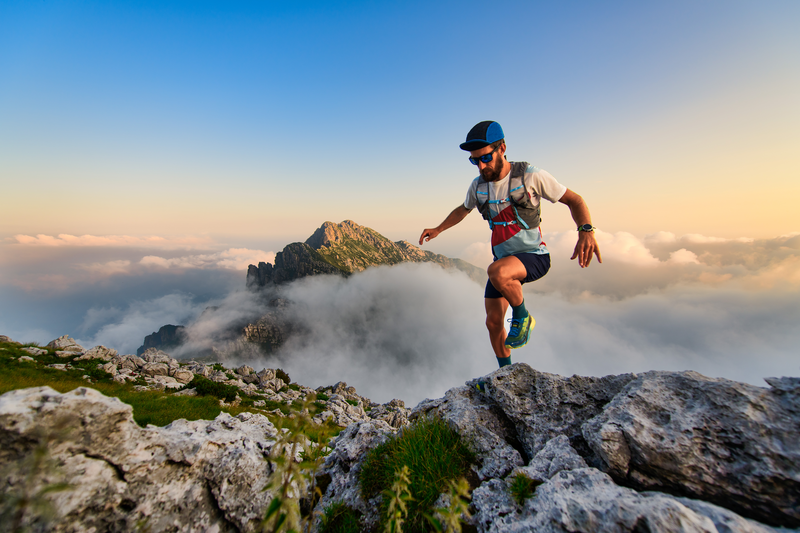
95% of researchers rate our articles as excellent or good
Learn more about the work of our research integrity team to safeguard the quality of each article we publish.
Find out more
BRIEF RESEARCH REPORT article
Front. Cardiovasc. Med. , 13 April 2022
Sec. General Cardiovascular Medicine
Volume 9 - 2022 | https://doi.org/10.3389/fcvm.2022.798954
Objective: Left ventricular hypertrophy (LVH) is a common complication of hypertension and microRNAs (miRNAs) are considered to play an important role in cardiac hypertrophy development. This study evaluated the relationship between circulating miRNAs and LVH in hypertensive patients.
Methods: Two cohorts [exploratory (n = 42) and validation (n = 297)] of hypertensive patients were evaluated by clinical, laboratory and echocardiography analysis. The serum expression of 754 miRNAs in the exploratory cohort and 6 miRNAs in the validation cohort was evaluated by the TaqMan OpenArray® system and quantitative polymerase chain reaction, respectively.
Results: Among the 754 analyzed miRNAs, ten miRNAs (miR-30a-5p, miR-let7c, miR-92a, miR-451, miR-145-5p, miR-185, miR-338, miR-296, miR-375, and miR-10) had differential expression between individuals with and without LVH in the exploratory cohort. Results of multivariable regression analysis adjusted for confounding variables showed that three miRNAs (miR-145-5p, miR-451, and miR-let7c) were independently associated with LVH and left ventricular mass index in the validation cohort. Functional enrichment analysis demonstrated that these three miRNAs can regulate various genes and pathways related to cardiac remodeling. Furthermore, in vitro experiments using cardiac myocytes demonstrated that miR-145-5p mimic transfection up-regulated the expression of brain and atrial natriuretic peptide genes, which are markers of cardiac hypertrophy, while anti-miR-145-5p transfection abrogated the expression of these genes in response to norepinephrine stimulus.
Conclusions: Our data demonstrated that circulating levels of several miRNAs, in particular miR-145-5p, miR-451, and let7c, were associated with LVH in hypertensive patients, indicating that these miRNAS may be potential circulating biomarkers or involved in hypertension-induced LV remodeling.
Hypertension is characterized by high blood pressure (BP) levels and is associated with target-organs damage and increased risk of cardiovascular events (1–3). Sustained BP elevation increases left ventricular (LV) wall tension, and may commonly lead to a compensatory increase in LV mass (LVM), known as left ventricular hypertrophy (LVH). Importantly, LVH is associated with an increased risk of cardiovascular morbidity and mortality (4, 5).
Alternative factors, including genetic (6), epigenetic (7) and environmental (8) factors, can contribute to LVH. MicroRNAs (miRNAs) are a class of small non-coding RNAs that modulate gene expression at the post-transcriptional level. They are believed to comprise about 1% to 5% of the human genome, and currently at least 2,654 mature miRNA sequences have been described (9). Several studies in humans and experimental models have suggested an important role of miRNAs in cardiac hypertrophy (10), but little is known regarding the impact of miRNAs on hypertension-induced LV remodeling. In this regard, an association between selected circulating miRNAs and LVM has been reported in hypertensive individuals (11–15).
This study aimed at identifying circulating miRNAs related to LVH in hypertensive individuals and evaluating the functional role of selected miRNAs on markers of cardiac myocyte hypertrophy in vitro.
Additional detailed methods are included in the Supplementary Data.
The authors declare that all supporting data are available within the article (and in the Data Supplement).
The present study included two cohorts of consecutive hypertensive patients followed at the Hypertension Outpatient Clinic of the Clinics Hospital of the University of Campinas who were enrolled from 2018 to 2019. The exploratory cohort included 42 patients (26 with LVH and 16 without LVH) and the validation cohort comprised 297 patients (162 with LVH and 135 without LVH). Exclusion criteria were age under 18 years, identifiable causes of secondary hypertension, evidence of significant cardiac valve disease and hypertrophic cardiomyopathy. The research was carried out in accordance with the Declaration of Helsinki. This study was approved by the Human Research Ethics Committee of the University of Campinas, and all patients gave written informed consent to participate.
Clinical data were gathered from each participant and included information on: age, sex, smoking, hypertension, diabetes mellitus, use of antihypertensive medications, body mass index (BMI), BP, heart rate. BP and heart rate were measured in the sitting position using a validated digital oscillometric device (HEM-705CP; Omron Healthcare, Kyoto, Japan) with appropriate cuff sizes. BMI was calculated as body weight divided by height squared (kg/m2). Fasting low density lipoprotein (LDL)-cholesterol, high-density lipoprotein (HDL)-cholesterol, triglycerides, creatinine, and glucose levels were measured using standard laboratory techniques. Hypertension was defined as systolic BP ≥140 mmHg or diastolic ≥90 mmHg or use of antihypertensive medications. Diabetes mellitus was diagnosed if fasting blood glucose was ≥126 mg/dl or when participants were taking hypoglycemic medications.
Echocardiography was performed by a single physician using a Vivid q device (General Electric, Milwaukee, Wisconsin, USA) equipped with a 3S-RS transducer, as previously described (16–18). LVMI was calculated as LV mass/body surface area. Relative wall thickness (RWT) was measured as 2*posterior wall thickness/LV diastolic diameter. LVH was defined as LVMI ≥95 g/m2 and ≥ 115 g/m2 in women and men, respectively. LV geometric patterns were defined as follows: normal geometry (No LVH and RWT ≤0.42), concentric remodeling (No LVH and RWT >0.42), eccentric hypertrophy (LVH and RWT ≤0.42) and concentric hypertrophy (LVH and RWT >0.42). LV ejection fraction was estimated by the Simpson's method.
Samples from both cohorts were extracted using the miRNeasy Serum/Plasma Kit (Qiagen). The quality of miRNA was assessed by measuring the percentage of miRNAs in the amount of small RNA using a Bioanalyzer 2,100 (Agilent, Santa Clara, CA), as previously reported (19). In the exploratory cohort, the miRNA profile was analyzed by the TaqMan OpenArray Human MicroRNA system, a quantitative polymerase chain reaction (qPCR)-based miRNA array platform that contains 754 microRNAs on a microfluidic platform across two sets of primer pools, panel A and B (LifeTechnologies). Data were normalized using the global normalization method as suggested by the manufacturer and previous reports (20). Six circulating miRNAs with the highest fold change in the exploratory study were chosen for the validation in the validation cohort by qRT-PCR using a customized plate (LifeTechnologies). Reverse transcription was performed using the SuperScript®III First Strand Synthesis Kit. Specific miRNA PCR primers were synthesized by Life Technologies. Real-time PCR assays were performed with the TaqMan Master Mix (Life Technologies) on a 7900HT FAST Real- Time PCR System (Life Technologies). The comparative Ct (ΔΔCt) method to quantify relative gene expression was used, and fold change (FC) was calculated as FC = 2- ΔΔCt, where Ct is defined as the PCR cycle number at which the fluorescence meets the threshold in the amplification plot (21). Data were normalized using a geometric mean of U6 snRNA (non-coding small nuclear RNA) and miR-16 as the housekeeping genes.
To understand the biological relevance of differentially expressed miRNAs, we performed functional enrichment analysis. The miRNAs differentially expressed between patients with and without LVH that significantly correlated with LVMI were uploaded into miRWalk (version 2) (22). To strengthen the data, only mRNAs predicted in at least four of five tools (miRanda, miRDB, miRWalk, RNA22, and TargetScan) were considered as possible miRNA targets. We used the Database for Annotation, Visualization, and Integrated Discovery (DAVID) to determine the enriched pathways. Only pathways with more than 10 genes, fold enrichment >1.5, and Fisher's exact test p-value < 0.05 were considered (23).
We used the adult mouse atrial muscle cell line HL-1, which has been extensively used to assess mechanisms related to cardiac myocyte hypertrophy (24, 25), to evaluate the functional impact of selected miRNAs. HL-1 cells were cultured at 37°C and 5% CO2 atmosphere in Claycomb medium (Sigma, 51800C) supplemented with 2 mM L-Glutamine (Sigma, G7513), penicillin-streptomycin (Sigma, P4333) and 10 %(v/v) FCS (Sigma; F2442) in the absence or presence of 100 μM of norepinephrine (Sigma, A0937) as a hypertrophic stimulus (26, 27) for 2 weeks (28) on gelatin-fibronectin [0.02 % (w/v) gelatin; 5 mg/ml fibronectin] (Sigma, F1141) pre-coated plates (29). Then, HL-1 cells were transiently transfected with miR-145-5p mimetic, anti-miR-145-5p or negative control miRNA (Applied Biosystems, ThermoFisher Scientific, Grand Island, NY) at final concentrations of 30 nM. This assay was carried out using the Lipofectamine 2000 Reagent (Invitrogen, Carlsbad, CA) following the manufacturer's instructions. Cells were harvested for subsequent analyses 48 h after the transfection. All transfection experiments were carried out in triplicate.
For detecting the in vitro expression of miRNAs and markers of cardiac myocyte hypertrophy, total RNA from cardiac myocytes was isolated using Mirvana Paris Kit (Applied Biosystems, CA). We evaluated the expression of brain natriuretic peptide (Nppb) and atrial natriuretic peptide (Nppa) genes, which are markers of cardiac myocyte hypertrophy, and miR-145-5p using the TaqMan Gene Expression Assays Kit (Applied Biosystems, CA). The polymerase chain reaction was performed in a StepOnePlus ™ System (ThermoFisher) real-time PCR system. Gapdh (Mm99999915_g1) was used as endogenous control for Nppb and Nppa expression, while U6 snRNA was used to normalize miR-145-5p expression.
Continuous variables with normal or non-normal distribution are presented as mean ± standard deviation (SD) or median [25th, 75th percentiles]. Differences in continuous variables with normal or non-normal distribution between the studied groups (with and without LVH) were evaluated by unpaired student's t-test and Mann-Whitney U-test, respectively. Chi-square test was used to compare categorical variables. Differences in continuous variables derived from cell assays were evaluated by one-way analysis of variance (ANOVA) followed by the Tukey test. The correlation between echocardiographic variables and log-transformed expression of miRNAs was assessed by the Person's Method. Multivariable linear regression analysis evaluated the association of log-transformed expression of circulating miRNAs with LVH, LVMI, and LV geometric patterns in the validation cohort, adjusting for variables that have been reported to influence LV remodeling: age, sex, diabetes, body mass index, systolic blood pressure, creatinine, smoking, and antihypertensive classes (5). p-value < 0.05 was considered statistically significant. SPSS 15.0 software was used for statistical analyses.
The characteristics of the exploratory cohort (n = 42; 57.7 ± 8.5 years, 52% males) and the validation cohort (n = 297; 61.3 ± 12.1 years, 43% males) are shown in the Supplementary Table S1. The cohorts had similar characteristics except for higher BP and rates of diabetes and use of diuretics, greater LV wall thickness and relative wall thickness values, and lower LDL-cholesterol levels in the validation cohort.
Clinical, laboratory, and echocardiographic features of the exploratory and validation cohorts according to the presence or not of LVH are presented in Table 1. There were no differences in clinical and laboratory characteristics between participants according to LVH status, except for greater systolic BP and creatinine levels in LVH patients of the validation cohort compared with those without LVH. In addition, patients with LVH had greater LV dimensions, LV mass and RWT than those without LVH in both cohorts.
The analysis of serum expression of 754 miRNAs using the OpenArray MicroRNA System in the exploratory cohort showed that 357 miRNAs were expressed in at least one of the two groups (with and without LVH). For the differential analysis, only 122 miRNAS that were expressed in at least 50% of the participants within each group were considered (Supplementary Table S2). Of these, 10 miRNAs (miR-30a-5p, miR-let7c, miR-92a, miR-451, miR-145-5p, miR-185, miR-338, miR-296, miR-375 and miR-10) were significantly upregulated in patients with LVH compared with those without LVH (Figure 1A, Supplementary Table S3 and Figure S1). Of these 10 miRNAs, six miRNAs with the highest fold change in the exploratory study (miR-30a-5p, miR-let7c, miR-92a, miR-451, miR-145-5p, miR-185) were chosen to have their serum expression measured by qRT-PCR in the validation cohort. All except one miRNA (miR-185) showed higher expression in LVH patients when compared with those without LVH in the validation cohort (Figure 1B and Supplementary Table S3). Bivariate correlation analysis was then performed to evaluate the relationship of circulating miRNAs with LVMI in the validation cohort. Of the six tested miRNAs, miR-145-5p (r = 0.153; p = 0.011), miR-451 (r = 0.162; p = 0.016) and miR-let7c (r = 0.242; p < 0.001) significantly correlated with LVMI, while miR-92a (r = 0.098; p = 0.10), miR30a-5p (r = 0.093; p = 0.12) and miR-185 (r = −0.155; p = 0.07) did not. Systolic BP was positively correlated with miR-30a-5p (r = 0.146; p = 0.015) and miR-451 (r = 0.213; p = 0.002), while no correlation between the studied miRNAs and the presence of diabetes was observed (Supplemental Table S4).
Figure 1. Differential expression analysis of miRNAs obtained in serum of hypertensive patients. (A) Differentially expressed serum miRNAs (fold change) in patients with left ventricular hypertrophy (LVH) compared with patients without LVH in the exploratory cohort. (B) Differentially expressed serum miRNAs (fold change) in patients with LVH compared with patients without LVH in the validation cohort. Box and whisker plots are represented for each miRNA and indicate the fold change in expression of LVH patients compared with patients without LVH. p-values from independent Mann-Whitney test are presented. †p < 0.001; *p < 0.05.
Results of multivariable linear regression analyses adjusted for potentially relevant confounders (age, sex, body mass index, systolic blood pressure, diabetes mellitus, creatinine, smoking, and antihypertensive medications) showed that miR-30a-5p, miR-let7c, miR-92a, miR-451, miR-145-5p remained associated with LVH, while only miR-145-5p, miR-let7c, and miR-451 showed an independent association with LVMI in the validation cohort (Supplementary Table S5). As a secondary analysis, we evaluated the relationship between LV geometric patterns and selected miRNAs (miR-30a-5p, miR-let7c, miR-92a, miR-451, miR-145-5p, miR-185) adjusted for potential confounders in the validation cohort. Compared with the normal geometric pattern, patients with concentric hypertrophy had greater expression of miR-let7c, miR-92a, miR-145-5p, miR-30a-5p, and miR-451, while patients with eccentric hypertrophy had greater expression of miR-let7c and miR-145-5p (Supplementary Table S6).
Using the miRWALK2.0 software, 1030 predicted genes were identified to be targeted by the three miRNAs that were associated with both LVH and LVMI (miR-145-5p, miR-let7c and miR-451). The number of genes targeted by each of the miRNAs is shown in the Venn diagram presented in Figure 2A. Figure 2B shows the predicted pathways of the aforementioned miRNAs. Pathways related to cardiac remodeling and vascular diseases, and metabolic and inflammatory pathways were predicted, including calcium and adrenergic signaling pathways, focal adhesion and hypertrophic cardiomyopathy.
Figure 2. Pathway enrichment analysis of predicted target genes. (A) Gene ontology analysis for the three miRNAs (miR-145-5p, miR-451 and miR-let7c) that correlated with both LVH and LVMI in the validation cohort. Venn's diagram of genes targeted by each of the three miRNAs with p < 0.05. (B)- Gene set enrichment analyses of pathways targeted by miR-145-5p, miR-451 and miR-let7c.
Due to controversial results in the literature (30–32), we decided to assess whether miR-145 is involved in the regulation of cardiac myocyte hypertrophy in vitro. We first evaluated the expression of this miRNA in HL-1-cells. We found that miR-145-5p was constitutively expressed in these cells and that its expression increased by ≈ 3-fold after norepinephrine stimulus and by ≈10-fold after transfection with miR-145-5p mimic (Figure 3A). The expression of Nppa and Nppb significantly increased in HL-1-cells transfected with miR-145-5p mimic (30nM) and in response to norepinephrine (Figures 3B,C). By contrast, transfection of anti-miR-145-5p (30nM) markedly decreased norepinephrine-induced expression of Nppa and Nppb when compared with cells transfected with negative control (Figures 3B,C).
Figure 3. Effects of miR-145-5p on cardiac myocyte hypertrophy. (A) The mRNA levels of miR-145-5p were increased in HL-1 cells treated with norepinephrine or transfected with miR-145-5p mimic (30 nM). Relative miR-145-5p expression corresponded to average expressions (fold change) normalized to U6. (B,C) HL-1 cells transfected with miR-145-5p mimic (30 nM) increased the mRNA level of Nppa (atrial natriuretic peptide gene) and Nppb (brain natriuretic peptide gene), while transfection with miR-145-5p inhibitor (30 nM) abrogated norepinephrine-induced increases in Nppa and Nppb. Relative expressions of hypertrophy markers were expressed as the average expressions (fold change) normalized to Glyceraldehyde-3-posphate dehydrogenase (GAPDH), by RT-PCR analysis. Data are presented as mean ± SEM (n = 3). Differences in the expression of Nppa, Nppb and miR-145 were assessed by one-way ANOVA and Tukey post hoc test. *p < 0.05; **p < 0.001.
In the present study, three main results were reported. First, we found a differential expression of 10 miRNAs from 754 studied miRNAs between hypertensive patients with and without LVH in an exploratory cohort. Second, we confirmed a differential expression of 5 miRNAs (miR-30a-5p, miR-let7c, miR-92a, miR-451, and miR-145-5p) in an extended sample of hypertensive patients (validation cohort). Of these miRNAs, miR-145-5p, miR-451, and let7-c expression levels were significantly associated with both LVH and LVMI even after adjusting for relevant confounders. Third, results of cell culture assays demonstrated a role of miR-145-5p in the development of cardiac myocyte hypertrophy. These findings provide novel evidence regarding the potential value of previously undisclosed miRNAs as circulating biomarkers or potential mediators of hypertension-induced LV remodeling.
Several studies have suggested that miRNAs play an important role in the LV remodeling and may also serve as circulating biomarkers of LVH (11, 33, 34). However, the knowledge regarding the relationship between circulating miRNAs and LVH in hypertensive individuals is scarce and has been restricted to the evaluation of a small number of pre-selected miRNAs in small samples of patients. In this regard, relationships between LVMI and circulating levels of miR-1, miR-133a, miR-26b, miR-208b, miR-499, and miR-21, miR-9 (12), miR-30e (13), miR-27b (14), miR-29 (15), miR-7, and miR-26 (34) have been reported. Notably, none of these miRNAs showed an association with LVH in our study. These divergences could be explained by differences in sample size, and in ethnic and clinical characteristics among the studied samples, and reinforce the need of confirmatory studies in alternative populations. It is also worth mentioning that the miRNAs which were associated with LVH in our validation cohort were derived from the evaluation of 754 miRNAs in an exploratory sample of 42 hypertensive individuals, thus strengthening the validity of our findings.
In our analysis, there was a significant association of circulating miR-145-5p levels with LVMI and LVH. We then evaluated the impact of this miRNA on cardiac myocyte hypertrophy in vitro. The results of cell assays showed that treatment with norepinephrine, a hypertrophic stimulus, increased the expression of miR-145-5p, while overexpression of miR-145-5p also upregulated the expression of markers of cardiac myocyte hypertrophy. In addition, transfection of anti-miR-145-5p markedly decreased norepinephrine-induced expression of cardiac myocyte hypertrophy markers. These data support the notion that miR-145-5p may play a role in the pathophysiology of cardiac myocyte hypertrophy. Conversely, our findings are in contrast with previous in vitro studies which showed that miR-145 transfection attenuated isoproterenol- and angiotensin II-induced cardiac myocyte hypertrophy (30, 31) but in agreement with the results of Xu et al. (32) who showed that miR-145 expression increases in induced hypertrophic cardiomyocytes by angiotensin II. The reasons for these discrepancies are not clear, but it is possible that differences in the experimental protocol among the studies might have played a role in this regard. In our protocol, HL-1 cells were treated or not with norepinephrine for 2 weeks, transfected with miR-145-5p or miR-145-5p antagonist, and then analyzed 48 h after. In the study by Lin et al. (30) H9C2 cells were overexpressed with miR-145-5p for 6 h, and then treated with Angiotensin-II for 24 h before analyses, while in the study by Li et al., neonatal rat cardiac myocytes from 1-day-old Sprague–Dawley rats were transfected with miR-145 adenovirus for 48 h, and then stimulated with isoproterenol for 24 h before analyses (31). Regardless of the divergences in the results of in vitro studies, our findings provide novel evidence that miR-145-5p may be a circulating marker of LV hypertrophy among hypertensive individuals.
Our data showed that not only miR-145-5p, but also miR-30a-5p, miR-let7c, miR-92a, miR-451 had an independent relationship with LVH in both validation and exploratory cohorts. Notably, these latter 4 miRNAs (miR-30a-5p, miR-let7c, miR-92a, miR-451) have been previously reported to be involved in adverse cardiac remodeling in experimental models (35–38). In addition, we found that only miR-145-5p, miR-let7c, and miR-451 were related to both LVH and LVMI, and therefore could be more specifically involved in the development of hypertension-induced LV hypertrophy. To better understand the biological relevance of these three miRNAs, functional enrichment analysis was performed aiming at identifying their targeted genes and pathways. We found that these miRNAs regulate genes and pathways related to cardiac remodeling and vascular diseases, including metabolic, inflammatory, focal adhesion, calcium and adrenergic signaling pathways. Overall, the current findings suggest that miR-145-5p, miR-let7c, and miR-451 comprise an attractive group of miRNAs that might play a role in hypertension-induced LV remodeling and could emerge as potential biomarkers or therapeutic targets in this regard.
Hypertension is classically assumed to induce LV concentric hypertrophy due to increases in LV wall stress (5). However, several other factors, including hemodynamic variables, as well as acquired and genetic factors may modulate LV geometry in hypertensive individuals, resulting in alternative LV geometric patterns, such as eccentric hypertrophy (5, 39). Available evidence obtained in experimental and clinical settings has also suggested that miRNAs may play a role in the development of LV geometric patterns (11, 40, 41). In our study, we found that patients with eccentric hypertrophy had increased expression of miR-miR-let7c, and miR-145-5p, while those with concentric hypertrophy had higher expression of miR-let7c, miR-92a, miR-145-5p, miR-30a-5p, and miR-451. As far as we know, this is the first study to describe the expression of these aforementioned miRNAs as a function of LV geometric patterns in hypertensive patients and further indicate that the assessment of LV geometry can also be a target in studies focusing on the role of miRNAs in hypertension-induced LV remodeling.
We acknowledge that our study has some limitations. First, we only selected six miRNAs for validation among the miRNAs that were differentially expressed between hypertensive patients with and without LVH in the exploratory cohort. Therefore, further studies should be performed to validate the remaining miRNAs whose expression was different between the two groups. Second, as any cross-sectional study, the influence of residual confounding cannot be excluded and the association between miRNAs and LV structural parameters cannot be assumed to be causal. Third, it is possible that the use of antihypertensive medications and clinical characteristics might have influenced our findings. In order to overcome this limitation, we included each antihypertensive class and potential relevant clinical confounders as independent variables in multivariable analyses regarding the validation cohort. Conversely, because the major aim of the exploratory cohort was to perform miRNA mining and due to its smaller number of enrolled patients, we opted to only perform unadjusted analyses for the relationship of miRNAs with LVH in this cohort.
In conclusion, starting from an analysis of 754 miRNAs, we found that the circulating levels of three miRNAs (miR-145-5p, miR-451, and let7-c) were associated with LVH and left ventricular mass index in hypertensive individuals. Furthermore, in vitro studies showed that miR-145-5p may have a pro-hypertrophic effect on cardiac myocytes. These findings suggest that miR-451 and let7c, and especially miR-145-5p, may be potential circulating biomarkers or may be involved hypertension-induced LV remodeling.
The original contributions presented in the study are included in the article/Supplementary Material, further inquiries can be directed to the corresponding author/s.
The studies involving human participants were reviewed and approved by was approved by the Ethics Committee of the State University of Campinas. 56841616.5.0000.5404. The patients/participants provided their written informed consent to participate in this study.
EL, RS, and WN designed this study, acquired and interpreted data, and wrote the manuscript. LP, EOM, LC-R, ERM, CV, JP-M, JM-S, OC-F, and AS acquired and/or interpreted data and revised manuscript for intellectual content. All authors read and agreed with the published version of the manuscript.
The study was supported by São Paulo Research Foundation (FAPESP 2017/23563-1) for Dr. Schreiber and by FAPESP 2013/07607-8.
The authors declare that the research was conducted in the absence of any commercial or financial relationships that could be construed as a potential conflict of interest.
All claims expressed in this article are solely those of the authors and do not necessarily represent those of their affiliated organizations, or those of the publisher, the editors and the reviewers. Any product that may be evaluated in this article, or claim that may be made by its manufacturer, is not guaranteed or endorsed by the publisher.
The Supplementary Material for this article can be found online at: https://www.frontiersin.org/articles/10.3389/fcvm.2022.798954/full#supplementary-material
BP, blood pressure; LV, left ventricular; LVM, left ventricular mass; LVMI, left ventricular mass index; LVH, left ventricular hypertrophy; miRNA, microRNA; Nppb, brain natriuretic peptide gene; Nppa, atrial natriuretic peptide gene; RT-qPCR, real-time quantitative polymerase chain reaction.
1. Williams B. The year in hypertension. J Am Coll Cardiol. (2009) 55:65–73. doi: 10.1016/j.jacc.2009.08.037
2. Nadruz W Jr, Claggett B, Henglin M, Shah AM, Skali H, Rosamond WD, et al. Racial disparities in risks of stroke. N Engl J Med. (2017) 376:2089–90. doi: 10.1056/NEJMc1616085
3. Nadruz W Jr, Claggett B, Henglin M, Shah AM, Skali H, Rosamond WD, et al. Widening racial differences in risks for coronary heart disease. Circulation. (2018) 137:1195–7. doi: 10.1161/CIRCULATIONAHA.117.030564
4. Lorell BH, Carabello BA. Left Ventricular hypertrophy. Circulation. (2000) 102:470–9. doi: 10.1161/01.CIR.102.4.470
5. Nadruz W Jr. Myocardial remodeling in hypertension. J Hum Hypertens. (2015) 29:1–6. doi: 10.1038/jhh.2014.36
6. Rossi GP, Ceolotto G, Caroccia B, Lenzini L. Genetic screening in arterial hypertension. Nat Rev Endocrinol. (2017) 13:289–98. doi: 10.1038/nrendo.2016.196
7. Gosev I, Zeljko M, Duric Z, Nikolic I, Gosev M, Ivcevic S, et al. Epigenome alterations in aortic valve stenosis and related left ventricular hypertrophy. Clin Epigenetics. (2017) 9:106. doi: 10.1186/s13148-017-0406-7
8. Sakamoto M, Matsutani D, Kayama Y. Possibility of a New Therapeutic Strategy for Left Ventricular Dysfunction in Type 2 Diabetes. J Clin Med Res. (2018) 10:799–805. doi: 10.14740/jocmr3584w
9. Kozomara A, Birgaoanu M, Griffiths-Jones S. miRBase: from microRNA sequences to function. Nucleic Acids Res. (2019) 47: D155–62. doi: 10.1093/nar/gky1141
10. Wehbe N, Nasser AS, Pintus G, Badran A, Eid AH, Baydoun E. MicroRNAs in cardiac hypertrophy. Int J Mol Sci. (2019) 20:4714. doi: 10.3390/ijms20194714
11. Kontaraki JE, Marketou ME, Parthenakis FI, Maragkoudakis S, Zacharis EA, Petousis S, et al. Hypertrophic and antihypertrophic microRNA levels in peripheral blood mono-nuclear cells and their relationship to left ventricular hypertrophy in patients with essential hypertension. J Am Soc Hypertens. (2015) 9:802–10. doi: 10.1016/j.jash.2015.07.013
12. Kontaraki JE, Marketou ME, Zacharis EA, Parthenakis FI, Vardas PE. MicroRNA-9 and microRNA-126 expression levels in patients with essential hypertension: potential markers of target-organ damage. J Am Soc Hypertens. (2014) 8:368–75. doi: 10.1016/j.jash.2014.03.324
13. Xu H, Xu Y, Yang J, Juang J. Circulating MicroRNA-30e Predicts left ventricular hypertrophy in essential hypertensive patients. Rev Invest Clin. (2021) 73:138–44. doi: 10.24875/RIC.20000447
14. Wang Y, Chen S, Gao Y, Zhang S. Serum MicroRNA-27b as a screening biomarker for left ventricular hypertrophy. Tex Heart Inst J. (2017) 44:385–9. doi: 10.14503/THIJ-16-5955
15. Huang Y, Tang S, Huang C, Chen J, Li J, Cai A, et al. Circulating miRNA29 family expression. levels in patients with essential hypertension as potential markers for left ventricular hypertrophy. Clin Exp Hypertens. (2017) 39:119–25. doi: 10.1080/10641963.2016.1226889
16. De Rossi G, Matos-Souza JR, Costa e Silva AA, Campos LF, Santos LG, Azevedo ER, et al. Physical activity and improved diastolic function in spinal cord-injured subjects. Med Sci Sports Exerc. (2014) 46:887–92. doi: 10.1249/MSS.0000000000000187
17. Lacchini R, Jacob-Ferreira ALB, Luizon MR, Coeli FB, Izidoro-Toledo TC, Gasparini S, et al. Matrix metalloproteinase 9 gene haplotypes affect left ventricular hypertrophy in hypertensive patients. Clin Chim Acta. (2010) 411:1940–4. doi: 10.1016/j.cca.2010.08.008
18. Lacchini R, Jacob-Ferreira ALB, Luizon MR, Gasparini S, Ferreira-Sae MC, Schreiber R, et al. Common matrix metalloproteinase 2 gene haplotypes may modulate left ventricular remodelling in hypertensive patients. J Hum Hypertens. (2012) 23:171–7. doi: 10.1038/jhh.2011.8
19. Li Y, Kowdley KV. Method for microRNA isolation from clinical sérum samples. Anal Biochem. (2012) 431:69–75. doi: 10.1016/j.ab.2012.09.007
20. Paim LR, Schreiber R, de Rossi G, Matos-Souza JR, Costa E Silva AA, Calegari DR, et al. Circulating microRNAs, vascular risk, and physical activity in spinal cord-injured subjects. J Neurotrauma. (2019) 3 6:845–52. doi: 10.1089/neu.2018.5880
21. Livak KJ, Schmittgen TD. Analysis of relative gene expression data using real-time quantitative PCR and the 2 -ΔΔCT method. Methods. (2001) 25:402–8. doi: 10.1006/meth.2001.1262
22. Dweep H, Sticht C, Pandey P, Gretz N. miRWalk—database: prediction of possible miRNA binding sites by “walking” the genes of three genomes. J Miomed Inform. (2011) 44:839–47. doi: 10.1016/j.jbi.2011.05.002
23. Zhang X, Wang X, Wu J, Peng J, Deng X, Shen Y, et al. The diagnostic values of circulating miRNAs for hypertension and bioinformatics analysis. Biosci Rep. (2018) 38:BSR20180525. doi: 10.1042/BSR20180525
24. White SM, Constantin PE, Claycomb WC. Cardiac physiology at the cellular level: use of cultured HL-1 cardiomyocytes for studies of cardiac muscle cell structure and function. Am J Physiol Heart Circ Physiol. (2004) 286:H823–9. doi: 10.1152/ajpheart.00986.2003
25. Hao L, Ren M, Rong B, Xie F, Lin MJ, Zhao YC, et al. TWEAK/Fn14 mediates atrial-derived HL-1 myocytes hypertrophy via JAK2/STAT3 signalling pathway. J Cell Mol Med. (2018) 22:4344–53. doi: 10.1111/jcmm.13724
26. Chen Y, Qiao X, Zhang L, Li X, Liu Q. Apelin-13 regulates angiotensin ii-induced Cx43 downregulation and autophagy via the AMPK/mTOR signaling pathway in HL-1 cells. Physiol Res. (2020) 69:813–22. doi: 10.33549/physiolres.934488
27. Landstrom AP, Kellen CA, Dixit SS, van Oort RJ, Garbino A, Weisleder N, et al. Junctophilin-2 expression silencing causes cardiocyte hypertrophy and abnormal intracellular calcium handling. Circ Heart Fail. (2011) 4:214–23. doi: 10.1161/CIRCHEARTFAILURE.110.958694
28. Bloch L, Ndongson-Dongmo B, Kusch A, Gragun D, Heller R, Huber O. Real-time monitoring of hypertrophy in HL-1 cardiomyocytes by impedance measurements reveals different modes of growth. Cytotechnology. (2016) 68:1897–907. doi: 10.1007/s10616-016-001-3
29. Claycomb WC, Lanson NA Jr, Stallworth BS, Egeland DB, Delcarpio JB, Bahinski A, et al. HL-1 cells: a cardiac muscle cell line that contracts and retains phenotypic characteristics of the adult cardiomyocyte. Proc Natl Acad Sci USA. (1998) 95:2979–84. doi: 10.1073/pnas.95.6.29.79
30. Lin KH, Kumar VB, Shanmugam T, Shibu MA, Chen RJ, Kuo CH, et al. miR-145-5p targets paxillin to attenuate angiotensin II-induced pathological cardiac hypertrophy via downregulation of Rac 1, pJNK, p-c-Jun, NFATc3, ANP and by Sirt-1 upregulation. Mol Cell Biochem. (2021). doi: 10.1007/s11010-021-04100-w;
31. Ruotian L, Guijun Y, Qun Z, Yue J, Haixiang S, Yali H, et al. miR-145 inhibits isoproterenol-induced cardiomyocyte hypertrophy by targeting the expression and localization of GATA6. FEBS Lett. (2013) 587:1754–61. doi: 10.1016/j.febslet.2013.04.018
32. Xu XD, Song XW Li Q, Wang GK, Jing Q, Qin YW. Attenuation of microRNA-22 derepressed PTEN to effectively protect rat cardiomyocytes from hypertrophy. J Cell Physiol. (2012) 227:1391–8. doi: 10.1002/jcp.22852
33. Sayed D, Hong C, Chen IY, Lypowy J, Abdellatif M. MicroRNAs play na essential role in the development of cardiac hypertrophy. Circ Res. (2007) 100:416–24. doi: 10.1161/01.RES/0000257913.42552.23
34. Kaneto CM, Nascimento JS, Moreira MCR, Ludovico ND, Santana AP, Silva RAA, et al. MicroRNA profiling identifies miR-7-5p and miR-26b-5p as differentially expressed in hypertensive patients with left ventricular hypertrophy. Braz J Med Biol Res. (2017) 50: e6211. doi: 10.1590/1414-431X20176211
35. Yin X, Peng C, Ning W, Li C, Ren Z, Zhang J, et al. miR-30a downregulation aggravates pressure overload-induced cardiomyocyte hypertrophy. Mol Cell Biochem. (2013) 379:1–6. doi: 10.1007/s11010-012-1552-z
36. Bellera N, Barba I, Rodriguez-Sinovas A, Ferret E, Asín MA, Gonzalez-Alujas MT, et al. Single intracoronary injection of encapsulated antagomir-92a promotes angiogenesis and prevents adverse infarct remodeling. J Am Heart Assoc. (2014) 3:e000946. doi: 10.1161/JAHA.114.000946
37. Liang C, Gao L, Liu Y, Liu Y, Yao R, Li Y, et al. Mir-451 antagonist protects against cardiac fibrosis in streptozotocin-induced diabetic mouse heart. Life Sci. (2019) 224:12–22. doi: 10.1016/j.lfs.2019.02.059
38. Tolonen AM, Magga J, Szabó Z, Viitala P, Gao E, Moilanen AM, et al. Inhibition of Let-7 microRNA attenuates myocardial remodeling and improves cardiac function postinfarction in mice. Pharmacol Res Perspect. (2014) 2:e00056. doi: 10.1002/prp2.56
39. Cuspidi C, Sala C, Negri F, Mancia G, Morganti A, Hypertension IS. Prevalence of left-ventricular hypertrophy in hypertension: na updated review of echocardiographic studies. J Hum Hypertens. (2012) 26:343–9. doi: 10.1038/jhh.2011.104
40. Raso A, Dirkx E, Philippen LE, Fernandez-Celis A, De Majo F, Sampaio-Pinto V, et al. Therapeutic delivery of miR-148a suppresses ventricular dilation in heart failure. Mol Ther. (2019) 27:584–99. doi: 10.1016/j.ymthe.2018.11.011
Keywords: hypertension, left ventricular hypertrophy, cardiac hypertrophy, microRNAs, miR-145-5p
Citation: Lopes ECP, Paim LR, Carvalho-Romano LFRS, Marques ER, Minin EOZ, Vegian CFL, Pio-Magalhães JA, Velloso LA, Coelho-Filho OR, Sposito AC, Matos-Souza JR, Nadruz W and Schreiber R (2022) Relationship Between Circulating MicroRNAs and Left Ventricular Hypertrophy in Hypertensive Patients. Front. Cardiovasc. Med. 9:798954. doi: 10.3389/fcvm.2022.798954
Received: 20 October 2021; Accepted: 24 March 2022;
Published: 13 April 2022.
Edited by:
Xiang Xie, First Affiliated Hospital of Xinjiang Medical University, ChinaReviewed by:
Yafeng Li, The Fifth Hospital of Shanxi Medical University, ChinaCopyright © 2022 Lopes, Paim, Carvalho-Romano, Marques, Minin, Vegian, Pio-Magalhães, Velloso, Coelho-Filho, Sposito, Matos-Souza, Nadruz and Schreiber. This is an open-access article distributed under the terms of the Creative Commons Attribution License (CC BY). The use, distribution or reproduction in other forums is permitted, provided the original author(s) and the copyright owner(s) are credited and that the original publication in this journal is cited, in accordance with accepted academic practice. No use, distribution or reproduction is permitted which does not comply with these terms.
*Correspondence: Roberto Schreiber, cm9iZXJ0b3NAdW5pY2FtcC5icg==; Wilson Nadruz, d2lsbmpAZmNtLnVuaWNhbXAuYnI=
Disclaimer: All claims expressed in this article are solely those of the authors and do not necessarily represent those of their affiliated organizations, or those of the publisher, the editors and the reviewers. Any product that may be evaluated in this article or claim that may be made by its manufacturer is not guaranteed or endorsed by the publisher.
Research integrity at Frontiers
Learn more about the work of our research integrity team to safeguard the quality of each article we publish.