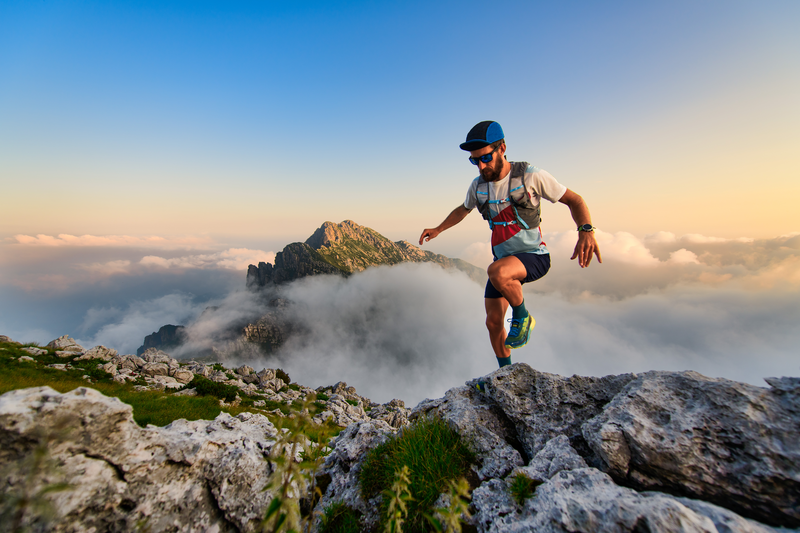
95% of researchers rate our articles as excellent or good
Learn more about the work of our research integrity team to safeguard the quality of each article we publish.
Find out more
ORIGINAL RESEARCH article
Front. Cardiovasc. Med. , 23 February 2022
Sec. Heart Failure and Transplantation
Volume 9 - 2022 | https://doi.org/10.3389/fcvm.2022.842554
Introduction: The determination of ventriculo-arterial coupling is gaining an increasing role in cardiovascular and sport medicine. However, its relevance in critically ill patients is still under investigation. In this study we measured the association between ventriculo-arterial coupling and oxygen consumption (VO2) response after hemodynamic interventions in cardiac surgery patients with acute circulatory instability.
Material and Methods: Sixty-one cardio-thoracic ICU patients (67 ± 12 years, 80% men) who received hemodynamic therapeutic interventions (fluid challenge or norepinephrine infusion) were included. Arterial pressure, cardiac output, heart rate, arterial (EA), and ventricular elastances (EV), total indexed peripheral resistances were assessed before and after hemodynamic interventions. VO2 responsiveness was defined as VO2 increase >15% following the hemodynamic intervention. Ventriculo-arterial coupling was assessed measuring the EA/EV ratio by echocardiography. The left ventricle stroke work to pressure volume area ratio (SW/PVA) was also calculated.
Results: In the overall cohort, 24 patients (39%) were VO2 responders, and 48 patients had high ventriculo-arterial (EA/EV) coupling ratio with a median value of 1.9 (1.6–2.4). Most of those patients were classified as VO2 responders (28 of 31 patients, p = 0.031). Changes in VO2 were correlated with those of indexed total peripheral resistances, EA, EA/EV and cardiac output. EA/EV ratio predicted VO2 increase with an AUC of 0.76 [95% CI: 0.62–0.87]; p = 0.001. In principal component analyses, EA/EV and SW/PVA ratios were independently associated (p < 0.05) with VO2 response following interventions.
Conclusions: VO2 responders were characterized by baseline high ventriculo-arterial coupling ratio due to high EA and low EV. Baseline EA/EV and SW/PVA ratios were associated with VO2 changes independently of the hemodynamic intervention used. These results underline the pathophysiological significance of measuring ventriculo-arterial coupling in patients with hemodynamic instability, as a potential therapeutic target.
Acute circulatory failure following cardiac surgery is characterized by an imbalance between oxygen delivery (DO2) and oxygen consumption (VO2) which results in tissue hypoxia and organ dysfunction (1). The challenge in clinical practice is the identification of parameters that are clinically relevant to become endpoints for titration of interventions. Increasing DO2 is an accepted goal for optimization following cardiac surgery, especially if it is decreased (2, 3). However, this increase might not be beneficial in all patients. Thus, a concomitant increase in VO2 and DO2 could identify those patients for whom DO2 increase through therapeutic interventions is most beneficial and cost-efficient.
Complementary to the classical heart-centered pressure-flow model, the ventriculo-arterial coupling (V-A coupling) concept describes the interactions between the ventricles and the large arteries from an integrated pressure-volume relationship (4–6). The left ventricle (LV) and the arterial system are described by their elastances [i.e. ventricular elastance (EV), arterial elastance (EA)], and V-A coupling is defined by the ratio of EA/EV (4). The efficacy and efficiency of the cardiovascular system are the result of regulated interactions between the heart and the vascular system. The optimal hemodynamic intervention in patients with acute circulatory failure would improve efficacy with the lowest energetic cost (high efficiency) for the cardiovascular system (7).
Cardiology studies have demonstrated that V-A coupling may represent a parameter that describes the energetic cost in particular when LV function is altered (8, 9). There is clear evidence that V-A coupling is a hemodynamic parameter associated with patient outcomes (8, 10–13). The relevance of V-A coupling as a parameter of hemodynamic optimization in patients with acute circulatory failure could be related to the fact that this represents a parameter of cardiovascular efficiency whereas the classical hemodynamic parameters are exclusively parameters of cardiovascular efficacy (2, 3). Guidelines regarding the implementation of V-A coupling in clinical use are available for cardiologists, particularly in the context of heart failure (14). However, studies evaluating V-A coupling-based hemodynamic algorithms in the setting of ICU acute circulatory failure are lacking.
The present study was designed to investigate the clinical relevance of V-A coupling in critically ill patients. We sought to analyse the effects of two types of interventions: fluid challenge or norepinephrine infusion on systemic oxygenation parameters (as indicators of cardiovascular efficacy) and on V-A coupling (as an indicator of cardiovascular efficiency).
The main objective of this study was to investigate the relationship between EA/EV ratio and changes in VO2 upon treatment of hemodynamic instability following cardiac surgery. The second objectives were to compare V-A coupling and oxygenation-derived parameters [central venous saturation (ScVO2), gap of CO2] as predictor of VO2 changes following hemodynamic treatment.
The study's objectives and procedures were approved by the local independent Ethics Committee (Comité de Protection des Personnes Nord-Ouest II CHU - Place V. Pauchet, 80054 AMIENS Cedex 1). All patients received written information and gave their verbal consent to participate. The present manuscript was drafted in compliance with the STROBE checklist for cohort studies (15).
This observational cohort study was performed in a university-affiliated cardiothoracic ICU between 2015 and 2017. We included patients aged 18 or over, under controlled positive ventilation, with hemodynamic instability, for whom the clinician decided to perform a fluid challenge and/or start a norepinephrine infusion. The indications for fluid challenge were: (1) arterial hypotension, defined as systolic arterial pressure (SAP) below 90 mmHg and/or a mean arterial pressure (MAP) below 65 mmHg, and/or (2) stroke volume (SV) variation of more than 10%, and/or (3) clinical signs of hypoperfusion. In the present study, fluid challenge always consisted of a 10-min infusion of 500 ml of lactated Ringer's solution (16). The indication for norepinephrine was persistent arterial hypotension (SAP <100 mmHg and/or MAP <65 mmHg) despite fluid challenge (11, 16). The exclusion criteria were permanent/persistent chronic atrial fibrillation, heart conduction block (type I, II and III atrioventricular blocks, left and right bundle branch blocks), the presence of an active pacemaker, poor acoustic window, more than mild aortic regurgitation, and right heart failure.
Stroke volume (SV; mL) and cardiac output (CO; l min−1) were measured using transthoracic echocardiography performed with a commercially available machine (CX50 ultrasound system Philips Medical System, Suresnes, France) equipped with a S5-1 Sector Array Transducer. The echocardiographic parameters were averaged from five measurements (regardless of the respiratory cycle). EV was estimated at the bedside using the non-invasive single beat method described by Chen et al. (17). This method is based on the assumption that time-variation of LV elastance is not influenced by loading conditions or heart rate. EV was calculated by the formula: EV = (Pd – (ENd(test) * Pes * 0.9)) / (SV * ENd(test)). ENd(test) was obtained from a group-averaged normalized elastance curve value at this same time td (ENd(avg)), baseline LV ejection fraction (LVEF) and the ratio of diastolic to systolic arterial pressure (17). We calculated the coefficient of variation (CV), precision and least significant change (LSC) for EV in ten patients. CV was 7.7% ± 0.6 and LSC was 10.9% ± 0.8. EA was estimated by using the formula EA= end-systolic pressure (ESP = 0.9 * SAP)/SV (18). SAP was measured by using invasive radial artery catheters. In healthy men and women, the mean EA/EV, EA, and EV values measured invasively at rest are respectively 1.0 ± 0.36, 2.2 ± 0.8 mmHg ml−1, and 2.3 ± 1.0 mmHg.ml−1 (18, 19). An abnormal EA/EV ratio was defined as a value >1.36 (19).
The total energy generated by each cardiac contraction is called the “pressure-volume area” (PVA), which is the sum of the external mechanical work exerted during systole (SW) and the potential energy (PE) stored at the end of systole: PVA = SW + PE (19). The PVA has been demonstrated to be linearly related to myocardial oxygen consumption (7, 19). SW is calculated as ESP x SV. Potential energy is calculated as ESP x ((ESV-V0)/2) and assumes that V0 is negligible when compared with ESV. We calculated total indexed peripheral resistance (TPRi) as TPRi = MAP-central venous pressure (CVP)/cardiac index (mmHg ml−1 m−2).
We recorded the ventilator settings (tidal volume, plateau pressure and end-expiratory pressure) at baseline. All parameters were measured on arterial and central venous blood gases (Supplementary File 1).
Anesthesia and cardiopulmonary bypass procedures were standardized for all patients. During the study period, the patients were mechanically ventilated in volume-controlled mode, with a tidal volume set to 7–9 ml kg−1 ideal body weight, and a positive end-expiratory pressure (PEEP) of 5–8 cm H2O, and sedated with Propofol (20). Ventilator settings (oxygen inspired fraction, tidal volume, respiratory rate and end positive pressure) were not modified during the study period.
The following clinical parameters were recorded: age, gender, weight, ventilation parameters, and primary diagnosis. After an equilibration period, HR, SAP, MAP, diastolic arterial pressure (DAP), CVP, SV, CO, and arterial/venous oxygen content were measured at baseline.
In the absence of preliminary data, we designed an observational study with a convenience sample of 61 consecutive patients. Such size could enable to demonstrate a correlation (0.3–0.5) between EA/Ev ratio and VO2 response with a power of 0.8 and alpha error of 0.05. The variables' distribution was assessed using a D'Agostino-Pearson test. Data are expressed as the number, proportion (in percent), mean ± standard deviation (SD) or the median [interquartile range (IQR)], as appropriate. Patients were classified as VO2 responders or non-responders as a function of the effect of hemodynamic interventions (fluid challenge or norepinephrine) on VO2. VO2 response was defined as an increase of more than 15% in the VO2. The non-parametric Wilcoxon rank sum test, Student's paired t-test, Student's t-test, the Mann-Whitney test, and the Fisher's test were used to assess statistical significance, as appropriate. Correlations were tested using Pearson's or Spearman's rank test, as appropriate.
Because we have analyzed several correlated hemodynamic and perfusion variables, we performed an exploratory principal component analysis to avoid collinearity. The principal component analysis transforms correlated variables into uncorrelated variables that may explain VO2 changes. A principal component analysis was carried out by including fourteen baseline variables. The VO2 changes following therapeutic interventions were included as a supplementary variable. Considering there were some differences between baseline characteristics between VO2 responders vs. non-responders in univariate, we performed a multivariate regression logistical model in order to determinate the independent adjusted associations. The conditions of validity of the multivariate model were checked, in order to have at least 5 responders for each variable included in the model. A receiver-operating characteristic (ROC) curve was established for the ability of ScVO2, CO2 gap, EV/EA ratio to predict an increase of more than 15% in VO2.
The threshold for statistical significance was set to p < 0.05. R software (version 3.5.0) with FactoMineR package was used for all statistical analyses.
Of the 65 included patients, four were excluded (Supplementary File 2), and so the final study population consisted of 61 subjects (Table 1). At baseline 48 patients (78%) were categorized as uncoupled, with a median EA/EV ratio of 1.9 (1.6–2.4) mainly related to abnormally low EV (1.1 (0.9–1.6)), as compared to preserved EA (2 (1.5–2.7)). In the overall population, 31 patients (48 %) were classified as VO2 responders. The percentage of VO2 responders did not differ between the two groups [16 (48%) out of 33 vs. 15 (54%) out of 28, p = 0.799]. Of the 6 patients with mitral regurgitation surgery, 2 were in the group of VO2 responders and 4 in the group of VO2 non responders (Fisher's test, p = 1).
At baseline, VO2 responders had higher EA/EV ratio, and lower SW/PVA ratio and VO2 than VO2 non-responders (Table 2, Figure 1). They also had a lower LVEF. Therapeutic interventions increased SAP, MAP, CO and DO2 in the overall population. VO2 responders were characterized by an increased SW/PVA ratio, and a decreased HR, and TPRi. VO2 non-responders were characterized by an increased EA, EV, ScVO2, TPRi, and a decreased gapCO2.
Table 2. Comparison of haemodynamic parameters in VO2 responders and VO2 non-responders. Values are expressed as the mean (SD) or the median (interquartile range).
Figure 1. Relationship between oxygen delivery (DO2) and oxygen consumption (VO2) in uncoupled and coupled patients before and after the hemodynamic intervention.
At baseline, VO2 responders had lower VO2, gapCO2, and higher EA/EV ratio, ScVO2 than VO2 non-responders. Fluid challenge increased SAP, MAP, and SV in VO2 responders and non-responders. VO2 responders were characterized by an increase in SV and CO decreased TPRi, EA, and an increased SW/PVA ratio and gapCO2. EV did not change. VO2 non-responders were characterized by an increase in SV, ScVO2, and a decreased HR.
At baseline, VO2 responders had lower SV, CO, SW/PVA ratio, VO2, and higher gapCO2, EA/EV ratio than VO2 non-responders. Norepinephrine infusion increased SAP, MAP, CO and DO2 in both groups. VO2 responders were characterized by an increased SV and CO, SW/PVA ratio. VO2 non-responders were characterized by an increased EA, EV, ScVO2.
In the overall cohort, changes in VO2 were correlated with those in SW/PVA ratio (r = 0.362, p = 0.003), EA (r = −0.446, p < 0.001), EA/EV (r = −0.256, p = 0.046), CO (r = 0.495, p < 0.001), ScVO2 (r = −0.522, p < 0.001), TPRi (r = −0.444, p < 0.001). The baseline SW/PVA ratio was correlated with DO2 (r = 0.339, p = 0.004), VO2 (r = 0.258, p = 0.045), and gapCO2 (r = −0.304, p = 0.017).
Baseline EA/EV was predictive of VO2 responsiveness, with an area under the curve (AUC) [95% confidence interval (95%CI)] of 0.76 ([0.62–0.87]; p = 0.001). The best cut-off was 2.1 with a gray zone between 1.8 and 2.4. With an AUC [95%CI] of 0.72 [0.59–0.85] (p = 0.004), baseline ScVO2 was predictive of VO2 responsiveness. With an AUC [95%CI] of 0.44 [0.29–0.58] (p = 0.05), gapCO2 was not predictive of VO2 responsiveness. When analyzing patients separately for fluid challenge or norepinephrine infusion, baseline EA/EV was predictive of VO2 responsiveness in fluid challenge group (AUC: 0.77 [0.59–0.95] (p = 0.008) and norepinephrine group (AUC: 0.74 [0.56–0.93] (p = 0.045).
These results were confirmed by the multivariate regression model adjusted on differences at baseline (LVEF, ScVO2, SW/PVA ratio, EA/EV ratio). Baseline EA/EV ratio (OR: 3.4 [1.1–10.3], p = 0.033) and ScVO2 (OR: 1.08 [1.01–1.15], p = 0.016) were independently associated with VO2 responsiveness, but not the baseline LVEF (OR: 1 [0.93–1.07], p = 0.993) and SW/PVA ratio (OR: 0.5 [0–13], p = 0.297). When using the principal component analysis, the 3 first principal components explained 61% of the variance (Supplementary Table 1, Supplementary Figures 1, 2). VO2 changes were significantly associated with the first (r = 0.31) and the third component (r = 0.52). EA, EV, EA/EV, and SW/PVA ratio were variables included in components associated to VO2 changes.
The main results of the present study are as follow: (1) most patients for whom fluid challenge or norepinephrine infusion increased VO2 had high V-A coupling ratio with lower left ventricle stroke work to pressure volume area ratio (SW/PVA) at baseline; (2) baseline EA/EV and SW/PVA ratios were associated with perfusion parameters and VO2 changes independently of the therapeutic intervention used.
When analyzing together fluid challenge or norepinephrine infusion, the only common profile is the increase in arterial pressure. VO2 responders have an increase in SV, CO and a decrease in TPRi. VO2 responder patients were uncoupled before interventions as they adapted to maintain tissue perfusion with a higher energetic cost for the same efficacy (preserving efficacy over efficiency). This was reflected by the lower EA/EV ratio in VO2-responders. Equally, VO2-responder patients had significantly lower SW/PVA values before hemodynamic intervention, which were associated to perfusion parameters. We demonstrated that the EA/EV ratio partly explains VO2 responsiveness and was independently associated with VO2 responsiveness. Both approaches credibly establish at least the statistical relevance of analyzing V-A coupling in patients with hemodynamic instability following cardiac surgery.
It has been shown that mechanical efficiency is greatest when EA = EV (i.e., EA/EV ratio = 1) (4, 6, 21). The patients of the present study were characterized by “normal” EA but much lower than normal EV values, resulting in 78% patients having high V-A coupling ratio. Burkhoff and Sagawa have also shown that the mechanical efficiency of the heart is more sensitive to EA, especially when EV is impaired, which is observed at baseline in the patients of the present study (21). “Sacrificing” efficiency to preserve efficacy for a limited period of time is a “physiological choice” observed in athletes (22). Patients with the most severe V-A coupling (i.e., the highest V-A coupling ratio) had the lowest VO2 (22). The consequences of long term “sacrificing efficiency,” (i.e., days) for the ICU patients are not known. For instance, the fact that catecholamine use is associated with increased mortality could be an example of deleterious long-term consequences of providing better cardiovascular performance at a high energetic cost (23).
Investigating the effects of two interventions on V-A coupling comes down to answering the question already raised many years ago: how effective is an increase in myocardial performance (i.e., an increase in SV) transmitted to the peripheral circulation (24). This transmission may be mediated by the V-A coupling (24). In this respect, if the increase in cardiac performance is transmitted to the circulation, this should result into opening new vascular beds, and if DO2 limits the VO2, this should result in an increase in VO2. This is what our results demonstrate, linking the increase in cardiac performance with the peripheral circulation through the V-A coupling.
Cardiologists have already integrated V-A coupling based hemodynamic approach in the treatment of chronic heart failure or arterial hypertension (9, 14, 25). In the ICU, several attempts of hemodynamic optimisation based on V-A coupling perspective were published (25, 26). Few studies have investigated the relationship between V-A coupling on one side and DO2 and VO2 on the other in ICU patients (10, 27). To the best of our knowledge, this is the first attempt that has specifically focused on VO2. V-A coupling has been already demonstrated as a factor limiting patients' adaptability to effort (8). Previous authors have studied the association of V-A coupling improvement and the time course of systemic oxygenation parameters in trauma patients (27, 28). Our results support their findings by demonstrating an association between V-A coupling, SW/PVA ratio, to perfusion parameters and further VO2 changes.
Sepsis and septic shock are characterized by different profiles of V-A coupling (i.e., different hemodynamic profiles) for which hemodynamic treatment may differ (29). More than two thirds of sepsis patients are V-A uncoupled (30, 31). The fact that most uncoupled patients showed an increase in VO2 after therapeutic interventions could be a further argument for targeting V-A coupling during resuscitation, as marker of tissular perfusion. This therapeutic option might help in better choosing the responsive patients, as a vasopressor-sparing strategy (32). The norepinephrine infusion alters V-A coupling with an increase in Ea >> Ev (30, 33). Targeting primarily the MAP of 65 mmHg might sustain an unnecessary (34) and possibly an unfavorable prolonged energetic state because of increased cardiac afterload (32). Algorithms using the monitoring dynamic arterial elastance were proposed in ICU and they demonstrated that early weaning of norepinephrine may be associated with better tissue perfusion (12, 35). As the dynamic arterial elastance was shown to be correlated with V-A coupling and microcirculation (36, 37), the connection with VO2 in our study might contribute with a new puzzle piece. One advantage of V-A coupling is that it can be non-invasively measured at bedside. Contrary to perfusion parameters, it does not require blood sampling. Further randomized study evaluating a hemodynamic strategy based on V-A coupling may confirmed these points.
Firstly, the analysis of two therapeutic interventions can make interpretation of the results difficult. The present objective was not to precisely analyze the individual effect of each therapy. Such demonstrations have been previously published (17). On the contrary, we would like to demonstrate that a hemodynamic approach based on the V-A coupling makes it possible to dispense with the hemodynamic treatment and a detailed analysis of each parameter. The fact that the association between V-A coupling and perfusion parameters was demonstrated in the population as a whole and in each treatment, group reinforces our results. As discussed, we believe that the effects of norepinephrine on VO2 may be due to its effects on CO and DO2 (38).
Secondly, the VO2/DO2 relationship is not linear. The VO2 responder group has lower values of VO2 that are below those in the non-responder group, even after hemodynamic treatment. We believe the lower value of VO2 in responder group may not have introduce bias. These observations are in relation with the fact that the hemodynamic response was defined by VO2 changes. The methods used to calculate EV and EA can potentially be criticized because we did not use a high-fidelity ventricular pressure catheter (17). We calculated ESP from a radial artery signal, which may differ from the aortic pressure signal. However, radial artery pressure has been reported to provide a good estimate of ESP (39). Although it can be argued that estimation of ESP from the radial artery has not been fully validated, any error in this method would only affect the precision of absolute values of EA and EV, but not the EA/EV ratio, as the error in end-systolic pressure would be similar. Despite these limitations, non-invasive evaluation of EV and EA was validated against the gold standard method and has been used in cardiac surgery (5–7). In the present study, EA and EV must be considered to be approximations of EA and EV. Despite these limitations, non-invasive evaluation was validated against the gold standard method, and have been used in the cardiology and cardiac surgical fields (14).
Thirdly, our findings generalizability is restricted by the low number of patients analyzed in this cohort. Considering the known heterogeneity of intensive care unit (ICU) patients, larger cohorts are required to perform a properly matching on different confounding factors. The clinical relevance of the relationship between V-A coupling and VO2 in the context of goal-directed therapy in critically ill patients is still to be validated. The results of well-designed interventional trials, such as the one published by Borlaug et al that used LV afterload reduction, are awaited (40).
In VO2 responders, V-A coupling was characterized by a high EA/EV ratio (due to high EA and low EV). Baseline EA/EV and SW/PVA ratios were associated with VO2 changes independently of the hemodynamic intervention used. Measuring V-A coupling may offer a new perspective of hemodynamic optimisation in the ICU by individualizing hemodynamic treatment and by analyzing both the efficacy and efficiency of hemodynamic interventions.
The raw data supporting the conclusions of this article will be made available by the authors, without undue reservation.
The studies involving human participants were reviewed and approved by (Comité de Protection des Personnes Nord-Ouest II CHU - Place V. Pauchet, 80054 AMIENS Cedex 1). The patients/participants provided their written informed consent to participate in this study.
SA, MN, and P-GG conceived the study, analyzed the data and drafted the manuscript. P-GG collected the data. DL, BP, and BB provided a critical review. All authors contributed to the article and approved the submitted version.
The authors declare that the research was conducted in the absence of any commercial or financial relationships that could be construed as a potential conflict of interest.
All claims expressed in this article are solely those of the authors and do not necessarily represent those of their affiliated organizations, or those of the publisher, the editors and the reviewers. Any product that may be evaluated in this article, or claim that may be made by its manufacturer, is not guaranteed or endorsed by the publisher.
The Supplementary Material for this article can be found online at: https://www.frontiersin.org/articles/10.3389/fcvm.2022.842554/full#supplementary-material
1. Schumacker PT. CSM. The concept of a critical oxygen delivery. Intensive Care Med. (1987) 13:223–9. doi: 10.1007/BF00265110
2. Osawa EA, Rhodes A, Landoni G, Galas FRBG, Fukushima JT, Park CHL, et al. Effect of perioperative goal-directed hemodynamic resuscitation therapy on outcomes following cardiac surgery: a randomized clinical trial and systematic review. Crit Care Med. (2016) 44:724–33. doi: 10.1097/CCM.0000000000001479
3. De Backer D. Detailing the cardiovascular profile in shock patients. Crit Care Lond Engl. (2017) 21:311. doi: 10.1186/s13054-017-1908-6
4. Sagawa K, Suga H, Shoukas AA, Bakalar KM. End-systolic pressure/volume ratio: a new index of ventricular contractility. Am J Cardiol. (1977) 40:748–53. doi: 10.1016/0002-9149(77)90192-8
5. Starling MR. Left ventricular-arterial coupling relations in the normal human heart. Am Heart J. (1993) 125:1659–66. doi: 10.1016/0002-8703(93)90756-Y
6. Asanoi H, Sasayama S, Kameyama T. Ventriculoarterial coupling in normal and failing heart in humans. Circ Res. (1989) 65:483–93. doi: 10.1161/01.RES.65.2.483
7. Takaoka H, Takeuchi M, Odake M, Yokoyama M. Assessment of myocardial oxygen consumption (Vo2) and systolic pressure-volume area (PVA) in human hearts. Eur Heart J. (1992) 13 Suppl E:85–90. doi: 10.1093/eurheartj/13.suppl_E.85
8. Aslanger E, Assous B, Bihry N, Beauvais F, Logeart D, Cohen-Solal A. Association between baseline cardiovascular mechanics and exercise capacity in patients with coronary artery disease. Anatol J Cardiol. (2016) 16:608–13. doi: 10.5152/AnatolJCardiol.2015.6471
9. Maurer MS, Sackner-Bernstein JD, El-Khoury Rumbarger L, Yushak M, King DL, Burkhoff D. Mechanisms underlying improvements in ejection fraction with carvedilol in heart failure. Circ Heart Fail. (2009) 2:189–96. doi: 10.1161/CIRCHEARTFAILURE.108.806240
10. Chang MC, Mondy JS, Meredith JW, Holcroft JW. Redefining cardiovascular performance during resuscitation: ventricular stroke work, power, and the pressure-volume diagram. J Trauma. (1998) 45:470–8. doi: 10.1097/00005373-199809000-00007
11. Guinot P-G, Longrois D, Kamel S, Lorne E, Dupont H. Ventriculo-Arterial Coupling Analysis Predicts the Hemodynamic Response to Norepinephrine in Hypotensive Postoperative Patients: A Prospective Observational Study. Crit Care Med. (2018) 46:e17–25. doi: 10.1097/CCM.0000000000002772
12. Guinot P-G, Abou-Arab O, Guilbart M, Bar S, Zogheib E, Daher M, et al. Monitoring dynamic arterial elastance as a means of decreasing the duration of norepinephrine treatment in vasoplegic syndrome following cardiac surgery: a prospective, randomized trial. Intensive Care Med. (2017) 43:643–51. doi: 10.1007/s00134-016-4666-z
13. Antonini-Canterin F, Enache R, Popescu BA, Popescu AC, Ginghina C, Leiballi E, et al. Prognostic value of ventricular-arterial coupling and B-type natriuretic peptide in patients after myocardial infarction: a five-year follow-up study. J Am Soc Echocardiogr. (2009) 22:1239–45. doi: 10.1016/j.echo.2009.08.009
14. Ikonomidis I, Aboyans V, Blacher J, Brodmann M, Brutsaert DL, Chirinos JA, et al. The role of ventricular-arterial coupling in cardiac disease and heart failure: assessment, clinical implications and therapeutic interventions. A consensus document of the European Society of Cardiology Working Group on Aorta & Peripheral Vascular Diseases, European Association of Cardiovascular Imaging, and Heart Failure Association. Eur J Heart Fail. (2019) 21:402–24. doi: 10.1002/ejhf.1436
15. von Elm E, Altman DG, Egger M, Pocock SJ, Gøtzsche PC, Vandenbroucke JP, et al. The Strengthening the Reporting of Observational Studies in Epidemiology (STROBE) statement: guidelines for reporting observational studies. Ann Intern Med. (2007) 147:573–7. doi: 10.7326/0003-4819-147-8-200710160-00010
16. Huette P, Abou-Arab O, Longrois D, Guinot P-G. Fluid expansion improve ventriculo-arterial coupling in preload-dependent patients: a prospective observational study. BMC Anesthesiol. (2020) 20:171. doi: 10.1186/s12871-020-01087-7
17. Chen CH, Fetics B, Nevo E, Rochitte CE, Chiou KR, Ding PA, et al. Noninvasive single-beat determination of left ventricular end-systolic elastance in humans. J Am Coll Cardiol. (2001) 38:2028–34. doi: 10.1016/S0735-1097(01)01651-5
18. Kelly RP, Ting CT, Yang TM, Liu CP, Maughan WL, Chang MS, et al. Effective arterial elastance as index of arterial vascular load in humans. Circulation. (1992) 86:513–21. doi: 10.1161/01.CIR.86.2.513
19. Kass DA. Ventricular arterial stiffening: integrating the pathophysiology. Hypertens. (2005) 46:185–93. doi: 10.1161/01.HYP.0000168053.34306.d4
20. Quintard H., l'Her E, Pottecher J, Adnet F, Constantin J-M, De Jong A, et al. Intubation and extubation of the ICU patient. Anaesth Crit Care Pain Med. (2017) 36:327–41. doi: 10.1016/j.accpm.2017.09.001
21. Burkhoff D, Sagawa K. Ventricular efficiency predicted by an analytical model. Am J Physiol. (1986) 250:R1021–1027. doi: 10.1152/ajpregu.1986.250.6.R1021
22. Sahlén A, Shahgaldi K, Aagaard P, Manouras A, Winter R, Braunschweig F. Altered ventriculo-arterial coupling during exercise in athletes releasing biomarkers after endurance running. Eur J Appl Physiol. (2012) 112:4069–79. doi: 10.1007/s00421-012-2396-7
23. Fellahi JL, Fischer MO, Daccache G, Gerard JL, Hanouz JL. Positive inotropic agents in myocardial ischemia-reperfusion injury: a benefit/risk analysis. Anesthesiology. (2013) 118:1460–5. doi: 10.1097/ALN.0b013e31828f4fc3
24. Freeman GL, Colston JT. Role of ventriculovascular coupling in cardiac response to increased contractility in closed-chest dogs. J Clin Invest. (1990) 86:1278–84. doi: 10.1172/JCI114835
25. Chirinos JA. Ventricular-arterial coupling: Invasive and non-invasive assessment. Artery Res. (2013) 7:2–14.. doi: 10.1016/j.artres.2012.12.002
26. Morelli A, Singer M, Ranieri VM, D'Egidio A, Mascia L, Orecchioni A, et al. Heart rate reduction with esmolol is associated with improved arterial elastance in patients with septic shock: a prospective observational study. Intensive Care Med. (2016) 42:1528–34. doi: 10.1007/s00134-016-4351-2
27. Chang MC, Martin RS, Scherer LA, Meredith JW. Improving ventricular-arterial coupling during resuscitation from shock: effects on cardiovascular function and systemic perfusion. J Trauma. (2002) 53:679–85. doi: 10.1097/00005373-200210000-00010
28. Martin RS, Norris PR, Kilgo PD, Miller PR, Hoth JJ, Meredith JW, et al. Validation of stroke work and ventricular arterial coupling as markers of cardiovascular performance during resuscitation. J Trauma. (2006) 60:930–4; discussion 934-935. doi: 10.1097/01.ta.0000217943.72465.52
29. Bouhemad B, Nicolas-Robin A, Arbelot C, Arthaud M, Féger F, Rouby J-J. Acute left ventricular dilatation and shock-induced myocardial dysfunction. Crit Care Med. (2009) 37:441–7. doi: 10.1097/CCM.0b013e318194ac44
30. Guarracino F, Ferro B, Morelli A, Bertini P, Baldassarri R, Pinsky MR. Ventriculoarterial decoupling in human septic shock. Crit Care Lond Engl. (2014) 18:R80. doi: 10.1186/cc13842
31. Zhou X, Pan J, Wang Y, Wang H, Xu Z, Zhuo W. Left ventricular-arterial coupling as a predictor of stroke volume response to norepinephrine in septic shock - a prospective cohort study. BMC Anesthesiol. (2021) 21:56. doi: 10.1186/s12871-021-01276-y
32. Guinot P-G, Martin A, Berthoud V, Voizeux P, Bartamian L, Santangelo E, et al. Vasopressor-Sparing Strategies in Patients with Shock: a Scoping-Review and an Evidence-Based Strategy Proposition. J Clin Med. (2021) 10:3164. doi: 10.3390/jcm10143164
33. Nguyen M, Mallat J, Marc J, Abou-Arab O, Bouhemad B, Guinot P-G. Arterial load and norepinephrine are associated with the response of the cardiovascular system to fluid expansion. Front Physiol. (2021) 12:707832. doi: 10.3389/fphys.2021.707832
34. Lamontagne F, Richards-Belle A, Thomas K, Harrison DA, Sadique MZ, Grieve RD, et al. Effect of Reduced Exposure to Vasopressors on 90-Day Mortality in Older Critically Ill Patients With Vasodilatory Hypotension: A Randomized Clinical Trial. JAMA. (2020) 323:938–49. doi: 10.1001/jama.2020.0930
35. Nguyen M, Abou-Arab O, Bar S, Dupont H, Bouhemad B, Guinot P-G. Echocardiographic measure of dynamic arterial elastance predict pressure response during norepinephrine weaning: an observational study. Sci Rep. (2021) 11:2853. doi: 10.1038/s41598-021-82408-9
36. Monge García MI, Jian Z, Hatib F, Settels JJ, Cecconi M, Pinsky MR. Dynamic Arterial Elastance as a Ventriculo-Arterial Coupling Index: An Experimental Animal Study. Front Physiol. (2020) 11:284. doi: 10.3389/fphys.2020.00284
37. Bar S, Nguyen M, Abou-Arab O, Dupont H, Bouhemad B, Guinot P-G. Dynamic arterial elastance is associated with the vascular waterfall in patients treated with norepinephrine: an observational study. Front Physiol. (2021) 12:583370. doi: 10.3389/fphys.2021.583370
38. Scheeren TW, Arndt JO. Different response of oxygen consumption and cardiac output to various endogenous and synthetic catecholamines in awake dogs. Crit Care Med. (2000) 28:3861–8. doi: 10.1097/00003246-200012000-00021
39. Haedersdal C, Madsen JK, Saunamäki K. The left ventricular end-systolic pressure and pressure-volume index. Comparison between invasive and auscultatory arm pressure measurements. Angiology. (1993) 44:959–64. doi: 10.1177/000331979304401206
Keywords: ventricular-arterial coupling, oxygen delivery, oxygen consumption, fluid therapy, norepinephrine, acute circulatory failure
Citation: Andrei S, Nguyen M, Longrois D, Popescu BA, Bouhemad B and Guinot P-G (2022) Ventriculo-Arterial Coupling Is Associated With Oxygen Consumption and Tissue Perfusion in Acute Circulatory Failure. Front. Cardiovasc. Med. 9:842554. doi: 10.3389/fcvm.2022.842554
Received: 23 December 2021; Accepted: 31 January 2022;
Published: 23 February 2022.
Edited by:
Nicolas Girerd, INSERM CIC1433 CIC Pierre Drouin, FranceReviewed by:
Aleksander Dokollari, St. Michael's Hospital, CanadaCopyright © 2022 Andrei, Nguyen, Longrois, Popescu, Bouhemad and Guinot. This is an open-access article distributed under the terms of the Creative Commons Attribution License (CC BY). The use, distribution or reproduction in other forums is permitted, provided the original author(s) and the copyright owner(s) are credited and that the original publication in this journal is cited, in accordance with accepted academic practice. No use, distribution or reproduction is permitted which does not comply with these terms.
*Correspondence: Stefan Andrei, c3RlZmFuLm0uYW5kcmVpQGdtYWlsLmNvbQ==
Disclaimer: All claims expressed in this article are solely those of the authors and do not necessarily represent those of their affiliated organizations, or those of the publisher, the editors and the reviewers. Any product that may be evaluated in this article or claim that may be made by its manufacturer is not guaranteed or endorsed by the publisher.
Research integrity at Frontiers
Learn more about the work of our research integrity team to safeguard the quality of each article we publish.