- 1Division of Diagnostics and Specialist Medicine, Department of Health, Medicine and Caring Sciences, Linköping University, Linköping, Sweden
- 2Department of Gastroenterology in Linköping and Department of Biomedical and Clinical Sciences, Linköping University, Linköping, Sweden
- 3Center for Medical Image Science and Visualization, Linköping University, Linköping, Sweden
- 4AMRA Medical AB, Linköping University, Linköping, Sweden
- 5Department of Radiation Physics and Department of Health, Medicine and Caring Sciences, Linköping University, Linköping, Sweden
- 6Department of Cardiology in Linköping and Department of Health, Medicine and Caring Sciences, Linköping University, Linköping, Sweden
- 7Division of Prevention, Rehabilitation and Community Medicine, Department of Health, Medicine and Caring Sciences, Linköping University, Linköping, Sweden
- 8Department of Clinical Physiology in Linköping and Department of Health, Medicine and Caring Sciences, Linköping University, Linköping, Sweden
Background: Different regional depots of fat have distinct metabolic properties and may relate differently to adverse cardiac remodeling. We sought to quantify regional depots of body fat and to investigate their relationship to cardiac structure and function in Type 2 Diabetes (T2D) and controls.
Methods: From the SCAPIS cohort in Linköping, Sweden, we recruited 92 subjects (35% female, mean age 59.5 ± 4.6 years): 46 with T2D and 46 matched controls. In addition to the core SCAPIS data collection, participants underwent a comprehensive magnetic resonance imaging examination at 1.5 T for assessment of left ventricular (LV) structure and function (end-diastolic volume, mass, concentricity, ejection fraction), as well as regional body composition (liver proton density fat fraction, visceral adipose tissue, abdominal subcutaneous adipose tissue, thigh muscle fat infiltration, fat tissue-free thigh muscle volume and epicardial adipose tissue).
Results: Compared to the control group, the T2D group had increased: visceral adipose tissue volume index (P < 0.001), liver fat percentage (P < 0.001), thigh muscle fat infiltration percentage (P = 0.02), LV concentricity (P < 0.001) and LV E/e'-ratio (P < 0.001). In a multiple linear regression analysis, a negative association between liver fat percentage and LV mass (St Beta −0.23, P < 0.05) as well as LV end-diastolic volume (St Beta −0.27, P < 0.05) was found. Epicardial adipose tissue volume and abdominal subcutaneous adipose tissue volume index were the only parameters of fat associated with LV diastolic dysfunction (E/e'-ratio) (St Beta 0.24, P < 0.05; St Beta 0.34, P < 0.01, respectively). In a multivariate logistic regression analysis, only visceral adipose tissue volume index was significantly associated with T2D, with an odds ratio for T2D of 3.01 (95% CI 1.28–7.05, P < 0.05) per L/m2 increase in visceral adipose tissue volume.
Conclusions: Ectopic fat is predominantly associated with cardiac remodeling, independently of type 2 diabetes. Intriguingly, liver fat appears to be related to LV structure independently of VAT, while epicardial fat is linked to impaired LV diastolic function. Visceral fat is associated with T2D independently of liver fat and abdominal subcutaneous adipose tissue.
Introduction
Visceral adipose tissue (VAT) is associated with metabolic cardiovascular disease risk factors (1, 2), above and beyond the abdominal subcutaneous adipose tissue (ASAT). Data from cardiovascular imaging studies also show that the volume of VAT is associated with subclinical cardiac remodeling, in terms of increased left ventricular (LV) concentricity and impaired diastolic relaxation (3–5). Fat can also accumulate in and around normally lean tissues, such as the heart, skeletal muscle and liver, a process termed 'ectopic fat infiltration' (6). In addition to VAT, both hepatic and cardiac ectopic fat depots have previously been linked to increased cardiovascular risk (7, 8), as well as adverse LV structural remodeling and dysfunction (9–11).
How different fat depots affect cardiovascular risk factors is still unclear. Ectopic and visceral fat depots are thought to act through both local and systemic effects (6), and probably affect the cardiovascular system differently. Based on data from the Framingham Heart Study, Lee et al. demonstrated that VAT and liver fat were more strongly associated with cardiometabolic risk factors, compared to other sites for ectopic fat accumulation (12). In contrast, a recent analysis from the Multi-Ethnic Study of Atherosclerosis found that the total amount of pericardial fat, but not hepatic fat, was independently associated with risk of atherosclerotic events over a median of 12.2 years follow-up (13).
Since VAT is related to the overall burden of ectopic fat (14), it might not constitute the primary mediator for adverse cardiac remodeling. Given the close interrelationship between VAT, ectopic fat and gluco-metabolic disease, the causal pathways need to be explored in more detail. Thus, there is a need for studies performing more comprehensive analyses of regional fat depots. Magnetic resonance imaging (MRI) allows for accurate assessment of whole-body adipose tissue, including accurate assessment of liver fat, which can be challenging with other imaging modalities (15). Further studies also need to consider T2D in order to distinguish the characteristics of separate fat depots, as diabetes per se is likely to affect cardiac remodeling (16).
In the present study, advanced MRI techniques were utilized to accurately assess whole-body adipose tissue, including liver fat, epicardial fat, visceral fat, abdominal subcutaneous fat, and skeletal muscle fat in participants with T2D and matched controls. We sought to investigate the relationship between these regional depots of fat to measures of LV structure and function as well as to T2D. We hypothesized that regional fat depots differ in their associations to cardiac remodeling and T2D.
Materials and methods
Study design and population
We conducted an exploratory cross-sectional study that was a sub-study within SCAPIS (17). We included 92 participants in the ages 50–64 years from the SCAPIS cohort in Linköping. We first included 46 participants with T2D. They were identified as having T2D according to health forms, fP-glucose ≥7.0 mmol/L on two occasions or HbA1c ≥ 48 mmol/mol. Additionally, 46 control subjects were recruited, reported as not having T2D according to the health forms, fP-glucose <6.0 mmol/L and HbA1c <42 mmol/mol. The control subjects were matched to the diabetes group on an individual basis with regard to age, sex, and smoking. General exclusion criteria were contraindications to MRI and significantly irregular ventricular rhythm.
Ethics
All participants provided written informed consent to participate. The study was performed in accordance with the Declaration of Helsinki and approved by the Linköping Ethical Review Board (Dnr 2016/229-31).
SCAPIS data
From the core SCAPIS data in Linköping, we had access to the following data:
• Demographic variables (sex, age, alcohol consumption, and smoking)
• Self-reported cardiovascular morbidity and drug use
• Anthropometric measurements (body weight, height, and BMI)
• Routine clinical biochemistry (glucose status, lipid status, highly sensitive CRP)
• Echocardiography (E/A-ratio, E/e'-ratio).
Echocardiography was performed using a GE Vivid E95 rev 201 or E9 (GE Vingmed Ultrasound, Horten, Norway) according to current guidelines from EACVI/ASE (18) (see Supplementary material for details on data acquisition and analysis).
Acquisition of MRI data
After inclusion, all 92 participants underwent a comprehensive MRI examination at Linköping University Hospital between November 2017 and July 2018. Data were acquired in a single examination using a Philips Achieva dStream 1.5 T MRI-scanner (Philips Healthcare, Best, The Netherlands) and included a neck-to-knee four-echo mDixon sequence, liver proton magnetic resonance spectroscopy (1H-MRS) sequence, cardiac 3D cine balanced steady state-free precession (bSSFP) sequence and cardiac 3D Dixon sequence. Figure 1 shows example images from the whole-body and cardiac Dixon sequences as well as the liver H-1 spectroscopy sequence. See Supplementary material for details on the magnetic resonance sequences.
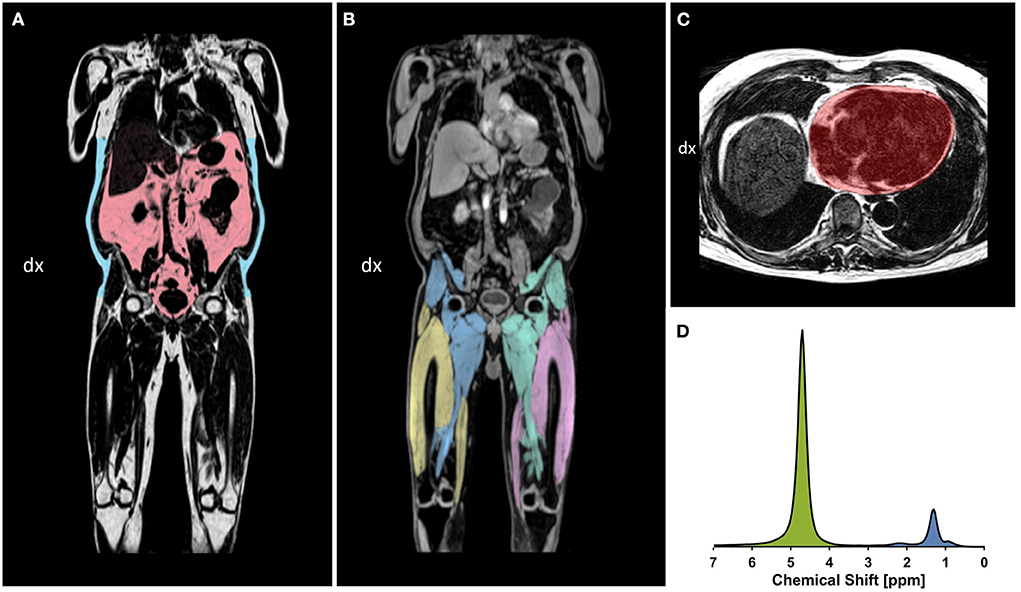
Figure 1. (A,B) example coronal slices of whole-body Dixon images. (A) Fat images with the VAT (red) and ASAT segmented (blue). (B) Water images with the segmented thigh muscle groups: right posterior thigh (blue), right anterior thigh (yellow), left posterior thigh (green), and left anterior thigh (pink). (C) Cardiac fat image in transversal view from the 3D Dixon sequence, showing the segmented region of interest (red) following the epicardial border. (D) Liver H-1 MR spectrum obtained at 1.5 T, showing the water resonance at 4.76 ppm (green), and the major fatty-acyl chain resonances at 1.21 ppm (methylene), as well as 0.9 ppm (methyl) and 2.2 ppm (alpha-olefinic etc) (blue). All lipid resonances were included in the integration procedure.
Analysis of MRI data
Abdominal and thigh composition
Images from the neck-to-knee mDixon sequence were analyzed and automatically segmented using the AMRA® Researcher (AMRA Medical AB, Linköping, Sweden) post-processing software. Briefly, the image analysis consisted of (1) image calibration, (2) fusion of image stacks, (3) image segmentation, and (4) quantification of fat and muscle volumes (15, 19, 20) and included manual quality control by a blinded trained operator. The following parameters were obtained:
• Abdominal subcutaneous adipose tissue (ASAT): the subcutaneous adipose tissue volume in the abdomen from the top of the femoral head to the top of the thoracic vertebra T9.
• Visceral adipose tissue (VAT): the adipose tissue volume within the abdominal cavity, excluding adipose tissue outside the abdominal skeletal muscles and adipose tissue and lipids within the cavity and posterior of the spine and back muscles.
• Fat-tissue free muscle volume (FFMV): the volume of all voxels with a fat fraction below 50%, i.e., “viable muscle tissue”, in the thighs.
• Muscle fat infiltration (MFI): the mean fat fraction in the FFMV of the right and left anterior thigh.
VAT, ASAT and FFMV were indexed to height squared (21) and denoted with the suffix “-i”, e.g. VATi.
Liver fat infiltration
To quantify liver fat infiltration, we measured 'Proton Density Fat Fraction' (PDFF) using MRS, which provides good precision on low fat concentrations and robustness toward artifacts. PDFF was measured using a 1H-MRS PRESS-sequence as described in previous publications (22, 23). Post-processing of the MRS data was performed by quantifying the integrals of water and fat resonances, respectively, using jMRUI and the AMARES algorithm (24, 25). In analyzing the spectra, we included all resonances representing different lipid moieties. The integrals were also corrected for systematic differences in T1 and T2 for fat and water, which were assumed to be as follows T1: 236 ms (fat) and 663 ms (water) and T2: 58.5 ms (fat) and 43.4 ms (water) (22, 26, 27).
Epicardial adipose tissue
The epicardial adipose tissue (EAT) volumes were determined by analyzing the transversal image stacks from the cardiac 3D Dixon sequence. ROI-drawing was performed using the open-source software MITK (version 2018.04.2) (28). Segmentation was performed in end-diastole from the most caudal slice where myocardium was visible to the first slice in the cranial direction where the lumen of right branch of the pulmonary artery displayed continuity. The adipose tissue volumes were then calculated using a separate script in MATLAB (MathWorks, Natick, MA), as described by Homsi et al. (29).
Left ventricular structure and function
Images from the cardiac 3D cine bSSFP sequence were analyzed using software Segment CMR (version 3.2 R8452, Medviso AB, Lund, Sweden) (30), to acquire structural (mass, end-diastolic volume and concentricity) and functional (stroke volume and ejection fraction) LV measures. To do this, epicardial and endocardial borders of the LV myocardium were segmented manually at end-diastole and end-systole using short axis images. The papillary muscles were regarded as part of the blood pool and LV mass (LVM) was acquired from the end-diastolic segmentation.
Stroke volume (SV) was calculated as: stroke volume = end-diastolic volume – end-systolic volume. Ejection fraction (EF) was calculated as: ejection fraction = stroke volume / end-diastolic volume. As a measure of concentricity, the ratio between LV mass and LV end-diastolic volume (LVEDV) was calculated for each participant, termed LV concentricity (LVC). Where applicable, cardiac parameters (including epicardial adipose tissue) were indexed to body surface area using the Mosteller method (31): BSA (m2) = (height (cm) × weight (kg)/3,600)1/2. Just as body composition variables, indexed cardiac parameters were denoted with the suffix “-i”.
Statistics
For all statistical analyses, SPSS IBM version 26 was used. A P-value of <0.05 was considered statistically significant. Normality of distribution was assessed using the Kolmogorov-Smirnoff test. For group comparisons of continuous variables, independent sample T-test was used for parametric and Mann-Whitney U test was used for non-parametric variables. Chi-square tests were used to compare categorical variables. A Bonferroni correction was used for group comparison of the MRI-derived body composition parameters as well as the structural and functional LV parameters.
To test the associations between cardiac remodeling and fat infiltration, linear regression analyses were performed. Structural and functional LV variables were deployed as dependent variables and the metrics of fat as independent variables. Regression analysis was performed in three steps. The crude regression model (Crude model) was a simple linear regression between each fat parameter and each LV parameter. Model 1 was a multiple linear regression with each fat parameter deployed separately, adjusted for sex and diabetes. Model 2 was a multiple linear regression with liver fat, epicardial fat and visceral fat deployed simultaneously in a single model, adjusted for sex and diabetes. MFI data were missing in 25% of the participants and was therefore not used in Model 2. Liver fat distribution was skewed despite stratifying for sex and diabetes, and therefore logarithmically transformed for this analysis. For every model we calculated the overall R2-value as well as P-value and standardized beta coefficients for each independent variable.
A logistic regression analysis was performed with the measures of body composition as predictors and the state of T2D as outcome. In the univariate model, each variable was deployed separately. We also performed a multivariate model including liver fat, visceral and abdominal subcutaneous fat simultaneously.
Results
Group comparison of demographic and basic clinical data
Between the groups, there were no statistical differences in age, sex, smoking, prevalence of frequent alcohol consumption or self-reported cardiac disease. The T2D group displayed significantly higher BMI (29.6 vs. 26.2 kg/m2, P < 0.001), higher P-glucose (8.4 vs. 5.3 mmol/L, P < 0.001), higher HbA1c (54 vs. 34 mmol/mol, P < 0.001) compared to the normoglycemic group. The T2D group also displayed significantly lower total cholesterol (4.3 vs. 5.7 mmol/L, P < 0.001), lower LDL cholesterol (2.2 vs. 3.6 mmol/L, P < 0.001) and higher self-reported usage of lipid-lowering medication (41.3 vs. 6.5 %, P < 0.001) (see Table 1).
Group comparison of body composition parameters
As compared to controls the T2D group had a significantly higher VATi volume (1.94 vs. 1.35 L/m2, P < 0.001), higher liver fat percentage (11.7 vs. 5.7 %, P < 0.001) and MFI percentage (9.4 vs. 7.7 %, P = 0.02) (see Table 2).
Group comparison of LV parameters
The T2D group displayed a higher E/e'-ratio (13.4 vs. 10.1, P < 0.001), indicating impaired diastolic function, and increased LV concentricity, (0.91 vs. 0.79 g/mL, P < 0.001). There were no significant differences in ejection fraction, LVEDVi or LVMi (see Table 3).
Linear regression analyses
In general, liver fat was associated with structural LV parameters. In the Crude model (not shown in tables), there was a positive relationship between liver fat and LVC (standardized beta=0.381, P < 0.001). The association was still significant when including diabetes and sex (standardized beta= 0.229, P < 0.05), but not when including VATi and EATi (Model 2). The negative associations of liver fat to LVMi and LVEDVi were still significant in Model 2, P < 0.05 for both. All parameters of fat had a significant positive relationship with E/e'-ratio in the Crude model. When adjusting for diabetes, only EATi and ASATi were significantly related to E/e', P < 0.05 for both. VATi was positively associated with both LVC and E/e' in the Crude model, although these associations were non-significant when adjusting for diabetes and sex (Model 1). Table 4 displays the results from the linear regression analyzes in model 1 and 2. Figure 2 shows scatterplots for the crude associations between liver fat, EATi and VATi, and LV parameters.
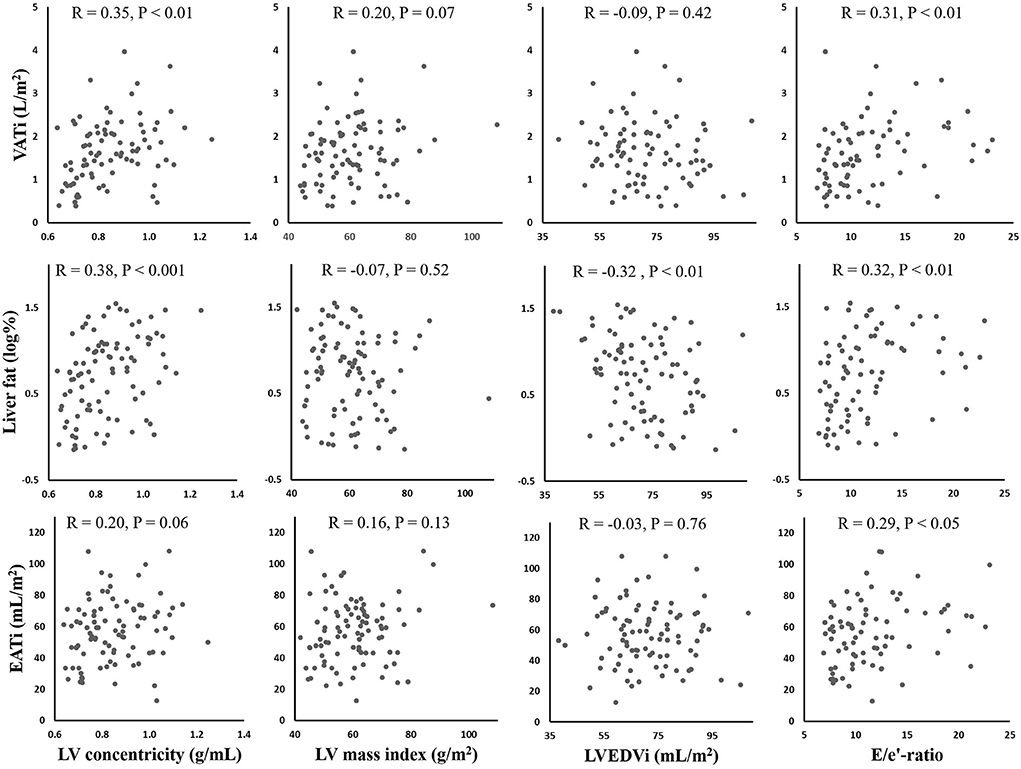
Figure 2. Scatterplots showing the correlations between fat depots (Y-axes) and left ventricular parameters (X-axes). Pearson's correlation coefficients (R) and P-values are provided for each plot. VATi, visceral adipose tissue index; EATi, epicardial adipose tissue index; LV, left ventricular; EDVi, end-diastolic volume index; E, early diastolic velocity of mitral inflow; e', early diastolic mitral annular velocity.
For Model 1 and Model 2, results were consistent when replacing diabetes status with BMI (not shown in tables). When adding adjustment for self-reported cardiac disease to sex and diabetes (not shown in tables), results in Model 1 and 2 were overall directionally consistent. Results in Model 1 persisted. Associations in Model 2 were slightly attenuated, as liver fat was borderline significant as predictor for LVMi (standardized beta = −0.20, P = 0.070) and LVEDVi (standardized beta = −0.25, P = 0.053).
Logistic regression analysis
In the univariate analyses, EATi, VATi, liver fat and MFI were significantly associated to T2D (P < 0.05 for all). In the multivariate model (including ASATi, VATi and liver fat), only VATi remained statistically significant (P < 0.05), with an odds ratio (OR) increase for T2D of 3.01 for each L/m2 increment in VATi (see Table 5).
Discussion
In our well-characterized study population, we found that liver fat infiltration, but not visceral fat, was related to structural LV parameters, including negative relationships to chamber mass and end-diastolic volume. Among the visceral and ectopic fat depots analyzed, the epicardial fat volume was the only parameter associated with diastolic function when adjusting for diabetes and sex. Visceral fat was significantly related to T2D, independently of liver fat and abdominal subcutaneous fat.
Association between regional fat and LV structure and function
Several large population-based studies have previously reported significant associations between visceral adipose tissue (VAT) and structural LV parameters. Neeland et al. (4) demonstrated that VAT was linked to increased LV concentricity and lower LVEDV. Other studies have reported positive relationships between VAT and LV concentricity (5), and negative relationships between VAT and LVEDV (32). Noticeably, neither of these studies analyzed or accounted for liver fat infiltration. A study that did analyze both VAT and liver fat was published by Schlett et al. (3). The authors found that both measures of fat were positively associated with LV concentricity and negatively with LVEDV, independently of diabetes. However, Schlett et al. did not analyze VAT and liver fat in the same regression model, which is a possible explanation for the discrepancy to our results. Our findings could indicate that liver fat acts as an interlink for the previously reported associations between VAT and LV structural remodeling, possibly due to the potentially progressive and inflammatory nature of fatty liver disease. For example, it has been demonstrated that patients with non-alcoholic fatty liver disease, especially non-alcoholic steatohepatitis, have increased systemic levels of inflammatory cytokines (33). Individuals with hepatic steatosis have also been shown to have altered LV metabolism in terms of reduced phosphocreatinine/adenosine triphosphate ratio, while still having preserved LV function (34).
Interestingly, while liver fat was related to LV structural parameters in our study, it was not significantly associated with any functional LV measures after adjustment for sex and diabetes. Among the visceral and ectopic fat depots, EAT was the only parameter in model 1 that was significantly positively associated with E/e' ratio, an indicator of LV diastolic dysfunction. Although this association was non-significant in model 2, perhaps due to lack of power, our results support previous findings that epicardial fat relates to LV function without a significant association to structural remodeling. A meta-analysis stated that EAT was associated with LV diastolic dysfunction, but the associations between EAT and LV structure were found to be largely inconsistent and uncertain (10). There are several postulated ways through which EAT could impair cardiac ventricular diastolic function. For example, through paracrine inflammatory mechanisms, molecular lipotoxic effects of free fatty acids (35, 36) as well as by physical obstructive effects (37). Given the close relationship between cardiac fat depots, epicardial fat may also act as a marker of intramyocardial triglyceride content, which too has been linked to impairment of LV function (36, 38–40). Finally, it is possible that altered hemodynamic factors, such as heart rate and blood pressure, influence the associations between ectopic fat infiltration and cardiac remodeling.
Diastolic impairment was the main cardiac functional abnormality found in this study population, related to both diabetes and to increasing volumes of EAT (independently of diabetes), while there were no significant findings regarding ejection fraction between the groups or in the regression analyses. Moreover, lower LVEDV, lower LVM and higher LV concentricity in the left ventricle was associated with liver fat. Taken together, these findings closely resemble the cardiac phenotype seen in heart failure with preserved ejection fraction, where patients are typically seen with preserved or increased ejection fraction and low or normal LVEDV (41). The role of ectopic fat in the development of heart failure with preserved ejection fraction may warrant further research, especially since previous studies have recognized obesity as a risk factor for this type of heart failure (42).
Association between regional fat and metabolic disease
The high proportion of individuals with T2D and matched design makes our study well suited to assess the relationship between individual regional fat depots and T2D using logistic regression. In the univariate models we found VAT as well as EAT, liver fat and MFI, but not ASAT, to be significant predictors of T2D. This is in line with the generally held view that ASAT and VAT have distinct metabolic and endocrine properties (43). And again, since visceral fat is closely related to the overall burden of ectopic fat (14), it is not surprising that both VAT and liver fat were significant predictors of T2D in our univariate analysis. In the multivariate analysis (including ASAT, VAT, and liver fat) VAT was a significant predictor of T2D while liver fat was non-significant. It is interesting that VAT, but not liver fat, was a significant predictor since several previous studies have demonstrated an independent role of fatty liver disease in T2D development (44, 45). It should be emphasized, however, that our analysis was employed to assess associations to clinically manifest disease. More sensitive and non-dichotomous measures of gluco-metabolic derangement, such as insulin sensitivity index, could yield additional insight into the roles of hepatic and visceral fat in development of metabolic disease. Nonetheless, our findings do support the hypothesis that different regional fat depots have dissimilar effects on cardiovascular and metabolic health.
Limitations
Due to the relatively small sample size, our data may lack statistical power to detect additional significant relationships between measures of fat, cardiac parameters and T2D. The rather small sample size limited the number of variables in the multiple linear regression analysis that could be adjusted for, in order to avoid overfitting. Moreover, we purposively limited the number of adjusting variables in the regression analyzes, in order to avoid adjusting for factors that potentially lie in the causal pathway between regional adiposity and cardiac remodeling. Finally, participants with previous or present cardiovascular disease were not excluded. As associations in model 2 were slightly attenuated when adjusting for self-reported cardiac disease, the observed associations between ectopic fat and LV remodeling could possibly be linked indirectly through various cardiac disorders.
Conclusions
In the present study, the application of advanced MR-techniques permitted comprehensive characterizations of whole-body fat depots including liver fat, epicardial fat, visceral fat, abdominal subcutaneous fat, and skeletal muscle fat in participants with T2D and matched controls. Our findings show that epicardial fat is associated with LV diastolic dysfunction. The findings also propose that previously reported relations between visceral fat and LV structure could be interlinked by the levels of liver fat infiltration. On the other hand, visceral fat was associated with T2D independently of liver and abdominal subcutaneous fat. Larger follow-up studies are needed to establish these results.
Data availability statement
The raw data supporting the conclusions of this article can be made available by the authors, upon reasonable request. Requests to access the datasets should be directed to C-JC, Y2FybC1qb2hhbi5jYXJsaGFsbEBsaXUuc2U=.
Ethics statement
The studies involving human participants were reviewed and approved by Linköping Ethical Review Board. The patients/participants provided their written informed consent to participate in this study.
Author contributions
C-JC, JE, OL, PL, and TE conceived and planned the study. C-JC, CÖ, ES, JE, MK, OL, PL, and TE were involved in data collection. CE, MK, and TS analyzed the data. CE and C-JC carried out the statistics. CE, C-JC, ME, and OL interpreted the results. CE drafted the manuscript. All authors read and critically revised the manuscript and approved the final version.
Funding
This work was funded by the Swedish Research Council, the Swedish Heart and Lung Foundation, and through ALF Grants Region Östergötland.
Acknowledgments
We thank Jasin Beij, Charlotte Brage, Mikael Forsgren, Mats Fredrikson, Markus Henningsson, Christian Simonsson, Jonathan Sundin, and Jens Tellman for their assistance with administration, technical input, statistics, and data management.
Conflict of interest
Authors MK and OL are employees of AMRA Medical AB. Authors OL and PL are stockholders in AMRA Medical AB. Author ME reports personal fees from Advisory Board AMRA Medical AB.
The remaining authors declare that the research was conducted in the absence of any commercial or financial relationships that could be construed as a potential conflict of interest.
Publisher's note
All claims expressed in this article are solely those of the authors and do not necessarily represent those of their affiliated organizations, or those of the publisher, the editors and the reviewers. Any product that may be evaluated in this article, or claim that may be made by its manufacturer, is not guaranteed or endorsed by the publisher.
Supplementary material
The Supplementary Material for this article can be found online at: https://www.frontiersin.org/articles/10.3389/fcvm.2022.813427/full#supplementary-material
Abbreviations
T2D, type 2 diabetes mellitus; VAT, visceral adipose tissue; ASAT, abdominal subcutaneous adipose tissue; EAT, epicardial adipose tissue; MFI, thigh muscle fat infiltration; FFMV, fat tissue-free muscle volume; BMI, body mass index; LV, left ventricular; LVEDV, left ventricular end-diastolic volume; LVM, left ventricular mass; LVC, left ventricular concentricity; EF, ejection fraction; E, early diastolic velocity of mitral inflow; A, late diastolic velocity of mitral inflow; e', early diastolic mitral annular velocity; NAFLD, non-alcoholic fatty liver disease; MRI, magnetic resonance imaging; PDFF, proton density fat fraction; PRESS, Point-RESolved Spectroscopy; SCAPIS, Swedish CArdioPulomonary bioImage Study.
References
1. Hiuge-Shimizu A, Kishida K, Funahashi T, Ishizaka Y, Oka R, Okada M, et al. Absolute value of visceral fat area measured on computed tomography scans and obesity-related cardiovascular risk factors in large-scale Japanese general population (the VACATION-J study). Ann Med. (2012) 44:82–92. doi: 10.3109/07853890.2010.526138
2. Fox CS, Massaro JM, Hoffmann U, Pou KM, Maurovich-Horvat P, Liu CY, et al. Abdominal visceral and subcutaneous adipose tissue compartments: association with metabolic risk factors in the framingham heart study. Circulation. (2007) 116:39–48. doi: 10.1161/CIRCULATIONAHA.106.675355
3. Schlett CL, Lorbeer R, Arndt C, Auweter S, Machann J, Hetterich H, et al. Association between abdominal adiposity and subclinical measures of left-ventricular remodeling in diabetics, prediabetics and normal controls without history of cardiovascular disease as measured by magnetic resonance imaging: Results from the KORA-FF4 S. Cardiovasc Diabetol. (2018) 17:1–12. doi: 10.1186/s12933-018-0721-0
4. Neeland IJ, Gupta S, Ayers CR, Turer AT, Rame JE, Das SR, et al. Relation of regional fat distribution to left ventricular structure and function. Circ Cardiovasc Imaging. (2013) 6:800–7. doi: 10.1161/CIRCIMAGING.113.000532
5. Abbasi SA, Hundley WG, Bluemke DA, Jerosch-Herold M, Blankstein R, Petersen SE, et al. Visceral adiposity and left ventricular remodeling: the multi-ethnic study of atherosclerosis. Nutr Metab Cardiovasc Dis. (2015) 25:667–76. doi: 10.1016/j.numecd.2015.03.016
6. Britton KA, Fox CS. Ectopic fat depots and cardiovascular disease. Circulation. (2011) 124:837–41. doi: 10.1161/CIRCULATIONAHA.111.077602
7. Adams LA, Anstee QM, Tilg H, Targher G. Non-Alcoholic fatty liver disease and its relationship with cardiovascular disease and other extrahepatic diseases. Gut. (2017) 66:1138–53. doi: 10.1136/gutjnl-2017-313884
8. Spearman JV, Renker M, Schoepf UJ, Krazinski AW, Herbert TL, De Cecco CN, et al. Prognostic value of epicardial fat volume measurements by computed tomography: a systematic review of the literature. Eur Radiol. (2015) 25:3372–81. doi: 10.1007/s00330-015-3765-5
9. Jung JY, Park SK, Ryoo JH, Oh CM, Kang JG, Lee JH, et al. Effect of non-alcoholic fatty liver disease on left ventricular diastolic function and geometry in the Korean general population. Hepatol Res. (2017) 47:522–32. doi: 10.1111/hepr.12770
10. Nerlekar N, Muthalaly RG, Wong N, Thakur U, Wong DTL, Brown AJ, Marwick TH. Association of volumetric epicardial adipose tissue quantification and cardiac structure and function. J Am Heart Assoc. (2018) 7:e009975. doi: 10.1161/JAHA.118.009975
11. Bonapace S, Perseghin G, Molon G, Canali G, Bertolini L, Zoppini G, et al. Nonalcoholic fatty liver disease is associated with left ventricular diastolic dysfunction in patients with type 2 diabetes. Diabetes Care. (2012) 35:389–95. doi: 10.2337/dc11-1820
12. Lee JJ, Pedley A, Hoffmann U, Massaro JM, Levy D, Long MT. Visceral and intrahepatic fat are associated with cardiometabolic risk factors above other ectopic fat depots: the framingham heart study. Am J Med. (2018) 131:684–92.e12. doi: 10.1016/j.amjmed.2018.02.002
13. Shah R V, Anderson A, Ding J, Budoff M, Rider O, Petersen SE, et al. Pericardial, but not hepatic, fat by CT is associated with cv outcomes and structure: the multi-ethnic study of atherosclerosis. JACC Cardiovasc Imaging. (2017) 10:1016–27. doi: 10.1016/j.jcmg.2016.10.024
14. Neeland IJ, Ross R, Després JP, Matsuzawa Y, Yamashita S, Shai I, et al. Visceral and ectopic fat, atherosclerosis, and cardiometabolic disease: a position statement. Lancet Diabetes Endocrinol. (2019) 7:715–25. doi: 10.1016/S2213-8587(19)30084-1
15. West J, Romu T, Thorell S, Lindblom H, Berin E, Holm ACS, et al. Precision of MRI-based body composition measurements of postmenopausal women. PLoS ONE. (2018) 13:1–16. doi: 10.1371/journal.pone.0192495
16. Seferović PM, Paulus WJ. Clinical diabetic cardiomyopathy: a two-faced disease with restrictive and dilated phenotypes. Eur Heart J. (2015) 36:1718–27. doi: 10.1093/eurheartj/ehv134
17. Bergström G, Berglund G, Blomberg A, Brandberg J, Engström G, Engvall J, et al. The Swedish CArdioPulmonary BioImage Study: objectives and design. J Intern Med. (2015) 278:645–59. doi: 10.1111/joim.12384
18. Lang RM, Badano LP, Mor-Avi V, Afilalo J, Armstrong A, Ernande L, et al. Recommendations for cardiac chamber quantification by echocardiography in adults: an update from the American society of echocardiography and the European association of cardiovascular imaging. Eur Heart J Cardiovasc Imaging. (2015) 16:233–71. doi: 10.1093/ehjci/jev014
19. Karlsson A, Rosander J, Romu T, Tallberg J, Grönqvist A, Borga M, et al. Automatic and quantitative assessment of regional muscle volume by multi-atlas segmentation using whole-body water-fat MRI. J Magn Reson Imaging. (2015) 41:1558–69. doi: 10.1002/jmri.24726
20. Leinhard OD, Johansson A, Rydell J, Smedby O, Nystrom F, Lundberg P, et al. Quantitative abdominal fat estimation using MRI. In: Proceedings—International Conference on Pattern Recognition (2008).
21. Heymsfield SB, Gallagher D, Mayer L, Beetsch J, Pietrobelli A. Scaling of human body composition to stature: New insights into body mass index. Am J Clin Nutr. (2007) 86:82–91. doi: 10.1093/ajcn/86.1.82
22. Nasr P, Forsgren MF, Ignatova S, Dahlström N, Cedersund G, Leinhard OD, et al. Using a 3% proton density fat fraction as a cut-off value increases sensitivity of detection of hepatic steatosis, based on results from histopathology analysis. Gastroenterology. (2017) 153:53–55.e7. doi: 10.1053/j.gastro.2017.03.005
23. Karlsson M, Ekstedt M, Dahlström N, Forsgren MF, Ignatova S, Norén B, et al. Liver R2* is affected by both iron and fat: a dual biopsy-validated study of chronic liver disease. J Magn Reson Imaging. (2019) 50:325–33. doi: 10.1002/jmri.26601
24. Vanhamme L, Van Den Boogaart A, Van Huffel S. Improved Method for Accurate and Efficient Quantification of MRS Data with Use of Prior Knowledge. J Magn Reson. (1997) 129:35–43. doi: 10.1006/jmre.1997.1244
25. Naressi A, Couturier C, Devos JM, Janssen M, Mangeat C, De Beer R, Graveron-Demilly D. Java-based graphical user interface for the MRUI quantitation package. In: Magnetic Resonance Materials in Physics, Biology and Medicine. Heidelberg, Germany: Springer Science and Business Media LLC (2001). p. 141–52.
26. Thomsen C, Becker U, Winkler K, Christoffersen P, Jensen M, Henriksen O. Quantification of liver fat using magnetic resonance spectroscopy. Magn Reson Imaging. (1994) 12:487–95. doi: 10.1016/0730-725X(94)92543-7
27. Lee SS, Lee Y, Kim N, Kim SW, Byun JH, Park SH, et al. Hepatic fat quantification using chemical shift MR imaging and MR spectroscopy in the presence of hepatic iron deposition: validation in phantoms and in patients with chronic liver disease. J Magn Reson Imaging. (2011) 33:1390–8. doi: 10.1002/jmri.22583
28. Wolf I, Vetter M, Wegner I, Böttger T, Nolden M, Schöbinger M, et al. The medical imaging interaction toolkit. Med Image Anal. (2005) 9:594–604. doi: 10.1016/j.media.2005.04.005
29. Homsi R, Meier-Schroers M, Gieseke J, Dabir D, Luetkens JA, Kuetting DL, et al. 3D-Dixon MRI based volumetry of peri- and epicardial fat. Int J Cardiovasc Imaging. (2016) 32:291–9. doi: 10.1007/s10554-015-0778-8
30. Heiberg E, Sjögren J, Ugander M, Carlsson M, Engblom H, Arheden H. Design and validation of Segment—freely available software for cardiovascular image analysis. BMC Med Imaging. (2010) 10:1. doi: 10.1186/1471-2342-10-1
31. Mosteller RD. Simplified calculation of body-surface area. N Engl J Med. (1987) 317:1098. doi: 10.1056/NEJM198710223171717
32. Van Hout MJP, Dekkers IA, Westenberg JJM, Schalij MJ, Scholte AJHA, Lamb HJ. The impact of visceral and general obesity on vascular and left ventricular function and geometry: a cross-sectional magnetic resonance imaging study of the UK Biobank. Eur Heart J Cardiovasc Imaging. (2020) 21:273–81. doi: 10.1093/ehjci/jez279
33. Haukeland JW, Damås JK, Konopski Z, Løberg EM, Haaland T, Goverud I, et al. Systemic inflammation in nonalcoholic fatty liver disease is characterized by elevated levels of CCL2. J Hepatol. (2006) 44:1167–74. doi: 10.1016/j.jhep.2006.02.011
34. Perseghin G, Lattuada G, De Cobelli F, Esposito A, Belloni E, Ntali G, et al. Increased mediastinal fat and impaired left ventricular energy metabolism in young men with newly found fatty liver. Hepatology. (2008) 47:51–8. doi: 10.1002/hep.21983
35. Iacobellis G. Local and systemic effects of the multifaceted epicardial adipose tissue depot. Nat Rev Endocrinol. (2015) 11:363–71. doi: 10.1038/nrendo.2015.58
36. Kankaanpää M, Lehto HR, Pärkkä JP, Komu M, Viljanen A, Ferrannini E, et al. Myocardial triglyceride content and epicardial fat mass in human obesity: relationship to left ventricular function and serum free fatty acid levels. J Clin Endocrinol Metab. (2006) 91:4689–95. doi: 10.1210/jc.2006-0584
37. Iacobellis G, Leonetti F, Singh N. M Sharma A. Relationship of epicardial adipose tissue with atrial dimensions and diastolic function in morbidly obese subjects. Int J Cardiol. (2007) 115:272–3. doi: 10.1016/j.ijcard.2006.04.016
38. Gao Y, Ren Y, Guo YK, Liu X, Xie LJ, Jiang L, Shen MT, Deng MY, Yang ZG. Metabolic syndrome and myocardium steatosis in subclinical type 2 diabetes mellitus: a 1 H-magnetic resonance spectroscopy study. Cardiovasc Diabetol. (2020) 19:70. doi: 10.1186/s12933-020-01044-1
39. Rijzewijk LJ, van der Meer RW, Smit JWA, Diamant M, Bax JJ, Hammer S, et al. Myocardial steatosis is an independent predictor of diastolic dysfunction in type 2 diabetes mellitus. J Am Coll Cardiol. (2008) 52:1793–9. doi: 10.1016/j.jacc.2008.07.062
40. Hata Y, Koike Y, Kimura N, Mochizuki J, Okamoto S, Matsumi H, et al. Longitudinal effect of myocardial fat deposition on left ventricular diastolic function: a retrospective cohort study. Int J Cardiovasc Imaging. (2022) 38:955–61. doi: 10.1007/s10554-021-02483-x
41. Katz AM, Rolett EL. Heart failure: When form fails to follow function. Eur Heart J. (2016) 37:449–54. doi: 10.1093/eurheartj/ehv548
42. Dunlay SM, Roger VL, Redfield MM. Epidemiology of heart failure with preserved ejection fraction. Nat Rev Cardiol. (2017) 14:591–602. doi: 10.1038/nrcardio.2017.65
43. Neeland IJ, Hughes C, Ayers CR, Malloy CR, Jin ES. Effects of visceral adiposity on glycerol pathways in gluconeogenesis. Metabolism. (2017) 67:80–9. doi: 10.1016/j.metabol.2016.11.008
44. Linge J, Whitcher B, Borga M, Dahlqvist Leinhard O. Sub-phenotyping metabolic disorders using body composition: an individualized, nonparametric approach utilizing large data sets. Obesity. (2019) 27:1190–9. doi: 10.1002/oby.22510
Keywords: ectopic fat, left ventricular structure, left ventricular diastolic function, cardiac remodeling, magnetic resonance imaging, type 2 diabetes, visceral fat
Citation: Edin C, Ekstedt M, Scheffel T, Karlsson M, Swahn E, Östgren CJ, Engvall J, Ebbers T, Leinhard OD, Lundberg P and Carlhäll C-J (2022) Ectopic fat is associated with cardiac remodeling—A comprehensive assessment of regional fat depots in type 2 diabetes using multi-parametric MRI. Front. Cardiovasc. Med. 9:813427. doi: 10.3389/fcvm.2022.813427
Received: 11 November 2021; Accepted: 30 June 2022;
Published: 28 July 2022.
Edited by:
Otto Alexander Sanchez, University of Minnesota Twin Cities, United StatesReviewed by:
Sven Månsson, Lund University, SwedenGrigorios Korosoglou, GRN Klinik Weinheim, Germany
Copyright © 2022 Edin, Ekstedt, Scheffel, Karlsson, Swahn, Östgren, Engvall, Ebbers, Leinhard, Lundberg and Carlhäll. This is an open-access article distributed under the terms of the Creative Commons Attribution License (CC BY). The use, distribution or reproduction in other forums is permitted, provided the original author(s) and the copyright owner(s) are credited and that the original publication in this journal is cited, in accordance with accepted academic practice. No use, distribution or reproduction is permitted which does not comply with these terms.
*Correspondence: Carl Edin, Y2FybC5lZGluQGxpdS5zZQ==