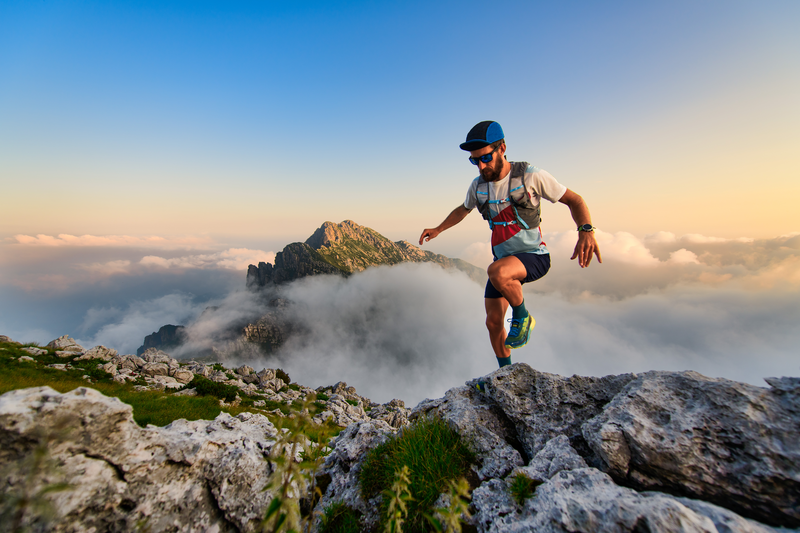
95% of researchers rate our articles as excellent or good
Learn more about the work of our research integrity team to safeguard the quality of each article we publish.
Find out more
MINI REVIEW article
Front. Cardiovasc. Med. , 29 March 2022
Sec. Thrombosis and Haemostasis
Volume 9 - 2022 | https://doi.org/10.3389/fcvm.2022.864899
This article is part of the Research Topic Anticoagulation in Cardiovascular Diseases: Evolving role, unmet needs and grey areas View all 26 articles
Most patients present for catheter ablation of atrial fibrillation (CAAF) with residual or full effect of vitamin K antagonists (VKAs) or direct oral anticoagulants (DOACs). In daily practice, it has been observed that the activated clotting time (ACT) was actually poorly sensitive to the effect of DOACs and that patients on DOACs required more unfractionated heparin (UFH) to achieve the ACT target of 300 s during the procedure, leading some authors to worry about potential overdosing. Conversely, we hypothesize that these higher doses of UFH are necessary to achieve adequate hemostasis during CAAF regardless of the residual effect of DOACs. During CAAF, thrombosis is promoted mainly by the presence of thrombogenic sheaths and catheters in the bloodstream. Preclinical data suggest that only high doses of DOACs are able to mitigate catheter-induced thrombin generation, whereas low dose UFH already do so. In addition, the effect of UFH seems to be lower in patients on DOACs, compared to patients on VKAs, explaining part of the differences observed in heparin requirements. Clinical studies could not identify increased bleeding risk in patients on DOACs compared to those on VKAs despite similar efficacy during CAAF procedures. Moreover, targeting a lower ACT was associated with an increased periprocedural thrombotic risk for both DOAC and VKA patients. Therefore, the low sensitivity of the ACT to the residual effect of DOACs should not be a major concern in its use in the interventional cardiology laboratory.
Atrial fibrillation (AF) is associated with a significant thrombotic risk, requiring long-term anticoagulation in patients with intermediate or high thrombotic risk (1–4). Nowadays, vitamin K antagonists (VKAs) and direct oral anticoagulants (DOACs) are the main anticoagulants for stroke prevention in non-valvular AF (1–3). Over the years, catheter ablation of atrial fibrillation (CAAF) has become a first- or second-line treatment for symptomatic AF (5). However, the procedure is associated with a thrombotic risk and requires the administration of high-dose unfractionated heparin (UFH; between 50 and 120 units per kg just before or immediately after transseptal puncture), exposing patients to a risk of bleeding (5); reported incidences of bleeding (e.g., groin bleeding/hematoma, cardiac tamponade) and embolic (e.g., transient ischemic attacks, strokes) complications during hospitalization being ~1.9 and 0.2%, respectively (6). Before ablation, most patients receive anticoagulation for at least 3 weeks to reduce the thromboembolic risk associated with CAAF (5).
Historically, VKAs therapy was interrupted and “bridged” with low molecular weight heparins (LMWH) before and after CAAF. In 2014, the COMPARE randomized trial identified a lower rate of periprocedural stroke and minor bleeding when warfarin was continued with an INR in the therapeutic range (i.e., between 2.0 and 3.0) throughout the periprocedural period, compared with discontinuation with LMWH bridging (7). With the introduction of DOACs in non-valvular AF patients, due to the concern of potentially major bleeding and the lack of a convenient reversal agent, DOACs were discontinued in the preprocedural period. Since then, several randomized trials have compared CAAF with uninterrupted DOAC vs. uninterrupted VKA approaches and found no significant differences between the two groups in terms of thrombotic and bleeding complications (8–15). This approach is now recommended over discontinuation and bridging (5). An acceptable alternative to avoid high DOAC peak plasma concentrations during CAAF is to skip one or two DOAC doses before the procedure (16). However, clinical trials that analyzed uninterrupted or minimally interrupted approaches for DOACs were all underpowered.
During the procedure, repeated measurements of the activated clotting time (ACT) each 10–15 min intervals until therapeutic anticoagulation and then at 15–30 min intervals are recommended to guide UFH administration (5). The test, which consists in measuring the time to clot formation in whole blood after complete activation of the contact pathway (e.g., celite or kaolin), is poorly sensitive to DOACs, especially to direct factor Xa inhibitors, even at high concentrations corresponding to the peak effect (17). An ACT maintained above 300 s is recommended during CAAF on VKA therapy based on studies visualizing a lower incidence of thrombi in left heart chambers and according to observational studies (5, 18–20). This threshold is applied similarly for CAAF on DOAC therapy despite a lower level of evidence. However, higher UFH doses are required to achieve the ACT goal of 300 s when DOACs are on board, compared to VKAs (8, 15, 18, 21–27), which corresponds to a potential overdosage according to some authors (21, 28). Conversely, we hypothesize that these higher doses of UFH are necessary to achieve adequate hemostasis during the procedure and that considering the residual effect of DOACs would not be as important as expected. The following paper will get some insights into the mechanisms of thrombosis during CAAF and the possibilities to manage them.
In addition to possible pre-existing thrombi that may be dislodged by the catheters or by fluctuations in heart rhythm during the procedure, several mechanisms have been advocated to explain thrombi formation during CAAF (29, 30). First, direct endothelial damage may result from the passage of sheaths and catheters from the femoral vein to the left atrium and from thermal injuries during the ablation procedure; radiofrequency ablation could be more thrombogenic than cryoablation by this mean (31). Second, the contact pathway is activated on the surface of foreign materiel (sheaths, catheters) in the bloodstream (Figure 1) and by cell debris such as DNA or polyphosphates released during thermoablation. Occasionally, emboli may also arise from coagulum or char formation on the ablation electrode, which can be limited by proper technique and does not seem to depend on hemostasis and anticoagulation (32). Of those thrombus/coagulum sources, those formed in the left atrium are particularly dangerous because they are more likely to embolize into the systemic circulation, whereas emboli formed in peripheral veins or right chambers can only embolize into the systemic circulation through an interatrial communication.
Figure 1. Contact pathway activation at the surface of catheters and targets of anticoagulant drugs. Factor XIIa auto-activates on contact with negatively charged surfaces. High molecular weight kininogen (HMWK) is also adsorbed and serves as a cofactor for FXIIa auto-activation. FXIIa then activates FXI to initiate thrombin generation, but also prekallikrein (PK) to kallikrein, resulting in further FXIIa generation. FXIa activates FIX, which forms the intrinsic tenase with FVIIIa. Intrinsic tenase and prothrombinase complexes form on negatively charged surfaces, classically on the surface of activated platelets, but possibly also on the surface of catheters. The thrombin generated then amplifies the reaction by promoting the activation of factors XI, VIII and V, but it is also a potent platelet activator. In addition to the generation of thrombin initiated by FXIIa, other thrombotic mechanisms take place on the surface of catheters (not shown in the figure): the adsorption of proteins such as fibrinogen, which promotes the adhesion and activation of platelets and leukocytes, potentially increasing thrombin generation and thrombogenesis (47–51). FXIIa, kallikrein and thrombin also activate the complement system which promotes platelet activation and thrombin generation (53–57). The effect of anticoagulant drugs is also depicted: unfractionated heparin (UFH) potentiates the inhibitory effect of antithrombin (AT) mainly on free activated factors X and II, but also to a lesser extent on free activated factors IX, XI and XII (86); clot-bound FXa and FIIa, and FXa within the prothrombinase complex, are less accessible to inhibition by AT (87–89). Direct oral anticoagulants (direct F Xa inhibitors (xabans) and the direct thrombin inhibitor (DTI) dabigatran) directly inhibit activated factor II or X, free, within the prothrombinase complex and clot-bound (89–92). Contact pathway inhibitors specifically target factor XI or XII; truncated antibodies against FXIa or FXIIa are shown in the figure, but this category also includes small inhibiting molecules, antisense oligonucleotides and small interfering RNAs (82).
During CAAF, intracardiac ultrasound can be used to directly visualize thrombi formed in the cardiac chambers. Small observational studies identified that thrombi form primarily on transseptal sheaths or on mapping catheters (19, 20, 33–36). Less frequently, thrombi are also seen in the left atrium, pulmonary vein or left atrial appendix; however, as some of these thrombi can be extracted by strong suction through the sheath during its removal, some authors emphasized that those thrombi could have been initially related to the sheath or the catheter itself (35). It should be noted that no thrombi are generally observed on the ablation lesion itself during the procedure and radiofrequency ablation is not associated with an increase in in vivo thrombin generation markers (such as thrombin-antithrombin complexes), compared with the mapping phase or with single electrophysiological studies (33, 37–39). Taken together, these data suggest that sheaths/catheters may be the main source of intracardiac thrombus formation during CAAF.
The thrombogenicity of catheters has been studied in preclinical models (40–46). Yau et al. demonstrated in vitro that clot forms three times faster in the presence of catheters than in their absence (41). They identified that coagulation was activated on the surface of catheters via the contact pathway, as the procoagulant effect of the catheters was reduced or reversed by corn trypsin inhibitor, in plasma deficient in factor XI or XII, or in rabbits treated with antisense oligonucleotide for factor XII or XI (41, 42, 45). Another research group demonstrated that Ixodes ricinus Contact Pathway Inhibitor (Ir-CPI) also has the potential to reduce the procoagulant effect of catheters in vitro (46). Interestingly, they showed that catheters were still able to induce a procoagulant effect in factor XII deficient plasma which could be abolished by the presence of Ir-CPI, suggesting that factor XII is not the only coagulation factor implicated in the activation of the coagulation cascade by catheters (46). As Ir-CPI is a dual inhibitor of both factor XII and factor XI, it is suggested that factor XI is also involved in the thrombogenesis mechanism of catheter-induced thrombosis.
As catheters are the primary site of thrombus formation during CAAF and as these catheters generate thrombin via the contact pathway, contact pathway inhibition may represent the primary target of anticoagulation during CAAF. Although tissue factor (TF) pathway could also contribute to thrombogenesis during the procedure (endothelial lesions by the passage of the sheaths and following cellular destruction during transseptal puncture or the application of thermal energy), UFH at concentrations required to block contact activation would also provide protection on TF-initiated thrombin generation.
Besides direct activation of coagulation upon contact with negatively charged surfaces, other mechanisms can contribute to thrombin generation during CAAF. First, proteins such as fibrinogen are adsorbed to the catheter surface, which promotes platelet adhesion and activation (47–49). Leukocytes can also adhere to adsorbed fibrinogen and platelets (50, 51); leukocytes may then degranulate and promote inflammation. Neutrophils extracellular traps can also activate the coagulation contact pathway and contribute to thrombin generation (52). In addition, the complement system may be activated by FXIIa, kallikrein and thrombin, which may then promote thrombosis through platelet activation and direct thrombin generation (53–57). However, it is less clear to what extent these mechanisms would contribute to thrombogenesis and how clinicians might manage them. Finally, direct measurement of haemostasis proteins/biomarkers in cardiac chambers could be more sensitive and help to understand pathogenesis more precisely (58, 59).
Among the conventional anticoagulants, heparins are preferred for contact inhibition in the acute setting [e.g., CAAF, extracorporeal circuits, mechanical heart valves (MHV)] (Figure 1). Previous work identified that inhibition of catheter-induced thrombin generation was more effective with UFH than with LMWH and poorly effective with fondaparinux, which was also ineffective at blocking FXIIa- and FXIa-initiated thrombin generation (41). Similar results were also observed in a rabbit model of catheter thrombosis (41). This could be due to the greater anti-IIa activity of UFH, compared to LMWH or fondaparinux, or to its upstream effect on free FIXa (60).
Whereas, UFH strongly inhibits contact-initiated thrombin generation, the ability of DOACs to do so may be much less. For example, only dabigatran concentrations of 200 ng/mL and above were able to attenuate in vitro polyurethane catheter-induced thrombin generation, whereas UFH concentrations as low as 0.02 IU/mL could already do so (43). No data are available regarding the ability of direct anti-Xa to mitigate catheter-induced thrombin generation. However, preclinical studies are available in other contact pathway activation models such as mechanical heart valves (MHV). As with catheters models, dabigatran, but also apixaban and rivaroxaban had limited ability to suppress MHV-induced thrombin generation at concentrations consistent with those observed in therapeutically anticoagulated patients (61, 62). Therefore, it is questionable whether full consideration of residual DOACs levels is relevant for thrombosis prevention during CAAF.
Another important aspect to consider is the pharmacodynamic interaction between UFH and oral anticoagulants. Unfortunately, few data are available regarding this topic. A pharmacokinetic-pharmacodynamic study performed in CAAF patients identified that the response to intravenous UFH was similar between patients on dabigatran and patients without baseline anticoagulation (same ACT increase for a given UFH bolus), but was enhanced in patients on VKAs (increased ACT increase for a given UFH bolus) (63). However, dabigatran was often skipped for one dose before the procedure, probably resulting in low plasma concentrations, which limits the findings of the study. Using thrombin generation, other authors identified a reduced response to the in vitro addition of 0.1 IU/mL UFH to the plasma of patients on DOACs (both at Cpeak and Ctrough), compared with the plasma of patients on VKA or healthy volunteers. The response to UFH was greater for samples with dabigatran than for samples with direct anti-Xa inhibitors (64). Finally, Yau et al. identified a synergistic effect on delaying the time to catheter occlusion in rabbits when low-dose dabigatran and UFH were administered concomitantly (43).
During CAAF, UFH administration is guided using the ACT. A variety of devices and cartridges are available, differing in the activator used (e.g., celite, kaolin, glass beads or a combination of these) and the method of measurement (e.g., rotation of a tube, a plunger or movement through capillaries) (17). Systematic differences exist between available ACT devices [which may be more than 100 s in heparinized patients (65–68)], which cannot be used interchangeably (65–71). However, in clinical guidelines, fixed ACT targets (i.e., 300 s) are proposed without differentiating devices (5), which adds variability in the level of anticoagulation achieved from center to center. In addition, various preanalytical variables may influence the ACT, such as blood collection technique (e.g., site of blood collection, amount of blood discarded before sampling, velocity of aspiration during sampling) and processing (e.g., time-interval between collection and analysis, agitation of the sample, prewarming of the reagent) (72, 73). As a result, this could also lead to huge variations in UFH dose administered.
Although the excellent correlation between ACT and UFH concentrations with in vitro spiking of whole blood (74, 75), the association between ACT and ex vivo heparin levels assessed with an anti-Xa assay is poor, especially at high UFH concentrations such as those used during CAAF (i.e., 1–2 IU/mL) (76). Unlike UFH, the ACT shows poor sensitivity to DOACs in vitro, especially to direct factor Xa inhibitors (inability to achieve ACTs >200 s even at supratherapeutic concentrations), whereas its sensitivity to dabigatran, the only direct factor IIa inhibitor, is better (77). When using samples from patients on DOAC, the correlation with direct factor Xa inhibitors levels is even worse (28). As a result, and because of uninterrupted preoperative anticoagulation attitudes, some patients may present in the interventional cardiology laboratory with therapeutic DOACs blood levels with only small ACT prolongations, especially for direct factor Xa inhibitors (78, 79).
Some authors suggested that the higher doses of UFH administered to patients on DOACs, compared with patients on VKAs, could be detrimental by adding to the residual effect of uninterrupted DOACs, putting patients at increased bleeding risk (21, 28). However, meta-analyses of randomized trials and observational studies comparing uninterrupted VKA and DOAC treatment approaches are reassuring, identifying no increase in bleeding risk with DOACs compared with VKAs (80, 81); dabigatran was even safer than VKAs in the RE-CIRCUIT randomized trial [absolute risk difference −5.3% (95% confidence interval: −8.4 to −2.2%), p < 0.001] (10). Furthermore, these increased doses of UFH appear to be necessary to prevent thrombosis during CAAF. Indeed, another meta-analysis identified that, similarly to the uninterrupted VKAs approach, achieving an ACT >300 s for patients on DOAC therapy was associated with a reduced risk of thromboembolic events, compared with an ACT target of <300 s (18). Overall, this suggests that the lack of integration of DOACs levels by the ACT and the hassle of administrating higher UFH doses with the uninterrupted DOAC approach are not worrying in terms of clinical endpoints. Worse, aiming for lower ACTs for fear of overanticoagulation could be deleterious by increasing thrombotic risk.
Due to the expected predominant role of the contact pathway in procedural thrombosis, contact pathway inhibitors are attractive for anticoagulation during CAAF. Contact pathway inhibitors are pharmacologic agents targeting specifically factor XII or XI using truncated antibodies, small inhibiting molecules, antisense oligonucleotides (ASO) or small interfering RNAs (82). These molecules are able to profoundly block contact activation with no effect on hemostasis initiated by TF exposure. At present, these molecules have been used successfully in preclinical models of extracorporeal life support (83, 84), and were associated with excellent thrombosis prevention with minimal bleeding risk (sometimes lower than UFH). In human, FXI ASO were more effective than LMWH to prevent venous thrombosis after total knee arthroplasty with a lower incidence of clinically relevant bleeding (85). However, the utilization of these molecules during CAAF should be done with caution as the contribution of TF-induced thrombin generation to thrombosis during the procedure remains unresolved. Whether this mechanism does significantly contribute to thrombosis, and whether residual VKAs or DOACs concentrations in the context of uninterrupted approaches would be enough to counter this specific risk would deserve to be carefully studied.
Although the activated clotting time is poorly sensitive to the effect of direct factor Xa inhibitors, the latter may not be very effective in mitigating catheter-induced thrombin generation, at least at concentrations encountered in the interventional cardiology laboratory. Furthermore, the higher UFH doses required to achieve the ACT target of 300 s in patients on uninterrupted DOAC therapy, compared with those required in an uninterrupted VKA approach, do not appear to dangerously compromise the hemostatic competence of those patients, as evidenced by available randomized controlled trials and meta-analysis. Although the reliability of the ACT for assessing overall coagulation in the presence of high-dose heparin may still be questioned, its low sensitivity to the residual effect of direct factor Xa inhibitors is not a major concern in its use in the interventional cardiology laboratory.
MH, FM, and SL wrote the first draft of the manuscript. All authors contributed to manuscript writing, revision, read, and approved the submitted version.
JD is the CEO and founder of QUALIblood s.a., a contract research organization manufacturing the DP-Filter, is a coinventor of the DP-Filter (patent application number: PCT/ET2019/052903) and reports personal fees from Daiichi-Sankyo, Mithra Pharmaceuticals, Stago, Roche and Roche Diagnostics outside the submitted work. FM reports institutional fees from Stago, Werfen, Nodia, Roche Sysmex and Bayer. He also reports speaker fees from Boehringer Ingelheim, Bayer Healthcare, Bristol-Myers Squibb, Pfizer, Stago, Sysmex and Aspen all outside the submitted work.
The remaining authors declare that the research was conducted in the absence of any commercial or financial relationships that could be construed as a potential conflict of interest.
All claims expressed in this article are solely those of the authors and do not necessarily represent those of their affiliated organizations, or those of the publisher, the editors and the reviewers. Any product that may be evaluated in this article, or claim that may be made by its manufacturer, is not guaranteed or endorsed by the publisher.
The authors would like to thank Professor Thomas Lecompte (Université de Nancy) for providing very sound and helpful advice on the content of the manuscript. The authors also thank the Fondation Mont-Godinne for the research grant awarded to MH.
1. Lip GYH, Banerjee A, Boriani G, Chiang CE, Fargo R, Freedman B, et al. Antithrombotic therapy for atrial fibrillation: CHEST guideline and expert panel report. Chest. (2018) 154:1121–201. doi: 10.1016/j.chest.2018.07.040
2. Andrade JG, Aguilar M, Atzema C, Bell A, Cairns JA, Cheung CC, et al. The 2020 Canadian cardiovascular society/canadian heart rhythm society comprehensive guidelines for the management of atrial fibrillation. Can J Cardiol. (2020) 36:1847–948. doi: 10.1016/j.cjca.2020.09.001
3. Hindricks G, Potpara T, Dagres N, Arbelo E, Bax JJ, Blomstrom-Lundqvist C, et al. 2020 ESC Guidelines for the diagnosis management of atrial fibrillation developed in collaboration with the European Association for Cardio-Thoracic Surgery (EACTS): The Task Force for the diagnosis management of atrial fibrillation of the European Society of Cardiology (ESC) Developed with the special contribution of the European Heart Rhythm Association (EHRA) of the ESC. Eur Heart J. (2021) 42:373–498. doi: 10.1093/eurheartj/ehab648
4. January CT, Wann LS, Calkins H, Chen LY, Cigarroa JE, Cleveland JC Jr., et al. 2019 AHA/ACC/HRS focused update of the 2014 AHA/ACC/HRS guideline for the management of patients with atrial fibrillation: A Report of the American College of Cardiology/American Heart Association Task Force on Clinical Practice Guidelines and the Heart Rhythm Society. J Am Coll Cardiol. (2019) 74:104–32. doi: 10.1016/j.jacc.2019.01.011
5. Calkins H, Hindricks G, Cappato R, Kim YH, Saad EB, Aguinaga L, et al. 2017 HRS/EHRA/ECAS/APHRS/SOLAECE expert consensus statement on catheter and surgical ablation of atrial fibrillation. Europace. (2018) 20:e1–160. doi: 10.1093/europace/eux274
6. Kusano K, Yamane T, Inoue K, Takegami M, Nakao YM, Miyamoto Y, et al. The Japanese catheter ablation registry (J-AB): a prospective nationwide multicenter registry in Japan. Annual report in 2018. J Arrhythm. (2020) 36:953–61. doi: 10.1002/joa3.12445
7. Di Biase L, Burkhardt JD, Santangeli P, Mohanty P, Sanchez JE, Horton R, et al. Periprocedural stroke and bleeding complications in patients undergoing catheter ablation of atrial fibrillation with different anticoagulation management: results from the Role of Coumadin in Preventing Thromboembolism in Atrial Fibrillation (AF) Patients Undergoing Catheter Ablation (COMPARE) randomized trial. Circulation. (2014) 129:2638–44. doi: 10.1161/CIRCULATIONAHA.113.006426
8. Cappato R, Marchlinski FE, Hohnloser SH, Naccarelli GV, Xiang J, Wilber DJ, et al. Uninterrupted rivaroxaban vs. uninterrupted vitamin K antagonists for catheter ablation in non-valvular atrial fibrillation. Eur Heart J. (2015) 36:1805–11. doi: 10.1093/eurheartj/ehv177
9. Kuwahara T, Abe M, Yamaki M, Fujieda H, Abe Y, Hashimoto K, et al. Apixaban versus warfarin for the prevention of periprocedural cerebral thromboembolism in atrial fibrillation ablation: multicenter prospective randomized study. J Cardiovasc Electrophysiol. (2016) 27:549–54. doi: 10.1111/jce.12928
10. Calkins H, Willems S, Gerstenfeld EP, Verma A, Schilling R, Hohnloser SH, et al. Uninterrupted dabigatran versus warfarin for ablation in atrial fibrillation. N Engl J Med. (2017) 376:1627–36. doi: 10.1056/NEJMoa1701005
11. Di Biase L, Callans D, Haeusler KG, Hindricks G, Al-Khalidi H, Mont L, et al. Rationale and design of AXAFA-AFNET 5: an investigator-initiated, randomized, open, blinded outcome assessment, multi-centre trial to comparing continuous apixaban to vitamin K antagonists in patients undergoing atrial fibrillation catheter ablation. Europace. (2017) 19:132–8. doi: 10.1093/europace/euw368
12. Kimura T, Kashimura S, Nishiyama T, Katsumata Y, Inagawa K, Ikegami Y, et al. Asymptomatic cerebral infarction during catheter ablation for atrial fibrillation: comparing uninterrupted rivaroxaban and warfarin (ASCERTAIN). JACC Clin Electrophysiol. (2018) 4:1598–609. doi: 10.1016/j.jacep.2018.08.003
13. Reynolds MR, Allison JS, Natale A, Weisberg IL, Ellenbogen KA, Richards M, et al. A prospective randomized trial of apixaban dosing during atrial fibrillation ablation: the AEIOU trial. JACC Clin Electrophysiol. (2018) 4:580–8. doi: 10.1016/j.jacep.2017.11.005
14. Kirchhof P, Haeusler KG, Blank B, De Bono J, Callans D, Elvan A, et al. Apixaban in patients at risk of stroke undergoing atrial fibrillation ablation. Eur Heart J. (2018) 39:2942–55. doi: 10.1093/eurheartj/ehy176
15. Hohnloser SH, Camm J, Cappato R, Diener HC, Heidbuchel H, Mont L, et al. Uninterrupted edoxaban vs. vitamin K antagonists for ablation of atrial fibrillation: the ELIMINATE-AF trial. Eur Heart J. (2019) 40:3013–21. doi: 10.1093/eurheartj/ehz190
16. Yu HT, Shim J, Park J, Kim TH, Uhm JS, Kim JY, et al. When is it appropriate to stop non-vitamin K antagonist oral anticoagulants before catheter ablation of atrial fibrillation? A multicentre prospective randomized study. Eur Heart J. (2019) 40:1531–7. doi: 10.1093/eurheartj/ehy870
17. Horton S, Augustin S. Activated clotting time (ACT). In: Monagle P, editor. Haemostasis: Methods and Protocols. Totowa, NJ: Humana Press (2013). p. 155–67.
18. Briceno DF, Villablanca PA, Lupercio F, Kargoli F, Jagannath A, Londono A, et al. Clinical impact of heparin kinetics during catheter ablation of atrial fibrillation: meta-analysis and meta-regression. J Cardiovasc Electrophysiol. (2016) 27:683–93. doi: 10.1111/jce.12975
19. Wazni OM, Rossillo A, Marrouche NF, Saad EB, Martin DO, Bhargava M, et al. Embolic events and char formation during pulmonary vein isolation in patients with atrial fibrillation: impact of different anticoagulation regimens and importance of intracardiac echo imaging. J Cardiovasc Electrophysiol. (2005) 16:576–81. doi: 10.1111/j.1540-8167.2005.40480.x
20. Ren JF, Marchlinski FE, Callans DJ, Gerstenfeld EP, Dixit S, Lin D, et al. Increased intensity of anticoagulation may reduce risk of thrombus during atrial fibrillation ablation procedures in patients with spontaneous echo contrast. J Cardiovasc Electrophysiol. (2005) 16:474–7. doi: 10.1046/j.1540-8167.2005.40465.x
21. Benali K, Verain J, Hammache N, Guenancia C, Hooks D, Magnin-Poull I, et al. Running after activated clotting time values in patients receiving direct oral anticoagulants: a potentially dangerous race. Results from a prospective study in atrial fibrillation catheter ablation procedures. J Clin Med. (2021) 10:4240. doi: 10.3390/jcm10184240
22. Nagao T, Inden Y, Yanagisawa S, Kato H, Ishikawa S, Okumura S, et al. Differences in activated clotting time among uninterrupted anticoagulants during the periprocedural period of atrial fibrillation ablation. Heart Rhythm. (2015) 12:1972–8. doi: 10.1016/j.hrthm.2015.04.016
23. Konduru SV, Cheema AA, Jones P, Li Y, Ramza B, Wimmer AP. Differences in intraprocedural ACTs with standardized heparin dosing during catheter ablation for atrial fibrillation in patients treated with dabigatran vs. patients on uninterrupted warfarin. J Interv Card Electrophysiol. (2012) 35:277–84; discussion 84. doi: 10.1007/s10840-012-9719-9
24. Bin Abdulhak AA, Kennedy KF, Gupta S, Giocondo M, Ramza B, Wimmer AP. Effect of pre-procedural interrupted apixaban on heparin anticoagulation during catheter ablation for atrial fibrillation: a prospective observational study. J Interv Card Electrophysiol. (2015) 44:91–6. doi: 10.1007/s10840-015-0048-7
25. Zeljkovic I, Brusich S, Scherr D, Velagic V, Traykov V, Pernat A, et al. Differences in activated clotting time and total unfractionated heparin dose during pulmonary vein isolation in patients on different anticoagulation therapy. Clin Cardiol. (2021) 44:1177–82. doi: 10.1002/clc.23681
26. Dillier R, Ammar S, Hessling G, Kaess B, Pavaci H, Buiatti A, et al. Safety of continuous periprocedural rivaroxaban for patients undergoing left atrial catheter ablation procedures. Circ Arrhythm Electrophysiol. (2014) 7:576–82. doi: 10.1161/CIRCEP.114.001586
27. Bassiouny M, Saliba W, Rickard J, Shao M, Sey A, Diab M, et al. Use of dabigatran for periprocedural anticoagulation in patients undergoing catheter ablation for atrial fibrillation. Circ Arrhythm Electrophysiol. (2013) 6:460–6. doi: 10.1161/CIRCEP.113.000320
28. Martin AC, Kyheng M, Foissaud V, Duhamel A, Marijon E, Susen S, et al. Activated clotting time monitoring during atrial fibrillation catheter ablation: does the anticoagulant matter? J Clin Med. (2020) 9:350. doi: 10.3390/jcm9020350
29. Weitz JI, Healey JS, Skanes AC, Verma A. Periprocedural management of new oral anticoagulants in patients undergoing atrial fibrillation ablation. Circulation. (2014) 129:1688–94. doi: 10.1161/CIRCULATIONAHA.113.005376
30. Martin AC, Godier A, Narayanan K, Smadja DM, Marijon E. Management of intraprocedural anticoagulation in patients on non-vitamin k antagonist oral anticoagulants undergoing catheter ablation for atrial fibrillation: understanding the gaps in evidence. Circulation. (2018) 138:627–33. doi: 10.1161/CIRCULATIONAHA.117.033326
31. Khairy P, Chauvet P, Lehmann J, Lambert J, Macle L, Tanguay JF, et al. Lower incidence of thrombus formation with cryoenergy versus radiofrequency catheter ablation. Circulation. (2003) 107:2045–50. doi: 10.1161/01.CIR.0000058706.82623.A1
32. Demolin JM, Eick OJ, Munch K, Koullick E, Nakagawa H, Wittkampf FH. Soft thrombus formation in radiofrequency catheter ablation. Pac Clin Electrophysiol. (2002) 25:1219–22. doi: 10.1046/j.1460-9592.2002.01219.x
33. Ren JF, Marchlinski FE, Callans DJ. Left atrial thrombus associated with ablation for atrial fibrillation: identification with intracardiac echocardiography. J Am Coll Cardiol. (2004) 43:1861–7. doi: 10.1016/j.jacc.2004.01.031
34. Maleki K, Mohammadi R, Hart D, Cotiga D, Farhat N, Steinberg JS. Intracardiac ultrasound detection of thrombus on transseptal sheath: incidence, treatment, and prevention. J Cardiovasc Electrophysiol. (2005) 16:561–5. doi: 10.1111/j.1540-8167.2005.40686.x
35. Bruce CJ, Friedman PA, Narayan O, Munger TM, Hammill SC, Packer DL, et al. Early heparinization decreases the incidence of left atrial thrombi detected by intracardiac echocardiography during radiofrequency ablation for atrial fibrillation. J Interv Card Electrophysiol. (2008) 22:211–9. doi: 10.1007/s10840-008-9270-x
36. Asbach S, Biermann J, Bode C, Faber TS. Early heparin administration reduces risk for left atrial thrombus formation during atrial fibrillation ablation procedures. Cardiol Res Pract. (2011) 2011:615087. doi: 10.4061/2011/615087
37. Lee DS, Dorian P, Downar E, Burns M, Yeo EL, Gold WL, et al. Thrombogenicity of radiofrequency ablation procedures: what factors influence thrombin generation? Europace. (2001) 3:195–200. doi: 10.1053/eupc.2001.0167
38. Dorbala S, Cohen AJ, Hutchinson LA, Menchavez-Tan E, Steinberg JS. Does radiofrequency ablation induce a prethrombotic state? Analysis of coagulation system activation and comparison to electrophysiologic study. J Cardiovasc Electrophysiol. (1998) 9:1152–60. doi: 10.1111/j.1540-8167.1998.tb00086.x
39. Parizek P, Haman L, Pleskot M, Pecka M, Bukac J, Stransky P, et al. Hemostatic changes before and during electrophysiologic study and radiofrequency catheter ablation. Int J Hematol. (2011) 93:452–7. doi: 10.1007/s12185-011-0806-y
40. Raaz U, Kaeberich A, Maegdefessel L, Buerke M, Busshardt M, Schubert S, et al. The direct thrombin inhibitor argatroban effectively prevents cardiac catheter thrombosis in vitro. Thromb Haemost. (2010) 103:808–14. doi: 10.1160/TH09-07-0456
41. Yau JW, Stafford AR, Liao P, Fredenburgh JC, Roberts R, Weitz JI. Mechanism of catheter thrombosis: comparison of the antithrombotic activities of fondaparinux, enoxaparin, and heparin in vitro and in vivo. Blood. (2011) 118:6667–74. doi: 10.1182/blood-2011-07-364141
42. Yau JW, Stafford AR, Liao P, Fredenburgh JC, Roberts R, Brash JL, et al. Corn trypsin inhibitor coating attenuates the prothrombotic properties of catheters in vitro and in vivo. Acta Biomater. (2012) 8:4092–100. doi: 10.1016/j.actbio.2012.07.019
43. Yau JW, Liao P, Fredenburgh JC, Roberts RS, Weitz JI. Only high levels of dabigatran attenuate catheter thrombosis in vitro and in rabbits. Thromb Haemost. (2014) 112:79–86. doi: 10.1160/TH13-12-1047
44. Kaeberich A, Raaz U, Vogt A, Maedgefessel L, Neuhart E, Krezel C, et al. In vitro comparison of the novel, dual-acting FIIa/FXa-inhibitor EP217609C101, unfractionated heparin, enoxaparin, and fondaparinux in preventing cardiac catheter thrombosis. J Thromb Thrombolysis. (2014) 37:118–30. doi: 10.1007/s11239-013-0938-4
45. Yau JW, Liao P, Fredenburgh JC, Stafford AR, Revenko AS, Monia BP, et al. Selective depletion of factor XI or factor XII with antisense oligonucleotides attenuates catheter thrombosis in rabbits. Blood. (2014) 123:2102–7. doi: 10.1182/blood-2013-12-540872
46. Pireaux V, Tassignon J, Demoulin S, Derochette S, Borenstein N, Ente A, et al. Anticoagulation with an inhibitor of factors XIa and XIIa during cardiopulmonary bypass. J Am Coll Cardiol. (2019) 74:2178–89. doi: 10.1016/j.jacc.2019.08.1028
47. Savage B, Ruggeri ZM. Selective recognition of adhesive sites in surface-bound fibrinogen by glycoprotein IIb-IIIa on nonactivated platelets. J Biol Chem. (1991) 266:11227–33. doi: 10.1016/S0021-9258(18)99152-0
48. Vroman L, Adams AL. Identification of rapid changes at plasma-solid interfaces. J Biomed Mater Res. (1969) 3:43–67. doi: 10.1002/jbm.820030106
49. Tsai WB, Grunkemeier JM, Horbett TA. Human plasma fibrinogen adsorption and platelet adhesion to polystyrene. J Biomed Mater Res. (1999) 44:130–9. doi: 10.1002/(SICI)1097-4636(199902)44:2<130::AID-JBM2>3.0.CO
50. Morley DJ, Feuerstein IA. Adhesion of polymorphonuclear leukocytes to protein-coated and platelet adherent surfaces. Thromb Haemost. (1989) 62:1023–8. doi: 10.1055/s-0038-1651046
51. Wright SD, Weitz JI, Huang AJ, Levin SM, Silverstein SC, Loike JD. Complement receptor type three (CD11b/CD18) of human polymorphonuclear leukocytes recognizes fibrinogen. Proc Natl Acad Sci USA. (1988) 85:7734–8. doi: 10.1073/pnas.85.20.7734
52. Gould TJ, Vu TT, Swystun LL, Dwivedi DJ, Mai SH, Weitz JI, et al. Neutrophil extracellular traps promote thrombin generation through platelet-dependent and platelet-independent mechanisms. Arterioscler Thromb Vasc Biol. (2014) 34:1977–84. doi: 10.1161/ATVBAHA.114.304114
53. Ghebrehiwet B, Randazzo BP, Dunn JT, Silverberg M, Kaplan AP. Mechanisms of activation of the classical pathway of complement by Hageman factor fragment. J Clin Invest. (1983) 71:1450–6. doi: 10.1172/JCI110898
54. DiScipio RG. The activation of the alternative pathway C3 convertase by human plasma kallikrein. Immunology. (1982) 45:587–95.
55. Huber-Lang M, Sarma JV, Zetoune FS, Rittirsch D, Neff TA, McGuire SR, et al. Generation of C5a in the absence of C3: a new complement activation pathway. Nat Med. (2006) 12:682–7. doi: 10.1038/nm1419
56. Durigutto P, Macor P, Pozzi N, Agostinis C, Bossi F, Meroni PL, et al. Complement activation and thrombin generation by MBL bound to beta2-glycoprotein I. J Immunol. (2020) 205:1385–92. doi: 10.4049/jimmunol.2000570
57. Polley MJ, Nachman RL. Human platelet activation by C3a and C3a des-arg. J Exp Med. (1983) 158:603–15. doi: 10.1084/jem.158.2.603
58. Toth NK, Csanadi Z, Hajas O, Kiss A, Nagy-Balo E, Kovacs KB, et al. Intracardiac hemostasis and fibrinolysis parameters in patients with atrial fibrillation. Biomed Res Int. (2017) 2017:3678017. doi: 10.1155/2017/3678017
59. Hajas O, Bagoly Z, Toth NK, Urbancsek R, Kiss A, Kovacs KB, et al. Intracardiac fibrinolysis and endothelium activation related to atrial fibrillation ablation with different techniques. Cardiol Res Pract. (2020) 2020:1570483. doi: 10.1155/2020/1570483
60. Beguin S, Dol F, Hemker HC. Factor IXa inhibition contributes to the heparin effect. Thromb Haemost. (1991) 66:306–9. doi: 10.1055/s-0038-1646412
61. Jaffer IH, Fredenburgh JC, Stafford A, Whitlock RP, Weitz JI. Rivaroxaban and dabigatran for suppression of mechanical heart valve-induced thrombin generation. Ann Thorac Surg. (2020) 110:582–90. doi: 10.1016/j.athoracsur.2019.10.091
62. Jaffer IH, Stafford AR, Fredenburgh JC, Whitlock RP, Chan NC, Weitz JI. Dabigatran is less effective than warfarin at attenuating mechanical heart valve-induced thrombin generation. J Am Heart Assoc. (2015) 4:e002322. doi: 10.1161/JAHA.115.002322
63. Edrich T, Frendl G, Michaud G, Paschalidis I. Heparin requirements for full anticoagulation are higher for patients on dabigatran than for those on warfarin - a model-based study. Clin Pharmacol. (2015) 7:19–27. doi: 10.2147/CPAA.S72185
64. Vitulli A, Dirienzo L, Mancazzo F, Dellanoce C, Paoletti O, Testa S, et al. Plasma from patients under direct oral anticoagulants (DOACs) displays a strong resistance to heparin compared to patients under vitamin K antagonists (VKA). Res Pract Thromb Haemost. (2020) 4(Suppl. 1):1–1311. doi: 10.1002/rth2.1239
65. Bosch YP, Ganushchak YM, de Jong DS. Comparison of ACT point-of-care measurements: repeatability and agreement. Perfusion. (2006) 21:27–31. doi: 10.1191/0267659106pf844oa
66. Nilsson S, Appelblad M, Svenmarker S. Can we rely on the activated clotting time to measure heparin anticoagulation? A clinical evaluation of two ACT monitors. J Extra Corpor Technol. (2020) 52:212−7. doi: 10.1182/ject-2000005
67. Li H, Serrick C, Rao V, Yip PM. A comparative analysis of four activated clotting time measurement devices in cardiac surgery with cardiopulmonary bypass. Perfusion. (2021) 36:610–9. doi: 10.1177/0267659120949351
68. Vranken NPA, Theelen A, Orbons T, Barenbrug PJC, Weerwind PW. Point-of-care measurement of kaolin activated clotting time during cardiopulmonary bypass: a single sample comparison between ACT plus and i-STAT. J Extra Corpor Technol. (2021) 53:57–61. doi: 10.1182/ject-2000046
69. Thenappan T, Swamy R, Shah A, Nathan S, Nichols J, Bond L, et al. Interchangeability of activated clotting time values across different point-of-care systems. Am J Cardiol. (2012) 109:1379–82. doi: 10.1016/j.amjcard.2011.12.033
70. Korpi-Steiner NL, Walz JM, Schanzer A, Rao LV. Comparison of point-of-care activated clotting time methods in different clinical settings in a large academic medical center. J Appl Lab Med. (2017) 2:356–66. doi: 10.1373/jalm.2017.024414
71. Dirkmann D, Nagy E, Britten MW, Peters J. Point-of-care measurement of activated clotting time for cardiac surgery as measured by the Hemochron signature elite and the Abbott i-STAT: agreement, concordance, and clinical reliability. BMC Anesthesiol. (2019) 19:174. doi: 10.1186/s12871-019-0846-z
72. Jobes DR, Ellison N, Campbell FW. Limit(ation)s for ACT. Anesth Analg. (1989) 69:142–4. doi: 10.1213/00000539-198907000-00037
73. Lasne D, Bauters A, Le Querrec A, Bourdin C, Voisin S, Societe Francaise de Biologie C, et al. [Guidelines for certification of activated clotting time (ACT) according to the EN ISO 22870 standards]. Ann Biol Clin. (2015) 73:225–54. doi: 10.1684/abc.2015.1035
74. Despotis GJ, Alsoufiev AL, Spitznagel E, Goodnough LT, Lappas DG. Response of kaolin ACT to heparin: evaluation with an automated assay and higher heparin doses. Ann Thorac Surg. (1996) 61:795–9. doi: 10.1016/0003-4975(95)00821-7
75. Marmur JD, Anand SX, Bagga RS, Fareed J, Pan CM, Sharma SK, et al. The activated clotting time can be used to monitor the low molecular weight heparin dalteparin after intravenous administration. J Am Coll Cardiol. (2003) 41:394–402. doi: 10.1016/S0735-1097(02)02762-6
76. Dieplinger B, Egger M, Luft C, Hinterreiter F, Pernerstorfer T, Haltmayer M, et al. Comparison between activated clotting time and anti-activated factor X activity for the monitoring of unfractionated heparin therapy in patients with aortic aneurysm undergoing an endovascular procedure. J Vasc Surg. (2018) 68:400–7. doi: 10.1016/j.jvs.2017.11.079
77. Dincq AS, Lessire S, Chatelain B, Gourdin M, Dogne JM, Mullier F, et al. Impact of the direct oral anticoagulants on activated clotting time. J Cardiothorac Vasc Anesth. (2017) 31:e24–7. doi: 10.1053/j.jvca.2016.09.002
78. Godier A, Dincq AS, Martin AC, Radu A, Leblanc I, Antona M, et al. Predictors of pre-procedural concentrations of direct oral anticoagulants: a prospective multicentre study. Eur Heart J. (2017) 38:2431–9. doi: 10.1093/eurheartj/ehx403
79. Sairaku A, Nakano Y, Onohara Y, Hironobe N, Matsumura H, Shimizu W, et al. Residual anticoagulation activity in atrial fibrillation patients with temporary interrupted direct oral anticoagulants: comparisons across 4 drugs. Thromb Res. (2019) 183:119–23. doi: 10.1016/j.thromres.2019.10.006
80. Wu S, Yang YM, Zhu J, Wan HB, Wang J, Zhang H, et al. Meta-analysis of efficacy and safety of new oral anticoagulants compared with uninterrupted vitamin K antagonists in patients undergoing catheter ablation for atrial fibrillation. Am J Cardiol. (2016) 117:926–34. doi: 10.1016/j.amjcard.2015.12.027
81. Brockmeyer M, Lin Y, Parco C, Karathanos A, Krieger T, Schulze V, et al. Uninterrupted anticoagulation during catheter ablation for atrial fibrillation: no difference in major bleeding and stroke between direct oral anticoagulants and vitamin K antagonists in an updated meta-analysis of randomised controlled trials. Acta Cardiol. (2021) 76:288–95. doi: 10.1080/00015385.2020.1724689
82. Grover SP, Mackman N. Intrinsic pathway of coagulation and thrombosis. Arterioscler Thromb Vasc Biol. (2019) 39:331–8. doi: 10.1161/ATVBAHA.118.312130
83. Naito N, Ukita R, Wilbs J, Wu K, Lin X, Carleton NM, et al. Combination of polycarboxybetaine coating and factor XII inhibitor reduces clot formation while preserving normal tissue coagulation during extracorporeal life support. Biomaterials. (2021) 272:120778. doi: 10.1016/j.biomaterials.2021.120778
84. Larsson M, Rayzman V, Nolte MW, Nickel KF, Bjorkqvist J, Jamsa A, et al. A factor XIIa inhibitory antibody provides thromboprotection in extracorporeal circulation without increasing bleeding risk. Sci Transl Med. (2014) 6:222ra17. doi: 10.1126/scitranslmed.3006804
85. Buller HR, Bethune C, Bhanot S, Gailani D, Monia BP, Raskob GE, et al. Factor XI antisense oligonucleotide for prevention of venous thrombosis. N Engl J Med. (2015) 372:232–40. doi: 10.1056/NEJMoa1405760
86. Holmer E, Kurachi K, Soderstrom G. The molecular-weight dependence of the rate-enhancing effect of heparin on the inhibition of thrombin, factor Xa, factor IXa, factor XIa, factor XIIa and kallikrein by antithrombin. Biochem J. (1981) 193:395–400. doi: 10.1042/bj1930395
87. Rezaie AR. Prothrombin protects factor Xa in the prothrombinase complex from inhibition by the heparin-antithrombin complex. Blood. (2001) 97:2308–13. doi: 10.1182/blood.V97.8.2308
88. Brufatto N, Ward A, Nesheim ME. Factor Xa is highly protected from antithrombin-fondaparinux and antithrombin-enoxaparin when incorporated into the prothrombinase complex. J Thromb Haemost. (2003) 1:1258–63. doi: 10.1046/j.1538-7836.2003.00254.x
89. van Ryn J, Hauel N, Waldmann L, Wienen W. Dabigatran inhibits both clot-bound and fluid phase thrombin in vitro: effects compared to heparin and hirudin. Blood. (2007) 110:3998. doi: 10.1182/blood.V110.11.3998.3998
90. Depasse F, Busson J, Mnich J, Le Flem L, Gerotziafas G, Samama M. Effect of BAY 59-7939 – a novel, oral, direct factor Xa inhibitor – on clot-bound factor Xa activity in vitro [Abstract no. P1104]. J Thromb Haemost. (2005) 3.
91. Perzborn E, Strassburger J, Wilmen A, Pohlmann J, Roehrig S, Schlemmer KH, et al. In vitro and in vivo studies of the novel antithrombotic agent BAY 59-7939–an oral, direct Factor Xa inhibitor. J Thromb Haemost. (2005) 3:514–21. doi: 10.1111/j.1538-7836.2005.01166.x
Keywords: atrial fibrillation, catheter ablation, direct oral anticoagulant, unfractionated heparin, activated clotting time
Citation: Hardy M, Douxfils J, Dincq A-S, Sennesael A-L, Xhaet O, Mullier F and Lessire S (2022) Uninterrupted DOACs Approach for Catheter Ablation of Atrial Fibrillation: Do DOACs Levels Matter? Front. Cardiovasc. Med. 9:864899. doi: 10.3389/fcvm.2022.864899
Received: 29 January 2022; Accepted: 28 February 2022;
Published: 29 March 2022.
Edited by:
Roberto Pola, Università Cattolica del Sacro Cuore, ItalyReviewed by:
Zsuzsa Bagoly, University of Debrecen, HungaryCopyright © 2022 Hardy, Douxfils, Dincq, Sennesael, Xhaet, Mullier and Lessire. This is an open-access article distributed under the terms of the Creative Commons Attribution License (CC BY). The use, distribution or reproduction in other forums is permitted, provided the original author(s) and the copyright owner(s) are credited and that the original publication in this journal is cited, in accordance with accepted academic practice. No use, distribution or reproduction is permitted which does not comply with these terms.
*Correspondence: Michael Hardy, bWljaGFlbC5oYXJkeUB1Y2xvdXZhaW4uYmU=
†These authors have contributed equally to this work
Disclaimer: All claims expressed in this article are solely those of the authors and do not necessarily represent those of their affiliated organizations, or those of the publisher, the editors and the reviewers. Any product that may be evaluated in this article or claim that may be made by its manufacturer is not guaranteed or endorsed by the publisher.
Research integrity at Frontiers
Learn more about the work of our research integrity team to safeguard the quality of each article we publish.