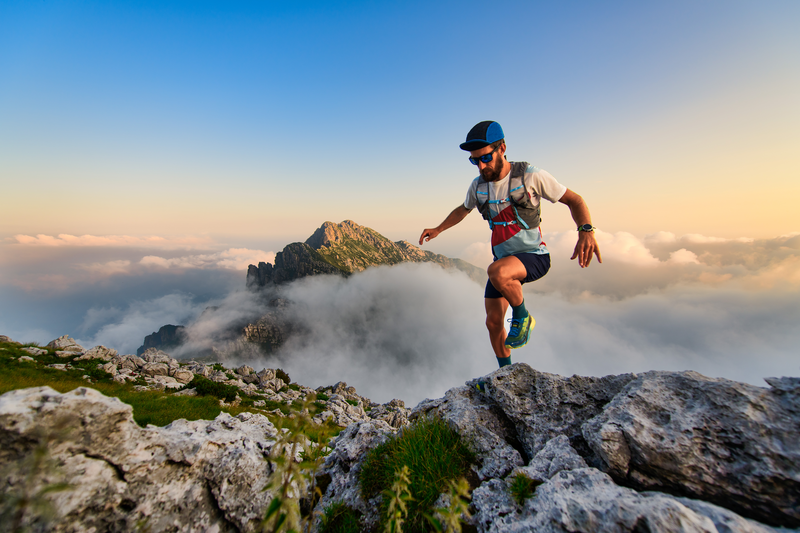
94% of researchers rate our articles as excellent or good
Learn more about the work of our research integrity team to safeguard the quality of each article we publish.
Find out more
SYSTEMATIC REVIEW article
Front. Cardiovasc. Med. , 15 April 2022
Sec. Cardiovascular Biologics and Regenerative Medicine
Volume 9 - 2022 | https://doi.org/10.3389/fcvm.2022.857364
This article is part of the Research Topic Insights in Cardiovascular Biologics and Regenerative Medicine: 2021 View all 7 articles
Objective: Effective interventions that might limit myocardial ischemia-reperfusion (I/R) injury are still lacking. Coenzyme Q10 (CoQ10) may exert cardioprotective actions that reduce myocardial I/R injury. We conducted this meta-analysis to assess the potential cardioprotective effect of CoQ10 in animal models of myocardial I/R injury.
Methods: We searched PubMed and Embase databases from inception to February 2022 to identify animal studies that compared the effect of CoQ10 with vehicle treatment or no treatment on myocardial infarct size in models of myocardial I/R injury. Means and standard deviations of the infarct size measurements were pooled as the weighted mean difference with 95% confidence interval (CI) using the random-effects model. Subgroup analyses were also conducted according to animals' species, models' type, and reperfusion time.
Results: Six animal studies (4 in vivo and 2 ex vivo) with 116 animals were included. Pooled analysis suggested that CoQ10 significantly reduced myocardial infarct size by −11.36% (95% CI: −16.82, −5.90, p < 0.0001, I2 = 94%) compared with the control group. The significance of the pooled effect estimate was maintained in rats, Hartley guinea pigs, and Yorkshire pigs. However, it became insignificant in the subgroup of rabbits −5.29% (95% CI: −27.83, 17.26; I2 = 87%). Furthermore, CoQ10 significantly reduced the myocardial infarct size regardless of model type (either in vivo or ex vivo) and reperfusion time (either ≤ 4 h or >4 h).
Conclusion: Coenzyme Q10 significantly decreased myocardial infarct size by 11.36% compared with the control group in animal models of myocardial I/R injury. This beneficial action was retained regardless of model type and reperfusion time.
Ischemic heart disease (IHD) is still the leading cause of death worldwide (1). A total of 197 million cases and 9.14 million deaths of IHD have been recorded globally in 2019 (1). Acute myocardial infarction (AMI) is the most serious form of IHD that is caused by decreased or complete cessation of the blood supply to a part of the cardiac muscle leading to ischemia and infarction of the affected portion. The American Heart Association (AHA) estimates that one American will experience an event of AMI nearly every 40 s and about 14% of AMI cases will result in death (2). Despite the progress in myocardial reperfusion methods over the past decade (e.g., pharmacological thrombolysis and percutaneous coronary intervention [PCI]), the mortality and morbidity associated with AMI and its sequelae (e.g., heart failure [HF]) are still significant (3, 4). One important flaw of reperfusion strategies is the development of myocardial ischemia-reperfusion (I/R) injury, which eventually constitutes up to 50% of the infarct size (3, 5). The exact pathophysiology of I/R injury is not completely understood (6). However, possible underlying mechanisms include mitochondrial damage, oxidative stress, inflammation, and excess calcium (7–9). Infarct size is a crucial prognostic factor in patients with AMI (10). Therefore, there is a large need for effective cardioprotective approaches that aim for infarct size limitation.
Coenzyme Q10 (CoQ10), also known as ubiquinone, is a lipophilic benzoquinone that presents in the cell membranes all over the body, particularly in the mitochondria (11). It plays a key role in ATP production through the electron transport chain and oxidative phosphorylation (12). It also exhibits antioxidant and membrane-stabilizing functions inside and outside the mitochondria (13, 14). Since the heart is a very active organ that requires much energy, it normally contains high levels of CoQ10 (15). Low levels of myocardial CoQ10 have been observed in many cardiac diseases such as IHD, cardiomyopathy, and chronic HF (16–19). In multiple reports, CoQ10 reduced creatine kinase (CK) leakage during myocardial I/R injury (20–22). Therefore, CoQ10 may represent a promising cardioprotective agent in case of cardiac I/R injury. We conducted this systematic review and meta-analysis to assess the potential cardioprotective effect of CoQ10 and its related molecular mechanisms in myocardial I/R injury in animal studies.
We followed the preferred reporting items for systematic reviews and meta-analyses (PRISMA) during this study preparation (Supplementary Table 1). The protocol of this meta-analysis was not prospectively registered.
We systematically searched both PubMed and Embase databases from inception to February 2022 using a combination of related keywords and MeSH terms as follows: (Coenzyme Q10 OR CoQ10 OR Ubiquinone OR “Ubiquinone”[Mesh]) AND (infarct OR infarction OR myocardial infarction OR myocardial injury OR myocardial necrosis OR myocardial death OR “Myocardial Infarction”[Mesh]) AND (size OR area OR region OR part OR portion OR zone). We did not use any restriction filters throughout the search. We also manually searched related review articles for potential missing studies (23).
Experimental studies were included if they met the following predefined criteria: (1) being an animal study on experimental models of myocardial I/R injury (either in vivo or ex vivo), (2) compared CoQ10 (either ubiquinol or ubiquinone form) with vehicle treatment or no treatment, and (3) data on myocardial infarct size, defined as the percentage of infarct zone over the area at risk or the total ventricular myocardium, were reported in both groups.
Exclusion criteria were as follows: (1) in vitro studies, (2) studies that included animals with cardiovascular (CV) comorbidities (e.g., obesity and diabetes mellitus), (3) studies with non-English or inaccessible text, (4) retracted studies that contained false or fabricated data, and (5) studies that missed any of the inclusion criteria.
The following information was extracted from each study: (1) first author's name, (2) publication year, (3) study location, (4) animal characteristics (i.e., species, weight, age, and sex), (5) treatment group characteristics (i.e., sample size, vehicle type, intervention dose, duration, and route of administration), (6) type of used anesthesia, (7) methods of model preparation, (8) data on the infarct size in each group, and (9) data on secondary outcomes assessing the cardiac function that included left ventricular ejection fraction (LVEF), LV developed pressure (LVDP), and LV dP/dtmax.
The risk of bias was assessed using the SYRCLE's risk of bias tool (24). This tool includes 10 domains as follows: (1) sequence generation, (2) baseline characteristics, (3) allocation concealment, (4) random housing, (5) blinding of the investigator, (6) random outcome assessment, (7) blinding of the outcome assessor, (8) incomplete outcome data, (9) selective outcome reporting, and (10) other sources of bias. Each domain is judged either the low, high, or unclear risk of bias. Disagreements were settled by discussion.
Means and standard deviations (SDs) of the infarct size measurements in the included studies were pooled as weighted mean difference (WMD) with 95% confidence interval (CI) using the DerSimonian and Laird random-effects model to address inter-study heterogeneity. If one of the included studies reported standard error (SE) instead of SD, we calculated SD using the formula: SD = SE × square root (sample size) (25). Heterogeneity was judged by visual inspection of the generated forest plot and measured by both I2 and χ2 tests. To test the result's robustness, leave-one-out sensitivity analysis was applied by removing one study successively and performing the analysis again. To address potential heterogeneity, we also conducted subgroup analyses according to animals' species, models' type (either in vivo or ex vivo), and reperfusion time (either ≤ 4 h or >4 h).
Publication bias was judged by Begg's funnel plot and Egger's test (26). In addition, the trim and fill approach was applied in the case of an asymmetrical funnel plot to adjust for the potential publication bias (27). All analyses were done by RevMan version 5.3 (The Cochrane Collaboration, Oxford, UK) and Comprehensive Meta-Analysis version 3 (Biostat, New Jersey, USA).
We identified 330 records through the literature search. Of them, 300 records remained after the exclusion of duplicates. Initial title/abstract screening resulted in 13 potentially relevant studies. Following the more detailed screening of their full texts, 6 relevant animal studies (4 in vivo and 2 ex vivo) were finally included in this systematic review and meta-analysis (28–33). All steps of study selection are summarized in Figure 1.
A total of 116 animals were enrolled in the included studies. Each relevant group (i.e., CoQ10 and control groups) included 58 animals. Four included studies (28, 30, 32, 33) were conducted in the United States, one (31) in China, and one (29) in India. The year of publication ranged from 1996 to 2017. Two of the included studies (28, 33) used New Zealand White rabbits, two used (29, 31) rats (Sprague Dawley or Wistar), one (30) used Hartley guinea pigs, and one (32) used Yorkshire pigs. All included animals were males. Ischemia was induced by blockage of the left anterior descending (LAD) artery in two studies (31, 32), the left main coronary artery in two studies (29, 33), the left circumflex artery in one study (28), and the aorta/atria in one study (30). Infarct size was reported in all included studies as a percentage of the area at risk except for one study as a percentage of the total left ventricle (32). More details on the characteristics of the included studies are shown in Table 1.
According to SYRCLE's risk of bias tool, all included studies showed a low risk of bias concerning the following three domains: baseline characteristics, selective outcome reporting, and other sources of bias. No studies reported any information about sequence generation, allocation concealment, random housing, blinding of the investigator, or random outcome assessment. One study by Liang et al. (31) reported blinding of the outcome assessor. A study by Khan et al. (29) was at a high risk of bias due to incomplete outcome data reporting. The risk of bias assessment is summarized in Table 2.
Pooled analysis of 6 studies including 116 animals revealed that CoQ10 significantly reduced myocardial infarct size by −11.36% (95% CI: −16.82, −5.90, p < 0.0001, Figure 2) compared with the control group in experimental models of myocardial I/R injury. Significant between-study heterogeneity was observed in this meta-analysis (χ2 = 132.87, p < 0.00001, I2 = 94%). The significance of the pooled effect estimate did not alter when we applied the leave-one-out sensitivity analysis, indicating the robustness of the observed result.
Figure 2. Forest plot displaying the results of meta-analysis of coenzyme Q10 effect on myocardial infarct size in models of myocardial ischemia-reperfusion injury compared with the control group. CI, confidence interval; df, degrees of freedom; SD, standard deviation; IV, inverse variance.
In subgroup analysis, according to included animal species, the significance of the pooled effect estimate was maintained in rats, Hartley guinea pigs, and Yorkshire pigs. However, it became insignificant in the subgroup including rabbits −5.29% (95% CI: −27.83, 17.26; I2 = 87%; Table 3). Moreover, CoQ10 significantly reduced the myocardial infarct size regardless of model type (either in vivo −10.14% [95% CI: −16.22 to −4.07] or ex vivo −15.81% [95% CI: −21.76 to −9.85]) and reperfusion time (either ≤ 4 h −13.43% [95% CI: −26 to −0.85] or >4 h −4.93% [95% CI: −7.78 to −2.08]).
Significant heterogeneity was observed in all studied subgroups except for the subgroup included ex vivo studies (I2 = 0%; χ2, p = 0.848). All details on subgroup analyses are summarized in Table 3.
Data on LVEF were reported only in the study by Liang et al. (31). Significant improvement in LVEF was observed in CoQ10 group (mean: [SD] 67.12 [6.18]) compared with the control group 59.12 (5.81). Data on LVDP were reported in the studies by Lekli et al. (30) and Maulik et al. (32), while data on LV dP/dtmax were reported in the studies by Maulik et al. (32) and Liang et al. (31). Recovery of LVDP and LV dP/dtmax was significantly better in CoQ10 group compared with the control group. The summary of data on cardiac function outcomes is shown in Table 4.
Visual inspection of the generated funnel plot suggested potential publication bias in the observed result (Figure 3). The trim and fill approach adjusted the pooled effect estimate for this potential bias as follows: −12.78% (95% CI: −18.23, −7.32) by imputing one study to the left of its mean. The significance of the effect estimate was not changed after this adjustment. On the contrary, Egger's test detected insignificant publication bias in the current analysis (two-tailed p-value = 0.18).
Figure 3. Corrected funnel plot showing publication bias in animal studies that compared the effect of coenzyme Q10 with vehicle treatment or no treatment on myocardial infarct size in models of myocardial ischemia-reperfusion injury.
To the best of our knowledge, this is the first meta-analysis to explore the potential cardioprotective limiting effect on infarct size of CoQ10 in myocardial I/R injury. Our meta-analysis of 6 studies including 116 experimental models of myocardial I/R injury suggested that CoQ10 significantly decreased myocardial infarct size by 11.36% compared with the control group. In addition, this beneficial effect was preserved irrespective of model type (either in vivo or ex vivo) and reperfusion time (either ≤ 4 h or >4 h). Likewise, the significant improvement of cardiac function parameters (e.g., LVEF and LVDP) with CoQ10 was observed in multiple included studies. These results are of interest in the context of involved molecular mechanisms and their implications in informing future research on the promise of CoQ10 as a cardioprotective agent.
There are a number of different mechanisms, which may account for the cardioprotective effect of CoQ10 seen in our analysis. First, CoQ10 has been shown in numerous studies to act as an antioxidant, increasing the levels of superoxide dismutase and glutathione and decreasing the levels of lipid peroxidation (31). This antioxidative activity is crucial as oxidative stress is believed to play a significant role in myocardial I/R injury (3). Following reperfusion of an ischemic heart, there is an increased production of free oxygen radicals that induces further cellular damage. Specifically, dysfunction of the mitochondrial electron transport chain results in the increased production of free oxygen radicals (34). These radicals then damage cardiolipin, an important component of the inner mitochondrial membrane (35). Cardiolipin damage may precipitate further leakage of electrons from the mitochondria (36), leading to the formation of greater amounts of superoxide anion radicals, therefore precipitating a vicious cycle that causes severe cellular damage (37). In multiple studies, CoQ10 inhibited oxidative inactivation of CK and reduced its leakage during myocardial I/R insult (21, 22, 38, 39).
Additionally, CoQ10 has been shown to reduce the levels of p53 (31), which is a well-recognized pro-apoptotic protein (40). P53 exerts its pro-apoptotic effect by enhancing the transcription of a group of pro-apoptotic members of the bcl-2 family, named BH3-only proteins (40–42). These proteins inhibit the anti-apoptotic members of the bcl-2 family and may enhance other pro-apoptotic bcl-2 proteins such as BAX and BAK (43). Therefore, by inhibiting the activity of p53, CoQ10 may reduce cellular apoptosis and thus reduce infarct size. In addition to its ability to diminish pro-apoptotic activity, CoQ10 has also been shown to increase the gene expression of anti-apoptotic bcl-2, leading to decreased apoptotic activity and preserved cellular structures in the setting of I/R injury (29). Besides, Khan et al. (29) observed that CoQ10 reduced apoptotic DNA levels through inhibition of caspase-9 and cytochrome-C release into the cytoplasm.
It has been reported that CoQ10 increased the levels of adenosine triphosphate (ATP) and creatine phosphate and enhanced the aerobic efficiency of the myocardium in I/R injury (31, 39). Increased production of nitric oxide was also observed with CoQ10 resulting in coronary vasodilatation (29).
Autophagy is a vital protective pathway that acts by the self-ingestion of damaged proteins and organelles (44). Owing to this pathway, minimal levels of energy may be sufficient for cell survival under stress conditions such as I/R injury (44, 45). Therefore, enhanced autophagy may be essential for cardioprotection in I/R injury (45, 46). It has been reported that CoQ10 increased several proteins responsible for the activity of autophagy such as Atg5, beclin-1, and LC-3II/LC3-I ratio (31). It also has been involved in the regulation of mitochondrial autophagy (through increasing levels of LC3-II, PINK, and parkin) and attenuation of mitochondrial dysfunction (47). Moreover, CoQ10 has been shown to increase the expression of ubiquitin proteins in I/R animal models (32). The ubiquitin-proteasome system is important for a cell to degrade its own dysfunctional contents. The system works as follows: dysfunctional substances are tagged by ubiquitin proteins, in a process called ubiquitination. Then, proteosomes recognize these tags and subsequently remove the dysfunctional constituents of the cell (48–50). Importantly, the coupling of ubiquitin to proteasomal activity requires so-called ubiquitin receptors, which recruit the ubiquitinated protein to the proteasome for degradation (51).
In I/R injury, oxidative stress leads to the formation of dysfunctional oxidized proteins, and it is the proteasome (particularly the 20S proteasome), which is primarily responsible for the removal of such hazardous proteins (52). Accordingly, it is not surprising that recent research has shown that inhibiting the proteasome system exacerbates I/R injury (53). In addition, Hu et al. have recently shown that knocking out ubiquilin 1, a ubiquitin receptor, in I/R mice models led to an accumulation of ubiquitinated proteins, ultimately resulting in larger infarct size compared to mice with increased ubiquilin 1 activity, in whom infarct area was smaller (54).
Furthermore, Tian et al. have demonstrated that pharmacological proteasomal inhibition leads to increased activation of protein kinase C delta (PKCδ) and decreased activation of PKCε (53). The changes in the ratios of these two isozymes, through their effects on mitochondrial functions, lead to increased apoptosis and thus exacerbate I/R injury (53, 55–57). In sum, these findings suggest that CoQ10, by increasing the levels of ubiquitin proteins, may enhance proteasomal activity, decrease apoptotic activity, and ultimately conserve myocardial cells after I/R injury.
Coenzyme Q10 has also been found to reduce levels of angiotensin-converting enzyme (ACE) in patients following MI (58). This is important for two reasons: first, ACE is a known inducer of remodeling following MI (59); therefore, by reducing ACE levels, CoQ10 reduces remodeling and preserves cardiac function. Second, inhibition of ACE can reduce the afterload imposed on the heart, thereby alleviating adverse structural cardiac changes (60). Coenzyme Q10 has been found to reduce peripheral resistance and thus afterload (61), which may be partially mediated by its effect on ACE. Supporting this postulate is a meta-analysis showing an attenuated benefit of CoQ10 in cohorts using ACE inhibitors (62), which suggests that CoQ10 exerts some of its beneficial effects, at least partially, by its effect on the renin-angiotensin-aldosterone system. All involved potential mechanisms of action are summarized in Figure 4.
Figure 4. Summary of involved potential mechanisms of action of coenzyme Q10 reducing effect on myocardial infarct size in models of myocardial ischemia-reperfusion injury. SOD, superoxide dismutase; GSH, glutathione; CK, creatine kinase; UPS, ubiquitin-proteasome system; ACE, angiotensin-converting enzyme; NO, nitric oxide; VD, vasodilatation; ATP, adenosine triphosphate; PCr, phosphocreatine; CoQ10, coenzyme Q10; IS, infarct size; CI, confidence interval. This figure was produced on diagrams.net, using SMART materials (Servier Medical Art; smart.servier.com) that are approved under a Creative Commons Attribution 3.0 Unported License.
In line with our results, the benefits of CoQ10 in terms of cardioprotection/CV prevention in MI (and other CV diseases) have been observed in clinical studies; however, its effect on the infarct size has not been investigated in humans. In a double-blind randomized controlled trial (RCT), Singh et al. (58) assessed the effects of CoQ10 (120 mg/day for 24 weeks), compared with placebo, on parameters of left ventricular remodeling in 55 patients with post-MI LVEF <50%. This study revealed that CoQ10 significantly reduced the wall thickness opposite the infarction site from (mean [SD]) 12.2 (2) to 10 (1.8) mm compared with placebo (p < 0.01). It also significantly suppressed changes in the sphericity index and wall thickening at the infarction site. Huang et al. (63) reported that higher plasma levels of CoQ10, measured 1 month after primary PCI, were associated with better left ventricular performance/remodeling after 6 months of follow-up in 55 patients with ST-segment elevation MI (STEMI). Low plasma levels of CoQ10 have been observed in patients with cardiomyopathy (17, 64). In a cohort of 236 patients with chronic HF, lower levels of CoQ10 were associated with the increased risk of all-cause mortality (hazard ratio [HR]: 1.99; 95% CI: 1.21–3.30, p = 0.007) (18). In patients with CV disease admitted to the coronary care unit, low plasma CoQ10 (less than 0.59 mg/L, or 0.46 mg/L) was an independent predictor of both in-hospital and long-term mortality (65, 66). In another RCT including 144 patients with AMI, CoQ10 (120 mg/day) was compared with placebo for 28 days in terms of CV prevention (67). In comparison with placebo, CoQ10 significantly reduced total cardiac events (15 vs. 30.9%, p = 0.02), angina pectoris (9.5 vs. 28.1%, p < 0.05), total arrhythmias (9.5 vs. 25.3%, p < 0.05), and poor left ventricular function (8.2 vs. 22.5%, p < 0.05). In 2003, Singh et al. (68) also compared, in a double-blind RCT, the effect of CoQ10 (120 mg/day) with vitamin B for 1 year on CV events in 144 patients with recent AMI. Coenzyme Q10 significantly reduced the total cardiac events (24.6 vs. 45.0%, p < 0.02) and non-fatal MI (13.7 vs. 25.3%, p < 0.05) compared with vitamin B. In a meta-analysis of 8 clinical trials with 327 patients undergoing cardiac surgery with cardiopulmonary bypass, CoQ10 (30–600 mg/day) for 12 h to 14 days before surgery significantly reduced inotropic drugs requirement and incidence of ventricular arrhythmias after surgery, with no significant effect in terms of cardiac index, the incidence of atrial fibrillation, or duration of hospital stay (69). In a recent Cochrane review that included 11 RCTs with 1,573 patients with HF, CoQ10 reduced all-cause mortality (risk ratio [RR]: 0.58; 95% CI: 0.35–0.95) and HF-related hospitalization (RR: 0.62; 95% CI: 0.49–0.78) compared with the control group (70). A significant improvement in LVEF was also observed with CoQ10 supplementation in comparison with the control group (MD: 1.77; 95% CI: 0.09–3.44) (70). In a cohort of 443 Swedish healthy elderly individuals, CoQ10 (200 mg/day) combined with selenium (200 μg/day) for 4 years resulted in reduced CV death compared with placebo (5.9 vs. 12.6%; p = 0.015), a favorable effect that persisted for 10 years after the intervention (HR: 0.51; 95% CI: 0.36–0.74, p = 0.0003) (71, 72). Multiple RCTs reported, in line with the previous results, significant improvements in the quality of life of chronic HF patients with CoQ10 supplementation (alone or combined with other micronutrients) compared with placebo (73, 74).
Myocardial infarct size has been identified as an important prognostic parameter in MI (10). In a patient-level meta-analysis including 2,632 patients from 10 randomized primary PCI trials, myocardial infarct size measured within 1 month after PCI was significantly associated with the increased risk of all-cause mortality (HR: 1.19; 95% CI: 1.18–1.20, p < 0.0001) and hospitalization for HF (HR: 1.20; 95% CI: 1.19–1.21, p < 0.0001) for every 5% increase. Therefore, infarct size reduction may be a clinically plausible explanation for the above-mentioned promising results of CoQ10 as a cardioprotective agent.
Experimental studies explored the cardioprotective potential of numerous antioxidant agents (e.g., vitamins C and E, N-acetyl cysteine, and allopurinol) based on the central role of oxidative stress in myocardial I/R injury (75, 76). In fact, several preclinical studies have shown promising results with these agents. For example, Ferrari et al. (77) reported that vitamin E infusion in isolated rabbit hearts (20 min before hypoxia) decreased the depletion of ATP and CP and preserved the mitochondrial function and the myocardium ultrastructure. A combination of vitamins C and E reduced infarct size in ischemic, reperfused pigs' heart by LAD artery ligation for 45 min followed by 3 days of reperfusion (78). N-acetyl cysteine (a glutathione precursor, 100 mg/kg), given 2 h after LAD artery ligation followed by 2 h of reperfusion in dogs, significantly reduced ventricular arrhythmias and myocardial infarct size (37 [12.6%]) compared with the control group (55 [7.0%]) (79). Allopurinol, a xanthine oxidase inhibitor, was reported to enhance coronaries relaxation (80) and limit myocardial infarct size in dogs (81, 82) and also in rats (83). Despite these positive results of antioxidants in animal studies, results from large-scale clinical studies on cardioprotection were disappointing (84). Long-term supplementation of vitamins E and C in clinical trials did not show benefit in terms of CV prevention (85–89). In a relatively large RCT that included 251 patients with STEMI undergoing PCI, N-acetyl cysteine did not show any clinical benefit in terms of CV prevention or with respect to myocardial I/R injury limitation compared with placebo (90). As for allopurinol, multiple small trials have shown positive cardioprotective results (e.g., decreased incidence of arrhythmia and improved myocardial efficiency and cardiac function) in patients undergoing coronary artery bypass grafting and in cardiomyopathy (91–93). However, other several trials failed to show benefit (94–97). It has always been a challenge to translate the results of experimental animal studies to human clinical studies (98). Lack of reproducibility of experimental studies, methodological defects (e.g., selection and performance bias), and large disparities (e.g., presence of comorbidities, different cardioprotective endpoints [i.e., infarct size vs. mortality rate] and inconsistency in dosing and timing of the intervention) in study design between animal experiments and clinical studies are possible causes of translational failure (84, 99, 100). Although low methodological quality may apply to the included animal studies, the combination of consistent evidence derived from animal and clinical studies suggests an important role for CoQ10 as a cardioprotective molecule following MI. However, large well-designed RCTs with longer durations of follow-up are warranted to further assess the potential cardioprotective benefits of CoQ10 in MI.
Animal studies play a critical role in understanding molecular mechanisms in a variety of diseases. However, there are large anatomical and physiological differences between used animal species and humans, especially with smaller animal models (101). Most of the included studies in this meta-analysis are based on small animal models. Thus, more high-quality animal studies on CoQ10's cardioprotective effects in larger models of myocardial I/R injury are still needed for better assessment of the suitable dosing and timing of CoQ10 and understanding of the involved mechanisms of action (101). Human equivalent doses of CoQ10 (based on body surface area) that ranged from 9.7 to 233 mg/day, for an adult person weighting 60 kg, were used in the included studies (102). However, higher doses of CoQ10, preferably given through intravenous or intracoronary routes, should be considered/assessed in future studies for multiple reasons. First, because of its relatively high molecular weight (863.34 g/mol) and insolubility in water, poor oral bioavailability has been a limitation for CoQ10 supplementation, which may become more evident in large MI complicated by peripheral hypoperfusion (23, 103). In rats, only a small part of orally supplemented CoQ10 was found to reach the circulation, spleen, and liver with none reached the heart or kidney (104, 105). However, CoQ10 as a lipid microsphere given intravenously reached both the heart and kidney as well as other tissues in rats (104, 106). Second, CoQ10 supplementation was found to be highly safe (107). In patients with Parkinson's disease, doses of 1,200 mg/day, and even 2,400 mg/day were well tolerated, compared with placebo (108). Finally, statins are commonly used drugs in patients with IHD that have been observed to additionally reduce CoQ10 levels (109). Therefore, considering higher doses of CoQ10 in patients on statins is reasonable. According to the included studies, pretreatment with CoQ10 for 3–30 days before induction of I/R injury seems to be favorable for prophylaxis of MI. Other timings, which may be more applicable in patients with unpredictable acute event (i.e., before or at early reperfusion), should be adequately assessed in future preclinical studies (3). Nevertheless, as mentioned before, higher doses of CoQ10 administered through intravenous or intracoronary routes may be needed in these timings to effectively increase the heart concentrations of CoQ10 (21). More animal studies on CoQ10 cardioprotective potential with a background of other comorbidities (e.g., hypertension, obesity, and diabetes mellitus) are needed for a better clinical insight. In addition, proof-of-concept clinical trials should include infarct size as an endpoint when assessing CoQ10's cardioprotective effects (10).
Our meta-analysis has some limitations. First, significant heterogeneity was observed in the current analysis. Variability in animals' characteristics and methodological differences (e.g., model type, risk of bias sources, and method of ischemia) among the included studies may explain this heterogeneity. Nevertheless, random-effects model and subgroup analyses were applied to address this heterogeneity. Second, potential publication bias was suggested by funnel plot visual inspection. However, the trim and fill approach was used to adjust for this bias. Third, most of the included studies were based on smaller animal models, which are less similar to humans compared with larger ones. Fourth, data on cardiac function were not adequately reported in the included studies. Fifth, all included studies did not report any information about multiple domains of bias assessment (e.g., sequence generation and allocation concealment). Sixth, infarct size assessments were short-term, ranging from 45 min to 72 h after reperfusion. Finally, all included animals were without CV comorbidity, not reflecting cases of MI in clinical practice that may have multiple CV risk factors (e.g., obesity, diabetes mellitus, and hypertension).
Coenzyme Q10 significantly decreased myocardial infarct size by 11.36% compared with the control group in animal models of myocardial I/R injury. Additionally, this beneficial action was retained regardless of model type (either in vivo or ex vivo) and reperfusion time (either ≤ 4 h or >4 h). Significant improvements in cardiac function parameters were also observed with CoQ10. High-quality large animal studies are still needed to confirm these results and to further explore the involved mechanisms. Moreover, these results provide the rationale for future large well-designed RCTs with longer durations of follow-up to assess their translation into clinical application.
The original contributions presented in the study are included in the article/Supplementary Material, further inquiries can be directed to the corresponding author/s.
KA helped in literature search, screening, data extraction, data analysis, and manuscript writing. AS helped in screening, data extraction, and manuscript writing. MB helped in study design, supervision, coordination, manuscript writing, and revision. All authors have approved the final article.
The authors declare that the research was conducted in the absence of any commercial or financial relationships that could be construed as a potential conflict of interest.
All claims expressed in this article are solely those of the authors and do not necessarily represent those of their affiliated organizations, or those of the publisher, the editors and the reviewers. Any product that may be evaluated in this article, or claim that may be made by its manufacturer, is not guaranteed or endorsed by the publisher.
The Supplementary Material for this article can be found online at: https://www.frontiersin.org/articles/10.3389/fcvm.2022.857364/full#supplementary-material
1. Roth GA, Mensah GA, Johnson CO, Addolorato G, Ammirati E, Baddour LM, et al. Global burden of cardiovascular diseases and risk factors, 1990–2019: update from the GBD 2019 study. J Am Coll Cardiol. (2020) 76:2982–3021. doi: 10.1016/j.jacc.2020.11.010
2. Benjamin EJ, Muntner P, Alonso A, Bittencourt MS, Callaway CW, Carson AP, et al. Heart disease and stroke statistics-2019 update: a report from the American Heart Association. Circulation. (2019) 139:e56–e528. doi: 10.1161/CIR.0000000000000659
3. Heusch G. Myocardial ischaemia–reperfusion injury and cardioprotection in perspective. Nat Rev Cardiol. (2020) 17:773–89. doi: 10.1038/s41569-020-0403-y
4. Lee KL, Woodlief LH, Topol EJ, Weaver WD, Betriu A, Col J, et al. Predictors of 30-day mortality in the era of reperfusion for acute myocardial infarction: results from an international trial of 41 021 patients. Circulation. (1995) 91:1659–68. doi: 10.1161/01.CIR.91.6.1659
5. Hausenloy DJ, Yellon DM. Myocardial ischemia-reperfusion injury: A neglected therapeutic target. J Clin Invest. (2013) 123:92–100. doi: 10.1172/JCI62874
6. Turer AT, Hill JA. Pathogenesis of myocardial ischemia-reperfusion injury and rationale for therapy. Am J Cardiol. (2010) 106:360–8. doi: 10.1016/j.amjcard.2010.03.032
7. Eltzschig HK, Eckle T. Ischemia and reperfusion-from mechanism to translation. Nat Med. (2011) 17:1391–401. doi: 10.1038/nm.2507
8. Kwon JS, Kim YS, Cho AS, Cho HH, Kim JS, Hong MH, et al. The novel role of mast cells in the microenvironment of acute myocardial infarction. J Mol Cell Cardiol. (2011) 50:814–25. doi: 10.1016/j.yjmcc.2011.01.019
9. Herrmann J. Peri-procedural myocardial injury: 2005 Update. Eur Heart J. (2005) 26:2493–519. doi: 10.1093/eurheartj/ehi455
10. Stone GW, Selker HP, Thiele H, Patel MR, Udelson JE, Ohman EM, et al. Relationship between infarct size and outcomes following primary pci patient-level analysis from 10 randomized trials. J Am Coll Cardiol. (2016) 67:1674–83. doi: 10.1016/j.jacc.2016.01.069
11. Kommuru TR, Ashraf M, Khan MA, Reddy IK. Stability and bioquivalence studies of two marketed formulations of coenzyme Q10 in beagle dogs. Chem Pharm Bull. (1999) 47:1024–8.
12. Ernster L, Dallner G. Biochemical, physiological and medical aspects of ubiquinone function. Biochim Biophys Acta (BBA)-Molecular Basis Dis. (1995) 1271:195–204.
13. Greenberg S, Frishman WH. Co-enzyme Q10: A new drug for cardiovascular disease. J Clin Pharmacol. (1990) 30:596–608.
14. Overvad K, Diamant B, Holm L, Hølmer G, Mortensen SA, Stender S. Coenzyme Q 10 in health and disease. Eur J Clin Nutr. (1999) 53:764–70.
15. Åberg F, Appelkvist E-L, Dallner G, Ernster L. Distribution and redox state of ubiquinones in rat and human tissues. Arch Biochem Biophys. (1992) 295:230–4. doi: 10.1016/0003-9861(92)90511-T
16. Kalén A, Appelkvist E-L, Dallner G. Age-related changes in the lipid compositions of rat and human tissues. Lipids. (1989) 24:579–84.
17. Seneş M, Erbay AR, Yilmaz FM, Topkaya BÇ, Zengi O, Dogan M, et al. Coenzyme Q10 and high-sensitivity C-reactive protein in ischemic and idiopathic dilated cardiomyopathy. Clin Chem Lab Med. (2008) 46:382–6. doi: 10.1515/CCLM.2008.061
18. Molyneux SL, Florkowski CM, George PM, Pilbrow AP, Frampton CM, Lever M, et al. Coenzyme Q10: an independent predictor of mortality in chronic heart failure. J Am Coll Cardiol. (2008) 52:1435–41. doi: 10.1016/j.jacc.2008.07.044
19. Mortensen SA. Perspectives on therapy of cardiovascular diseases with oenzyme Q 10 (Ubiquinone). Clin Investig. (1993) 71:S116–23.
20. Konishi T, Nakamura Y, Konishi T, Kawai C. Improvement in recovery of left ventricular function during reperfusion with coenzyme Q10 in isolated working rat heart. Cardiovasc Res. (1985) 19:38–43. doi: 10.1093/cvr/19.1.38
21. Sunamori M, Tanaka H, Maruyama T, Sultan I, Sakamoto T, Suzuki A. Clinical experience of coenzyme Q10 to enhance intraoperative myocardial protection in coronary artery revascularization. Cardiovasc Drugs Ther. (1991) 5:297–300. doi: 10.1007/BF00054751
22. Chello M, Mastroroberto P, Romano R, Bevacqua E, Pantaleo D, Ascione R, et al. Protection by coenzyme Q10 from myocardial reperfusion injury during coronary artery bypass grafting. Ann Thorac Surg. (1994) 58:1427–32. doi: 10.1016/0003-4975(94)91928-3
23. Martelli A, Testai L, Colletti A, Cicero AFG. Coenzyme Q10: clinical applications in cardiovascular diseases. Antioxidants. (2020) 9:41. doi: 10.3390/antiox9040341
24. Hooijmans CR, Rovers MM, de Vries RBM, Leenaars M, Ritskes-Hoitinga M, Langendam MW. SYRCLE's risk of bias tool for animal studies. BMC Med Res Methodol. (2014) 14:43. doi: 10.1186/1471-2288-14-43
25. Altman DG, Bland JM. Standard deviations and standard errors. BMJ. (2005) 331:903. doi: 10.1136/bmj.331.7521.903
26. Egger M, Davey Smith G, Schneider M, Minder C. Bias in meta-analysis detected by a simple, graphical test. BMJ. (1997) 315:629–34.
27. Duval S, Tweedie R. Trim and fill: A simple funnel-plot-based method of testing and adjusting for publication bias in meta-analysis. Biometrics. (2000) 56:455–63.
28. Birnbaum Y, Hale SL, Kloner RA. The effect of coenzyme Q10 on infarct size in a rabbit model of ischemia/reperfusion. Cardiovasc Res. (1996) 32:861–868. doi: 10.1016/0008-6363(96)00127-7
29. Khan N, Abid M, Ahmad A, Abuzinadah M, Basheikh M, Kishore K. Cardioprotective effect of coenzyme Q10on apoptotic myocardial cell death by regulation of bcl-2 gene expression. J Pharmacol Pharmacother. (2017) 8:122–7. doi: 10.4103/jpp.JPP_47_17
30. Lekli I, Das S, Das S, Mukherjee S, Bak I, Juhasz B, et al. Coenzyme Q9 provides cardioprotection after converting into coenzyme Q10. J Agric Food Chem. (2008) 56:5331–7. doi: 10.1021/jf800035f
31. Liang S, Ping Z, Ge J. Coenzyme Q10 regulates antioxidative stress and autophagy in acute myocardial ischemia-reperfusion injury. Oxid Med Cell Longev. (2017) 2017:181. doi: 10.1155/2017/9863181
32. Maulik N, Yoshida T, Engelman RM, Bagchi D, Otani H, Das DK. Dietary coenzyme Q10 supplement renders swine hearts resistant to ischemia-reperfusion injury. Am J Physiol - Hear Circ Physiol. (2000) 278:H1084–90. doi: 10.1152/ajpheart.2000.278.4.h1084
33. Verma DD, Hartner WC, Thakkar V, Levchenko TS, Torchilin VP. Protective effect of coenzyme Q10-loaded liposomes on the myocardium in rabbits with an acute experimental myocardial infarction. Pharm Res. (2007) 24:2131–7. doi: 10.1007/s11095-007-9334-0
34. Ambrosio G, Zweier JL, Duilio C, Kuppusamy P, Santoro G, Elia PP, et al. Evidence that mitochondrial respiration is a source of potentially toxic oxygen free radicals in intact rabbit hearts subjected to ischemia and reflow. J Biol Chem. (1993) 268:18532–41.
35. Paradies G, Petrosillo G, Pistolese M, Ruggiero FM. Reactive oxygen species generated by the mitochondrial respiratory chain affect the complex III activity via cardiolipin peroxidation in beef-heart submitochondrial particles. Mitochondrion. (2001) 1:151–9. doi: 10.1016/S1567-7249(01)00011-3
36. Petrosillo G, Ruggiero FM, Di Venosa N, Paradies G. Decreased complex III activity in mitochondria isolated from rat heart subjected to ischemia and reperfusion: role of reactive oxygen species and cardiolipin. FASEB J. (2003) 17:714–6. doi: 10.1096/fj.02-0729fje
37. Paradies G, Petrosillo G, Pistolese M, Ruggiero FM. The effect of reactive oxygen species generated from the mitochondrial electron transport chain on the cytochrome c oxidase activity and on the cardiolipin content in bovine heart submitochondrial particles. FEBS Lett. (2000) 466:323–6. doi: 10.1016/S0014-5793(00)01082-6
38. Yamamoto F, Yamamoto H, Yoshida S, Ichikawa H, Takahashi A, Tanaka K, et al. The effects of several pharmacologic agents upon postischemic recovery. Cardiovasc Drugs Ther. (1991) 5:301–8. doi: 10.1007/BF00054752
39. Crestanello JA, Kamelgard J, Lingle DM, Mortensen SA, Rhode M, Whitman GJR, et al. Elucidation of a tripartite mechanism underlying the improvement in cardiac tolerance to ischemia by coenzyme Q10 pretreatment. J Thorac Cardiovasc Surg. (1996) 111:443–50. doi: 10.1016/S0022-5223(96)70455-5
40. Aubrey BJ, Kelly GL, Janic A, Herold MJ, Strasser A. How does p53 induce apoptosis and how does this relate to p53-mediated tumour suppression? Cell Death Differ. (2018) 25:104–13. doi: 10.1038/cdd.2017.169
41. Nakano K, Vousden KH. PUMA, a novel proapoptotic gene, is induced by p53. Mol Cell. (2001) 7:683–94. doi: 10.1016/S1097-2765(01)00214-3
42. Chipuk JE, Kuwana T, Bouchier-Hayes L, Droin NM, Newmeyer DD, Schuler M, et al. Direct activation of Bax by p53 mediates mitochondrial membrane permeabilization and apoptosis. Science (80-). (2004) 303:1010–4. doi: 10.1126/science.1092734
43. Chipuk JE, Green DR. How do BCL-2 proteins induce mitochondrial outer membrane permeabilization? Trends Cell Biol. (2008) 18:157–64. doi: 10.1016/j.tcb.2008.01.007
44. Nishida K, Otsu K. Autophagy during cardiac remodeling. J Mol Cell Cardiol. (2016) 95:11–8. doi: 10.1016/j.yjmcc.2015.12.003
45. Schiattarella GG, Hill JA. Therapeutic targeting of autophagy in cardiovascular disease. J Mol Cell Cardiol. (2016) 95:86–93. doi: 10.1016/j.yjmcc.2015.11.019
46. Khan S, Salloum F, Das A, Xi L W, Vetrovec G, C, Kukreja R. Rapamycin confers preconditioning-like protection against ischemia-reperfusion injury in isolated mouse heart and cardiomyocytes. J Mol Cell Cardiol. (2006) 41:256–64. doi: 10.1016/j.yjmcc.2006.04.014
47. Sun J, Zhu H, Wang X, Gao Q, Li Z, Huang H. CoQ10 ameliorates mitochondrial dysfunction in diabetic nephropathy through mitophagy. J Endocrinol. (2019) 240:445–65. doi: 10.1530/JOE-18-0578
48. Kobayashi M, Higa JK, Matsui T. The role of ubiquitin in cardiac ischemia-reperfusion injury. Am J Physiol - Hear Circ Physiol. (2019) 316:H583–5. doi: 10.1152/ajpheart.00018.2019
49. Wilkinson KD, Urban MK, Haas AL. Ubiquitin is the ATP-dependent proteolysis factor I of rabbit reticulocytes. J Biol Chem. (1980) 255:7529–32.
50. Rose IA, Warms JVB, Hershko A. A high molecular weight protease in liver cytosol. J Biol Chem. (1979) 254:8135–8.
51. Wang X, Terpstra EJM. Ubiquitin receptors and protein quality control. J Mol Cell Cardiol. (2013) 55:73–84. doi: 10.1016/j.yjmcc.2012.09.012
52. Pickering AM, Linder RA, Zhang H, Forman HJ, Davies KJA. Nrf2-dependent induction of proteasome and Pa28αβ regulator are required for adaptation to oxidative stress. J Biol Chem. (2012) 287:10021–31. doi: 10.1074/jbc.M111.277145
53. Tian Z, Zheng H, Li J, Li Y, Su H, Wang X. Genetically induced moderate inhibition of the proteasome in cardiomyocytes exacerbates myocardial ischemia-reperfusion injury in Mice. Circ Res. (2012) 111:532–42. doi: 10.1161/CIRCRESAHA.112.270983
54. Hu C, Tian Y, Xu H, Pan B, Terpstra EM, Wu P, et al. Inadequate ubiquitination-proteasome coupling contributes to myocardial ischemia-reperfusion injury. J Clin Invest. (2018) 128:5294–306. doi: 10.1172/JCI98287
55. Jaburek M, Costa ADT, Burton JR, Costa CL, Garlid KD. Mitochondrial PKCε and mitochondrial ATP-sensitive K+ channel copurify and coreconstitute to form a functioning signaling module in proteoliposomes. Circ Res. (2006) 99:878–83. doi: 10.1161/01.RES.0000245106.80628.d3
56. Baines CP, Song C-X, Zheng Y-T, Wang G-W, Zhang J, Wang O-L, et al. Protein kinase Cε interacts with and inhibits the permeability transition pore in cardiac mitochondria. Circ Res. (2003) 92:873–80. doi: 10.1161/01.RES.0000069215.36389.8D
57. Downey JM, Davis AM, Cohen M V. Signaling pathways in ischemic preconditioning. Heart Fail Rev. (2007) 12:181–8. doi: 10.1007/s10741-007-9025-2
58. Singh RB, Fedacko J, Mojto V, Pella D. Coenzyme Q10 modulates remodeling possibly by decreasing angiotensin-converting enzyme in patients with acute coronary syndrome. Antioxidants. (2018) 7:18. doi: 10.3390/antiox7080099
59. Harada K, Sugaya T, Murakami K, Yazaki Y, Komuro I. Angiotensin II type 1A receptor knockout mice display less left ventricular remodeling and improved survival after myocardial infarction. Circulation. (1999) 100:2093–9. doi: 10.1161/01.CIR.100.20.2093
60. Michel J-B. Relationship between decrease in afterload and beneficial effects of ACE inhibitors in experimental cardiac hypertrophy and congestive heart failure. Eur Heart J. (1990) 11:17–26. doi: 10.1093/eurheartj/11.suppl_d.17
61. Digiesi V, Cantini F, Oradei A, Bisi G, Guarino GC, Brocchi A, et al. Coenzyme Q10 in essential hypertension. Mol Aspects Med. (1994) 15:s257–63. doi: 10.1016/0098-2997(94)90036-1
62. Sander S, Coleman CI, Patel AA, Kluger J, Michael White C. The impact of coenzyme q10 on systolic function in patients with chronic heart failure. J Card Fail. (2006) 12:464–72. doi: 10.1016/j.cardfail.2006.03.007
63. Huang C-H, Kuo C-L, Huang C-S, Tseng W-M, Lian IB, Chang C-C, et al. High plasma coenzyme Q10 concentration is correlated with good left ventricular performance after primary angioplasty in patients with acute myocardial infarction. Med (United States). (2016) 95:501. doi: 10.1097/MD.0000000000004501
64. Folkers K, Vadhanavikit S, Mortensen SA. Biochemial rationale and myocardial tissue data on the effective therapy of cardiomyopathy with coenzyme Q10. Proc Natl Acad Sci U S A. (1985) 82:901–4. doi: 10.1073/pnas.82.3.901
65. Shimizu M, Miyazaki T, Takagi A, Sugita Y, Yatsu S, Murata A, et al. Low circulating coenzyme Q10 during acute phase is associated with inflammation, malnutrition, and in-hospital mortality in patients admitted to the coronary care unit. Heart Vessels. (2017) 32:668–73. doi: 10.1007/s00380-016-0923-x
66. Shimizu M, Miyazaki T, Takagi A, Sugita Y, Ouchi S, Aikawa T, et al. Low coenzyme Q10 levels in patients with acute cardiovascular disease are associated with long-term mortality. Heart Vessels. (2021) 36:401–7. doi: 10.1007/s00380-020-01698-7
67. Singh RB, Wander GS, Rastogi A, Shukla PK, Mittal A, Sharma JP, et al. Randomized, double-blind placebo-controlled trial of coenzyme Q10 in patients with acute myocardial infarction. Cardiovasc Drugs Ther. (1998) 12:347–53. doi: 10.1023/A:1007764616025
68. Singh RB, Neki NS, Kartikey K, Pella D, Kumar A, Niaz MA, et al. Effect of coenzyme Q10 on risk of atherosclerosis in patients with recent myocardial infarction. Mol Cell Biochem. (2003) 246:75–82. doi: 10.1023/A:1023408031111
69. De Frutos F, Gea A, Hernandez-Estefania R, Rabago G. Prophylactic treatment with coenzyme Q10 in patients undergoing cardiac surgery: Could an antioxidant reduce complications? a systematic review and meta-analysis. Interact Cardiovasc Thorac Surg. (2015) 20:254–9. doi: 10.1093/icvts/ivu334
70. Al Saadi T, Assaf Y, Farwati M, Turkmani K, Al-Mouakeh A, Shebli B, et al. Coenzyme Q10 for heart failure. Cochrane Database Syst Rev. (2021) 2021:3. doi: 10.1002/14651858.CD008684.pub3
71. Alehagen U, Johansson P, Björnstedt M, Rosén A, Dahlström U. Cardiovascular mortality and N-terminal-proBNP reduced after combined selenium and coenzyme Q10 supplementation: A 5-year prospective randomized double-blind placebo-controlled trial among elderly Swedish citizens. Int J Cardiol. (2013) 167:1860–6. doi: 10.1016/j.ijcard.2012.04.156
72. Alehagen U, Aaseth J, Johansson P. Reduced cardiovascular mortality 10 years after supplementation with selenium and coenzyme q10 for four years: Follow-up results of a prospective randomized double-blind placebo-controlled trial in elderly citizens. PLoS One. (2015) 10:1641. doi: 10.1371/journal.pone.0141641
73. Witte KKA, Nikitin NP, Parker AC, Von Haehling S, Volk H-D, Anker SD, et al. The effect of micronutrient supplementation on quality-of-life and left ventricular function in elderly patients with chronic heart failure. Eur Heart J. (2005) 26:2238–44. doi: 10.1093/eurheartj/ehi442
74. Hofman-Bang C, Rehnqvist N, Swedberg K, Wiklund I, Åström H. Coenzyme Q10 as an adjunctive in the treatment of chronic congestive heart failure. J Card Fail. (1995) 1:101–7. doi: 10.1016/1071-9164(95)90011-X
75. Rodrigo R, Libuy M, Feliú F, Hasson D. Molecular basis of cardioprotective effect of antioxidant vitamins in myocardial infarction. Biomed Res Int. (2013) 2013:463. doi: 10.1155/2013/437613
76. Goszcz K, Deakin SJ, Duthie GG, Stewart D, Leslie SJ, Megson IL. Antioxidants in cardiovascular therapy: panacea or false hope? Front Cardiovasc Med. (2015) 2:29. doi: 10.3389/fcvm.2015.00029
77. Ferrari R, Visioli O, Guarnieri C, Caldarera M. Vitamin E and the heart: Possible role as antioxidant. Acta Vitaminol Enzymol. (1983) 5:11–22.
78. Klein HH, Pich S, Lindert S, Nebendahl K, Niedmann P, Kreuzer H. Combined treatment with vitamins E and C in experimental myocardial infarction in pigs. Am Heart J. (1989) 118:667–73. doi: 10.1016/0002-8703(89)90577-2
79. Šochman J, Kolc J, Vrána M, Fabián J. Cardioprotective effects of N-acetylcysteine: the reduction in the extent of infarction and occurrence of reperfusion arrhythmias in the dog. Int J Cardiol. (1990) 28:191–6. doi: 10.1016/0167-5273(90)90060-I
80. Sobey CG, Dalipram RA, Dusting GJ, Woodman OL. Impaired endothelium-dependent relaxation of dog coronary arteries after myocardial ischaemia and reperfusion: prevention by amlodipine, propranolol and allopurinol. Br J Pharmacol. (1992) 105:557–62. doi: 10.1111/j.1476-5381.1992.tb09018.x
81. Akizuki S, Yoshida S, Chambers DE, Eddy LJ, Parmley LF, Yellon DM, et al. Infarct size limitation by the xanthine oxidase inhibitor, allopurinol, in closed-chest dogs with small infarcts. Cardiovasc Res. (1985) 19:686–92. doi: 10.1093/cvr/19.11.686
82. Chambers DE, Parks DA, Patterson G, Roy R, McCord JM, Yoshida S, et al. Xanthine oxidase as a source of free radical damage in myocardial ischemia. J Mol Cell Cardiol. (1985) 17:145–52. doi: 10.1016/S0022-2828(85)80017-1
83. Montor SG, Thoolen MJMC, Mackin WM, Timmermans PBMWM. Effect of azapropazone and allopurinol on myocardial infarct size in rats. Eur J Pharmacol. (1987) 140:203–7. doi: 10.1016/0014-2999(87)90806-5
84. Qin C, Yap S, Woodman OL. Antioxidants in the prevention of myocardial ischemia/reperfusion injury. Expert Rev Clin Pharmacol. (2009) 2:673–95. doi: 10.1586/ecp.09.41
85. Khan SU, Khan MU, Riaz H, Valavoor S, Zhao D, Vaughan L, et al. Effects of nutritional supplements and dietary interventions on cardiovascular outcomes. Ann Intern Med. (2019) 171:190–8. doi: 10.7326/M19-0341
86. Yusuf S, Dagenais G, Pogue J, Bosch J, Sleight P. Vitamin E supplementation and cardiovascular events in high-risk patients. N Engl J Med. (2000) 342:154–60. doi: 10.1056/nejm200001203420302
87. Collins R, Armitage J, Parish S, Sleight P, Peto R. MRC/BHF Heart Protection Study of antioxidant vitamin supplementation in 20 536 high-risk individuals: A randomised placebo-controlled trial. Lancet. (2002) 360:23–33. doi: 10.1016/S0140-6736(02)09328-5
88. Marchioli R. Dietary supplementation with N-3 polyunsaturated fatty acids and vitamin E after myocardial infarction: Results of the GISSI-Prevenzione trial. Lancet. (1999) 354:447–55. doi: 10.1016/S0140-6736(99)07072-5
89. Cook NR, Albert CM, Gaziano JM, Zaharris E, MacFadyen J, Danielson E, et al. A randomized factorial trial of vitamins C and E and beta carotene in the secondary prevention of cardiovascular events in women: results from the women's antioxidant cardiovascular study. Arch Intern Med. (2007) 167:1610–8. doi: 10.1001/archinte.167.15.1610
90. Thiele H, Hildebrand L, Schirdewahn C, Eitel I, Adams V, Fuernau G, et al. Impact of high-dose n-acetylcysteine versus placebo on contrast-induced nephropathy and myocardial reperfusion injury in unselected patients with st-segment elevation myocardial infarction undergoing primary percutaneous coronary intervention. The LIPSIA-. J Am Coll Cardiol. (2010) 55:2201–9. doi: 10.1016/j.jacc.2009.08.091
91. Guan W, Osanai T, Kamada T, Hanada H, Ishizaka H, Onodera H, et al. Effect of allopurinol pretreatment on free radical generation after primary coronary angioplasty for acute myocardial infarction. J Cardiovasc Pharmacol. (2003) 41:699–705. doi: 10.1097/00005344-200305000-00005
92. Cappola TP, Kass DA, Nelson GS, Berger RD, Rosas GO, Kobeissi ZA, et al. Allopurinol improves myocardial efficiency in patients with idiopathic dilated cardiomyopathy. Circulation. (2001) 104:2407–11. doi: 10.1161/hc4501.098928
93. Castelli P, Maria Condemi A, Brambillasca C, Fundarò P, Botta M, Lemma M, et al. Improvement of cardiac function by allopurinol in patients undergoing cardiac surgery. J Cardiovasc Pharmacol. (1995) 25:119–25. doi: 10.1097/00005344-199501000-00019
94. Nasr G, Maurice C. Allopurinol and global left myocardial function in heart failure patients. J Cardiovasc Dis Res. (2010) 1:191–5. doi: 10.4103/0975-3583.74262
95. Greig D, Alcaino H, Castro PF, Garcia L, Verdejo HE, Navarro M, et al. Xanthine-oxidase inhibitors and statins in chronic heart failure: Effects on vascular and functional parameters. J Hear Lung Transplant. (2011) 30:408–13. doi: 10.1016/j.healun.2010.10.003
96. Givertz MM, Anstrom KJ, Redfield MM, Deswal A, Haddad H, Butler J, et al. Effects of xanthine oxidase inhibition in hyperuricemic heart failure patients: The xanthine oxidase inhibition for hyperuricemic heart failure patients (EXACT-HF) study. Circulation. (2015) 131:1763–71. doi: 10.1161/CIRCULATIONAHA.114.014536
97. Gavin AD, Struthers AD. Allopurinol reduces B-type natriuretic peptide concentrations and haemoglobin but does not alter exercise capacity in chronic heart failure. Heart. (2005) 91:749–53. doi: 10.1136/hrt.2004.040477
98. McGonigle P, Ruggeri B. Animal models of human disease: Challenges in enabling translation. Biochem Pharmacol. (2014) 87:162–71. doi: 10.1016/j.bcp.2013.08.006
99. Bart van der Worp H, Howells DW, Sena ES, Porritt MJ, Rewell S, O'Collins V, et al. Can animal models of disease reliably inform human studies? PLoS Med. (2010) 7:1–8. doi: 10.1371/journal.pmed.1000245
100. Heusch G. Critical Issues for the Translation of Cardioprotection. Circ Res. (2017) 120:1477–86. doi: 10.1161/CIRCRESAHA.117.310820
101. Tsang HG, Rashdan NA, Whitelaw CBA, Corcoran BM, Summers KM, MacRae VE. Large animal models of cardiovascular disease. Cell Biochem Funct. (2016) 34:113–32. doi: 10.1002/cbf.3173
102. Nair AB, Jacob S. A simple practice guide for dose conversion between animals and human. J basic Clin Pharm. (2016) 7:27–31. doi: 10.4103/0976-0105.177703
103. Califf RM, Bengtson JR. Cardiogenic shock. N Engl J Med. (1994) 330:1724–1730. doi: 10.1056/NEJM199406163302406
104. Bhagavan HN, Chopra RK. Coenzyme Q10: Absorption, tissue uptake, metabolism and pharmacokinetics. Free Radic Res. (2006) 40:445–53. doi: 10.1080/10715760600617843
105. Zhang Y, Aberg F, Appelkvist E-L, Dallner G, Ernster L. Uptake of dietary coenzyme Q supplement is limited in rats. J Nutr. (1995) 125:446–53.
106. Alessandri MG, Scalori V, Giovannini L, Mian M, Bertelli AAE. Plasma and tissue concentrations of coenzyme Q10 in the rat after intravenous administration by a microsphere delivery system or in a new type of solution. Int J Tissue React. (1988) 10:99–102.
107. Arenas-Jal M, Suñé-Negre JM, García-Montoya E. Coenzyme Q10 supplementation: Efficacy, safety, and formulation challenges. Compr Rev Food Sci Food Saf. (2020) 19:574–94. doi: 10.1111/1541-4337.12539
108. Zhu Z-G, Sun M-X, Zhang W-L, Wang W-W, Jin Y-M, Xie C-L. The efficacy and safety of coenzyme Q10 in Parkinson's disease: a meta-analysis of randomized controlled trials. Neurol Sci. (2017) 38:215–24. doi: 10.1007/s10072-016-2757-9
Keywords: ubiquinone, animals, rats, coenzyme Q10, pre-clinical, myocardial reperfusion injury
Citation: Awad K, Sayed A and Banach M (2022) Coenzyme Q10 Reduces Infarct Size in Animal Models of Myocardial Ischemia-Reperfusion Injury: A Meta-Analysis and Summary of Underlying Mechanisms. Front. Cardiovasc. Med. 9:857364. doi: 10.3389/fcvm.2022.857364
Received: 18 January 2022; Accepted: 15 March 2022;
Published: 15 April 2022.
Edited by:
Salvatore Pepe, Royal Children's Hospital, AustraliaReviewed by:
Carmine Rocca, University of Calabria, ItalyCopyright © 2022 Awad, Sayed and Banach. This is an open-access article distributed under the terms of the Creative Commons Attribution License (CC BY). The use, distribution or reproduction in other forums is permitted, provided the original author(s) and the copyright owner(s) are credited and that the original publication in this journal is cited, in accordance with accepted academic practice. No use, distribution or reproduction is permitted which does not comply with these terms.
*Correspondence: Kamal Awad, a2FtYWwyMjUyNDRAbWVkaWNpbmUuenUuZWR1LmVn; Maciej Banach, bWFjaWVqYmFuYWNoNzdAZ21haWwuY29t
Disclaimer: All claims expressed in this article are solely those of the authors and do not necessarily represent those of their affiliated organizations, or those of the publisher, the editors and the reviewers. Any product that may be evaluated in this article or claim that may be made by its manufacturer is not guaranteed or endorsed by the publisher.
Research integrity at Frontiers
Learn more about the work of our research integrity team to safeguard the quality of each article we publish.