- 1Department of Cardiothoracic and Vascular Surgery, German Heart Center Berlin, Berlin, Germany
- 2DZHK (German Centre for Cardiovascular Research), Partner Site Berlin, Berlin, Germany
- 3Charité – Universitätsmedizin Berlin, Berlin, Germany
- 4Eidgenössiche Technische Hochschule Zürich, Department of Health Sciences and Technology, Translational Cardiovascular Technology, Zurich, Switzerland
Background: Surgical ventricular restoration (SVR) leads to functional improvement by volume reduction and restoration of left ventricular (LV) geometry. Our purpose was to refine the planning for SVR using cardiac computed tomography (CCT).
Methods: The possibility to anticipate the postoperative residual LV volume was assessed using CCT in 205 patients undergoing SVR combined with coronary artery bypass grafting (77%), mitral valve repair/replacement (19%) and LV thrombectomy (19%). The potential of CCT to guide the procedure was evaluated. Additionally, the predictive value of CCT characteristics on survival was addressed.
Results: 30-day, 1- and 5-year survival was 92.6, 82.7, and 72.1%, respectively, with a marked reduction of NYHA class III-IV quota after surgery (95.1% vs. 20.5% in the follow-up). Both pre- and postoperative LV end-systolic volume index (LVESVI) were predictive of all defined endpoints according to the following tertiles: preoperative: <74 ml/m2, 74–114 ml/m2 and >114 ml/m2; postoperative: <58 ml/m2, 58–82 ml/m2 and >82 ml/m2. On average, a 50 ml/m2 increase of preoperative LVESVI was associated with a 35% higher hazard of death (p = 0.043). Aneurysms limited to seven antero-apical segments (1–7) were associated with a lower death risk (n = 60, HR 0.52, CI 0.28–0.96, p = 0.038). LVESVI predicted by CCT was found to correlate significantly with effectively achieved LVESVI (r = 0.87 and r = 0.88, respectively, p < 0.0001).
Conclusions: CCT-guided SVR can be performed with good mid-term survival and significant improvement in HF severity. CCT-based assessment of achievable postoperative LV volume helps estimate the probability of therapeutic success in individual patients.
Introduction
Chronic ischemic heart failure (HF) after myocardial infarction (MI) is an important indicator of poor prognosis despite advances in drug and device therapy (8). The development of therapeutic concepts addressing ischemic left ventricular (LV) remodeling due to scar formation is essential to improve survival and amelioration of HF symptoms in this patient cohort.
Surgical ventricular restoration (SVR) of anterior LV aneurysms (9–11) is an established treatment option for LV remodeling after MI. It can be performed, if required, in combination with revascularization and mitral valve (MV) repair/replacement (1, 12, 13). Despite disillusioning reports from the STICH trial (2) with its known methodological limitations (14), other studies report excellent outcomes for acute reverse remodeling following SVR. Essential factors of clinical success are appropriate patient selection (15, 16) combined with adequate surgical volume reduction and reshaping of the LV (3, 17).
The analysis of LV volume, scar localization and extension as well as the amount of geometrical distortion play a key role in the preoperative assessment for SVR. Image guidance and a tailored surgical procedure are of paramount importance for a successful SVR. With its high spatial resolution of up to 0.4 mm and an acceptable time resolution of up to 75 ms, cardiac computed tomography (CCT) enables the analysis of a primarily acquired three-dimensional data set, the detailed examination of the cardiac anatomy and the assessment of the functional and geometrical remodeling of the cardiac chambers, based on exact true volume detection, with very high accuracy (4). Advanced CCT software tools allow for analyzing LV segmental wall motion abnormalities (WMA) and estimating the aneurysm volume.
This study aimed to refine surgical planning for SVR and to investigate factors that allow for predicting whether the therapeutic goals (sufficient volume reduction, sufficient residual volume, improved ventricular geometry) can be successfully achieved using the volumetric, geometric and functional tools of CCT. We analyzed our single-center early and mid-term results and the factors determining survival and improvement in HF symptoms after CCT-guided SVR for anterior LV aneurysms.
Materials and Methods
Study Design
Using a modified Dor technique in most cases, SVR was performed in patients with severe HF symptoms, well-defined antero-septal LV akinesia or dyskinesia and preserved contractility of residual myocardium after anterior MI. The procedure was combined with coronary revascularization, MV repair or replacement and LV thrombectomy, when indicated. Endpoints were 30-day mortality, death from any cause in the follow-up period, implantation of a LV assist device and heart transplantation.
The diagnosis of LV aneurysm was made by echocardiography or angiography and confirmed by CCT. Echocardiography and CCT were repeated during the first postoperative week. The pre- and postoperative data were analyzed and compared retrospectively. Clinical, demographic and procedural data were retrospectively collected.
Study Population
We retrospectively analyzed the data of 205 consecutive patients with coronary artery disease who underwent SVR for anterior LV aneurysms in our hospital between November 2005 and January 2016. All patients had a previous anterior MI with severe LV systolic dysfunction and symptomatic HF.
Written informed consent for surgery was obtained from all patients or their representatives. The study was performed according to the principles of the Declaration of Helsinki and approved by the ethics committee of the Charité – Universitätsmedizin Berlin (EA2/177/20).
Surgical Technique
All SVR procedures were performed through a median sternotomy approach under cardiopulmonary bypass (CPB). After coronary artery bypass grafting (CABG), LV repair and MV repair or replacement were performed. Antero-apical SVR was routinely performed using a modified Dor procedure with several Fontan sutures along the aneurysm perimeter without patch application to exclude the aneurysm and restore LV geometry (9). This technique enables the effective exclusion of scarred area, achieving the required target LV volume and reconstruction of the LV apex. Only few patients (12.2%) with specific local findings, for example a ventricular septal defect after a huge antero-apical MI, required a patch repair.
Intraoperative transesophageal echocardiography was routinely performed to assess the adequacy of LV repair. Mitral surgery was performed in patients with echocardiographically proven MR grade ≥2. All surgeons had access to the complete preoperative CCT assessment.
MSCT Measurements
LV Volumetric Study
CCT measurements have been extensively described previously (5). The data were obtained and analyzed in a single lab by use of the uniform protocols. In brief, contrast-enhanced ECG-triggered cardiac scanning was performed using a first- or second-generation dual-source scanner (Somatom Definition, Somatom Definition Flash, Siemens AG, Erlangen, Germany). To study the anatomy and geometry of the LV, the data set was reconstructed with a slice thickness of 0.75 mm and reconstruction increment of 0.4 mm, from early systole to end diastole in steps of 10%. LV volumes and systolic function were assessed using a dedicated CCT evaluation software (syngo.via Cardiac Function, Siemens AG) and applying a 3D threshold segmentation algorithm. The timepoints of the end diastole and end systole were estimated automatically and adjusted by the investigator if required.
LV end-diastolic and end-systolic volume (LVEDV, LVESV) were obtained (Figure 1) and then indexed to body surface area (LVEDVI/LVESVI). Stroke volume (SV) was calculated by subtracting LVESV from LVEDV; left ventricular ejection fraction (LVEF) was obtained by dividing SV by LVEDV; cardiac output (CO) was calculated as a product of SV and cardiac rate.
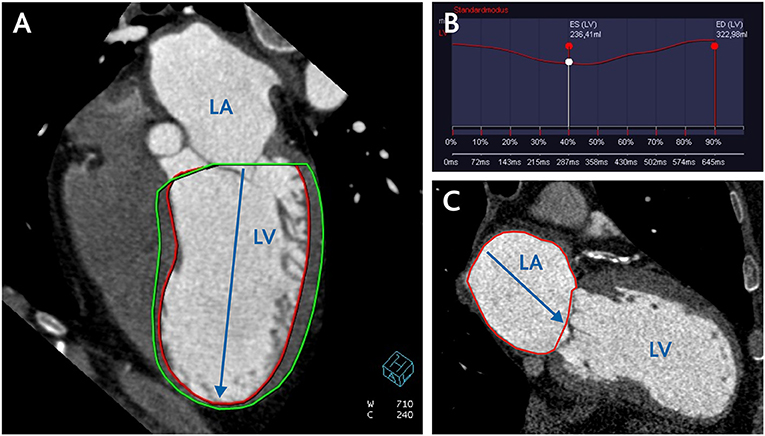
Figure 1. CCT assessment of left ventricular and left atrial volume. (A) CCT reconstruction of four-chamber view for semi-automatic measurement of LV volume and calculation of LV volumetric sphericity index (LVSI) according to the formula LVSI = LV Volume/LV long axis 3 × π/6. (B) filling curve of LV. (C) two-chamber view for measurement of LA area and calculation of LA volume according to the formula (0.85 × A1 × A2)/L. LV, left ventricle; LA, left atrium; A1 and A2, LA area in two- and four-chamber view; L, length of the left atrium.
Assessment of the Anticipated Residual LV Volume and Aneurysm Volume
To separate the aneurysm volume in diastole and systole, a plane determined by three landmarks on the borders of scarred to intact LV myocardium (antero-septal, lateral and inferior) was used. The residual volumes were then assessed using the same CCT evaluation software tool (Figure 2). This enabled the estimation of the anticipated postoperative LVEDV/LVEDVI and LVESV/LVESVI. Aneurysm volume (AnV/AnVI) was then calculated as the subtraction of anticipated from original volumes.
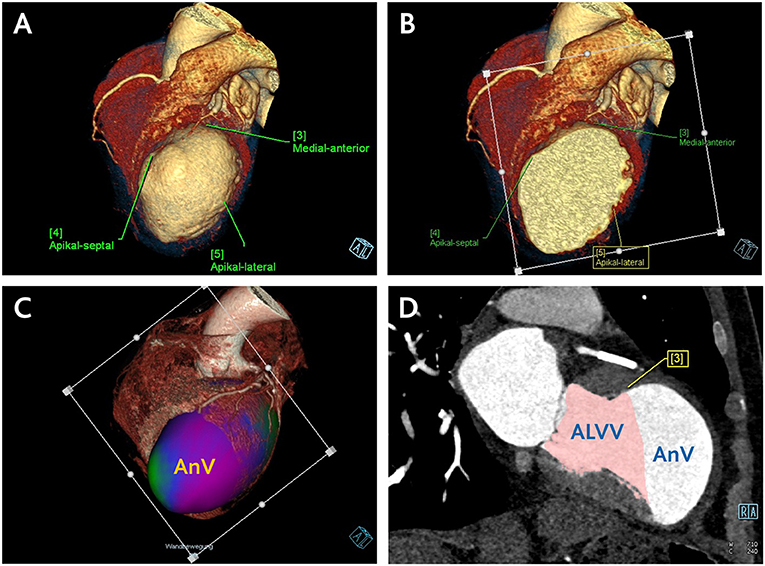
Figure 2. Central Picture. CCT assessment of anticipated residual LV volume and aneurysm volume. (A) Definition of three landmarks on the borders of scarred to intact LV myocardium. (B) Positioning of the plane determined by the defined landmarks. (C) Separation of the aneurysm volume in a three-dimensional data set using the defined plane in the systole and diastole. (D) Estimation of the anticipated postoperative LV volume by means of manually corrected LV borders along the defined plane using the same CCT volumetric software tool. Subsequent calculation of the aneurysm volume through subtraction of anticipated from original LV volume. ALVV, anticipated left ventricular volume; AnV, aneurysm volume.
Analysis of LV Segmental WMA
Local WMA were estimated semiquantitatively using a 17-segment model with separate assessment of papillary muscles. Akinetic or dyskinetic segments involved in an aneurysm or scar formation of other localizations were detected.
Automated cluster analysis was performed to establish the different scar patterns. The identified clusters were then tested for their influence on survival after SVR.
Patients were divided into defined subgroups according to their LVA localization and extension: antero-apical LVA (segments 7, 8, 13, 14, 15, 16, 17)—subgroup1; antero-apical and basal septal or basal anterior LVA (plus segments 1 or 9)—subgroup 2; antero-apical LVA and additional scar of any other localization—subgroup 3.
Morphological characteristics of the aneurysm in terms of myocardial disruption, apposition of thrombotic masses and pericardial effusion were also evaluated.
LV Volumetric Sphericity Index
The systolic and diastolic LV volumetric sphericity index (LVSI) was calculated on the basis of end-diastolic and end-systolic LV volume and LV long axis length in two-chamber view according to the empiric formula (7) (Figure 1),
Left Atrium Volume
The left atrium (LA) volume was calculated at the end systole on the basis of planimetric measured LA area in two-chamber view (A1) and four-chamber view (A2) and LA length (L) according to the simplified empiric formula (6) (Figure 1),
Follow-Up Data Collection
Follow-up was performed in 94.6% of the cases, either during a routine clinical evaluation in our outpatient department or by telephone contact using the short form Health Survey (SF-12) (18). In patients, in whom the follow-up was not possible, national death registry check was performed. Mean follow-up time in the overall population was 1,600 ± 1,106-days. Median follow-up time was 1,528-days.
Statistical Analysis
Continuous variables are presented as mean ± SD. Parametric and non-parametric tests were used where required. For parametric variables, means were compared with the paired, two-tailed student t-test. Categorical variables are presented as numbers with percentages and compared using chi-square tests. Actuarial survival curves were produced using the Kaplan-Meier analysis with a long-rank test. For the evaluation of survival differences dependent on LV volumetric parameters, the patient population was subgrouped according to tertiles of LVESVI with further application of survival analysis. A cluster analysis of scarred ventricle segments detected in CCT was performed using nearest neighbor algorithm to reveal patterns of segmental wall motion abnormalities associated with better or worse survival.
Cox proportional hazard regression modeling was applied, and both univariable and multivariable hazard ratios were calculated to assess independent predictive factors of combined endpoint of all-cause mortality, implantation of a LV assist device and heart transplantation. A p-value <0.05 was considered statistically significant. The data were analyzed with SPSS 23 (SPSS, Chicago, IL, USA).
Results
Preoperative patient characteristics are presented in Table 1. There were 10 (4.9%) patients in New York Heart Association (NYHA) class II of HF, 179 (87.3%) in class III and 16 in class IV (7.8%); the mean NYHA class was 3.02 ± 0.35. The median preoperative LVEF, estimated via echocardiography, was 30% (11–70). 36 (17.6%) patients had a concomitant MR of grade ≥2.
A modified Dor procedure without patch application was performed in 180 patients (87.8%). while 25 patients underwent SVR with patch. One hundred and fifty eight patients (77.1%) had associated myocardial revascularization. Thirty nine patients (19%) underwent concomitant MV surgery (MV repair in 35 and MV replacement in four patients). LV thrombectomy was performed in 39 patients (19%).
Fifteen patients (7.3%) died in hospital within 30-days due to multiorgan failure (n = 9), septic shock (n = 4), electromechanical dissociation (n = 1) or stroke (n = 1). The operative and postoperative data are summarized in Table 1.
Early and Late Outcomes
The median survival period was 51.1 months (range between 1-day and 121.4 months) and the mean survival time was 51.6 ± 37.8 months. Overall, 30-day mortality was 7.3% (n = 15). One- and 5-year survival was 82.7 and 72.1%, respectively (Figure 3).
NYHA Class Change in Survivors
During follow-up we observed a remarkable improvement in HF symptoms after SVR, with a positive redistribution of NYHA class in the whole population in the sense of a strong reduction of NYHA class III-IV quota after surgery in survivors (from 95.1% preoperatively to 20.5% in the early follow-up, p < 0.001) (Figure 4).
Predictive Factors of 30-Day Mortality
Hospital and 30-day mortality were identical in our series. Thirteen of the 15 patients who died in hospital underwent combined SVR and CABG surgery, six underwent combined SVR, CABG and valvular surgery, and 10 required an intra-aortic balloon pump (IABP). Univariate logistic regression revealed factors potentially predictive of 30-day mortality: long CPB time and cross-clamp time, requirement of IABP or other temporary mechanical circulatory support systems, urgent surgery, postoperative sepsis and renal failure contributed to the higher 30-day mortality rate (Supplementary Table 1). Multivariate logistic regression showed that CPB time (p < 0.001) and postoperative LVEF (p < 0.001) were independent predictive factors of 30-day mortality. CPB time longer than 163 min was associated with an adverse outcome (sensitivity 0.765, specificity 0.789, AUC=0.890). Severity of preoperative HF, preoperative LVEF and CCT LV volumetric parameters did not play a substantial role in 30-day mortality.
Late Mortality and Survival Determinants
Univariate logistic regression showed a potential predictive role in all-cause 5-year mortality of such factors as diabetes mellitus, peripheral artery disease, renal failure and atrial fibrillation. Preoperative MR ≥2+, preoperative LAVI, SVI, CI and postoperative LVEDVI, LVESVI and LAVI also had a potential predictive role in all-cause mortality (Supplementary Table 2). The multivariate regression analysis revealed that preoperative LVEDD and preoperative LAVI as well as patient age, presence of diabetes mellitus and renal failure were independent significant predictive factors of all adverse outcomes (Table 2).
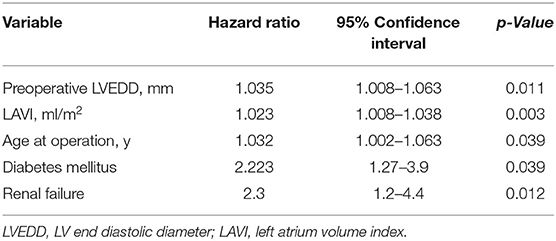
Table 2. Multivariate logistic regression analysis for an adverse outcome (all-cause death, ventricular assist device or heart transplantation).
There was no difference in survival between men and women as shown by the Kaplan-Meier analysis (p = 0.593) and by the univariate regression analysis (male sex HR = 0.87; 95%CI 0.52–1.45).
Changes in LV Geometry and Function
The changes in LV geometric and functional parameters measured by echocardiography and CCT after SVR are presented in Table 3.
LV volumetric parameters measured in CCT in 160 patients were significantly reduced after SVR: LVEDVI decreased about 33.0 ± 17.0% (p < 0.001) and LVESVI about 40.8 ± 21.5% (p < 0.001). LVEF measured in CCT increased significantly from 34.1 ± 12.1% to 43.1 ± 13.9% (p < 0.001) after SVR. The diastolic LV SI increased from 0.40 ± 0.10 to 0.52 ± 0.18 (p < 0.001); the systolic LV SI increased from 0.31 ± 0.10 to 0.36 ± 0.17 (p < 0.001).
Survival Stratified by Preoperative LVESVI and Postoperative LVESVI
Survival stratification according to tertiles of preoperative LVESVI revealed a significantly higher survival rate in the lower tertile group (≤74 ml/m2) than in the groups with a LVESVI of 74–114 ml/m2 and ≥114 ml/m2 (p = 0.048). Five-year survival in the group with LVESVI ≤74 ml/m2 was 83.4% compared to 67.3 and 64.3% in the two other groups. An average 50 ml increase of preoperative LVESVI was associated with a 35% increase of the hazard of death (p = 0.043) (Figure 5A).
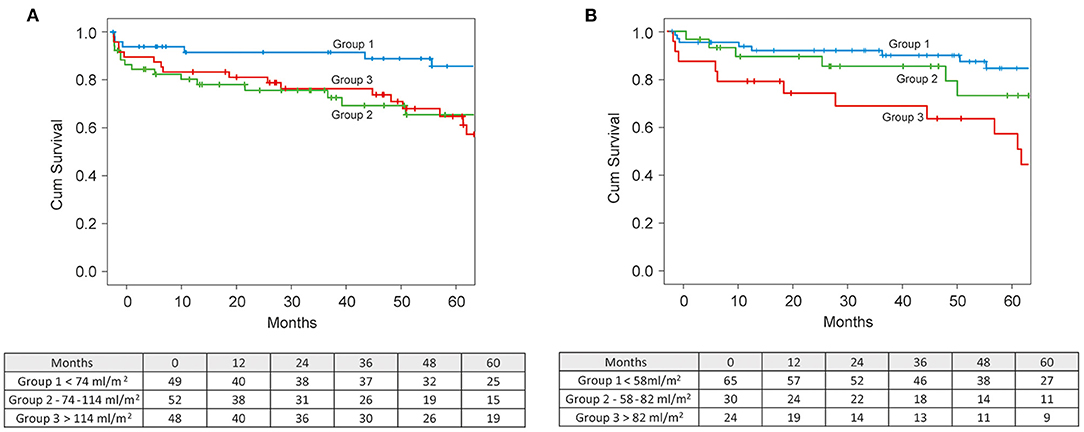
Figure 5. (A) Kaplan-Meier survival curves stratified by preoperative LVESVI. (B) Kaplan-Meier survival curves stratified by achieved postoperative LVESVI.
Survival stratification according to tertiles of the postoperative LVESVI demonstrated a significant difference in survival between tertiles (p = 0.016), with the lowest survival rate of 60.2% in the highest tertile of postoperative LVESVI (>66.2 ml/m2) compared to the survival rates of 84.6 and 85.3% in the middle (41.6–66.2 ml/m2) and lowest (<41.6 ml/m2) teriles, respectively (Figure 5B).
Correlation Between Anticipated and Achieved Residual LV Volume and Estimated Aneurysm Volume
We observed a significant correlation between anticipated and effectively achieved postoperative LVEDV and LVESV (r = 0.903 and r = 0.904, respectively, p < 0.0001), and their indexed values (r = 0.87 and r = 0.88, respectively, p < 0.0001) (Figure 6). Anticipated LVEDVI was only 7.2 ± 18.0 ml/m2 higher than achieved LVEDVI (p = 0.00002), and anticipated LVESVI was only 2.3 ± 16.1 ml/m2 higher than achieved LVESVI (p = 0.109) (Table 4).
Mean estimated diastolic and systolic AnV were 88.5 ± 54.2 ml and 80.5 ± 56.4 ml, respectively. The relation of the estimated AnV to the actual preoperative LVEDV and LVESV was 28.9 ± 11.3% and 37.8 ± 14.7%, respectively. This relation closely matched with the effectively postoperative achieved relation of excluded part of LV volume to the whole LV volume—LVEDV by 33.1 ± 1.6% and LVESV by 41.2 ± 21.7%.
Analysis of LV Segmental WMA
Aneurysms limited to seven antero-apical segments (1–7)—group 1—were associated with a lower death risk (n = 60, HR 0.52, CI 0.28–0.96, p = 0.038). The Kaplan-Meier analysis showed significantly better survival in these patients compared to all other patients (p = 0.035)—group 2 and 3—with a 5-year survival rate of 84.7% (95% CI 71.3–92.1) vs. 64% (95% CI 50.8–74.6) (Table 5).
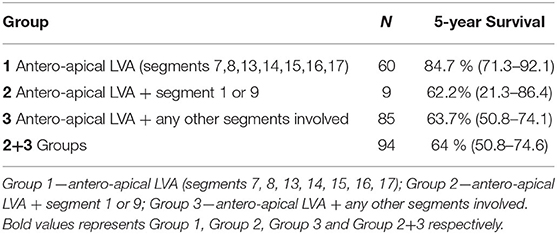
Table 5. Five-year survival rates in defined subgroups of patients according to their LVA localization and extension.
Furthermore, an automated cluster analysis based on the frequency of segment involvement and its impact on survival after SVR allowed for identifying the similar scar pattern (Cluster 1) with a significantly better outcome (Figure 7) compared to all other patients with HR 0.491 (0.263–0.916, p = 0.025) and a 5-year survival rate of 82.2% vs. 63.7% in all other patients (p = 0.022).
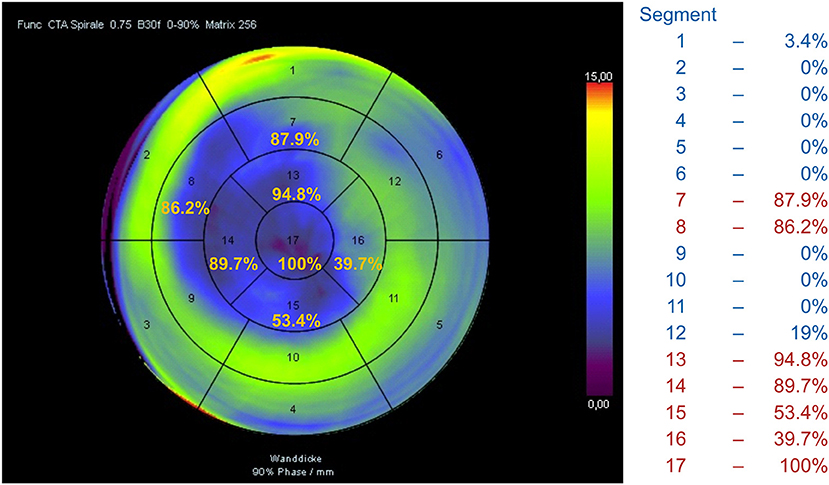
Figure 7. Automated cluster analysis based on the frequency of segment involvement allowed for identifying the scar pattern (Cluster 1) with a significantly better outcome compared to all other patients.
Left Atrium Volume
LAVI decreased significantly from 60.7 ± 19.2 ml/m2 to 50.6 ± 18.7 ml/m2 (p < 0.001). Dividing by tertiles of preoperative LAVI revealed that patients in the lowest tertile (<49.5 ml/m2) had a lower risk of death than patients in the middle (49.5–67.6 ml/m2) and in the highest tertile (>67.6 ml/m2) of LAVI (long rank p = 0.005), with a 5-year survival in patients with LAVI <49.5 ml/m2 of 83.4% compared to the survival rate of patients in the middle and highest tertiles of 71.6 and 60.8%, respectively.
Mitral Regurgitation
MR was reduced after surgery from 1.02 ± 0.80 to 0.36 ± 0.45 (p < 0.001) in the whole population and from 0.79 ± 0.56 to 0.37 ± 0.44 (p < 0.001) in patients without concomitant MV surgery.
Role of LVSI
Both systolic and diastolic SI increased significantly in all patients after SVR (Table 3) due to a substantial shortening of the LV long axis in relation to the LV volume reduction. However, the multivariate regression analysis (χ2=1.7, p = 0.428) provided no evidence for an effect of pre-operative diastolic SI on survival.
Discussion
This study is based on our experience of SVR performed between 2005 and 2016, since the modified Dor procedure was implemented as a standard technique in our institution.
The main results of the present analysis are that both CCT-derived preoperative and postoperative LVESVI are predictive for mid-term survival. The possibility to predict the volume reduction following SVR through the separation of the aneurysm volume using a commercially available CCT evaluation software tool allows for better therapy planning and patient selection. The CCT-based analysis of local WMA provides additional information for risk assessment: aneurysms limited to seven antero-apical segments were associated with a lower risk of mortality. The follow-up analysis shows a remarkable positive improvement in NYHA class after surgery as evidence for an improvement in HF symptoms.
Diagnostic Testing
Accurate assessment of the LV geometrical distortion, aneurysm localization and extension, and viability of the remaining myocardium is decisive for a successful SVR (19, 20). The most advanced imaging modality with excellent standardized approaches for these purposes is cardiac magnetic resonance imaging (MRI) (19); however, an increasing number of patients with ischemic cardiomyopathy become carriers of implantable devices (in our cohort up to 40%), limiting the applicability of MRI.
Despite excellent spatial and temporal resolution, echocardiography depends highly on exact geometric alignment, patient anatomical characteristics and the operator's skills, and it often has limitations in the visualization of the apical region. Furthermore, 2D echocardiography only allows for calculating the ventricle volume based on the empiric approximated formula, and 3D real-time volume detection is not widespread or sufficiently validated. In this study we complementarily employed echocardiography to evaluate the LV diameters, systolic function and severity of MR. The profound analysis of further echocardiographic data in the represented patient cohort, especially the prognostic role of speckle-tracking echocardiography (LV global longitudinal and basal longitudinal strain), is presented separately (21).
Our reasons to use CCT for SVR planning were the possibility of exact true volume detection and geometrical analysis in primarily acquired three-dimensional data sets combined with a short examination time and lack of technical restrictions even in critical patients. Previous studies (4, 22) demonstrated that CCT is a valuable tool to evaluate LV and MV geometry and function. In our previous study (5) we showed that CCT data enable the precise analysis of LV volume, geometry and local WMA as well as the reliable recognition of aneurysm borders, thrombotic apposition and presence of LV pseudoaneurysm. This study focuses on prognostic important volumetric and functional characteristics and on the validation of the CCT-based separation of the aneurysm volume from the residual LV volume and thereby on improvement of the therapy targeting, with the further aim to avoid the restrictive residual LV volume.
However, we are well aware that CCT has clear limitations in detecting scar transmurality and viability of the remaining myocardium compared to MRI.
Thirty-Day Mortality
In our series the overall 30-day mortality was 7.3%. Dor et al. (19) found hospital mortality rates of 8.1 and 4.8% in early and late series of patients who underwent SVR. Garatti et al. (23) reported a 30-day mortality of 8.3% in patients after SVR for anterior aneurysm. Jeganathan et al. (13) reported a hospital mortality of 13.3% in patients who underwent combined SVR and MV surgery. Their group identified NYHA class IV symptoms, preoperative atrial fibrillation, previous cardiac surgery and presence of ischemic MR as significant risk factors for increased hospital mortality. In our study, multivariate logistic regression showed that CPB time (p < 0.001) and postoperative LV EF (p < 0.001) but not the severity of preoperative HF, preoperative LV EF or CCT LV volume were independent predictive factors of 30-day mortality.
Late Mortality and Survival Determinants
In our study population, 1- and 5-year actuarial survival rates were 82.7 and 72.1%, which is comparable to most published studies. A multivariate regression analysis revealed that preoperative LVEDD and LAVI as well as the patient's age, presence of diabetes mellitus and renal failure were independent significant predictive factors of all adverse outcomes (Table 2).
Efficient LV volume reduction toward a physiological range of a LVESVI of <60–70 ml/m2 is essential to improve survival after surgical repair. Di Donato et al. (7) showed that a postoperative LVESVI of >60 ml/m2 is an independent predictor of mortality. The analysis of the STICH trial data (2) showed that a statistically significant reduction in mortality was achieved only in patients attaining an LVESVI of <70 ml/m2. In our study group, both the preoperative and the postoperative LVESVI had a strong effect on survival. A cut-off point of ≤74 ml/m2 for preoperative LVESVI revealed the best 5-year survival rate of 86% (Figure 5A). An average 50 ml increase of preoperative LVESVI was associated with a 35% increase of the hazard of death (p = 0.043). A postoperative LVESVI of ≥82 ml/m2 was strongly predictive of all-cause mortality, with a lowest 5-year survival rate of 49% (Figure 5B).
The other important task is to avoid the restrictive residual LV volume after surgery. There were five patients with LVESVI <20 ml/m2 after surgery in our series, only one of them showed no improvement in HF symptoms during follow-up.
In this context, the possibility to predict the volume reduction using CCT volumetric tools is extremely valuable for better therapy planning and appropriate patient selection.
A CCT-based analysis of LV segmental WMA yielded additional valuable findings; it showed that the scar pattern typically involving seven antero-apical segments is associated with a better 5-year survival rate of 82.2% compared to 63.7% in all other patients with more segments involved (p = 0.022).
We observed a significant reduction of LAVI as immediate effect of SVR, probably through the improvement in LV diastolic function after SVR and/or in correlation with the reduction of MR, both in the whole population and in patients who underwent SVR only (Table 2). Preoperative LAVI was identified as an independent significant predictive factor of all adverse outcomes with a 5-year survival in patients with LAVI <49.5 ml/m2 of 83.4%, compared to a survival rate of 71.6 and 60.8% in patients in the middle and highest tertiles, respectively.
In our series both diastolic and systolic LVSI increased immediately after surgery due to a substantial shortening of the LV long axis in relation to LV volume reduction, but it is not clear whether this effect is necessarily negative. In the follow-up study of the STICH trial, Choi et al. (17) also reported a postoperative increase of the SI in the CABG+SVR group and an association of higher baseline SI with poorer postoperative survival both in CABG and CABG+SVR groups. In our series, multivariate proportional hazards regression modeling (χ2 = 1.7, p = 0.428) revealed no evidence for an effect of pre-operative diastolic SI on survival.
Study Limitations
First, the study is subject to the usual limitations inherent to a retrospective analysis of prospectively collected data sets. Second, CCT imaging data were not available in all patients. Third, postoperative CCT data were obtained only several days after surgery and documented the immediate effects of SVR on LV volumes and function. However, they do not reflect the effects of subsequent ventricular reverse remodeling. Fourth, CCT-based assessment of local WMA as semiquantitative detection of akinetic or dyskinetic myocardial segments involved in an aneurysm or scar formation of other localizations is dependent on the operator's skills and only offers rough scar detection. Due to the high radiation burden, we did not consider the use of CCT-based viability assessment. The CCT-based strain analysis has clear limitations because of the low temporal resolution. The analysis of systolic and diastolic LV function, myocardial dyssynergy and viability based on a two-dimensional speckle-tracking echocardiography is not included in this study. Fifth, the provided CCT-based procedure to separate the aneurysm volume using a plane determined by three landmarks on the borders of scarred to intact LV myocardium and subsequent estimation of residual volumes using the commercially available CCT volumetric tool only allows for an approximate estimation of the possible volume reduction and does not incorporate the modeling of the surgical procedure in the sense of the creation of a neoapex.
Conclusions
The results of this study suggest that CCT-guided SVR can be performed with good mid-term survival and significant relief of HF symptoms due to LV volume reduction, reverse remodeling and functional improvement. The modified Dor procedure is an adequate surgical approach to achieve the therapeutic goals of SVR. CCT as an alternative modality to MRI can deliver relevant data for surgical planning as well as important predictive parameters for adverse outcome. The provided possibility to predict the achievable volume reduction through the separation of the aneurysm volume using a commercially available CCT volumetric tool allows for better therapy planning and patient selection.
Data Availability Statement
The data analyzed in this study is subject to the following licenses/restrictions: anonymized data. Requests to access these datasets should be directed to Natalia Solowjowa, c29sb3dqb3dhQGRoemIuZGU=.
Ethics Statement
The study was performed according to the principles of the Declaration of Helsinki and approved by the ethics committee of the Charité – Universitätsmedizin Berlin (EA2/177/20). Written informed consent for surgery was obtained from all patients or their representatives.
Author Contributions
NS performed the MSCT examinations, designed the study, analyzed and interpreted the data, and wrote the manuscript. ON and YH analyzed and interpreted the data and helped writing the manuscript. AM helped design the study, analyzed and interpreted the data, and helped writing the manuscript. FH and VF performed surgery and revised the manuscript critically for important intellectual content. CK performed surgery, conceived and designed the study, analyzed and interpreted the data, and wrote the manuscript. All authors contributed to the article and approved the submitted version.
Funding
This work was supported by the DZHK (German Centre for Cardiovascular Research) and the BMBF (German Ministry of Education and Research).
Conflict of Interest
VF disclosed financial relationships with the following entities: Medtronic GmbH, Biotronik SE & Co, Abbott GmbH & Co KG, Boston Scientific, Edwards Lifesciences, Berlin Heart, Novartis Pharma GmbH, JOTEC GmbH, and Zurich Heart.
The remaining authors declare that the research was conducted in the absence of any commercial or financial relationships that could be construed as a potential conflict of interest.
Publisher's Note
All claims expressed in this article are solely those of the authors and do not necessarily represent those of their affiliated organizations, or those of the publisher, the editors and the reviewers. Any product that may be evaluated in this article, or claim that may be made by its manufacturer, is not guaranteed or endorsed by the publisher.
Acknowledgments
We thank Hannah Laumann for editorial assistance and Helge Haselbach as well as Nadja Kugler for graphics.
Supplementary Material
The Supplementary Material for this article can be found online at: https://www.frontiersin.org/articles/10.3389/fcvm.2022.763073/full#supplementary-material
References
1. Prucz RB, Weiss ES, Patel ND, Nwakanma LU, Baumgartner WA, Conte JV. Coronary artery bypass grafting with or without surgical ventricular restoration: a comparison. Ann Thorac Surg. (2008) 86:806–14; discussion −14. doi: 10.1016/j.athoracsur.2008.05.009
2. Michler RE, Rouleau JL, Al-Khalidi HR, Bonow RO, Pellikka PA, Pohost GM, et al. Insights from the stich trial: change in left ventricular size after coronary artery bypass grafting with and without surgical ventricular reconstruction. J Thorac Cardiovasc Surg. (2013) 146:1139–45 e6. doi: 10.1016/j.jtcvs.2012.09.007
3. Menicanti LA. Reduce or reshape, this is the question! Eur J Cardiothorac Surg. (2015) 47:480–1. doi: 10.1093/ejcts/ezu254
4. Asferg C, Usinger L, Kristensen TS, Abdulla J. Accuracy of multi-slice computed tomography for measurement of left ventricular ejection fraction compared with cardiac magnetic resonance imaging and two-dimensional transthoracic echocardiography: a systematic review and meta-analysis. Eur J Radiol. (2012) 81:e757–62. doi: 10.1016/j.ejrad.2012.02.002
5. Solowjowa N, Penkalla A, Dandel M, Novikov A, Pasic M, Weng Y, et al. Multislice computed tomography-guided surgical repair of acquired posterior left ventricular aneurysms: demonstration of mitral valve and left ventricular reverse remodelling. Interact Cardiovasc Thorac Surg. (2016) 23:383–90. doi: 10.1093/icvts/ivw137
6. Lester SJ, Ryan EW, Schiller NB, Foster E. Best method in clinical practice and in research studies to determine left atrial size. Am J Cardiol. (1999) 84:829–32. doi: 10.1016/S0002-9149(99)00446-4
7. Di Donato M, Castelvecchio S, Menicanti L. End-systolic volume following surgical ventricular reconstruction impacts survival in patients with ischaemic dilated cardiomyopathy. Eur J Heart Fail. (2010) 12:375–81. doi: 10.1093/eurjhf/hfq020
8. Ponikowski P, Voors AA, Anker SD, Bueno H, Cleland JGF, Coats AJS, et al. ESC scientific document group 2016 esc guidelines for the diagnosis and treatment of acute and chronic heart failure: The task force for the diagnosis and treatment of acute and chronic heart failure of the european society of cardiology (ESC) developed with the special contribution of the Heart Failure Association (HFA) of the ESC. Eur Heart J. (2016) 37:2129–200. doi: 10.1093/eurheartj/ehw128
9. Dor V, Civaia F, Alexandrescu C, Sabatier M, Montiglio F. Favorable effects of left ventricular reconstruction in patients excluded from the surgical treatments for ischemic heart failure (stich) trial. J Thorac Cardiovasc Surg. (2011) 141:905–16:16 e1–4. doi: 10.1016/j.jtcvs.2010.10.026
10. Dor V, Saab M, Coste P, Kornaszewska M, Montiglio F. Left ventricular aneurysm: a new surgical approach. J Thorac Cardiovasc Surg. (1989) 37:11–9. doi: 10.1055/s-2007-1013899
11. Jatene AD. Left ventricular aneurysmectomy. Resection or reconstruction. J Thorac Cardiovasc Surg. (1985) 89:321–31. doi: 10.1016/S0022-5223(19)38781-1
12. Castelvecchio S, Parolari A, Garatti A, Gagliardotto P, Mossuto E, Canciani A, et al. Surgical ventricular restoration plus mitral valve repair in patients with ischaemic heart failure: risk factors for early and mid-term outcomesdagger. Eur J Cardiothorac Surg. (2016) 49:e72–8; discussion e8–9. doi: 10.1093/ejcts/ezv478
13. Jeganathan R, Maganti M, Badiwala MV, Rao V. Concomitant mitral valve surgery in patients undergoing surgical ventricular reconstruction for ischaemic cardiomyopathy. Eur J Cardiothorac Surg. (2013) 43:1000–5. doi: 10.1093/ejcts/ezs499
14. Buckberg GD. Surgical ventricular restoration after flawed stich trial: results when guidelines followed. Eur J Cardiothorac Surg. (2016) 50:702–3. doi: 10.1093/ejcts/ezw133
15. Calafiore AM, Iaco AL, Kheirallah H, Sheikh AA, Al Sayed H, El Rasheed M, et al. Outcome of left ventricular surgical remodelling after the stich trial. Eur J Cardiothorac Surg. (2016) 50:693–701. doi: 10.1093/ejcts/ezw103
16. Castelvecchio S, Menicanti L, Ranucci M. Development and validation of a risk score for predicting operative mortality in heart failure patients undergoing surgical ventricular reconstruction. Eur J Cardiothorac Surg. (2015) 47:e199–205. doi: 10.1093/ejcts/ezv023
17. Choi JO, Daly RC, Lin G, Lahr BD, Wiste HJ, Beaver TM, et al. Impact of surgical ventricular reconstruction on sphericity index in patients with ischaemic cardiomyopathy: follow-up from the stich trial. Eur J Heart Fail. (2015) 17:453–63. doi: 10.1002/ejhf.256
18. Ware J, Kosinski M, Keller S. Sf-12: How to Score the sf-12 Physical and Mental Health Summary Scales. Boston, MA: The Health Institute, New England Medical Center (1995).
19. Dor V, Sabatier M, Montiglio F, Civaia F, DiDonato M. Endoventricular patch reconstruction of ischemic failing ventricle. A single center with 20 years experience. Advantages of magnetic resonance imaging assessment. Heart Fail Rev. (2004) 9:269–86. doi: 10.1007/s10741-005-6804-5
20. Schuster A, Morton G, Chiribiri A, Perera D, Vanoverschelde JL, Nagel E. Imaging in the management of ischemic cardiomyopathy: special focus on magnetic resonance. J Am Coll Cardiol. (2012) 59:359–70. doi: 10.1016/j.jacc.2011.08.076
21. Nemchyna O, Solowjowa N, Dandel M, Hrytsyna Y, Knierim J, Schoenrath F et al. Predictive value of two-dimensional speckle-tracking echocardiography in patients undergoing surgical ventricular restoration. Front Cardio Med. (2022) 9:824467.
22. Debonnaire P, Palmen M, Marsan NA, Delgado V. Contemporary imaging of normal mitral valve anatomy and function. Curr Opin Cardiol. (2012) 27:455–64. doi: 10.1097/HCO.0b013e328354d7b5
Keywords: heart failure, myocardial infarction, aneurysm, imaging—computed tomography, cardiac computed tomography, surgical ventricular reconstruction
Citation: Solowjowa N, Nemchyna O, Hrytsyna Y, Meyer A, Hennig F, Falk V and Knosalla C (2022) Surgical Restoration of Antero-Apical Left Ventricular Aneurysms: Cardiac Computed Tomography for Therapy Planning. Front. Cardiovasc. Med. 9:763073. doi: 10.3389/fcvm.2022.763073
Received: 23 August 2021; Accepted: 21 February 2022;
Published: 28 March 2022.
Edited by:
Zahra K. Motamed, McMaster University, CanadaReviewed by:
Filippo Cademartiri, Gabriele Monasterio Tuscany Foundation (CNR), ItalyPatrick Klein, St. Antonius Hospital, Netherlands
Copyright © 2022 Solowjowa, Nemchyna, Hrytsyna, Meyer, Hennig, Falk and Knosalla. This is an open-access article distributed under the terms of the Creative Commons Attribution License (CC BY). The use, distribution or reproduction in other forums is permitted, provided the original author(s) and the copyright owner(s) are credited and that the original publication in this journal is cited, in accordance with accepted academic practice. No use, distribution or reproduction is permitted which does not comply with these terms.
*Correspondence: Christoph Knosalla, a25vc2FsbGFAZGh6Yi5kZQ==