- 1Obesity and Comorbidities Research Center, University of Campinas, Campinas, Brazil
- 2Department of Internal Medicine, School of Medical Sciences, University of Campinas, Campinas, Brazil
- 3Department of Radiology, School of Medical Sciences, University of Campinas, Campinas, Brazil
- 4School of Nursing, University of Campinas, Campinas, Brazil
Severe acute respiratory syndrome coronavirus 2 (SARS-CoV-2) employs angiotensin-converting enzyme 2 (ACE2) as its receptor for cell entrance, and studies have suggested that upon viral binding, ACE2 catalytic activity could be inhibited; therefore, impacting the regulation of the renin-angiotensin-aldosterone system (RAAS). To date, only few studies have evaluated the impact of SARS-CoV-2 infection on the blood levels of the components of the RAAS. The objective of this study was to determine the blood levels of ACE, ACE2, angiotensin-II, angiotensin (1–7), and angiotensin (1–9) at hospital admission and discharge in a group of patients presenting with severe or critical evolution of coronavirus disease 2019 (COVID-19). We showed that ACE, ACE2, angiotensin (1–7), and angiotensin (1–9) were similar in patients with critical and severe COVID-19. However, at admission, angiotensin-II levels were significantly higher in patients presenting as critical, compared to patients presenting with severe COVID-19. We conclude that blood levels of angiotensin-II are increased in hospitalized patients with COVID-19 presenting the critical outcome of the disease. We propose that early measurement of Ang-II could be a useful biomarker for identifying patients at higher risk for extremely severe progression of the disease.
Introduction
Angiotensin-converting enzyme 2 (ACE-2) acts as the receptor for severe acute respiratory syndrome coronavirus 2 (SARS-CoV-2; 1) and viral binding and internalization are believed to impair ACE-2 catalytic activity, impacting the inactivation of angiotensin II (Ang-II; 2–4). It has been proposed that abnormal regulation of the renin-angiotensin-aldosterone system (RAAS) could explain cardiovascular and inflammatory instability, which play important roles in the severe progression of coronavirus disease 2019 (COVID-19; 5–7). This is further supported by the fact that hypertension, diabetes, and obesity, conditions that are related to abnormal regulation of the RAAS, are the main risk factors for the severe progression of COVID-19 (8, 9). In addition, a recent meta-analysis has shown that pharmacological inhibition of the RAAS is associated with reduced mortality of patients with COVID-19 (10). Nevertheless, there are still several questions that have not been properly answered in this field.
Angiotensin II is produced from angiotensin I (Ang-I) as a result of the catalytic action of angiotensin-converting enzyme 1 (ACE-1). Ang-II plays an important role in the regulation of blood volume, blood pressure, cardiac function, and electrolyte balance (11, 12). Moreover, increased Ang-II can lead to mitochondrial oxidative damage, reactive oxygen species overproduction, and increased interleukin-6 (IL-6); thus, boosting inflammation (13, 14). Ang-II is also involved in the regulation of insulin action, as both signaling systems interact at the intracellular level (15, 16). Therefore, determining the potential involvement of Ang-II in severe COVID-19 could provide advances in the diagnosis and treatment of the disease.
Currently, only few studies have measured the components of the RAAS in the blood of patients with COVID-19 and the results are mostly controversial (17–19). In this study, we determined the blood levels of the RAAS components in patients with severe progression of COVID-19. We showed that, at hospital admission, Ang-II was increased in the blood of patients that presented with an extremely severe progression of COVID-19. We propose that early measurement of Ang-II could be a useful marker for identifying patients at higher risk for extremely severe progression of the disease.
Methods
Study Cohort and Data Collection
This study was conducted at the Clinics Hospital at the University of Campinas in Brazil. Sample and data collection were performed within the study “Safety and Outcomes Associated with the Pharmacological Inhibition of the Kinin–Kallikrein System in Severe COVID-19” (20), a phase II, single-center, three-arm parallel-group, open-label, randomized clinical trial. Thirty patients diagnosed with COVID-19, as confirmed by real time-PCR analysis of nasopharyngeal swab samples, were enrolled from 22 April–14 June 2020. The study was approved by the ethics committee of the University of Campinas (protocol CAEE: 30227920.9.0000.5404), conducted according to the Declaration of Helsinki and Good Clinical Practice principles (ICH 1996) and registered with the Brazilian Clinical Trials Registry1 – Universal Trial Number, U1111-1250-1843. Written consent forms to participate in this study were obtained from all patients or substitute decision-makers (for patients lacking decision-making capacity). We retrospectively analyzed the medical history, physical examination, and radiological evaluation results obtained from all patients. Epidemiological, clinical, laboratory, and radiological parameters and treatment, as well as outcome data, were obtained from electronic medical records. The data collection forms were reviewed independently by two researchers. Blood samples were collected on admission and at hospital discharge. For patient #3, who died on the 21st day of hospitalization, blood samples were collected on day 21; and for patient #15, who died on day 17 of hospitalization, blood samples were collected on day 14. For the two deceased patients, length of stay (LOS) was considered 28 days (maximum LOS predicted in the study). Hematological and biochemical parameters in blood samples were determined using automated methods.
Definition of Clinical Status
According to the original study, all 30 patients with COVID-19 were symptomatic at hospital admission and typical pneumonia was confirmed by computed tomography of the chest and scored (CT score) by two lung expert radiologists, according to parameters described elsewhere (21). COVID-19 diagnosis was confirmed by SARS-CoV-2 RT-PCR and the oxygen saturation measured was ≤94% at ambient air or Pa02/FiO2 ≤ 300 mm Hg. In addition, considering LOS, patients were divided into two groups: severe and critical, according to a definition published elsewhere (20, 22, 23). Patients with LOS for up to 5 days were defined as severe (n = 11) and patients with LOS ≥ 13 days were defined as critical (n = 12; Supplementary Table 1). Patients with LOS between 6–12 days were not further evaluated in this study (n = 7).
Determination of Serum Parameters
ELISA methods were used to determine triggering receptor expressed on myeloid cells-1 (TREM-1, RD Systems; catalog number DTRM10C), lactate dehydrogenase (MyBioSource; catalog number MBS162869), and serum IgG against SARS-CoV-2 spike protein (Cell Signaling; catalog number 20154). Albumin was determined using a colorimetric assay (LaborLab; catalog number 1770010). C-reactive protein (CRP) was determined using an ELISA kit (Thermo Fisher; catalog number KHA0031). Cytokines were determined using multiplex Human Cytokine 25-Plex Panel (Invitrogen; catalog number LHC0009) and ELISA kits (Interleukin-1beta, RD Systems; catalog number HSLB00C; IL-6, RD Systems; catalog number D6050; eotaxin-2, RayBiotech; catalog number O00175).
Determination of Angiotensin Pathway Components
ELISA kits were also used to measure ACE (ACE MyBioSource; catalog number MBS2700706), ACE2 (ACE 2 MyBioSource; catalog number MBS2703852), angiotensin (1–7; ANG 1-7 MyBioSource; catalog number MBS2607668), angiotensin (1–9; ANG 1–9 MyBioSource; catalog number MBS162788), and angiotensin 2 (ANG II MyBioSource; catalog number MBS7003599).
Real-Time Reverse Transcription PCR Assay
Gene expression of cytokines and immune cell molecular markers were determined by RT-PCR. Blood was collected using the PAXgene® Blood RNA tube and frozen at –80°C for further analysis. Total RNA from whole blood was extracted and purified using PAXgene Blood RNA Kit (PreAnalytix; catalog number 762174). cDNA samples were obtained from 600 ng of RNA using High-Capacity cDNA Reverse Transcription Kit (Applied Biosystems; catalog number 4368813). Gene expression analyses were performed using the StepOne™ Real-Time PCR System (Applied Biosystems). Each PCR well contained 5 ng of cDNA, 0.25 μl of specific primer, 3.0 μl of Taqman Universal master mix (Applied Biosystems; catalog number 4369016), and RNase-free water to 7.5 μl of the final volume. Primers were purchased from Applied Biosystems: IL6 – Hs00985639_m1; CXCL8 – Hs00174103_m1; CCL2 – Hs00234140_m1; TNFα – Hs00174128_m1; IL10 – Hs00961622_m1; IL1β – Hs0155410_m1; CCR3 – Hs00266213_m1; TLR7 – Hs01933259_m1; CD11B – Hs00167304_m1; CD15 – Hs1106466_s1; CD66b – Hs00266198_m1; IL5RA – Hs00602482_m1; and CD16A – Hs02388314_m1. Human Gapdh (Cat. Number 4326317E), and PPIA (Hs04401210_s1) were employed in all samples as normalizing transcripts. PCR efficiency was calculated before the experiments, and the 2^-DDCT method was used to calculate gene expression.
Statistical Analysis
All statistical analyses were performed using GraphPad Prism 8.0 and SPSS version 22.0. Values are presented as mean and standard deviation (SD) in the tables and means and standard error of the mean in the figures. Before the analyses, a Kolmogorov–Smirnov test was used to evaluate the normal distribution of variables. If the variable had non-parametric distribution, the equivalent non-parametric test was used. For most analyses, three different variables associated with disease severity were considered: total duration of the disease, LOS (hospitalization), and length of intensive care. Associations between duration of the disease, LOS, and length of intensive care with clinical parameters and biochemical markers were performed using Spearman or Person coefficient. A p-value < 0.05 was considered significant.
Results
Clinical, Laboratorial, and Radiological Characteristics of Patients at Admission
Supplementary Table 1 presents the age, sex, date of hospital admission, LOS, and disease severity of each patient included in the study. Baseline clinical and laboratorial data are presented in Table 1. Compared to severe patients, critical patients presented higher CT scores, lower diastolic blood pressure, higher blood lactate dehydrogenase, and higher CRP. There were no differences in comorbidities among the groups (Supplementary Table 2).
Defining the Clinical, Laboratorial, and Radiological Parameters Associated With Poor Outcomes
To validate this cohort as representative of the expected outcomes of patients with severe COVID-19, we measured several parameters and evaluated their association with poor prognosis, defined by the number of days from the beginning of symptoms to hospital discharge; the number of days in hospital; and, the number of days in the intensive care unit. As depicted in Figure 1, admission CT score, variation in delta-troponin, baseline D-dimer, change in D-dimer during hospitalization, and baseline CRP were positively correlated with poorer prognosis. In addition, baseline creatinine was positively correlated with total disease time, whereas the change in blood eosinophils correlated with time in hospital. Conversely, variation in red blood cell counts and hematocrit variation were negatively correlated with prognosis.
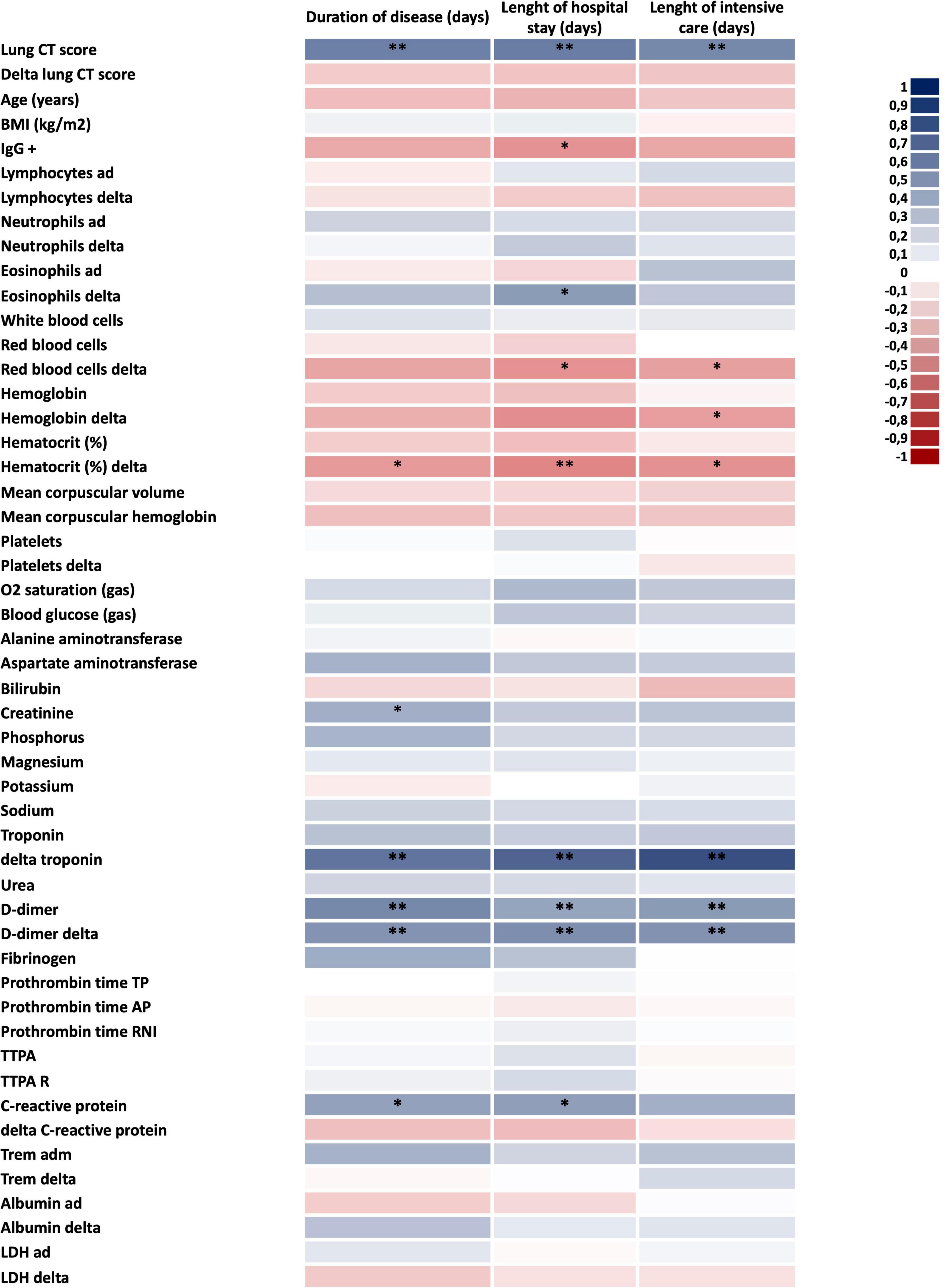
Figure 1. Defining the factors associated with COVID-19 severity. Heatmap of clinical and laboratorial parameters potentially associated with COVID-19 severity. Blue colors mean direct correlation; red colors mean inverse correlation. In all n = 28; *p < 0.05 and **p < 0.01. BMI, body mass index; CT, computed tomography; ICU, intensive care unit; LDH, lactate dehydrogenase; and TTPA, partial activated thromboplastin time.
Blood Cytokines/Chemokine Associated With Poor Prognosis
We further validated the cohort by determining the blood levels of three cytokines and one chemokine known to be increased in the blood of patients with severe COVID-19. In Figure 2, we show that admission blood IL-6, IL-8, IL-1β, and monocyte chemoattractant protein-1 were significantly higher in patients with poorer prognoses.
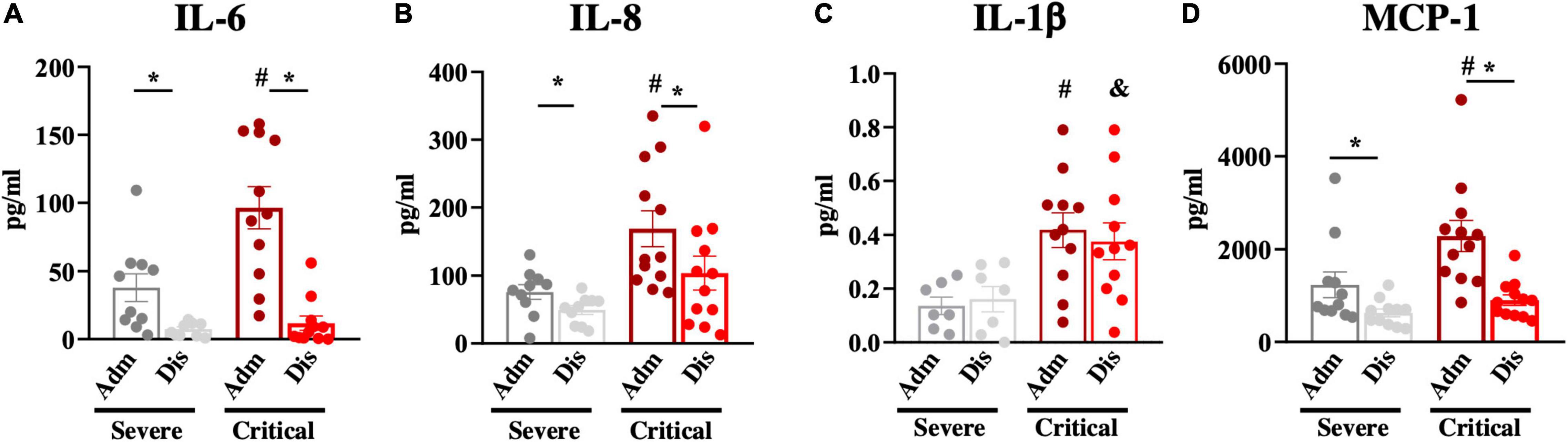
Figure 2. Blood inflammatory markers. Inflammatory markers; interleukin-6 (IL-6; A), interleukin-8 (IL-8; B), interleukin-1beta (IL-1β; C), and monocyte chemoattractant protein-1 (MCP-1; D) were determined in blood samples at hospital admission (Adm) and discharge (Dis). *p < 0.05; #p < 0.05 vs. severe at admission; &p < 0.05 vs. severe at discharge.
Admission Plasma Angiotensin II Levels Predict Critical Coronavirus Disease 2019
Next, we determined the plasma levels of key components of the RAAS (Figure 3A) at admission and discharge. There were no significant differences in soluble ACE2 (Figure 3B), as well as in the variation of soluble ACE2, from admission to discharge (Figure 3C). Plasma ACE levels were also similar in both groups and underwent no changes from admission to discharge (Figure 3D). Conversely, admission Ang-II levels were significantly higher (approximately 2-fold higher) in patients presenting with critical COVID-19 compared to patients presenting with severe disease (Figure 3E). Moreover, there was a significantly greater decrease in Ang-II plasma levels from admission to discharge in critical patients compared to severe patients (Figure 3F). Importantly, all patients presenting Ang-II plasma levels greater than 300 pg/ml presented a critical outcome of the disease. There were no differences in the plasma levels of Ang (1–7; Figure 3G) and Ang (1–9; Figure 3H) between the groups. As the existence of comorbidities could represent a bias in the analysis, we evaluated RAAS components by comparing patients with and without comorbidities. As shown in Supplementary Table 3, only Ang (1–7) was different among the groups presenting higher levels at admission in patients without comorbidities.
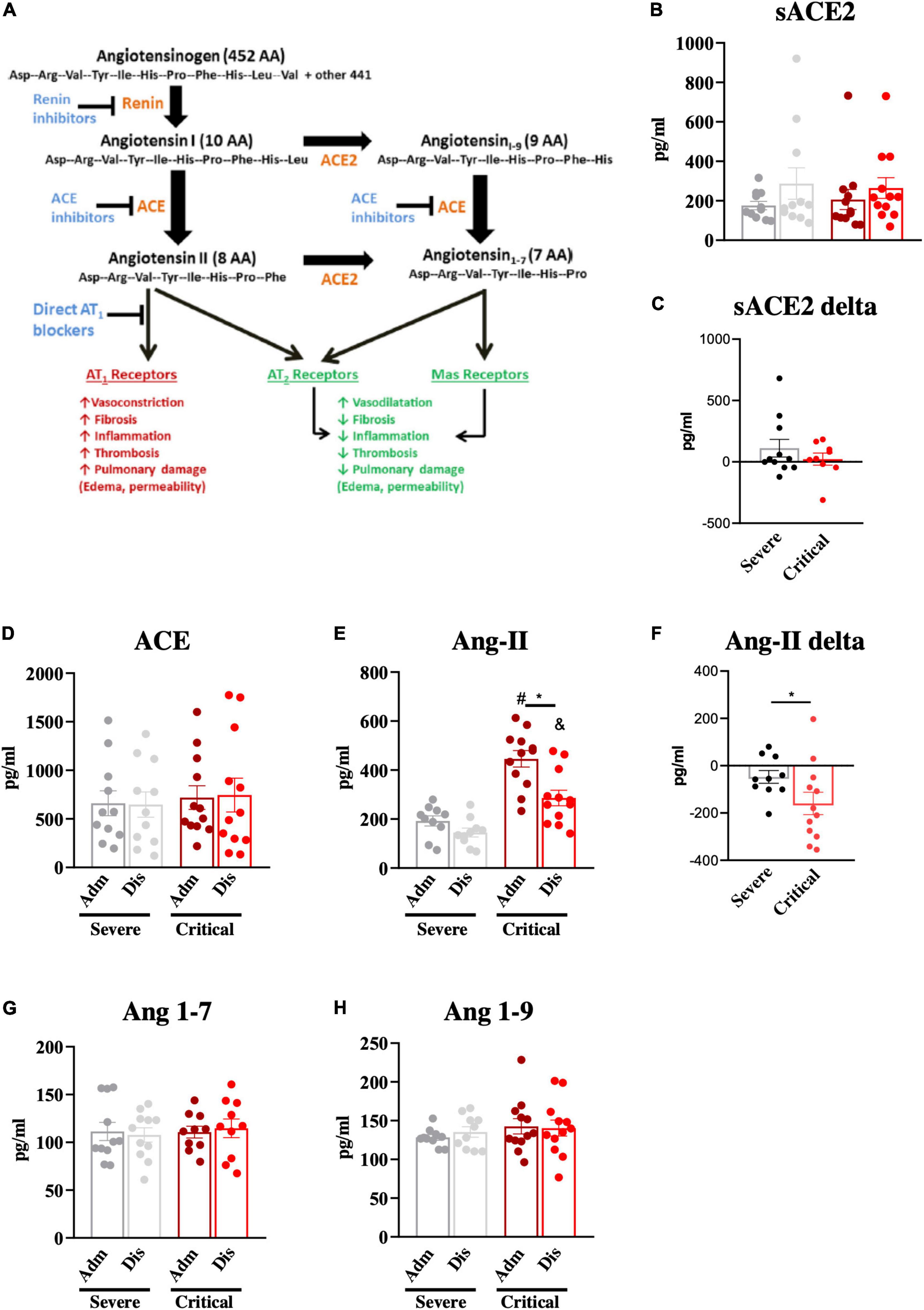
Figure 3. Components of the renin-angiotensin-aldosterone system. The renin-angiotensin-aldosterone system and its main effects are depicted in (A). Components of the renin-angiotensin-aldosterone system; soluble angiotensin-converting enzyme-2 (sACE2; B, C), angiotensin-converting enzyme (ACE; D), angiotensin-II (Ang-II; E, F), angiotensin 1–7 (Ang 1–7; G) and angiotensin 1–9 (Ang 1–9; H) were determined in blood samples at hospital admission (Adm) and discharge (Dis). The variations of sACE2 (C) and Ang-II (F) from admission to discharge were determined. *p < 0.05; #p < 0.05 vs. severe at admission; &p < 0.05 vs. severe at discharge.
Discussion
This study shows that at hospital admission, plasma levels of Ang-II are higher in patients with COVID-19 presenting with the critical disease compared to patients presenting with severe disease. This data indicates Ang-II may be a potential biomarker that could help identify hospitalized patients with poorer COVID-19 prognosis and, also stimulate further research aimed at developing therapeutic strategies to mitigate disease severity. It is important to highlight that other biomarkers have been previously identified and seem to be associated with the pathological process of COVID-19. The incorporation of Ang-II to this list could help to understand the mechanisms leading to severe forms of the disease, as well as provide an advance in the capacity of predicting critical outcomes and the early need for intensive care (24).
The patients included in the study were ranked for severity based on the LOS. We decided for using this approach because, as a retrospective study that asked if admission levels of components of the RAAS could be good biomarkers for predicting the outcomes of severe COVID-19, we reasoned that upon employment of other known markers of severity, such as blood pressure, oxygen requirements, and imaging progression, we could generate a bias in the interpretation of the results that could mask the real value of RAAS components in the context.
Despite the undisputed importance of studying the RAAS in COVID-19, there are only few studies that have actually measured the components of this system in patients. Most of the studies have simply compared non-infected vs. SARS-CoV-2-infected patients without considering disease severity and clinical outcomes. In this context, two studies reported no differences in the blood levels of Ang-II (18, 25) and one study reported a reduction of Ang-II and an increase in angiotensin (1–7; 26). It is currently known that SARS-CoV-2 infection can lead to a large spectrum of clinical outcomes that range from sore throat, mild fever, and tiredness to severe lung damage, cardiovascular dysfunction, and coagulopathy (22, 23). Approximately 81% of patients with COVID-19 present with mild or moderate disease and are not admitted for hospital care (27); conversely, of the remaining symptomatic patients that require hospitalization, three-fourths have severe disease and one-fourth have critical disease (27). The studies that have evaluated the components of the RAAS, comparing SARS-CoV-2-infected vs. non-infected patients, could provide important mechanistic advances if they showed that SARS-CoV-2 infection, independently of severity, could modulate the components of the RAAS. In at least one study, the treatment of rodents with purified spike protein induced an increase in Ang-II, providing an interesting experimental proof-of-concept to support the hypothesis that SARS-CoV-2 binding to ACE2 can indeed impact the regulation of the RAAS (28, 29). However, the few clinical studies available to date present conflicting results. In some studies, there were methodological issues, such as small sample size and inappropriate assays for measuring RAAS peptides; and in others, statistical power was insufficient to confirm or refute the impact of SARS-CoV-2 infection on the RAAS. Thus, further studies should be performed in order to provide a definitive answer to this important question.
Identifying biomarkers that are modulated upon SARS-CoV-2 infection, independently of disease severity, is important and could provide advances in the understanding of the mechanisms of disease, but they have limited value in population screening, as the majority of infected patients have either no or very mild symptoms and do not require medical intervention (23). This is the case for IL-6, TNF-α, IL-1β, and IL-10, which present early increases in the blood of patients that will progress with severe disease as compared with patients presenting only mild symptoms that require no hospitalization (30). Conversely, identifying biomarkers that segregate hospitalized patients who present with severe or critical diseases could provide therapeutic advances, as patients at greater risk could be given intensive care promptly upon admission. Prior to this study, there were only two reports on the measurement of the components of the RAAS in the plasma of patients with COVID-19 presenting with distinct clinical statuses. One study compared patients during an early phase of COVID-19 manifestations vs. patients with prolonged disease (31). The authors reported a reduction of ACE2 expression in cells and an increase in the blood level of Ang-II in patients presenting with prolonged disease (31). Another study evaluated patients with severe COVID-19 vs. uninfected subjects (17), documenting an increase in the blood levels of Ang-II in severe patients, and particularly, a positive correlation between blood Ang-II and viral load (17). Of note, the blood levels of Ang-II in severe patients in this study were similar to the levels (approximately 400 pg/ml) we found in our study of patients with critical COVID-19.
Despite the advance provided by this study, there are weaknesses that could be addressed in future work: (i) the number of patients is small, but it is still similar to other previous studies that evaluated the RAAS in patients with COVID-19 (18, 25, 26, 32); (ii) as this is a retrospective study, we could not determine the real-time correlation between the rate of clinical improvement and changes in the blood levels of RAAS components; (iii) as the study was performed in the very beginning of COVID-19 pandemic, we were not equipped to perform determination of viral load or spike protein amount; and (iv) there are unsolved methodological critics regarding the accuracy of the available Ang-II determination methods (32–34).
In conclusion, blood levels of angiotensin-II are increased in hospitalized patients with COVID-19 presenting the critical outcome of the disease. This could place Ang-II alongside D-dimer (35), ferritin (36), CRP (37), IL-6, and tumor necrosis factor-alpha (38) as early biomarkers for prognosis and, therefore, with the ability to guide the clinical decision.
Data Availability Statement
The raw data supporting the conclusions of this article will be made available by the authors, without undue reservation.
Ethics Statement
The studies involving human participants were reviewed and approved by Ethics Committee of the University of Campinas. The patients/participants provided their written informed consent to participate in this study.
Author Contributions
LV and AS are the supervisors and lead contacts, conceived the study, raised funds, organized the protocol, and wrote the manuscript. RC and BB performed laboratory analysis. MM-P and EA organized data. EM coordinated the clinical team. AP, LR, RU, AB, TN, and MA screened, enrolled, and followed-up patients. MM provided clinical supervision support for the preparation and execution of the trial. RD and SD designed and performed radiological analysis. MM-P performed the statistical analysis. All authors read and approved the final manuscript.
Funding
The study was fully sponsored by public grants provided by the University of Campinas and the São Paulo Research Foundation. The sponsors had no involvement with study design, data collection, data analysis, interpretation of results, and manuscript preparation. São Paulo Research Foundation grants #2013/07607-8 and #2020/04522-5; University of Campinas grant #2298/20.
Conflict of Interest
The authors declare that the research was conducted in the absence of any commercial or financial relationships that could be construed as a potential conflict of interest.
Publisher’s Note
All claims expressed in this article are solely those of the authors and do not necessarily represent those of their affiliated organizations, or those of the publisher, the editors and the reviewers. Any product that may be evaluated in this article, or claim that may be made by its manufacturer, is not guaranteed or endorsed by the publisher.
Acknowledgments
We thank Erika Roman, Joseane Morari, and Gerson for their technical assistance.
Supplementary Material
The Supplementary Material for this article can be found online at: https://www.frontiersin.org/articles/10.3389/fcvm.2022.847809/full#supplementary-material
Footnotes
References
1. Hoffmann M, Kleine-Weber H, Schroeder S, Krüger N, Herrler T, Erichsen S, et al. SARS-CoV-2 cell entry depends on ACE2 and TMPRSS2 and is blocked by a clinically proven protease inhibitor. Cell. (2020) 181:271–80.e8.
2. Ou X, Liu Y, Lei X, Li P, Mi D, Ren L, et al. Characterization of spike glycoprotein of SARS-CoV-2 on virus entry and its immune cross-reactivity with SARS-CoV. Nat Commun. (2020) 11:1620.
3. Shang J, Wan Y, Luo C, Ye G, Geng Q, Auerbach A, et al. Cell entry mechanisms of SARS-CoV-2. Proc Natl Acad Sci U S A. (2020) 117:11727–34.
4. Sidarta-Oliveira D, Jara CP, Ferruzzi AJ, Skaf MS, Velander WH, Araujo EP, et al. SARS-CoV-2 receptor is co-expressed with elements of the kinin-kallikrein, renin-angiotensin and coagulation systems in alveolar cells. Sci Rep. (2020) 10:19522. doi: 10.1038/s41598-020-76488-2
5. Lloyd-Jones G, Oudkerk M. COVID-19: angiotensin II in development of lung immunothrombosis and vasculitis mimics. Lancet Rheumatol. (2021) 3:e325–6.
6. Aksoy H, Karadag AS, Wollina U. Angiotensin II receptors: impact for COVID-19 severity. Dermatol Ther. (2020) 33:e13989.
7. Gu H, Xie Z, Li T, Zhang S, Lai C, Zhu P, et al. Angiotensin-converting enzyme 2 inhibits lung injury induced by respiratory syncytial virus. Sci Rep. (2016) 6:19840.
8. Aghili SMM, Ebrahimpur M, Arjmand B, Shadman Z, Pejman Sani M, Qorbani M, et al. Obesity in COVID-19 era, implications for mechanisms, comorbidities, and prognosis: a review and meta-analysis. Int J Obes. (2021) 45:998–1016. doi: 10.1038/s41366-021-00776-8
9. Stefan N, Birkenfeld AL, Schulze MB. Global pandemics interconnected – obesity, impaired metabolic health and COVID-19. Nat Rev Endocrinol. (2021) 17:135–49. doi: 10.1038/s41574-020-00462-1
10. Guo X, Zhu Y, Hong Y. Decreased mortality of COVID-19 with renin-angiotensin-aldosterone system inhibitors therapy in patients with hypertension: a meta-analysis. Hypertension. (2020) 76:e13–4.
11. Schmieder RE, Hilgers KF, Schlaich MP, Schmidt BM. Renin-angiotensin system and cardiovascular risk. Lancet. (2007) 369:1208–19.
12. Boehm M, Nabel EG. Angiotensin-converting enzyme 2–a new cardiac regulator. N Engl J Med. (2002) 347:1795–7.
13. Yin JX, Yang RF, Li S, Renshaw AO, Li YL, Schultz HD, et al. Mitochondria-produced superoxide mediates angiotensin II-induced inhibition of neuronal potassium current. Am J Physiol Cell Physiol. (2010) 298:C857–65. doi: 10.1152/ajpcell.00313.2009
14. Han Y, Runge MS, Brasier AR. Angiotensin II induces interleukin-6 transcription in vascular smooth muscle cells through pleiotropic activation of nuclear factor-kappa B transcription factors. Circ Res. (1999) 84:695–703. doi: 10.1161/01.res.84.6.695
15. Velloso LA, Folli F, Sun XJ, White MF, Saad MJ, Kahn CR. Cross-talk between the insulin and angiotensin signaling systems. Proc Natl Acad Sci U S A. (1996) 93:12490–5.
16. Calegari VC, Alves M, Picardi PK, Inoue RY, Franchini KG, Saad MJ, et al. Suppressor of cytokine signaling-3 Provides a novel interface in the cross-talk between angiotensin II and insulin signaling systems. Endocrinology. (2005) 146:579–88. doi: 10.1210/en.2004-0466
17. Liu Y, Yang Y, Zhang C, Huang F, Wang F, Yuan J, et al. Clinical and biochemical indexes from 2019-nCoV infected patients linked to viral loads and lung injury. Sci China Life Sci. (2020) 63:364–74. doi: 10.1007/s11427-020-1643-8
18. Rieder M, Wirth L, Pollmeier L, Jeserich M, Goller I, Baldus N, et al. Serum ACE2, angiotensin II, and aldosterone levels are unchanged in patients with COVID-19. Am J Hypertens. (2021) 34:278–81. doi: 10.1093/ajh/hpaa169
19. Wu Z, Hu R, Zhang C, Ren W, Yu A, Zhou X. Elevation of plasma angiotensin II level is a potential pathogenesis for the critically ill COVID-19 patients. Crit Care. (2020) 24:290. doi: 10.1186/s13054-020-03015-0
20. Mansour E, Palma AC, Ulaf RG, Ribeiro LC, Bernardes AF, Nunes TA, et al. Safety and outcomes associated with the pharmacological inhibition of the kinin-kallikrein system in severe COVID-19. Viruses. (2021) 13:309. doi: 10.3390/v13020309
21. Pan F, Ye T, Sun P, Liang B, Li L, Zheng D, et al. Time course of lung changes at chest CT during recovery from coronavirus disease 2019 (COVID-19). Radiology. (2020) 295:715–21.
24. Samprathi M, Jayashree M. Biomarkers in COVID-19: an up-to-date review. Front Pediatr. (2020) 8:607647. doi: 10.3389/fped.2020.607647
25. Henry BM, Benoit S, Lippi G, Benoit J. Letter to the Editor - Circulating plasma levels of angiotensin II and aldosterone in patients with coronavirus disease 2019 (COVID-19): a preliminary report. Prog Cardiovasc Dis. (2020) 63:702–3. doi: 10.1016/j.pcad.2020.07.006
26. Valle Martins AL, da Silva FA, Bolais-Ramos L, de Oliveira GC, Ribeiro RC, Pereira DAA, et al. Increased circulating levels of angiotensin-(1-7) in severely ill COVID-19 patients. ERJ Open Res. (2021) 7:00114–2021. doi: 10.1183/23120541.00114-2021
27. Wu Z, McGoogan JM. Characteristics of and important lessons from the coronavirus disease 2019 (COVID-19) outbreak in china: summary of a report of 72314 cases from the Chinese center for disease control and prevention. JAMA. (2020) 323:1239–42. doi: 10.1001/jama.2020.2648
28. Kai H, Kai M. Interactions of coronaviruses with ACE2, angiotensin II, and RAS inhibitors-lessons from available evidence and insights into COVID-19. Hypertens Res. (2020) 43:648–54. doi: 10.1038/s41440-020-0455-8
29. Kuba K, Imai Y, Rao S, Gao H, Guo F, Guan B, et al. A crucial role of angiotensin converting enzyme 2 (ACE2) in SARS coronavirus-induced lung injury. Nat Med. (2005) 11:875–9.
30. Schultze JL, Aschenbrenner AC. COVID-19 and the human innate immune system. Cell. (2021) 184:1671–92.
31. Osman IO, Melenotte C, Brouqui P, Million M, Lagier JC, Parola P, et al. Expression of ACE2, soluble ACE2, angiotensin I, angiotensin II and Angiotensin-(1-7) is modulated in COVID-19 patients. Front Immunol. (2021) 12:625732. doi: 10.3389/fimmu.2021.625732
32. Doerschug KC, Delsing AS, Schmidt GA, Ashare A. Renin-angiotensin system activation correlates with microvascular dysfunction in a prospective cohort study of clinical sepsis. Crit Care. (2010) 14:R24. doi: 10.1186/cc8887
34. van Kats JP, de Lannoy LM, Jan Danser AH, van Meegen JR, Verdouw PD, Schalekamp MA. Angiotensin II type 1 (AT1) receptor-mediated accumulation of angiotensin II in tissues and its intracellular half-life in vivo. Hypertension. (1997) 30:42–9. doi: 10.1161/01.hyp.30.1.42
35. Thoreau B, Galland J, Delrue M, Neuwirth M, Stepanian A, Chauvin A, et al. D-Dimer level and neutrophils count as predictive and prognostic factors of pulmonary embolism in severe non-ICU COVID-19 patients. Viruses. (2021) 13:758. doi: 10.3390/v13050758
36. Qeadan F, Tingey B, Gu LY, Packard AH, Erdei E, Saeed AI. Prognostic values of serum ferritin and D-Dimer trajectory in patients with COVID-19. Viruses. (2021) 13:419. doi: 10.3390/v13030419
37. Ali A, Noman M, Guo Y, Liu X, Zhang R, Zhou J, et al. Myoglobin and C-reactive protein are efficient and reliable early predictors of COVID-19 associated mortality. Sci Rep. (2021) 11:5975.
Keywords: angiotensin converting enzyme 2, coronavirus, inflammation, lung hypertension, renin, lung, SARS-CoV-2, biomarker
Citation: Camargo RL, Bombassaro B, Monfort-Pires M, Mansour E, Palma AC, Ribeiro LC, Ulaf RG, Bernardes AF, Nunes TA, Agrela MV, Dertkigil RP, Dertkigil SS, Araujo EP, Nadruz W, Moretti ML, Velloso LA and Sposito AC (2022) Plasma Angiotensin II Is Increased in Critical Coronavirus Disease 2019. Front. Cardiovasc. Med. 9:847809. doi: 10.3389/fcvm.2022.847809
Received: 03 January 2022; Accepted: 31 May 2022;
Published: 24 June 2022.
Edited by:
Alaa Omar, Mount Sinai St. Luke’s Hospital, United StatesReviewed by:
Diana Maria Ronderos, Bronx Lebanon Hospital, United StatesFrancisco Cano, University of Chile, Chile
Copyright © 2022 Camargo, Bombassaro, Monfort-Pires, Mansour, Palma, Ribeiro, Ulaf, Bernardes, Nunes, Agrela, Dertkigil, Dertkigil, Araujo, Nadruz, Moretti, Velloso and Sposito. This is an open-access article distributed under the terms of the Creative Commons Attribution License (CC BY). The use, distribution or reproduction in other forums is permitted, provided the original author(s) and the copyright owner(s) are credited and that the original publication in this journal is cited, in accordance with accepted academic practice. No use, distribution or reproduction is permitted which does not comply with these terms.
*Correspondence: Licio A. Velloso, bGF2ZWxsb3NAdW5pY2FtcC5icg==
†These authors have contributed equally to this work