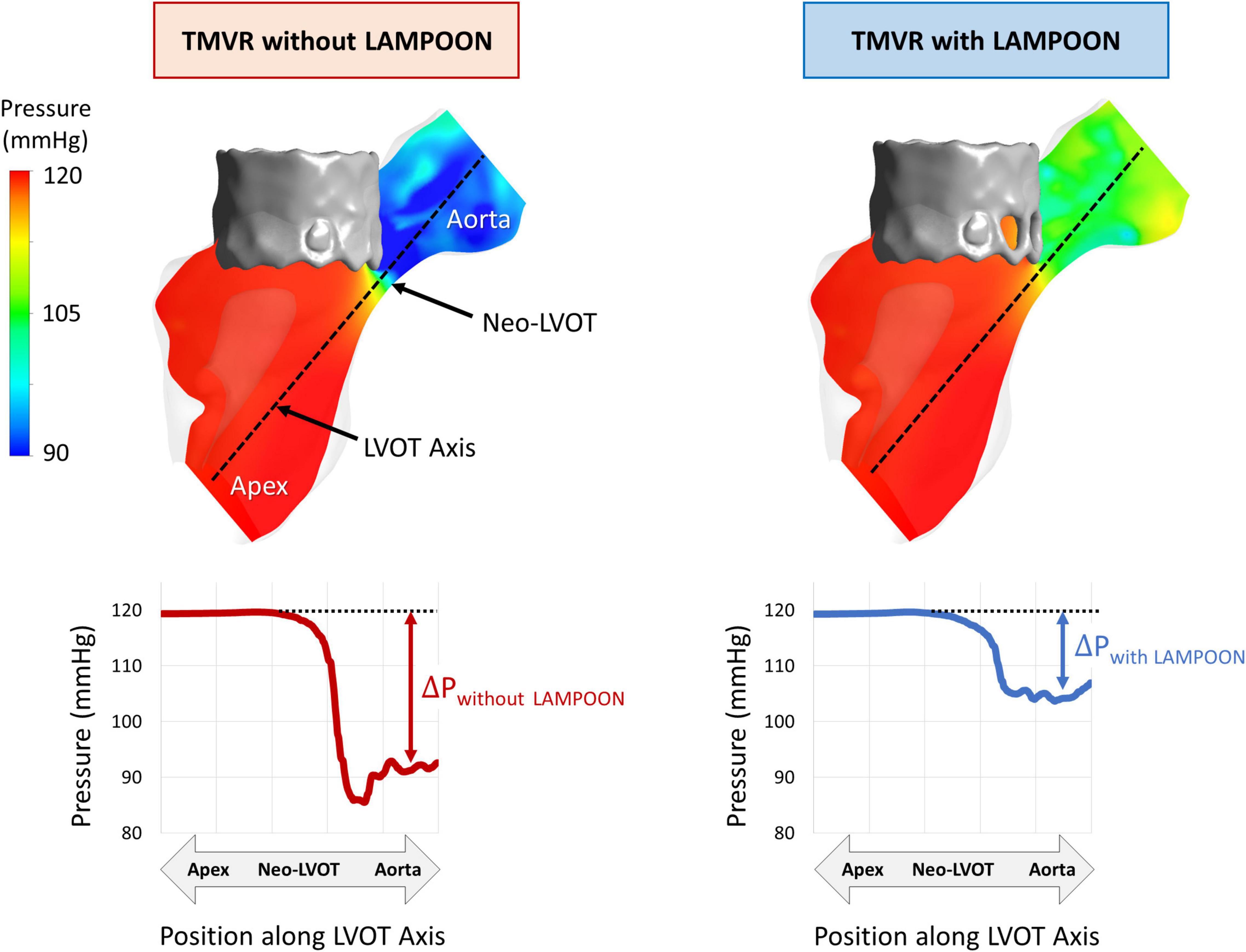
Figure 3. Effect of LAMPOON on left ventricular pressure fields. Simulated pressure fields for a representative subject (Subject 5) are shown in color maps (red = highest pressure, blue = lowest pressure). The left column shows the simulated pressure drop at the LVOT for the virtual control procedure (TMVR without LAMPOON). The right column shows the simulated pressure drop at the LVOT for the actual performed procedure (TMVR with LAMPOON). The simulated LVOT gradient is substantially reduced following LAMPOON. In this subject, LAMPOON exposed three cells of the transcatheter valve, corresponding to a total increase in flow area of 87 mm2.
Comparison of Simulated Hemodynamics
As compared to TMVR without LAMPOON, TMVR with LAMPOON resulted in lower Vpeak, lower ΔPpeak, and higher EOApeak by 14 ±7% (p = 0.006), 31 ±17% (p = 0.01), and 17 ±9% (p = 0.002), respectively (Figure 4). Following LAMPOON, Vpeak decreased from 2.6 ±1.1 m/s to 2.2 ±0.9 m/s, ΔPpeak decreased from 17.5 ±19.6 mmHg to 10.0 ± 8.8 mmHg, and EOApeak increased from 1.6 ±0.6 cm2 to 1.8 ±0.7 cm2 (p < 0.05 for all comparisons). Subject 7 had a substantially larger simulated LVOT gradient than the other subjects and could be considered an outlier (Figure 4A). However, when we excluded this subject from the analysis, we observed the same significant trend for the effect of LAMPOON on simulated LVOT gradient (p = 0.02).
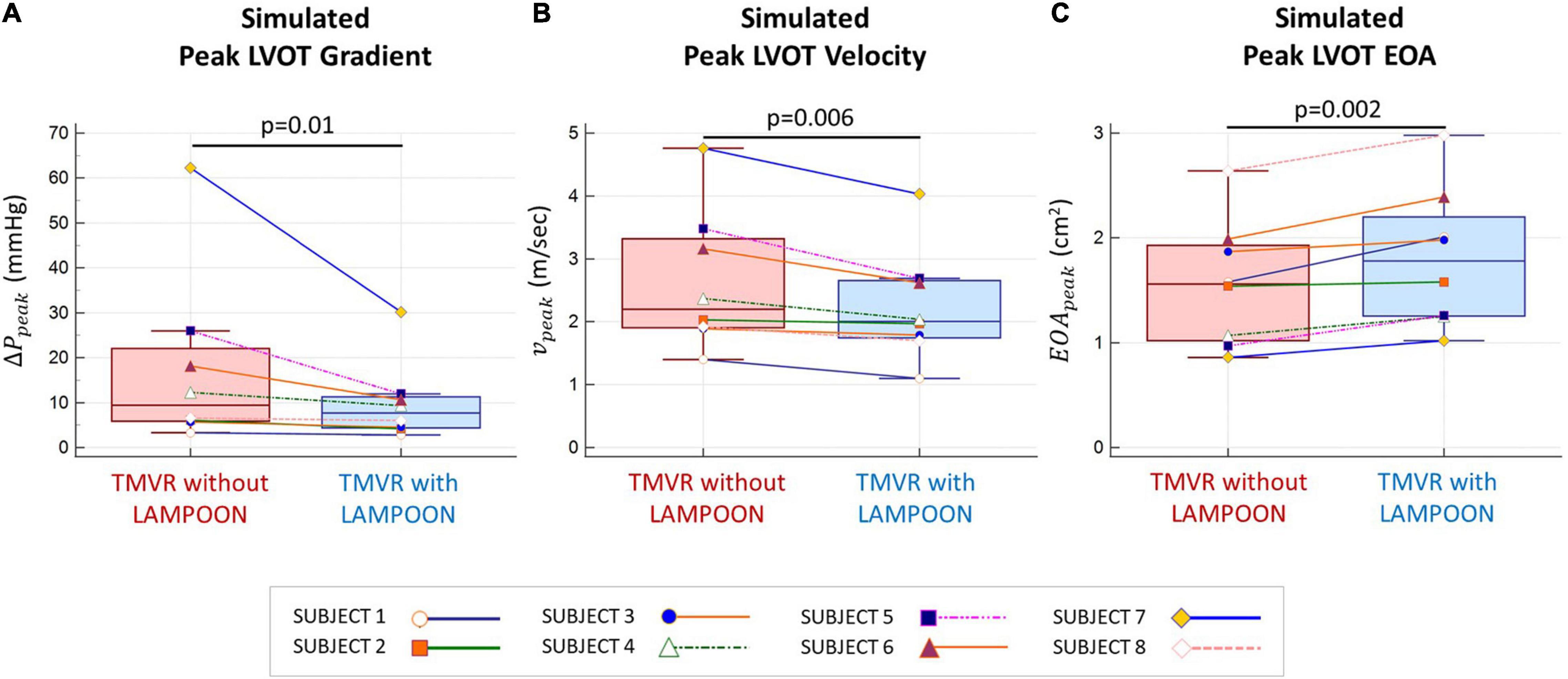
Figure 4. Comparison of simulated hemodynamics following TMVR with and without LAMPOON. Simulated hemodynamics following TMVR with LAMPOON were superior to those following TMVR without LAMPOON for all subjects. Following LAMPOON, the simulated peak outflow gradient (ΔPpeak) and velocity (vpeak) decreased in all subjects (p < 0.05 for both comparisons) (A,B). Additionally, the simulated peak effective orifice area (EOApeak) increased following LAMPOON in all subjects (p < 0.05) (C). LVOT, left ventricular outflow tract.
Relationship Between Neo-Left Ventricular Outflow Tract Area and LAMPOON Efficacy
LAMPOON was most effective in subjects who had a small post-procedure neo-LVOT area, as measured on post-procedure CT (Figure 5). The largest hemodynamic improvement with LAMPOON was observed in Subject 7 who had the smallest post-procedure neo-LVOT area across all subjects (49 mm2). This subject experienced a 32 mmHg reduction in the simulated LVOT gradient following LAMPOON. 3D flow patterns in this subject were also shown to substantially improve following LAMPOON, as evidenced by more centrally directed flow along the LVOT (Supplementary Video 1).
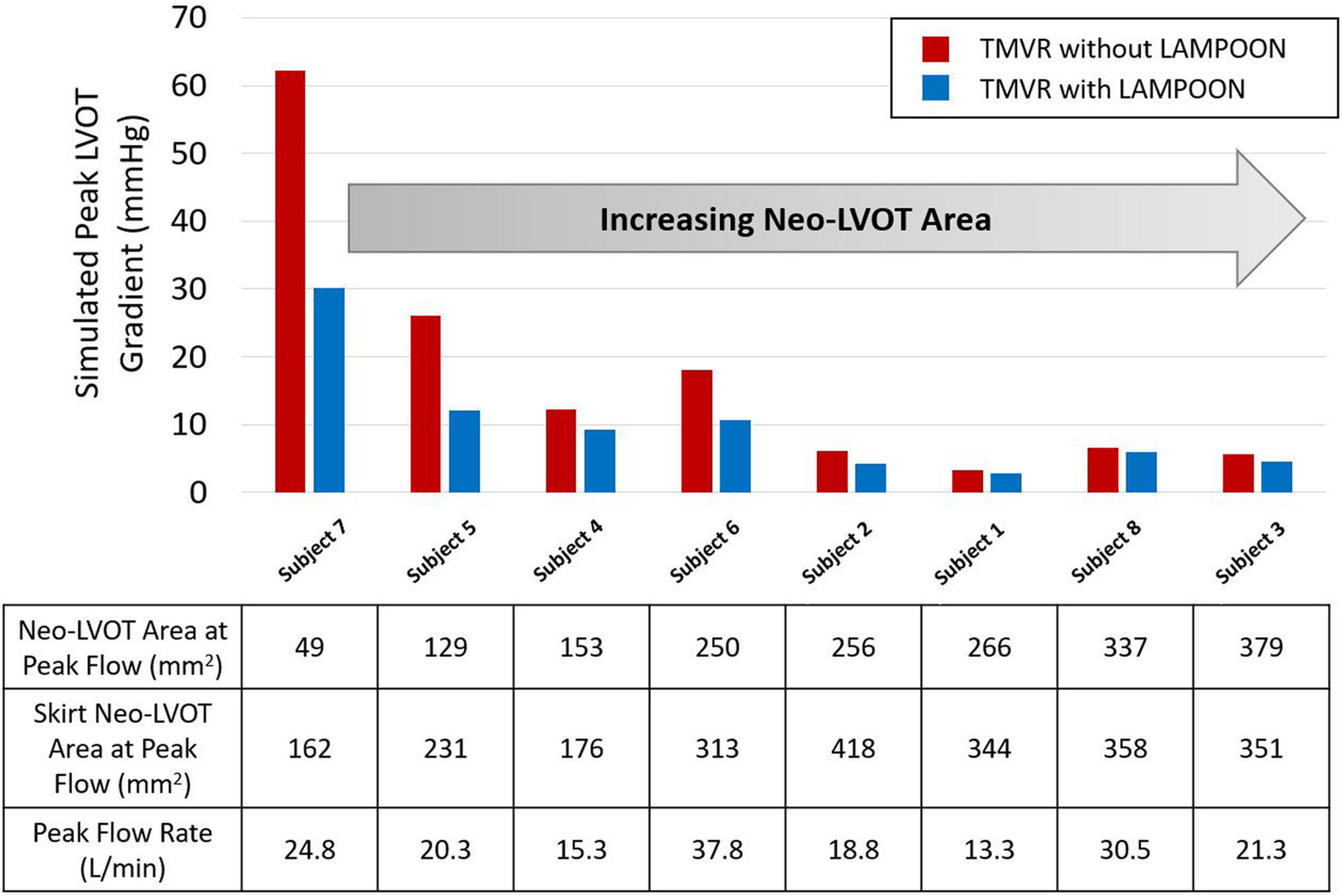
Figure 5. Relationship between post-procedure neo-LVOT area and hemodynamic improvement with LAMPOON. Subjects with a smaller post-procedure neo-LVOT area experienced a greater hemodynamic improvement with LAMPOON. The largest improvement in simulated LVOT gradient (32 mmHg reduction in gradient) was observed in the subject with the smallest post-procedure neo-LVOT area (49 mm2). As neo-LVOT area increased beyond 250 mm2, there was a smaller hemodynamic improvement with LAMPOON (<2 mmHg reduction in gradient). Neo-LVOT area = cross-sectional area at the narrowest point of the neo-left ventricular outflow tract. Skirt neo-LVOT area = cross-sectional area of the neo-left ventricular outflow tract measured at the level of the transcatheter valve skirt.
As the post-procedure neo-LVOT area increased beyond 250 mm2, LAMPOON began to demonstrate a smaller hemodynamic effect. For all subjects with a post-procedure neo-LVOT area greater than 250 mm2, the simulated LVOT gradient reduced by less than 2 mmHg following LAMPOON. This finding is also illustrated in Figure 6 which shows simulations of flow patterns in two representative subjects with different neo-LVOT dimensions.
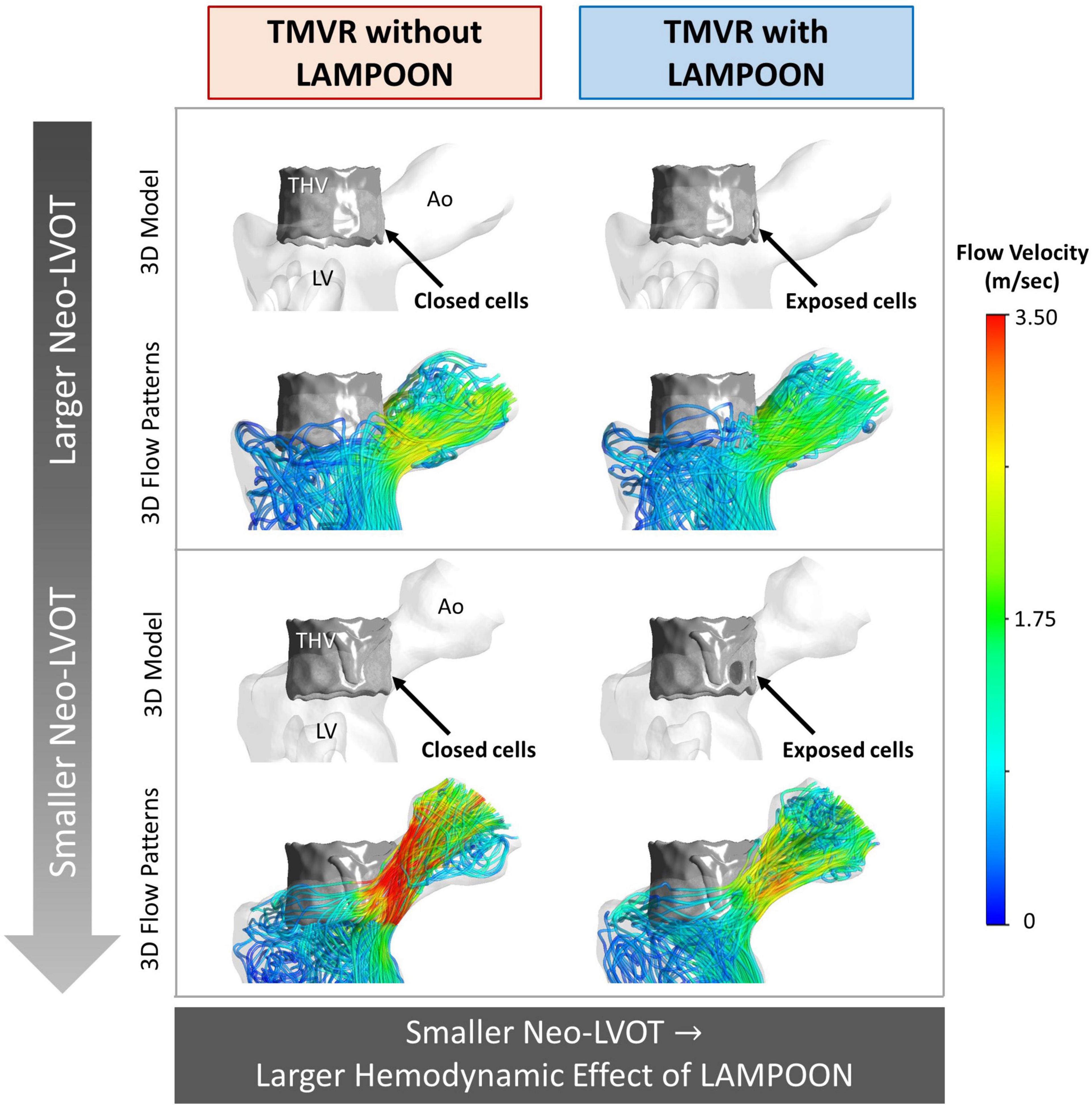
Figure 6. Flow simulations demonstrating the relationship between neo-LVOT size and LAMPOON efficacy. Two representative subjects are shown, where the subject shown in the top panel (Subject 6) has a larger neo-LVOT area as compared to the subject shown in the bottom panel (Subject 7). 3D models and simulated flow patterns are shown for the conditions of TMVR with and without LAMPOON. As neo-LVOT area decreases, the hemodynamic effect of LAMPOON increases. The total area of the transcatheter valve cells exposed by the splayed anterior leaflet were identical in both subjects (88 mm2). Ao, aorta; LV, left ventricle; neo-LVOT, neo-left ventricular outflow tract; THV, transcatheter heart valve.
Discussion
This study evaluated the hemodynamic impact of LAMPOON in TMVR using patient-specific in silico models. The main finding was that LAMPOON improved outflow hemodynamics in all subjects, with a larger hemodynamic effect in the setting of a smaller post-procedure neo-LVOT area. Because of the impossibility of performing a controlled clinical trial of TMVR with vs. without LAMPOON, the present in silico study is the first to provide quantitative insight into the hemodynamic effect of LAMPOON.
Left ventricular outflow tract obstruction is a significant problem for the broader adoption of TMVR as a therapy for patients with mitral valve disease who are not otherwise surgical candidates. The risk of LVOT obstruction is a primary reason for screen failure in TMVR device trials (10). For patients at prohibitive risk of obstruction, LAMPOON is a potential option to mitigate the risk of obstruction. To date, all clinical studies of LAMPOON to have been single-arm studies to assess clinical safety and feasibility of the technique (4, 11, 12).
The hemodynamic impact of LAMPOON in TMVR has not previously been investigated since patients who are considered for LAMPOON are anticipated to be at prohibitive risk of LVOT obstruction and therefore are not considered anatomical candidates for the control procedure (TMVR without LAMPOON) which would be needed to compare hemodynamic outcomes. The only way to quantitatively assess the hemodynamic impact of the LAMPOON procedure would be to perform a controlled in vivo (animal), in vitro (bench), or in silico (computational) study. In vivo studies are not always feasible due to procedural and anatomical limitations of the animal model. In vitro studies are more feasible but are also often very tedious to perform and can be costly. We chose to perform a controlled in silico study, which allowed us to use each subject as their own virtual comparator.
In silico modeling revealed that LAMPOON leads to a consistent improvement in hemodynamics following TMVR. Mechanistically, splaying the anterior mitral leaflet allows additional flow into the LVOT which decreases LVOT velocity and pressure gradient, while increasing the effective orifice area. The average number of transcatheter valve cells exposed by LAMPOON was 2 ±0.6 cells which corresponded to a total increase in outflow area of 70 ±20 mm2. On average, the area of a single exposed cell was 36 ±7 mm2. This small increment in outflow area led to a critical improvement in simulated hemodynamics, particularly for subjects with a smaller post-procedure neo-LVOT area (Figure 5). In this study, TMVR without LAMPOON was modeled by artificially closing the exposed transcatheter valve cells to mimic an intact anterior leaflet. However, in the clinical setting, the anterior leaflet may not only drape the cells but also hang lower beyond the valve frame. Thus, the actual improvement in hemodynamics with LAMPOON may be even more dramatic than what was calculated in this study.
Flow simulations in this study also validated the importance of the skirt neo-LVOT concept when predicting the risk of LVOT obstruction following TMVR with LAMPOON. The skirt neo-LVOT area is measured at the level of the transcatheter valve skirt, and represents the main impediment to forward flow following TMVR with anterior leaflet resection or LAMPOON (8). In the subject with the smallest post-procedure neo-LVOT area (Subject 7), there was the largest hemodynamic improvement with LAMPOON (>30 mmHg decrease in simulated LVOT gradient). However, despite this improvement, this subject still had a 30 mmHg simulated LVOT gradient post-LAMPOON due to a small skirt neo-LVOT area (162 mm2). While initial clinical data suggested a skirt neo-LVOT area of <150 mm2 should be avoided to prevent significant LVOT obstruction (catheterization gradient >30 mmHg), a more recent study from our group has shown that a skirt neo-LVOT area >180 mm2 is ideal to ensure survival with measurable clinical benefit (e.g., improvement in Kansas City Cardiomyopathy Questionnaire Score) (9). Thus, flow simulations in this study corroborate the clinical observation that LVOT obstruction may still occur following LAMPOON in the case of a small skirt neo-LVOT, and confirm that the skirt neo-LVOT should be routinely and carefully evaluated prior to performing TMVR with LAMPOON.
This study also highlighted that there can be differences between the anticipated risk of LVOT obstruction and the actual risk of LVOT obstruction in patients undergoing TMVR. All subjects in this study were initially thought to be at prohibitive risk for obstruction based on a predicted end-systolic neo-LVOT area of <200 mm2 measured on baseline CT. However, the neo-LVOT area measured on post-procedure CT was found to be substantially larger (>100 mm2) than the predicted neo-LVOT area at baseline in four subjects (Subjects 1, 2, 3, and 8) (Table 1), indicating that the actual risk of obstruction was substantially lower than the anticipated risk of obstruction. These four subjects experienced the least simulated hemodynamic benefit with LAMPOON. These data suggests that, in patients undergoing a valve-in-ring or valve-in-mitral annular calcification procedure, the anticipated risk of obstruction may in fact differ from the actual risk based on factors such as valve deployment depth (more atrial vs. more ventricular) or the valve deployment orientation (coaxial vs. canted with respect to the mitral valve). Personalized computational modeling may eventually help in better predicting the hemodynamic outcome of patients (13), and should account for a range of valve deployment scenarios. Recent interventional techniques have also enabled more predictable deployment of the transcatheter valve in the predicted landing zone, and may help improve future predictions of LVOT obstruction risk (14, 15).
In silico studies have the potential to provide key insights into the performance of novel interventional procedures such as LAMPOON which may be impossible to evaluate in a controlled clinical trial. The advantages of in silico studies are to (1) avoid the costs associated with conventional clinical trials, (2) avoid risk to patients, and (3) study unique metrics which may be difficult or impossible to attain in a clinical setting. Within the realm of structural heart disease interventions, prior in silico studies have evaluated para-valvular regurgitation in transcatheter aortic valve replacement (16, 17), device size selection for left atrial appendage occlusion (18), and LVOT hemodynamics in the setting of TMVR (7, 19, 20). In the future, in silico trials may also be used to supplement conventional clinical trial evidence and support the regulatory process of new devices and procedures (21). With appropriate validation, in silico data can represent a potentially valuable form of evidence which can be obtained at a reduced cost and without risk to patients (22).
Limitations
This was a retrospective study with a small sample size. As adoption of the LAMPOON technique expands, larger datasets will be needed to corroborate the findings of this study. The modeling of blood flow in this study using CFD may be limited given the assumptions of fixed left ventricular walls with zero wall velocity during peak systole, and steady flow boundary conditions. Since the pressure gradient across a normal aortic valve should be negligible relative to the gradient across an obstructed LVOT, we did not model an aortic valve in our simulations for simplicity. The anterior mitral leaflet was modeled depending on whether the leaflet could be reliably segmented on the post-procedure CT dataset. The posterior mitral leaflet was not modeled since this leaflet is not affected by the LAMPOON procedure and should not impact flow in the LVOT. The value of our CFD workflow is to provide a simplified, rapid approach to simulate LVOT hemodynamics. This method has been previously validated and has demonstrated good accuracy (<10% error) when compared to clinical hemodynamic measurements (7). Despite the discussed limitations, this study provides unique insight into the hemodynamic effect of LAMPOON which would have been impossible to study in a clinical setting.
Conclusion
A controlled in silico study was performed to investigate the hemodynamic impact of LAMPOON in TMVR. LAMPOON achieved a critical increment in outflow area which resulted in a consistent improvement in hemodynamic outcomes and 3D flow patterns following TMVR. Patients with a smaller post-procedure neo-LVOT area experienced a greater hemodynamic improvement with LAMPOON. This study demonstrates the potential for in silico studies to evaluate procedures virtually which may improve cost-effectiveness and patient safety as compared to a conventional clinical trial.
Data Availability Statement
Data can be made available upon reasonable request to NHLBI.
Ethics Statement
All subject data were acquired and analyzed under Institutional Review Board approvals at the National Institutes of Health (NCT03015194). The patients/participants provided their written informed consent to participate in this study.
Author Contributions
KK, ZW, VS, RL, VB, AY, and JO: conception and design of the work. EP, AG, JK, RL, and VB: acquisition and compilation of clinical data. KK and ZW: development of methods for computer simulations. KK, ZW, AS, PB, and EP: initial drafting of manuscript. All authors analysis and interpretation of data, critical review of manuscript for important intellectual content, and final approval of manuscript.
Funding
This study was supported by American Heart Association Pre-doctoral Fellowship 20PRE35200392 (Kohli) and National Institutes of Health (NIH) grant R01EB027774 (Oshinski).
Conflict of Interest
KK has served as a consultant for Abbott Vascular. PB has served as a consultant for Edwards Lifesciences, Tendyne, Neovasc, and Circle Imaging. AG has served as a proctor for Edwards Lifesciences, Medtronic, and Abbott Vascular; and has served as a consultant for and is an equity holder in Transmural Systems. VB has served as a consultant for Edwards Lifesciences and Abbott Vascular; and has served as a consultant for and is an equity holder in Transmural Systems. JK and RL were co-inventors on patents, assigned to the NIH, on devices for leaflet laceration.
The remaining authors declare that the research was conducted in the absence of any commercial or financial relationships that could be construed as a potential conflict of interest.
Publisher’s Note
All claims expressed in this article are solely those of the authors and do not necessarily represent those of their affiliated organizations, or those of the publisher, the editors and the reviewers. Any product that may be evaluated in this article, or claim that may be made by its manufacturer, is not guaranteed or endorsed by the publisher.
Acknowledgments
We would like to acknowledge helpful technical discussions with the members of the Cardiovascular Fluid Mechanics (CFM) Laboratory at Georgia Tech. We gratefully acknowledge Daniel A. Herzka, Ph.D. for de-identifying and compiling the entire cohort of subject image data utilized in this study. We also acknowledge the use of ANSYS software which was provided through an Academic Partnership between ANSYS, Inc. and the CFM Lab. Finally, we acknowledge Vivian Truong for her assistance with cardiac image post-processing.
Supplementary Material
The Supplementary Material for this article can be found online at: https://www.frontiersin.org/articles/10.3389/fcvm.2022.869259/full#supplementary-material
Abbreviations
CFD, computational fluid dynamics; CT, computed tomography; LAMPOON, intentional laceration of the anterior mitral leaflet to prevent left ventricular outflow tract obstruction; LVOT, left ventricular outflow tract; TMVR, transcatheter mitral valve replacement.
References
1. Yoon SH, Whisenant BK, Bleiziffer S, Delgado V, Dhoble A, Schofer N, et al. Outcomes of transcatheter mitral valve replacement for degenerated bioprostheses, failed annuloplasty rings, and mitral annular calcification. Eur Heart J. (2018). 40, 441–451. doi: 10.1093/eurheartj/ehy590
2. Babaliaros VC, Greenbaum AB, Khan JM, Rogers T, Wang DD, Eng MH, et al. Intentional percutaneous laceration of the anterior mitral leaflet to prevent outflow obstruction during transcatheter mitral valve replacement: first-in-human experience. JACC Cardiovasc Interv. (2017) 10:798–809. doi: 10.1016/j.jcin.2017.01.035
3. Babaliaros VC, Lederman RJ, Gleason PT, Khan JM, Kohli K, Sahu A, et al. The art of SAPIEN 3 transcatheter mitral valve replacement in valve-in-ring and valve-in-mitral-annular-calcification procedures. JACC Cardiovasc Interv. (2021) 14:2195–214. doi: 10.1016/j.jcin.2021.08.044
4. Khan JM, Babaliaros VC, Greenbaum AB, Foerst JR, Yazdani S, McCabe JM, et al. Anterior leaflet laceration to prevent ventricular outflow tract obstruction during transcatheter mitral valve replacement. J Am Coll Cardiol. (2019) 73:2521–34. doi: 10.1016/j.jacc.2019.02.076
5. Case BC, Lisko JC, Babaliaros VC, Greenbaum AB, Satler L, Ben-Dor I, et al. Lampoon techniques to prevent or manage left ventricular outflow tract obstruction in transcatheter mitral valve replacement. Ann Cardiothorac Surg. (2021) 10:172–9. doi: 10.21037/ACS-2020-MV-25
6. Kohli K, Wei ZA, Yoganathan AP, Oshinski JN, Leipsic J, Blanke P. Transcatheter mitral valve planning and the Neo-LVOT: utilization of virtual simulation models and 3D printing. Curr Treat Options Cardiovasc Med. (2018) 20:99. doi: 10.1007/s11936-018-0694-z
7. Kohli K, Wei ZA, Sadri V, Netto T, Lisko JC, Greenbaum AB, et al. A simplified in silico model of left ventricular outflow in patients after transcatheter mitral valve replacement with anterior leaflet laceration. Ann Biomed Eng. (2021) 49:1449–61. doi: 10.1007/s10439-021-02740-z
8. Khan JM, Rogers T, Babaliaros VC, Fusari M, Greenbaum AB, Lederman RJ. Predicting left ventricular outflow tract obstruction despite anterior mitral leaflet resection: the “Skirt NeoLVOT.”. JACC Cardiovasc Imaging. (2018) 11:1356–9. doi: 10.1016/j.jcmg.2018.04.005
9. Kohli K, Wei ZA, Sadri V, Khan JM, Lisko JC, Netto T, et al. Dynamic nature of the LVOT following transcatheter mitral valve replacement with LAMPOON: new insights from post-procedure imaging. Eur Heart J Cardiovasc Imaging. (2022) 23:650–62. doi: 10.1093/ehjci/jeab074
10. Coisne A, Pontana F, Tchétché D, Richardson M, Longère B, Vahdat O, et al. Transcatheter mitral valve replacement: factors associated with screening success and failure. Eurointervention. (2019) 15:e983–9. doi: 10.4244/eij-d-19-00444
11. Kasel AM, Rumpf M, Frangieh AH, Mayr P, Joner M, Xhepa E, et al. International LAMPOON: first european experience with laceration of the anterior mitral valve leaflet prior to transseptal transcatheter mitral valve implantation. Eurointerv J. (2018) 14:746–9. doi: 10.4244/eij-d-18-00201
12. Lisko JC, Greenbaum AB, Khan JM, Kamioka N, Gleason PT, Byku I, et al. Antegrade intentional laceration of the anterior mitral leaflet to prevent left ventricular outflow. Circ Cardiovasc Interv. (2020) 13:e008903. doi: 10.1161/CIRCINTERVENTIONS.119.008903
13. Kohli K, Wei A, Sadri V, Pierce E, Zhang Y, Wang D, et al. Framework for planning TMVR using 3-D imaging, in silico modeling, and virtual reality. Struct Heart J Heart Team. (2020) 4:336–41. doi: 10.1080/24748706.2020.1762268
14. Greenbaum AB, Lisko JC, Gleason PT, Kamioka N, Metcalf DP, Greenbaum MA, et al. Annular-to-apical “emory angle” to ensure coaxial mitral implantation of the SAPIEN 3 valve. JACC Cardiovasc Interv. (2020) 13:2447–50. doi: 10.1016/j.jcin.2020.07.024
15. Greenbaum AB, Perdoncin E, Paone G, Grubb KJ, Xie JX, Gleason PT, et al. Tableside skirt modification of the SAPIEN 3 valve to reduce paravalvular leak during transcatheter mitral valve replacement. JACC Cardiovasc Interv. (2021) 14:932–4. doi: 10.1016/j.jcin.2021.01.016
16. Mao W, Wang Q, Kodali S, Sun W. Numerical parametric study of paravalvular leak following a transcatheter aortic valve deployment into a patient-specific aortic root. J Biomech Eng. (2018) 140:1–11. doi: 10.1115/1.4040457
17. Halim J, Brouwer J, Lycke M, Swaans MJ, Van der Heyden J. Transcatheter aortic valve replacement: impact of pre-procedural FEops HEARTguide assessment on device size selection in borderline annulus size cases. Neth Heart J. (2021) 29:654–61. doi: 10.1007/s12471-021-01620-4
18. Buysschaert I, Viaene D. Clinical impact of preprocedural CT-based 3D computational simulation of left atrial appendage occlusion with amulet. J Interv Cardiol. (2021) 2021:9972228. doi: 10.1155/2021/9972228
19. de Vecchi A, Marlevi D, Nordsletten DA, Ntalas I, Leipsic J, Bapat V, et al. Left ventricular outflow obstruction predicts increase in systolic pressure gradients and blood residence time after transcatheter mitral valve replacement. Sci Rep. (2018) 8:15540. doi: 10.1038/s41598-018-33836-7
20. Alharbi Y, Otton J, Muller DWM, Geelan-Small P, Lovell NH, Al Abed A, et al. Predicting the outcome of transcatheter mitral valve implantation using image-based computational models. J Cardiovasc Comput Tomogr. (2019). 14, 335–342. doi: 10.1016/j.jcct.2019.11.016
21. Pappalardo F, Russo G, Tshinanu FM, Viceconti M. In silico clinical trials: concepts and early adoptions. Brief Bioinformatics. (2019) 20:1699–708. doi: 10.1093/bib/bby043
Keywords: transcatheter mitral valve replacement (TMVR), LAMPOON, left ventricular outflow tract obstruction, neo-LVOT, computational fluid dynamics (CFD), computed tomography (CT), personalized computational modeling
Citation: Kohli K, Wei ZA, Sadri V, Siefert AW, Blanke P, Perdoncin E, Greenbaum AB, Khan JM, Lederman RJ, Babaliaros VC, Yoganathan AP and Oshinski JN (2022) Assessing the Hemodynamic Impact of Anterior Leaflet Laceration in Transcatheter Mitral Valve Replacement: An in silico Study. Front. Cardiovasc. Med. 9:869259. doi: 10.3389/fcvm.2022.869259
Received: 04 February 2022; Accepted: 22 April 2022;
Published: 09 June 2022.
Edited by:
Amirhossein Arzani, Northern Arizona University, United StatesReviewed by:
Zahra K. Motamed, McMaster University, CanadaWenbin Mao, University of South Florida, United States
Copyright © 2022 Kohli, Wei, Sadri, Siefert, Blanke, Perdoncin, Greenbaum, Khan, Lederman, Babaliaros, Yoganathan and Oshinski. This is an open-access article distributed under the terms of the Creative Commons Attribution License (CC BY). The use, distribution or reproduction in other forums is permitted, provided the original author(s) and the copyright owner(s) are credited and that the original publication in this journal is cited, in accordance with accepted academic practice. No use, distribution or reproduction is permitted which does not comply with these terms.
*Correspondence: Keshav Kohli, keshavkohli@gmail.com