- 1Univ Lyon, CarMeN Laboratory, INSERM, INRA, INSA Lyon, Université Claude Bernard Lyon 1, Lyon, France
- 2Olea Medical, La Ciotat, France
- 3Grenoble Institut Neurosciences, INSERM, U1216, Univ. Grenoble Alpes, Grenoble, France
- 4CREATIS, CNRS UMR-5220, INSERM U1206, Université Lyon 1, Villeurbanne, France
- 5Neuroradiology Department, Hospices Civils of Lyon, Lyon, France
- 6Stroke Department, Hospices Civils of Lyon, Lyon, France
The ischemic penumbra is defined as the severely hypoperfused, functionally impaired, at-risk but not yet infarcted tissue that will be progressively recruited into the infarct core. Early reperfusion aims to save the ischemic penumbra by preventing infarct core expansion and is the mainstay of acute ischemic stroke therapy. Intravenous thrombolysis and mechanical thrombectomy for selected patients with large vessel occlusion has been shown to improve functional outcome. Given the varying speed of infarct core progression among individuals, a therapeutic window tailored to each patient has recently been proposed. Recent studies have demonstrated that reperfusion therapies are beneficial in patients with a persistent ischemic penumbra, beyond conventional time windows. As a result, mapping the penumbra has become crucial in emergency settings for guiding personalized therapy. The penumbra was first characterized as an area with a reduced cerebral blood flow, increased oxygen extraction fraction and preserved cerebral metabolic rate of oxygen using positron emission tomography (PET) with radiolabeled O2. Because this imaging method is not feasible in an acute clinical setting, the magnetic resonance imaging (MRI) mismatch between perfusion-weighted imaging and diffusion-weighted imaging, as well as computed tomography perfusion have been proposed as surrogate markers to identify the penumbra in acute ischemic stroke patients. Transversal studies comparing PET and MRI or using longitudinal assessment of a limited sample of patients have been used to define perfusion thresholds. However, in the era of mechanical thrombectomy, these thresholds are debatable. Using various MRI methods, the original penumbra definition has recently gained a significant interest. The aim of this review is to provide an overview of the evolution of the ischemic penumbra imaging methods, including their respective strengths and limitations, as well as to map the current intellectual structure of the field using bibliometric analysis and explore future directions.
1. Introduction
Stroke is the second most common cause of death and a leading cause of disability in occidental countries, and the 20- to 30-year projection forecasts around 20–30% increase of its burden (1, 2).
In case of acute ischemic stroke (AIS), occlusion of a cerebral blood vessel causes a variable decrease of blood flow in the downstream parenchyma. Three major zones have been identified: (i) the irreversibly damaged ischemic core; (ii) the ischemic penumbra, defined as the severely hypoperfused, electrically silent, at risk brain tissue; and (iii) the oligemia, a mildly hypoperfused area with preserved neuronal function (3, 4). As time elapses, the core progresses within the ischemic penumbra, but this area can be salvaged if perfusion is restored (5).
Therefore, the primary target of AIS treatment is to restore brain perfusion as soon as possible in order to preserve the ischemic penumbra. Reperfusion therapies, such as intravenous (IV) thrombolysis with recombinant tissue plasminogen activator (rt-PA) and mechanical thrombectomy (MT) for selected patients with large vessel occlusion (LVO), have been shown to improve functional outcome within a time window of 4.5 and 6 h, respectively (6–8).
Strict time windows, however, have been questioned as the infarct core progresses at different rates across individuals. The primary pathophysiological variable distinguishing “fast progressors” from “slow progressors” is the collateral status, and particularly the functionality of the leptomeningeal anastomoses (9). Therefore, a growing paradigm has been the shift from a “time-based” to a “tissue-based” approach, thus using a therapeutic window tailored to each patient's unique pathophysiology. Recent clinical trials that included penumbral imaging in their eligibility criteria demonstrated the benefit of reperfusion therapy beyond conventional time windows of up to 9 h for IV thrombolysis and up to 24 h for MT (10). Mapping the ischemic penumbra has therefore become critical when managing AIS patients in order to identify those who are the most amenable to reperfusion strategies beyond conventional time windows (11, 12).
The aim of this review is to provide an overview of the ischemic penumbra imaging methods from positron emission tomography (PET) imaging, the historical one, to multiparametric magnetic resonance imaging (MRI) and computed tomography (CT) that can be used as operational surrogate in AIS to guide therapeutic decisions. Using co-citation analysis, a bibliometric analysis of the field was carried out to observe the emergence of these methods. Additionally, the bibliographic coupling focusing on the MT era sketches the current state of research in order to position future contributions to the field.
2. [15O]-PET: The Gold Standard of Ischemic Penumbra Imaging
Initially, the ischemic penumbra was identified in baboons as severely hypoperfused and electrically silent tissue but without massive release of extracellular potassium. This definition relies on the measured electrical response as well as pH and potassium variation throughout the occlusion time (13, 14). [15O]-PET imaging on baboons validated the existence of the ischemic penumbra with metabolic parameters (3, 15). The transition from electrical activity to imaging parameters established a first shift of paradigm in the field (16).
The existence of the ischemic penumbra in humans was demonstrated using PET imaging 40 years ago and confirmed the data obtained in baboons (17). The mapping of cerebral blood flow (CBF) and O2 metabolism biomarkers such as the oxygen extraction fraction (OEF) and the cerebral metabolic rate of oxygen (CMRO2) is obtained through a series of PET acquisitions following inhalation and injection of molecules labeled with15O radioactive tracer. The steady state method was first implemented, and consists in maintaining the tracer's concentration in the blood to a constant level throughout the acquisition period. This method was replaced by a bolus injection and inhalation method that drastically reduced the radioactive doses required for image acquisition (18). Two acquisitions are required in the imaging pipeline to obtain the CMRO2 parameter map (19). The injection of a labeled water ([15O]H2O) bolus provides CBF mapping. The time activity curve following the injection enables the numerical calculation of the image-derived arterial input function (IDIF) and may prevent invasive procedures to obtain the arterial blood curves (20). The second bolus acquisition is performed with an inhaled [15O]O2 tracer. Oxygen extraction from capillaries to cerebral tissues is modeled as a single-tissue compartment kinetic model providing CMRO2 mapping (21). These acquisitions are then combined to compute the OEF mapping as described mathematically by Kudomi et al. (20). The post-processing pipeline for metabolic penumbra imaging with [15O]-PET is described in Figure 1 (22).
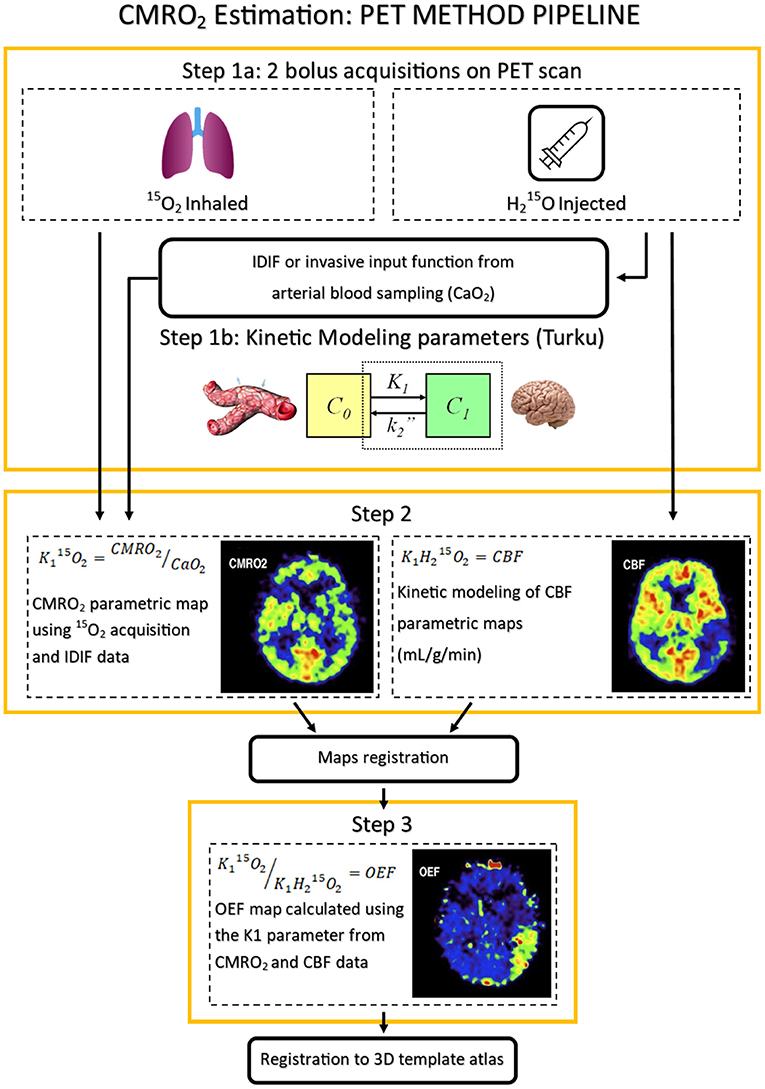
Figure 1. Oxygen metabolism [15O]-Positron Emission Tomography (PET) imaging pipeline. PET images published in JNM. Heiss WD. Radionuclide imaging in ischemic stroke. J Nucl Med. (2014) 55:1831–41. © SNMMI. CMRO2, cerebral metabolic rate of oxygen; IDIF, image-derived input function; CaO2, arterial blood oxygen content; VOI, volume of interest; CBF, cerebral blood flow; OEF, oxygen extraction fraction; K1, tracer delivery rate.
The relevance of the cerebral oxygen metabolism in predicting tissue outcome in ischemic stroke patients was demonstrated using [15O]-PET imaging (23). CBF distinguished penumbra (CBF 8-20 mL/100g/min) from ischemic core (CBF <8 mL/100g/min) and oligemia (CBF 20-50 mL/100g/min) (24, 25). Although the OEF was identified as a key factor in evaluating the transition from ischemic to infarcted tissues (26), CMRO2 was found to be the most accurate predictive parameter (27). Infarcted regions had a significant drop of CMRO2 below the threshold of 1.4 mL/100g/min whereas viable tissues were characterized by a maintained oxygen metabolism level above that threshold. The ischemic penumbra PET imaging pattern, initially called “misery perfusion”, is therefore characterized as a hypoperfused region (CBF 8–20 mL/100g/min) with increased OEF and relatively preserved CMRO2 (CMRO2 ≥ 1.4 mL/100g/min) (28). These thresholds were established through longitudinal studies in AIS patients prior to any reperfusion therapy. Few data are available in the setting of IV thrombolysis (29).
[15O]-PET has been fundamental in demonstrating the existence of the ischemic penumbra in humans, thus driving the development of reperfusion therapies. However, it is impractical in the emergency setting and exposes patients to high doses of radioactivity (18).
Notably, two other PET radiotracers have also been proposed as markers of neuronal integrity: (i) 11C-flumazenil (FMZ) a ligand selective for the central benzodiazepine receptor, whose binding is reduced in irreversible tissue damage (30, 31); (ii) (18)F-misonidazole (FMISO) which is trapped within hypoxic cells but is not specific to the penumbra (32). Although they are both straightforward PET radiotracers compared to 15O, their use is not extended to the AIS emergency setting.
Alternatively, single photon emission computed tomography (SPECT) using 99mTc-HMPAO (99m-Technetium hexamethylpropyleneamineoxime) or ECD (99mTc-ethyl-cysteinate-dimer) also provides data on perfusion in brain tissue. Although relative thresholds of CBF reduction have been proposed, the combination of SPECT and diffusion-weighted MRI seems more accurate to distinguish the core from the penumbra (33–36). However, SPECT can not be used in clinical emergency setting.
3. Evolution of Penumbra Imaging in Clinical Emergency Settings
Reperfusion therapies, such as IV thrombolysis with recombinant tissue plasminogen activator (rt-PA) and mechanical thrombectomy (MT) for selected patients with large vessel occlusion (LVO), have been shown to improve clinical outcome in AIS patients. While penumbra imaging is not formally required for patient's eligibility in early time windows, it has been proposed for the selection of patients who are the most amenable to reperfusion strategies in later time windows. Operational penumbra imaging methods, that are feasible in emergency setting, are required for this purpose. In this context, CT and MRI modalities have been introduced to estimate the penumbra in clinical routine (37, 38).
Hemodynamic parameters maps can be computed using CT or MR perfusion imaging. Perfusion data processing requires the manual or automatic selection of an arterial input function, which is used for the deconvolution of each voxel's concentration time curve. Deconvolution can be done using a variety of techniques, ranging from the classical singular value decomposition (SVD)-based algorithms (39) to more advanced Bayesian approaches (40). Perfusion parameters such as the cerebral blood flow (CBF), cerebral blood volume (CBV), mean transit time (MTT), time to peak (TTP), time to maximum (Tmax), or the arterial delay can be used to distinguish tissue with a perfusion deficit from the infarct core and the normal tissue. Furthermore, the apparent diffusion coefficient (ADC) parameter derived from diffusion weighted MRI (DWI) is an excellent surrogate for detecting the infarct core (41, 42). A new definition based on the mismatch between infarct core and the tissue with a perfusion deficit provides the perfusion penumbra as opposed to the originally defined metabolic penumbra obtained with [15O]-PET. A parallel between these two penumbra can be found on Figure 2 (22).
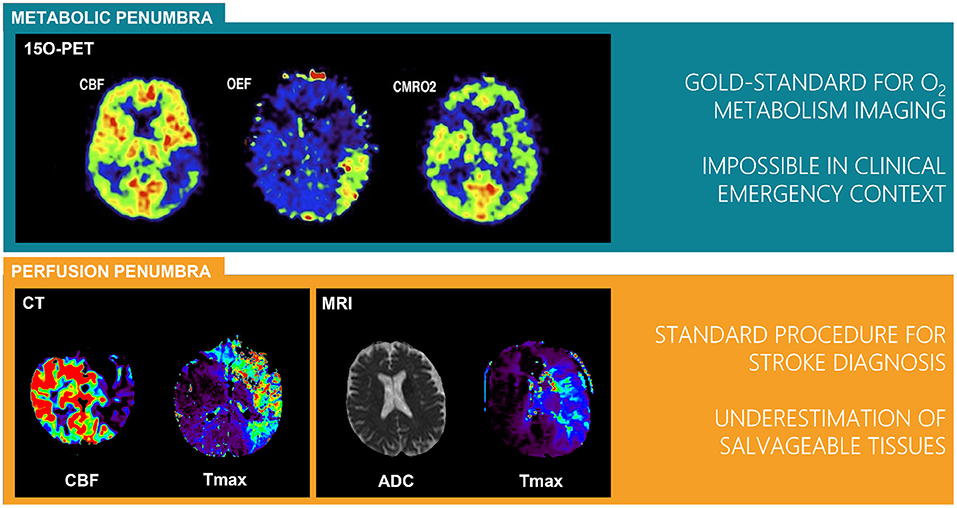
Figure 2. Definitions of perfusion and metabolic penumbra from the gold standard [15O]-Positron Emission Tomography (PET) to the widely distributed Computed Tomography (CT) and Magnetic Resonance Imaging (MRI) perfusion imaging. PET images published in JNM. Heiss WD. Radionuclide imaging in ischemic stroke. J Nucl Med. (2014) 55:1831–41. © SNMMI.; CT and MRI images from Olea Medical. CBF, cerebral blood flow; CMRO2, cerebral metabolic rate of oxygen; OEF, oxygen extraction fraction; ADC, apparent diffusion coefficient; Tmax, time to maximum of residual function.
To differentiate the penumbra from the oligemia, perfusion thresholds were initially defined using relative CBF and CBV parameters opposed to the contralateral control region (CCR) (38, 43). With the development of deconvolution methods, a longitudinal study conducted in a small sample of patients treated with IV thrombolysis has shown that a Tmax > 6s provides an accurate estimate of critically hypoperfused tissue in MRI (44); the same threshold was later approved for CT. This threshold is commonly used to obtain the perfusion penumbra by subtracting the lesion core. This definition has been used as a criteria for selecting patients in clinical trials using the RAPID software (iSchemaView Inc., Menlo Park, CA) for image processing (11).
The lesion core definition, on the other hand, differs between the two imaging modalities. In MRI, the DWI-derived ADC parameter distinguishes the core with a threshold of ADC <0.62 × 10−3mm2\s (45). However, methods to identify the lesion core on ADC maps may vary and semi-automated as well as manual methods are still favored in clinical settings. In contrast, with CT imaging, the CBF parameter derived from perfusion acquisition defines the core in opposition to the CCR (CBF <30% of CCR) (46). Figure 3 summarizes the pipeline of both modalities.
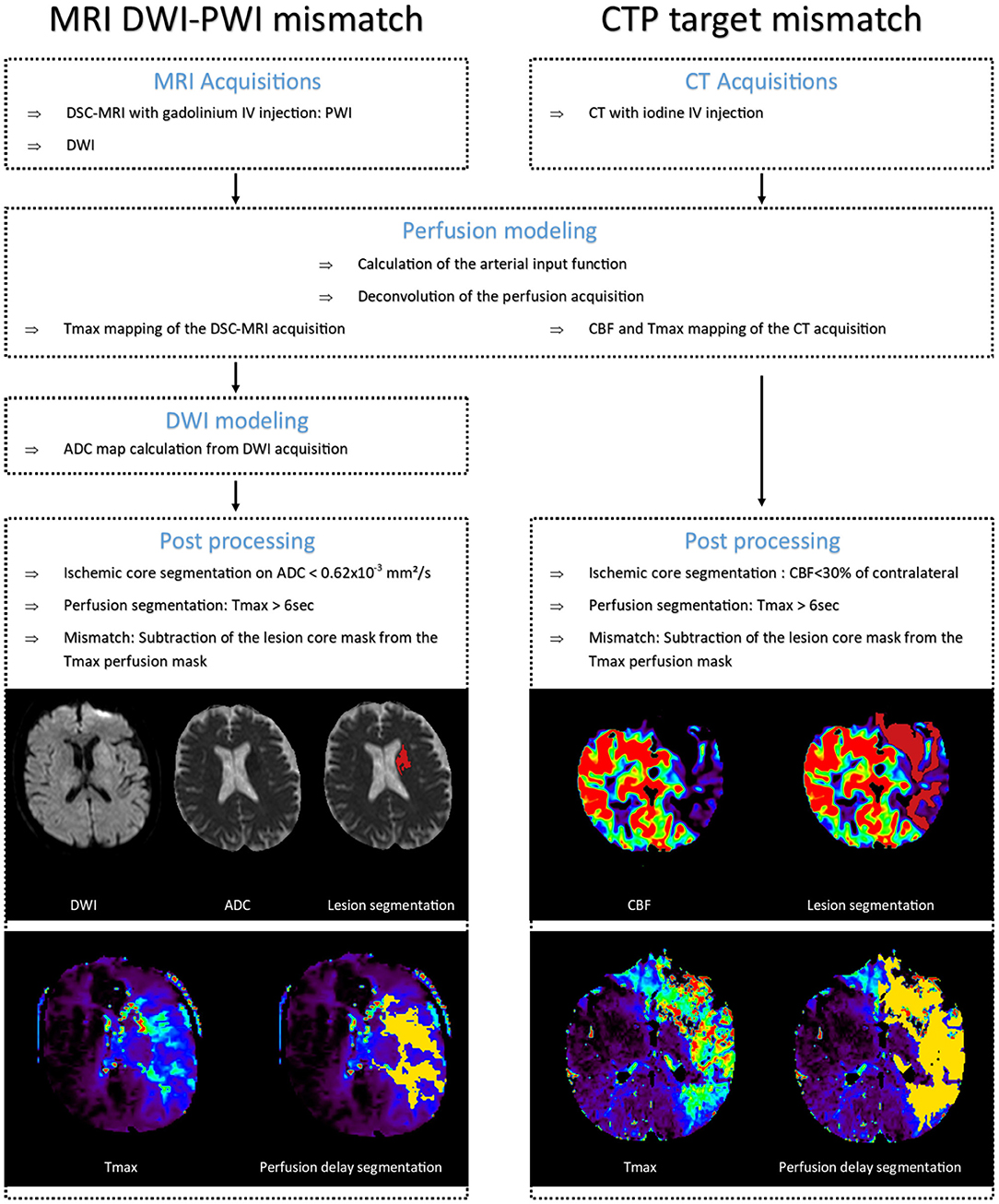
Figure 3. CT and MRI pipelines. In dark red: segmented infarct core. In yellow: segmented perfusion delay on Tmax. Images from Olea Medical. MRI, magnetic resonance imaging; DWI, diffusion weighted imaging; PWI, perfusion weighted imaging; CTP, computed tomography perfusion; DSC, dynamic susceptibility contrast; IV, intravenous bolus; CBF, cerebral blood flow; Tmax, time to maximum of residual function; ADC, apparent diffusion coefficient.
CT and MRI have been used in clinical trials to select patients with persistent penumbra in order to assess the benefit of reperfusion therapies in later time windows. These trials have demonstrated that reperfusion therapies benefit these selected patients for up to 9 h for intravenous (IV) thrombolysis (10) and up to 16–24 h for MT (11, 12). The shift from a “time-based” to a “tissue-based” approach has significantly improved stroke management by extending therapeutic windows in patients with persistent penumbra, i.e. those with a good collateral status (47, 48). CT and MRI are therefore used in daily practice to guide therapy in patients managed beyond the conventional time window. While clinical trials used RAPID or Olea Sphere with above-mentioned thresholds for image processing, other post-processing software (Philips, Siemens, Vitrea) have been developed with alternative perfusion imaging thresholds adapted to the deconvolution method. However comparative studies have shown significant differences in their predictive accuracy of final infarct volume in the setting of MT (49, 50).
This historical association between imaging and AIS management was followed by a fast evolution of therapeutic and imaging methods in the field of AIS care. Figure 4 illustrates the timeline with the major evolutions and key-factors.
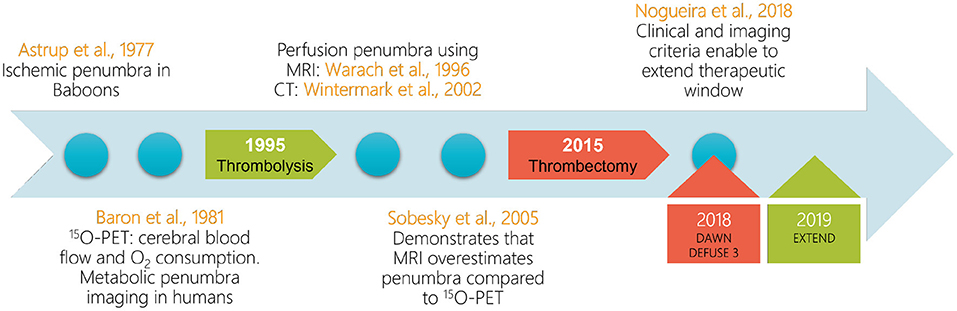
Figure 4. Summarized development timeline of the penumbra imaging field. Circles: the major contributions to the acute ischemic stroke research field. Arrows: the clinical validation years for reperfusion therapies. Rectangles: the major clinical trials using advanced imaging to extend therapeutic windows. Red and green code for mechanical thrombectomy and intravenous thrombolysis clinical trials, respectively. PET, positron emission tomography; MRI, magnetic resonance imaging; CT, computed tomography.
The scientific literature review of this field is a very challenging task as there are over 1000 articles on penumbra imaging in scientific databases, with about 50% of these articles published since 2015, in the era of MT. Therefore, in terms of data quantity, bibliometric analysis was a relevant approach to provide an overview of the field and an insight into the knowledge of the thrombectomy era. The bibliometric analysis was carried out in accordance with the methodology recommendations of Donthu et al. (51) and the material and methods used for this analysis can be found in Supplementary Material.
The co-citation analysis is a bibliometric method that calculates how frequently two articles are cited together. It uncovers fundamental publications and enables a mapping of the field's foundation knowledge and evolutions. The analysis included the 75 most co-cited documents. This threshold increased the network's legibility and highlighted the most important contributions. The resulting network can be found in Figure 5 where cluster denomination is based on predominance of the topic.
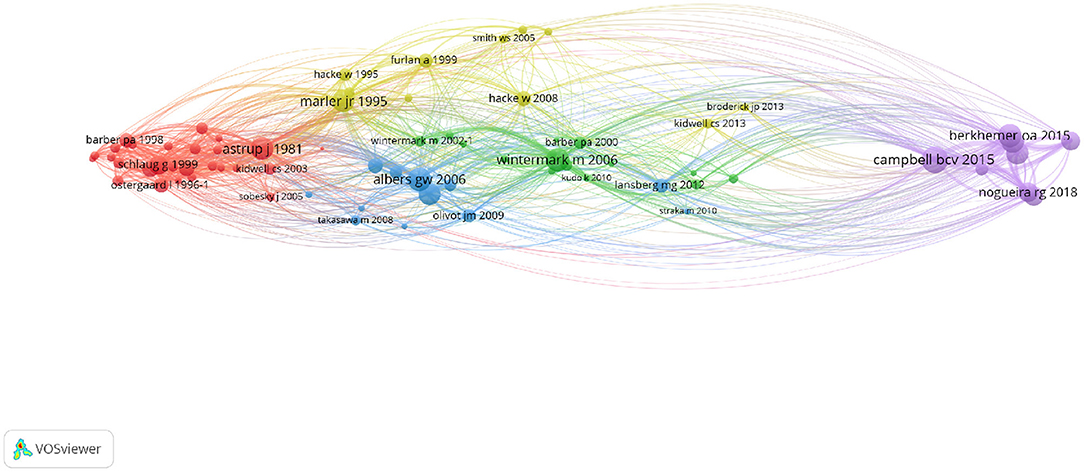
Figure 5. Co-citation network providing an overview of the acute ischemic stroke research field evolutions through its most contributing publications: Red cluster [1981–2005]: MRI diffusion-perfusion mismatch; Green cluster [2000–2013]: perfusion CT; Blue cluster [2003–2012]: Eligibility for thrombolysis; Yellow cluster [1995–2013]: Thrombolysis combined to endovascular therapies; Purple cluster [2015–2018]: Mechanical thrombectomy. MRI, magnetic resonance imaging; CT, computed tomography.
The applied mapping method retraces the timeline developed in Figure 4. Thus, the query defined for data collection (described in Supplementary Material) is relevant as the fundamental knowledge of the field obtained through co-citation analysis is similar to the current understanding of the field. The resulting clusters reveal the main research topics in the field as well as their interdependence. These observations, along with the geographical position (physical location within the network) of the various network elements, provide additional interpretation material.
While the majority of the articles in the red cluster relates to MRI sequences to evaluate lesion core and perfusion delay mismatch (16/27 articles), it also includes articles about threshold and biomarkers of penumbral tissues using PET, CT, and MRI. These articles represent 10/27 documents within this cluster. This cluster also includes the first publication defining the ischemic penumbra (3). The detailed exploration of the content enables the following elaborations: this cluster contains all major articles on penumbra definition from the theory to the first clinical applications through new thresholds definitions and comparison to [15O]-PET imaging. Several studies have compared MRI to [15O]-PET back-to-back in ischemic stroke patients and demonstrated that MRI overestimated the penumbra (52, 53). Two major limitations for MRI definition of the penumbra have been identified: (i) ADC reduction is considered to be a reflection of the ischemic core but a part of the ADC lesion may reverse (54); (ii) Distinguishing perfusion deficit from oligemia is challenging and several thresholds have been proposed, as previously demonstrated.
All of the articles in the green cluster are focused on CT imaging. Perfusion CT and associated thresholds are covered in 10/16 publications. 3 articles elaborate on other CT imaging modalities [Dynamic CT perfusion (46) and CT angiography (55)]. 2 publications relate to the ASPECTS clinical trial (56, 57). Finally, one publication compares CT perfusion method to MRI diffusion-perfusion mismatch (58). This cluster is centered on CT imaging methods in AIS management from the universally accepted scoring system to specific methods to evaluate ischemic tissue perfusion. Because of its rapid feasibility and widespread availability, CT has been the first-line imaging procedure in a majority of countries worldwide. In the 16 major clinical trials conducted since 2012, while 50% based their eligibility criteria on either CT or MR imaging, 43.75% were focused only on CT and 6.25% only on MRI (59, 60). This reflects the use of CT as the primary imaging modality for AIS in most stroke units in daily practice due to high availability, lack of contraindications, and reduced scan-duration. However, MRI is highly feasible in AIS setting, offers additional information regarding the tissue state and prevents from radiation exposure without introducing longer delay compared with the CT-selected patients and may improve outcome despite the potential delays in workflow time metrics (61–65).
The blue cluster contains a majority of articles related to thrombolytic therapies (7/12). Within this subcluster, 5 articles are related to clinical trials that aimed to extend therapeutic time windows [DIAS (66), DIAS-2 (67) and EPITHET (68)] or identifying MRI findings of patients who are likely to benefit from reperfusion therapies in later time window [DEFUSE (69, 70)]. In addition, 3 articles are focused on the post-processing MRI sequences: (i) The reference article on SVD deconvolution for MR perfusion (39); (ii) A retrospective analysis of the EPITHET clinical trial aiming to define a standardized MRI procedure for perfusion-diffusion mismatch (71); (iii) The RAPID solution for automating the task (72). Notably, two publications aimed to compare MRI and PET imaging of the ischemic penumbra (73, 74). Thus, this cluster introduces reperfusion therapy along with clinical trials aiming to increase therapeutic windows as well as imaging methods for selecting patients.
The yellow cluster relates to reperfusion therapies and is almost evenly split between IV thrombolysis (6/11) and MT (4/11) as well as combined methods. Three publications are related to IV thrombolysis clinical trials [PROACT II (75), ECASS II (76), ATLANTIS, and NINDS (77)]. The 4 articles related to MT refer to clinical trials for clot retriever devices (78–80) and imaging eligibility criteria (81). This cluster demonstrates the connection between the two treatments, frequently combined in clinical settings (82).
The last cluster, in purple, is centered on thrombectomy with a majority of publications referring to the major clinical trials demonstrating the benefit of MT and extending the therapeutic window with two subclusters in 2015(5/9) and 2018(2/9). In 2015, MR CLEAN was the first to demonstrate that MT outperformed IV thrombolysis alone in patients within 6 h of last known normal with LVO (83). Five other clinical trials [EXTEND IA (84), SWIFT PRIME (85), REVASCAT (86), ESCAPE (87), THRACE (88)] published in 2015 and 2016 and conducted in selected patients up to 12 h from stroke onset have confirmed these results. Among these clinical trials, 4 (EXTEND IA, SWIFT PRIME, REVASCAT, ESCAPE) included advanced imaging to select patients with limited core or good collateral circulation. In 2016, a meta-analysis of individual patient data enrolled in the 5 first clinical trials showed that MT reduced disability at 3 months with a number needed to treat 5 to prevent 1 patient from experiencing disability (8). Therefore, AHA guidelines, also found in this cluster (89), recommended to treat AIS patients with LVO and ASPECTS1≥6, although the role of additional imaging-based eligibility criteria is not well-established (90). In 2018, DEFUSE 3 and DAWN extended the therapeutic window to 16 and 24 h from time last known well, respectively, in highly selected patients with persistent penumbra (11, 12). DEFUSE 3 defined the penumbra with advanced imaging, CT or MRI, whereas DAWN defined the penumbra as a mismatch between the severity of the clinical deficit and the infarct volume. This last cluster highlights the key role of penumbra imaging in recent clinical trials that improved AIS management. Moreover, additional elaborations are possible knowing that 50% of the articles in this field were published since 2015, it demonstrates the major impact of these clinical trials on further research.
The detailed analysis of each cluster reveals a general trend that is shared by all clusters: the interdependence of imaging assessment, clinical trials and therapeutic solutions and windows. The recurrent presence of PET comparison with other imaging methods, on the other hand, is representative of the ongoing reassessment of relevance for clinical alternatives to penumbra imaging. The mapping obtained with the co-citation analysis is representative of the intellectual structure of the field. Furthermore, this analysis demonstrates the major publications and fundamental knowledge that serve as foundation for research in the thrombectomy era.
4. Penumbra Imaging in the Thrombectomy Era
The impact of MT and associated clinical trials on research for AIS management was compelling as in the course of 6 years over 500 articles were published on the topic (query described in Supplementary Material). The bibliographic coupling method was chosen in order to review this large corpus of articles.
The bibliographic coupling is the process of associating articles based on the number of references they share (91). This analysis is based on the hypothesis that articles sharing a large number of common references have similar content. The aim for this technique is to provide an intellectual mapping of the field by identifying thematic clusters. Applied to a specific time frame, it uncovers niche themes as well as recent development of the field (51). In the current review, all articles published since 2015 that matched the query described in Supplementary Material were included in the analysis. Figure 6 presents an annotated version of the network with cluster nomination based on the predominance of a theme within the cluster. The Supplementary Material contains the original figure as well as the described calculation method.
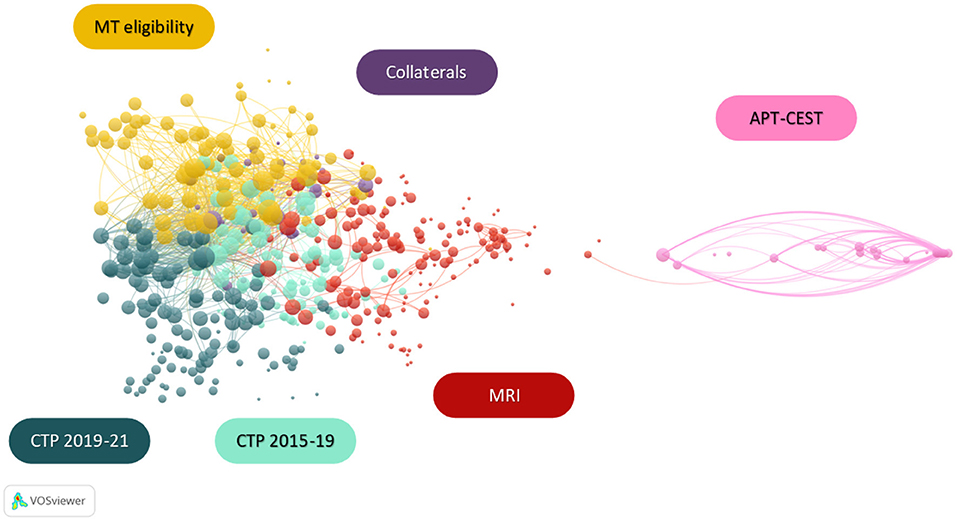
Figure 6. Bibliographic coupling network in the era of mechanical thrombectomy [2015–2021] with labeled clusters based on predominance of themes within the clusters. MRI, magnetic resonance imaging; CTP, computed tomography perfusion; MT, mechanical thrombectomy; APT, amide proton transfer; CEST, chemical exchange saturation transfer.
The resulting figure is densely populated and therefore interpretation of the bibliographic coupling is a challenging task. Following guidelines described in Supplementary Material, the most connected articles of each cluster were reviewed in order to elaborate on their content.
The presence of collaterals and MT eligibility clusters shows the emergence of tissue based patient selection criteria and a more personalized medicine. It also appears in imaging method clusters where tissue characterization is more and more present throughout time.
The majority of articles in the collaterals thematic cluster explore the relation between collateral circulation and infarct core growth (92–94). Other publications use MRI and CT imaging to identify markers of collateral status (95, 96).
The MT eligibility thematic cluster contains a selection of articles assessing the relevance of computed tomography perfusion (CTP) and MRI in patient selection, either with a comparison of the two methods (59, 97), or with the expression of a need for alternative imaging methods (98). This is consistent with the publications promoting tissue-based eligibility criteria over the conventional time windows (99–101). This cluster also includes guidelines intended to personalize AIS management through advanced imaging assessment (102, 103).
The cluster's thematic predominance and time frame also highlighted a change of course in the CTP research field in 2019. A detailed analysis of these clusters revealed the evolution of CTP imaging considerations in AIS management. The first cluster ranging from 2015 to 2019 contains a majority of publications aimed at validating perfusion thresholds and determining reliable eligibility parameters from this imaging modality (104–107). It also shows the emergence of studies to evaluate core and perfusion penumbra volumes as well as their relationship to collateral circulation (32, 108). The second cluster ranging from 2019 to 2021 includes publications retrospectively analyzing CTP data from the major clinical trials concomitant with the development of MT, confirming the growing interest in imaging-based patients selection for reperfusion therapies (109–111). This time frame also shows an emergence of automated CTP imaging post-processing to provide target mismatch volume as well as infarct growth prediction (112–116).
Publications aiming to automate post-processing of imaging modalities are also largely represented in the MRI cluster with articles describing numerous methods based on machine learning (117–119). This tendency is encouraged by the need for fast identification of penumbral tissue. Additionally, this group contains a subcluster focused on the validation of MRI for MT eligible patient selection, including comparisons with PET imaging (120, 121). If the majority of the articles validating MRI are focused on PWI-DWI mismatch, however, MRI modalities and sequences providing additional parameters such as hypoperfusion with DWI-susceptibility weighted imaging (SWI) mismatch are also present in this cluster (122, 123).
These imaging modality-focused clusters are representative of the central position of imaging in AIS care. The most frequent eligibility criteria for clinical trials on reperfusion therapies are CTP target mismatch and MRI DWI-PWI mismatch. However, because they differ in defining the lesion core, the accuracy of those methods is often compared. While these two methods are widespread, other imaging modalities, not relying on perfusion imaging, have been proposed. The DWI-FLAIR (Fluid-Attenuated Inversion Recovery) mismatch is a surrogate marker of lesions within 4.5 h of symptom onset used as selection criteria in the WAKE-UP trial that assessed the benefit of IV rt-PA in patients with unknown time of onset of stroke (124). Regarding CT, multiphase CT angiography (CTA) provides information on collateral status and has been used to select patients in the ESCAPE-NA1 trial that tested the efficacy and safety of nerinetide in AIS patients treated with MT within a 12 h window (125, 126). Of interest, patients included in the latter trial (control arm) had similar outcome than patients included in DAWN and DEFUSE-3 trials despite having larger lesion volume.
The thematic predominance of each cluster in relation to their physical position within the network also provides elaboration material. While the five groups on the left represent generic concepts, the isolated cluster on the right is focused on the specific amid proton transfer modality of chemical exchange saturation transfer imaging (APT-CEST). Additionally, the MRI cluster is closer and marginally connected to the APT-CEST cluster as the latter is an imaging modality of the former. In the context of ischemic stroke, APT-CEST provides pH-weighted imaging, a marker of tissue micro-environment which variation influences hemoglobin affinity for O2 (127, 128). The presence of this cluster is an evidence of the growing need for imaging parameters representative of tissue activity and metabolism rather than perfusion delay markers.
5. Future Directions
As initially demonstrated with PET imaging, penumbral tissues are described by a relatively preserved O2 metabolism within a region with perfusion deficit. With the change of paradigm operating and promoting tissue based eligibility criteria for reperfusion therapies, there is a need for an operational imaging parameter to evaluate the metabolic state of the tissues. The current knowledge of the field raises legitimate concerns about other imaging modalities providing metabolic parameters.
An additional exploration of the bibliometric coupling network was carried out in order to further investigate the location of oxygen metabolism imaging methods. The method of investigation is described in Supplementary Material.
The detailed exploration of the clusters has shown that oxygen metabolism related biomarkers (OEF and CMRO2) imaging methods are split into 2 clusters. Three articles are contained within the MRI cluster and relate to MRI based modalities. The CTP [2019–2021] cluster contains 3 articles related to these biomarkers, however, 2/3 methods in this group are based on MRI modalities. Regarding the methods developed, 4/6 publications are based on variations of blood oxygen level dependent (BOLD) functional MRI principles (129–132). Another article provided OEF mapping through quantitative susceptibility mapping MRI (133). Lastly, dynamic CTP provided OEF and CMRO2 mapping based on extrapolated data from perfusion acquisition; this method remains theoretical (134).
In addition to the search for these specific biomarkers, the bibliographic coupling also contains a selection of alternative marker imaging based on hemodynamics. The MRI cluster presents 3 articles for hemodynamic assessment as a marker of tissue activity with MRI SWI (135, 136) and MRI T2⋆-weighted imaging (137). Two articles relate to near infrared spectroscopy for hemodynamic, side-bed, AIS patient monitoring. This imaging modality, as well as photoacoustic, do not provide whole brain imaging and are therefore not addressed in this review.
From 2015 to 2021, articles providing metabolic parameters accounted for less than 2% of the corpus of scientific literature. Their importance within this network is minor compared to the imaging concepts that have been studied for the past two decades. The widespread distribution of these articles throughout the network indicates that the scientific community fails to reach a consensus on oxygen metabolism marker imaging, but MRI methodologies predominate. Table 1 provides an overview of these MRI methods, the majority of which are based on the quantification of the BOLD effect. An example of a multiparametric qBOLD approach is described in Figure 7.
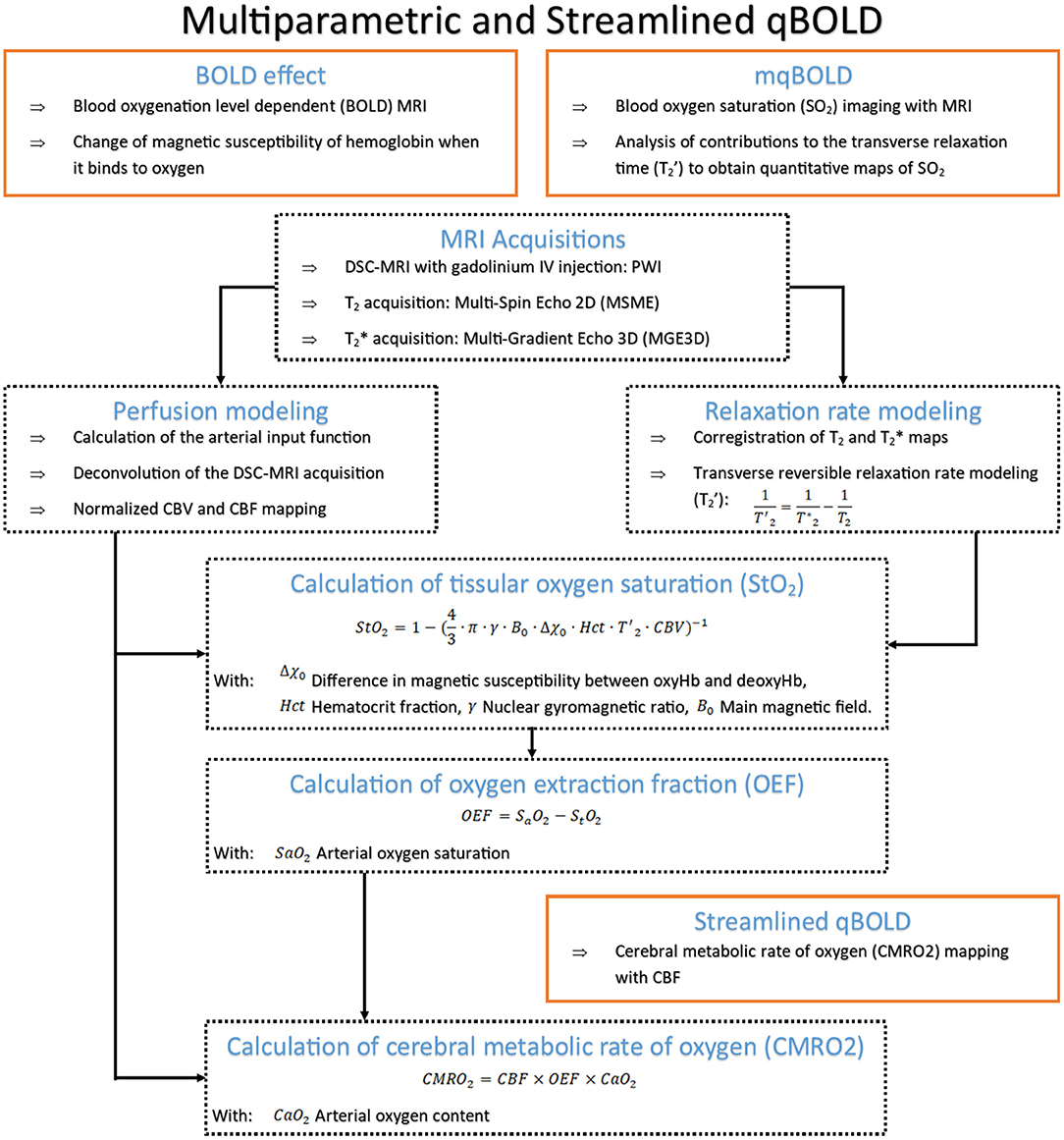
Figure 7. Detailed principle and pipeline for OEF mapping with the multiparametric quantitative Blood Oxygen Level Dependent (mqBOLD) method and CMRO2 mapping with streamlined qBOLD. MRI, magnetic resonance imaging; DSC, dynamic susceptibility contrast; IV, intravenous bolus; CBV, cerebral blood volume; CBF, cerebral blood flow.
It seems that these methods have the potential to provide the relevant markers of the ischemic penumbra. Yet, the current state of research in this field has not reached clinical validation. The methods described in Table 1 have been either validated for other pathologies or validated on ischemic stroke preclinical-models. Therefore, there are no known thresholds to qualify the metabolic penumbra in AIS clinical context with these methods. Moreover, technical issues still remain.
The original qBOLD method (140) is fast and non-invasive. However, it requires a special MR sequence usually not accessible in a clinical environment and the estimates are only valid if the signal to noise ratio (SNR) is very high. The mqBOLD approach (142) can be used with standard MR sequences but it requires the injection of a contrast agent and the co-registration of the maps is a challenging task due to the variations in resolution and potential distortions within the sequences. Moreover, the numerous parameters estimated for the calculations and the corresponding models only allows an approximated mapping of CMRO2 and OEF without considering tissue specificity (such as white vs. gray matter). An exciting alternative consists in the fusion of the qBOLD approach with a new MRI framework called MR fingerprinting (MRF) where complex MR sequences are directly linked to advanced numerical simulations (145). This new perspective would enable to shorten acquisition time and allow the quantification of several biomarkers simultaneously. Initial studies using MRF to quantify brain oxygenation in both humans and rodents (146, 147) have been very encouraging and the method is now under validation in preclinical AIS models and future intended contributions aim in defining thresholds for the metabolic penumbra to extend it to clinical settings.
In the present review, MRI advances are vastly discussed, but there are also developments for CTP, e.g. the calculation of penumbra and core from multiphase CTA acquisitions (148).
6. Conclusion
Conducting a bibliometric analysis of the field enabled to describe penumbra imaging over time and its close parallel relationship with the change of paradigm operating in AIS management. The key role of imaging was highlighted as well as the necessity for biomarkers of the cerebral tissue metabolic state in clinical emergency settings. Up to date the scientific community fails to reach a consensus on imaging and post processing modalities to meet these new requirements. MR CMRO2 methods and MR vascular fingerprinting for AIS models are particularly promising and deserve further exploration.
Author Contributions
EC-S, LM, and TB proposed and initiated the review focus and scheme. LC designed, conducted, and analyzed the bibliometric study. LM supervised the manuscript drafting and clinical focus. The CMRO2 pipelines were initially designed by JD and EC-S and adapted by LC. The other figures were prepared by LC and overviewed by LM, TB, and EC-S. DR provided the clinical images for the figures. LC drafted the manuscript and interpreted the bibliometric data with LM, EC-S, and TB. LC, TB, TC, JD, OE, GB, NN, T-HC, EC-S, and LM reviewed the literature in their respective field of expertise and revised the manuscript critically for important intellectual content. All authors approved the final version of the manuscript submitted.
Funding
This work was supported by ANR CYCLOPS and CMRO2 (ANR-15-CE17-0020 and ANR-21-CE17-0028), the RHU MARVELOUS (ANR-16-RHUS-0009) of Lyon University, under the Investissements d'Avenir program of the French National Research Agency (ANR). The Ph.D. salary of LC (Cifre, Olea Medical) is co-funded by the French Ministry of Higher Education and Research (ANRT).
Conflict of Interest
LC, DR, and TB are employees of Olea Medical, a company developing the OleaSphere platform including pipelines for ischemic penumbra.
The remaining authors declare that the research was conducted in the absence of any commercial or financial relationships that could be construed as a potential conflict of interest.
Publisher's Note
All claims expressed in this article are solely those of the authors and do not necessarily represent those of their affiliated organizations, or those of the publisher, the editors and the reviewers. Any product that may be evaluated in this article, or claim that may be made by its manufacturer, is not guaranteed or endorsed by the publisher.
Acknowledgments
We acknowledge the IRIS team, the RHU MARVELOUS and its Scientific Advisory Board for fruitful discussions about the ischemic penumbra imaging.
Supplementary Material
The Supplementary Material for this article can be found online at: https://www.frontiersin.org/articles/10.3389/fcvm.2022.861913/full#supplementary-material
Abbreviations
ADC, apparent diffusion coefficient; AHA, american stroke association; AIS, acute ischemic stroke; APT, amide proton transfer; ASPECTS, alberta stroke program early CT score; BOLD, blood oxygen level dependent; CBF, cerebral blood flow; CBV, cerebral blood volume; CCR, contralateral control region; CEST, chemical exchange saturation transfer; CMRO2, cerebral metabolic rate of oxygen; CT, computed tomography; CTP, CT perfusion; DSC, dynamic susceptibility contrast; DWI, diffusion weighted imaging; FMZ, 11C-flumazenil; FMISO, (18)F-misonidazole; FLAIR, fluid-attenuated inversion recovery; IV, intravenous; LVO, large vessel occlusion; mqBOLD, multiparametric qBOLD; MRI, magnetic resonance imaging; MRF, MR fingerprinting; MT, mechanical thrombectomy; MTT, mean transit time; OEF, oxygen extraction fraction; PET, positron emission tomography; PWI, perfusion weighted imaging; qBOLD, quantitative BOLD; QSM, quantitative susceptibility mapping; rt-PA, recombinant tissue plasminogen activator; SNR, signal to noise ratio; SPECT, single photon emission computed tomography; SVD, singular value decomposition; SWI, susceptibility weighted imaging; Tmax, time to maximum of residual function; TTP, time to peak.
Footnotes
1. ^ASPECTS, Alberta Stroke Program Early CT Score.
References
1. Wafa HA, Wolfe CDA, Emmett E, Roth GA, Johnson CO, Wang Y. Burden of stroke in Europe. Stroke. (2020) 51:2418–27. doi: 10.1161/STROKEAHA.120.029606
2. Ovbiagele B, Goldstein LB, Higashida RT, Howard VJ, Johnston SC, Khavjou OA, et al. Forecasting the future of stroke in the United States: a policy statement from the American Heart Association and American Stroke Association. Stroke. (2013) 44:2361–75. doi: 10.1161/STR.0b013e31829734f2
3. Astrup J, Siesjo BK, Symon L. Thresholds in cerebral ischemia - the ischemic penumbra. Stroke. (1981) 12:723–5. doi: 10.1161/01.STR.12.6.723
4. Baron JC. Mapping the ischaemic penumbra with PET: implications for acute stroke treatment. Cerebrovasc Dis. (1999) 9:193–201. doi: 10.1159/000015955
5. Jones TH, Morawetz RB, Crowell RM, Marcoux FW, FitzGibbon SJ, DeGirolami U, et al. Thresholds of focal cerebral ischemia in awake monkeys. J Neurosurg. (1981) 54:773–82. doi: 10.3171/jns.1981.54.6.0773
6. National Institute of Neurological Disorders and Stroke RT-PA Stroke Study Group. Tissue plasminogen activator for acute ischemic stroke. N Engl J Med. (1995). 333:1581–8. doi: 10.1056/NEJM199512143332401
7. Hacke W, Kaste M, Bluhmki E, Brozman M, Davalos A, Guidetti D, et al. Thrombolysis with alteplase 3 to 4.5 hours after acute ischemic stroke. N Engl J Med. (2008) 359:1317–29. doi: 10.1056/NEJMoa0804656
8. Goyal M, Menon BK, van Zwam WH, Dippel DWJ, Mitchell PJ, Demchuk AM, et al. Endovascular thrombectomy after large-vessel ischaemic stroke: a meta-analysis of individual patient data from five randomised trials. Lancet. (2016) 387:1723–31. doi: 10.1016/S0140-6736(16)00163-X
9. Vagal A, Aviv R, Sucharew H, Reddy M, Hou Q, Michel P, et al. Collateral clock is more important than time clock for tissue fate. Stroke. (2018) 49:2102–7. doi: 10.1161/STROKEAHA.118.021484
10. Campbell BCV, Ma H, Ringleb PA, Parsons MW, Churilov L, Bendszus M, et al. Extending thrombolysis to 45–9 h and wake-up stroke using perfusion imaging: a systematic review and meta-analysis of individual patient data. Lancet. (2019) 394:139–47. doi: 10.1016/S0140-6736(19)31053-0
11. Albers GW, Marks MP, Kemp S, Christensen S, Tsai JP, Ortega-Gutierrez S, et al. Thrombectomy for stroke at 6 to 16 hours with selection by perfusion imaging. N Engl J Med. (2018) 378:708–18. doi: 10.1056/NEJMoa1713973
12. Nogueira RG, Jadhav AP, Haussen DC, Bonafe A, Budzik RF, Bhuva P, et al. Thrombectomy 6 to 24 hours after stroke with a mismatch between deficit and infarct. N Engl J Med. (2018) 378:11–21. doi: 10.1056/NEJMoa1706442
13. Branston NM, Strong AJ, Symon L. Extracellular potassium activity, evoked potential and tissue blood flow. Relationships during progressive ischaemia in baboon cerebral cortex. J Neurol Sci. (1977) 32:305–21. doi: 10.1016/0022-510X(77)90014-4
14. Astrup J, Symon L, Branston NM, Lassen NA. Cortical evoked potential and extracellular K+ and H+ at critical levels of brain ischemia. Stroke. (1977) 8:51–7. doi: 10.1161/01.STR.8.1.51
15. Astrup J, Symon L, Branston NM, Lassen NA. Thresholds of cerebral ischemia. In: Schmiedek P, Gratzl O, Spetzler RF, editors. Microsurgery for Stroke. New York, NY: Springer. (1977). p. 16–21. doi: 10.1007/978-1-4612-6349-4_3
16. Warach S. Measurement of the ischemic penumbra with MRI: it's about time. Stroke. (2003) 34:2533–4. doi: 10.1161/01.STR.0000092395.19554.9A
17. Baron JC, Bousser MG, Comar D, Soussaline F, Castaigne P. Noninvasive tomographic study of cerebral blood flow and oxygen metabolism in vivo. Eur Neurol. (1981) 20:273–84. doi: 10.1159/000115247
18. Fan AP, An H, Moradi F, Rosenberg J, Ishii Y, Nariai T, et al. Quantification of brain oxygen extraction and metabolism with [15O]-gas PET: a technical review in the era of PET/MRI. Neuroimage. (2020) 220:117136. doi: 10.1016/j.neuroimage.2020.117136
19. Holden JE, Eriksson L, Roland PE, Stone-Elander S, Widen L, Kesselberg M. Direct comparison of single-scan autoradiographic with multiple-scan least-squares fitting approaches to PET CMRO2 estimation. J Cereb Blood Flow Metab. (1988) 8:671–80. doi: 10.1038/jcbfm.1988.114
20. Kudomi N, Watabe H, Hayashi T, Iida H. Separation of input function for rapid measurement of quantitative CMRO2 and CBF in a single PET scan with a dual tracer administration method. Phys Med Biol. (2007) 52:1893–908. doi: 10.1088/0031-9155/52/7/009
21. Mintun MA, Raichle ME, Martin WR, Herscovitch P. Brain oxygen utilization measured with O-15 radiotracers and positron emission tomography. J Nuclear Med. (1984) 25:177–87.
22. Heiss WD, Sobesky J, Hesselmann V. Identifying thresholds for penumbra and irreversible tissue damage. Stroke. (2004) 35(11 Suppl 1):2671–4. doi: 10.1161/01.STR.0000143329.81997.8a
23. Lenzi GL, Frackowiak RS, Jones T. Cerebral oxygen metabolism and blood flow in human cerebral ischemic infarction. J Cereb Blood Flow Metab. (1982) 2:321–35. doi: 10.1038/jcbfm.1982.33
24. Furlan M, Marchal G, Derlon JM, Baron JC, Viader F. Spontaneous neurological recovery after stroke and the fate of the ischemic penumbra. Ann Neurol. (1996) 40:216–26. doi: 10.1002/ana.410400213
25. Marchal G, Benali K, Iglesias S, Viader F, Derlon JM, Baron JC. Voxel-based mapping of irreversible ischaemic damage with PET in acute stroke. Brain. (1999) 122:2387–400. doi: 10.1093/brain/122.12.2387
26. Wise RJ, Bernardi S, Frackowiak RS, Legg NJ, Jones T. Serial observations on the pathophysiology of acute stroke. The transition from ischaemia to infarction as reflected in regional oxygen extraction. Brain J Neurol. (1983) 106 (Pt 1):197–222. doi: 10.1093/brain/106.1.197
27. Powers WJ, Grubb RL, Darriet D, Raichle ME. Cerebral blood flow and cerebral metabolic rate of oxygen requirements for cerebral function and viability in humans. J Cereb Blood Flow Metab. (1985) 5:600–8. doi: 10.1038/jcbfm.1985.89
28. Marchal G, Beaudouin V, Rioux P, de la Sayette V, Le Doze F, Viader F, et al. Prolonged persistence of substantial volumes of potentially viable brain tissue after stroke. Stroke. (1996) 27:599–606. doi: 10.1161/01.STR.27.4.599
29. Heiss WD, Grond M, Thiel A, von Stockhausen HM, Rudolf J, Ghaemi M, et al. Tissue at risk of infarction rescued by early reperfusion: a positron emission tomography study in systemic recombinant tissue plasminogen activator thrombolysis of acute stroke. J Cereb Blood Flow Metab. (1998) 18:1298–307. doi: 10.1097/00004647-199812000-00004
30. Heiss WD, Kracht LW, Thiel A, Grond M, Pawlik G. Penumbral probability thresholds of cortical flumazenil binding and blood flow predicting tissue outcome in patients with cerebral ischaemia. Brain. (2001) 124:20–9. doi: 10.1093/brain/124.1.20
31. Heiss WD, Kracht L, Grond M, Rudolf J, Bauer B, Wienhard K, et al. Early [11C]Flumazenil/H2O positron emission tomography predicts irreversible ischemic cortical damage in stroke patients receiving acute thrombolytic therapy. Stroke. (2000) 31:366–9. doi: 10.1161/01.STR.31.2.366
32. Agarwal S, Matys T, Marrapu ST, Scoffings DJ, Mitchell J, Jones PS, et al. Is CT-based perfusion and collateral imaging sensitive to time since stroke onset? Front Neurol. (2015) 6:70. doi: 10.3389/fneur.2015.00070
33. Mahagne MH, David O, Darcourt J, Migneco O, Dunac A, Chatel M, et al. Voxel-based mapping of cortical ischemic damage using Tc 99m L,L-ethyl cysteinate dimer SPECT in acute stroke. J Neuroimaging. (2004) 14:23–32. doi: 10.1177/1051228403258081
34. Hirano T, Read SJ, Abbott DF, Baird AE, Yasaka M, Infeld B, et al. Prediction of the final infarct volume within 6 h of stroke using single photon emission computed tomography with technetium-99m hexamethylpropylene amine oxime. Cerebrovasc Dis. (2001) 11:119–27. doi: 10.1159/000047623
35. Giubilei F, Lenzi GL, Di Piero V, Pozzilli C, Pantano P, Bastianello S, et al. Predictive value of brain perfusion single-photon emission computed tomography in acute ischemic stroke. Stroke. (1990) 21:895–900. doi: 10.1161/01.STR.21.6.895
36. Karonen JO, Nuutinen J, Kuikka JT, Vanninen EJ, Vanninen RL, Partanen PL, et al. Combined SPECT and diffusion-weighted MRI as a predictor of infarct growth in acute ischemic stroke. J Nuclear Med. (2000) 41:788–94. doi: 10.1148/radiology.217.3.r00dc02886
37. Warach S, Dashe JF, Edelman RR. Clinical outcome in ischemic stroke predicted by early diffusion-weighted and perfusion magnetic resonance imaging: a preliminary analysis. J Cereb Blood Flow Metab. (1996) 16:53–9. doi: 10.1097/00004647-199601000-00006
38. Wintermark M, Reichhart M, Thiran JP, Maeder P, Chalaron M, Schnyder P, et al. Prognostic accuracy of cerebral blood flow measurement by perfusion computed tomography, at the time of emergency room admission, in acute stroke patients. Ann Neurol. (2002) 51:417–32. doi: 10.1002/ana.10136
39. Wu O, Østergaard L, Weisskoff RM, Benner T, Rosen BR, Sorensen AG. Tracer arrival timing-insensitive technique for estimating flow in MR perfusion-weighted imaging using singular value decomposition with a block-circulant deconvolution matrix. Magnet Reson Med. (2003) 50:164–74. doi: 10.1002/mrm.10522
40. Boutelier T, Kudo K, Pautot F, Sasaki M. Bayesian hemodynamic parameter estimation by bolus tracking perfusion weighted imaging. IEEE Trans Med Imaging. (2012) 31:1381–95. doi: 10.1109/TMI.2012.2189890
41. Oppenheim C, Grandin C, Samson Y, Smith A, Duprez T, Marsault C, et al. Is there an apparent diffusion coefficient threshold in predicting tissue viability in hyperacute stroke? Stroke. (2001) 32:2486–91. doi: 10.1161/hs1101.098331
42. Barber PA, Darby DG, Desmond PM, Yang Q, Gerraty RP, Jolley D, et al. Prediction of stroke outcome with echoplanar perfusion- and diffusion-weighted MRI. Neurology. (1998) 51:418–26. doi: 10.1212/WNL.51.2.418
43. Schlaug G, Benfield A, Baird AE, Siewert B, Lovblad KO, Parker RA, et al. The ischemic penumbra: operationally defined by diffusion and perfusion MRI. Neurology. (1999) 53:1528–1528. doi: 10.1212/WNL.53.7.1528
44. Olivot JM, Mlynash M, Thijs VN, Kemp S, Lansberg MG, Wechsler L, et al. Optimal Tmax threshold for predicting penumbral tissue in acute stroke. Stroke. (2009) 40:469–75. doi: 10.1161/STROKEAHA.108.526954
45. Purushotham A, Campbell BCV, Straka M, Mlynash M, Olivot JM, Bammer R, et al. Apparent diffusion coefficient threshold for delineation of ischemic core. Int J Stroke. (2015) 10:348–53. doi: 10.1111/ijs.12068
46. Mayer TE, Hamann GF, Baranczyk J, Rosengarten B, Klotz E, Wiesmann M, et al. Dynamic CT perfusion imaging of acute stroke. Am J Neuroradiol. (2000) 21:1441–9.
47. Baron JC. Protecting the ischaemic penumbra as an adjunct to thrombectomy for acute stroke. Nat Rev Neurol. (2018) 14:325–37. doi: 10.1038/s41582-018-0002-2
48. Ermine CM, Bivard A, Parsons MW, Baron JC. The ischemic penumbra: from concept to reality. Int J Stroke. (2021) 16:497–509. doi: 10.1177/1747493020975229
49. Austein F, Riedel C, Kerby T, Meyne J, Binder A, Lindner T, et al. Comparison of perfusion CT software to predict the final infarct volume after thrombectomy. Stroke. (2016) 47:2311–7. doi: 10.1161/STROKEAHA.116.013147
50. Rava RA, Snyder KV, Mokin M, Waqas M, Zhang X, Podgorsak AR, et al. Assessment of computed tomography perfusion software in predicting spatial location and volume of infarct in acute ischemic stroke patients: a comparison of Sphere, Vitrea, and RAPID. J Neurointervent Surg. (2021) 13:130–5. doi: 10.1136/neurintsurg-2020-015966
51. Donthu N, Kumar S, Mukherjee D, Pandey N, Lim WM. How to conduct a bibliometric analysis: an overview and guidelines. J Bus Res. (2021) 133:285–96. doi: 10.1016/j.jbusres.2021.04.070
52. Sobesky J, Zaro Weber O, Lehnhardt FG, Hesselmann V, Neveling M, Jacobs A, et al. Does the mismatch match the penumbra? Magnetic resonance imaging and positron emission tomography in early ischemic stroke. Stroke. (2005) 36:980–5. doi: 10.1161/01.STR.0000160751.79241.a3
53. Heiss WD, Weber OZ. Validation of MRI determination of the penumbra by PET measurements in ischemic stroke. J Nuclear Med. (2017) 58:187–93. doi: 10.2967/jnumed.116.185975
54. Guadagno JV, Warburton EA, Aigbirhio FI, Smielewski P, Fryer TD, Harding S, et al. Does the acute diffusion-weighted imaging lesion represent penumbra as well as core? A combined quantitative PET/MRI voxel-based study. J Cereb Blood Flow Metab. (2004) 24:1249–54. doi: 10.1097/01.WCB.0000141557.32867.6B
55. Miteff F, Levi CR, Bateman GA, Spratt N, McElduff P, Parsons MW. The independent predictive utility of computed tomography angiographic collateral status in acute ischaemic stroke. Brain. (2009) 132:2231–8. doi: 10.1093/brain/awp155
56. Barber PA, Demchuk AM, Zhang J, Buchan AM. Validity and reliability of a quantitative computed tomography score in predicting outcome of hyperacute stroke before thrombolytic therapy. ASPECTS Study Group. Alberta Stroke Programme Early CT Score. Lancet. (2000) 355:1670–4. doi: 10.1016/S0140-6736(00)02237-6
57. Pexman JH, Barber PA, Hill MD, Sevick RJ, Demchuk AM, Hudon ME, et al. Use of the Alberta Stroke Program Early CT Score (ASPECTS) for assessing CT scans in patients with acute stroke. Am J Neuroradiol. (2001) 22:1534–42.
58. Wintermark M, Reichhart M, Cuisenaire O, Maeder P, Thiran J, Schnyder P, et al. Comparison of admission perfusion computed tomography and qualitative diffusion- and perfusion-weighted magnetic resonance imaging in acute stroke patients. Stroke. (2002) 33:2025–31. doi: 10.1161/01.STR.0000023579.61630.AC
59. Bonney PA, Walcott BP, Singh P, Nguyen PL, Sanossian N, Mack WJ. The continued role and value of imaging for acute ischemic stroke. Neurosurgery. (2019) 85:S23–30. doi: 10.1093/neuros/nyz068
60. Mehta B, Leslie-Mazwi TM, Chandra RV, Chaudhry ZA, Rabinov JD, Hirsch JA, et al. Assessing variability in neurointerventional practice patterns for acute ischemic stroke. J Neurointervent Surg. (2013) 5(Suppl 1):i52–7. doi: 10.1136/neurintsurg-2012-010565
61. Provost C, Soudant M, Legrand L, Ben Hassen W, Xie Y, Soize S, et al. Magnetic resonance imaging or computed tomography before treatment in acute ischemic stroke. Stroke. (2019) 50:659–64. doi: 10.1161/STROKEAHA.118.023882
62. Simonsen CZ, Sarensen LH, Karabegovic S, Mikkelsen IK, Schmitz ML, Juul N, et al. MRI before intraarterial therapy in ischemic stroke: feasibility, impact, and safety. J Cereb Blood Flow Metab. (2014) 34:1076–81. doi: 10.1038/jcbfm.2014.57
63. Lee H, Yang Y, Liu B, Castro SA, Shi T. Patients with acute ischemic stroke who receive brain magnetic resonance imaging demonstrate favorable in-hospital outcomes. J Am Heart Assoc. (2020) 9:e016987. doi: 10.1161/JAHA.120.016987
64. Gerischer LM, Fiebach JB, Scheitz JF, Audebert HJ, Endres M, Nolte CH. Magnetic resonance imaging-based versus computed tomography-based thrombolysis in acute ischemic stroke: comparison of safety and efficacy within a cohort study. Cerebrovasc Dis. (2013) 35:250–6. doi: 10.1159/000347071
65. Kim JT, Cho BH, Choi KH, Park MS, Kim BJ, Park JM, et al. Magnetic resonance imaging versus computed tomography angiography based selection for endovascular therapy in patients with acute ischemic stroke. Stroke. (2019) 50:365–72. doi: 10.1161/STROKEAHA.118.023173
66. Hacke W, Albers G, Al-Rawi Y, Bogousslavsky J, Davalos A, Eliasziw M, et al. The Desmoteplase in Acute Ischemic Stroke Trial (DIAS): a phase II MRI-based 9-hour window acute stroke thrombolysis trial with intravenous desmoteplase. Stroke. (2005) 36:66–73. doi: 10.1161/01.STR.0000149938.08731.2c
67. Hacke W, Furlan AJ, Al-Rawi Y, Davalos A, Fiebach JB, Gruber F, et al. Intravenous desmoteplase in patients with acute ischaemic stroke selected by MRI perfusion-diffusion weighted imaging or perfusion CT (DIAS-2): a prospective, randomised, double-blind, placebo-controlled study. Lancet Neurol. (2009) 8:141–50. doi: 10.1016/S1474-4422(08)70267-9
68. Davis SM, Donnan GA, Parsons MW, Levi C, Butcher KS, Peeters A, et al. Effects of alteplase beyond 3 h after stroke in the Echoplanar Imaging Thrombolytic Evaluation Trial (EPITHET): a placebo-controlled randomised trial. Lancet Neurol. (2008) 7:299–309. doi: 10.1016/S1474-4422(08)70044-9
69. Albers GW, Thijs VN, Wechsler L, Kemp S, Schlaug G, Skalabrin E, et al. Magnetic resonance imaging profiles predict clinical response to early reperfusion: the diffusion and perfusion imaging evaluation for understanding stroke evolution (DEFUSE) study. Ann Neurol. (2006) 60:508–17. doi: 10.1002/ana.20976
70. Olivot JM, Mlynash M, Thijs VN, Purushotham A, Kemp S, Lansberg MG, et al. Geography, structure, and evolution of diffusion and perfusion lesions in diffusion and perfusion imaging evaluation for understanding stroke evolution (DEFUSE). Stroke. (2009) 40:3245–51. doi: 10.1161/STROKEAHA.109.558635
71. Butcher K, Parsons M, MacGregor L, Barber P, Chalk J, Bladin C, et al. Refining the perfusion-diffusion mismatch hypothesis. Stroke. (2005) 36:1153–9. doi: 10.1161/01.STR.0000166181.86928.8b
72. Straka M, Albers GW, Bammer R. Real-time diffusion-perfusion mismatch analysis in acute stroke. J Magnet Reson Imaging. (2010) 32:1024–37. doi: 10.1002/jmri.22338
73. Takasawa M, Jones PS, Guadagno JV, Christensen S, Fryer TD, Harding S, et al. How reliable is perfusion MR in acute stroke? Stroke. (2008) 39:870–7. doi: 10.1161/STROKEAHA.107.500090
74. Zaro-Weber O, Moeller-Hartmann W, Heiss WD, Sobesky J. MRI perfusion maps in acute stroke validated with 15O-water positron emission tomography. Stroke. (2010) 41:443–9. doi: 10.1161/STROKEAHA.109.569889
75. Furlan A, Higashida R, Wechsler L, Gent M, Rowley H, Kase C, et al. Intra-arterial prourokinase for acute ischemic stroke. The PROACT II study: a randomized controlled trial. Prolyse in acute cerebral thromboembolism. JAMA. (1999) 282:2003–11. doi: 10.1001/jama.282.21.2003
76. Hacke W, Kaste M, Fieschi C, von Kummer R, Davalos A, Meier D, et al. Randomised double-blind placebo-controlled trial of thrombolytic therapy with intravenous alteplase in acute ischaemic stroke (ECASS II). Second European-Australasian Acute Stroke Study Investigators. Lancet. (1998) 352:1245–51. doi: 10.1016/S0140-6736(98)08020-9
77. Hacke W, Donnan G, Fieschi C, Kaste M, von Kummer R, Broderick JP, et al. Association of outcome with early stroke treatment: pooled analysis of ATLANTIS, ECASS, and NINDS rt-PA stroke trials. Lancet. (2004) 363:768–74. doi: 10.1016/S0140-6736(04)15692-4
78. Smith WS, Sung G, Starkman S, Saver JL, Kidwell CS, Gobin YP, et al. Safety and efficacy of mechanical embolectomy in acute ischemic stroke: results of the MERCI trial. Stroke. (2005) 36:1432–8. doi: 10.1161/01.STR.0000171066.25248.1d
79. Smith WS, Sung G, Saver J, Budzik R, Duckwiler G, Liebeskind DS, et al. Mechanical thrombectomy for acute ischemic stroke: final results of the Multi MERCI trial. Stroke. (2008) 39:1205–12. doi: 10.1161/STROKEAHA.107.497115
80. Penumbra Pivotal Stroke Trial Investigators. The penumbra pivotal stroke trial: safety and effectiveness of a new generation of mechanical devices for clot removal in intracranial large vessel occlusive disease. Stroke. (2009) 40:2761–8. doi: 10.1161/STROKEAHA.108.544957
81. Kidwell CS, Jahan R, Gornbein J, Alger JR, Nenov V, Ajani Z, et al. A trial of imaging selection and endovascular treatment for ischemic stroke. N Engl J Med. (2013) 368:914–23. doi: 10.1056/NEJMoa1212793
82. Broderick JP, Palesch YY, Demchuk AM, Yeatts SD, Khatri P, Hill MD, et al. Endovascular therapy after intravenous t-PA versus t-PA alone for stroke. N Engl J Med. (2013) 368:893–903. doi: 10.1056/NEJMoa1214300
83. Berkhemer OA, Fransen PSS, Beumer D, van den Berg LA, Lingsma HF, Yoo AJ, et al. A randomized trial of intraarterial treatment for acute ischemic stroke. N Engl J Med. (2015) 372:11–20. doi: 10.1056/NEJMoa1411587
84. Campbell BCV, Donnan GA, Lees KR, Hacke W, Khatri P, Hill MD, et al. Endovascular stent thrombectomy: the new standard of care for large vessel ischaemic stroke. Lancet Neurol. (2015) 14:846–54. doi: 10.1016/S1474-4422(15)00140-4
85. Saver JL, Goyal M, Bonafe A, Diener HC, Levy EI, Pereira VM, et al. Stent-retriever thrombectomy after intravenous t-PA vs. t-PA alone in stroke. N Engl J Med. (2015) 372:2285–95. doi: 10.1056/NEJMoa1415061
86. Jovin TG, Chamorro A, Cobo E, de Miquel MA, Molina CA, Rovira A, et al. Thrombectomy within 8 hours after symptom onset in ischemic stroke. N Engl J Med. (2015) 372:2296–306. doi: 10.1056/NEJMoa1503780
87. Goyal M, Demchuk AM, Menon BK, Eesa M, Rempel JL, Thornton J, et al. Randomized assessment of rapid endovascular treatment of ischemic stroke. N Engl J Med. (2015) 372:1019–30. doi: 10.1056/NEJMoa1414905
88. Bracard S, Ducrocq X, Mas JL, Soudant M, Oppenheim C, Moulin T, et al. Mechanical thrombectomy after intravenous alteplase versus alteplase alone after stroke (THRACE): a randomised controlled trial. Lancet Neurol. (2016) 15:1138–47. doi: 10.1016/S1474-4422(16)30177-6
89. Powers WJ, Rabinstein AA, Ackerson T, Adeoye OM, Bambakidis NC, Becker K, et al. 2018 guidelines for the early management of patients with acute ischemic stroke: a guideline for healthcare professionals from the American Heart Association/American Stroke Association. Stroke. (2018). 49:e46–110. doi: 10.1161/STR.0000000000000158
90. Powers WJ, Rabinstein AA, Ackerson T, Adeoye OM, Bambakidis NC, Becker K, et al. Guidelines for the early management of patients with acute ischemic stroke: 2019 update to the 2018 guidelines for the early management of acute ischemic stroke: a guideline for healthcare professionals from the American Heart Association/American Stroke Association. Stroke. (2019). 50:e344–418. doi: 10.1161/STR.0000000000000211
91. Kessler MM. Bibliographic coupling between scientific papers. Am Document. (1963) 14:10–25. doi: 10.1002/asi.5090140103
92. Jiang B, Ball RL, Michel P, Li Y, Zhu G, Ding V, et al. Factors influencing infarct growth including collateral status assessed using computed tomography in acute stroke patients with large artery occlusion. Int J Stroke. (2019) 14:603–12. doi: 10.1177/1747493019851278
93. Rusanen H, Saarinen JT, Sillanpaa N. Collateral circulation predicts the size of the infarct core and the proportion of salvageable penumbra in hyperacute ischemic stroke patients treated with intravenous thrombolysis. Cerebrovasc Dis. (2015) 40:182–90. doi: 10.1159/000439064
94. von Baumgarten L, Thierfelder KM, Beyer SE, Baumann AB, Bollwein C, Janssen H, et al. Early CT perfusion mismatch in acute stroke is not time-dependent but relies on collateralization grade. Neuroradiology. (2016) 58:357–65. doi: 10.1007/s00234-016-1643-8
95. Potreck A, Seker F, Hoffmann A, Pfaff J, Nagel S, Bendszus M, et al. A novel method to assess pial collateralization from stroke perfusion MRI: subdividing T-max into anatomical compartments. Eur Radiol. (2017) 27:618–26. doi: 10.1007/s00330-016-4415-2
96. Kawano H, Bivard A, Lin L, Spratt NJ, Miteff F, Parsons MW, et al. Relationship between collateral status, contrast transit, and contrast density in acute ischemic stroke. Stroke. (2016) 47:742–9. doi: 10.1161/STROKEAHA.115.011320
97. Catanese L, Tarsia J, Fisher M. Acute ischemic stroke therapy overview. Circ Res. (2017) 120:541–58. doi: 10.1161/CIRCRESAHA.116.309278
98. El-Tawil S, Wardlaw J, Ford I, Mair G, Robinson T, Kalra L, et al. Penumbra and re-canalization acute computed tomography in ischemic stroke evaluation: PRACTISE study protocol. Int J Stroke. (2017) 12:671–8. doi: 10.1177/1747493017696099
99. Smith WS. Endovascular stroke therapy. Neurotherapeutics. (2019) 16:360–8. doi: 10.1007/s13311-019-00724-5
100. Vakharia K, Atwal GS, Levy E I. Management of acute ischemic thrombosis. Neurosurg Clin N Am. (2018) 29:595. doi: 10.1016/j.nec.2018.06.012
101. Wu L, Wu W, Tali ET, Yuh WT. Oligemia, penumbra, infarction understanding hypoperfusion with neuroimaging. Neuroimaging Clin N Am. (2018) 28:599–609. doi: 10.1016/j.nic.2018.06.013
102. Yamagami H, Hayakawa M, Inoue M, Iihara K, Ogasawara K, Toyoda K, et al. Guidelines for mechanical thrombectomy in Japan, the fourth edition, march 2020: a guideline from the Japan stroke society, the Japan neurosurgical society, and the Japanese society for neuroendovascular therapy. Neurol Med Chirurgica. (2021) 61:163–92. doi: 10.2176/nmc.nmc.st.2020-0357
103. Yu W, Jiang WJ. A simple imaging guide for endovascular thrombectomy in acute ischemic stroke: from time window to perfusion mismatch and beyond. Front Neurol. (2019) 10:502. doi: 10.3389/fneur.2019.00502
104. Bivard A, Kleinig T, Miteff F, Butcher K, Lin L, Levi C, et al. Ischemic core thresholds change with time to reperfusion: a case control study. Ann Neurol. (2017) 82:995–1003. doi: 10.1002/ana.25109
105. d'Esterre CD, Boesen ME, Ahn SH, Pordeli P, Najm M, Minhas P, et al. Time-dependent computed tomographic perfusion thresholds for patients with acute ischemic stroke. Stroke. (2015) 46:3390–7. doi: 10.1161/STROKEAHA.115.009250
106. Flottmann F, Broocks G, Faizy TD, Ernst M, Forkert ND, Grosser M, et al. CT-perfusion stroke imaging: a threshold free probabilistic approach to predict infarct volume compared to traditional ischemic thresholds. Sci Rep. (2017) 7:6679. doi: 10.1038/s41598-017-06882-w
107. Lin L, Bivard A, Krishnamurthy V, Levi CR, Parsons MW. Whole-brain CT perfusion to quantify acute ischemic penumbra and core. Radiology. (2016) 279:876–87. doi: 10.1148/radiol.2015150319
108. Cheripelli BK, Huang X, McVerry F, Muir KW. What is the relationship among penumbra volume, collaterals, and time since onset in the first 6h after acute ischemic stroke? Int J Stroke. (2016) 11:338–46. doi: 10.1177/1747493015620807
109. Campbell BCV, Majoie CBLM, Albers GW, Menon BK, Yassi N, Sharma G, et al. Penumbral imaging and functional outcome in patients with anterior circulation ischaemic stroke treated with endovascular thrombectomy versus medical therapy: a meta-analysis of individual patient-level data. Lancet Neurol. (2019) 18:46–55. doi: 10.1016/S1474-4422(18)30314-4
110. Carbone F, Busto G, Padroni M, Bernardoni A, Colagrande S, Dallegri F, et al. Radiologic cerebral reperfusion at 24h predicts good clinical outcome. Transl Stroke Res. (2019) 10:178–88. doi: 10.1007/s12975-018-0637-8
111. Chen C, Parsons MW, Levi CR, Spratt NJ, Lin L, Kleinig T, et al. What is the “optimal” target mismatch criteria for acute ischemic stroke? Front Neurol. (2021) 11:590766. doi: 10.3389/fneur.2020.590766
112. Cimflova P, Volny O, Mikulik R, Tyshchenko B, Belaskova S, Vinklarek J, et al. Detection of ischemic changes on baseline multimodal computed tomography: expert reading vs. brainomix and RAPID software. J Stroke Cerebrovasc Dis. (2020) 29:104978. doi: 10.1016/j.jstrokecerebrovasdis.2020.104978
113. Perez-Pelegri M, Biarnes C, Thio-Henestrosa S, Remollo S, Gimeno A, Cuba V, et al. Higher agreement in endovascular treatment decision-making than in parametric quantifications among automated CT perfusion software packages in acute ischemic stroke. J. Xray Sci Technol. (2021) 29:823–34. doi: 10.3233/XST-210898
114. Psychogios MN, Sporns PB, Ospel J, Katsanos AH, Kabiri R, Flottmann FA, et al. Automated Perfusion Calculations vs. visual scoring of collaterals and CBV-ASPECTS has the machine surpassed the eye? Clin Neuroradiol. (2021) 31:499–506. doi: 10.1007/s00062-020-00974-3
115. Krusche C, Bartulos CR, Abu-Mugheisib M, Haimerl M, Wiggermann P. Dynamic perfusion analysis in acute ischemic stroke: a comparative study of two different softwares. Clin Hemorheol Microcirc. (2021) 79:55–63. doi: 10.3233/CH-219106
116. Shi Z, Li J, Zhao M, Zhang M, Wang T, Chen L, et al. Baseline cerebral ischemic core quantified by different automatic software and its predictive value for clinical outcome. Front Neurosci. (2021) 15:608799. doi: 10.3389/fnins.2021.608799
117. Yu Y, Xie Y, Thamm T, Gong E, Ouyang J, Christensen S, et al. Tissue at risk and ischemic core estimation using deep learning in acute stroke. Am J Neuroradiol. (2021) 42:1030–7. doi: 10.3174/ajnr.A7081
118. Vupputuri A, Ghosh N. Multi-view iterative random walker for automated salvageable tissue delineation in ischemic stroke from multi-sequence MRI. J Neurosci Methods. (2021) 360:109260. doi: 10.1016/j.jneumeth.2021.109260
119. Benzakoun J, Charron S, Turc G, Hassen WB, Legrand L, Boulouis G, et al. Tissue outcome prediction in hyperacute ischemic stroke: Comparison of machine learning models. J Cereb Blood Flow Metab. (2021) 41:3085–96. doi: 10.1177/0271678X211024371
120. Zaro-Weber O, Moeller-Hartmann W, Siegmund D, Kandziora A, Schuster A, Heiss WD, et al. MRI-based mismatch detection in acute ischemic stroke: optimal PWI maps and thresholds validated with PET. J Cereb Blood Flow Metab. (2017) 37:3176–83. doi: 10.1177/0271678X16685574
121. Zaro-Weber O, Fleischer H, Reiblich L, Schuster A, Moeller-Hartmann W, Heiss WD. Penumbra detection in acute stroke with perfusion magnetic resonance imaging: validation with O-15-positron emission tomography. Ann Neurol. (2019) 85:875–86. doi: 10.1002/ana.25479
122. Darwish EAF, Abdelhameed-El-Nouby M, Geneidy E. Mapping the ischemic penumbra and predicting stroke progression in acute ischemic stroke: the overlooked role of susceptibility weighted imaging. Insights Imaging. (2020) 11:11–16. doi: 10.1186/s13244-019-0810-y
123. Lu X, Meng L, Zhou Y, Wang S, Fawaz M, Wang M, et al. Quantitative susceptibility-weighted imaging may be an accurate method for determining stroke hypoperfusion and hypoxia of penumbra. Eur Radiol. (2021) 31:6323–33. doi: 10.1007/s00330-020-07485-2
124. Thomalla G, Simonsen CZ, Boutitie F, Andersen G, Berthezene Y, Cheng B, et al. MRI-guided thrombolysis for stroke with unknown time of onset. N Engl J Med. (2018) 379:611–22. doi: 10.1056/NEJMoa1804355
125. Hill MD, Goyal M, Menon BK, Nogueira RG, McTaggart RA, Demchuk AM, et al. Efficacy and safety of nerinetide for the treatment of acute ischaemic stroke (ESCAPE-NA1): a multicentre, double-blind, randomised controlled trial. Lancet. (2020) 395:878–87. doi: 10.1016/S0140-6736(20)30258-0
126. Menon BK, Ospel JM, McTaggart RA, Nogueira RG, Demchuk AM, Poppe A, et al. Imaging criteria across pivotal randomized controlled trials for late window thrombectomy patient selection. J Neurointervent Surg. (2020). doi: 10.1136/neurintsurg-2020-016902
127. Harston GWJ, Tee YK, Blockley N, Okell TW, Thandeswaran S, Shaya G, et al. Identifying the ischaemic penumbra using pH-weighted magnetic resonance imaging. Brain. (2015) 138:36–42. doi: 10.1093/brain/awu374
128. Heo HY, Zhang Y, Burton TM, Jiang S, Zhao Y, van Zijl PCM, et al. Improving the detection sensitivity of pH-weighted amide proton transfer MRI in acute stroke patients using extrapolated semisolid magnetization transfer reference signals. Magnet Reson Med. (2017) 78:871–80. doi: 10.1002/mrm.26799
129. Gersing AS, Ankenbrank M, Schwaiger BJ, Toth V, Janssen I, Kooijman H, et al. Mapping of cerebral metabolic rate of oxygen using dynamic susceptibility contrast and blood oxygen level dependent MR imaging in acute ischemic stroke. Neuroradiology. (2015) 57:1253–61. doi: 10.1007/s00234-015-1592-7
130. Seiler A, Blockley NP, Deichmann R, Noeth U, Singer OC, Chappell MA, et al. The relationship between blood flow impairment and oxygen depletion in acute ischemic stroke imaged with magnetic resonance imaging. J Cereb Blood Flow Metab. (2019) 39:454–65. doi: 10.1177/0271678X17732448
131. Stone AJ, Harston GWJ, Carone D, Okell TW, Kennedy J, Blockley NP. Prospects for investigating brain oxygenation in acute stroke: experience with a non-contrast quantitative BOLD based approach. Hum Brain Mapp. (2019) 40:2853–66. doi: 10.1002/hbm.24564
132. Zhang S, Cho J, Nguyen TD, Spincemaille P, Gupta A, Zhu W, et al. Initial experience of challenge-free MRI-based oxygen extraction fraction mapping of ischemic stroke at various stages: comparison with perfusion and diffusion mapping. Front Neurosci. (2020) 14:535441. doi: 10.3389/fnins.2020.535441
133. Fan AP, Khalil AA, Fiebach JB, Zaharchuk G, Villringer A, Villringer K, et al. Elevated brain oxygen extraction fraction measured by MRI susceptibility relates to perfusion status in acute ischemic stroke. J Cereb Blood Flow Metab. (2020) 40:539–51. doi: 10.1177/0271678X19827944
134. Brugnara G, Herweh C, Neuberger U, Bo Hansen M, Ulfert C, Mahmutoglu MA, et al. Dynamics of cerebral perfusion and oxygenation parameters following endovascular treatment of acute ischemic stroke. J Neurointervent Surg. (2020). 14:78–83. doi: 10.1136/neurintsurg-2020-017163
135. Park MG, Yeom JA, Baik SK, Park KP. Total mismatch of diffusion-weighted imaging and susceptibility-weighted imaging in patients with acute cerebral ischemia. J Neuroradiol. (2017) 44:308–12. doi: 10.1016/j.neurad.2017.04.002
136. Polan RM, Poretti A, Huisman TAGM, Bosemani T. Susceptibility-weighted imaging in pediatric arterial ischemic stroke: a valuable alternative for the noninvasive evaluation of altered cerebral hemodynamics. Am J Neuroradiol. (2015) 36:783–8. doi: 10.3174/ajnr.A4187
137. Dani KA, Moreton FC, Santosh C, Lopez R, Brennan D, Schwarzbauer C, et al. Oxygen challenge magnetic resonance imaging in healthy human volunteers. J Cereb Blood Flow Metab. (2017) 37:366–76. doi: 10.1177/0271678X15627827
138. Fiat D, Hankiewicz J, Liu S, Trbovic S, Brint S. 17O magnetic resonance imaging of the human brain. Neurol Res. (2004). 26:803–8. doi: 10.1179/016164104X5156
139. Delapaz R, Gupte P. Potential application of 17O MRI to human ischemic stroke. Adv Exp Med Biol. (2011) 701:215–22. doi: 10.1007/978-1-4419-7756-4_29
140. He X, Yablonskiy DA. Quantitative BOLD: mapping of human cerebral deoxygenated blood volume and oxygen extraction fraction: default state. Magnet Reson Med. (2007) 57:115–26. doi: 10.1002/mrm.21108
141. Bouvier J, Detante O, Tahon F, Attye A, Perret T, Chechin D, et al. Reduced CMRO2 and cerebrovascular reserve in patients with severe intracranial arterial stenosis: a combined multiparametric qBOLD oxygenation and BOLD fMRI study. Hum Brain Mapp. (2015) 36:695–706. doi: 10.1002/hbm.22657
142. Christen T, Lemasson B, Pannetier N, Farion R, Segebarth C, Ramy C, et al. Evaluation of a quantitative blood oxygenation level-dependent (qBOLD) approach to map local blood oxygen saturation. NMR Biomed. (2011) 24:393–403. doi: 10.1002/nbm.1603
143. Dimov AV, Christoforidis GA, Saadat N, Liu MM, Jeong YI, Roth S, et al. QSM in canine model of acute cerebral ischemia: a pilot study. Magnet Reson Med. (2021) 85:1602–10. doi: 10.1002/mrm.28498
144. Cho J, Lee J, An H, Goyal MS, Su Y, Wang Y. Cerebral oxygen extraction fraction (OEF): Comparison of challenge-free gradient echo QSM+qBOLD (QQ) with 15O PET in healthy adults. J Cereb Blood Flow Metab. (2021) 41:1658–68. doi: 10.1177/0271678X20973951
145. Ma D, Gulani V, Seiberlich N, Liu K, Sunshine JL, Duerk JL, et al. Magnetic resonance fingerprinting. Nature. (2013) 495:187–92. doi: 10.1038/nature11971
146. Christen T, Pannetier NA, Ni WW, Qiu D, Moseley ME, Schuff N, et al. MR vascular fingerprinting: a new approach to compute cerebral blood volume, mean vessel radius, and oxygenation maps in the human brain. Neuroimage. (2014) 89:262–70. doi: 10.1016/j.neuroimage.2013.11.052
147. Lemasson B, Pannetier N, Coquery N, Boisserand LSB, Collomb N, Schuff N, et al. MR vascular fingerprinting in stroke and brain tumors models. Sci Rep. (2016) 6:37071. doi: 10.1038/srep37071
Keywords: cerebral metabolic rate of oxygen, MRI, PET, ischemic thresholds, penumbra, thrombolysis, thrombectomy
Citation: Chalet L, Boutelier T, Christen T, Raguenes D, Debatisse J, Eker OF, Becker G, Nighoghossian N, Cho T-H, Canet-Soulas E and Mechtouff L (2022) Clinical Imaging of the Penumbra in Ischemic Stroke: From the Concept to the Era of Mechanical Thrombectomy. Front. Cardiovasc. Med. 9:861913. doi: 10.3389/fcvm.2022.861913
Received: 25 January 2022; Accepted: 11 February 2022;
Published: 09 March 2022.
Edited by:
Moritz Wildgruber, LMU Munich University Hospital, GermanyReviewed by:
Paul Reidler, LMU Munich University Hospital, GermanyAnastasios Mpotsaris, München Hospital, Germany
Copyright © 2022 Chalet, Boutelier, Christen, Raguenes, Debatisse, Eker, Becker, Nighoghossian, Cho, Canet-Soulas and Mechtouff. This is an open-access article distributed under the terms of the Creative Commons Attribution License (CC BY). The use, distribution or reproduction in other forums is permitted, provided the original author(s) and the copyright owner(s) are credited and that the original publication in this journal is cited, in accordance with accepted academic practice. No use, distribution or reproduction is permitted which does not comply with these terms.
*Correspondence: Laura Mechtouff, bGF1cmEubWVjaHRvdWZmJiN4MDAwNDA7Y2h1LWx5b24uZnI=