- 1Barbra Streisand Women's Heart Center, Cedars-Sinai Smidt Heart Institute, Cedars-Sinai Medical Center, Los Angeles, CA, United States
- 2Cedars-Sinai Smidt Heart Institute, Cedars-Sinai Medical Center, Los Angeles, CA, United States
- 3Biomedical Imaging Research Institute, Cedars-Sinai Medical Center, Los Angeles, CA, United States
- 4Department of Imaging, Mark Taper Imaging Institute, Cedars-Sinai Medical Center, Los Angeles, CA, United States
- 5Division of Rheumatology and Department of Biomedical Sciences, Department of Medicine, Cedars-Sinai Medical Center, Los Angeles, CA, United States
Chest pain is a common symptom in patients with systemic lupus erythematosus, an autoimmune disease that is associated with increased cardiovascular morbidity and mortality. While chest pain mechanisms can be multifactorial and often attributed to non-coronary or non-cardiac cardiac etiologies, emerging evidence suggests that ischemia with no obstructive coronary arteries (INOCA) is a prevalent condition in patients with chest pain and no obstructive coronary artery disease. Coronary microvascular dysfunction is reported in approximately half of SLE patients with suspected INOCA. In this mini review, we highlight the cardiovascular risk assessment, mechanisms of INOCA, and diagnostic approach for patients with SLE and suspected CMD.
Introduction
Cardiovascular disease (CVD) is a significant contributor to mortality in patients with systemic lupus erythematosus (SLE), with cardiovascular mortality approximately 2.7-fold higher than the general population (1). Despite advancements in treatment for SLE that have improved prognosis, CVD remains a major comorbidity, manifesting as accelerated atherosclerosis and myocardial infarction. Because SLE patients are predominantly female, inadequate CVD risk assessment and underdiagnosis in SLE patients may contribute to CVD morbidity and mortality, which are worse overall for women compared to men with ischemic heart disease (2). Young women with SLE are particularly at risk (3), as those in the 35-to 44-year age group have a 50-fold increase in risk of myocardial infarction compared to age-matched reference women (4). Patients with SLE frequently report chest pain in the absence of obstructive coronary artery disease (CAD), and their chest pain is often attributed to a non-ischemic etiology (5). However, in the past decade, advances in the non-invasive diagnosis of ischemia and no obstructive CAD (INOCA) have determined that coronary microvascular dysfunction (CMD) is highly prevalent in patients with SLE. In this mini review, we highlight the cardiovascular risk assessment, mechanisms of INOCA, and diagnostic approach for patients with SLE and suspected CMD.
Cardiovascular Risk Assessment in SLE Patients
Traditional cardiovascular risk factors only partially explain the increased CVD risk in SLE patients (6). Thus, traditional cardiovascular risk scores underestimate CVD risk in SLE patients (7). In an analysis of 263 asymptomatic patients with SLE and no prior CVD, risks of non-fatal MI and CVD mortality were over 10-fold higher than what would be expected based on traditional risk factors alone, and presence of SLE increased CVD risk at least 4-fold compared with predictions based on Framingham Risk Score (FRS) models (8). A modified FRS for SLE in which each item is multiplied by 2 was found to be a more accurate predictor of CAD in patients with SLE (9), but this approach does not reflect SLE-specific factors that contribute to heterogeneity of CVD risk in SLE patients. In a cohort of 210 SLE patients without prior CVD or diabetes mellitus (93% female, mean age 45 ± 12 years), both generic risk scores and “SLE-adapted” CVD risk scores underestimated high CVD risk as defined by carotid and femoral atherosclerotic plaque presence (10). Compared to modified risk scores that use a multiplication factor for the presence of SLE (e.g., modified FRS), the QRESEARCH risk estimator version 3 (QRISK3) includes SLE as an independent CVD risk factor and improved prediction of subclinical atherosclerotic CVD (10, 11).
SLE-related factors (e.g., as age at diagnosis, cumulative disease duration, disease activity, and cumulative dose of prednisone or cyclophosphamide) have been found to correlate with coronary atherosclerosis, and elevated c-reactive protein level was associated with CVD events in SLE patients (12, 13). Derivation of a SLE-specific cardiovascular risk equation (SLE-CRE) that incorporates both traditional CVD risk factors and SLE-specific risk factors (SELENA-SLEDAI disease activity score, low C3, and lupus anticoagulant) may better estimate 10-year CVD risk among patients with SLE compared to existing FRS or Pooled Cohort Risk Equation risk scores (14). In a recent single-center analysis of 1,887 SLE patients (88% female, age 39 ± 15 years), SLE-CRE had the highest sensitivity (61%) but lowest specificity (64%) for predicting CVD events compared to QRISK3, FRS, and modified FRS; the authors recommended the modified FRS as the best performer with its simple scoring system (15). However, further studies in larger cohorts are needed to improve the precision of CVD risk assessment in SLE patients. The American College of Cardiology (ACC)/American Heart Association (AHA) prevention guideline recommends to consider the presence of chronic inflammatory or autoimmune disease as a risk-enhancing factor (16) but does not provide specific recommendations regarding risk calculators.
Mechanisms of Ischemia in Patients with no Obstructive CAD
Chest pain and discomfort are frequently reported by patients with SLE, with high prevalence of angina in comparison to the general population (5). SLE patients with chest pain often present a diagnostic dilemma as they are frequently dismissed when coronary angiography demonstrates non-obstructive CAD or normal epicardial coronary arteries, which is more common among women than men with chest pain (17). Given previous limitations in diagnostic technology, these symptoms are often attributed to psychosomatic pain, chest wall pain, pericarditis/myocarditis, esophageal pain, or myofascial pain (18). Failure to accurately diagnose INOCA has significant clinical implications. Despite the absence of obstructive epicardial CAD, women with persistent stable angina have an elevated risk for CVD events, including all-cause and CVD mortality and progression to obstructive CAD (19, 20). Mechanisms of INOCA are predominantly attributed to coronary microvascular dysfunction (CMD) and/or coronary vasospasm, one or both of which are diagnosed in up to 4 in 5 patients undergoing invasive evaluation for INOCA (21–25). CMD is defined as an attenuated coronary blood flow response or coronary flow reserve (CFR), increased microvascular resistance, microvascular vasospasm, impaired myocardial perfusion reserve, and/or myocardial ischemia in the absence of obstructive CAD (<50% epicardial stenosis or fractional flow reserve >0.80) (26). CMD has an estimated prevalence of approximately 50% in individuals with no obstructive CAD undergoing non-invasive stress tests (27, 28). Epicardial coronary artery spasm is defined as >90% constriction either spontaneously or in response to a provocative stimulus (29).
Endothelial dysfunction, smooth muscle cell dysfunction, and vascular remodeling are major pathogenetic mechanisms in CMD and vasospasm. Endothelial dysfunction results from a reduced production or action of endothelium-derived relaxing factors (nitric oxide, vasodilator prostaglandins, and endothelium-dependent hyperpolarization factors), leading to the inability of the small resistance vessels (prearterioles and arterioles) or the large conduit vessels (epicardial arteries) to vasodilate adequately in response to myocardial demand, therefore leading to ischemia (30). Endothelial dysfunction is caused by aging, hypertension, hyperlipidemia, diabetes, obesity, chronic inflammatory disease, and smoking, and it is a precursor to coronary atherosclerosis (31, 32). In addition, patients with CMD and/or vasospasm may have enhanced coronary vasoconstrictive reactivity related to inflammation, rho kinase-induced myosin light-chain phosphorylation, and increased production of vasoconstrictive mediators (e.g., endothelin-1, serotonin, catecholamines), contributing to smooth muscle cell hyper-reactivity (30). The autonomic nervous system, a key regulator of vascular tone, can also contribute to coronary vasomotor dysfunction, such as heightened sympathetic nervous system activity and increased vagal tone in patients with vasospastic angina, and may be implicated in SLE (33). Finally, vascular remodeling and capillary rarefaction may occur in patients with hypertensive heart disease, aortic stenosis, infiltrative heart diseases, or chronic kidney disease (34). These functional and structural alterations of the coronary microvascular and epicardial arterial system contribute to the imbalance of vasodilating and vasoconstricting responses to stress, resulting in a supply-demand mismatch in coronary blood flow and ischemia.
Microvascular involvement is known to affect multiple organ systems in SLE, for example, in the kidney (lupus nephritis), digestive system (intestinal vasculitis), pulmonary vasculature (pulmonary hypertension, pulmonary vasculitis) and skin (livedo reticularis, cutaneous vasculitis). Although cardiac microvascular involvement occurs in other autoimmune diseases (such as systemic sclerosis and rheumatoid arthritis), the possibility of CMD in SLE has not been well established (35–39). Brachial endothelial dysfunction is significantly impaired in young SLE patients (40). Recent studies have indicated that skin microvascular dysfunction is present in young SLE patients with and without CVD risk factors (41) and associated with elevated carotid intimal media thickness and aortic atherosclerosis (42). Given this propensity for SLE to affect the microvasculature and the endothelium, the cardiovascular involvement is an important consideration beyond the well-known risk for atherosclerotic CAD.
Inflammatory Mechanisms of SLE Coronary Vascular Dysfunction and Atherosclerosis
The chronic inflammatory state of SLE plays a crucial role in accelerating endothelial dysfunction, atherosclerosis, and autonomic dysfunction. Endothelial dysfunction and early atherosclerosis in SLE have been attributed to dysregulation of prothrombotic cell death, and inflammatory mediators (Figure 1) (43–50).
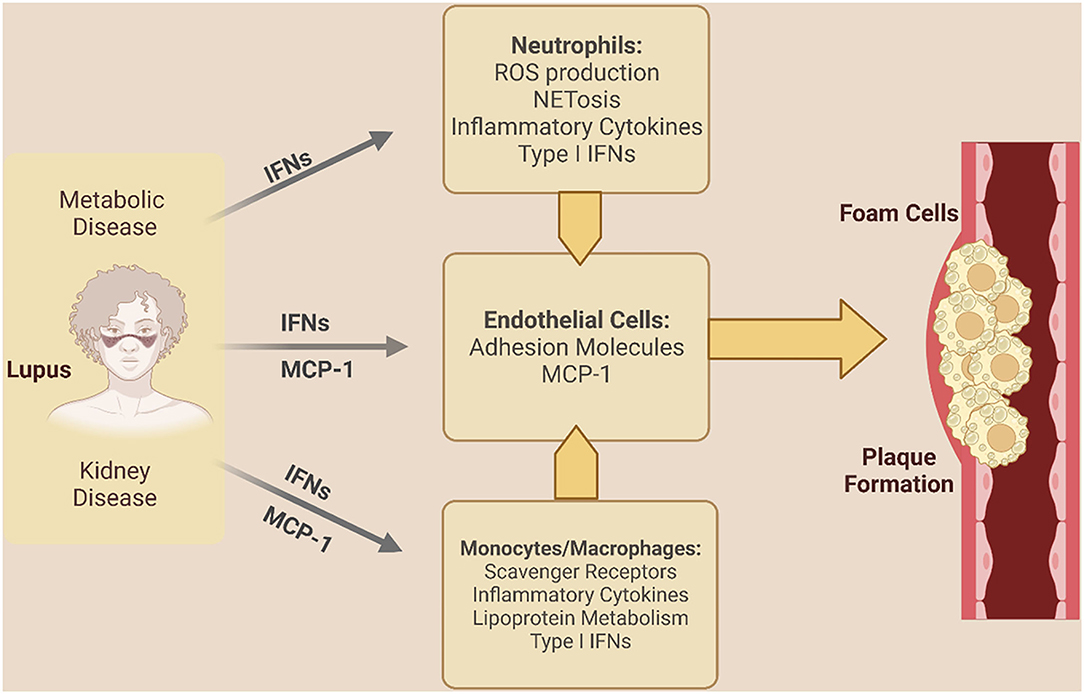
Figure 1. Inflammatory mechanisms driving coronary vascular dysfunction in SLE. Lupus-specific and non-lupus specific factors drive inflammation and coronary vascular dysfunction in SLE. IFNs indicate interferons; MCP-1, monocyte chemoattractant protein 1; NET, neutrophil extracellular trap; ROS, reactive oxygen species. [Created in Biorender. Adapted from (43)].
Monocyte/Macrophage Function
In SLE patients, type I interferons (IFNs) drive an increase in elevated inflammatory chemokines monocyte chemotactic protein-1 [MCP-1] and macrophage inflammatory protein 1α, triggering recruitment of monocytes into the subendothelial space and the upregulation of scavenger receptors such as CD36 (elevated in SLE monocytes) and scavenger receptor A (SR-A) (51–54). IFNs also promote formation of macrophage foam cells with the uptake of oxidized low-density lipoprotein (55–58). Indeed, a correlation between MCP-1 levels and carotid artery intima thickness is observed in SLE, underscoring its potential importance in promoting plaque development in SLE (59).
Neutrophil Function
Type I IFNs and other inflammatory mediators elevated in SLE also contribute to vascular damage by inducing endothelial dysfunction and reduced nitric oxide mediated vasodilation (60–63). Neutrophils in SLE are a potent source of type I IFNs–either releasing it directly themselves or triggering its production via the release of neutrophil extracellular traps or NETs (64–67). In SLE, NETs from inflammatory neutrophils termed low density neutrophils (LDNs) or granulocytes have been found to contain matrix metalloproteinases, resulting in damage to endothelial cells and drive the release of inflammatory cytokines and type I IFNs, promoting endothelial dysfunction (68–71). Levels of LDNs are associated with coronary plaque burden and endothelial dysfunction (72, 73), thus underscoring the role for these neutrophils in SLE-associated cardiovascular disease and potentially CMD.
Metabolic Dysregulation
Among SLE patients, renal dysfunction and higher corticosteroid doses are associated with metabolic syndrome, prevalence of which is enhanced in SLE patients and further contributes to increased CVD risk (74). Body mass index is significantly related to insulin resistance in SLE patients, independently of age, sex, race, and corticosteroid use (75). Chronic inflammation may be a potential contributor to enhanced risk of developing metabolic syndrome and insulin resistance in SLE patients, via TNFα release from adipocytes (76–78). In addition to secreting pro-inflammatory cytokines, white adipose tissue also secretes adipokines such as leptin and adiponectin, both of which are responsible for regulating energy homeostasis and metabolism. Leptin also drives inflammation and upregulates oxidative stress, not only in neutrophils and monocytes, but also in endothelial cells and cardiomyocytes (79–82). Indeed, leptin levels are increased in SLE, with evidence that the increase confers enhanced risk of atherosclerosis in these patients (83, 84).
Antiphospholipid Syndrome
Gene profiling has also revealed specific molecular pathways in the pathogenesis of atherosclerosis and CVD in SLE patients with and without antiphospholipid syndrome (APS). Antiphospholipid antibodies are known to trigger inflammatory cascades with increased expression of cytokines, chemokines, and mediators of endothelial dysfunction, as well as accelerate the influx of oxidized low-density lipoproteins into macrophages, promoting atherosclerosis development (85). From microarray expression profiling in monocytes of patients with SLE with and without APS, IgG-anticardiolipin titers were significantly related to inflammatory, endothelial dysfunction, and oxidative stress markers, as well as were independently predicted both atherosclerosis and thrombosis in SLE patients with APS (85).
Advanced Cardiac Imaging for Diagnosis of CMD in SLE Patients
Advanced cardiac imaging studies in the past decade have improved understanding of CMD prevalence in SLE patients. Stress transthoracic doppler echocardiography (TTDE), positron emission tomography (PET), and cardiac magnetic resonance imaging (CMR) are recommended by society guidelines for the diagnosis of CMD, with test choice guided by local availability and expertise (86, 87).
Transthoracic Doppler Echocardiography
TTDE is an established method of CMD evaluation, by measuring CFR in the left anterior descending coronary artery (88, 89). In a study including 21 SLE patients (mean age 60 ± 11 years) and 23 control subjects (mean age 65 ± 10 years) with comparable CVD risk factors, coronary artery calcium scores, and no obstructive CAD, the prevalence of CMD (defined as CFR < 2.5) was higher in the SLE group (67%) compared to the control group (26%), with an odds ratio of 16.7 for CMD in SLE patients after adjusting for age, body mass index, anemia, and hemoglobin level (89). TTDE CFR has also been demonstrated to be reduced in young SLE patients (n = 18, mean age 29 ± 6 years) compared to age-, sex- and race-matched healthy controls, supporting the hypothesis that coronary microvascular impairment occurs early in SLE patients (90).
Stress Cardiac Positron Emission Tomography
Stress cardiac PET utilizes radioactive tracers in patients at rest and with vasodilator stress to quantify absolute myocardial blood flow (MBF) and detect impaired myocardial flow reserve (MFR) suggestive of CMD (91). Cardiac PET MFR < 2.0 has been found to be prognostic in both women and men with INOCA and recommended as a diagnostic threshold for CMD (28). In a recent study of 42 middle-aged SLE patients (mean age 61.2 ± 0.5 years, 97% women) with no obstructive CAD who underwent cardiac PET for evaluation of suspected CAD, MFR < 2.0 consistent with CMD was seen in 57.1% of the patients, and global MFR was significantly reduced compared to matched controls (1.9 ± 0.5 vs. 2.4 ± 0.7, P < 0.0001) despite a similar degree of non-obstructive CAD burden and similar myocardial blood flow at rest (92). MFR was reduced in the presence or absence of chronic kidney disease, whether due to lupus nephritis or other causes. MFR was not associated with SLE disease duration nor presence of antiphospholipid antibodies. However, MFR was inversely related to SLE disease activity, consistent with a prior PET study of 13 SLE patients (93). Although prognostic utility of PET MFR has not been reported in SLE patients only, several studies have demonstrated that impaired PET MFR predicts adverse cardiovascular events and all-cause mortality in patients with autoimmune rheumatic diseases including SLE (37, 94, 95).
Stress Cardiac Magnetic Resonance Imaging
Stress CMR has emerged as a diagnostic and prognostic tool for the evaluation of CMD in patients with no obstructive CAD (96, 97), and may be preferred in SLE patients with concern for myocarditis or pericardial disease, given the standing of CMR as a primary modality of diagnosis for these disease processes (98). CMR measures of coronary blood flow include the semiquantitative myocardial perfusion reserve index (MPRI) <1.84 and quantitative MFR < 2.19, which are sensitive and specific for diagnosing CMD in women and men with angina and no obstructive CAD (99, 100). Furthermore, MPRI ≤ 1.47 independently predicts of major adverse cardiac events in patients with INOCA (101). A study of 20 young women with SLE and angina (mean age 40.6 years) found that visually-identified stress-induced circumferential subendocardial hypoperfusion consistent with global endocardial ischemia was more common in the SLE patients compared with age and sex-matched asymptomatic healthy controls (44 vs. 0%, P = 0.014) (102). MPRI trended lower in patients with SLE vs. controls (2.0 ± 0.4 vs. 2.3 ± 0.4, P = 0.16), despite absence of obstructive CAD with low burden of coronary atherosclerosis and low-to-moderate SLE disease activity in the SLE patients. MPRI did not correlate with SLE duration or SLE disease activity in this relatively healthy SLE cohort, but presence of SLE was found to be a predictor of subepicardial and subendocardial MPRI (102). In a 5-year follow-up study, a majority of the women reported persistent chest pain but only 25% had a decrease in their MPRI, which occurred in the absence of coronary atherosclerosis progression (103). All individuals with improved CMR findings were concomitantly on aspirin, beta-blocker therapy and cholesterol-lowering agents at follow-up, although clinical trials are needed to understand impact of disease modifying agents and optimal preventive therapy in SLE patients with CMD. Long-term studies to determine prognostic utility of CMR MPRI or MPR in SLE patients are needed.
Invasive Coronary Function Testing for Diagnosis of CMD and Vasospasm
Invasive testing allows for a more comprehensive delineation of CMD vs. vasospasm in patients with INOCA (17), although prevalence of coronary vasomotor dysfunction in SLE patients has not been reported in invasive coronary function studies. Since SLE patients not uncommonly have Raynaud's phenomenon, a vasospastic disorder of the fingers or toes, coronary vasospasm has been hypothesized to contribute to angina in SLE patients, although confirmatory studies are lacking. Invasive measures of CMD predict mortality and major adverse cardiac events, independent of cardiovascular risk factors (104). Acetylcholine provocation of epicardial vasospasm predicts myocardial infarction and repeated angiography (105). Furthermore, stratified medical therapy based on invasive diagnosis of CMD vs. epicardial vasospasm improved angina and quality of life in a randomized clinical trial (21). However, the diagnostic and prognostic utility of invasive coronary function testing for SLE patients is unknown.
Discussion
Chest pain is a frequent complaint of SLE patients in the absence of obstructive CAD, and ischemic mechanisms such as CMD and coronary vasospasm should be considered in the diagnostic algorithm. Given the higher risk of CVD mortality and morbidity attributed to SLE due to the inflammatory and metabolic pathophysiologic mechanisms, early identification and prevention of CVD risk factors is warranted, although available risk scores underestimate CVD risk in SLE patients. SLE patients presenting with chest pain should undergo clinical risk assessment and subsequent evaluation for CAD, with the consideration of CMD and/or coronary vasospasm evaluation in those with no obstructive CAD (Figure 2). Society guidelines recommend intensification of preventive and anti-ischemic therapies in patients with stable angina and suspected INOCA, particularly those with known coronary atherosclerosis, but evidence-based treatment specific to INOCA is lacking (86, 87).
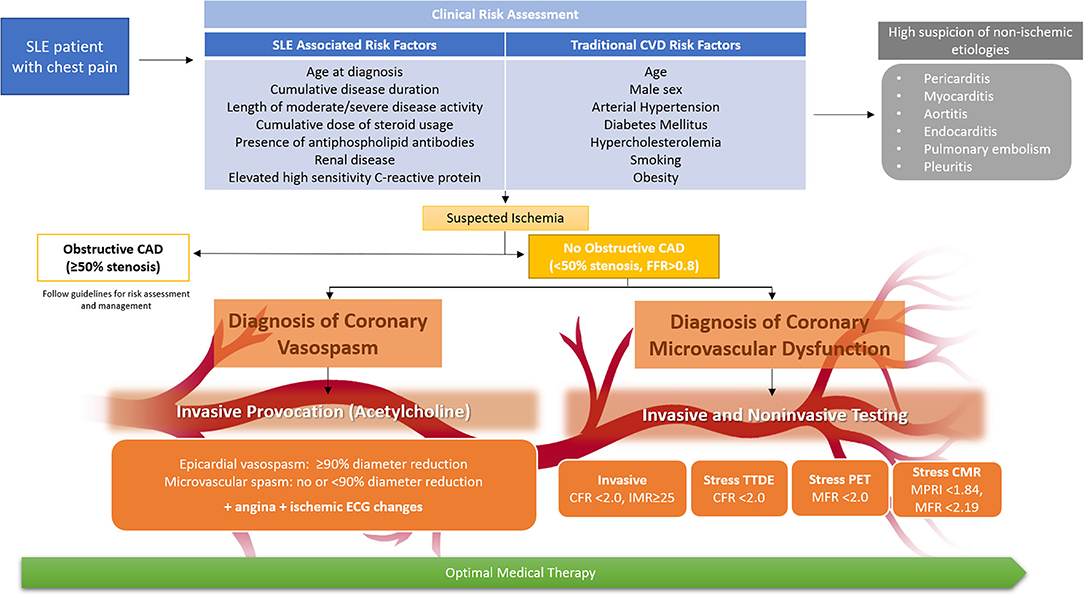
Figure 2. Clinical risk assessment of SLE patients with chest pain and algorithm for diagnosis of coronary microvascular dysfunction and vasospasm. Non-invasive and invasive testing allow assessment of coronary microvascular dysfunction, while invasive acetylcholine provocation testing can additionally assess coronary vasospasm. While CMR may be preferred for concomitant assessment of myocarditis, test choice should be guided by local expertise and availability. All SLE patients with angina should receive optimal medical therapy, including lifestyle intervention and intensive pharmacologic therapy (anti-ischemic and preventive therapy). CAD indicates coronary artery disease; CFR, coronary flow reserve; CMR, cardiac magnetic resonance imaging; CVD, cardiovascular disease; ECG, electrocardiogram; FFR, fractional flow reserve; IMR, index of microcirculatory restriction; MFR, myocardial flow reserve; MPRI, myocardial perfusion reserve index; PET, positron emission tomography; and TTDE, transthoracic doppler echocardiography.
CMD evaluation of SLE patients to date consistently demonstrates impaired flow or perfusion reserve compared to matched controls (Table 1). A relationship between CMD and SLE disease activity was reported in several of the studies, but overall no relationship between CMD and disease duration, risk factors, or steroid use, although larger cohorts may be needed to further evaluate predictors of CMD in SLE patients. Blood biomarkers of inflammation were not reported in the majority of studies. Several studies also reported higher resting velocities or myocardial flow in SLE patients compared to controls, suggesting underlying differences in coronary blood flow autoregulation, suspected due to vasomotor and autonomic dysfunction. Although studies comparing CMD in SLE vs. other autoimmune rheumatic diseases are limited, CMD has been reported in autoimmune rheumatic diseases such as rheumatoid arthritis and systemic sclerosis, strengthening the link between inflammation and CMD (36). While the pathogenic inflammatory mechanisms of various autoimmune rheumatic diseases are increasingly described, differences in the inflammatory mechanisms that contribute to CMD are not well understood.
Significant knowledge gaps exist in SLE patients with INOCA, including the prevalence of coronary vasospasm, contribution of autonomic dysfunction to ischemia and chest pain, role of disease-modifying antirheumatic drugs on CMD and cardiovascular outcomes, non-invasive and invasive strategies to identify high risk patients for targeted preventive therapy, and optimal therapy of microvascular and vasospastic angina. While inflammatory mechanisms of SLE are increasingly understood, mechanistic pathways underlying the pathobiology of SLE-specific coronary vasomotor dysfunction remain unknown. Future prospective research studies are needed to address these questions in the risk assessment. diagnosis and treatment of SLE patients with INOCA.
Author Contributions
All authors contributed with conceptualization, writing—review and editing, validation, and funding. All authors contributed to the article and approved the submitted version.
Funding
This work was supported by research funding from NIH R01HL146158 (CB and JW), R01HL124649 (CB), R01HL153500 (JW), U54AG065141 (CB), K23HL125941 (JW), R01AI164504 (CJ), the Office of the Assistant Secretary of Defense for Health Affairs through the Department of Defense Lupus Research Program Award Number W81XWH-18-1-0709 (CJ), the Barbra Streisand Women's Cardiovascular Research and Education Program (CB), the Linda Joy Pollin Women's Heart Health Program (CB), the Erika Glazer Women's Heart Health Project (CB), and the Adelson Family Foundation (CB).
Conflict of Interest
CB has served as consultant for Sanofi, Abbott Diagnostics, and iRhythm. DB has served as a consultant for Bayer and received software royalties from Cedars-Sinai Medical Center. JW has served as consultant and advisory board member for Abbott Vascular.
The remaining authors declare that the research was conducted in the absence of any commercial or financial relationships that could be construed as a potential conflict of interest.
Publisher's Note
All claims expressed in this article are solely those of the authors and do not necessarily represent those of their affiliated organizations, or those of the publisher, the editors and the reviewers. Any product that may be evaluated in this article, or claim that may be made by its manufacturer, is not guaranteed or endorsed by the publisher.
References
1. Yurkovich M, Vostretsova K, Chen W, Avina-Zubieta JA. Overall and cause-specific mortality in patients with systemic lupus erythematosus: a meta-analysis of observational studies. Arthritis Care Res. (2014) 66:608–16. doi: 10.1002/acr.22173
2. Aggarwal NR, Patel HN, Mehta LS, Sanghani RM, Lundberg GP, Lewis SJ, et al. Sex differences in ischemic heart disease: advances, obstacles, and next steps. Circ Cardiovasc Qual Outcomes. (2018) 11:e004437. doi: 10.1161/CIRCOUTCOMES.117.004437
3. Ward MM. Premature morbidity from cardiovascular and cerebrovascular diseases in women with systemic lupus erythematosus. Arthritis Rheum. (1999) 42(2):338–46. doi: 10.1002/1529-0131(199902)42:2<338::AID-ANR17>3.0.CO;2-U
4. Manzi S, Meilahn EN, Rairie JE, Conte CG, Medsger TA, Jansen-McWilliams L, et al. Age-specific incidence rates of myocardial infarction and angina in women with systemic lupus erythematosus: comparison with the framingham study. Am J Epidemiol. (1997) 145:408–15. doi: 10.1093/oxfordjournals.aje.a009122
5. Ishimori ML, Gal NJ, Rogatko A, Berman DS, Wilson A, Wallace DJ, et al. Prevalence of angina in patients with systemic lupus erythematosus. Arthritis Res Ther. (2012) 14:A62. doi: 10.1186/ar3996
6. Zeller CB, Appenzeller S. Cardiovascular disease in systemic lupus erythematosus: the role of traditional and lupus related risk factors. Curr Cardiol Rev. (2008) 4:116–22. doi: 10.2174/157340308784245775
7. Skamra C, Ramsey-Goldman R. Management of cardiovascular complications in systemic lupus erythematosus. Int J Clin Rheumtol. (2010) 5:75–100. doi: 10.2217/ijr.09.73
8. Esdaile JM, Abrahamowicz M, Grodzicky T, Li Y, Panaritis C, du Berger R, et al. Traditional framingham risk factors fail to fully account for accelerated atherosclerosis in systemic lupus erythematosus. Arthritis Rheum. (2001) 44:2331–7. doi: 10.1002/1529-0131(200110)44:10<2331::aid-art395>3.0.co;2-i
9. Urowitz MB, Ibanez D, Su J, Gladman DD. Modified framingham risk factor score for systemic lupus erythematosus. J Rheumatol. (2016) 43:875–9. doi: 10.3899/jrheum.150983
10. Drosos GC, Konstantonis G, Sfikakis PP, Tektonidou MG. Underperformance of clinical risk scores in identifying vascular ultrasound-based high cardiovascular risk in systemic lupus erythematosus. Eur J Prev Cardiol. (2020) 2047487320906650. doi: 10.1177/2047487320906650
11. Vazquez-Del Mercado M, Perez-Vazquez FJ, Gomez-Banuelos E, Chavarria-Avila E, Llamas-Garcia A, Arrona-Rios KI, et al. Subclinical parameters of arterial stiffness and arteriosclerosis correlate with Qrisk3 in systemic lupus erythematosus. PLoS ONE. (2018) 13:e0207520. doi: 10.1371/journal.pone.0207520
12. Romero-Diaz J, Vargas-Vorackova F, Kimura-Hayama E, Cortazar-Benitez LF, Gijon-Mitre R, Criales S, et al. Systemic lupus erythematosus risk factors for coronary artery calcifications. Rheumatology. (2012) 51:110–9. doi: 10.1093/rheumatology/ker307
13. Pons-Estel GJ, Gonzalez LA, Zhang J, Burgos PI, Reveille JD, Vila LM, et al. Predictors of cardiovascular damage in patients with systemic lupus erythematosus: data from lumina (Lxviii), a multiethnic Us cohort. Rheumatology. (2009) 48:817–22. doi: 10.1093/rheumatology/kep102
14. Petri MA, Barr E, Magder LS. Development of a systemic lupus erythematosus cardiovascular risk equation. Lupus Sci Med. (2019) 6:e000346. doi: 10.1136/lupus-2019-000346
15. Sivakumaran J, Harvey P, Omar A, Tayer-Shifman O, Urowitz MB, Gladman DD, et al. Assessment of cardiovascular risk tools as predictors of cardiovascular disease events in systemic lupus erythematosus. Lupus Sci Med. (2021) 8:e000448. doi: 10.1136/lupus-2020-000448
16. Arnett DK, Blumenthal RS, Albert MA, Buroker AB, Goldberger ZD, Hahn EJ, et al. 2019 Acc/Aha guideline on the primary prevention of cardiovascular disease: a report of the American college of cardiology/American heart association task force on clinical practice guidelines. J Am Coll Cardiol. (2019) 74:e177–232. doi: 10.1016/j.jacc.2019.03.010
17. Bairey Merz CN, Pepine CJ, Walsh MN, Fleg JL. Ischemia and no obstructive coronary artery disease (Inoca): developing evidence-based therapies and research agenda for the next decade. Circulation. (2017) 135:1075–92. doi: 10.1161/CIRCULATIONAHA.116.024534
18. Ishimori ML, Anderson L, Weisman MH, Mehta PK, Bairey Merz CN, Wallace DJ. Microvascular angina: an underappreciated cause of sle chest pain. J Rheumatol. (2013) 40:746–7. doi: 10.3899/jrheum.121277
19. Kenkre TS, Malhotra P, Johnson BD, Handberg EM, Thompson DV, Marroquin OC, et al. Ten-year mortality in the wise study (Women's Ischemia Syndrome Evaluation). Circ Cardiovasc Qual Outcomes. (2017) 10:e003863. doi: 10.1161/CIRCOUTCOMES.116.003863
20. Johnson BD, Shaw LJ, Pepine CJ, Reis SE, Kelsey SF, Sopko G, et al. Persistent chest pain predicts cardiovascular events in women without obstructive coronary artery disease: results from the Nih-Nhlbi-sponsored women's ischaemia syndrome evaluation (Wise) study. Eur Heart J. (2006) 27:1408–15. doi: 10.1093/eurheartj/ehl040
21. Ford TJ, Stanley B, Good R, Rocchiccioli P, McEntegart M, Watkins S, et al. Stratified medical therapy using invasive coronary function testing in angina: the cormica trial. J Am Coll Cardiol. (2018) 72:2841–55. doi: 10.1016/j.jacc.2018.09.006
22. Sara JD, Widmer RJ, Matsuzawa Y, Lennon RJ, Lerman LO, Lerman A. Prevalence of coronary microvascular dysfunction among patients with chest pain and nonobstructive coronary artery disease. JACC Cardiovasc Interv. (2015) 8:1445–53. doi: 10.1016/j.jcin.2015.06.017
23. Aziz A, Hansen HS, Sechtem U, Prescott E, Ong P. Sex-related differences in vasomotor function in patients with angina and unobstructed coronary arteries. J Am Coll Cardiol. (2017) 70:2349–58. doi: 10.1016/j.jacc.2017.09.016
24. Wei J, Mehta PK, Johnson BD, Samuels B, Kar S, Anderson RD, et al. Safety of coronary reactivity testing in women with no obstructive coronary artery disease: results from the nhlbi-sponsored wise (Women's Ischemia Syndrome Evaluation) study. JACC Cardiovasc Interv. (2012) 5:646–53. doi: 10.1016/j.jcin.2012.01.023
25. Lee BK, Lim HS, Fearon WF, Yong AS, Yamada R, Tanaka S, et al. Invasive evaluation of patients with angina in the absence of obstructive coronary artery disease. Circulation. (2015) 131:1054–60. doi: 10.1161/CIRCULATIONAHA.114.012636
26. Ong P, Camici PG, Beltrame JF, Crea F, Shimokawa H, Sechtem U, et al. International standardization of diagnostic criteria for microvascular angina. Int J Cardiol. (2018) 250:16–20. doi: 10.1016/j.ijcard.2017.08.068
27. Aribas E, van Lennep JER, Elias-Smale SE, Piek JJ, Roos M, Ahmadizar F, et al. Prevalence of microvascular angina among patients with stable symptoms in the absence of obstructive coronary artery disease: a systematic review. Cardiovasc Res. (2022) 118:763–71. doi: 10.1093/cvr/cvab061
28. Murthy VL, Naya M, Taqueti VR, Foster CR, Gaber M, Hainer J, et al. Effects of sex on coronary microvascular dysfunction and cardiac outcomes. Circulation. (2014) 129:2518–27. doi: 10.1161/CIRCULATIONAHA.113.008507
29. Beltrame JF, Crea F, Kaski JC, Ogawa H, Ong P, Sechtem U, et al. International standardization of diagnostic criteria for vasospastic angina. Eur Heart J. (2017) 38:2565–8. doi: 10.1093/eurheartj/ehv351
30. Godo S, Suda A, Takahashi J, Yasuda S, Shimokawa H. Coronary microvascular dysfunction. Arterioscler Thromb Vasc Biol. (2021) 41:1625–37. doi: 10.1161/ATVBAHA.121.316025
31. Corban MT, Lerman LO, Lerman A. Endothelial dysfunction. Arterioscler Thromb Vasc Biol. (2019) 39:1272–4. doi: 10.1161/ATVBAHA.119.312836
32. Gimbrone MA, Garcia-Cardena G. Endothelial cell dysfunction and the pathobiology of atherosclerosis. Circ Res. (2016) 118:620–36. doi: 10.1161/CIRCRESAHA.115.306301
33. Lanza GA, Careri G, Crea F. Mechanisms of coronary artery spasm. Circulation. (2011) 124:1774–82. doi: 10.1161/CIRCULATIONAHA.111.037283
34. Taqueti VR, Di Carli MF. Coronary microvascular disease pathogenic mechanisms and therapeutic options: Jacc state-of-the-art review. J Am Coll Cardiol. (2018) 72:2625–41. doi: 10.1016/j.jacc.2018.09.042
35. Amigues I, Russo C, Giles JT, Tugcu A, Weinberg R, Bokhari S, et al. Myocardial microvascular dysfunction in rheumatoid arthritisquantitation by (13)N-ammonia positron emission tomography/computed tomography. Circ Cardiovasc Imaging. (2019) 12:e007495. doi: 10.1161/CIRCIMAGING.117.007495
36. Faccini A, Kaski JC, Camici PG. Coronary microvascular dysfunction in chronic inflammatory rheumatoid diseases. Eur Heart J. (2016) 37:1799–806. doi: 10.1093/eurheartj/ehw018
37. Liao KP, Huang J, He Z, Cremone G, Lam E, Hainer JM, et al. Coronary microvascular dysfunction in rheumatoid arthritis compared to diabetes mellitus and association with all-cause mortality. Arthritis Care Res. (2021) 73:159–65. doi: 10.1002/acr.24108
38. Faccini A, Agricola E, Oppizzi M, Margonato A, Galderisi M, Sabbadini MG, et al. Coronary microvascular dysfunction in asymptomatic patients affected by systemic sclerosis - limited Vs. diffuse form. Circ J. (2015) 79:825–9. doi: 10.1253/circj.CJ-14-1114
39. Vacca A, Montisci R, Garau P, Siotto P, Piga M, Cauli A, et al. Prognostic impact of coronary microcirculation abnormalities in systemic sclerosis: a prospective study to evaluate the role of non-invasive tests. Arthritis Res Ther. (2013) 15:R8. doi: 10.1186/ar4136
40. Lima DS, Sato EI, Lima VC, Miranda F, Hatta FH. Brachial endothelial function is impaired in patients with systemic lupus erythematosus. J Rheumatol. (2002) 29:292–7. https://www.jrheum.org/content/29/2/292.long
41. Koletsos N, Gkaliagkousi E, Lazaridis A, Triantafyllou A, Anyfanti P, Dolgyras P, et al. Skin microvascular dysfunction in systemic lupus erythematosus patients with and without cardiovascular risk factors. Rheumatology. (2021) 60:2834–41. doi: 10.1093/rheumatology/keaa722
42. Svensson C, Eriksson P, Bjarnegard N, Jonasson H, Stromberg T, Sjowall C, et al. Impaired microcirculation and vascular hemodynamics in relation to macrocirculation in patients with systemic lupus erythematosus. Front Med. (2021) 8:722758. doi: 10.3389/fmed.2021.722758
43. Tumurkhuu G, Montano E, Jefferies C. Innate immune dysregulation in the development of cardiovascular disease in lupus. Curr Rheumatol Rep. (2019) 21:46. doi: 10.1007/s11926-019-0842-9
44. Ruiz-Limon P, Barbarroja N, Perez-Sanchez C, Aguirre MA, Bertolaccini ML, Khamashta MA, et al. Atherosclerosis and cardiovascular disease in systemic lupus erythematosus: effects of in vivo statin treatment. Ann Rheum Dis. (2015) 74:1450–8. doi: 10.1136/annrheumdis-2013-204351
45. Colonna L, Lood C, Elkon KB. Beyond apoptosis in lupus. Curr Opin Rheumatol. (2014) 26:459–66. doi: 10.1097/BOR.0000000000000083
46. Saxena V, Lienesch DW, Zhou M, Bommireddy R, Azhar M, Doetschman T, et al. Dual roles of immunoregulatory cytokine tgf-beta in the pathogenesis of autoimmunity-mediated organ damage. J Immunol. (2008) 180:1903–12. doi: 10.4049/jimmunol.180.3.1903
47. Wigren M, Nilsson J, Kaplan MJ. Pathogenic immunity in systemic lupus erythematosus and atherosclerosis: common mechanisms and possible targets for intervention. J Intern Med. (2015) 278:494–506. doi: 10.1111/joim.12357
48. Wigren M, Svenungsson E, Mattisson IY, Gustafsson JT, Gunnarsson I, Zickert A, et al. Cardiovascular disease in systemic lupus erythematosus is associated with increased levels of biomarkers reflecting receptor-activated apoptosis. Atherosclerosis. (2018) 270:1–7. doi: 10.1016/j.atherosclerosis.2018.01.022
49. Svenungsson E, Engelbertsen D, Wigren M, Gustafsson JT, Gunnarsson I, Elvin K, et al. Decreased levels of autoantibodies against apolipoprotein B-100 antigens are associated with cardiovascular disease in systemic lupus erythematosus. Clin Exp Immunol. (2015) 181:417–26. doi: 10.1111/cei.12651
50. Mahajan A, Herrmann M, Munoz LE. Clearance deficiency and cell death pathways: a model for the pathogenesis of sle. Front Immunol. (2016) 7:35. doi: 10.3389/fimmu.2016.00035
51. McCarthy EM, Smith S, Lee RZ, Cunnane G, Doran MF, Donnelly S, et al. The association of cytokines with disease activity and damage scores in systemic lupus erythematosus patients. Rheumatology. (2014) 53:1586–94. doi: 10.1093/rheumatology/ket428
52. Zivkovic V, Cvetkovic T, Mitic B, Stamenkovic B, Stojanovic S, Radovanovic-Dinic B, et al. Monocyte chemoattractant protein-1 as a marker of systemic lupus erythematosus: an observational study. Rheumatol Int. (2018) 38:1003–8. doi: 10.1007/s00296-017-3888-x
53. Lee YH, Song GG. Urinary Mcp-1 as a biomarker for lupus nephritis: a meta-analysis. Z Rheumatol. (2017) 76:357–63. doi: 10.1007/s00393-016-0109-z
54. Tabas I, Bornfeldt KE. Macrophage phenotype and function in different stages of atherosclerosis. Circ Res. (2016) 118:653–67. doi: 10.1161/CIRCRESAHA.115.306256
55. Boshuizen MC, Hoeksema MA, Neele AE, van der Velden S, Hamers AA, Van den Bossche J, et al. Interferon-beta promotes macrophage foam cell formation by altering both cholesterol influx and efflux mechanisms. Cytokine. (2016) 77:220–6. doi: 10.1016/j.cyto.2015.09.016
56. Ramji DP, Davies TS. Cytokines in atherosclerosis: key players in all stages of disease and promising therapeutic targets. Cytokine Growth Factor Rev. (2015) 26:673–85. doi: 10.1016/j.cytogfr.2015.04.003
57. Ding D, Su D, Li X, Li Z, Wang Y, Qiu J, et al. Serum levels of monocyte chemoattractant protein-1 and all-cause and cardiovascular mortality among patients with coronary artery disease. PLoS ONE. (2015) 10:e0120633. doi: 10.1371/journal.pone.0120633
58. Park JK, Kim JY, Moon JY, Ahn EY, Lee EY, Lee EB, et al. Altered lipoproteins in patients with systemic lupus erythematosus are associated with augmented oxidative stress: a potential role in atherosclerosis. Arthritis Res Ther. (2016) 18:306. doi: 10.1186/s13075-016-1204-x
59. Tan C, Liu Y, Li W, Deng F, Liu X, Wang X, et al. Associations of matrix metalloproteinase-9 and monocyte chemoattractant protein-1 concentrations with carotid atherosclerosis, based on measurements of plaque and intima-media thickness. Atherosclerosis. (2014) 232:199–203. doi: 10.1016/j.atherosclerosis.2013.11.040
60. Denny MF, Thacker S, Mehta H, Somers EC, Dodick T, Barrat FJ, et al. Interferon-alpha promotes abnormal vasculogenesis in lupus: a potential pathway for premature atherosclerosis. Blood. (2007) 110:2907–15. doi: 10.1182/blood-2007-05-089086
61. Somers EC, Zhao W, Lewis EE, Wang L, Wing JJ, Sundaram B, et al. Type I interferons are associated with subclinical markers of cardiovascular disease in a cohort of systemic lupus erythematosus patients. PLoS ONE. (2012) 7:e37000. doi: 10.1371/journal.pone.0037000
62. Buie JJ, Renaud LL, Muise-Helmericks R, Oates JC. Ifn-alpha negatively regulates the expression of endothelial nitric oxide synthase and nitric oxide production: implications for systemic lupus erythematosus. J Immunol. (2017) 199:1979–88. doi: 10.4049/jimmunol.1600108
63. Reynolds JA, Haque S, Williamson K, Ray DW, Alexander MY, Bruce IN. Vitamin D improves endothelial dysfunction and restores myeloid angiogenic cell function via reduced cxcl-10 expression in systemic lupus erythematosus. Sci Rep. (2016) 6:22341. doi: 10.1038/srep22341
64. Lood C, Blanco LP, Purmalek MM, Carmona-Rivera C, De Ravin SS, Smith CK, et al. Neutrophil extracellular traps enriched in oxidized mitochondrial DNA are interferogenic and contribute to lupus-like disease. Nat Med. (2016) 22:146–53. doi: 10.1038/nm.4027
65. Yu Y, Su K. Neutrophil extracellular traps and systemic lupus erythematosus. J Clin Cell Immunol. (2013) 4:139. doi: 10.4172/2155-9899.1000139
66. Knight JS, Kaplan MJ. Lupus neutrophils: 'net' gain in understanding lupus pathogenesis. Curr Opin Rheumatol. (2012) 24:441–50. doi: 10.1097/BOR.0b013e3283546703
67. Apel F, Andreeva L, Knackstedt LS, Streeck R, Frese CK, Goosmann C, et al. The cytosolic DNA sensor cgas recognizes neutrophil extracellular traps. Sci Signal. (2021) 14:eaax7942. doi: 10.1126/scisignal.aax7942
68. Carlucci PM, Purmalek MM, Dey AK, Temesgen-Oyelakin Y, Sakhardande S, Joshi AA, et al. Neutrophil subsets and their gene signature associate with vascular inflammation and coronary atherosclerosis in lupus. JCI Insight. (2018) 3:e99276. doi: 10.1172/jci.insight.99276
69. Carmona-Rivera C, Kaplan MJ. Low-density granulocytes: a distinct class of neutrophils in systemic autoimmunity. Semin Immunopathol. (2013) 35:455–63. doi: 10.1007/s00281-013-0375-7
70. Carmona-Rivera C, Zhao W, Yalavarthi S, Kaplan MJ. Neutrophil extracellular traps induce endothelial dysfunction in systemic lupus erythematosus through the activation of matrix metalloproteinase-2. Ann Rheum Dis. (2015) 74:1417–24. doi: 10.1136/annrheumdis-2013-204837
71. Mohan S, Barsalou J, Bradley TJ, Slorach C, Reynolds JA, Hasni S, et al. Endothelial progenitor cell phenotype and function are impaired in childhood-onset systemic lupus erythematosus. Arthritis Rheumatol. (2015) 67:2257–62. doi: 10.1002/art.39149
72. Van Avondt K, Fritsch-Stork R, Derksen RH, Meyaard L. Ligation of signal inhibitory receptor on leukocytes-1 suppresses the release of neutrophil extracellular traps in systemic lupus erythematosus. PLoS ONE. (2013) 8:e78459. doi: 10.1371/journal.pone.0078459
73. Liu Y, Kaplan MJ. Cardiovascular disease in systemic lupus erythematosus: an update. Curr Opin Rheumatol. (2018) 30:441–8. doi: 10.1097/BOR.0000000000000528
74. Parker B, Urowitz MB, Gladman DD, Lunt M, Bae SC, Sanchez-Guerrero J, et al. Clinical associations of the metabolic syndrome in systemic lupus erythematosus: data from an international inception cohort. Ann Rheum Dis. (2013) 72:1308–14. doi: 10.1136/annrheumdis-2012-202106
75. Chung CP, Oeser A, Solus JF, Gebretsadik T, Shintani A, Avalos I, et al. Inflammation-associated insulin resistance: differential effects in rheumatoid arthritis and systemic lupus erythematosus define potential mechanisms. Arthritis Rheum. (2008) 58:2105–12. doi: 10.1002/art.23600
76. del Aguila LF, Claffey KP, Kirwan JP. Tnf-Alpha impairs insulin signaling and insulin stimulation of glucose uptake in C2c12 muscle cells. Am J Physiol. (1999) 276:E849–55. doi: 10.1152/ajpendo.1999.276.5.E849
77. Hotamisligil GS. Molecular mechanisms of insulin resistance and the role of the adipocyte. Int J Obes Relat Metab Disord. (2000) 24:S23–7. doi: 10.1038/sj.ijo.0801497
78. Ormseth MJ, Swift LL, Fazio S, Linton MF, Raggi P, Solus JF, et al. Free fatty acids are associated with metabolic syndrome and insulin resistance but not inflammation in systemic lupus erythematosus. Lupus. (2013) 22:26–33. doi: 10.1177/0961203312462756
79. Bouloumie A, Marumo T, Lafontan M, Busse R. Leptin induces oxidative stress in human endothelial cells. FASEB J. (1999) 13:1231–8. doi: 10.1096/fasebj.13.10.1231
80. Dong F, Zhang X, Ren J. Leptin regulates cardiomyocyte contractile function through endothelin-1 receptor-NADPH oxidase pathway. Hypertension. (2006) 47:222–9. doi: 10.1161/01.HYP.0000198555.51645.f1
81. Fernandez-Riejos P, Najib S, Santos-Alvarez J, Martin-Romero C, Perez-Perez A, Gonzalez-Yanes C, et al. Role of leptin in the activation of immune cells. Mediators Inflamm. (2010) 2010:568343. doi: 10.1155/2010/568343
82. Kelesidis T, Kelesidis I, Chou S, Mantzoros CS. Narrative review: the role of leptin in human physiology: emerging clinical applications. Ann Intern Med. (2010) 152:93–100. doi: 10.7326/0003-4819-152-2-201001190-00008
83. Garcia-Gonzalez A, Gonzalez-Lopez L, Valera-Gonzalez IC, Cardona-Munoz EG, Salazar-Paramo M, Gonzalez-Ortiz M, et al. Serum leptin levels in women with systemic lupus erythematosus. Rheumatol Int. (2002) 22:138–41. doi: 10.1007/s00296-002-0216-9
84. McMahon M, Skaggs BJ, Sahakian L, Grossman J, FitzGerald J, Ragavendra N, et al. High plasma leptin levels confer increased risk of atherosclerosis in women with systemic lupus erythematosus, and are associated with inflammatory oxidised lipids. Ann Rheum Dis. (2011) 70:1619–24. doi: 10.1136/ard.2010.142737
85. Perez-Sanchez C, Barbarroja N, Messineo S, Ruiz-Limon P, Rodriguez-Ariza A, Jimenez-Gomez Y, et al. Gene profiling reveals specific molecular pathways in the pathogenesis of atherosclerosis and cardiovascular disease in antiphospholipid syndrome, systemic lupus erythematosus and antiphospholipid syndrome with lupus. Ann Rheum Dis. (2015) 74:1441–9. doi: 10.1136/annrheumdis-2013-204600
86. Gulati M, Levy PD, Mukherjee D, Amsterdam E, Bhatt DL, Birtcher KK, et al. 2021 Aha/Acc/Ase/Chest/Saem/Scct/Scmr guideline for the evaluation and diagnosis of chest pain: a report of the American college of Cardiology/American heart association joint committee on clinical practice guidelines. Circulation. (2021) 144:e368–454. doi: 10.1161/CIR.0000000000001029
87. Kunadian V, Chieffo A, Camici PG, Berry C, Escaned J, Maas A, et al. An eapci expert consensus document on ischaemia with non-obstructive coronary arteries in collaboration with European society of cardiology working group on coronary pathophysiology & microcirculation endorsed by coronary vasomotor disorders international study group. Eur Heart J. (2020) 41:3504–20. doi: 10.1093/eurheartj/ehaa503
88. Prescott E, Abildstrom SZ, Aziz A, Merz NB, Gustafsson I, Halcox J, et al. Improving diagnosis and treatment of women with angina pectoris and microvascular disease: the ipower study design and rationale. Am Heart J. (2014) 167:452–8. doi: 10.1016/j.ahj.2014.01.003
89. Kakuta K, Dohi K, Sato Y, Yamanaka T, Kawamura M, Ogura T, et al. Chronic inflammatory disease is an independent risk factor for coronary flow velocity reserve impairment unrelated to the processes of coronary artery calcium deposition. J Am Soc Echocardiogr. (2016) 29:173–80. doi: 10.1016/j.echo.2015.09.001
90. Hirata K, Kadirvelu A, Kinjo M, Sciacca R, Sugioka K, Otsuka R, et al. Altered coronary vasomotor function in young patients with systemic lupus erythematosus. Arthritis Rheum. (2007) 56:1904–9. doi: 10.1002/art.22702
91. Murthy VL, Bateman TM, Beanlands RS, Berman DS, Borges-Neto S, Chareonthaitawee P, et al. Clinical quantification of myocardial blood flow using pet: joint position paper of the snmmi cardiovascular council and the asnc. J Nucl Cardiol. (2018) 25:269–97. doi: 10.1007/s12350-017-1110-x
92. Weber BN, Stevens E, Barrett L, Bay C, Sinnette C, Brown JM, et al. Coronary microvascular dysfunction in systemic lupus erythematosus. J Am Heart Assoc. (2021) 10:e018555. doi: 10.1161/JAHA.120.018555
93. Recio-Mayoral A, Mason JC, Kaski JC, Rubens MB, Harari OA, Camici PG. Chronic inflammation and coronary microvascular dysfunction in patients without risk factors for coronary artery disease. Eur Heart J. (2009) 30:1837–43. doi: 10.1093/eurheartj/ehp205
94. Weber BN, Stevens E, Perez-Chada LM, Brown JM, Divakaran S, Bay C, et al. Impaired coronary vasodilator reserve and adverse prognosis in patients with systemic inflammatory disorders. JACC Cardiovasc Imaging. (2021) 14:2212–20. doi: 10.1016/j.jcmg.2020.12.031
95. Feher A, Boutagy NE, Oikonomou EK, Liu YH, Miller EJ, Sinusas AJ, et al. Association between impaired myocardial flow reserve on (82)rubidium positron emission tomography imaging and adverse events in patients with autoimmune rheumatic disease. Circ Cardiovasc Imaging. (2021) 14:e012208. doi: 10.1161/CIRCIMAGING.120.012208
96. Patel AR, Salerno M, Kwong RY, Singh A, Heydari B, Kramer CM. Stress cardiac magnetic resonance myocardial perfusion imaging: jacc review topic of the week. J Am Coll Cardiol. (2021) 78:1655–68. doi: 10.1016/j.jacc.2021.08.022
97. Indorkar R, Kwong RY, Romano S, White BE, Chia RC, Trybula M, et al. Global coronary flow reserve measured during stress cardiac magnetic resonance imaging is an independent predictor of adverse cardiovascular events. JACC Cardiovasc Imaging. (2019) 12:1686–95. doi: 10.1016/j.jcmg.2018.08.018
98. Caforio ALP, Adler Y, Agostini C, Allanore Y, Anastasakis A, Arad M, et al. Diagnosis and management of myocardial involvement in systemic immune-mediated diseases: a position statement of the European society of cardiology working group on myocardial and pericardial disease. Eur Heart J. (2017) 38:2649–62. doi: 10.1093/eurheartj/ehx321
99. Thomson LE, Wei J, Agarwal M, Haft-Baradaran A, Shufelt C, Mehta PK, et al. Cardiac magnetic resonance myocardial perfusion reserve index is reduced in women with coronary microvascular dysfunction. a national heart, lung, and blood institute-sponsored study from the women's ischemia syndrome evaluation. Circ Cardiovasc Imaging. (2015) 8:e002481. doi: 10.1161/CIRCIMAGING.114.002481
100. Rahman H, Scannell CM, Demir OM, Ryan M, McConkey H, Ellis H, et al. High-resolution cardiac magnetic resonance imaging techniques for the identification of coronary microvascular dysfunction. JACC Cardiovasc Imaging. (2021) 14:978–86. doi: 10.1016/j.jcmg.2020.10.015
101. Zhou W, Lee JCY, Leung ST, Lai A, Lee TF, Chiang JB, et al. Long-term prognosis of patients with coronary microvascular disease using stress perfusion cardiac magnetic resonance. JACC Cardiovasc Imaging. (2021) 14:602–11. doi: 10.1016/j.jcmg.2020.09.034
102. Ishimori ML, Martin R, Berman DS, Goykhman P, Shaw LJ, Shufelt C, et al. Myocardial ischemia in the absence of obstructive coronary artery disease in systemic lupus erythematosus. JACC Cardiovasc Imaging. (2011) 4:27–33. doi: 10.1016/j.jcmg.2010.09.019
103. Sandhu VK, Wei J, Thomson LEJ, Berman DS, Schapira J, Wallace D, et al. Five-year follow-up of coronary microvascular dysfunction and coronary artery disease in systemic lupus erythematosus: results from a community-based lupus cohort. Arthritis Care Res. (2020) 72:882–7. doi: 10.1002/acr.23920
104. AlBadri A, Bairey Merz CN, Johnson BD, Wei J, Mehta PK, Cook-Wiens G, et al. Impact of abnormal coronary reactivity on long-term clinical outcomes in women. J Am Coll Cardiol. (2019) 73:684–93. doi: 10.1016/j.jacc.2018.11.040
Keywords: systemic lupus erythematosus, chest pain, coronary microvascular dysfunction, coronary vasospasm, ischemic heart disease
Citation: Manchanda AS, Kwan AC, Ishimori M, Thomson LEJ, Li D, Berman DS, Bairey Merz CN, Jefferies C and Wei J (2022) Coronary Microvascular Dysfunction in Patients With Systemic Lupus Erythematosus and Chest Pain. Front. Cardiovasc. Med. 9:867155. doi: 10.3389/fcvm.2022.867155
Received: 31 January 2022; Accepted: 28 February 2022;
Published: 15 April 2022.
Edited by:
Sophie Mavrogeni, Onassis Cardiac Surgery Center, GreeceReviewed by:
Jas Sara, Mayo Clinic, United StatesFilippo Cademartiri, Gabriele Monasterio Tuscany Foundation (CNR), Italy
Copyright © 2022 Manchanda, Kwan, Ishimori, Thomson, Li, Berman, Bairey Merz, Jefferies and Wei. This is an open-access article distributed under the terms of the Creative Commons Attribution License (CC BY). The use, distribution or reproduction in other forums is permitted, provided the original author(s) and the copyright owner(s) are credited and that the original publication in this journal is cited, in accordance with accepted academic practice. No use, distribution or reproduction is permitted which does not comply with these terms.
*Correspondence: Janet Wei, janet.wei@cshs.org