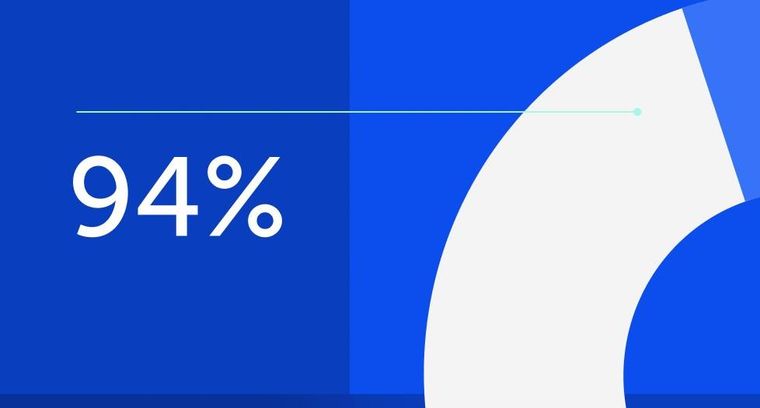
94% of researchers rate our articles as excellent or good
Learn more about the work of our research integrity team to safeguard the quality of each article we publish.
Find out more
REVIEW article
Front. Cardiovasc. Med., 09 January 2023
Sec. Cardiovascular Imaging
Volume 9 - 2022 | https://doi.org/10.3389/fcvm.2022.1094796
This article is part of the Research TopicMultimodality Imaging of Left Ventricular Assist Devices: Applications in advanced heart failureView all 7 articles
Patients suffering from end-stage heart failure tend to have high mortality rates. With growing numbers of patients progressing into severe heart failure, the shortage of available donors is a growing concern, with less than 10% of patients undergoing cardiac transplantation (CTx). Fortunately, the use of left ventricular assist devices (LVADs), a variant of mechanical circulatory support has been on the rise in recent years. The expansion of LVADs has led them to be incorporated into a variety of clinical settings, based on the goals of therapy for patients ailing from heart failure. However, with an increase in the use of LVADs, there are a host of complications that arise with it. One such complication is the development and progression of aortic regurgitation (AR) which is noted to adversely influence patient outcomes and compromise pump benefits leading to increased morbidity and mortality. The underlying mechanisms are likely multifactorial and involve the aortic root-aortic valve (AV) complex, as well as the LVAD device, patient, and other factors, all of them alter the physiological mechanics of the heart resulting in AV dysfunction. Thus, it is imperative to screen patients before LVAD implantation for AR, as moderate or greater AR requires a concurrent intervention at the time of LVADs implantation. No current strict guidelines were identified in the literature search on how to actively manage and limit the development and/or progression of AR, due to the limited information. However, some recommendations include medical management by targeting fluid overload and arterial blood pressure, along with adjusting the settings of the LVADs device itself. Surgical interventions are to be considered depending on patient factors, goals of care, and the underlying pathology. These interventions include the closure of the AV, replacement of the valve, and percutaneous approach via percutaneous occluding device or transcatheter aortic valve implantation. In the present review, we describe the interaction between AV and LVAD placement, in terms of patient management and prognosis. Also it is provided a comprehensive echocardiographic strategy for the precise assessment of AV regurgitation severity.
Aortic valve pathophysiology and the associated risk factors predisposing to AR development in patients with left ventricular assist device (LVAD) should be taken into account considering the increasing number of patients with end-stage heart failure (HF) that temporarily or permanently are implanted, despite being on optimal medical management (1) while waiting for cardiac transplantation (CTx). This is particularly important since CTx is vital for enhancing life expectancy, quality of life (QoL), and functional status (2, 3), however, organ donor shortage is an ongoing obstacle and, as a result, fewer than 10% of patients with severe, refractory HF receive CTx (4, 5). An alternative for this patient cohort is mechanical circulatory support with LVAD (6, 7). LVADs work by unloading the left ventricle (LV) and providing appropriate pressure, thus increasing cardiac output. The subsequent structural and functional changes improve survival and QoL for end-stage HF patients, despite being tethered to an external power source (7–10). However, long-term LVAD placement has side effects, related to the device, heart function, or cardiac valves, including the aortic valve (AV). Co-existing cardiac valve dysfunction can make LVAD implantation and effectiveness more challenging. Multiple factors can be responsible for AV malfunction, compromising the benefits of the device (11). Aortic regurgitation (AR) affects at least 25–30% of patients within the first year of implantation, which can lead to poor pump efficiency, worsening HF, and increased mortality (12, 13). However, there is no agreement on the prognostic role of AR in LVAD patients in terms of outcomes (14–18) and therapeutic management (19–21).
In the present review, we describe the interaction between AV and LVAD placement, in terms of patient management and prognosis. We also provide a comprehensive echocardiographic strategy for the precise assessment of AV regurgitation.
The function of the LVAD is to reduce the workload of the LV by pumping blood via a LV apical cannula to the aorta, to maintain systemic perfusion (Figure 1).
Figure 1. Left ventricular assist device (LVAD) structure with the corresponding echocardiographic images for the inflow cannula and outflow cannula. LV, left ventricle; LA, left atrium; RV, right ventricle; AR, aortic regurgitation.
Left ventricular assist devices can be divided into pulsatile or continuous flow. Pulsatile flow LVADs (PF-LVAD), mimic the heart’s natural rhythmic motion (e.g., Berlin heart), whereas continuous flow LVADs (CFL-VAD) use a motor operating at a fixed speed, resulting in continuous ejection of blood into the aorta. This causes a decreased pulse pressure and a non-palpable peripheral pulse. CF-LVAD can be further subdivided into axial flow (HeartMate II, Jarvik 2000, and DeBakey) and centrifugal flow (VentrAssist, HeartWare, Levacor, and HearMate III). Axial flow employs a turbine rotation, pushing blood into the outflow cannula, whereas centrifugal flow draws blood along the rotor’s axis and propels it tangentially into the systemic circulation. Continuous flow LVAD has substantially prolonged the longevity of the pump (22).
Between 2011 and 2022, there has been a progressive increase in LVAD implantation (26,688 devices placed), with a drop in 2020, because of the COVID-19 pandemic effect on cardiac surgical volumes in the United States (23). At present, HeartMate III (Abbott) is the most employed LVAD in clinical practice (24).
Left ventricular assist device is employed in a variety of clinical settings, based on the goals of therapy:
a) Short term: Bridge to recovery. These are patients awaiting cardiac surgery, or who suffer from ventricular arrhythmias, cardiogenic shock, or heart failure that are refractory to medical management.
b) Medium term: Bridge to Transplantation (BTT) and Bridge To candidacy (BTC). These patients require additional support while awaiting CTx or are currently unfit for CTx, but not have absolute contraindications (23, 25–27). BTC patients have improved utilization rates, increasing from 26.9% (2011–2015) to 32.5% in (2015–2020) (23).
c) Long-term: Destination Therapy (DT). Patients who are ineligible for CTx due to age, comorbidities, or psychosocial factors can have prolonged survival with LVAD implantation. Due to the new heart allocation, heart strategy by the United Network for Organ Sharing (UNOS) in 2018, DT has increased from 42.7% (2011–2015) to 50.4% (2015–2020) (28). Reported survival rates for DT are 82.8 and 74.1% at 1 and 2 years, respectively (23). LVAD implantation is also used as a DT in facilities that lack CTx facilities (29).
Left ventricular assist device implantation is associated with several complications (Table 1). Despite the complication rate, LVAD use has shown a significant reduction in morbidity rate from any cause, as compared with medical treatment, as demonstrated by the REMATCH trial (6). The 2-year survival rates for patients using the latest CF-LVAD (HeartMate III) are equivalent to those following CTx, with survival rates of 80 and 70% at 1- and 2-years, respectively (30, 31). An overall survival rate of 74.5% was seen when fully magnetized centrifugal-flow LVADs were used in real-world population using 2-year results from the ELEVATE registry (32). The MOMENTUM 3 trial demonstrated that, in centrifugal-flow LVADs, the 2-year survival rate was 84.5%, while the stroke-free and need for reoperation rate, due to LVAD malfunction, was 76.9% (24), confirmed by the more recent MOMENTUM 3, 5 years outcome (33). Additionally, even though numerous patients have been planned to be implanted as DT or BTT (mid and long term destination), some of these can turn in bridge to recovery when weaning criteria are satisfy, included no AR or maximum grade I AR. In these selected patients with HF secondary to chronic cardiomyopathy who underwent LVAD removal for complete or even incomplete cardiac recovery, had a 66% freedom from HF and 10.6% mortality after 5 year post-weaning (34).
Risk factors for progression of AR in LVAD patients include longer duration of LVAD placement, use of CF-LVAD, DT, older age, female gender, smaller BMI, and mild pre-implant AR (12–14, 21, 35, 36). Short-term data suggests that 10–55% of patients will develop de novo AR over the first 6 months post-implantation (14, 36). This also seems to hold true in patients implanted as DT (18). At 2.5-year follow-up, 43.2% of those patients with mild AR pre-LVAD developed moderate to severe AR (18). Moreover, it was reported that at 2 years follow-up, 33% of the LVAD patients developed more than moderate AR and only 30% of AVs opened at least partially or intermittently.
There is no uniform consensus on mortality and morbidity on the effect of AR in LVAD patients. Although many studies have shown a progressive increase in de novo and progression of AR over time (16), most of them show no difference in survival between patients with AR compared to those who did not develop AR (13, 16–18, 36, 37). For example, Holly et al. (16) reported that 15.2% of patients developed severe AR out of 237 implanted with CF-LVAD but no difference in survival was noted when patients who developed AR were compared with those who did not develop AR. Cowger et al. (13) found that the development of AR is a common phenomenon and did not affect mortality except in those with pre-LVAD implantation significant right ventricle (RV) dysfunction. In general the hypothesis of those that support no direct relation between AR and mortality, is that patients with AR are more likely to have more complications including mitral and tricuspid regurgitation, hemolysis, worse RV function at long-term follow-up, higher hospital readmissions for HF, and more probable to remain in NYHA class III (13, 18, 21, 37–39) (Figure 2).
Figure 2. Effect of aortic regurgitation (AR) severity on left ventricle (LV) and right ventricle (RV) structure and hemodynamic.
On the other hand, the INTERMACS study (14) found that patients on LVAD with significant AR had increased mortality compared to those with no AR (49.1% vs. 36.5% at 5-year, p < 0.001) after adjustment for confounders. Moreover, patients with severe AR had at least moderate mitral regurgitation (MR), leading to lower systolic blood pressures and cardiac output, and higher rehospitalization rates for HF. Auvil et al. (40), in their cohort of patients, found that moderate AR was an independent predictor of 2-year mortality after LVAD implantation and there was a significant difference in survival among patients with no AR, mild and moderate AR. On the other hand, they did not find any difference among AR groups in terms of 6-min walking test and prevalence of RV failure. In Table 2 are summarized all the studies we found on Pubmed using keywords as LVAD, AR and mortality/survival, excluding case reports (13–18, 36–48).
Table 2. Literature review of multiple studies studying the association between left ventricular assist device (LVAD) and AR.
Studies have been performed to assess the relationship between different LVAD devices and AR (49–51), and, as previously mentioned, AR is a determinant in morbidity and mortality which are described in detail in Table 2. As compared to PF-LVAD, CF-LVADs provide an overall larger reduction in LV end-diastolic pressure and volume but also cause derangements of the pressure-volume loop. CF-LVADs also improve LV systolic flow, aortic flow, LV systolic pressure, and mean arterial pressure. However, PF-LVADs have lower rates of developing more advanced AR than CF-LVAD patients (15, 35, 36, 52). Park et al. (42) described that patients with CF-LVAD were two times more likely to develop AR than patients on PF-LVAD support. Hatano et al. (52) considered AR frequency among multiple device brands, either CF-LVAD or PF-LVAD, and found that CF-LVAD was an independent risk factor for the development of AR. Kagawa et al. (15) reported that 30% of their 316 patients, developed AR at 1 year from CF-LVAD implantation. The effect of the different LVAD was explained by Imamura et al. (53). According to the authors, PF-LVAD unloads the LV only during the diastolic time, whereas CF-LVAD unloads the LV throughout the all cardiac cycle with a constant transvalvular pressure less than 0 mmHg that avoid AV opening. The time of AV opening was higher, AR rate lower, LV diameter in diastole lower and higher LV ejection fraction in PF-LVAD patients compare to CF-LVAD. It seems that CF-LVAD per se has a LV remodeling effect that ultimately contributes to the development of AR. Moreover, complete LV unloading is associated with higher myocardial fibrosis and cardiac stiffness with decreased coronary flow which promotes inflammation and myocardial fibrosis (54–56).
Although pulsatility may have some advantages, the improvements in size, reliability, efficacy, and durability of the current generation of CF-LVADs have made them the LVAD of choice. The latest generation of CF-LVAD allow some intermittent aortic valve opening for washing of the aortic root. HeartMate 3 LVADs have demonstrated a certain degree of pulse pressure in animal models. Actually it represents 80–90% of all implants since the U.S. FDA approval in October 2018 (57).
However, AR, as said, develops over time in CF-rotatory-LVAD pump and the recirculation of blood flow is more severe in CF than PF system with more severe reduction in blood flow to the end organs and signs of HF, despite the increase of LVAD speed. For these reasons, AR more than mild should be addressed before or during support device implantation (58).
Newer LVAD’s have built-in artificial pulsatile, but their impact on the AV remains to be established (CorWave has been awarded the Medtech Award 2021) (59).
The altered physiological mechanics of the heart, resulting from LVAD-host integration might cause the development of AV dysfunction (60).
Under normal conditions, the aortic root-aortic valve complex guarantees unidirectional, intermittent blood flow under optimal conditions, with laminar flow, minimal shear stress and resistance, and complete diastolic coaptation of the AV leaflets (61). Moreover, the AV endothelium has important regulatory functions, acting as a paracrine structure releasing regulatory cytokines (including tumor necrosis factor-α), anti-thrombotic and vasoactive factors (nitric oxide). The AV endothelium is also side-specific, sensitive to local flow and shear stress, with the aortic side exposed to higher flow, pressure, and turbulence than the ventricular side (62).
The high shear stress produced by CF-LVADs is found throughout the whole cardiac cycle, exposing the aortic valve leaflets and aortic wall to continuous turbulent flow and higher arterial pressures. Normally, the valve leaflets relax in the open position when stretch and stress are low, allowing nutrient flow to the tissue. With CF-LVADs, the leaflets are usually persistently closed, so that the valve leaflets are under maximum stretch with a continuously high transvalvular gradient. This provides a stimulus for collagen production and increased inflammation, causing adverse remodeling of the valve leaflets, with retraction, degenerative involvement, and focal nodular calcification (20, 35, 63). In contrast to what naturally occurs in AV disease, focal lesions occur on the ventricular side of the AV as a consequence of valve dysregulation due to constant high transvalvular pressure (60). Post-transplant autoptic evaluation of LVAD hearts, revealed extensive tissue remodeling of the AV, in particular commissure fusion in 71–88% of patients (64, 65). In addition, it is also postulated that, as a consequence of the closed aortic valve cusps and blood stasis on the ventricular side, thrombus formation is more likely (66), along with forming a commissural fusion and accelerating degenerative processes of the AV (60, 67, 68).
Shear stress can also affect the aortic wall, as the outflow graft can be smaller than the native aorta, with higher velocity and flow. The consequence of this process is aortic dilatation, with progressive thinning of the aorta media layer, decrease in smooth muscle cells and elastic fibers of the media layer (69–71). Finally, the implantation position of the outflow anastomosis is also important, suggested to be 2 cm above the sino-tubular junction and more than 90 degree inclination, to reduce blood flow stagnation in the aortic root and thrombi formation with normal wall shear stress and moderate local pressure values (72). If the cannula is too far away from the AV, in the ascending aorta or descending aorta, the reduced blood washout near the AV can promote thrombus formation (73). On the other hand, if the outflow cannula is attached too close to the AV, can be responsible of the AV cusps distortion and mal-coaptation due to increased local pressure, diastolic transvalvular pressure gradient promoting AR (74, 75).
Absence of AV opening, distortion of AV cusps and dilatation of the aortic root are factors that predispose to de novo AR and worsening of pre-existing AR. This pathophysiology is seen in animal models, leading to aortic atrophy and worsening AR (69).
In addition to the factors related to the interaction LVAD-host, there are also patient factors that predispose to developing AR. In the metanalysis of Deo et al. (76), that considered 7 observational studies (657 patients), 65% of the them used CFLVAD, it was found that pre-operative parameters affecting the development of AR were older age, female sex, and low body surface area. Mitral regurgitation was also independently related to the development of AR. Post-LVAD implantation factors that played a role in the development of AR were larger aortic root and aortic sinus diameter, closed aortic valve and longer duration of support (15, 16, 76).
According to European Society of Cardiology (ESC)/American Society of Echocardiography (ASE) guidelines (77), it is crucial to evaluate the structure and functions of cardiac muscle and valves, with a keen focus on AV, using transthoracic (TTE) and/or transesophageal (TEE) echocardiography before LVAD implantation, during implantation and follow-up. Echocardiography, either TTE or TEE, are sensitive enough to detect valve anatomy and function. In Table 3 are summarized the measurements that have to be taken to select the patient for implantation and eventually plan other cardiac procedures (i.e., ASD closure). Table 4 reports the specific measurements for AR stratification while Table 5 illustrates the timing and parameters to be evaluated during implantation, post-procedure, and during follow-up (12, 20).
Table 3. Echocardiographic parameters pre-left ventricular assist device (LVAD) implantation (80, 120).
Table 4. Aortic regurgitation (AR) evaluation pre-left ventricular assist device (LVAD) implantation (78, 121–123).
Table 5. Intraoperative evaluation and post-left ventricular assist device (LVAD) (77).
Stratification of AR severity pre-LVAD implantation is fundamental for patient management and should be done according to guidelines for accurate long-term prognosis since some of the parameters are predictors of future development of AR (78–80). Table 3 summarizes all the TTE parameters that should be considered and reported during the pre-implantation assessment. Patients with moderate to severe AR require surgical intervention (repair or replacement of the aortic valve). It is always important to remember in AR that the pressure difference between the two chambers (LV and aorta), the diastolic characteristic of the LV, and the LV volume all have an impact on the regurgitant flow. If the AR assessment by TEE is performed under sedation or general anesthesia, it is possible that there is a high LV diastolic pressure, low systemic resistances, and a small pressure gradient across the LV-aorta, which underestimates the degree of AR and can be avoided by increasing the blood pressure. As previously stated, sinus of Valsalva, sinus tubular junction and ascending aortic diameter should be documented since they are predictors of future AR development (Tables 3, 4).
Table 5 lists the variables to be verified during the procedure and the timing of the evaluation. During LVAD implantation transesophageal echocardiography (TEE) will be used either for the bi-ventricular function evaluation or AV function or correct alignment of the inflow-outflow cannula. The increased severity of AR following LVAD implantation necessitates a referral to the surgeon. The reason might be underestimation of AR during preimplantation or high suction of the inflow cannula (high pump speed). In terms of AV evaluation, it is critical to determine if AV opens, quantifying the opening (intermittent, continuous, by M-Mode), and the severity of AR if present. The optimal pump speed is the one that allows for at least intermittent AV opening (77).
Traditional TTE tends to underestimate the severity of AR as it occurs throughout the cardiac cycle due to insufficient residual LV contractile forces to oppose the backflow in systole, thus even a relatively small AV orifice can account for severe AR. Aortic flow, as a result, becomes highly dependent on global hemodynamics such as LV pre-load, residual contractility, and heart rate (77). Furthermore, the quantitative parameters (pressure half time, vena contracta, proximal iso-velocity surface area) are unreliable in this context since the determinant factor to the assessment of regurgitant severity in a CF-LVAD patient is the measure of flow over time. In addition to the traditional parameters, other specific measurements are suggested to improve AR severity stratification such as those reported in Table 5. For example, pulsed wave (PW) Doppler of the outflow cannula is important for documenting laminar, unidirectional, low-peak velocity flows, and no regurgitation. The PW sample volume is placed at least 1 cm from the anastomosis (Table 5) and a pulsatile flow pattern is characterized by phasic changes in flow throughout the cardiac cycle, reaching the maximum during systole and minimum during diastole. This signal can be used to calculate two parameters: (1) peak systolic-to-diastolic (S/D) velocity ratio, which correlates negatively with AR, and (2) diastolic acceleration time, which correlates positively with AR (Figure 3) (81, 82). Diastolic acceleration time is the diastolic slope from the onset to the end of diastole and the S/D ratio is calculated by dividing the peak systolic velocity by the end diastolic peak velocity. An S/D ratio less than 5.0 and/or a diastolic acceleration time greater than 49.0 cm/s corresponds to at least moderate AR, defined as a regurgitant fraction > 30%. The relationship between diastolic flow on the outflow cannula and AR is self-evident: as AR worsens, diastolic flow through the outflow cannula increases (81). However, in our experience, it is not always easy to be accurate since the outflow cannula might not be seen and/or the PW sample is not aligned with the flow of the cannula.
Figure 3. (A) Severe continuous (holo-systolic and holo-diastolic) aortic regurgitation (AR). Green arrow: outflow cannula. Pulse Doppler (PW) at about 1 cm from the outflow cannula (bold green arrow). Diastolic acceleration time 49 ms (orange line), S/D ratio 93/41 = 2.3 (<5 significant AR) (Yellow head arrow: systolic wave; green head arrow: end Diastolic velocity). Acceleration time 240 ms. (B) No AR. S/D 53/10 = 5. Acceleration time 49.
As previously mentioned, the duration of AR should be considered when the AR is quantified (measure of flow over time). For this reason, the calculation of regurgitant volume (RVol) can be estimated by measuring PISA by M-mode, AR by CW, and the duration of AR (ms) adjusted by heart rate (83) (Table 5).
Importantly, the degree of AV opening can be significantly reduced or intermittent, depending on the LVAD speed. Ideally, LVAD support aims to open the aortic valve every two or three beats. M-Mode of the AV long-axis can help to quantify the degree of AV opening (intermittent opening, in which part of the cardiac cycle, extend AV opening) and if color is added also the AR characteristics (extent of AR into systolic period -electrical and mechanical-) It is suggested to acquire at low speed (25 mm/s) and at least 3–5 cardiac cycles.
Following LVAD implantation, it is recommended to perform routine TTE according to the algorithm shown in the flow chart (Figure 4).
Standard comprehensive echocardiography will be performed, with a particular emphasis on LV and RV dimensions, systolic function, inflow and outflow cannula interrogation, valvular apparatus included AV function (AR or aortic stenosis, AV normally open or closure time and its relationship with the cardiac cycle) (Figure 5). It is recommended to record 3–5 cycles. TTE should be performed according to the guidelines and remain consistent during the follow-up (77, 78). Since the quantification of AR in LVAD patients is quite challenging, a multiparametric approach is mandatory.
Figure 5. Upper part: (A) No aortic regurgitation (AR); (B) trivial AR; (C) mild AR; (D) severe continuous AR (VC 4 mm). Lower part: (E) Closed AV; (F) aortic valve open in end diastole and systole. No AR; (G) aortic valve opened in systole. No AR; (H) same patient of panel (D). M-Mode showing severe systolic-diastolic AR. The valve was closed during the cardiac cycle.
The consequence of AR determines backward flow through the AV during diastole that contribute to energy loss and reducing systemic flow and worsening HF. The re-entering of blood in the LVAD causes a vicious regurgitant flow loop with the consequence that the pump needs to run at a higher speed to maintain the cardiac output and extend the time of shear stress to the blood increasing hemolysis and thrombogenicity (84). Aortic insufficiency can be managed before or after LVAD implantation, according to the severity of regurgitation.
If the AR is mild, the patient is treated medically. When AR is more than mild, AV needs to be tackled before or at the time of device implantation to prevent its progression. The intervention of choice is AV replacement with a bio-prosthesis as described below. If the patient already has an AV replaced with a mechanical valve at the time of device implantation, it is recommended to replace it with a bioprosthetic valve, or bypass the valve from the circulation to avoid thromboembolic complications (74, 85). To the best of our knowledge, there are no studies or case report reporting the experience of transcutaneous aortic valve implantation (TAVI) in pre-LVAD patients. This is most likely because TAVI in AR is an off-label procedure, as explained later, and standard aortic valve replacement (SAVR) is often the best option.
Patients with moderate to severe AR after device implantation should be promptly treated since it affects the patient either clinically or prognostically as previously explained (Figure 3) (12–15, 60). When approaching a patient with AR, it is important to determine whether he/she is asymptomatic or symptomatic. When an invasive approach is needed, the options include AV replacement with a bioprosthetic valve by SAVR or permanent AV closure or TAVI.
Management indications are still unclear for patients developing AR on LVAD. Since there is a clear association between the development of AR secondary to permanently closed AV, it is suggested to adjust the LVAD settings to lower pump speed to obtain intermittent AV opening and reducing the risk of de novo AR or AR progression (12). On the other hand, it is important to keep in mind that once the LVAD pump speed is reduced other potential complications, such as HF symptoms, increasing LV dimensions, increase MR severity, and LV filling pressure can occur.
Medical management, as described below, can help in AR improvement.
In this case, there are two options, non-surgical and surgical.
1. Medical management can be a starting option, targeting fluid overload with diuretics, reducing afterload with vasodilators (12) along with utilizing angiotensin-converting enzyme inhibitors, calcium channel blockers, or beta blockers (86).
2. Device settings can be adjusted to the lowest speed for intermittent AV opening and improved best functional class (20) but is rarely sufficient (86). Intermittent low-speed algorithm has been developed to simulate normal physiological conditions and AV valve movement, reducing the likelihood of de novo AR or AR progression (43, 87).
If LVAD is expected to be implanted for more than 1 year (12), different AV procedures are performed for different AV pathologies. For degenerative disease (cusp prolapse or malcoaptation) the closure of the AV can be considered while the replacement with a bioprosthetic valve is recommended for calcified valves (12, 88). Bioprosthetic valve is considered to be favorable, however, due to a longer cardioplegic arrest, there is the potential for thromboembolic complications of the prosthesis (89–91), along with increased operative risk and a fivefold increase in 30-day mortality (92). Replacement with a mechanical valve is contraindicated because of the high risk of thrombosis (93). AV closure is contraindicated in patients with a plan of temporary LVAD since it leaves the patients completely dependent on the device and can lead to disastrous complications in those developing pump thrombosis or malfunction (12). Important to note is that patients with AV procedure and LVAD implantation at the same time have higher peri-operative and early mortality, but similar long-term outcomes to patients without simultaneous procedures (94). Furthermore, a recent minimally invasive technique of LVAD implantation with concomitant transapical transcatheter aortic valve replacement through a hybrid process has shown good early outcomes as it preserves the pericardial geometry and eliminates the need for cardioplegic arrest (95).
Importantly, AR can re-develop within the first year in up to 20% of patients having simultaneous AV procedures at the time of LVAD implantation (19% with AV repair, 5% for AV closure, and 9% after AV replacement) (94).
Prohibitive surgical risk patients can be candidates for percutaneous techniques including percutaneous occluding devices (PODs) or transcatheter aortic valve implantation (TAVI) (96–99). Although TAVI technique at the moment is considered the last line of treatment due to significant complications including mortality, it does look promising and will probably become the first choice of treatment in the near future. Moreover, research effort is put in developing new transcatheter heart valves (THV) dedicated specifically to AR (100, 101). The case of TAVI in AR, is an off-label procedure given that a non-calcific AR is the most common characteristic. In this case AR can be associated with aortic dilatation, aortic annulus enlargement in association with advanced LV and/or RV dysfunction and remodeling. Schneeberger et al. (102), used a self-expanding THV in 9 patients reporting no mortality at 30 days, two acute kidney injury and 2 patients with mild paravalvular leak. Belkin et al. (103) had a more complicated experience. Seven patients underwent TAVI: 2 patients had two procedures; 2 patients had an inadequate fixation with severe paravalvular leak that evolved into cardiogenic shock and death within the first day. In the rest of the 5 patients with successful valve deployment, AR improved significantly. Residual mild or moderate paravalvular leak was noted on five of the six surviving patients on immediate post-procedure TTE as well as at 6-month follow-up. Phan et al. (96) identified 29 patients from 2,116 electronic database search. Eight patients underwent TAVI and 21 POD. The results were similar in terms of AR improvement after the procedure. The POD group was complicated by migration of the device in 2 patients, TAVI group was complicated by device migration in 2 patients and 1 had significant post-implant paravalvular leak. The survival of patients with TAVI at 20 months was 35% while no patient survived beyond 20 months in POD group. A recent study on 148 hospitalized patients with a history of LVAD, compared the outcome of those who underwent TAVI or SAVR (87 TAVR vs. 61 SAVR). The 30-day all-cause readmission rate was numerically higher in the SAVR group, but the difference was not statistically significant, as well as the difference in mortality (104).
The most common complications associated with TAVI procedures are embolization of the device into the aorta or migration of the prosthesis in the left ventricle (due to the absence of calcium for stabilizing the valve and the vacuum effect of the inflow cannula), as well as the intra-and paravalvular leaks that either occurs after the valve is released or develop during the follow-up period and call for additional intervention (Figure 6). Prior to TAVI, right ventricular dysfunction, dilatation, and pulmonary hypertension—even those that are not clinically evident—must be carefully assessed. Even though there is not enough data in literature, it is required to use medications like milrinone, nitric oxide, and phosphodiesterase inhibitors prior to the procedure along with carefully managing fluid overload during the procedure to lower the risk of RV failure following TAVI. The main causes of periprocedural death are extensive, widespread organ damage and RV failure (kidney, liver) (105). Table 6 summarizes all the different management options with advantages and disadvantages.
Figure 6. Transcatheter aortic valve implantation (TAVI) procedure. 32 years old gentleman with severe dilated cardiomyopathy, severe reduced systolic function (Ejection Fraction less than 10%), severe dilated and reduce systolic function of the right ventricle (RV), on left ventricular assist device (LVAD) as Bridge To Therapy (BTT) developed severe aortic regurgitation (AR). On transthoracic (TTE) the patients also had severely dilated RV, severe tricuspid regurgitation (TR) and associated severe liver and kidney dysfunction on hemodialysis. The patient underwent TAVI procedure with mild to moderate residual intravalvular AR. Five days after the procedure, TTE was repeated showing the presence of severe PV leak and the possible protrusion of the prosthesis into left ventricular outflow tract (LVOT). The patient died after 10 days from the procedure due to end stage heart failure and multi organ failure. (A) Pre-TAVI implantation, (B) TTE, mild to moderate paravalvular leak during TAVI procedure and before aortic valve (AV) deployment, (C) parasternal long axis TTE just after deployment, mild to moderate intra and paravalvular leak, (D–F) 5 days later S/P TAVR with likely severe intra and paravalvular leak, possible protrusion of the AV prosthesis into LV. (D) Color M-mode with AR in systole and diastole. (E) Shot axis. (F) Parasternal long axis.
Table 6. Managing aortic regurgitation (AR) complication that develops post-left ventricular assist device (LVAD) transplantation (12, 89–92).
The ability of LVAD support to function as a BTT, BTC, and DT has greatly increased its appeal in recent years. A major concern with LVAD implantation is the development of de novo AR, which is estimated to have a prevalence of 10–55% in the first 6 months, and the worsening of pre-existing AR. The AV is considerably impacted by the LVAD; hence, this factor should be carefully considered along with the aortic dimension. The specific hemodynamics of AR make it difficult for TTE/TEE to accurately identify this condition, which is crucial because AR affects mortality and morbidity. It is advised that AR be addressed at the time of LVAD implantation if it is moderate or worse before the implantation. Intervention must be taken into consideration if moderate or worse AR occurs after implantation. Non-invasive, medical, and/or LVAD pump modification settings, or invasive management options are available. Since patients frequently have advanced HF with severely compromised end organ damage and high-risk surgery, TAVI is regarded as a possible alternative to invasive therapies, even though it is currently off-label.
OV, AM, AS, and AE-S equally contributed in writing the manuscript and editing. KF, AE, HA, NK, MA, DA-B, ARS, and FT assisted in visualization and editing. All authors contributed to the article and approved the submitted version.
The authors declare that the research was conducted in the absence of any commercial or financial relationships that could be construed as a potential conflict of interest.
All claims expressed in this article are solely those of the authors and do not necessarily represent those of their affiliated organizations, or those of the publisher, the editors and the reviewers. Any product that may be evaluated in this article, or claim that may be made by its manufacturer, is not guaranteed or endorsed by the publisher.
1. Truby L, Rogers J. Advanced heart failure: epidemiology, diagnosis, and therapeutic approaches. JACC Heart Fail. (2020) 8:523–36. doi: 10.1016/j.jchf.2020.01.014
2. Lund L, Edwards L, Kucheryavaya A, Dipchand A, Benden C, Christie J, et al. The registry of the international society for heart and lung transplantation: thirtieth official adult heart transplant report–2013; focus theme: age. J Heart Lung Transplant. (2013) 32:951–64. doi: 10.1016/j.healun.2013.08.006
3. Frazier O, Rose E, Oz M, Dembitsky W, McCarthy P, Radovancevic B, et al. Multicenter clinical evaluation of the HeartMate vented electric left ventricular assist system in patients awaiting heart transplantation. J Thorac Cardiovasc Surg. (2001) 122:1186–95. doi: 10.1067/mtc.2001.118274
4. Peura J, Colvin-Adams M, Francis G, Grady K, Hoffman T, Jessup M, et al. Recommendations for the use of mechanical circulatory support: device strategies and patient selection: a scientific statement from the American heart association. Circulation. (2012) 126:2648–67. doi: 10.1161/CIR.0b013e3182769a54
5. Struber M, Lange R, Gummert J, Beyersdorf F, Jurmann M, Schmid C, et al. Alternatives to heart transplantation. Symposium of the “Treatment of End-stage Heart and Lung Failure” working group on October 22, 2005 in Munich. Thorac Cardiovasc Surg. (2007) 55(Suppl. 2):S147–67. doi: 10.1055/s-2007-965414
6. Rose E, Gelijns A, Moskowitz A, Heitjan D, Stevenson L, Dembitsky W, et al. Long-term use of a left ventricular assist device for end-stage heart failure. N Engl J Med. (2001) 345:1435–43. doi: 10.1056/NEJMoa012175
7. Slaughter M, Rogers J, Milano C, Russell S, Conte J, Feldman D, et al. Advanced heart failure treated with continuous-flow left ventricular assist device. N Engl J Med. (2009) 361:2241–51. doi: 10.1056/NEJMoa0909938
8. Rogers J, Pagani F, Tatooles A, Bhat G, Slaughter M, Birks E, et al. Intrapericardial left ventricular assist device for advanced heart failure. N Engl J Med. (2017) 376:451–60. doi: 10.1056/NEJMoa1602954
9. Heidenreich P, Bozkurt B, Aguilar D, Allen L, Byun J, Colvin M, et al. 2022 AHA/ACC/HFSA Guideline for the management of heart failure: a report of the American college of cardiology/American heart association joint committee on clinical practice guidelines. Circulation. (2022) 145:e895–1032. doi: 10.1161/CIR.0000000000001073
10. Miller W, Hodge D, Tointon S, Rodeheffer R, Nelson S, Gibbons R. Relationship of myocardial perfusion imaging findings to outcome of patients with heart failure and suspected ischemic heart disease. Am Heart J. (2004) 147:714–20. doi: 10.1016/j.ahj.2003.10.045
11. Rao V, Slater J, Edwards N, Naka Y, Oz M. Surgical management of valvular disease in patients requiring left ventricular assist device support. Ann Thorac Surg. (2001) 71:1448–53. doi: 10.1016/S0003-4975(01)02479-1
12. Cowger J, Rao V, Massey T, Sun B, May-Newman K, Jorde U, et al. Comprehensive review and suggested strategies for the detection and management of aortic insufficiency in patients with a continuous-flow left ventricular assist device. J Heart Lung Transplant. (2015) 34:149–57. doi: 10.1016/j.healun.2014.09.045
13. Cowger J, Aaronson K, Romano M, Haft J, Pagani F. Consequences of aortic insufficiency during long-term axial continuous-flow left ventricular assist device support. J Heart Lung Transplant. (2014) 33:1233–40. doi: 10.1016/j.healun.2014.06.008
14. Truby L, Garan A, Givens R, Wayda B, Takeda K, Yuzefpolskaya M, et al. Aortic insufficiency during contemporary left ventricular assist device support: analysis of the INTERMACS registry. JACC Heart Fail. (2018) 6:951–60. doi: 10.1016/j.jchf.2018.07.012
15. Kagawa H, Aranda-Michel E, Kormos R, Keebler M, Hickey G, Wang Y, et al. Aortic insufficiency after left ventricular assist device implantation: predictors and outcomes. Ann Thorac Surg. (2020) 110:836–43. doi: 10.1016/j.athoracsur.2019.12.030
16. Holley C, Fitzpatrick M, Roy S, Alraies M, Cogswell R, Souslian L, et al. Aortic insufficiency in continuous-flow left ventricular assist device support patients is common but does not impact long-term mortality. J Heart Lung Transplant. (2017) 36:91–6. doi: 10.1016/j.healun.2016.07.018
17. Patil N, Sabashnikov A, Mohite P, Garcia D, Weymann A, Zych B, et al. De novo aortic regurgitation after continuous-flow left ventricular assist device implantation. Ann Thorac Surg. (2014) 98:850–7. doi: 10.1016/j.athoracsur.2014.05.030
18. Tanaka Y, Nakajima T, Fischer I, Wan F, Kotkar K, Moon M, et al. The impact of uncorrected mild aortic insufficiency at the time of left ventricular assist device implantation. J Thorac Cardiovasc Surg. (2020) 160:1490.e–500.e. doi: 10.1016/j.jtcvs.2020.02.144
19. Holtz J, Teuteberg J. Management of aortic insufficiency in the continuous flow left ventricular assist device population. Curr Heart Fail Rep. (2014) 11:103–10. doi: 10.1007/s11897-013-0172-6
20. Bouabdallaoui N, El-Hamamsy I, Pham M, Giraldeau G, Parent M, Carrier M, et al. Aortic regurgitation in patients with a left ventricular assist device: a contemporary review. J Heart Lung Transplant. (2018) 37:1289–97. doi: 10.1016/j.healun.2018.07.002
21. Jorde U, Uriel N, Nahumi N, Bejar D, Gonzalez-Costello J, Thomas S, et al. Prevalence, significance, and management of aortic insufficiency in continuous flow left ventricular assist device recipients. Circ Heart Fail. (2014) 7:310–9. doi: 10.1161/CIRCHEARTFAILURE.113.000878
22. Shah K, Tang D, Cooke R, Harton S, Flattery M, Katlaps G, et al. Implantable mechanical circulatory support: demystifying patients with ventricular assist devices and artificial hearts. Clin Cardiol. (2011) 34:147–52. doi: 10.1002/clc.20825
23. Shah P, Yuzefpolskaya M, Hickey G, Breathett K, Wever-Pinzon O, Ton V, et al. Twelfth interagency registry for mechanically assisted circulatory support report: readmissions after left ventricular assist device. Ann Thorac Surg. (2022) 113:722–37. doi: 10.1016/j.athoracsur.2021.12.011
24. Mehra M, Goldstein D, Uriel N, Cleveland J Jr., Yuzefpolskaya M, Salerno C, et al. Two-year outcomes with a magnetically levitated cardiac pump in heart failure. N Engl J Med. (2018) 378:1386–95. doi: 10.1056/NEJMoa1800866
25. McDonagh T, Metra M, Adamo M, Gardner R, Baumbach A, Bohm M, et al. 2021 ESC Guidelines for the diagnosis and treatment of acute and chronic heart failure. Eur Heart J. (2021) 42:3599–726.
26. Golbus J, Gupta K, Colvin M, Cascino T, Aaronson K, Kumbhani D, et al. Changes in type of temporary mechanical support device use under the new heart allocation policy. Circulation. (2020) 142:1602–4. doi: 10.1161/CIRCULATIONAHA.120.048844
27. Hanff T, Harhay M, Kimmel S, Molina M, Mazurek J, Goldberg L, et al. Trends in mechanical support use as a bridge to adult heart transplant under new allocation rules. JAMA Cardiol. (2020) 5:728–9. doi: 10.1001/jamacardio.2020.0667
29. Brinkley D, DeNofrio D, Ruthazer R, Vest A, Kapur N, Couper G, et al. Outcomes after continuous-flow left ventricular assist device implantation as destination therapy at transplant versus nontransplant centers. Circ Heart Fail. (2018) 11:e004384. doi: 10.1161/CIRCHEARTFAILURE.117.004384
30. Kirklin J, Naftel D, Pagani F, Kormos R, Stevenson L, Blume E, et al. Seventh INTERMACS annual report: 15,000 patients and counting. J Heart Lung Transplant. (2015) 34:1495–504. doi: 10.1016/j.healun.2015.10.003
31. Theochari C, Michalopoulos G, Oikonomou E, Giannopoulos S, Doulamis I, Villela M, et al. Heart transplantation versus left ventricular assist devices as destination therapy or bridge to transplantation for 1-year mortality: a systematic review and meta-analysis. Ann Cardiothorac Surg. (2018) 7:3–11. doi: 10.21037/acs.2017.09.18
32. Zimpfer D, Gustafsson F, Potapov E, Pya Y, Schmitto J, Berchtold-Herz M, et al. Two-year outcome after implantation of a full magnetically levitated left ventricular assist device: results from the ELEVATE registry. Eur Heart J. (2020) 41:3801–9. doi: 10.1093/eurheartj/ehaa639
33. Mehra M, Goldstein D, Cleveland J, Cowger J, Hall S, Salerno C, et al. Five-year outcomes in patients with fully magnetically levitated vs axial-flow left ventricular assist devices in the MOMENTUM 3 randomized trial. JAMA. (2022) 328:1233–42. doi: 10.1001/jama.2022.16197
34. Dandel M, Weng Y, Siniawski H, Stepanenko A, Krabatsch T, Potapov E, et al. Heart failure reversal by ventricular unloading in patients with chronic cardiomyopathy: criteria for weaning from ventricular assist devices. Eur Heart J. (2011) 32:1148–60. doi: 10.1093/eurheartj/ehq353
35. Cowger J, Pagani F, Haft J, Romano M, Aaronson K, Kolias T. The development of aortic insufficiency in left ventricular assist device-supported patients. Circ Heart Fail. (2010) 3:668–74. doi: 10.1161/CIRCHEARTFAILURE.109.917765
36. Pak S, Uriel N, Takayama H, Cappleman S, Song R, Colombo P, et al. Prevalence of de novo aortic insufficiency during long-term support with left ventricular assist devices. J Heart Lung Transplant. (2010) 29:1172–6. doi: 10.1016/j.healun.2010.05.018
37. Gasparovic H, Jakus N, Brugts J, Pouleur A, Timmermans P, Rubis P, et al. Impact of progressive aortic regurgitation on outcomes after left ventricular assist device implantation. Heart Vessels. (2022) 37:1985–94.
38. Naganuma M, Akiyama M, Sasaki K, Maeda K, Ito K, Suzuki T, et al. Aortic insufficiency causes symptomatic heart failure during left ventricular assist device support. Tohoku J Exp Med. (2021) 255:229–37. doi: 10.1620/tjem.255.229
39. Imamura T, Kinugawa K, Fujino T, Inaba T, Maki H, Hatano M, et al. Aortic insufficiency in patients with sustained left ventricular systolic dysfunction after axial flow assist device implantation. Circ J. (2015) 79:104–11. doi: 10.1253/circj.CJ-14-0944
40. Auvil B, Chung J, Ameer A, Han J, Helmers M, Birati E, et al. Moderate aortic insufficiency with a left ventricular assist device portends a worse long-term survival. ASAIO J. (2020) 66:780–5. doi: 10.1097/MAT.0000000000001071
41. da Rocha E, Meyer A, Eifert S, Garbade J, Mohr F, Strueber M. Influence of aortic valve opening in patients with aortic insufficiency after left ventricular assist device implantation. Eur J Cardiothorac Surg. (2016) 49:784–7. doi: 10.1093/ejcts/ezv204
42. Park J, Brady P, Clavell A, Maleszewski J, Nkomo V, Pislaru S, et al. Predictors and long-term impact of de novo aortic regurgitation in continuous flow left ventricular assist devices using vena contracta. ASAIO J. (2022) 68:691–7. doi: 10.1097/MAT.0000000000001564
43. Saeed D, Westenfeld R, Maxhera B, Keymel S, Sherif A, Sadat N, et al. Prevalence of de novo aortic valve insufficiency in patients after heartware VAD implantation with an intermittent low-speed algorithm. ASAIO J. (2016) 62:565–70. doi: 10.1097/MAT.0000000000000391
44. Hiraoka A, Cohen J, Shudo Y, MacArthur J Jr., Howard J, Fairman A, et al. Evaluation of late aortic insufficiency with continuous flow left ventricular assist devicedagger. Eur J Cardiothorac Surg. (2015) 48:400–6. doi: 10.1093/ejcts/ezu507
45. Aggarwal A, Raghuvir R, Eryazici P, Macaluso G, Sharma P, Blair C, et al. The development of aortic insufficiency in continuous-flow left ventricular assist device-supported patients. Ann Thorac Surg. (2013) 95:493–8. doi: 10.1016/j.athoracsur.2012.09.020
46. Soleimani B, Haouzi A, Manoskey A, Stephenson E, El-Banayosy A, Pae W. Development of aortic insufficiency in patients supported with continuous flow left ventricular assist devices. ASAIO J. (2012) 58:326–9. doi: 10.1097/MAT.0b013e318251cfff
47. Toda K, Fujita T, Domae K, Shimahara Y, Kobayashi J, Nakatani T. Late aortic insufficiency related to poor prognosis during left ventricular assist device support. Ann Thorac Surg. (2011) 92:929–34. doi: 10.1016/j.athoracsur.2011.04.115
48. Bhagra S, Bhagra C, Ozalp F, Butt T, Ramesh B, Parry G, et al. Development of de novo aortic valve incompetence in patients with the continuous-flow HeartWare ventricular assist device. J Heart Lung Transplant. (2016) 35:312–9. doi: 10.1016/j.healun.2015.10.022
49. Koenig S, Pantalos G, Gillars K, Ewert D, Litwak K, Etoch S. Hemodynamic and pressure-volume responses to continuous and pulsatile ventricular assist in an adult mock circulation. ASAIO J. (2004) 50:15–24. doi: 10.1097/01.MAT.0000104816.50277.EB
50. Bartoli C, Giridharan G, Litwak K, Sobieski M, Prabhu S, Slaughter M, et al. Hemodynamic responses to continuous versus pulsatile mechanical unloading of the failing left ventricle. ASAIO J. (2010) 56:410–6. doi: 10.1097/MAT.0b013e3181e7bf3c
51. Garcia S, Kandar F, Boyle A, Colvin-Adams M, Lliao K, Joyce L, et al. Effects of pulsatile- and continuous-flow left ventricular assist devices on left ventricular unloading. J Heart Lung Transplant. (2008) 27:261–7. doi: 10.1016/j.healun.2007.12.001
52. Hatano M, Kinugawa K, Shiga T, Kato N, Endo M, Hisagi M, et al. Less frequent opening of the aortic valve and a continuous flow pump are risk factors for postoperative onset of aortic insufficiency in patients with a left ventricular assist device. Circ J. (2011) 75:1147–55. doi: 10.1253/circj.CJ-10-1106
53. Imamura T, Kinugawa K, Nitta D, Hatano M, Kinoshita O, Nawata K, et al. Advantage of pulsatility in left ventricular reverse remodeling and aortic insufficiency prevention during left ventricular assist device treatment. Circ J. (2015) 79:1994–9. doi: 10.1253/circj.CJ-15-0419
54. Ootaki Y, Kamohara K, Akiyama M, Zahr F, Kopcak M Jr., Dessoffy R, et al. Phasic coronary blood flow pattern during a continuous flow left ventricular assist support. Eur J Cardiothorac Surg. (2005) 28:711–6. doi: 10.1016/j.ejcts.2005.08.008
55. Muranaka H, Marui A, Tsukashita M, Wang J, Nakano J, Ikeda T, et al. Prolonged mechanical unloading preserves myocardial contractility but impairs relaxation in rat heart of dilated cardiomyopathy accompanied by myocardial stiffness and apoptosis. J Thorac Cardiovasc Surg. (2010) 140:916–22. doi: 10.1016/j.jtcvs.2010.02.006
56. Kinoshita M, Takano H, Taenaka Y, Mori H, Takaichi S, Noda H, et al. Cardiac disuse atrophy during LVAD pumping. ASAIO Trans. (1988) 34:208–12.
57. Molina E, Shah P, Kiernan M, Cornwell W III, Copeland H, Takeda K, et al. The society of thoracic surgeons intermacs 2020 annual report. Ann Thorac Surg. (2021) 111:778–92. doi: 10.1016/j.athoracsur.2020.12.038
58. Hetzer R, Javier M, Dandel M, Loebe M, Javier Delmo E. Mechanical circulatory support systems: evolution, the systems and outlook. Cardiovasc Diagn Ther. (2021) 11:309–22. doi: 10.21037/cdt-20-283
59. DAIC. Innovative New Pulsatile LVAD Wins 2021 HealthTech Award. (2021). Available online at: https://www.dicardiology.com/content/innovative-new-pulsatile-lvad-wins-2021-healthtech-award (accessed october 20, 2021)
60. John R, Mantz K, Eckman P, Rose A, May-Newman K. Aortic valve pathophysiology during left ventricular assist device support. J Heart Lung Transplant. (2010) 29:1321–9. doi: 10.1016/j.healun.2010.06.006
61. Torii R, El-Hamamsy I, Donya M, Babu-Narayan S, Ibrahim M, Kilner P, et al. Integrated morphologic and functional assessment of the aortic root after different tissue valve root replacement procedures. J Thorac Cardiovasc Surg. (2012) 143:1422–8. doi: 10.1016/j.jtcvs.2011.12.034
62. Farrar E, Huntley G, Butcher J. Endothelial-derived oxidative stress drives myofibroblastic activation and calcification of the aortic valve. PLoS One. (2015) 10:e0123257. doi: 10.1371/journal.pone.0123257
63. Mannava S, Moparthy K, Wheeler L, Natarajan V, Zucker S, Fink E, et al. Depletion of deoxyribonucleotide pools is an endogenous source of DNA damage in cells undergoing oncogene-induced senescence. Am J Pathol. (2013) 182:142–51. doi: 10.1016/j.ajpath.2012.09.011
64. May-Newman K, Mendoza A, Abulon D, Joshi M, Kunda A, Dembitsky W. Geometry and fusion of aortic valves from pulsatile flow ventricular assist device patients. J Heart Valve Dis. (2011) 20:149–58.
65. Mudd J, Cuda J, Halushka M, Soderlund K, Conte J, Russell S. Fusion of aortic valve commissures in patients supported by a continuous axial flow left ventricular assist device. J Heart Lung Transplant. (2008) 27:1269–74. doi: 10.1016/j.healun.2008.05.029
66. Cheng A, Williamitis C, Slaughter M. Comparison of continuous-flow and pulsatile-flow left ventricular assist devices: is there an advantage to pulsatility? Ann Cardiothorac Surg. (2014) 3:573–81.
67. El-Hamamsy I, Balachandran K, Yacoub M, Stevens L, Sarathchandra P, Taylor P, et al. Endothelium-dependent regulation of the mechanical properties of aortic valve cusps. J Am Coll Cardiol. (2009) 53:1448–55. doi: 10.1016/j.jacc.2008.11.056
68. Fried J, Nazif T, Colombo P. A new frontier for TAVR: aortic insufficiency in CF-LVAD patients. J Heart Lung Transplant. (2019) 38:927–9. doi: 10.1016/j.healun.2019.06.024
69. Nishimura T, Tatsumi E, Takaichi S, Taenaka Y, Wakisaka Y, Nakatani T, et al. Prolonged nonpulsatile left heart bypass with reduced systemic pulse pressure causes morphological changes in the aortic wall. Artif Organs. (1998) 22:405–10. doi: 10.1046/j.1525-1594.1998.06137.x
70. Yoshida S, Fukushima S, Miyagawa S, Toda K, Sawa Y. Visualization of vortex flow and shear stress in the aortic root during left ventricular assist device support. J Thorac Cardiovasc Surg. (2017) 154:877.e–8.e. doi: 10.1016/j.jtcvs.2017.01.060
71. Conlin A, Parnes L. Treatment of sudden sensorineural hearing loss: I. A systematic review. Arch Otolaryngol Head Neck Surg. (2007) 133:573–81. doi: 10.1001/archotol.133.6.573
72. Callington A, Long Q, Mohite P, Simon A, Mittal T. Computational fluid dynamic study of hemodynamic effects on aortic root blood flow of systematically varied left ventricular assist device graft anastomosis design. J Thorac Cardiovasc Surg. (2015) 150:696–704. doi: 10.1016/j.jtcvs.2015.05.034
73. May-Newman K, Hillen B, Dembitsky W. Effect of left ventricular assist device outflow conduit anastomosis location on flow patterns in the native aorta. ASAIO J. (2006) 52:132–9. doi: 10.1097/01.mat.0000201961.97981.e9
74. Wang T, Hernandez A, Felker G, Milano C, Rogers J, Patel C. Valvular heart disease in patients supported with left ventricular assist devices. Circ Heart Fail. (2014) 7:215–22. doi: 10.1161/CIRCHEARTFAILURE.113.000473
75. Robert L, Kormos L. Mechanical Circulatory Support: A Companion to Braunwald’s Heart Disease: Expert Consult: Online and Print. 1st ed. Philadelphia, PA: Saunders (2012).
76. Deo S, Sharma V, Cho Y, Shah I, Park S. De novo aortic insufficiency during long-term support on a left ventricular assist device: a systematic review and meta-analysis. ASAIO J. (2014) 60:183–8. doi: 10.1097/MAT.0000000000000042
77. Stainback R, Estep J, Agler D, Birks E, Bremer M, Hung J, et al. Echocardiography in the management of patients with left ventricular assist devices: recommendations from the American society of echocardiography. J Am Soc Echocardiogr. (2015) 28:853–909. doi: 10.1016/j.echo.2015.05.008
78. Zoghbi W, Adams D, Bonow R, Enriquez-Sarano M, Foster E, Grayburn P, et al. Recommendations for noninvasive evaluation of native valvular regurgitation: a report from the American society of echocardiography developed in collaboration with the society for cardiovascular magnetic resonance. J Am Soc Echocardiogr. (2017) 30:303–71.
79. Flores A, Essandoh M, Yerington G, Bhatt A, Iyer M, Perez W, et al. Echocardiographic assessment for ventricular assist device placement. J Thorac Dis. (2015) 7:2139–50.
80. Todaro M, Khandheria B, Paterick T, Umland M, Thohan V. The practical role of echocardiography in selection, implantation, and management of patients requiring LVAD therapy. Curr Cardiol Rep. (2014) 16:468. doi: 10.1007/s11886-014-0468-5
81. Grinstein J, Kruse E, Sayer G, Fedson S, Kim G, Sarswat N, et al. Novel echocardiographic parameters of aortic insufficiency in continuous-flow left ventricular assist devices and clinical outcome. J Heart Lung Transplant. (2016) 35:976–85. doi: 10.1016/j.healun.2016.05.009
82. Vandenberghe S, Segers P, Antaki J, Meyns B, Verdonck P. Hemodynamic modes of ventricular assist with a rotary blood pump: continuous, pulsatile, and failure. ASAIO J. (2005) 51:711–8. doi: 10.1097/01.mat.0000179251.40649.45
83. Han H, Hong E, Tat E, Hahn R, Cobey FA. Novel approach to quantification of aortic regurgitation in left ventricular assist device. J Cardiothorac Vasc Anesth. (2022) 36:1218–20. doi: 10.1053/j.jvca.2021.11.040
84. Kirklin J, Naftel D, Kormos R, Pagani F, Myers S, Stevenson L, et al. Interagency registry for mechanically assisted circulatory support (INTERMACS) analysis of pump thrombosis in the HeartMate II left ventricular assist device. J Heart Lung Transplant. (2014) 33:12–22. doi: 10.1016/j.healun.2013.11.001
85. Cohn W, Demirozu Z, Frazier O. Surgical closure of left ventricular outflow tract after left ventricular assist device implantation in patients with aortic valve pathology. J Heart Lung Transplant. (2011) 30:59–63. doi: 10.1016/j.healun.2010.08.025
86. Takeda K, Ahmad U, Malaisrie S, Lee R, McCarthy P, McGee E Jr. Successful implantation of HeartWare HVAD left ventricular assist device with concomitant ascending and sinus of valsalva aneurysms repair. J Artif Organs. (2012) 15:204–6. doi: 10.1007/s10047-012-0628-6
87. Uriel N, Adatya S, Maly J, Kruse E, Rodgers D, Heatley G, et al. Clinical hemodynamic evaluation of patients implanted with a fully magnetically levitated left ventricular assist device (HeartMate 3). J Heart Lung Transplant. (2017) 36:28–35. doi: 10.1016/j.healun.2016.07.008
88. Dranishnikov N, Stepanenko A, Potapov E, Dandel M, Siniawski H, Mladenow A, et al. Simultaneous aortic valve replacement in left ventricular assist device recipients: single-center experience. Int J Artif Organs. (2012) 35:489–94. doi: 10.5301/ijao.5000102
89. Bryant A, Holman W, Nanda N, Vengala S, Blood M, Pamboukian S, et al. Native aortic valve insufficiency in patients with left ventricular assist devices. Ann Thorac Surg. (2006) 81:e6–8. doi: 10.1016/j.athoracsur.2005.08.072
90. Barbone A, Rao V, Oz M, Naka Y. LVAD support in patients with bioprosthetic valves. Ann Thorac Surg. (2002) 74:232–4. doi: 10.1016/S0003-4975(01)03514-7
91. Feldman C, Silver M, Sobieski M, Slaughter M. Management of aortic insufficiency with continuous flow left ventricular assist devices: bioprosthetic valve replacement. J Heart Lung Transplant. (2006) 25:1410–2. doi: 10.1016/j.healun.2006.10.004
92. Pal J, Klodell C, John R, Pagani F, Rogers J, Farrar D, et al. Low operative mortality with implantation of a continuous-flow left ventricular assist device and impact of concurrent cardiac procedures. Circulation. (2009) 120(Suppl. 11):S215–9. doi: 10.1161/CIRCULATIONAHA.108.844274
93. Feldman D, Pamboukian S, Teuteberg J, Birks E, Lietz K, Moore S, et al. The 2013 international society for heart and lung transplantation guidelines for mechanical circulatory support: executive summary. J Heart Lung Transplant. (2013) 32:157–87. doi: 10.1016/j.healun.2012.09.013
94. Robertson J, Naftel D, Myers S, Prasad S, Mertz G, Itoh A, et al. Concomitant aortic valve procedures in patients undergoing implantation of continuous-flow left ventricular assist devices: an INTERMACS database analysis. J Heart Lung Transplant. (2015) 34:797–805. doi: 10.1016/j.healun.2014.11.008
95. Schaefer A, Treede H, Bernhardt A, Wagner F, Reichenspurner H, Deuse T. Concomitant minimally invasive HVAD and transapical aortic valve implantation. ASAIO J. (2015) 61:209–12. doi: 10.1097/MAT.0000000000000177
96. Phan K, Haswell J, Xu J, Assem Y, Mick S, Kapadia S, et al. Percutaneous transcatheter interventions for aortic insufficiency in continuous-flow left ventricular assist device patients: a systematic review and meta-analysis. ASAIO J. (2017) 63:117–22. doi: 10.1097/MAT.0000000000000447
97. Alkhouli M, Melito B, Ling F. Percutaneous aortic valve closure for patients with left ventricular assist device-associated aortic insufficiency. Catheter Cardiovasc Interv. (2016) 88:1170–3. doi: 10.1002/ccd.26314
98. Parikh K, Mehrotra A, Russo M, Lang R, Anderson A, Jeevanandam V, et al. Percutaneous transcatheter aortic valve closure successfully treats left ventricular assist device-associated aortic insufficiency and improves cardiac hemodynamics. JACC Cardiovasc Interv. (2013) 6:84–9. doi: 10.1016/j.jcin.2012.08.021
99. Retzer E, Sayer G, Fedson S, Nathan S, Jeevanandam V, Friant J, et al. Predictors of survival following trans-catheter aortic valve closure for left ventricular assist device associated aortic insufficiency. Catheter Cardiovasc Interv. (2016) 87:971–9. doi: 10.1002/ccd.26280
100. Costanzo P, Bamborough P, Peterson M, Deva D, Ong G, Fam N. Transcatheter aortic valve implantation for severe pure aortic regurgitation with dedicated devices. Interv Cardiol. (2022) 17:e11. doi: 10.15420/icr.2021.19
101. Kan T, Gu L, Lu H, Cao J, Zhu Z, Wu D, et al. Improved transcatheter aortic valve implantation for aortic regurgitation using a new-type stent: the first preclinical experience. J Cardiothorac Surg. (2020) 15:276. doi: 10.1186/s13019-020-01327-4
102. Schneeberger Y, Seiffert M, Schaefer A, Bhadra O, Schofer N, Pecha S, et al. TAVI for pure non-calcified aortic regurgitation using a self-expandable transcatheter heart valve. Front Cardiovasc Med. (2021) 8:743579. doi: 10.3389/fcvm.2021.743579
103. Belkin M, Imamura T, Fujino T, Kanelidis A, Holzhauser L, Ebong I, et al. Transcatheter aortic valve replacement in left ventricular assist device patients with aortic regurgitation. Struct Heart. (2020) 4:107–12. doi: 10.1080/24748706.2019.1706793
104. Zaidi S, Minhas A, Sagheer S, ManeshGangwani K, Dani S, Goel S, et al. Clinical outcomes of transcatheter aortic valve replacement (TAVR) Vs. surgical aortic valve replacement (SAVR) in patients with durable left ventricular assist device (LVAD). Curr Probl Cardiol. (2022) 47:101313. doi: 10.1016/j.cpcardiol.2022.101313
105. Dhillon A, Jones B, Hodson R, Korngold E. Transcatheter aortic valve replacement for severe aortic regurgitation in patients with a left ventricular assist device. J Invasive Cardiol. (2022) 34:E369–73.
106. Varshney A, DeFilippis E, Cowger J, Netuka I, Pinney S, Givertz M. Trends and outcomes of left ventricular assist device therapy: JACC focus seminar. J Am Coll Cardiol. (2022) 79:1092–107. doi: 10.1016/j.jacc.2022.01.017
107. Klovaite J, Gustafsson F, Mortensen S, Sander K, Nielsen L. Severely impaired von Willebrand factor-dependent platelet aggregation in patients with a continuous-flow left ventricular assist device (HeartMate II). J Am Coll Cardiol. (2009) 53:2162–7. doi: 10.1016/j.jacc.2009.02.048
108. Steinlechner B, Dworschak M, Birkenberg B, Duris M, Zeidler P, Fischer H, et al. Platelet dysfunction in outpatients with left ventricular assist devices. Ann Thorac Surg. (2009) 87:131–7. doi: 10.1016/j.athoracsur.2008.10.027
109. Crow S, John R, Boyle A, Shumway S, Liao K, Colvin-Adams M, et al. Gastrointestinal bleeding rates in recipients of nonpulsatile and pulsatile left ventricular assist devices. J Thorac Cardiovasc Surg. (2009) 137:208–15. doi: 10.1016/j.jtcvs.2008.07.032
110. Acharya D, Loyaga-Rendon R, Morgan C, Sands K, Pamboukian S, Rajapreyar I, et al. INTERMACS analysis of stroke during support with continuous-flow left ventricular assist devices: risk factors and outcomes. JACC Heart Fail. (2017) 5:703–11. doi: 10.1016/j.jchf.2017.06.014
111. Shah P, Birk S, Cooper L, Psotka M, Kirklin J, Barnett S, et al. Stroke and death risk in ventricular assist device patients varies by ISHLT infection category: an INTERMACS analysis. J Heart Lung Transplant. (2019) 38:721–30. doi: 10.1016/j.healun.2019.02.006
112. Mehra M, Crandall D, Gustafsson F, Jorde U, Katz J, Netuka I, et al. Aspirin and left ventricular assist devices: rationale and design for the international randomized, placebo-controlled, non-inferiority ARIES HM3 trial. Eur J Heart Fail. (2021) 23:1226–37. doi: 10.1002/ejhf.2275
113. Bellavia D, Iacovoni A, Scardulla C, Moja L, Pilato M, Kushwaha S, et al. Prediction of right ventricular failure after ventricular assist device implant: systematic review and meta-analysis of observational studies. Eur J Heart Fail. (2017) 19:926–46. doi: 10.1002/ejhf.733
114. Logstrup B, Nemec P, Schoenrath F, Gummert J, Pya Y, Potapov E, et al. Heart failure etiology and risk of right heart failure in adult left ventricular assist device support: the European registry for patients with mechanical circulatory support (EUROMACS). Scand Cardiovasc J. (2020) 54:306–14. doi: 10.1080/14017431.2020.1781239
115. Ziv O, Dizon J, Thosani A, Naka Y, Magnano A, Garan H. Effects of left ventricular assist device therapy on ventricular arrhythmias. J Am Coll Cardiol. (2005) 45:1428–34. doi: 10.1016/j.jacc.2005.01.035
116. Harding J, Piacentino V III, Rothman S, Chambers S, Jessup M, Margulies K. Prolonged repolarization after ventricular assist device support is associated with arrhythmias in humans with congestive heart failure. J Card Fail. (2005) 11:227–32. doi: 10.1016/j.cardfail.2004.08.158
117. Refaat M, Chemaly E, Lebeche D, Gwathmey J, Hajjar R. Ventricular arrhythmias after left ventricular assist device implantation. Pacing Clin Electrophysiol. (2008) 31:1246–52. doi: 10.1111/j.1540-8159.2008.01173.x
118. Gopinathannair R, Cornwell W, Dukes J, Ellis C, Hickey K, Joglar J, et al. Device therapy and arrhythmia management in left ventricular assist device recipients: a scientific statement from the American heart association. Circulation. (2019) 139:e967–89. doi: 10.1161/CIR.0000000000000673
119. Martina J, de Jonge N, Sukkel E, Lahpor J. Left ventricular assist device-related systolic aortic regurgitation. Circulation. (2011) 124:487–8. doi: 10.1161/CIRCULATIONAHA.111.020891
120. Raina A, Seetha Rammohan H, Gertz Z, Rame J, Woo Y, Kirkpatrick J. Postoperative right ventricular failure after left ventricular assist device placement is predicted by preoperative echocardiographic structural, hemodynamic, and functional parameters. J Card Fail. (2013) 19:16–24. doi: 10.1016/j.cardfail.2012.11.001
121. Schoenhagen P, Drude L, Klein H, Garcia M. Quantitative doppler-echocardiographic determination of regurgitant volume in patients with aortic insufficiency. Open Cardiovasc Med J. (2008) 2:12–9. doi: 10.2174/1874192400802010012
122. Lancellotti P, Tribouilloy C, Hagendorff A, Moura L, Popescu B, Agricola E, et al. European association of echocardiography recommendations for the assessment of valvular regurgitation. Part 1: aortic and pulmonary regurgitation (native valve disease). Eur J Echocardiogr. (2010) 11:223–44. doi: 10.1093/ejechocard/jeq030
123. Zoghbi W, Adams D, Bonow R, Enriquez-Sarano M, Foster E, Grayburn P, et al. Recommendations for noninvasive evaluation of native valvular regurgitation a report from the American society of echocardiography developed in collaboration with the society for cardiovascular magnetic resonance. J Indian Acad Echocardiogr Cardiovasc Imaging. (2020) 4:58–121. doi: 10.4103/2543-1463.282191
Keywords: heart transplant, left ventricular assist device (LVAD), aortic regurgitation, transcatheter aortic valve implantation (TAVI), surgical intervention
Citation: Vriz O, Mushtaq A, Shaik A, El-Shaer A, Feras K, Eltayeb A, Alsergnai H, Kholaif N, Al Hussein M, Albert-Brotons D, Simon AR and Tsai FW (2023) Reciprocal interferences of the left ventricular assist device and the aortic valve competence. Front. Cardiovasc. Med. 9:1094796. doi: 10.3389/fcvm.2022.1094796
Received: 10 November 2022; Accepted: 22 December 2022;
Published: 09 January 2023.
Edited by:
Valeria Pergola, University Hospital of Padua, ItalyReviewed by:
Giulia Mattesi, University of Padua, ItalyCopyright © 2023 Vriz, Mushtaq, Shaik, El-Shaer, Feras, Eltayeb, Alsergnai, Kholaif, Al Hussein, Albert-Brotons, Simon and Tsai. This is an open-access article distributed under the terms of the Creative Commons Attribution License (CC BY). The use, distribution or reproduction in other forums is permitted, provided the original author(s) and the copyright owner(s) are credited and that the original publication in this journal is cited, in accordance with accepted academic practice. No use, distribution or reproduction is permitted which does not comply with these terms.
*Correspondence: Olga Vriz, b2xnYXZyaXpAeWFob28uY29t
†These authors have contributed equally to this work and share first authorship
Disclaimer: All claims expressed in this article are solely those of the authors and do not necessarily represent those of their affiliated organizations, or those of the publisher, the editors and the reviewers. Any product that may be evaluated in this article or claim that may be made by its manufacturer is not guaranteed or endorsed by the publisher.
Research integrity at Frontiers
Learn more about the work of our research integrity team to safeguard the quality of each article we publish.