- 1Tianjin Key Laboratory of Ionic-Molecular Function of Cardiovascular Disease, Department of Cardiology, Tianjin Institute of Cardiology, Second Hospital of Tianjin Medical University, Tianjin, China
- 2Department of Cardiology, Tianjin Medical University General Hospital, Tianjin, China
- 3Department of Urology, Second Hospital of Tianjin Medical University, Tianjin, China
Background: Early detection of subclinical cardiotoxicity of immune checkpoint inhibitor (ICI) therapy can be challenging.
Objective: To evaluate subclinical cardiac dysfunction using two-dimensional speckle tracking imaging (2D-STI) and three-dimensional echocardiography in Chinese patients.
Methods: Fifty-five consecutive patients with malignant tumors treated by immunotherapy were included. They were examined by echocardiography before immunotherapy and after immunotherapy. Left ventricular ejection fraction (LVEF) was calculated in three-dimensional imaging. Moreover, left ventricular global longitudinal peak systolic strain (LVGLS), left ventricular global circumferential peak systolic strain (LVGCS), right ventricular global longitudinal systolic strain (RVGLS), right ventricular free wall longitudinal peak systolic strain (RVFWLS), and tricuspid annular plane systolic excursion (TAPSE) were evaluated. Clinical and laboratory parameters were recorded. Cardiac toxicity events were defined as the presence of heart failure symptoms, LVEF reduction, and increase in troponin. Subclinical cardiac toxicity was defined as cardiac dysfunction associated with ICI treatment, with absent or delayed ICI-associated cardiotoxicity clinical symptoms.
Results: Compared with baseline, the LVGLS, TAPSE, and RVGLS significantly deteriorated after ICI treatment [(–18.63 ± 2.53)% vs. (–17.35 ± 2.58)%, P = 0.000; 18.29 ± 6.23 vs. 14.57 ± 3.81, P = 0.0001; and (–18.45 ± 4.65)% vs. (–14.98 ± 3.85)%, P = 0.0001, respectively]. LVGLS (–17.35 ± 2.58, P = 0.000), TAPSE (14.57 ± 3.81, P = 0.0001), and RVGLS [(–14.98 ± 3.85)%, P = 0.0001] were decreased after ICI immunotherapy. Kaplan-Meier curve analysis showed that LVGLS was more sensitive than the cardiac toxicity events to assess ICI-related subclinical cardiac dysfunction (log-rank P = 0.205). The ROC curve showed that the cutoff value of ΔLVGLS was -13%.
Conclusion: Subclinical cardiac dysfunction can be detected using two-dimensional speckle-tracking imaging. LVGLS, RVGLS, and TAPSE are more sensitive indices for detection.
Clinical trial registration: [https://www.chictr.org.cn/showprojen.aspx?proj=27498], identifier [ChiCTR1800016216].
1 Introduction
Immune checkpoint inhibitors (ICIs) including PD1/PDL1 were the novel immunotherapy drugs for various cancers; however, early detection of subclinical cardiotoxicity ICI therapy has been challenging (1). With few recent exceptions, the vast majority of articles on the toxicities of checkpoint inhibitors have underestimated or even neglected cardiac toxicity (2). According to reports, PD1/PDL1 immunotherapy can lead to myocardial damage, and cardiotoxicity can be often fatal and is associated with a poor prognosis (3). Thus, continuous monitoring during immunotherapy for early detection of left ventricular (LV) dysfunction is necessary.
Echocardiography enables the identification of left ventricular dysfunction, cardiac valves, and pericardial diseases and is one of the most important clinical tools in the diagnosis and management of myocardial damage. However, the traditional ultrasound indices are not sufficiently sensitive for the detection of early changes in cardiac function (4). Two-dimensional strain parameters, especially left ventricular longitudinal strain, have been shown to precede a decrease in left ventricular ejection fraction (LVEF) during PD1/PDL1 immunotherapy (5). Global longitudinal strain (GLS) has been recommended as a sensitive index by ASE/EACI and ESC guidelines during the follow-up of chemotherapy (6, 7). In this study, we evaluated the cardiac dysfunction associated with ICI treatment in Chinese patients by using speckle-tracking imaging and three-dimensional echocardiography.
2 Materials and methods
2.1 Study population
The study protocol was approved by the medical Ethical Review Boards of the Tianjin Medical University Second Hospital (number: KY2018K090). The study was registered with the Chinese Clinical Trial Registry (registration number: ChiCTR18000162216). All participants provided informed consent. A total of 55 consecutive patients hospitalized in the Urology department and Oncology Department of Tianjin Medical University Second Hospital from June 2018 to October 2020 who were naive to ICI treatment were enrolled in the study.
The inclusion criteria included the following: (1) 18–90 years old, (2) cancer confirmed by histopathological results, and (3) normal liver and renal function.
The exclusion criteria were patients with existing (1) heart failure, (2) myocardial infarction, (3) myocarditis, (4) cardiomyopathy, (5) severe valvular diseases, (6) patients who were pregnant or were lactating, (7) patients with poor echocardiography image qualities, and (8) those with less than 6 months survival.
2.2 Laboratory characteristics and clinical history
Laboratory findings included cardiac enzymes [troponin, creatine kinase (CK), and creatine kinase MB (CKMB)], blood routine test (hemoglobin and platelet levels), liver function [albumin, alanine transaminase (ALT), aspartate aminotransferase (AST), and total bilirubin], renal function (urea, creatinine, and uric acid), glucose and thyroid function [triiodothyronine (T3), tetraiodothyronine (T4), and thyroid-stimulating hormone (TSH)], and clinical history including gender, age, height, weight, blood pressure, heart rate, hypertension, diabetes mellitus, heart diseases, smoking, and alcohol intake history, which were recorded at baseline. Transthoracic echocardiograms were performed before immunotherapy, and pre-ICI and after ICI administration.
2.3 Transthoracic echocardiography
The echocardiographic images were performed in the left recumbent position of the patients. Echocardiographic measurements were based on current guidelines for assessing cardiovascular structure and function. All patients underwent transthoracic echocardiography using a Philips IE33 ultrasound system equipped with an X5-1 (2.5–3.5 MHz) probe. Images of at least five cycles of sinus rhythm were digitally stored in the original Digital Imaging and Communications in Medicine format for offline analysis. The images were recorded in the following standard views: parasternal long-axis view, parasternal short-axis view, apical four-chamber view, apical three-chamber view, and apical two-chamber view.
2.4 General two-dimensional echocardiography parameters
Left atrial diameter (LAD), left ventricular end-diastolic diameter (LVEDD), left ventricular end-systolic diameter (LVESD), left ventricle posterior wall thickness (LVPWT), and interventricular septum thickness (IVST) were measured in the parasternal long-axis view. The right ventricular area change rate (RVFAC) was calculated via the maximum and minimum areas of the right ventricle [(RVAmax–RVAmin)/RVAmax]. The tricuspid annular plane systolic excursion (TAPSE) was measured in the M mode of the four-chamber view. Mitral inflow velocities and mitral annulus velocities were obtained by pulsed and tissue Doppler echocardiography, respectively. Left atrial volume (LAV) was measured in three-dimensional echocardiography.
2.5 Two-dimensional strain parameters and three-dimensional echocardiography
The QLAB 10.8 software was used to analyze the images offline. The left ventricle was divided into 17 myocardial segments, and the GLS was calculated as the average longitudinal strain at the end of the systole. The left ventricular global circumference strain (GCS) was measured in the parasternal short-axis views (SAX B, SAX M, and SAX A) according to the software instructions. The right ventricular longitudinal strain and free-wall longitudinal strain were measured accordingly at AP4 view. Left ventricular end-systolic volume (LVESV), left ventricular end-diastolic volume (LVEDV), and 3D LVEF were calculated in three-dimensional echocardiography imaging of a four-chamber view.
2.6 Definition of cardiac toxicity and subclinical cardiac toxicity
Cardiac toxicity was defined as LVEF less than 53% or decreasing more than 10% in LVEF or 15% in GLS when compared with the baseline value (6, 8). In addition, cardiac toxicity was also defined as a >5% decrease in LVEF along with the presence of heart failure symptoms, troponin increased extending normal range combined with the patient having the sign of myocarditis, cardiovascular death, cardiogenic shock, and cardiac arrest. TAPSE less than 16 mm represented right ventricular systolic dysfunction (9). Subclinical cardiac toxicity was defined as early changes in left ventricular and right ventricular mechanics after ICI treatment, but clinical symptoms of ICI-associated were absent or happened later. The cutoff follow-up time was 220 days. According to reports, the cutoff of LVGLS, RVGLS, and RVFWLS was –18, –17.3, and –19.5%, respectively (10–12).
2.7 Statistical analysis
The IBM SPSS statistics 20.0 software (IBM Corporation, Armonk, NY, USA) was used for statistical analysis. All continuous variables were expressed as means ± standard deviation and compared by paired t-test. Categorical data were presented as numbers (%) and compared through the chi-square test. The data were analyzed using two-tailed tests, and P < 0.05 indicated that the difference was statistically significant. Subgroup analysis was performed. Cox regression was employed for survival analysis. ICI-related cardiotoxicity occurred time and survival cumulative percentage was analyzed through the Kaplan–Meier curve, and the P-value was calculated by log-rank test. The receiver operator characteristic (ROC) curve was done for analyzing LVGLS.
3 Results
3.1 Patient clinical characteristics
In total, 55 patients met the criteria of this study. Among them, 40 (72.3%) were male patients with a mean age of 62, 17 patients had hypertension, nine patients had diabetes mellitus, five patients had a history of cardiovascular diseases, and 23 patients had a smoking history. Their cardiac enzymes (troponin, CK, and CKMB), routine blood results (hemoglobin and platelet), and liver and renal function (albumin, ALT, AST, total bilirubin, urea, creatinine, and uric acid) were within normal ranges. The baseline clinical data of the patients are shown in Table 1. All patients had a normal left ventricular ejection fraction of more than 53% at the baseline. The most common indications for ICIs were urinary system malignancies and lung cancer (87.3%) (Table 1). Patients were more likely to have received single ICI therapy and later combination ICI therapy with chemotherapy, and clinical laboratory results are shown in Table 2. Compared with the pre-ICI data, all the aforementioned clinical laboratory results were similar to the post-ICI data, except for the AST parameter, though AST was in the normal range either before or after ICI treatment.
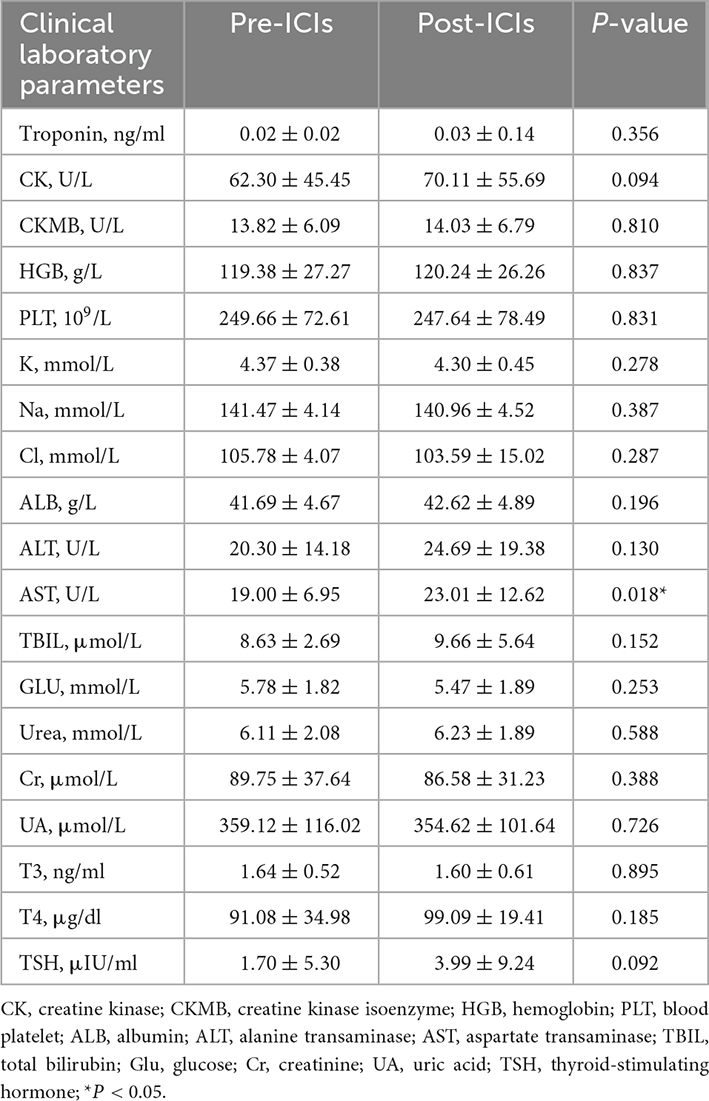
Table 2. Patients’ clinical laboratory data before and after immune checkpoint inhibitors (ICIs) treatment.
3.2 Echocardiography results
Compared with the pre-ICI data, RVFAC [(52.16 ± 12.18)% vs. (48.62 ± 9.84)%, P = 0.064] had no significant change after ICI treatment. mPAP (16.77 ± 8.71 vs. 22.03 ± 9.08, P = 0.0001), IVST (9.15 ± 1.86 vs. 9.74 ± 1.95, P = 0.004), LVEDD (46.03 ± 4.06 vs. 47.27 ± 3.59, P = 0.01), LVEDS (25.47 ± 3.86 vs. 27.39 ± 4.34, P = 0.002), LVPWT (8.75 ± 1.41 vs. 9.39 ± 1.41, P = 0.0001), E/e’ ratio (10.09 ± 2.51 vs. 11.04 ± 2.45, P = 0.011), LAD (35.09 ± 4.93 vs. 35.87 ± 4.63, P = 0.038), and LAV (30.40 ± 10.17 vs. 34.79 ± 12.32, P = 0.005) were significantly increased and LV3DEF [(60.89 ± 11.77)% vs. (57.00 ± 8.18)%, P = 0.022] was significantly decreased, while they were in normal range either before or after ICI treatment. Compared with the baseline data, the LVEF, LVGLS, LVGCS, TAPSE, RVGLS, and RVFWLS were significantly worsened after ICI treatment [(64.49 ± 4.36)% vs. (60.75 ± 4.43)%, P = 0.0001; (–18.63 ± 2.53)% vs. (–17.35 ± 2.58)%, P = 0.000; (–28.83 ± 5.09)% vs. (–24.98 ± 4.51)%, P = 0.0001; 18.29 ± 6.23 vs. 14.57 ± 3.81, P = 0.0001; (–18.45 ± 4.65)% vs. (–14.98 ± 3.85)%, P = 0.0001; and (–25.49 ± 6.42)% vs. (–21.33 ± 6.00)%, P = 0.001, respectively] (Figure 1). LVEF, LVGCS, and RVFWLS were in the normal range either before or after ICI treatment. LVGLS (–17.57 ± 2.65, P = 0.001), TAPSE (14.57 ± 3.81, P = 0.0001), and RVGLS (–14.98 ± 3.85%, P = 0.0001) were below their normal range individually after ICI immunotherapy. Echocardiography results are shown in Table 3. Subgroup analysis of LVGLS showed similar results in age, gender, and drug subgroups (Table 4).
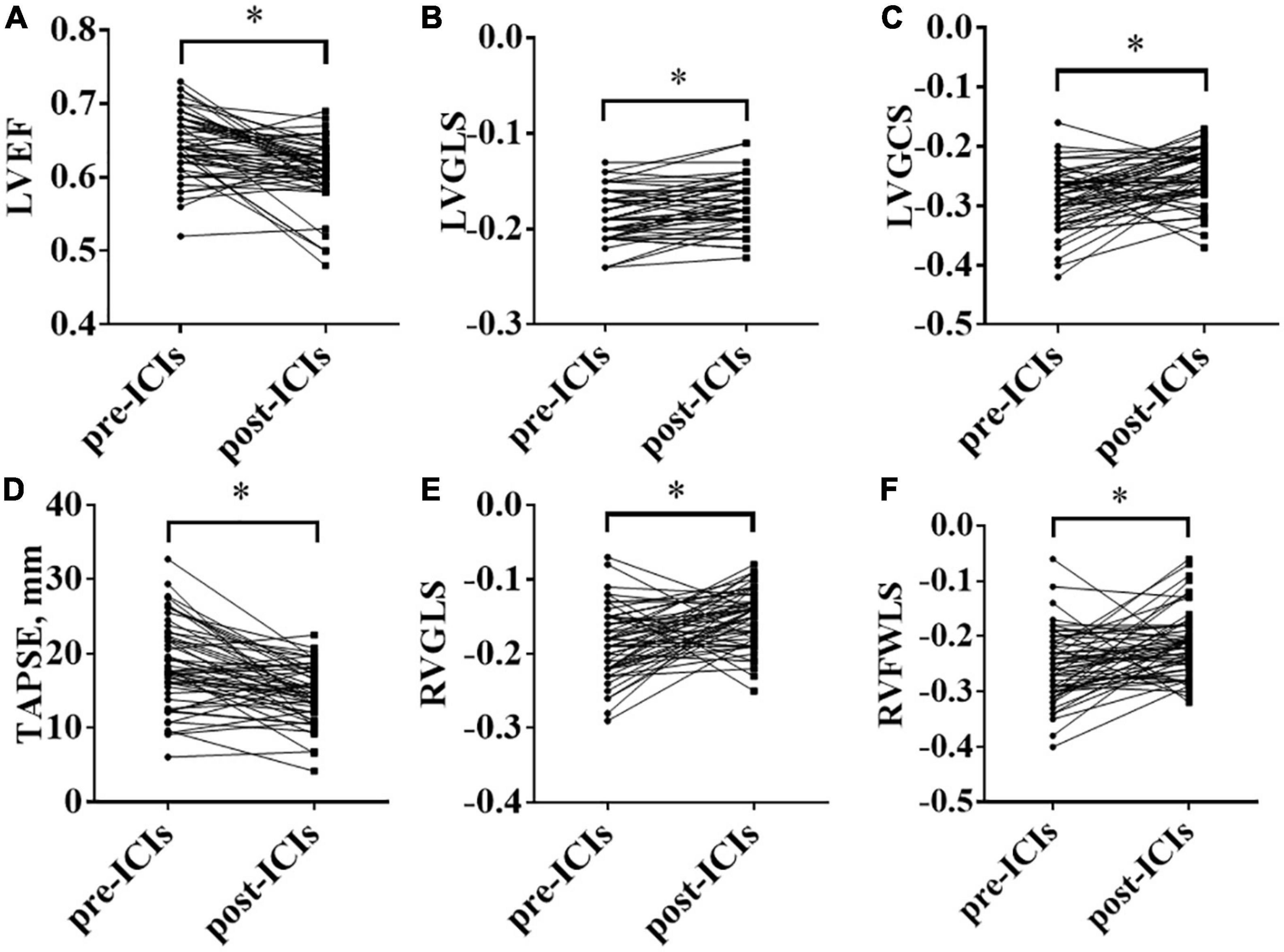
Figure 1. Changes in left ventricular ejection fraction (LVEF), left ventricular global longitudinal peak systolic strain (LVGLS), left ventricular global circumferential peak systolic strain (LVGCS), tricuspid annular plane systolic excursion (TAPSE), right ventricular global longitudinal systolic strain (RVGLS), and right ventricular free-wall longitudinal peak systolic strain (RVFWLS) before and after immune checkpoint inhibitors (ICIs) treatment; (A): LVEF; (B): LVGLS; (C): LVGCS; (D): TAPSE; (E): RVGLS; (F): RVFWLS; *P < 0.05.
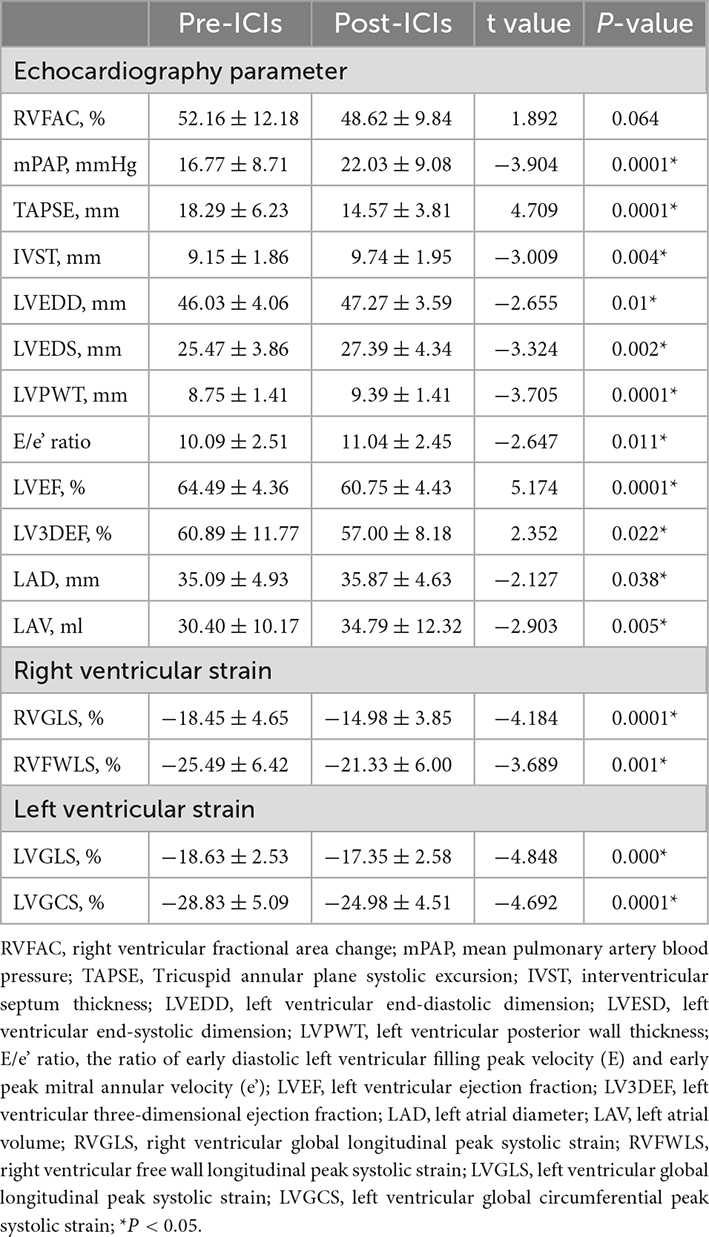
Table 3. Patients’ echocardiography data before and after immune checkpoint inhibitors (ICIs) treatment.
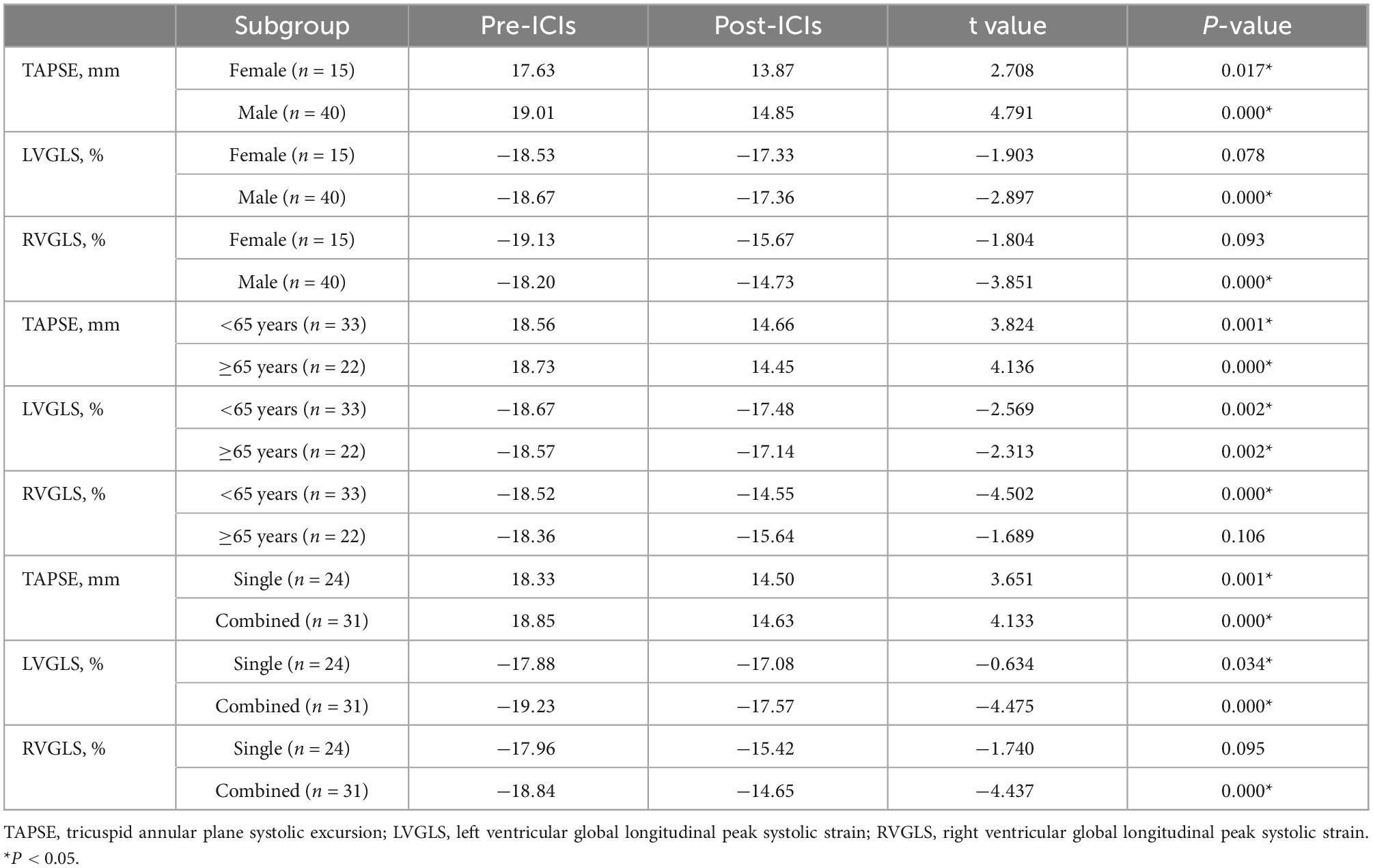
Table 4. Subgroup analysis of echocardiography data before and after immune checkpoint inhibitors (ICIs) treatment.
3.3 Prognosis analysis by Kaplan–Meier curve
Fifty-five patients enrolled in the present study were followed up for 220 days. Six (10.9%) patients died, 15 (27.3%) patients had more than 15% LVGLS decrease, 10 (18.2%) patients had cardiac toxicity (LVEF reduced by 10% and less than 53%), and four (7.3%) patients developed interstitial pneumonia. The KM curve analysis showed that the standard of LVGLS decreased by more than 15% and was more sensitive than the cardiac toxicity events to assess ICI-related subclinical cardiac toxicity, but there was no statistical significance (log-rank P = 0.205) (Figure 2). Kaplan–Meier curve analysis demonstrated that patients with decreased LVEF, LVGLS, RVGLS, and TAPSE tended to have higher rates of long-term mortality, although statistical significance was not reached (Figure 2). Cox regression analysis clarified two factors significantly related to the survival rates, including the single or combined treatment and LVGLS decreased or preserved (Table 5). The ROC curve for analyzing the LVGLS showed that the area under the ROC curve was 0.693, and the cutoff value of ΔLVGLS was –13% (Figure 3).
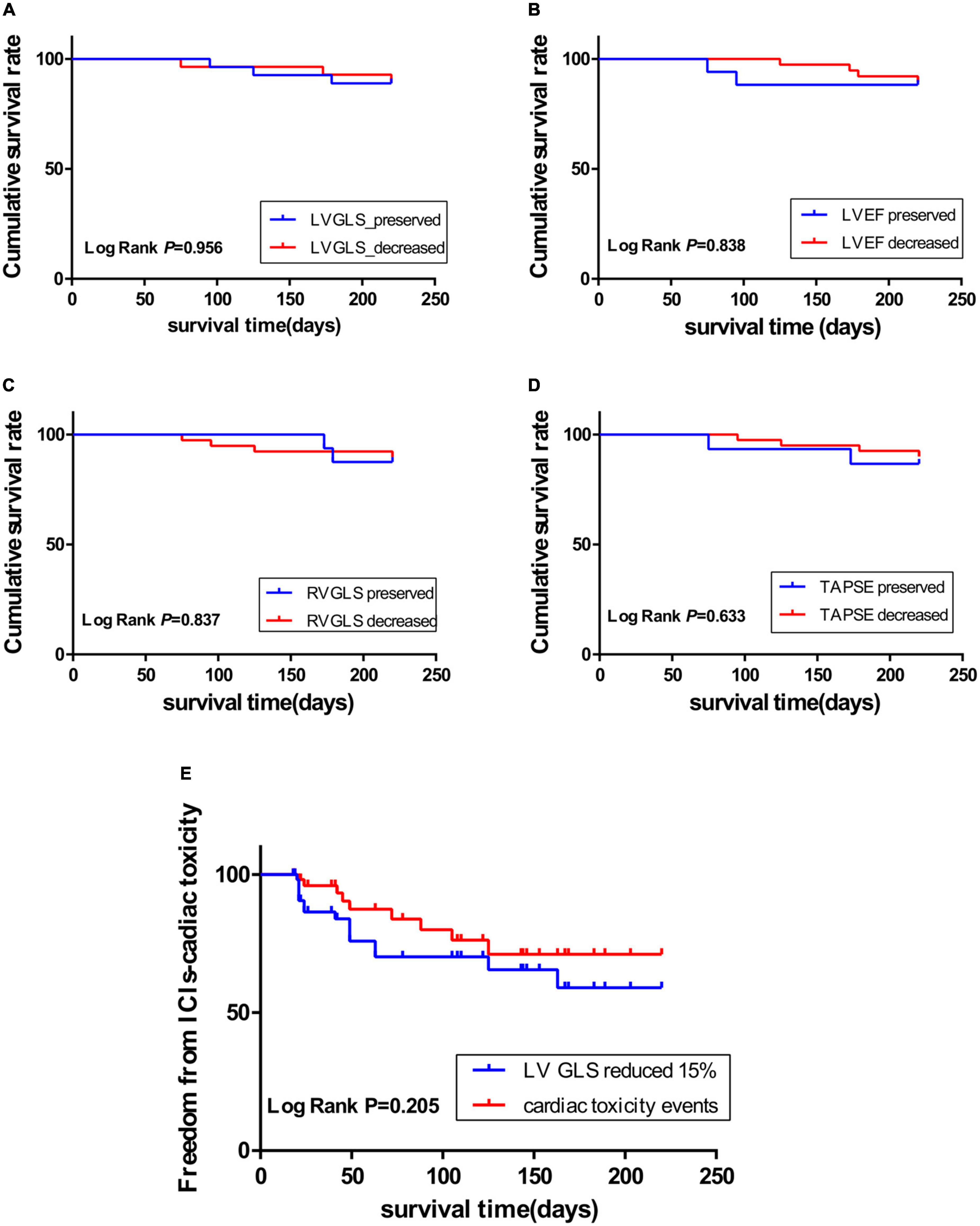
Figure 2. Kaplan–Meier curve showing the cumulative survival rate according to decreased or preserved left ventricular global longitudinal peak systolic strain (LVGLS), left ventricular ejection fraction (LVEF), right ventricular global longitudinal systolic strain (RVGLS), and tricuspid annular plane systolic excursion (TAPSE); (A): LVGLS; (B): LVEF; (C): RVGLS; (D): TAPSE. (E): Kaplan–Meier curve showing the immune checkpoint inhibitor (ICI)-related cardiotoxicity survival time through LVGLS reduced by more than 15% (blue curve), and events (red curve) including heart failure symptoms, troponin increased exceeding the normal range, and LVEF reduced by more than 10% and less than 53%.
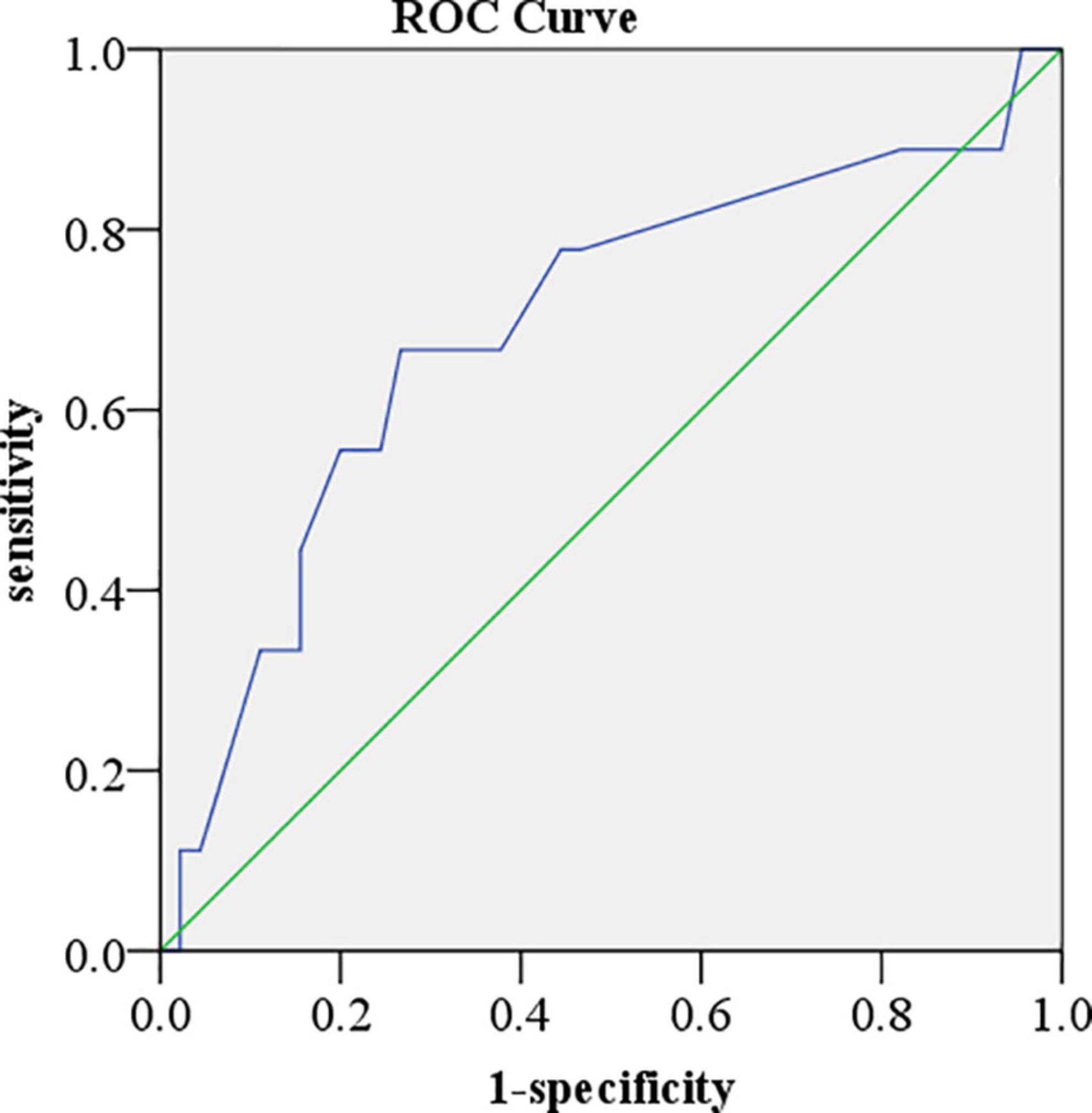
Figure 3. Receiver operator characteristic curve for analyzing left ventricular global longitudinal peak systolic strain (LVGLS). The area under the receiver operator characteristic (ROC) curve was 0.693. The cutoff value of ΔLVGLS was –13%.
4 Discussion
The present study showed that LVGLS, RVGLS, and TAPSE were significantly reduced to less than the normal range after ICI administration. Decreased LVGLS reflected left ventricular dysfunction while RVGLS and TAPSE reflected right ventricular dysfunction. By contrast, other parameters including LVEF, LVEDD, LVEDS, and RVFAC were within normal limits following ICI immunotherapy. Thus, it would appear that LVGLS, RVGLS, and TAPSE are more sensitive indices for the early detection of ICI-related subclinical myocardial toxicity.
4.1 ICI-associated cardiac dysfunction
Recent reports have expanded the effect of ICI therapy on the cardiovascular system to include an increase in cardiac dysfunction without myocarditis, arrhythmias, venous thromboembolic disease, accelerated atherosclerosis, and atherosclerosis-related cardiovascular events (13). The risk factors associated with ICI-related cardiotoxicity include male sex, sleep apnea, higher BMI, history of radiation, and low neutrophil-to-lymphocyte ratios (14, 15). However, single ICI did not increase the risk of cardiotoxicity compared with chemotherapy and combination immunotherapy did not increase the risk of cardiotoxicity compared with single ICI (16). The association between immune checkpoint inhibitor therapy and an increase in cardiovascular events is not only limited to events occurring within the first few weeks after starting therapy but also can include events that occur months to years after therapy (13). The median time from treatment initiation to clinical manifestation of ICI-related events was 30 days for myocarditis, 30 days for pericardial disorders, and 55 days for vasculitis (17). The current gold standard for myocarditis diagnosis is histological findings of endomyocardial lymphocyte infiltration with myocyte necrosis (18). Transthoracic echocardiogram (TTE) and elevated cardiac biomarkers, including serum troponin, CK-MB, total CK, and natriuretic peptide levels are important tests in the evaluation of suspected myocarditis (19). TTE can also detect other cardiac manifestations, including pericardial effusion or pericardial thickening and intra-cardiac thrombi (19). However, there were no standard examinations to detect ICI-associated cardiac dysfunction. Our study found that LVGLS, RVGLS, and TAPSE were more sensitive indices for the early detection of ICI-related subclinical myocardial toxicity. Given the involvement of immune checkpoints in many aspects of cardiovascular homeostasis, the mechanisms are probably multiple and interrelated (20). Therefore, the change in myocardial mechanics during PD1/PDL1 immunotherapy requires continuous monitoring. For the early recognition of ICI-related cardiac events, a basic assessment of cardiac status, including ECG, echocardiography, and troponin, should be considered before starting ICI therapy (21).
4.2 ICIs and right ventricular parameters
Previous studies found that the cutoff value of RVGLS and RVFWLS was –17.3 and –19.5%, respectively (10, 11). In pathological situations, right ventricular remodeling can occur when ventricular dilatation is produced by pressure overload (22). Because the interventricular septum is shared by the left ventricle and right ventricle, the RVGLS is influenced by both left and right ventricular function. It is reported that RVFWLS was the prior method to assess the right ventricle function of patients with pulmonary arterial hypertension (23). A slight change in right systolic function in patients with tumors could be identified through TAPSE (24). TAPSE less than 16 mm represents right ventricular systolic dysfunction (9). The study showed that compared with the baseline, TAPSE was significantly decreased post-ICIs. Kaplan–Meier curve analysis presented patients with reduced TAPSE had a higher incidence of death. The result coincidentally proved the important role of TAPSE to evaluate right ventricular systolic function.
4.3 ICIs and left ventricular parameters
Left and right ventricular toxicity can occur simultaneously (25). LVGLS and RVGLS were important indicators to assess the function of both chambers and can provide an early indicator of prognosis in patients with cancer. Our research demonstrated that LVGLS and RVGLS after ICI immunotherapy were significantly reduced than the baseline and lower than their normal range. Our results also proved that LV and RV functions were injured simultaneously after the administration of ICIs. Among the 55 patients, 15 patients were diagnosed with subclinical cardiac toxicity, according to the definition of LVGLS, reduced by more than 15%. Adverse cardiovascular events occurred in nine patients. The Kaplan–Meier curve analysis demonstrated that the criteria of LVGLS reduced by more than 15% were more sensitive than cardiac toxicity events to predict subclinical cardiac toxicity. LVEF is a load-dependent index, and preload, afterload, and myocardial contractility can all affect the LVEF value. All of these effects may lead to errors that affect the accuracy and sensitivity of LVEF (4, 12, 26).
4.4 ICIs and laboratory findings
Our early findings found no obvious increase in cTnI or NT-proBNP during follow-up, except in two cases. Laboratory parameters, including cTnI or NT-proBNP, were not optimum guidance for cardiotoxicity. Lena et al. (27) reported in EcoR Registry that the number of patients with increased cTnI or NT-proBNP was higher in patients with chemotherapy-induced cardiomyopathy than in patients without cardiotoxicity.
5 Limitations
There were several limitations of our study. First, a small number of patients were enrolled, and a single-center study was included. Further investigations in a multicenter and larger pool pattern will be needed to confirm the potential role of 2D-STI and 3D echocardiography in predicting ICI-related subclinical myocardial toxicity. Second, the heterogeneity of the patients was inevitable not only due to the small group of patients but also the complexity of individuals. Third, the follow-up study time was not sufficiently long, and the prognostic value of the different echocardiography indexes in the PD1/PDL1-related cardiotoxicity has not been thoroughly elucidated to date.
6 Conclusion
The cardiotoxicity of PD1/PDL1 immunotherapy can manifest as impaired left and right ventricular systolic function. LVGLS, RVGLS, and TAPSE are more sensitive indices for the early detection of subclinical cardiotoxicity.
Data availability statement
The raw data supporting the conclusions of this article will be made available by the authors, without undue reservation.
Ethics statement
The studies involving human participants were reviewed and approved by Medical Ethical Review Boards of the Tianjin Medical University Second Hospital. The patients/participants provided their written informed consent to participate in this study.
Author contributions
HF was responsible for the manuscript concept, design, and definition of intellectual content. AX and MY were responsible for data analysis, statistical analysis, and manuscript preparation. XZ, GM, TW, and GZ were responsible for the literature search, clinical studies, and data acquisition. HH was responsible for manuscript editing and review. All authors contributed to the article and approved the submitted version.
Funding
This study was funded by the Tianjin Natural Science Foundation (16JCYBJC25000, 21JCYBJC01740, and 21JCYBJC01460), the Key Laboratory Scientific Research Foundation of Second Hospital of Tianjin Medical University (2018ZDSYS03 and 2019ZDSYS03), the Clinical Study of Second Hospital of Tianjin Medical University (2019LC03), the Tianjin Key Medical Discipline (Specialty) Construction Project, the Tianjin Key Medical Discipline (Specialty) Construction Project (TJYXZDXK-029A), and the National Natural Science Foundation of China (No. 82100342).
Conflict of interest
The authors declare that the research was conducted in the absence of any commercial or financial relationships that could be construed as a potential conflict of interest.
Publisher’s note
All claims expressed in this article are solely those of the authors and do not necessarily represent those of their affiliated organizations, or those of the publisher, the editors and the reviewers. Any product that may be evaluated in this article, or claim that may be made by its manufacturer, is not guaranteed or endorsed by the publisher.
References
1. Zhang N, Tse G, Liu T. Neutrophil-lymphocyte ratio in the immune checkpoint inhibitors-related atherosclerosis. Eur Heart J. (2021) 42:2215. doi: 10.1093/eurheartj/ehab158
2. Ganatra S, Neilan T. Immune checkpoint inhibitor-associated myocarditis. Oncologist. (2018) 23:879–86. doi: 10.1634/theoncologist.2018-0130
3. Behravesh S, Shomali N, Danbaran GR, Aslani S, Hemmatzadeh M, Hosseinzadeh R, et al. Cardiotoxicity of immune checkpoint inhibitors: An updated review. Biotechnol Appl Biochem. (2022) 69:61–69. doi: 10.1002/bab.2081
4. Ewer M, Lenihan D. Left ventricular ejection fraction and cardiotoxicity: is our ear really to the ground? J Clin Oncol. (2008) 26:1201–3. doi: 10.1200/JCO.2007.14.8742
5. Poterucha J, Kutty S, Lindquist R, Li L, Eidem B. Changes in left ventricular longitudinal strain with anthracycline chemotherapy in adolescents precede subsequent decreased left ventricular ejection fraction. J Am Soc Echocardiogr. (2012) 25:733–40. doi: 10.1016/j.echo.2012.04.007
6. Zamorano J, Lancellotti P, Rodriguez Muñoz D, Aboyans V, Asteggiano R, Galderisi M, et al. 2016 ESC position paper on cancer treatments and cardiovascular toxicity developed under the auspices of the ESC committee for practice guidelines: the task force for cancer treatments and cardiovascular toxicity of the European society of cardiology (ESC). Eur Heart J. (2016) 37:2768–801. doi: 10.1093/eurheartj/ehw211
7. Santoro C, Arpino G, Esposito R, Lembo M, Paciolla I, Cardalesi C, et al. 2D and 3D strain for detection of subclinical anthracycline cardiotoxicity in breast cancer patients: a balance with feasibility. Eur Heart J Cardiovasc Imaging. (2017) 18:930–6. doi: 10.1093/ehjci/jex033
8. Lang R, Badano L, Mor-Avi V, Afilalo J, Armstrong A, Ernande L, et al. Recommendations for cardiac chamber quantification by echocardiography in adults: an update from the American society of echocardiography and the European association of cardiovascular imaging. J Am Soc Echocardiogr. (2015) 28:1–39.e14. doi: 10.1016/j.echo.2014.10.003
9. Rudski L, Lai W, Afilalo J, Hua L, Handschumacher M, Chandrasekaran K, et al. Guidelines for the echocardiographic assessment of the right heart in adults: a report from the American society of echocardiography endorsed by the European association of echocardiography, a registered branch of the european society of cardiology, and the Canadian society of echocardiography. J Am Soc Echocardiogr. (2010) 23:685–713. doi: 10.1016/j.echo.2010.05.010
10. Anwar S, Harris M, Whitehead K, Keller M, Goldmuntz E, Fogel M, et al. The impact of the right ventricular outflow tract patch on right ventricular strain in tetralogy of fallot: a comparison with valvar pulmonary stenosis utilizing cardiac magnetic resonance. Pediatr Cardiol. (2017) 38:617–23. doi: 10.1007/s00246-016-1558-5
11. Garcia-Martin A, Moya-Mur J, Carbonell-San Roman S, Garcia-Lledo A, Navas-Tejedor P, Muriel A, et al. Four chamber right ventricular longitudinal strain versus right free wall longitudinal strain. Prognostic value in patients with left heart disease. Cardiol J. (2016) 23:189–94. doi: 10.5603/CJ.a2015.0079
12. Negishi T, Negishi K. Echocardiographic evaluation of cardiac function after cancer chemotherapy. J Echocardiogr. (2018) 16:20–7. doi: 10.1007/s12574-017-0344-6
13. Thuny F, Naidoo J, Neilan T. Cardiovascular complications of immune checkpoint inhibitors for cancer. Eur Heart J. (2022) 43:4458–68. doi: 10.1093/eurheartj/ehac456
14. Mahmood S, Fradley M, Cohen J, Nohria A, Reynolds K, Heinzerling L, et al. Myocarditis in patients treated with immune checkpoint inhibitors. J Am College Cardiol. (2018) 71:1755–64. doi: 10.1016/j.jacc.2018.02.037
15. Wu S, Bai H, Zhang L, He J, Luo X, Wang S, et al. Cardiovascular adverse events induced by immune checkpoint inhibitors: a real world study from 2018 to 2022. Front Cardiovasc Med. (2022) 9:969942. doi: 10.3389/fcvm.2022.969942
16. Zhang X, Ge N, Xiang Z, Liu T. Immune checkpoint inhibitor-related adverse cardiac events in patients with lung cancer: a systematic review and meta-analysis. Cancer Cell Int. (2022) 22:363. doi: 10.1186/s12935-022-02760-2
17. Salem J, Manouchehri A, Moey M, Lebrun-Vignes B, Bastarache L, Pariente A, et al. Cardiovascular toxicities associated with immune checkpoint inhibitors: an observational, retrospective, pharmacovigilance study. Lancet Oncol. (2018) 19:1579–89. doi: 10.1016/s1470-2045(18)30608-9
18. Guo C, Alexander M, Dib Y, Lau P, Weppler A, Au-Yeung G, et al. A closer look at immune-mediated myocarditis in the era of combined checkpoint blockade and targeted therapies. Eur J Cancer. (2020) 124:15–24. doi: 10.1016/j.ejca.2019.09.009
19. Baik A, Tsai K, Oh D, Aras M. Mechanisms and clinical manifestations of cardiovascular toxicities associated with immune checkpoint inhibitors. Clin Sci (Lond). (2021) 135:703–24. doi: 10.1042/cs20200331
20. Baik A, Oluwole O, Johnson D, Shah N, Salem J, Tsai K, et al. Mechanisms of cardiovascular toxicities associated with immunotherapies. Circ Res. (2021) 128:1780–801. doi: 10.1161/CIRCRESAHA.120.315894
21. Liang S, Yang J, Lin Y, Li T, Zhao W, Zhao J, et al. Immune myocarditis overlapping with myasthenia gravis due to Anti-PD-1 treatment for a chordoma patient: a case report and literature review. Front Immunol. (2021) 12:682262. doi: 10.3389/fimmu.2021.682262
22. Mercurio V, Palazzuoli A, Correale M, Lombardi C, Passantino A, Ravera A, et al. Right heart dysfunction: from pathophysiologic insights to therapeutic options: a translational overview. J Cardiovasc Med (Hagerstown). (2018) 19:613–23. doi: 10.2459/JCM.0000000000000700
23. Werther Evaldsson A, Ingvarsson A, Smith J, Radegran G, Roijer A, Waktare J, et al. Echocardiographic right ventricular strain from multiple apical views is superior for assessment of right ventricular systolic function. Clin Physiol Funct Imaging. (2019) 39:168–76. doi: 10.1111/cpf.12552
24. Keramida K, Farmakis D. Right ventricular involvement in cancer therapy-related cardiotoxicity: the emerging role of strain echocardiography. Heart Fail Rev. (2021) 26:1189–93. doi: 10.1007/s10741-020-09938-8
25. Frey M, Bergler-Klein J. Echocardiographic evaluation of patients undergoing cancer therapy. Eur Heart J Cardiovasc Imaging. (2021) 22:375–82. doi: 10.1093/ehjci/jeaa341
26. Shuai W, Kong B, Fu H, Shen C, Huang H. Loss of MD1 increases vulnerability to ventricular arrhythmia in diet-induced obesity mice via enhanced activation of the TLR4/MyD88/CaMKII signaling pathway. Nutr Metab Cardiovasc Dis. (2019) 29:991–8. doi: 10.1016/j.numecd.2019.06.004
Keywords: subclinical cardiotoxicity, echocardiography, strain, TAPSE, ICIs
Citation: Xu A, Yuan M, Zhan X, Zhao G, Mu G, Wang T, Hu H and Fu H (2022) Early detection of immune checkpoint inhibitor-related subclinical cardiotoxicity: A pilot study by using speckle tracking imaging and three-dimensional echocardiography. Front. Cardiovasc. Med. 9:1087287. doi: 10.3389/fcvm.2022.1087287
Received: 02 November 2022; Accepted: 01 December 2022;
Published: 21 December 2022.
Edited by:
Qingpeng Zhang, City University of Hong Kong, Hong Kong SAR, ChinaReviewed by:
Leilei Cheng, Fudan University, ChinaTiangang Zhu, Peking University People’s Hospital, China
Ying Liu, Dalian Medical University, China
Copyright © 2022 Xu, Yuan, Zhan, Zhao, Mu, Wang, Hu and Fu. This is an open-access article distributed under the terms of the Creative Commons Attribution License (CC BY). The use, distribution or reproduction in other forums is permitted, provided the original author(s) and the copyright owner(s) are credited and that the original publication in this journal is cited, in accordance with accepted academic practice. No use, distribution or reproduction is permitted which does not comply with these terms.
*Correspondence: Hailong Hu, ✉ aGhsbG92ZTIwMDRAMTYzLmNvbQ==; Huaying Fu, ✉ ZnVodWF5aW5nQHRtdS5lZHUuY24=
†These authors have contributed equally to this work and share first authorship