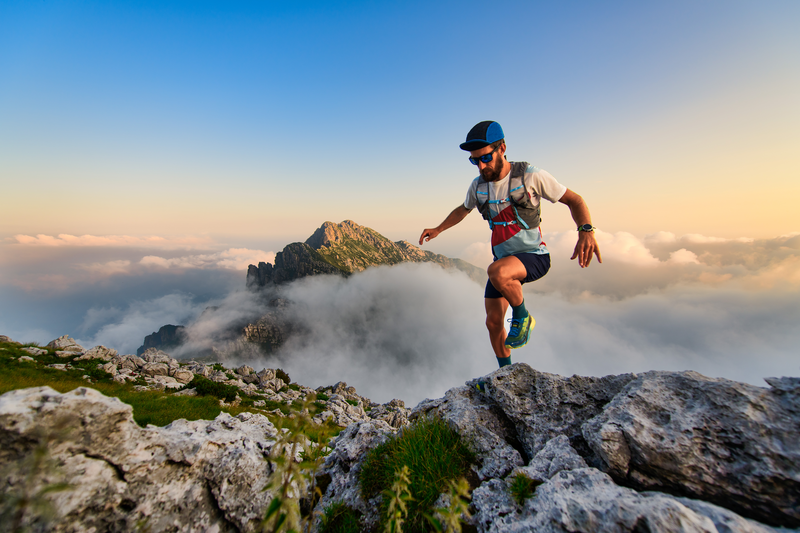
94% of researchers rate our articles as excellent or good
Learn more about the work of our research integrity team to safeguard the quality of each article we publish.
Find out more
SYSTEMATIC REVIEW article
Front. Cardiovasc. Med. , 28 April 2022
Sec. Cardiovascular Therapeutics
Volume 9 - 2022 | https://doi.org/10.3389/fcvm.2022.841654
Objective: Myocardial ischemia/reperfusion injury (IRI) is a common and serious complication in clinical practice. Sevoflurane conditionings have been identified to provide a protection against myocardial IRI in animal experiments, but their true clinical benefits remain controversial. Here, we aimed to analyze the preclinical evidences obtained in animal models of myocardial IRI and explore the possible reasons for controversial clinical benefits.
Methods: Our primary outcome was the difference in mean infarct size between the sevoflurane and control groups in animal models of myocardial IRI. After searching the databases of PubMed, Embase, Web of Science, and the Cochrane Library, a systematic review retrieved 37 eligible studies, from which 28 studies controlled comparisons of sevoflurane preconditioning (SPreC) and 40 studies controlled comparisons of sevoflurane postconditioning (SPostC) that were made in a pooled random-effects meta-analysis. In total, this analysis included data from 313 control animals and 536 animals subject to sevoflurane conditionings.
Results: Pooled estimates for primary outcome demonstrated that sevoflurane could significantly reduce the infarct size after myocardial IRI whether preconditioning [weighted mean difference (WMD): −18.56, 95% CI: −23.27 to −13.85, P < 0.01; I2 = 94.1%, P < 0.01] or postconditioning (WMD: −18.35, 95% CI: −20.88 to −15.83, P < 0.01; I2 = 90.5%, P < 0.01) was performed. Interestingly, there was significant heterogeneity in effect size that could not be explained by any of the prespecified variables by meta-regression and stratified analysis. However, sensitivity analysis still identified the cardioprotective benefits of sevoflurane conditionings with robust results.
Conclusion: Sevoflurane conditionings can significantly reduce infarct size in in-vivo models of myocardial IRI. Given the fact that there is a lack of consistency in the quality and design of included studies, more well-performed in-vivo studies with the detailed characterization of sevoflurane protocols, especially studies in larger animals regarding cardioprotection effects of sevoflurane, are still required.
Ischemic heart disease (IHD), especially acute myocardial infarction, is one of the leading causes of morbidity and mortality of the world populations (1). It is generally believed that timely and successful restoration of blood supply to the ischemic area is the most effective method to rescue the ischemic myocardium and improve clinical outcomes (2). In clinical practice, moreover, various treatment strategies for restoration of blood supply to the ischemic myocardium, such as primary percutaneous coronary intervention or coronary artery bypass grafting (CABG), have been widely used. As a “double-edged sword,” however, reperfusion of blood flow to ischemic myocardium can further worsen damage in the ischemic myocardium and even cause more deaths of cardiomyocytes, which are even attributable to about 50% of final myocardial infarct size (3, 4). This phenomenon is called as myocardial ischemia/reperfusion injury (IRI). For decades, abundant studies have been conducted, but the physiopathology mechanism underlying myocardial IRI remains elusive. The available literatures indicate that oxidative stress, apoptosis, and inflammation are extensively involved in the development of myocardial IRI (3, 5). Unfortunately, as an intractable clinical issue, there have been still no effective interventions against myocardial IRI (6). Hence, developing reasonable and practical countermeasures to provide protection against myocardial IRI are extremely essential.
Sevoflurane, an inhaled anesthetic with good pharmacological properties of stable induction and rapid recovery, is extensively applied for anesthesia of cardiac surgery in clinical practice (7). Early in 2007, a meta-analysis of small randomized clinical trials comparing inhaled anesthesia and total intravenous anesthesia (TIVA) has showed that both the sevoflurane and desflurane are associated with significant reductions of myocardial infarction (2.4 vs. 5.1%) and mortality (0.4 vs. 1.6%) (8). Furthermore, a RCT demonstrated a decreased 1-year mortality after CABG with cardiopulmonary bypass (CPB) in patients receiving sevoflurane anesthesia compared with TIVA (17.8 vs. 24.8%) (9). Similarly, animal experiments have also demonstrated that sevoflurane conditionings can provide a protection against myocardial IRI and the underlying mechanisms involve antioxidant, antiapoptosis, and anti-inflammation pathways (10, 11). However, a multicenter study found no difference in the occurrence of myocardial ischemia when comparing sevoflurane vs. TIVA in patients undergoing noncardiac surgery at risk for perioperative myocardial ischemia (12). These inconsistent findings have caused confusion of available evidence about cardioprotective benefits of sevoflurane conditionings.
Undeniably, there are still many differences between the preclinical and clinical studies due to the complexity of clinical situations. Actually, poor reporting of preclinical study protocols can potentially lead to unreliable experimental results and unnecessary clinical trials (13, 14). In contrast, well-designed animal studies can provide both the safe and effective information on relevant factors that may improve the possibility of success in future clinical trials (15). Furthermore, a systematic reviews of preclinical animal data may partly explain the underlying mechanisms of clinical diseases and indicate the directions of clinical treatments (16). Thus, well-designed experimental studies may provide a deep insight into the cardioprotective efficacy of sevoflurane and useful information on relevant factors that may influence outcomes. However, it must be noted that previous preclinical studies regarding cardioprotection of sevoflurane against myocardial IRI are usually small sample size and vary considerably in the quality of representation, resulting in a low power of evidence. Thus, this comprehensive systematic review and meta-analysis were conducted to pool the findings of previous animal studies regarding the cardioprotective effects of sevoflurane conditionings. The main aims of this analysis are to provide a comprehensive evaluation on cardioprotective efficacy of sevoflurane conditionings in in-vivo animal models of myocardial IRI and attempt to explore the possible reasons for controversial clinical benefits.
We systematically searched the PubMed, Embase, Web of Science, and the Cochrane Library for evidence of the cardioprotective effects of sevoflurane conditionings in animal models of myocardial IRI published from the inception to July 2021. The search strategy was using selected keywords and the Medical Subject Headings (MeSH) terms where appropriately specific to each database (Supplementary Material 1). Furthermore, the reference of review articles, meeting abstracts, and comments for additional citations were searched for relevant studies.
Studies that met the following criteria were included for further meta-analysis: (1) Sevoflurane vs. control treatment; (2) Infarct size was determined with blue dye (i.e., Evans blue)/2,3,5-Triphenyltetrazolium chloride (TTC) double staining and expressed as the percentage of infarct area over area at risk (AAR); (3) Animal models without risk factors of cardiovascular diseases (i.e., aging, diabetes, obesity, or hyperlipidemia); (4) Nonhuman setting; (5) Documented durations of ischemia and reperfusion; and (6) Documented times and dosage regimens of the sevoflurane conditionings. The ex-vivo or in-vitro studies assessing cardioprotective effects of sevoflurane conditionings were excluded.
Eligibility assessment was performed independently in an unblinded, standardized manner by Bin Hu and Tian Tian. Two reviewers extracted the data independently from included studies using predefined data fields and the discrepancies were resolved by consensus. The following information of each study is shown in Tables 1A,B (1) Studies' characteristics (i.e., first author's name, year of publication, countries, number of included animals, durations of ischemia, and reperfusion); (2) Animals' characteristics (i.e., species, sex, body weight/age, and anesthetics); (3) Intervention data (i.e., dosage and time of treatment), and (4) For each eligible study, if the associated information was present merely in figures, two reviewers (Bin Hu and Tian Tian) would use the Engauge Digitizer 11.1 to collect data from the statistical graphs independently (52). Then, the mean values would be adopted. If the complete data are still not available, the article with missing data will be excluded from data synthesis.
Table 1A. The characteristics of sevoflurane preconditioning (SPreC) studies included in the meta-analysis.
Table 1B. The characteristics of sevoflurane postconditioning (SPostC) studies included in the meta-analysis.
The quality of included studies was assessed and graded by two reviewers (Bin Hu and Tian Tian) based on the published criteria for animal experiments using the “Animal Research: Reporting of in vivo Experiments” (ARRIVE) guidelines 2.0 and a 12-item quality score (15, 53). The quality of study was assessed independently from data extraction and between assessors in an unblinded, standardized manner by two reviewers (Bin Hu and Tian Tian). Discrepancies were resolved by consensus or by another reviewer (Pei-Pei Hao), if necessary.
For statistical comparisons, sevoflurane interventions were divided into the two main groups: preconditioning (SPreC), in where sevoflurane was given at any time before the onset of ischemia and postconditioning (SPostC), in where sevoflurane was administrated during ischemia or at the beginning of reperfusion. The weighted (unstandardized) mean difference (WMD) in the infarct size between the sevoflurane and control groups was used as primary outcome to determine the cardioprotective efficacy of sevoflurane conditionings. The number of animals in the control group was corrected based on the number of comparisons for each series of experiments (n/number of comparisons) (54). To account for anticipated heterogeneity, effect sizes were pooled by using a random-effects meta-analysis, which considers the within-study and between-study variability and weights each study accordingly (15). The extent of heterogeneity among studies was assessed with the Cochran's Q test and further quantified by I2 statistics. Potential publication bias was assessed by visual inspection of a funnel plot for asymmetry and further detected by the Egger's and Begg's tests. If significant heterogeneity (P< 0.1) was found across the studies, sensitivity analysis was conducted by removing each study in turn. Furthermore, the univariate meta-regression and stratified analysis (i.e., countries, species, ischemia duration, reperfusion duration, and timing regimens of interventions) were proposed to explore the potential sources of heterogeneity for primary outcome. The STATA version 16.0 statistical software (STATA Corporation, College Station, Texas, USA) was applied for analysis of all the data and the GraphPad Prism for Windows (version 9, GraphPad Software Incorporation, San Diego, California, USA) was used for production of all the figures. A P-value <0.05 was considered as statistically significant.
Our initial search identified 1,149 records, including 375 duplicate reports. In total, 774 reports underwent title and abstract screening, which resulted in 698 exclusions. The remaining 76 reports were retrieved for evaluation of detailed full text. Eventually, 39 articles were further excluded; of them, 12 articles were due to difference of study protocols, 11 articles had TTC staining only, 6 articles were ex-vivo studies, 5 articles did not report infarct size, 2 articles were no sample size per group, 2 articles could not acquire full text, and 1 article used animal model with the risk factors of cardiovascular diseases (Figure 1). As a result, 37 literatures [(14 SPreC studies (10, 17, 19, 21, 22, 24–32), 20 SPostC studies (11, 33–51), and 3 both the SPreC and SPostC studies (18, 20, 23)] met our selection criteria and were included in the analysis. Of 37 included studies, 7 studies provided the associated information of infarct size merely in figures, including 3 SPreC studies (10, 30, 31) and 4 SPostC studies (37, 45, 49, 50). Thus, the Engauge Digitizer 11.1 was used to collect data from the statistical graphs and obtained the mean values.
From the 37 included studies, we extracted data on 68 controlled comparisons of sevoflurane conditionings in the models of myocardial IRI. These were split into 28 comparisons assessing SPreC and 40 comparisons evaluating SPostC. In total, our analysis included data from 313 control animals and 536 animals receiving sevoflurane conditionings with statistical correction.
In the pooled analysis using a random-effects model, SPreC compared with control treatment significantly diminished the infarct size (WMD: −18.56, 95% CI: −23.27 to −13.85, P < 0.01). Significant heterogeneity among studies was observed (I2 = 94.1%, P < 0.01) (Figure 2). By systematically excluding each study, the infarct size was still significantly reduced with SPreC over control treatment (Supplementary Table 1). Meta-regression (Table 2 and Supplementary Material 2) and stratified analysis (Table 3 and Supplementary Material 3) did not unmask significant impacts of prespecified covariates (i.e., countries, species, ischemia duration, reperfusion duration, and timing regimens of treatments) on the infarct size sparing benefit of SPreC. Meta-regression showed that country difference might be a source of significant heterogeneity (P< 0.05). Stratified analysis by countries showed that there was still significant heterogeneity between the groups. Especially, compared to other countries, Netherlands showed a large WMD and a wide 95% CI.
Figure 2. Pooled estimates of infarct size for sevoflurane preconditioning (SPreC) vs. control. Forest plots of meta-analysis of SPreC on myocardial infarct size pooled using a random-effects meta-analysis. The study ID is represented by last name of first author and year of publication. If a study ID is repeated, it indicates that same study involves different intervention protocols. Please refer to the details of intervention protocols in Table 1A.
Table 2. The univariate meta-regression analyses to determine potential sources of heterogeneity for infarct size sparing in the included studies of SPreC and SPostC.
Table 3. Stratified analysis of pooled estimates for infarct size sparing in the included studies of SPreC and SPostC.
In accordance with the above effect of SPreC, compared with control treatment, SPostC also significantly diminished the infarct size (WMD: −18.35, 95% CI: −20.88 to −15.83, P < 0.01). Again, significant heterogeneity among studies was observed (I2 = 90.5%, P < 0.01) (Figure 3). Sensitivity analysis by systematically removing each study provided a consistent estimation for the infarct size sparing benefit of SPostC (Supplementary Table 1). Similarly, meta-regression (Table 2 and Supplementary Material 2) and stratified analysis (Table 3 and Supplementary Material 3) showed no relationship between the prespecified covariates and pooled estimates.
Figure 3. Pooled estimates of infarct size for sevoflurane postconditioning (SPostC) vs. control. Forest plots of meta-analysis of SPostC on myocardial infarct size pooled using a random-effects meta-analysis. The study ID is represented by last name of first author and year of publication. If a study ID is repeated, it indicates that same study involves different intervention protocols. Please refer to the details of intervention protocols in Table 1B.
Reports achieved the median ARRIVE guidelines 2.0 score of 16 out of 21 (interquartile range, 15–17) (Figure 4A) and a median 12-item quality score of 7 out of 12 (interquartile range, 6–8) (Figure 4B). The publication bias for the included studies was detected both visually (funnel plot) and mathematically (Egger's and Begg's tests) by plotting the effect size (WMD) of each controlled comparison against its SD for the SPreC and SPostC groups. Absence of publication bias was identified by the Egger's (P = 0.92) and Begg's (P = 0.78) tests in spite of an asymmetrical funnel plot for the SPreC group (Figure 5A). However, for the SPostC group, visual inspection of funnel plots showed that many studies fell outside of 95% CI (Figure 5B). Particularly, publication bias was identified by Egger's test (P < 0.01), despite not indicated by Begg's test (P = 0.28).
Figure 4. Reporting of study quality indicators. Study quality was assessed using the Animal Research: Reporting of in vivo Experiments (ARRIVE) guidelines 2.0 on reporting (A) and a 12-item quality score (B). Values are expressed as the percentage of studies reporting each quality indicator.
Figure 5. Funnel plot for assessment of publication bias for the infarct size in SPreC (A) and SPostC (B). The vertical line represents the mean effect size. The plots were assessed visually, with further analysis of publication bias performed using the Egger's and Begg's tests.
The meta-analysis demonstrated that the included studies were particularly poor in some important areas of experimental design. For example, with respect to the ARRIVE guidelines 2.0, only 11% of included studies reported a sample size calculation, 16% of included studies performed a blinded assessment of outcomes, 5% of included studies declared the protocol registration, and 14% of included studies reported data access (Figure 4A). Regarding the 12-item quality score, moreover, only 27% of studies reported measurement of partial pressure of oxygen (PaO2) or oxygen saturation (SaO2), 38% of studies monitored the sevoflurane concentrations, and 22% of studies monitored the anesthesia depth (Figure 4B).
The main findings of this comprehensive systematic review and meta-analysis are that compared with control treatment, both the SPreC and SPostC can provide a significant protection against myocardial IRI, shown by a significant infarct size sparing. Furthermore, the robust effect is comparable whether sevoflurane conditioning is carried out before the onset of ischemia, during ischemia, or at the time of reperfusion based on in-vivo data over 800 animals. In addition, the benefits of sevoflurane conditionings on myocardial IRI are not significantly affected by countries, species, duration of ischemia or reperfusion, and timing regimens of drug administration.
Myocardial IRI is a common and serious complication in clinical practice and has aroused extensive attention. Whether with the help of mechanical or pharmacological interventions, it is essential to reestablish coronary blood flow to limit myocardial damage following ischemia (55). The pathogenesis underlying myocardial IRI is extraordinarily complex and multifactorial. Among the etiological factors of myocardial IRI, oxidative stress is a vital mechanism, which initiates at the onset of reperfusion and triggers subsequent series of pathophysiological processes. Essentially, oxidative stress is characterized as severe imbalance between exaggerated reactive oxygen species (ROS) generation and inhibited antioxidant defense systems. Thus, ROS is considered as major determinants for adverse ventricular remodeling via promoting myocardial interstitial fibrosis, cardiomyocyte hypertrophy, and induction of cell death (56). Meanwhile, excessive oxidative stress causes mitochondrial permeability transition pore (mPTP) opening and cytochrome C releasing, subsequently triggering the intrinsic apoptosis by activation of caspase-9/3 signaling pathway (57). After reperfusion injury, oxidative stress and apoptosis collectively contribute to the substantial loss of cardiomyocyte and consequently enlarged infarct area. These pathological mechanisms provide the rationale for therapeutic strategies targeted against myocardial IRI.
As sevoflurane is an inhalational anesthetic most commonly used in clinical practice, its cardioprotection has attracted wide concern in the past decades. A recent meta-analysis elaborates the importance of various mechanisms involved in preconditioning and postconditioning of halogenated gases for cardioprotection (58). Several signaling molecules and pathways have been shown to be involved in sevoflurane-induced cardioprotection, but detailed mechanisms are not fully understood yet. To better understand the cardioprotection of sevoflurane conditionings, this analysis also included the in-vivo evidence regarding the potential mechanisms of sevoflurane conditioning reported in available literatures (Supplementary Table 2). Substantial literatures indicate that cardioprotection of sevoflurane conditionings is closely related to its antioxidant, antiapoptosis, and anti-inflammation properties. For example, Zhao et al. (10) established mice model of myocardial IRI in vivo and adult mouse cardiomyocytes model of simulated ischemia and reoxygenation (SI/R) in vitro and demonstrated that SPreC compared with control treatment decreased superoxide generation by 43.6%. Furthermore, this antioxidant property was largely retained in the AMP-activated protein kinase dominant-negative (AMPK-DN) mice, but completely abolished in caveolin-3 knockout (Cav-3KO) mice. These results suggest that SPreC-mediated attenuation of superoxide generation is highly dependent on Cav-3 and only partially dependent on the AMPK signaling axis. In addition, this study showed that SPreC compared with control treatment significantly decreased caspase-3 activity, indicated inhibition of cardiomyocyte apoptosis involved in the cardioprotection of SPreC. Previous study had identified that microRNAs (miRNAs) were important targets for regulating myocardial reperfusion damage and were the valuable biomarkers of myocardial injury (5). The study of Tan et al. (49) on a rat model of myocardial IRI in vivo demonstrated that SPostC compared with control treatment significantly decreased myocardial malondialdehyde level, but increased myocardial glutathione and superoxide dismutase activities, indicating that SPostC can inhibit oxidative stress in ischemia/reperfused myocardium by activating miR-203. Besides, this study also showed that SPostC decreased serum proinflammation factors and cardiomyocyte apoptosis. Similarly, Qi et al. (11) found that SPostC significantly inhibited oxidative stress and apoptosis to provide a protect against myocardial IRI by upregulating miR-145 expression and downregulating granzyme K expression.
It must be emphasized that several interesting phenomena about cardioprotection of SPreC and SPostC deserve special attention. Qiao et al. (47) demonstrated that cardioprotection of SPostC is age dependent, i.e., a significant cardioprotection is achieved in young animals but not in old ones. Available evidence indicates that this age-dependent cardioprotection of SPostC is at least associated with the inability to activate Akt and Erk1/2 in old animals (41). Furthermore, Obal et al. (18) found that a combination of SPreC with SPostC can provide an additive protection against myocardial IRI, which is partly mediated by opening mitochondrial adenosine triphosphate-dependent potassium (mKATP) channels. In addition, various risk factors of cardiovascular diseases, such as obesity, hyperlipidemia, and diabetes, can significantly attenuate or even abolish the cardioprotective effects of sevoflurane conditionings (26, 50, 59). As a main aim of this analysis was to determine the cardioprotective effects of sevoflurane conditionings, the preclinical studies performed on animals with the risk factors of cardiovascular diseases were specifically excluded from this analysis. Nevertheless, it is advisable to consider the risk factors of cardiovascular diseases when determining the optimum protocols of sevoflurane conditionings.
Although many in-vivo experiments have shown that sevoflurane conditionings can provide an infarct size sparing, clinical trials in humans have yielded variable results regarding cardioprotection of sevoflurane conditionings. Several studies demonstrate that sevoflurane has the potential benefits of cardioprotection in patients undergoing coronary artery bypass surgery when it is administrated to maintain anesthesia at 1 minimum alveolar concentration (MAC), shown by improved clinical results and myocardial injury biomarkers after surgery (60, 61). A recent meta-analysis reports that halogenated agents including desflurane, isoflurane, and sevoflurane can reduce the occurrence of myocardial infarction, mortality rate, and mechanical ventilation need after cardiac surgery, especially for the patients undergoing CABG surgery (62). However, a meta-analysis of 79 randomized controlled trials (RCTs) including 6,219 patients with noncardiac surgery reports that cardioprotective benefits of sevoflurane are inconclusive (63). It should be noted that this meta-analysis uses perioperative myocardial infarction or deaths as the main endpoint and no case occurs in any of the included studies. Similarly, another systematic review and meta-analysis indicate that the use of volatile anesthetics such as sevoflurane, desflurane, or isoflurane is associated with decreased mortality and perioperative complications in patients undergoing cardiac surgery, but does not improve these outcomes in patients with noncardiac surgery (64). It is still unclear why cardioprotection of sevoflurane is significantly different between patients with cardiac and noncardiac surgeries. Thus, further studies are needed to determine whether cardioprotection of sevoflurane is really surgery type-dependent and which factors can impair the transformation of cardioprotective benefits provided by sevoflurane into improved clinical outcomes of patients.
Tracing the potential sources responsible for heterogeneity in a meta-analysis can guide preclinical and clinical study designs. As our analysis showed that there were high levels of heterogeneity between the included studies, both the meta-regression and stratified analysis were used to explore the potential sources of heterogeneity and determine whether some of the predefined experimental variables in this analysis could influence the observed effect size with cardioprotective efficacy of sevoflurane conditionings. In fact, this method for analyzing heterogeneity has been successfully applied to several promising preclinical interventions (65, 66). Our stratified analysis showed that animal species (dog, rabbit, rat, and mice) did not produce significant effects on either effect size or heterogeneity of both the SPreC and SPostC groups. In addition, meta-regression suggested that country difference might be a source of significant heterogeneity for SPreC. Further stratified analysis by countries showed that there was still significant heterogeneity between the groups. Compared to other countries, moreover, Netherlands showed a large WMD, with a wide 95% CI. This may partly explain the source of heterogeneity. Anyway, it should be aware that preclinical characterization of sevoflurane administration protocol in-vivo studies has not yet been identified and current studies have showed uneven quality. All of these are essential to determine the important parameters that will facilitate optimization of administration protocol in further clinical trials.
Certainly, both the poor methodological quality and publication bias can result in over- or under-estimation of effect size (67, 68). To determine the internal validity of included studies in this analysis, both the quality of study and publication bias were evaluated by the reporting quality assessment tools, i.e., the ARRIVE guidelines 2.0 and a 12-item quality score. Our results showed that the overall scores of the reporting quality assessment tools for the included studies were high, indicating the validity of this analysis. In fact, main contents of experimental process, such as confirmation of ischemia and monitoring of ECG, were well reported in the included studies. However, a number of aspects required by the ARRIVE guidelines 2.0 were poorly reported in the most included studies, particularly in sample size calculations (11%) and blinding (16%). These may inevitably raise concerns of statistical validity and result in exclusion of literature selection. Furthermore, appropriate monitoring of experimental animals, such as PaO2 or SaO2 (27%), depth of anesthesia (22%), and concentration of sevoflurane (38%), was also reported poorly in the included studies. According to a position article on improving the preclinical assessment of novel cardioprotective therapies (69), these factors are actually essential to ensure a high-quality study. Thus, failure to report high-quality studies may partly account for the observed heterogeneity of this analysis. Nevertheless, the effect sizes by sevoflurane conditionings were consistent and robust despite high heterogeneity was observed.
Finally, publication bias within the included studies was visually assessed by a funnel plot and further detected by the Egger's and Begg's tests. Notably, publication bias was displayed in the funnel plot and identified by Egger's test (P < 0.01) for the SPostC group. Visual analysis of the funnel plot suggested that neutral or negative studies might be underrepresented in the SPostC group. That is true, studies with neutral or negative data are often not given priority for publication. Fortunately, it is reassuring that the bias of our analysis does not result in a statistically significant impact on the overall effect.
This meta-analysis has included all the studies, which met our rigorous inclusion criteria. Besides, the studies that are not published and do not meet important quality criteria are not be included in our analysis. As the validity of a meta-analysis is highly depended on the quality of all the included studies, there are several limitations in this analysis that deserve special attention. First, there is only a small amount of data from large animals in our analysis, though large animals share more anatomical and physiological characteristics of heart with human. This may limit the interpretation and extension of our results. It is warranted that large animal experiments are needed to further confirm the favorable effects of sevoflurane conditionings on myocardial IRI in small animal models. Second, as mentioned in the discussion, this analysis only includes the studies that are conducted on normal animals without any risk factors of cardiovascular diseases, such as aging, diabetes, obesity, hyperlipidemia, hypertension, and others. Evidently, our results cannot be generalized to the animals with risk factors of cardiovascular diseases. Third, besides country differences may be a source of heterogeneity for SPreC studies, meta-regression fails to reveal any influence of other prespecified covariates on pooled estimates of infarct size. However, robustness of the data is evidenced by both the sensitivity analysis and stratified analysis, which confirm the benefits and reliability of sevoflurane conditionings in ameliorating myocardial IRI. Thus, the real reasons for high degree of heterogeneity among the included studies are unclear. To address above issues and confirm therapeutic effect of sevoflurane conditionings on myocardial IRI, we believe that more animal studies and RCTs are still required.
This comprehensive systematic review and meta-analysis demonstrate that sevoflurane conditionings can provide a robust and highly reproducible infarct size-sparing effect in animal models of myocardial IRI. Furthermore, this beneficial effect can be obtained when sevoflurane conditioning is carried out before the onset of ischemia, during ischemia, or during reperfusion, despite a high heterogeneity among the included studies is observed. Nonetheless, preclinical studies with a high-quality design, especially for those conducted on large animal models and the animals with risk factors of cardiovascular diseases, are still required to future determine the protection of sevoflurane conditionings against myocardial IRI and explore relevant mechanisms.
The original contributions presented in the study are included in the article/Supplementary Material, further inquiries can be directed to the corresponding author.
BH and F-SX contributed to the study design, planning, data analysis, and wrote the manuscript. TT, P-PH, W-CL, Y-GC, and T-YJ contributed to data analysis and revision of the manuscript. All authors had seen and approved the final version of the manuscript.
This study was performed with the financial support of the National Natural Science Foundation of China (No. 81470019 to F-SX).
The authors declare that the research was conducted in the absence of any commercial or financial relationships that could be construed as a potential conflict of interest.
All claims expressed in this article are solely those of the authors and do not necessarily represent those of their affiliated organizations, or those of the publisher, the editors and the reviewers. Any product that may be evaluated in this article, or claim that may be made by its manufacturer, is not guaranteed or endorsed by the publisher.
The Supplementary Material for this article can be found online at: https://www.frontiersin.org/articles/10.3389/fcvm.2022.841654/full#supplementary-material
Supplementary Table 1. Sensitivity analysis.
Supplementary Table 2. The proposed mechanism of cardioprotection by sevoflurane preconditioning (SPreC) and sevoflurane postconditioning (SPostC) in the included studies.
Supplementary Material 1. (A) Web of Science search history; (B) Cochrane search history; (C) Embase search history; and (D) PubMed search history.
Supplementary Material 2. Meta-regression.
Supplementary Material 3. Stratified analysis.
1. Virani S, Alonso A, Benjamin E, Bittencourt M, Callaway C, Carson A, et al. Heart Disease and Stroke Statistics-2020 Update: A Report From the American Heart Association. Circulation. (2020) 141:e139–596. doi: 10.1161/CIR.0000000000000757
2. Piao H, Takahashi K, Yamaguchi Y, Wang C, Liu K, Naruse K. Transient receptor potential melastatin-4 is involved in hypoxia-reoxygenation injury in the cardiomyocytes. PLoS ONE. (2015) 10:e0121703. doi: 10.1371/journal.pone.0121703
3. Yellon D, Hausenloy D. Myocardial reperfusion injury. N Engl J Med. (2007) 357:1121–35. doi: 10.1056/NEJMra071667
4. Hausenloy D, Yellon D. Myocardial ischemia-reperfusion injury: a neglected therapeutic target. J Clin Invest. (2013) 123:92–100. doi: 10.1172/JCI62874
5. Eltzschig HK, Eckle T. Ischemia and reperfusion–from mechanism to translation. Nat Med. (2011) 17:1391–401. doi: 10.1038/nm.2507
6. Davidson S, Ferdinandy P, Andreadou I, Bøtker H, Heusch G, Ibáñez B, et al. Multitarget strategies to reduce myocardial ischemia/reperfusion injury: JACC review topic of the week. J Am Coll Cardiol. (2019) 73:89–99. doi: 10.1016/j.jacc.2018.09.086
7. Lemoine S, Zhu L, Gérard JL, Hanouz JL. Sevoflurane-induced cardioprotection in coronary artery bypass graft surgery: randomised trial with clinical and ex-vivo endpoints. Anaesth Crit Care Pain Med. (2018) 37:217–23. doi: 10.1016/j.accpm.2017.05.009
8. Landoni G, Biondi-Zoccai GGL, Zangrillo A, Bignami E, D'Avolio S, Marchetti C, et al. Desflurane and sevoflurane in cardiac surgery: a meta-analysis of randomized clinical trials. J Cardiothorac Vasc Anesth. (2007) 21:502–11. doi: 10.1053/j.jvca.2007.02.013
9. Likhvantsev VV, Landoni G, Levikov DI, Grebenchikov OA, Skripkin YV, Cherpakov RA. Sevoflurane Versus Total Intravenous Anesthesia for Isolated Coronary Artery Bypass Surgery With Cardiopulmonary Bypass: A Randomized Trial. J Cardiothorac Vasc Anesth. (2016) 30:1221–7. doi: 10.1053/j.jvca.2016.02.030
10. Zhao J, Wang F, Zhang Y, Jiao L, Lau WB, Wang L, et al. Sevoflurane preconditioning attenuates myocardial ischemia/reperfusion injury via caveolin-3-dependent cyclooxygenase-2 inhibition. Circulation. (2013) 128:S121–S9. doi: 10.1161/CIRCULATIONAHA.112.000045
11. Qi Z, Li S, Su Y, Zhang J, Kang Y, Huang Y, et al. Role of microRNA-145 in protection against myocardial ischemia/reperfusion injury in mice by regulating expression of GZMK with the treatment of sevoflurane. J Cell Physiol. (2019). doi: 10.1002/jcp.28323
12. Lurati Buse GAL, Schumacher P, Seeberger E, Studer W, Schuman RM, Fassl J, et al. Randomized comparison of sevoflurane versus propofol to reduce perioperative myocardial ischemia in patients undergoing noncardiac surgery. Circulation. (2012) 126:2696–704. doi: 10.1161/CIRCULATIONAHA.112.126144
13. Bell R, Bøtker H, Carr R, Davidson S, Downey J, Dutka D, et al. 9th Hatter Biannual Meeting: position document on ischaemia/reperfusion injury, conditioning and the ten commandments of cardioprotection. Basic Res Cardiol. (2016) 111:41. doi: 10.1007/s00395-016-0558-1
14. Hausenloy D, Garcia-Dorado D, Bøtker H, Davidson S, Downey J, Engel F, et al. Novel targets and future strategies for acute cardioprotection: Position Paper of the European Society of Cardiology Working Group on Cellular Biology of the Heart. Cardiovasc Res. (2017) 113:564–85. doi: 10.1093/cvr/cvx049
15. Bromage D, Pickard J, Rossello X, Ziff O, Burke N, Yellon D, et al. Remote ischaemic conditioning reduces infarct size in animal in vivo models of ischaemia-reperfusion injury: a systematic review and meta-analysis. Cardiovasc Res. (2017) 113:288–97. doi: 10.1093/cvr/cvw219
16. Ritskes-Hoitinga M, Leenaars M, Avey M, Rovers M, Scholten R. Systematic reviews of preclinical animal studies can make significant contributions to health care and more transparent translational medicine. Cochrane Database Syst Rev. (2014). ED000078. doi: 10.1002/14651858.ED000078
17. Toller WG, Kersten JR, Pagel PS, Hettrick DA, Warltier DC. Sevoflurane reduces myocardial infarct size and decreases the time threshold for ischemic preconditioning in dogs. Anesthesiology. (1999) 91:1437–46. doi: 10.1097/00000542-199911000-00037
18. Obal D, Dettwiler S, Favoccia C, Scharbatke H, Preckel B, Schlack W. The influence of mitochondrial KATP-channels in the cardioprotection of preconditioning and postconditioning by sevoflurane in the rat in vivo. Anesth Analg. (2005) 101:1252–60. doi: 10.1213/01.ANE.0000181336.96511.32
19. Lange M, Smul TM, Blomeyer CA, Redel A, Klotz K-N, Roewer N, et al. Role of the beta1-adrenergic pathway in anesthetic and ischemic preconditioning against myocardial infarction in the rabbit heart in vivo. Anesthesiology. (2006) 105:503–10. doi: 10.1097/00000542-200609000-00014
20. Redel A, Stumpner J, Tischer-Zeitz T, Lange M, Smul TM, Lotz C, et al. Comparison of isoflurane-, sevoflurane-, and desflurane-induced pre- and postconditioning against myocardial infarction in mice in vivo. Exp Biol Med (Maywood). (2009) 234:1186–91. doi: 10.3181/0902-RM-58
21. Wang C, Xie H, Liu X, Qin Q, Wu X, Liu H, et al. Role of nuclear factor-κB in volatile anaesthetic preconditioning with sevoflurane during myocardial ischaemia/reperfusion. Eur J Anaesthesiol. (2010) 27:747–56. doi: 10.1097/EJA.0b013e32833bb3ba
22. Frädorf J, Huhn R, Weber NC, Ebel D, Wingert N, Preckel B, et al. Sevoflurane-induced preconditioning: impact of protocol and aprotinin administration on infarct size and endothelial nitric-oxide synthase phosphorylation in the rat heart in vivo. Anesthesiology. (2010) 113:1289–98. doi: 10.1097/ALN.0b013e3181f97fec
23. Tosaka S, Tosaka R, Matsumoto S, Maekawa T, Cho S, Sumikawa K. Roles of cyclooxygenase 2 in sevoflurane- and olprinone-induced early phase of preconditioning and postconditioning against myocardial infarction in rat hearts. J Cardiovasc Pharmacol Ther. (2011) 16:72–8. doi: 10.1177/1074248410380208
24. Xiao YY, Chang YT, Ran K, Liu JP. Delayed preconditioning by sevoflurane elicits changes in the mitochondrial proteome in ischemia-reperfused rat hearts. Anesth Analg. (2011) 113:224–32. doi: 10.1213/ANE.0b013e3182239b71
25. Zhang FJ, Ma LL, Wang WN, Qian LB, Yang MJ Yu J, et al. Hypercholesterolemia abrogates sevoflurane-induced delayed preconditioning against myocardial infarct in rats by alteration of nitric oxide synthase signaling. Shock. (2012) 37:485–91. doi: 10.1097/SHK.0b013e318249b7b6
26. Ma LL, Zhang FJ, Qian LB, Kong FJ, Sun JF, Zhou C, et al. Hypercholesterolemia blocked sevoflurane-induced cardioprotection against ischemia-reperfusion injury by alteration of the MG53/RISK/GSK3β signaling. Int J Cardiol. (2013) 168:3671–8. doi: 10.1016/j.ijcard.2013.06.037
27. Qiao S, Xie H, Wang C, Wu X, Liu H, Liu C. Delayed anesthetic preconditioning protects against myocardial infarction via activation of nuclear factor-κB and upregulation of autophagy. J Anesth. (2013) 27:251–60. doi: 10.1007/s00540-012-1494-3
28. Xie H, Liu X, Wang C, Zhu J, Yang C, Liu C, et al. The changes of technetium-99m-labeled annexin-V in delayed anesthetic preconditioning during myocardial ischemia/reperfusion. Mol Biol Rep. (2014) 41:131–7. doi: 10.1007/s11033-013-2845-3
29. Behmenburg F, Boekholt Y, van Caster P, Dorsch M, Heinen A, Hollmann MW, et al. Extended second window of protection of sevoflurane-induced preconditioning. J Cardiovasc Pharmacol. (2017) 70:284–9. doi: 10.1097/FJC.0000000000000517
30. Liu AJ, Pang CX, Liu GQ, Wang SD, Chu CQ Li LZ, et al. Ameliorative effect of sevoflurane on endoplasmic reticulum stress mediates cardioprotection against ischemia-reperfusion injury. Can J Physiol Pharmacol. (2019) 97:345–51. doi: 10.1139/cjpp-2018-0016
31. Xie D, Zhao J, Guo R, Jiao L, Zhang Y, Lau WB, et al. Sevoflurane pre-conditioning ameliorates diabetic myocardial ischemia/reperfusion injury via differential regulation of p38 and ERK. Sci Rep. (2020) 10:23. doi: 10.1038/s41598-019-56897-8
32. Hong L, Sun Y, An JZ, Wang C, Qiao SG. Sevoflurane preconditioning confers delayed cardioprotection by upregulating AMP-activated protein kinase levels to restore autophagic flux in ischemia-reperfusion rat hearts. Med Sci Monit. (2020) 26:e922176. doi: 10.12659/MSM.922176
33. Preckel B, Schlack W, Comfère T, Obal D, Barthel H, Thämer V. Effects of enflurane, isoflurane, sevoflurane and desflurane on reperfusion injury after regional myocardial ischaemia in the rabbit heart in vivo. Br J Anaesth. (1998) 81:905–12. doi: 10.1093/bja/81.6.905
34. Obal D, Preckel B, Scharbatke H, Müllenheim J, Höterkes F, Thämer V, et al. One MAC of sevoflurane provides protection against reperfusion injury in the rat heart in vivo. Br J Anaesth. (2001) 87:905–11. doi: 10.1093/bja/87.6.905
35. Obal D, Scharbatke H, Barthel H, Preckel B, Müllenheim J, Schlack W. Cardioprotection against reperfusion injury is maximal with only two minutes of sevoflurane administration in rats. Can J Anesth. (2003) 50:940–5. doi: 10.1007/BF03018744
36. Huhn R, Heinen A, Weber NC, Hollmann MW, Schlack W, Preckel B. Hyperglycaemia blocks sevoflurane-induced postconditioning in the rat heart in vivo: cardioprotection can be restored by blocking the mitochondrial permeability transition pore. Br J Anaesth. (2008) 100:465–71. doi: 10.1093/bja/aen022
37. Drenger B, Ostrovsky IA, Barak M, Nechemia-Arbely Y, Ziv E, Axelrod JH. Diabetes blockade of sevoflurane postconditioning is not restored by insulin in the rat heart: phosphorylated signal transducer and activator of transcription 3- and phosphatidylinositol 3-kinase-mediated inhibition. Anesthesiology. (2011) 114:1364–72. doi: 10.1097/ALN.0b013e31820efafd
38. Tai W, Shi E, Yan L, Jiang X, Ma H, Ai C. Diabetes abolishes the cardioprotection induced by sevoflurane postconditioning in the rat heart in vivo: roles of glycogen synthase kinase-3β and its upstream pathways. J Surg Res. (2012) 178:96–104. doi: 10.1016/j.jss.2012.02.021
39. Chen D, Cheng B, Zhou HY Li LH. The effect of sevoflurane postconditioning on cardioprotection against ischemia-reperfusion injury in rabbits. Mol Biol Rep. (2012) 39:6049–57. doi: 10.1007/s11033-011-1419-5
40. Xu Y, Ma LL, Zhou C, Zhang FJ, Kong FJ, Wang WN, et al. Hypercholesterolemic myocardium is vulnerable to ischemia-reperfusion injury and refractory to sevoflurane-induced protection. PLoS ONE. (2013) 8:e76652. doi: 10.1371/journal.pone.0076652
41. Li H, Zhou C, Chen D, Fang N, Yao Y, Li L. Failure to protect against myocardial ischemia-reperfusion injury with sevoflurane postconditioning in old rats in vivo. Acta Anaesthesiol Scand. (2013) 57:1024–31. doi: 10.1111/aas.12156
42. Zhang YL, Yao YT, Fang NX, Zhou CH, Gong JS Li LH. Restoration of autophagic flux in myocardial tissues is required for cardioprotection of sevoflurane postconditioning in rats. Acta Pharmacol Sin. (2014) 35:758–69. doi: 10.1038/aps.2014.20
43. Stumpner J, Tischer-Zeitz T, Frank A, Lotz C, Redel A, Lange M, et al. The role of cyclooxygenase-1 and−2 in sevoflurane-induced postconditioning against myocardial infarction. Semin Cardiothorac Vasc Anesth. (2014) 18:272–80. doi: 10.1177/1089253214523683
44. Gao S, Yang Z, Shi R, Xu D, Li H, Xia Z, et al. Diabetes blocks the cardioprotective effects of sevoflurane postconditioning by impairing Nrf2/Brg1/HO-1 signaling. Eur J Pharmacol. (2016) 779:111–21. doi: 10.1016/j.ejphar.2016.03.018
45. Lin J, Wang T, Li Y, Wang M, Li H, Irwin MG, et al. N-Acetylcysteine restores sevoflurane postconditioning cardioprotection against myocardial ischemia-reperfusion injury in diabetic rats. J Diabetes Res. (2016) 2016:9213034. doi: 10.1155/2016/9213034
46. Li H, Chen D, Fang N, Yao Y, Li L. Age-associated differences in response to sevoflurane postconditioning in rats. Scand Cardiovasc J. (2016) 50:128–36. doi: 10.3109/14017431.2015.1122830
47. Qiao SG, Sun Y, Sun B, Wang A, Qiu J, Hong L, et al. Sevoflurane postconditioning protects against myocardial ischemia/reperfusion injury by restoring autophagic flux via an NO-dependent mechanism. Acta Pharmacol Sin. (2019) 40:35–45. doi: 10.1038/s41401-018-0066-y
48. Huang G, Hao F, Hu X. Downregulation of microRNA-155 stimulates sevoflurane-mediated cardioprotection against myocardial ischemia/reperfusion injury by binding to SIRT1 in mice. J Cell Biochem. (2019) 120:15494–505. doi: 10.1002/jcb.28816
49. Tan J, Wu Z, Liu J, Zhang W, Yuan W, Peng H. MicroRNA-203-mediated inhibition of doublecortin underpins cardioprotection conferred by sevoflurane in rats after myocardial ischaemia-reperfusion injury. J Cell Mol Med. (2020) 24:9825–38. doi: 10.1111/jcmm.15566
50. Yu J, He J, Yang W, Wang X, Shi G, Duan Y, et al. Diabetes impairs the protective effects of sevoflurane postconditioning in the myocardium subjected to ischemia/ reperfusion injury in rats: important role of Drp1. BMC Cardiovasc Disord. (2021) 21:96. doi: 10.1186/s12872-021-01906-w
51. Gao S, Wang R, Dong S, Wu J, Perek B, Xia Z, et al. Inactivation of TOPK caused by hyperglycemia blocks diabetic heart sensitivity to sevoflurane postconditioning by impairing the PTEN/PI3K/Akt signaling. Oxid Med Cell Longev. (2021) 2021:6657529. doi: 10.1155/2021/6657529
52. Shi X, Chen Q, Wang F. Mesenchymal stem cells for the treatment of ulcerative colitis: a systematic review and meta-analysis of experimental and clinical studies. Stem Cell Res Ther. (2019) 10:266. doi: 10.1186/s13287-019-1336-4
53. Percie du Sert N, Hurst V, Ahluwalia A, Alam S, Avey M, Baker M, et al. The ARRIVE guidelines 2.0: updated guidelines for reporting animal research. BMJ Open Sci. (2020) 4:e100115. doi: 10.1136/bmjos-2020-100115
54. Vesterinen HM, Sena ES, Egan KJ, Hirst TC, Churolov L, Currie GL, et al. Meta-analysis of data from animal studies: a practical guide. J Neurosci Methods. (2014) 221:92–102. doi: 10.1016/j.jneumeth.2013.09.010
55. Prabhu S, Frangogiannis N. The biological basis for cardiac repair after myocardial infarction: from inflammation to fibrosis. Circ Res. (2016) 119:91–112. doi: 10.1161/CIRCRESAHA.116.303577
56. Münzel T, Camici GG, Maack C, Bonetti NR, Fuster V, Kovacic JC. Impact of oxidative stress on the heart and vasculature: part 2 of a 3-part series. J Am Coll Cardiol. (2017) 70:212–29. doi: 10.1016/j.jacc.2017.05.035
57. Mao ZJ, Lin H, Hou JW, Zhou Q, Wang Q, Chen YH, et al. Meta-analysis of resveratrol protects against myocardial ischemia/reperfusion injury: evidence from small animal studies and insight into molecular mechanisms. Oxi Med Cell Longev. (2019) 2019:5793867. doi: 10.1155/2019/5793867
58. Guerrero-Orriach JL, Escalona Belmonte JJ, Ramirez Fernandez A, Ramirez Aliaga M, Rubio Navarro M, Cruz Mañas J. Cardioprotection with halogenated gases: how does it occur? Drug Des Devel Ther. (2017) 11:837–49. doi: 10.2147/DDDT.S127916
59. Song T, Lv LY, Xu J, Tian ZY, Cui WY, Wang QS, et al. Diet-induced obesity suppresses sevoflurane preconditioning against myocardial ischemia-reperfusion injury: role of AMP-activated protein kinase pathway. Exp Biol Med (Maywood). (2011) 236:1427–36. doi: 10.1258/ebm.2011.011165
60. Xu R, Lu R, Jiang H, Li Q, Sun Y, Xu H, et al. Meta-analysis of protective effect of sevoflurane on myocardium during cardiac surgery. Eur Rev Med Pharmacol Sci. (2014) 18:1058–66.
61. Conzen P, Fischer S, Detter C, Peter K. Sevoflurane provides greater protection of the myocardium than propofol in patients undergoing off-pump coronary artery bypass surgery. Anesthesiology. (2003) 99:826–33. doi: 10.1097/00000542-200310000-00013
62. Landoni G, Lopez-Delgado JC, Sartini C, Tamà S, Zangrillo A. Halogenated Agents and Cardiovascular Surgery: Has Mortality Really Decreased? Curr Vasc Pharmacol. (2018) 16:336–43. doi: 10.2174/1570161115666171010121549
63. Landoni G, Fochi O, Bignami E, Calabrò M, D'Arpa M, Moizo E, et al. Cardiac protection by volatile anesthetics in non-cardiac surgery? A meta-analysis of randomized controlled studies on clinically relevant endpoints. HSR Pro Intensive Care Cardiovasc Anesth. (2009) 1:34–43.
64. Uhlig C, Bluth T, Schwarz K, Deckert S, Heinrich L, De Hert S, et al. Effects of volatile anesthetics on mortality and postoperative pulmonary and other complications in patients undergoing surgery: a systematic review and meta-analysis. Anesthesiology. (2016) 124:1230–45. doi: 10.1097/ALN.0000000000001120
65. Lim W, Messow C, Berry C. Cyclosporin variably and inconsistently reduces infarct size in experimental models of reperfused myocardial infarction: a systematic review and meta-analysis. Br J Pharmacol. (2012) 165:2034–43. doi: 10.1111/j.1476-5381.2011.01691.x
66. Mewton N, Bergerot C, Ovize M. Cyclosporine before PCI in acute myocardial infarction. N Engl J Med. (2016) 374:90. doi: 10.1056/NEJMc1514192
67. van der Worp HB, Howells DW, Sena ES, Porritt MJ, Rewell S, O'Collins V, et al. Can animal models of disease reliably inform human studies? PLoS Med. (2010) 7:e1000245. doi: 10.1371/journal.pmed.1000245
68. Sena ES, van der Worp HB, Bath PMW, Howells DW, Macleod MR. Publication bias in reports of animal stroke studies leads to major overstatement of efficacy. PLoS Biol. (2010) 8:e1000344. doi: 10.1371/journal.pbio.1000344
Keywords: sevoflurane, preconditioning, postconditioning, myocardial ischemia/reperfusion injury, pre-clinical trials, infarct size, meta-analysis
Citation: Hu B, Tian T, Hao P-P, Liu W-C, Chen Y-G, Jiang T-Y and Xue F-S (2022) The Protective Effect of Sevoflurane Conditionings Against Myocardial Ischemia/Reperfusion Injury: A Systematic Review and Meta-Analysis of Preclinical Trials in in-vivo Models. Front. Cardiovasc. Med. 9:841654. doi: 10.3389/fcvm.2022.841654
Received: 22 December 2021; Accepted: 16 March 2022;
Published: 28 April 2022.
Edited by:
Xiaofeng Yang, Temple University, United StatesReviewed by:
Giuseppe Alloatti, University of Turin, ItalyCopyright © 2022 Hu, Tian, Hao, Liu, Chen, Jiang and Xue. This is an open-access article distributed under the terms of the Creative Commons Attribution License (CC BY). The use, distribution or reproduction in other forums is permitted, provided the original author(s) and the copyright owner(s) are credited and that the original publication in this journal is cited, in accordance with accepted academic practice. No use, distribution or reproduction is permitted which does not comply with these terms.
*Correspondence: Fu-Shan Xue, eHVlZnVzaGFuQGFsaXl1bi5jb20=; ZnVzaGFueHVlQG91dGxvb2suY29t
Disclaimer: All claims expressed in this article are solely those of the authors and do not necessarily represent those of their affiliated organizations, or those of the publisher, the editors and the reviewers. Any product that may be evaluated in this article or claim that may be made by its manufacturer is not guaranteed or endorsed by the publisher.
Research integrity at Frontiers
Learn more about the work of our research integrity team to safeguard the quality of each article we publish.