- 1Institute of Cardiovascular Disease Research, Xuzhou Medical University, Xuzhou, China
- 2Department of Cardiology, The Affiliated Hospital of Xuzhou Medical University, Xuzhou, China
Background: At present, effective clinical therapies for myocardial ischemia-reperfusion injury (MIRI) are lacking. We investigated if luteolin conferred cardioprotective effects against MIRI and elucidated the potential underlying mechanisms.
Method: Four databases were searched for preclinical studies of luteolin for the treatment of MIRI. The primary outcomes were myocardial infarct size (IS) and intracardiac hemodynamics. The second outcomes were representative indicators of apoptosis, oxidative stress, and inflammatory. The Stata and RevMan software packages were utilized for data analysis.
Results: Luteolin administration was confirmed to reduce IS and ameliorate hemodynamics as compared to the control groups (p < 0.01). IS had decreased by 2.50%, 2.14%, 2.54% in three subgroups. Amelioration of hemodynamics was apparent in two different myocardial infarct models (model of left anterior descending branch ligation and model of global heart ischemia), as left ventricular systolic pressure improved by 21.62 and 35.40 mmHg respectively, left ventricular end-diastolic pressure decreased by 7.79 and 4.73 mmHg respectively, maximum rate of left ventricular pressure rise increased by 737.48 and 750.47 mmHg/s respectively, and maximum rate of left ventricular pressure decrease increased by 605.66 and 790.64 mmHg/s respectively. Apoptosis of cardiomyocytes also significantly decreased, as indicated by thelevels of MDA, an oxidative stress product, and expression of the inflammatory factor TNF-α (p < 0.001).
Conclusion: Pooling of the data demonstrated that luteolin exerts cardioprotective effects against MIRI through different signaling pathways. As possible mechanisms, luteolin exerts anti-apoptosis, anti-oxidation, and anti-inflammation effects against MIRI.
Introduction
Myocardial infarction is an acute heart condition characterized by decreased or complete cessation of blood flow to a portion of the myocardium, resulting in an imbalance between the supply and demand of oxygen to the myocardium and subsequent death of myocardiocytes (1, 2). Ultrastructural changes and mitochondrial abnormalities of cardiomyocytes are identified as early as 10 min after arterial occlusion (3, 4). However, cardiomyocyte necrosis can be detected hours later (5). The results of clinical studies have shown that appropriate and timely myocardial reperfusion therapy can effectively reduce ischemic injury (6, 7). Despite numerous benefits following recanalization of the coronary artery, postoperative mortality and morbidity remain significant (8). Moreover, the course of myocardial reperfusion itself can exacerbate myocardial systolic and diastolic function and expand myocardial infarct size (IS)–a phenomenon known as myocardial ischemia-reperfusion injury (MIRI) (9). Experimental studies have suggested that ~50% of the final IS is due to MIRI-induced cell death (10). From the emergence of this phenomenon, massive experimental studies of cardioprotective strategies against MIRI have been conducted (11). However, turning the application of these sorts of laboratory discoveries into treatments to improve patient outcomes have encountered significant obstacles primarily because of the multiple multi-factorial mechanisms underlying MIRI-induced cardiomyocyte injury (12). Hence, there is a need to assess the cardioprotective effects of potential strategies and elucidate the underlying mechanisms.
Although the herbs used in Traditional Chinese Medicine offer massive untapped potential for use in modern medicine, the underlying mechanisms remain unclear (13). Growing evidence suggests that dietary intake of flavonoids can reduce the incidence of ischemic heart disease (14). Luteolin, a 3', 4', 5, 7 tetra hydroxyl flavonoid derived from various plant sources, including broccoli, green pepper, and even peanut hulls, possess anti-apoptosis, antioxidant, anti-inflammatory, anti-tumor, and metabolic adjustment properties (15–18). So far, a variety of mechanisms of luteolin against MIRI have been identified. To accelerate the translation of cardioprotective effects of luteolin to clinical research, the empirical evidence and possible mechanisms of luteolin are summarized in this report.
Research on luteolin has been limited to preclinical trials, as most findings have been obtained from animal studies. However, the use of animal models has inherent flaws. For example, animal models generally only imitate a specific disease and cannot be implemented in adult animals without the induction of some comorbidities. Also, animal models are insufficient to reproduce the complicated pathophysiology in older adults with additional risk factors of myocardial infarction due to intrinsic heterogeneity. In addition, the conclusions of animal experiments are generally obtained from relatively small independent samples. Nonetheless, although animal studies are still necessary prior to preclinical studies, a well-designed quantitative meta-analysis with appropriate inclusion criteria can provide convincing evidence while minimizing bias. Hence, the aim of this review article was to summarize current knowledge of the cardioprotective effects of luteolin for treatment of MIRI.
Methods
Search Strategy
Relevant articles published up to February 15, 2022 were retrieved from the PubMed, Embase, Cochrane Library, and Web of Sciencedatabases using the key words “luteolin” and “myocardial ischemia” without limitations to the year of publication, article type, or species. The PubMed database was searched with the use of the following retrieval statement: {[(Myocardial Ischemia) OR (Myocardial Ischemias) OR (Ischemias, Myocardial) OR (Ischemia, Myocardial) OR (Heart Disease, Ischemic) OR (Ischemic Heart Disease) OR (Heart Diseases, Ischemic) OR (Diseases, Ischemic Heart) OR (Disease, Ischemic Heart) OR (Ischemic Heart Diseases)] AND [(Luteolin) OR (3′,4′,5,7-Tetrahydroxy-Flavone) OR (3′,4′,5,7-Tetrahydroxyflavone) OR (Luteoline)]}. Quotations in eligible articles were also traced to minimize the possibility of omission as much as possible. The ID of PROSPERO is CRD42021226773. The search strategy and exclusion criteria are presented in Figure 1.
Inclusion Criteria
Articles regarding preclinical studies on the Post-infarction cardioprotective effects of luteolin that met the following inclusion criteria were included in the meta-analysis: (1) use of an acute myocardial ischemia model induced by occlusion of the left anterior descending artery (LAD) or global Non-flow ischemia of the isolated heart; (2) the intervention group received pretreatment with luteolin monotherapy, while the MIRI group was administered normal saline, a vehicle, or no treatment with no restriction on the route of administration, time of pretreatment, or dosage; and (3) an experimental study with the use of an animal model with no restriction to the species, weight, or age, with the exception of sex. The primary indicators for evaluation were IS and hemodynamics. The hemodynamic indicators, as recorded with a pressure sensor, included: (1) left ventricular systolic pressure (LVSP); (2) left ventricular end-diastolic pressure (LVEDP); (3) maximum rate of left ventricular pressure rise (+dp/dtmax); and (4) maximum rate of left ventricular pressure decrease (-dp/dtmax). Secondary indicators, which included cardiomyocyte apoptotic rate, oxidative products, and inflammatory factors, reflected possible mechanisms of luteolin for treatment of MIRI.
Exclusion Criteria
The exclusion criteria included the following: (1) no mention of the process for reperfusion of the impaired myocardium; (2) editorials, comments, conference abstracts, correspondences, reviews, and case reports; (3) pretreatment with other flavonoids or the other compound of luteolin; (4) in vitro studies; (5) pronounced intrinsic cardiac effect of anesthetics; and (6) clinical studies.
Data Extraction
The data from the articles that met the inclusion criteria were included in a table with the following categories: (1) surname of the author and year of publication; (2) characteristics of the experimental animals of each study, which included sex, weight, and age; (3) establishment of an MIRI model and time of ischemia/reperfusion process; (4) staining and mode of identification of the infarcted myocardium; (5) method of treatment, including the administration route, dosage, and duration; (6) type of anesthetic; (7) outcome measures; and (8) related signaling pathways and corresponding inhibitors. The mean values and standard deviations of the control and intervention groups were extracted. If the results were indispensable but not explicitly shown, GetData Graph Digitizer software (http://getdata-graph-digitizer.com/) was used to assemble the data. When the luteolin dosages varied among the intervention groups, the data of which will be merged according the formula recommend by Cochrane Handbook (19). The formula is shown in Supplementary Figure 1. The indices detected by different methods in the same study were annotated as 1, 2, 3, etc. Data from the eligible articles were extracted by two independent authors and disputes were adjudicated by the corresponding author.
Risk of Bias in Individual Studies
The checklist for animal data was rehashed as follows: (1) sample size estimation; (2) generation of random sequence; (3) no simulated myocardial ischemia; (4) blinding of outcome assessment; (5) appropriate animal model without comorbidities; (6) no noticeable intrinsic cardiac effect of anesthetics; (7) description of temperature control; (8) compliance with guidelines regarding the welfare of animals used in scientific testing and research; (9) publication after peer review; and (10) statement of a potential conflict of interest. If an entry qualified, the study received a score of 1 on a 10-point scale. Finally, the total score of each study was calculated. Any dispute was adjudicated by the corresponding author.
Statistical Analysis
When there were differences in units or testing methods, or the presence of apparently exaggerated numbers, the extracted outcomes were converted to standardized mean difference (SMD) values with the 95% confidence interval (CI) to complete the summary statistics. In other cases, the weighted mean difference (WMD) and 95% CI were adopted. The sample size of an experimental study is usually smaller than of a clinical study. Therefore, Hedge's g rather than Cohen's d was implemented. Statistical heterogeneity between studies was calculated using the I squared (I2) statistic. Random effects were selected because of the small sample size and prevalent statistical heterogeneity in preclinical studies. To more accurately explore the effect of group size, prespecified subgroups were assessed by different methods. If heterogeneity was remarkable, subgroup analysis, sensitivity analysis, and/or meta-regression analysis were conducted. If the consistency of baseline data was poor, linear regression and multiple regression were utilized. If the same outcome index was reported by more than 10 articles, funnel plots and the Egger's test were used to assess publication bias. All quantitative outcomes were analyzed using Stata/SE version 12 (StataCorp LLC, College Station, TX, USA) and RevMan version 5.3 (The Cochrane Collaboration, London, England). A probability value of < 0.05 was considered statistically significant.
Results
Study Inclusion
In total, 162 articles were harvested in accordance with the search strategy and tracing of quotations. After the removal of 50 duplications, the remaining 112 studies were screened by browsing the article type. In addition, 25 reviews, six conference abstracts, two correspondences, and one editorial were also excluded as Non-original articles. Of the remaining 78 articles, 58 were also excluded: 25 because luteolin was not the primary focus or was mixed with other flavonoids, seven because of the other compound forms of luteolin (luteolin-7-O-glucoside and luteolin-7-beta-D-glucoside), eight due to the lack of a MIRI model, one that lacked the reperfusion method, three because of clinical trails and thirteen were not in vivo study. Finally, data were pooled from 21 articles.
Study Characteristics and Baseline Data Analysis
All 21 articles (20–40) were published within the past 10 years. Sixteen studies (20–28, 30, 31, 35–38, 40) had healthy adult murids for comparison, and 5 studies (29, 32–34, 39) had comorbidities murids for comparison. Based on the construction method, two types of ischemia models were used in the included articles: ligation of LAD and Non-flow ischemia of the global heart. Nineteen studies (20, 22, 24–40) used rats as study subjects, while two articles (21, 23) used mice as study subjects. Eventually, eighteen articles (20, 23–28, 30–40) were included for quantitative analysis while two article (21, 23) was excluded by species and one article (29) was excluded to avoid the interference of comorbidity on the effect size. Eighty comparisons with 415 animals were tracked to describe amelioration of MIRI with luteolin, of which 263 were classified as the intervention group while 152 were classified as the control group. All of the animals were adult male rats, including Wistar rats with a weight range of 220–250 g, and Sprague Dawley rats with a weight range of 140–300 g. Anesthetics used for surgery that had no pronounced intrinsic cardiac effect included urethane, isoflurane, and pentobarbital sodium. Each experimental group was pretreated with luteolin via intravenous injection, intraperitoneal injection, gavage, or cardiac perfusion. The dosages differed via the administration route. There was also a dose-gradient design in some of the studies. The time of luteolin pretreatment spanned from dozens of minutes to 2 weeks before surgery. Nine articles (22, 24–26, 28, 30, 31, 35, 37) assessed IS by staining with 2,3,7-triphenyl tetrazolium-chloride (TTC) or Evans Blue/TTC. IS was assessed as the ratio of weight to area of IS. Areas stained by Evans blue were defined as an area not at risk, while the remaining area was defined as an area at risk (AAR), which was theoretically an area of IS without recanalization of the infarcted coronary artery. The AAR included the area of viable myocardium stained by TTC and the area of IS that negatively stained. The methods for calculation of the area of IS included IS/AAR, IS/whole heart, and IS/left ventricle (IS/LV). The duration of ischemia was 30 min. The duration of reperfusion was 24 h in five articles and 1–3 h, usually 2 h, in the others. Quantitative analysis in only one article reported the ejection fraction and fractional shortening. Consequently, intracardiac hemodynamic parameters for evaluation of heart function evaluation were included in 8 articles (20, 22, 27, 30, 31, 35–38). The fundamental characteristics of individual studies are shown in Table 1, and the mechanisms are listed in Table 2.
In the group of LAD ligation, baseline data (i.e., administrations, dosages, pretreatment timing, and reperfusion duration) were inconsistent. It has been reported that oral bioavailability of luteolin was 26 ± 6% while intravenous bioavailability was usually set at 100% (41). Although the method of administration was different, the included studies had explored the optimal dosage or quoted the dosage designed by others. Regression analysis analysis by administration, dosages, timing regimen of pretreatment or reperfusion duration had no impact on the effect size of IS and hemodynamics (Supplementary Table 1). The different Research Groups have explored their optimal experimental conditions for the best experimental results. Hence, the effect size did not be significantly affected by baseline data. In the model of global ischemia, the baseline data except for reperfusion duration was consistent. Regression analysis by reperfusion duration had no impact on the effect size of hemodynamics (Supplementary Table 1).
Study Quality and Publication Bias
The lowest study quality score was 6 points on a 10-point scale, while the highest was 9 points. Of the 21 completed studies, 19.05% were assigned a quality score of 6 points, 19.05% a score of 7 points, 52.38% a score of 8 points, and 9.52% a score of 9 points. The majority of studies received relatively high scores. All of the included studies were published in peer-reviewed journals. Control of temperature and animal welfare were described in 21 studies. No study mentioned sample size estimation. The process of randomly assigning animals to each group was described in all 21 studies. Outcomes were assessed blindly in five studies (22, 25, 27, 29, 30). A total of 16 studies (20–28, 30, 31, 35–38, 40) included a control group comprised of healthy adult animals without complications. The administration of anesthetics had hardly any effect on heart function in 21 articles. All studies declared potential conflicts of interest. The methodological quality of individual studies is shown in Table 3. The Egger test (P > 0.05) (Supplementary Figure 2) and the funnel plot (Supplementary Figure 3) showed no significant publication bias for all indicators except LVEDP (P = 0.014). However, trim and filling method illustrates the better authenticity of LVEDP results (Supplementary Figure 3C). The value of LVEDP changes from −6.35 to −9.03 (95% CI = −11.20 to −6.86, P < 0.001) through trim and filling method. The new valuation does not cross the invalid line.
Effectiveness
Myocardial IS
Subgroups were established based on IS measurements (Figure 2). Within the subgroups, SMD with the 95% CI was calculated to determine the coexistence of the area ratio and weight ratio. There were three subgroups of IS. Quantitative analysis of two studies (24, 30) showed that luteolin administration led to a decrease in the IS/AAR ratio as compared to that of the control group [Figure 2, SMD = −2.50, 95% CI = −3.47 to −1.52, p < 0.00001; heterogeneity: χ2 = 0.03, df = 2 (p = 0.87); I2 = 0%]. Quantitative analysis of four studies (25, 28, 35, 37) showed that the IS/whole heart ratio was significantly decreased as compared to that of the control group [Figure 2, SMD = 2.14, 95% CI = 3.06 to −2.14, p < 0.0001; heterogeneity: χ2 = 4.90, df = 3 (p = 0.18); I2 = 39%]. Quantitative analysis of four studies (22, 24, 26, 31) showed that luteolin administration reduced the IS/LV ratio as compared to that of the control group [Figure 2, SMD = −2.54, 95% CI = −3.82 to −1.26, p = 0.0001; heterogeneity: χ2 = 4.66 df = 3 (p = 0.20); I2 = 36%]. The subgroup analysis for the MIRI model was also conducted. In regard to the LAD ligation model, quantitative analysis of seven studies (24–26, 28, 30, 35, 37) with eight comparisons showed that luteolin administration reduced the IS as compared to that of the control group [Supplementary Figure 4, SMD = −2.14, 95% CI = −2.68 to −1.59, p < 0.00001; heterogeneity: χ2 = 7.94, df = 7 (p = 0.34); I2 = 12%]. In regard to the global ischemia model, quantitative analysis of two studies (22, 31) showed that luteolin administration reduced the IS as compared to that of the control group [Supplementary Figure 4, SMD = −2.87, 95% CI = −4.72 to −1.03, p = 0.002; heterogeneity: χ2 = 1.89, df = 1 (p = 0.17); I2 = 47%].
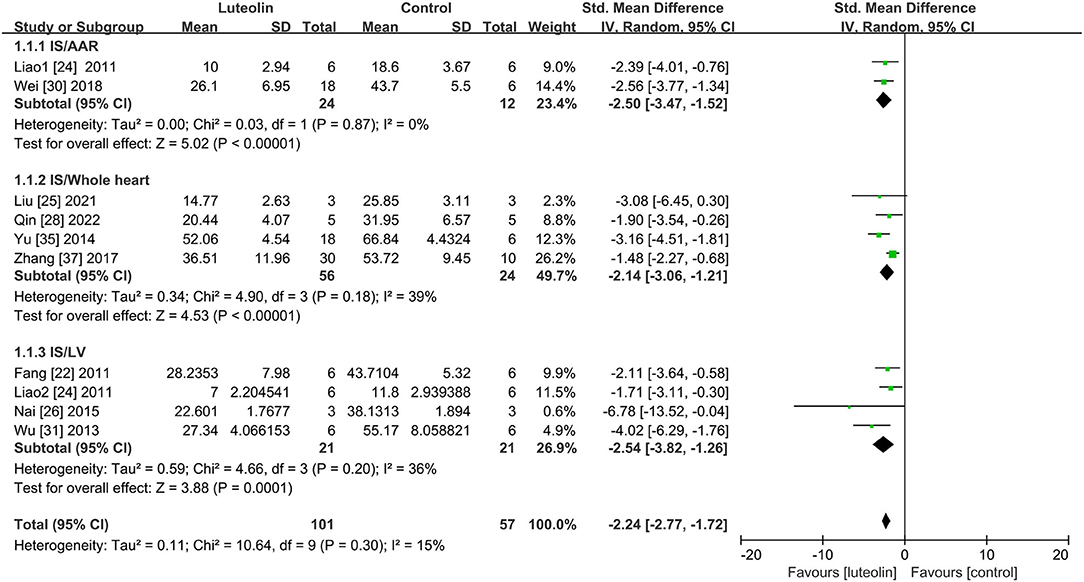
Figure 2. Forest plot showing changes in myocardial infarct size. IS, infarct size; AAR, area at risk; LV, left ventricular.
Hemodynamics
LVSP
In regard to the LAD ligation model, quantitative analysis of four studies (30, 35, 37, 38) showed that LVSP was significantly improved in the luteolin group as compared to that of the control group [Figure 3A, WMD = 21.62, 95% CI = 18.24 to 25.00, p < 0.00001; heterogeneity: χ2 = 2.75, df = 3 (p = 0.43); I2 = 0%]. In regard to the global ischemia model, quantitative analysis of five studies (20, 22, 27, 31, 36) with 10 comparisons showed that LVSP was significantly improved in the intervention group as compared to that of the control group [Figure 3A, WMD = 38.82, 95% CI = 32.82 to 44.82, p < 0.00001; heterogeneity: χ2 = 26.14, df = 9 (p = 0.002); I2 = 66%]. Here, sensitivity analysis was performed to determine the source of heterogeneity (Supplementary Figure 5). After the removal of one study (36), quantitative analysis of four studies (20, 22, 27, 31) with eight comparisons showed that LVSP was significantly improved as compared to that of the control group [Figure 3A, WMD = 35.40, 95% CI = 29.94 to 40.86, p < 0.00001; heterogeneity: χ2 = 10.05, df = 7 (p = 0.19); I2 = 30%].
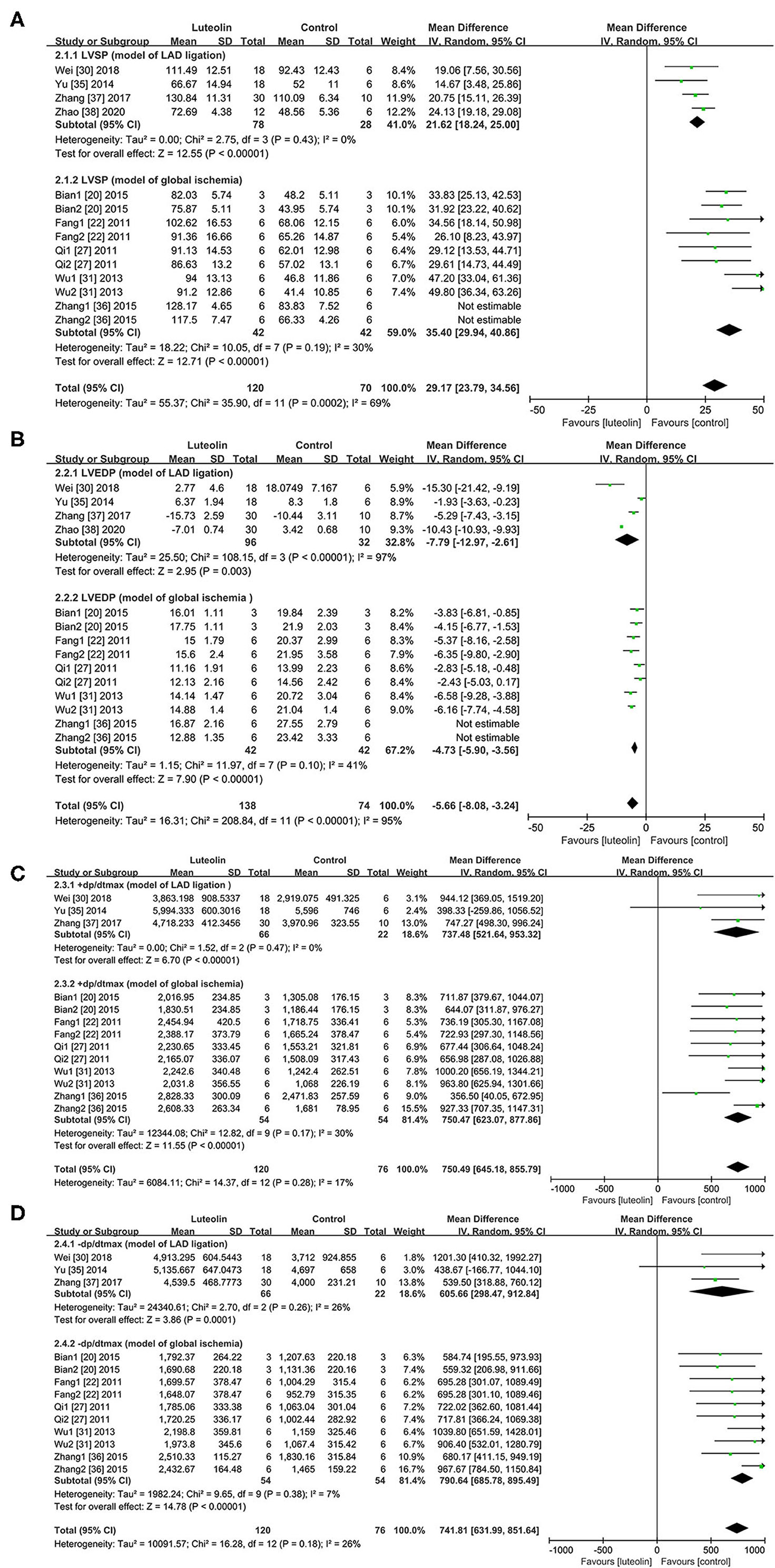
Figure 3. Forest plots for effect of luteolin on hemodynamics including (A) LVSP, (B) LVEDP, (C) +dp/dtmax, (D) -dp/dtmax. LVSP, left ventricular systolic pressure; LVEDP, left ventricular end-diastolic pressure; +dp/dtmax, maximum rate of left ventricular pressure rise; -dp/dtmax, maximum rate of left ventricular pressure decrease.
LVEDP
In regard to the LAD ligation model, Quantitative analysis of four studies (30, 35, 37, 38) showed that luteolin administration reduced LVEDP as compared to that of the control group [Figure 3B, WMD = −7.79, 95% CI = −12.97 to −2.61, p < 0.00001; heterogeneity: χ2 = 108.15, df = 3 (p < 0.00001); I2 = 97%]. Sensitivity analysis had no pronounced effect on the I2 values (Supplementary Figure 6).
In regard to the global ischemia model, three articles (32–34) that lacked a control group at the corresponding time point during reperfusion of the isolated heart were excluded from analysis. Thus, quantitative analysis of five studies (20, 22, 27, 31, 36) with 10 comparisons showed that luteolin administration significantly reduced LVEDP as compared to that of the control group [WMD = −5.85, 95% CI = −7.54 to −4.16, p < 0.00001; heterogeneity: χ2 = 38.43, df = 9 (p < 0.01); I2 = 77%]. After removal of one study (36) by sensitivity analysis (Supplementary Figure 7), the heterogeneity was decreased, indicating a meaningful change [Figure 3B, WMD = −4.73, 95% CI = −5.90 to −3.56, p < 0.01; heterogeneity: χ2 = 11.97, df = 7 (p = 0.10); I2 = 41%].
+dp/dtmax
In regard to the LAD ligation model, quantitative analysis of three (30, 35, 37) studiesshowed that luteolin administration increased +dp/dtmax as compared to that of the control group [Figure 3C, WMD = 737.48, 95% CI = 521.64 to 953.32, p < 0.00001; heterogeneity: χ2 = 1.52, df = 2 (p = 0.47); I2 = 0%]. In regard to the global ischemia model, quantitative analysis of five studies (20, 22, 27, 31, 36) with 10 comparisons showed that luteolin administration increased +dp/dtmax as compared to that of the control group [Figure 3C, WMD = 750.47, 95% CI = 623.09 to 877.86, p < 0.01; heterogeneity: χ2 = 12.82, df = 9 (p = 0.17); I2 = 30%].
-dp/dtmax
In regard to the LAD ligation model, quantitative analysis of three studies (30, 35, 37) showed that luteolin administration significantly increased -dp/dtmax as compared to that of the control group [Figure 3D, WMD = 605.66, 95% CI = 298.47 to 912.84, p = 0.0001; heterogeneity: χ2 = 2.70, df = 2 (p = 0.26); I2 = 26%].
In regard to the global ischemia model, quantitative analysis of five studies (20, 22, 27, 31, 36) with 10 comparisons showed that luteolin administration significantly increased -dp/dtmax as compared to that of the control group [Figure 3D, WMD = 790.64, 95% CI = 685.78 to 895.49, p < 0.01; heterogeneity: χ2 = 9.65, df = 9 (p = 0.38); I2 = 7%].
Cardioprotective Mechanisms of Luteolin
Anti-apoptosis
Only one study (30) included in the group of LAD ligation model. In regard to the global ischemia model, quantitative analysis of four studies (22, 27, 31, 40) showed that luteolin administration significantly decreased the proportion of apoptotic cells (TUNEL-positive cells) in the intervention group as compared to that of the control group [Figure 4A, WMD = −11.76, 95% CI = −12.90 to −10.63, p < 0.00001; heterogeneity: χ2 = 2.65, df = 3 (p = 0.45); I2 = 0%].
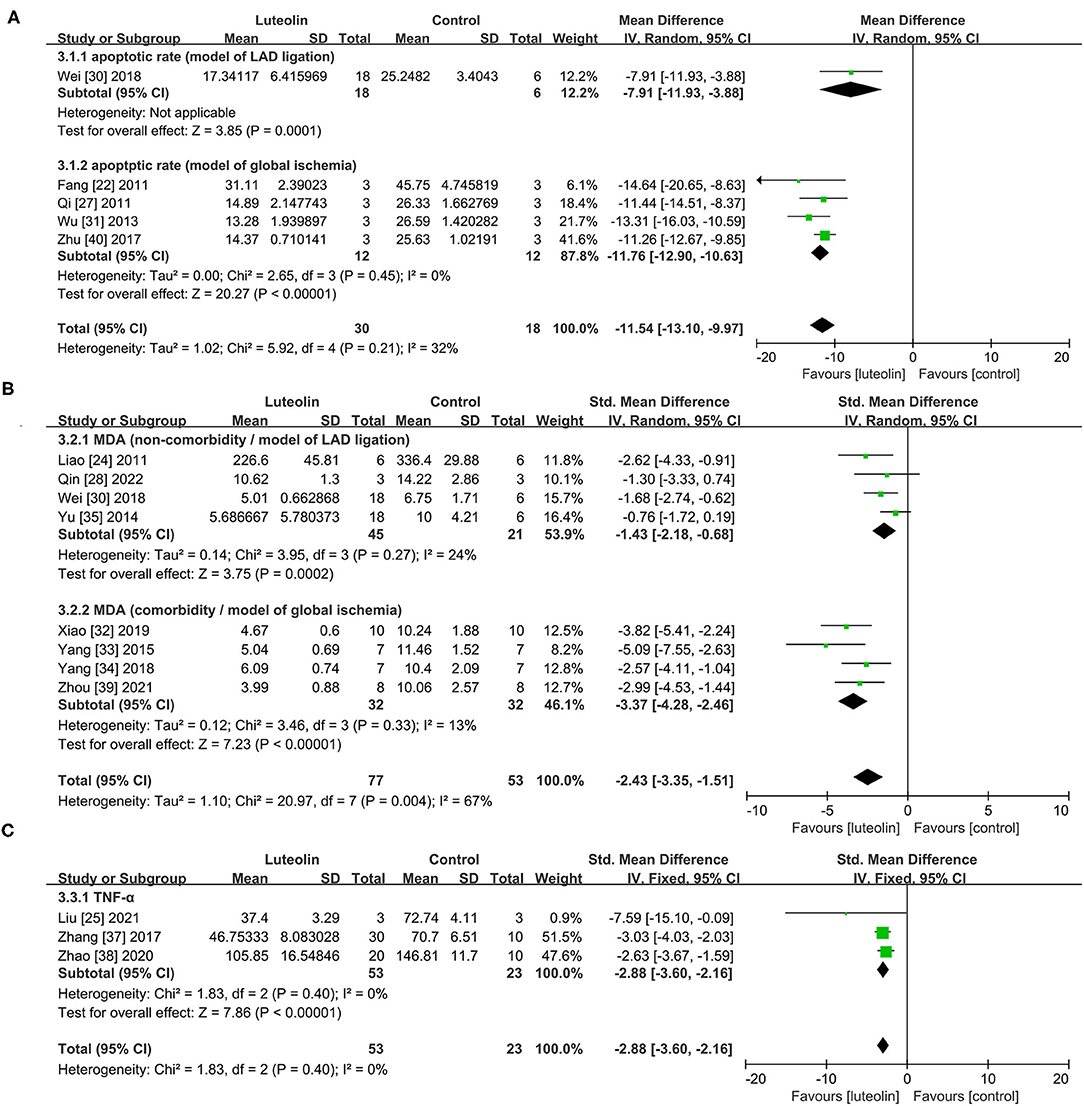
Figure 4. Forest plots for (A) apoptotic rate, (B) MDA, (C) TNF-α. LAD, left anterior descending branch; MDA, malondialdehyde; TNF-α, tumor necrosis factor alpha.
Anti-oxidation
Eight studies (24, 27, 30, 32–35, 39) showed that luteolin administration was significant for decreasing MAD level compared with the control group [Figure 4B, SMD = −2.43, 95% CI = −3.35 to −1.51, p < 0.00001; heterogeneity: χ2 = 20.97, df = 7 (p = 0.0004); I2 = 67%]. Subgroup analysis based on the comorbidities was subsequently conducted, which showed a more meaningful result [Non-comorbidity: SMD = −1.43, 95% CI = −2.18 to −0.68, p = 0.0002; heterogeneity: χ2 = 3.95, df = 3 (p = 0.27); I2 = 24%; comorbidity: SMD = −3.37, 95% CI = −4.28 to −2.46, p < 0.00001; heterogeneity: χ2 = 3.46, df = 3 (p = 0.33); I2 = 13%]. Coincidentally, the two different subgroups belonged to two different MIRI models.
Anti-inflammation
Quantitative analysis of three studies (25, 37, 38) showed that luteolin administration significantly decreased TNF-α levels [Figure 4C, SMD = −2.88, 95% CI = −3.60 to −2.16, p < 0.0001; heterogeneity: χ2 = 1.83, df = 2 (p = 0.40); I2 = 0%].
Discussion
Summary of Results
This meta-analysis is the first to assess the effects of luteolin administration for treatment of MIRI. Comprehensive analysis was conducted of 18 studies with 415 animals. According to the checklist, the included studies were of relatively high quality with a low risk of bias. All the included data were objective response variables. The issues of comorbidities and different MIRI models were addressed in advance. Baseline data (i.e., administrations, dosages, pretreatment timing, and reperfusion duration) did not have a significant effect on effect size of IS and hemodynamics. Preclinical evidence obtained from the meta-analysis confirmed that pretreatment with luteolin reduced IS and ameliorated hemodynamics in animal models. These potential cardioprotective effects were primarily due to the anti-apoptosis, anti-oxidation, and anti-inflammation properties of luteolin.
Limitations
The amount of data from mice is too small for further analysis. In regard to study quality, the included studies were credible but only four referred to a blind evaluation. Holman et al. (42) affirmed the necessity of blind outcome assessment while they also stated that experiment with an objective response variable did not need to be blind in animal researches. Hirst et al. (43) included 119,597 animals to evaluate the effects of blind outcome assessment which showed that subjective variable but not objective variable significantly increased effect sizes in the absence of blinding. In addition, there were notable differences among the experimental groups, which is likely the main reason for the absence of sample size calculation. As a result of the inclusion of more positive studies, the efficacy of luteolin may be overestimated. Besides, partial results were standardized by SMD with the 95% CI, thus the results should be carefully interpreted. The included studies employed multiple methods for the calculation of IS, which led to a smaller sample size in each subgroup.
Implications
Recent basic research studies have reported the superior efficacy of flavonoids for the treatment of MIRI. Epidemiological investigations suggest that dietary intake of flavonoids could effectively reduce the risk of cardiac events (44, 45). As compared with other isolated flavonoids from Ixeris sonchifolia, luteolin was reported as the best option against ischemia-induced injury (46). Luteolin is mainly metabolized in the liver, and intestines and the metabolites are absorbed in the gastrointestinal tract (47). By oral administration, the highest plasma concentration of luteolin, which has a half-life of about 5 h, occurs about 1 h later (45). The anti-tumor properties of luteolin have been reported in wide range of studies, although the focus of most current research seems to be shifting to cardioprotective effects. The initial focus of this meta-analysis was not only MIRI, but all Post-infarction cardiac injuries. However, with the exception of one article (48), most studies screened the effect of luteolin for treatment of MIRI in accordance with stringent standards. However, the focus of the present meta-analysis was the effect of luteolin administration on MIRI.
Myocardial Infarction Size and Apoptosis of Cardiomyocytes
From the included studies, AAR had no statistical significance in any of the experimental groups. The goal of treatment is to reduce reperfusion injury to the viable myocardium stained by TTC. Apoptosis of cardiomyocytes was detected at the infarcted border zone in the animal models, while reperfusion of the infarcted area further induced apoptosis (49). In addition, clinical studies have reported a close association of apoptosis with MIRI (50). With the development and popularization of percutaneous coronary intervention, more studies have attached great importance to protecting cardiomyocytes at areas of greater risk. Luteolin was reported to reduce IS to varying degrees in different models and by different measurement methods.
The anti-apoptosis effect of luteolin as the end-stage outcome of the physiological impact is regulated by various mechanisms. Of these, the phosphoinositide 3-kinase (PI3K)/AKT pathway is considered to participate in cell survival (51). Yet, the high ratio of Bcl-2/Bax was negatively correlated with vulnerability to activation of apoptosis pathways (52, 53). The phosphorylation of AKT regulated by luteolin increased the ratio of Bcl-2/Bax and decreased the proportion of TUNEL-positive cells (22, 27). Luteolin also up-regulated the expression of the anti-apoptotic proteins FGFR2 and LIF, which was related to the activation of Akt signalling (29). The MIRI-induced decrease in sarcoplasmic reticulum Ca2+-ATPase (SERCA2a) activity was facilitated by luteolin partly through the PI3K/Akt signaling pathway. Luteolin activation of the PI3K/Akt pathway was reported to exert antioxidation effects in a simulated ischemia-reperfusion model (32), while the cardioprotective effects of luteolin were partly reversed by the PI3K inhibitors LY294002 and wortmannin (22, 26, 29).
C-Jun N terminal kinase (JNK), extracellular signal-regulated kinases (ERKs), and P38 are downstream effectors of the mitogen-activated protein kinase (MAPK) pathway (54). Members of the MAPK family regulate apoptosis of cardiomyocytes. Wu et al. (31) found that luteolin and the JNK-inhibitor SP600125 both attenuated cardiomyocyte apoptosis and that the JNK and ERK1/2 pathways have opposing relationships, as luteolin-mediated down-regulation of JNK and up-regulation of ERK1/2 had an anti-apoptotic effect in an MIRI model. Activated ERK1/2 mediated SERCA2a activity, while this positive effect was abolished by the ERK1/2 inhibitor PD98059 (31). During the reperfusion period, activated P38 MAPK resulted in Ca2+ overload and an imbalance in mitochondrial transmembrane potential. Then, the impaired mitochondria released pro-apoptosis proteins, which led to the loss of cardiomyocytes (40). Wei et al. (30) suggested that luteolin enhanced peroxiredoxin II activation to ameliorate mitochondrial dysfunction. Luteolin possesses an anti-apoptosis effect and improves SERCA2a activity, equal to that of the P38-inhibitor SB203580 (40). Yu et al. (35) demonstrated that luteolin via reactive oxygen species (ROS) activation of the MAPK pathways inhibited cardiomyocyte apoptosis in an MIRI model.
The anti-apoptosis effects of luteolin are also involved other mechanisms. For example, Bian et al. (20) suggested that luteolin exerted apoptotic protective effects through miR-208b-3p regulation of small interfering RNA Ets1 expression. SUMO1, a SUMO isoform, was found to convey cardioprotective effects in an MIRI model and luteolin improved SUMO1 expression to reduce cardiomyocyte apoptosis (21), while upregulating the expression of the transcription factor Sp1 to reduce cardiomyocyte death (23).
Oxidative Stress and Ca2+ Overload
During myocardial infarct, myocardiocyte metabolism switches to anaerobic respiration, which leads to the accumulation of lactate and Ca2+ overload, while the opening of mitochondrial membrane permeability transition pores (MPTPs) is Pre-vented by the acidic condition. During reperfusion, ROS generated from reactivation of the electron transport chain, xanthine oxidase, and nicotinamide adenine dinucleotide phosphate (NADPH) oxidase prompts the opening of MPTPs and causes sarcoplasmic reticulum dysfunction. With the elimination of lactic acid, restoration of the mitochondrial membrane potential results in Ca2+ overload in the mitochondria, which further induces the opening of the MPTPs (55). Reoxygenation of the heart inflicts significant myocardial injury throughout the ischemic region that exceeds the damage caused by ischemia alone (56). SERCA2a, an ATP-dependent enzyme, pumps Ca2+ into the sarcoplasmic reticulum to prevent Ca2+ overload.
Superoxide radicals combined with NO produce peroxynitrite, which results in myocardial dysfunction (57). Liao et al. (24) found that luteolin down-regulates the expression of inducible nitric oxide synthase (NOS), but had no effect on the expression levels of endothelial NOS (eNOS) and neuronal NOS. However, in a rat model of diabetes, eNOS was activated by luteolin to diminish oxidative stress induced by MIRI (32, 33). Xiao et al. (32) suggested that luteolin could activate eNOS to trigger antioxidative effects mediated by nuclear factor E2-associated factor 2 (Nrf2), which attenuated MIRI in diabetic rats. Moreover, the anti-oxidative effect was abolished by the NOS inhibitor L-NAME and the Nrf2 inhibitor brusatol. Nrf2, as the key molecule in redox balance, initiates the transcription of downstream antioxidant enzymes (58). The antioxidative function of Nrf2 was also improved by luteolin-mediated activation of the PI3K/Akt pathway. With a similar mechanism, Zhang et al. (36) revealed that luteolin attenuated oxidative injury through the PI3K/Akt pathway. ROS activation of members of the MAPK family was inhibited by luteolin to reduce MIRI (32, 36). In addition, luteolin enhanced the expression of the antioxidant protein peroxiredoxin II (30).
Inflammation and Hemodynamics
The release of ROS, cytokines, and complement results in the accumulation of neutrophils after the onset of myocardial reperfusion (55). The luteolin-mediated anti-inflammatory effect focuses on the inflammasome NLRP3/NF-KB pathway. Luteolin generally enhances the expression of various upstream binding factors, such as sirt1 and Toll-like receptor 4, to reduce inflammatory injury in MIRI (38). Hemodynamics can reflect the systolic and diastolic functions of heart. Effective cardiomyocyte contraction and relaxation can not be separated from calcium recycling. SERCA2a modulate cardiac cytosolic Ca2+ levels, SERCA Overexpression attenuates cardiac microvascular I/R injury through mitochondrial quality control (59, 60). The mitochondrial calcium uniporter (MCU) transports free Ca2+ into the mitochondrial matrix, maintaining Ca2+ homeostasis, thus regulates the mitochondrial morphology and energy supply (61). SERCA2a Overexpression inhibite the overactive MCU to reduce MIRI (62). Luteolin enhances SERCA2a activity via sumoylation of lysine 585 and Sp1 upregulation (21, 23) to improve hemodynamics in MIRI.
Conclusions
The results of the present meta-analysis suggest that luteolin can act on different signaling pathways to reduce MIRI in animal models. As possible mechanisms, luteolin exerts anti-apoptosis, anti-oxidation, and anti-inflammation effects against MIRI. The main cardioprotective benefits of luteolin are the reduction of myocardial IS and the amelioration of intracardiac hemodynamics. There were some limitations to the methodology and study quality that reduce the strength of this evidence. Nonetheless, systematic inspection of these MIRI models provides preclinical evidence of the benefits of luteolin for clinical treatment of MIRI.
Data Availability Statement
The original contributions presented in the study are included in the article/Supplementary Material, further inquiries can be directed to the corresponding authors.
Author Contributions
QP and YL: contributed equally to this manuscript, wrote the manuscript, and made the figures. WM and RK: conducted the literature search, study selection, and analysis. DL and HZ: approved the final revisions of the manuscript submitted for publication. All authors contributed to the article and approved the submitted version.
Funding
This work was supported by the Natural Science Foundation of China [grant number 81570326] and the Science and Technology Innovation Special Fund of Xuzhou Science and Technology Bureau [grant number KC17094].
Conflict of Interest
The authors declare that the research was conducted in the absence of any commercial or financial relationships that could be construed as a potential conflict of interest.
Publisher's Note
All claims expressed in this article are solely those of the authors and do not necessarily represent those of their affiliated organizations, or those of the publisher, the editors and the reviewers. Any product that may be evaluated in this article, or claim that may be made by its manufacturer, is not guaranteed or endorsed by the publisher.
Supplementary Material
The Supplementary Material for this article can be found online at: https://www.frontiersin.org/articles/10.3389/fcvm.2022.685998/full#supplementary-material
Supplementary Figure 1. Data merge formula.
Supplementary Figure 2. Egger test. (A) IS, (B) LVSP, (C) LVEDP, (D) +dp/dtmax, (E) -dp/dtmax, (F) apoptotic rate, (G) MDA, (H) TNF-α.
Supplementary Figure 3. 3.1:trim and filling. (A) IS, (B) LVSP, (C) LVEDP, (D) +dp/dtmax, (E) -dp/dtmax, (F) apoptotic rate, (G) MDA, (H) TNF-α. 3.2: funnel plot (a) IS, (b) LVSP, (c) LVEDP, (d) +dp/dtmax, (e) -dp/dtmax, (f) apoptotic rate, (g) MDA, (h) TNF-α.
Supplementary Figure 4. Pooled estimates of myocardial infarct size for luteolin vs. vehicle in different MIRI models. Model of LAD ligation: the model was constructed by the left anterior descending coronary artery ligation. Model of global ischemia: heart was subjected to global no-flow ischemia without pacing.
Supplementary Figure 5. Sensitivity analysis.
Supplementary Figure 6. Sensitivity analysis.
Supplementary Figure 7. Sensitivity analysis.
Supplementary Table 1. Regression analysis in the model of LAD ligation and global ischemia.
References
1. Sandoval Y, Jaffe AS. Type 2 myocardial infarction: JACC review topic of the week. J Am Coll Cardiol. (2019) 73:1846–60. doi: 10.1016/j.jacc.2019.02.018
2. Thygesen K, Alpert JS, Jaffe AS, Chaitman BR, Bax JJ, Morrow DA, et al. Fourth universal definition of myocardial infarction (2018). J Am Coll Cardiol. (2018) 72:2231–64. doi: 10.1016/j.jacc.2018.08.1038
3. Jennings RB, Ganote CE. Structural changes in myocardium during acute ischemia. Circ Res. (1974) 35(Suppl 3):156–72.
4. Virmani R, Forman MB, Kolodgie FD. Myocardial reperfusion injury. Histopathological effects of perfluorochemical. Circulation. (1990) 81(3 Suppl):Iv57-68.
5. Ooi DS, Isotalo PA, Veinot JP. Correlation of antemortem serum creatine kinase, creatine kinase-MB, troponin I, and troponin T with cardiac pathology. Clin Chem. (2000) 46:338−44.
6. Ibáñez B, Heusch G, Ovize M F. van de Werf: Evolving therapies for myocardial ischemia/reperfusion injury. J Am Coll Cardiol. (2015) 65:1454–71. doi: 10.1016/j.jacc.2015.02.032
7. Montecucco F, Carbone F, Schindler TH. Pathophysiology of ST-segment elevation myocardial infarction: novel mechanisms and treatments. Eur Heart J. (2016) 37:1268–83. doi: 10.1093/eurheartj/ehv592
8. Cung TT, Morel O, Cayla G, Rioufol G, Garcia-Dorado D, Angoulvant D, et al. Cyclosporine before PCI in patients with acute myocardial infarction. N Engl J Med. (2015) 373:1021–31. doi: 10.1056/NEJMoa1505489
9. Fröhlich GM, Meier P, White SK, Yellon DM, Hausenloy DJ. Myocardial reperfusion injury: looking beyond primary PCI. Eur Heart J. (2013) 34:1714–22. doi: 10.1093/eurheartj/eht090
10. Yellon DM, Hausenloy DJ. Myocardial reperfusion injury. N Engl J Med. (2007) 357:1121–35. doi: 10.1056/NEJMra071667
11. Hausenloy DJ, Garcia-Dorado D, Bøtker HE, Davidson SM, Downey J, Engel FB, et al. Novel targets and future strategies for acute cardioprotection: position paper of the European society of cardiology working group on cellular biology of the heart. Cardiovasc Res. (2017) 113:564–85. doi: 10.1093/cvr/cvx049
12. Davidson SM, Ferdinandy P, Andreadou I, Bøtker HE, Heusch G, Ibáñez B, et al. Multitarget strategies to reduce myocardial ischemia/reperfusion injury: JACC review topic of the week. J Am Coll Cardiol. (2019) 73:89–99. doi: 10.1016/j.jacc.2018.09.086
13. Wang J, Wong YK, Liao F. What has traditional Chinese medicine delivered for modern medicine? Expert Rev Mol Med. (2018) 20:e4. doi: 10.1017/erm.2018.3
14. Parmenter BH, Croft KD, Hodgson JM, Dalgaard F, Bondonno CP, Lewis JR, et al. An overview and update on the epidemiology of flavonoid intake and cardiovascular disease risk. Food Funct. (2020) 11:6777–806. doi: 10.1039/d0fo01118e
15. Kempuraj D, Thangavel R, Kempuraj DD, Ahmed ME, Selvakumar GP, Raikwar SP, et al. Neuroprotective effects of flavone luteolin in neuroinflammation and neurotrauma. Biofactors. (2020) 47:190–7. doi: 10.1002/biof.1687
16. Iida K, Naiki T, Naiki-Ito A, Suzuki S, Kato H, Nozaki S, et al. Luteolin suppresses bladder cancer growth via regulation of mechanistic target of rapamycin pathway. Cancer Sci. (2020) 111:1165–79. doi: 10.1111/cas.14334
17. T. Boeing, de Souza P, Speca S, Somensi LB, Mariano LNB, Cury BJ M, et al. Luteolin prevents irinotecan-induced intestinal mucositis in mice through antioxidant and anti-inflammatory properties. Br J Pharmacol. (2020) 177:2393–408. doi: 10.1111/bph.14987
18. Pei J, Sun Q, Zhao L, Shi H, Tang F, Cao F. Efficient biotransformation of luteolin to isoorientin through adjusting induction strategy, controlling acetic acid, and increasing UDP-glucose supply in Escherichia coli. J Agric Food Chem. (2019) 67:331–40. doi: 10.1021/acs.jafc.8b05958
19. Cumpston M, Li T, Page MJ, Chandler J, Welch VA, Higgins JP, et al. Updated guidance for trusted systematic reviews: a new edition of the cochrane handbook for systematic reviews of interventions. Cochrane Database Syst Rev. (2019) 10:Ed000142. doi: 10.1002/14651858.Ed000142
20. Bian C, Xu T, Zhu H, Pan D, Liu Y, Luo Y, et al. Luteolin inhibits ischemia/reperfusion-induced myocardial injury in rats via downregulation of microRNA-208b-3p. PLoS One. (2015) 10:12–e0144877. doi: 10.1371/journal.pone.0144877
21. Du Y, Liu P, Xu T, Pan D, Zhu H, Zhai N, et al. Luteolin modulates SERCA2a leading to attenuation of myocardial ischemia/ reperfusion injury via sumoylation at lysine 585 in mice. Cell Physiol Biochem. (2018) 45:883–98. doi: 10.1159/000487283
22. Fang F, Li D, Pan H, Chen D, Qi L, Zhang R, et al. Luteolin inhibits apoptosis and improves cardiomyocyte contractile function through the PI3K/Akt pathway in simulated ischemia/reperfusion. Pharmacology. (2011) 88:149–58. doi: 10.1159/000330068
23. Hu Y, Zhang C, Zhu H, Wang S, Zhou Y, Zhao J, et al. Luteolin modulates SERCA2a via Sp1 upregulation to attenuate myocardial ischemia/reperfusion injury in mice. Sci Rep. (2020) 10:1–15407. doi: 10.1038/s41598-020-72325-8
24. Liao PH, Hung LM, Chen YH, Kuan YH, Zhang FBY, Lin RH, et al. Cardioprotective effects of luteolin during ischemia-reperfusion injury in rats. Circ J. (2011) 75:443–50. doi: 10.1253/circj.CJ-10-0381
25. Liu D, Luo H, Qiao C. SHP-1/STAT3 interaction is related to luteolin-induced myocardial ischemia protection. Inflammation. (2022) 45:88–99. doi: 10.1007/s10753-021-01530-y
26. Nai C, Xuan H, Zhang Y, Shen M, Xu T, Pan D, et al. Luteolin exerts cardioprotective effects through improving sarcoplasmic reticulum Ca2+-ATPase activity in rats during ischemia/reperfusion in vivo. Evid Based Complement Alternat Med. (2015) 2015:365854. doi: 10.1155/2015/365854
27. Qi L, Pan H, Li D, Fang F, Chen D, Sun H. Luteolin improves contractile function and attenuates apoptosis following ischemia-reperfusion in adult rat cardiomyocytes. Eur J Pharmacol. (2011) 668:201–7. doi: 10.1016/j.ejphar.2011.06.020
28. Qin X, Qin H, Li Z, Xue S, Huang B, Liu X, et al. Luteolin alleviates ischemia/reperfusion injury-induced no-reflow by regulating Wnt/β-catenin signaling in rats. Microvasc Res. (2022) 139:104266. doi: 10.1016/j.mvr.2021.104266
29. Sun D, Huang J, Zhang Z, Gao H, Li J, Shen M, et al. Luteolin limits infarct size and improves cardiac function after myocardium ischemia/reperfusion injury in diabetic rats. PLoS One. (2012) 7:e33491. doi: 10.1371/journal.pone.0033491
30. Wei B, Lin Q, Ji YG, Zhao YC, Ding LN, Zhou WJ, et al. Luteolin ameliorates rat myocardial ischaemia-reperfusion injury through activation of peroxiredoxin II. Br J Pharmacol. (2018) 175:3315–32. doi: 10.1111/bph.14367
31. Wu X, Xu T, Li D, Zhu S, Chen Q, Hu W, et al. ERK/PP1a/PLB/SERCA2a and JNK pathways are involved in luteolin-mediated protection of rat hearts and cardiomyocytes following ischemia/reperfusion. PLoS One. (2013) 8:12-e82957. doi: 10.1371/journal.pone.0082957
32. Xiao C, Xia M-L, Wang J, Zhou X-R, Lou Y-Y, Tang L-H, et al. Luteolin Attenuates Cardiac Ischemia/Reperfusion Injury in Diabetic Rats by Modulating Nrf2 Antioxidative Function. Oxid Med Cell Longev. (2019) 2019:2719252. doi: 10.1155/2019/2719252
33. Yang JT, Qian LB, Zhang FJ, Wang J, Ai H, Tang LH, et al. Cardioprotective effects of luteolin on ischemia/reperfusion injury in diabetic rats are modulated by eNOS and the mitochondrial permeability transition pathway. J Cardiovasc Pharmacol. (2015) 65:349–56. doi: 10.1097/fjc.0000000000000202
34. Yang J-T, Wang J, Zhou X-R, Xiao C, Lou Y-Y, Tang L-H, et al. Luteolin alleviates cardiac ischemia/reperfusion injury in the hypercholesterolemic rat via activating Akt/Nrf2 signaling. Naunyn-Schmiedebergs Archives of Pharmacology. (2018) 391:719–28. doi: 10.1007/s00210-018-1496-2
35. Yu D, Li M, Tian Y, Liu J, Shang J. Luteolin inhibits ROS-activated MAPK pathway in myocardial ischemia/reperfusion injury. Life Sci. (2015) 122:15–25. doi: 10.1016/j.lfs.2014.11.014
36. Zhang RQ, Li DY, Xu TD, Zhu SS, Pan HJ, Fang F, et al. Antioxidative effect of luteolin pretreatment on simulated ischemia/reperfusion injury in cardiomyocyte and perfused rat heart. Chin J Integr Med. (2015) 23:518–27. doi: 10.1007/s11655-015-2296-x
37. Zhang X, Du Q, Yang Y, Wang J, Dou S, Liu C, et al. The protective effect of Luteolin on myocardial ischemia/reperfusion (I/R) injury through TLR4/NF-κB/NLRP3 inflammasome pathway. Biomed Pharmacother. (2017) 91:1042–52. doi: 10.1016/j.biopha.2017.05.033
38. Zhao L, Zhou Z, Zhu C, Fu Z, Yu D. Luteolin alleviates myocardial ischemia reperfusion injury in rats via Siti1/NLRP3/NF-kappa B pathway. Int Immunopharmacol. (2020) 85:106680. doi: 10.1016/j.intimp.2020.106680
39. Zhou XR, Ru XC, Xiao C, Pan J, Lou YY, Tang LH, et al. Sestrin2 is involved in the Nrf2-regulated antioxidative signaling pathway in luteolin-induced prevention of the diabetic rat heart from ischemia/reperfusion injury. Food Funct. (2021) 12:3562–71. doi: 10.1039/d0fo02942d
40. Zhu S, Xu T, Luo Y, Zhang Y, Xuan H, Ma Y, et al. Luteolin enhances sarcoplasmic reticulum Ca2+-ATPase activity through p38 MAPK signaling thus improving rat cardiac function after ischemia/reperfusion. Cell Physiol Biochem. (2017) 41:999–1010. doi: 10.1159/000460837
41. Chen T, Li LP, Lu XY, Jiang HD, Zeng S. Absorption and excretion of luteolin and apigenin in rats after oral administration of Chrysanthemum morifolium extract. J Agric Food Chem. (2007) 55:273–7. doi: 10.1021/jf062088r
42. Holman L, Head ML, Lanfear R, Jennions MD. Evidence of experimental bias in the life sciences: why we need blind data recording. PLoS Biol. (2015) 13:e1002190. doi: 10.1371/journal.pbio.1002190
43. Hirst JA, Howick J, Aronson JK, Roberts N, Perera R, Koshiaris C, et al. The need for randomization in animal trials: an overview of systematic reviews. PLoS One. (2014) 9:e98856. doi: 10.1371/journal.pone.0098856
44. Hertog MG, Feskens EJ, Hollman PC, Katan MB, Kromhout D. Dietary antioxidant flavonoids and risk of coronary heart disease: the zutphen elderly study. Lancet. (1993) 342:1007–11. doi: 10.1016/0140-6736(93)92876-u
45. Wang L, Lee IM, Zhang SM, Blumberg JB, Buring JE, Sesso HD. Dietary intake of selected flavonols, flavones, and flavonoid-rich foods and risk of cancer in middle-aged and older women. Am J Clin Nutr. (2009) 89:905–12. doi: 10.3945/ajcn.2008.26913
46. Zhang YC, Gan FF, Shelar SB, Ng KY, Chew EH. Antioxidant and Nrf2 inducing activities of luteolin, a flavonoid constituent in Ixeris sonchifolia Hance, provide neuroprotective effects against ischemia-induced cellular injury. Food Chem Toxicol. (2013) 59:272–80. doi: 10.1016/j.fct.2013.05.058
47. Tang L, Feng Q, Zhao J, Dong L, Liu W, Yang C, et al. Involvement of UDP-glucuronosyltranferases and sulfotransferases in the liver and intestinal first-pass metabolism of seven flavones in C57 mice and humans in vitro. Food Chem Toxicol. (2012) 50:1460–7. doi: 10.1016/j.fct.2012.01.018
48. Hu J, Man W, Shen M, Zhang M, Lin J, Wang T, et al. Luteolin alleviates post-infarction cardiac dysfunction by up-regulating autophagy through Mst1 inhibition. J Cell Mol Med. (2016) 20:147–56. doi: 10.1111/jcmm.12714
49. Gottlieb RA, Burleson KO, Kloner RA, Babior BM, Engler RL. Reperfusion injury induces apoptosis in rabbit cardiomyocytes. J Clin Invest. (1994) 94:1621–8. doi: 10.1172/jci117504
50. Xu T, Li D, Jiang D. Targeting cell signaling and apoptotic pathways by luteolin: cardioprotective role in rat cardiomyocytes following ischemia/reperfusion. Nutrients. (2012) 4:2008–19. doi: 10.3390/nu4122008
51. Hand TW, Cui W, Jung YW, Sefik E, Joshi NS, Chandele A, et al. Differential effects of STAT5 and PI3K/AKT signaling on effector and memory CD8 T-cell survival. Proc Natl Acad Sci USA. (2010) 107:16601–6. doi: 10.1073/pnas.1003457107
52. Changizi Z, Moslehi A, Rohani AH, Eidi A. Chlorogenic acid induces 4T1 breast cancer tumor's apoptosis via p53, Bax, Bcl-2, and caspase-3 signaling pathways in BALB/c mice. J Biochem Mol Toxicol. (2021) 35:e22642. doi: 10.1002/jbt.22642
53. Ma M, Wang X, Liu N, Shan F, Feng Y. Low-dose naltrexone inhibits colorectal cancer progression and promotes apoptosis by increasing M1-type macrophages and activating the Bax/Bcl-2/caspase-3/PARP pathway. Int Immunopharmacol. (2020) 83:106388. doi: 10.1016/j.intimp.2020.106388
54. Luo Y, Shang P, Li D. Luteolin: a flavonoid that has multiple cardio-protective effects and its molecular mechanisms. Front Pharmacol. (2017) 8:692. doi: 10.3389/fphar.2017.00692
55. Hausenloy DJ, Yellon DM. Myocardial ischemia-reperfusion injury: a neglected therapeutic target. J Clin Invest. (2013) 123:92–100. doi: 10.1172/jci62874
56. Hearse DJ, Humphrey SM, Chain EB. Abrupt reoxygenation of the anoxic potassium-arrested perfused rat heart: a study of myocardial enzyme release. J Mol Cell Cardiol. (1973) 5:395–407. doi: 10.1016/0022-2828(73)90030-8
57. Wang P, Zweier JL. Measurement of nitric oxide and peroxynitrite generation in the postischemic heart. Evidence for peroxynitrite-mediated reperfusion injury. J Biol Chem. (1996) 271:29223–30. doi: 10.1074/jbc.271.46.29223
58. Shen Y, Liu X, Shi J, Wu X. Involvement of Nrf2 in myocardial ischemia and reperfusion injury. Int J Biol Macromol. (2019) 125:496–502. doi: 10.1016/j.ijbiomac.2018.11.190
59. Tan Y, Mui D, Toan S, Zhu P, Li R, Zhou H. SERCA overexpression improves mitochondrial quality control and attenuates cardiac microvascular ischemia-reperfusion injury. Mol Ther Nucleic Acids. (2020) 22:696–707. doi: 10.1016/j.omtn.2020.09.013
60. Pinton P, Giorgi C, Siviero R, Zecchini E, Rizzuto R. Calcium and apoptosis: ER-mitochondria Ca2+ transfer in the control of apoptosis. Oncogene. (2008) 27:6407–18. doi: 10.1038/onc.2008.308
61. Garbincius JF, Elrod JW. Mitochondrial calcium exchange in physiology and disease. Physiol Rev. (2022) 102:893–992. doi: 10.1152/physrev.00041.2020
Keywords: luteolin, myocardial ischemia-reperfusion injury, apoptosis, oxidation, inflammation
Citation: Pan Q, Liu Y, Ma W, Kan R, Zhu H and Li D (2022) Cardioprotective Effects and Possible Mechanisms of Luteolin for Myocardial Ischemia-Reperfusion Injury: A Systematic Review and Meta-Analysis of Preclinical Evidence. Front. Cardiovasc. Med. 9:685998. doi: 10.3389/fcvm.2022.685998
Received: 26 March 2021; Accepted: 22 March 2022;
Published: 25 April 2022.
Edited by:
Tommaso Gori, Johannes Gutenberg University Mainz, GermanyReviewed by:
Istvan Szokodi, University of Pécs, HungaryJian Yang, China Three Gorges University, China
Copyright © 2022 Pan, Liu, Ma, Kan, Zhu and Li. This is an open-access article distributed under the terms of the Creative Commons Attribution License (CC BY). The use, distribution or reproduction in other forums is permitted, provided the original author(s) and the copyright owner(s) are credited and that the original publication in this journal is cited, in accordance with accepted academic practice. No use, distribution or reproduction is permitted which does not comply with these terms.
*Correspondence: Dongye Li, dongyeli@xzhmu.edu.cn; Hong Zhu, hongzhumao@sohu.com
†These authors have contributed equally to this work