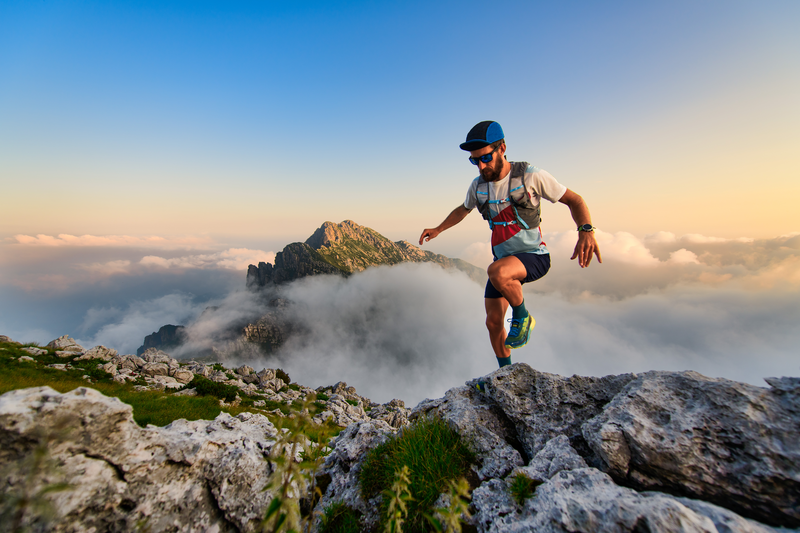
94% of researchers rate our articles as excellent or good
Learn more about the work of our research integrity team to safeguard the quality of each article we publish.
Find out more
REVIEW article
Front. Cardiovasc. Med. , 25 November 2022
Sec. General Cardiovascular Medicine
Volume 9 - 2022 | https://doi.org/10.3389/fcvm.2022.1063683
This article is part of the Research Topic Year in Review: Discussions in general cardiovascular medicine View all 5 articles
Abdominal aortic aneurysm (AAA) is an inflammatory vascular disease with high disability and mortality. Its susceptible risk factors include old age, being male, smoking, hypertension, and aortic atherosclerosis. With the improvement of screening techniques, AAA incidence and number of deaths caused by aneurysm rupture increase annually, attracting much clinical attention. Due to the lack of non-invasive treatment, early detection and development of novel treatment of AAA is an urgent clinical concern. The pathophysiology and progression of AAA are characterized by inflammatory destruction. The gut microbiota is an “invisible organ” that directly or indirectly affects the vascular wall inflammatory cell infiltration manifested with enhanced arterial wall gut microbiota and metabolites, which plays an important role in the formation and progression of AAA. As such, the gut microbiome may become an important risk factor for AAA. This review summarizes the direct and indirect effects of the gut microbiome on the pathogenesis of AAA and highlights the gut microbiome-mediated inflammatory responses and discoveries of relevant therapeutic targets that may help manage the development and rupture of AAA.
Abdominal aortic aneurysm (AAA) refers to the local full-thickness expansion of the subrenal aorta, in which the expansion diameter is >3 cm or >50% of the normal diameter. AAA is generally asymptomatic in the early stage, but the mortality rate can reach 80% following a rupture in the later stage (1). The main AAA risk factors are the age of >65 years, being male, and smoking history, while other risk factors include a family history of AAA, coronary heart disease(CHD), hypertension (HTN), peripheral artery disease, and previous myocardial infarction (2). The pathological characteristics of AAA mainly include an inflammatory response, vascular endothelial cells (VECs) damage, vascular smooth muscle cell (VSMC) apoptosis, extracellular matrix (ECM) degradation, and oxidative stress (3). Many leukocytes and inflammatory mediators are associated with the pathogenesis of AAA, including interleukin (IL)-1,−17, transforming growth factor (TGF)-β, and angiotensin II (AngII), which can infiltrate into aortic media and lead to smooth muscle cell depletion, generation of reactive oxygen species (ROS), and activation of matrix metalloproteinases (MMPs) causing ECM fragmentation (4).
The human gut microbiota is usually composed of four phyla: Bacteroidetes (9–42%), Firmicutes (30–52%), Proteobacteria (5–10%), and Actinobacteria (1–3%) (5), among which, Bacteroides, Faecalis, and Bifidobacterium are the most common genera in healthy adults. The pathogenic bacteria causing AAA originate from the skin, oral cavity, gastrointestinal tract, and respiratory tract and can infect the abdominal aorta through gut bacterial translocation, blood-borne transmission, and aortic aneurysm surgery. For example, Streptococcus colonizing the rectum can infect the abdominal aortic wall and induce AAA (6). Propionibacterium acnes, Propionibacterium granulosum, Actinomyces viscosus, Actinomyces naeslundii, and Eggerthella lenta are found in the aneurysm wall and intravascular plaque (7). Furthermore, the presence of Campylobacter and Campylobacter urealyticus may be related to the rupture of cerebral aneurysms (8). Additionally, compared to the non-AAA group, differences in gut microbiota were confirmed in both AAA mouse models (9) and patients with AAA (10). Gut microbiome dysbiosis can also cause oxidative stress injuries, as well as activate inflammatory cells and toll-like receptors (TLRs) through small molecules produced by microbial metabolites, which serve to intensify the remodeling of the arterial wall (11). Pro-inflammatory factors such as IL1-β, tumor necrosis factor (TNF)-α, interferon (IFN)-γ, and C-C motif chemokine ligand (CCL)-2 can accelerate the progression of AAA, while anti-inflammatory factors such as Arg1, IL10, and TGF-β can promote repair and prevent AAA rupture to a certain extent; therefore, the balance of these inflammatory factors regulated by the gut microbiome affects AAA pathological progression (12). These findings suggest that the gut microbiota contributes to the pathophysiology of AAA by modulating inflammation.
Nevertheless, the mechanism of the direct effect of gut microbiome species and metabolites on the pathogenesis of AAA is not so profound. The imbalance between symbiotic and pathological bacteria in the intestine may lead to changes in immune development and inappropriate inflammatory reactions, but it is unclear whether this imbalance is the cause or result of AAA. In this review, we focus on promoting the understanding of the pathogenesis of gut microbial-mediated AAA by summarizing the relevant findings of animal and human studies and seeking strategies to identify new therapeutic targets for AAA, with the aim to provide novel ideas for gut microbiome intervention in AAA.
Nearly 95% of patients with AAA have AS pathological changes, thus AS represents a vital independent risk factor for AAA (13). However, clinical anti-atherosclerotic drugs are incompletely effective for treating AAA, and AS plays a minor role in patients with AAA that are ≤ 45 mm (14). Recently, some investigations proposed that gut microbiome tightly linked AS with AAA. The changes at different stages of CHD were represented by Roseburia, Klebsiella, Clostridium IV, and Ruminococcaceae, which might affect AS by modulating the metabolic pathways of the host (15). A correlation between the diameter of AAA and gut microbiota of C57BL ApoE(−/−) mice suggested that Akkermansia, Odoribacter, Helicobacter, and Ruminococcus play important roles in the progression of AAA (9). Furthermore, the AAA group had a higher relative abundance of Leuconostocaceae, Ruminococcaceae, Weissella, and Faecalibacterium and a lower relative abundance of Firmicuteria, Selenomonadales, and Veillonellaceae (10). Indeed, Leuconostocaceae is a risk factor for AAA and CHD, whereas an inverse association has been found between Lachnospiraceae and cardiovascular risk factors (16). Thus, the diversity of gut microbiome may be involved in the onset of AS and AAA.
Although AAA is more common in males than in females, the prevalence increases with aging in both sexes (17). Increasing evidences show that the existence of aging cells greatly promotes the inflammatory state of aging blood vessels and can activate nuclear factor kappa-B(NF-κB), TLR, and MMPs in aging VECs, VSMCs, and ECM, resulting in an increased risk of AAA development (18, 19). The main characteristics of the gut microbiome composition of the elderly include reduced diversity, with Bacteroidetes as the dominant microbiota, and a lack of butyrate (20). Furthermore, a decrease in Bifidobacteria and increased levels of the mucin-degrading Akkermansia muciniphila have also been detected to a greater extent in elderly people compared to young adults (21). The decrease in probiotic-producing short-chain fatty acids (SCFAs) in the gut microbiome of the elderly plays a key role in the occurrence and progression of AAA. Aging can cause gut microbiome dysbiosis, leading to increased CD4+ T cell differentiation, and then trigger asystemic inflammatory response by bacterial-derived circulatory inflammatory factors. Aging also leads to oxidative stress by increasing TNF-α expression, which aggravates abnormal changes in the gut microbiota population of Bifidobacterium and the ratio of Firmicutes/Bacteroidetes, which leads to an increase in flavin-containing monooxygenase-3 and trimethylamine N-oxide (TMAO) (22).
Smoking is one of the most important modifiable risk factors of AAAs. Quitting smoking not only reduces the risk of developing an AAA but also limits the growth of AAA (23). It was demonstrated that smoking, age, and other factors can affect 8.87% of gut microbiome changes (24). Moreover, the relative abundance of Prevotella and Neisseria spp. is reduced for current smokers, and the relative abundance of Firmicumis increased, mainly Streptococcus spp., Veillonella spp., and Rothia (Actinobacteria) (25). Additionally, the differences in bacterial communities in current smokers may be related to the impairment of antibacterial defense caused by the immunosuppressive nature of tobacco (26). Indeed, it has been reported that nicotine from tobacco can stimulate the expression of MMPs in VSMCs, VECs, and inflammatory cells of the vascular wall and induce angiogenesis in aneurysm tissues (27).
Obesity is closely associated with the occurrence and progression of AAA (28). In human and mouse AAA lesions, IL18 co-localizes to its receptors at regions rich in adipocytes, which leads to AAA development through IL18 activation (29). Obesity can change the richness of microbes or their genes, and there is a stronger relationship between higher Prevotella relative abundance and body mass index in populations of different races (30). Obesity is also related to changes in the relative abundance of the two dominant bacterial divisions, the Bacteroidetes and the Firmicutes (31).
AngII-induced HTN can increase the pressure of AAA, promote cardiac hypertrophy, damage VECs, and activate the inflammatory response of VSMCs, which are potential causes of the pathogenesis and progression of AAA (32). Firmicutes/Bacteroidetes ratio was increased and the acetate-, butyrate-, and lactic acid-producing bacterial populations were decreased in HTN rats and a patient model (33). Lactococcus, Alistipes, and Subdoligranulum abundances were positively correlated with systolic blood pressure or diastolic blood pressure in hypertensive patients (34). Furthermore, microbial richness and diversity were significantly reduced, gut probiotics were reduced, and other gut microbiome components such as Prevotella and Klebsiella were excessively increased in people with pre-HTN and HTN (35). Among them, Klebsiella pneumoniae was first reported to cause AAA (36). Evidence has also shown that the genus Alistipes, harbored in patients with HTN, is positively correlated with AAA diameter (37).
Low-density lipoprotein (LDL) cholesterol cause VECs damage, abnormal proliferation of VSMCs, and finally, AAA progression. The abundances of Turicibacter, Lachnospira, Ruminococcus_gauvreauii_group, and Acetivibrio_ethanolgignens _group increased in hyperlipidemic rats, while those of Alistipes, Bacteroides, Ruminococcu, and Butyrivibrio were decreased (38). A high-fat and high-fructose diet can alter the gut microbiota composition of Syrian hamsters, leading to dyslipidemia. Among these, Ruminiclostridium 9 and Tyzzerella were positively correlated with fasting cholesterol levels, while the Tyzzerella and Ruminococceace NK4A214 groups were positively correlated with fasting triglyceride levels (39). Moreover, the abundances of Lachnospiraceae and Sutterellaceaecan were significantly decreased, while that of Prevotellaceae was significantly increased in high-fat high-sugar-fed mice, and such a diet led to changes in metabolites and microbiota (40). An HFD not only leads to a reduction in antimicrobial peptides, but also increases inflammation and upregulation of CCL2, IL-β1, and MMPs (41). Besides, “Mediterranean” and vegetarian diets have anti-inflammatory effects and can increase gut probiotics except for improving dyslipidemia (42).
Asthma is an independent risk factor for AAA rupture (43). Low relative abundances of Bifidobacterium, Ackermann, Lachnospira, Veillonella, Faecalibacterium, Rothia genera Ruminococcus gnavus, and Faecalis and high abundances of Candida, Rhodotorula fungi, Streptococcus and Bacteroides were associated with the highest risk of developing childhood atopy and asthma (44–46). Our laboratory also reported that asthma-induced AAA development involves an inflammatory reaction through the activation of eosinophil-derived IL4, eosinophil cationic protein (ECP; cationic proteins of EOS), and IgE and mast cell activation (47).
Chronic obstructive pulmonary disease (COPD) is independently and positively associated with AAA occurrence and rupture (48) but has no association with AAA growth (49). Bifidobacteriaceae, Eubacteriaceae, Lactobacillaceae, Micrococcaceae, Streptococcaceae, and Veillonellaceae were increased, whereas Desulfovibrionaceae, Gastranaerophilaceae, and Selenomonadaceae were decreased in patients with COPD (50). It was also found that the decrease in probiotics and the increase in Enterobacteriaceae and anaerobic bacteria can lead to an increased systemic inflammatory response, causing the occurrence and rupture of AAA (50).
Chronic kidney disease (CKD) induces systemic inflammation, a condition considered to increase the risk of AAA incidence rate and abdominal aortic diameter enlargement (51). CKD severity is an important predictor of perioperative mortality and long-term survival after AAA repair (52). The bacterial families of Actinobacteria, Firmicutes, and Proteobacteria have been found to show the greatest increase in patients with CKD compared to healthy controls (53). Furthermore, Bifidobacterium Catenulatum, Bifidobacterium longum, Bifidobacterium bifidum, Lactobacillus plantarum, Lactobacillus paracasei, and Klebsiella pneumonia are known to be decreased in patients with peritoneal dialysis (54). The sharp reduction in gut probiotics reduces the ability of the gut microbiome to remove toxins, which can aggravate the systemic inflammatory response. Simultaneously, colon-derived uremic toxins can lead to gut microbiota imbalance and aortic wall damage, which can cause the onset and progression of AAA.
Periodontitis is highly prevalent in patients with both stable and unstable AAA, and Porphyromonas gingivalis (Pg) has been shown to be closely correlated with AAA diameters and volumes (55). In addition to Pg, Aggregatibacter actinomycetemcomitans, Tannerellaforsythia, and Campylobacter rectus are also involved periodontal pathogens in patients with AAA (56). Pg promotes AAA progression through systemic inflammation using the following mechanism: after entering the blood, Pg binds to the TLR-2 receptor of the abdominal aorta, induces overexpression of MMPs inside the AAA wall or thrombus, and enhances the intraluminal thrombus (ILT) enrichment (55, 57).
A previous study demonstrated that the incidence of peptic ulcer disease is 22.6% in patients with AAA compared to 7.2% in the general necropsy population (58). Helicobacter pylori infection can cause peptic ulcers, and one of the pathogenicity factors of H. pylori is the cytokine-associated gene A (CagA). Indeed, CagA+ H. pylorico-culture with Lactobacillus acidophilus has been shown to induce cytokine patterns (e.g., IL-2, IL-4, IL-6, IL-10, and IFN-γ), contributing to the pathogenesis of AAA (59).
Interestingly, DM can cause arteriosclerosis, but the incidence or growth rate of AAA is lower in patients with diabetes (60). The gut microbiota is involved in insulin resistance, which is related to promote the progression of DM and AAA diameter (61–63). Importantly, in patients with newly diagnosed DM, the level of Lactobacillus is significantly increased, whereas the levels of Clostridium coccoides and Clostridium leptum are significantly decreased (64). Patients with DM also decreased the abundance of some universal butyric acid-producing bacteria, increased opportunistic pathogens, and rich functions of other microorganisms, all of which can reduce sulfate and stimulate bacterial defense mechanisms against oxidative stress injury (61, 65). Additionally, some hypoglycemic drugs, such as metformin, have the positive effect of increasing the life span of the gut probiotics (66), thereby making AAA less likely to occur or develop.
The existence of bidirectional interactions between microorganisms and drugs can be established through pharmacomicrobiomics (67), thus affecting the pathogenesis and progress of AAA. Doxycycline exposure results in Bacteroides expansion and Bifidobacterium and Lactobacillus reduction, which has an adverse impact on promoting AAA growth (68). Fluoroquinolones may also promote the occurrence or rupture of AAA (69). Moxifloxacin can reduce the abundance of alistipes, bilophila, butyciromonas, coprobacillus, fecalibacter, odoribacter, oscillibacter, parasutterella, Roseburia, and sutterella (70). Asthma medication can accelerate the growth or rupture of AAA. For instance, a combination of inhaled corticosteroids (ICSs) and oral glucocorticoids correlates positively with increased abundance of Proteobacteria and Pseudomonas and with decreased abundance of Bacteroidetes, Fusobacteria, and Prevotella (71). Patients with neutrophilic asthma using high doses of ICSs have been shown to have relative enrichment in Haemophilus and Moraxella species, members of the Proteobacteria phylum, and a reduced relative abundance of Streptococcus, Gemella, and Porphyromonas taxa compared to patients with eosinophilic asthma (72, 73). Additionally, other drugs (e.g., proton pump inhibitors, statins, metformin, β receptor blockers, ACE inhibitors, selective serotonin reuptake inhibitors, and antidepressants) are known to dramatically shift the microbiota profile and lead to less diverse changes in microbial composition (67).
Taken together, a total of five categories including 150 species of gut bacteria were related to AAA. The composition ratio of gut microbiome related to AAA risk factors is shown in Figure 1. Furthermore, the correlation between the gut microbiome and risk factors, and the pathological status of AAA are summarized in Supplementary Table 1.
Figure 1. Composition ratio of gut microbiome related to AAA risk factors. This review lists 150 gut bacteria related to AAA, which are divided into five categories according to bacterial phyla.
In the heart, aorta, and peripheral arteries, there is an increased accumulation of immune cells, including lymphocytes helper T cells (Th)1, Th2, Th17, and Tregs (74). IL-1, IL-6, IL-12, and TNF-α can lead to the accumulation of CD4+ T cells, whose effector cells can produce MMPs and cathepsins, causing aneurysm formation and rupture through damage to the aortic wall, inflammation, and loss of VSMCs (4). The systemic spread of inflammation is caused by a compromised gut barrier, and loss of antigen tolerance stimulates the differentiation of helper T cells to produce proinflammatory cytokines, including TNF-α, IL-1β, IL-6, IL-12, IL-23, and chemokines (75). H. pylori can also activate Th1 and Th2, causing AAA progression (59). Additionally, the role of L. acidophilus in inducing miRNA and apoptosis in CD4+ memory T cells was investigated and shown to directly affect the occurrence and prevention of AAA (76). Bacteroides fragilis polysaccharide A (PSA) has been shown to impact the Th1/Th2 ratio, which contributes to the development of the immune system. PSA is a component of the cell wall of Bifidobacterium fragilis, which induces the production of IL-10 through gut T cells (77), and the induction of Tregs has been shown to depend on IL-10-producing B cells. Segmented filamentous bacteria can promote the differentiation of Th17 cells (78), and activated Th17 cells play an important role in the occurrence and development of AAA. The interaction of gut bacteria with food mediates immune cell activation, cytokine production, and T lymphocyte proliferation through SCFA metabolism. Importantly, 80% of activated B cells in adults exist in the gut mucosal tissue. IgE action contributes to AngII perfusion-induced mouse AAA growth by increasing inflammation (79). In the process of AAA formation, immunoglobulin G (IgG) immune complexes infiltration interacts with activated Fc receptors (FcγR) in VSMCs to play a pathogenic role. Targeting FcγRs and/or downstream molecules to inhibit humoral immune damage in patients with AAA has become a new immunotherapeutic strategy (80).
Neutrophils are important inflammatory cells for AAA formation and progression, which are rich in MMPs and degrade ECM components. Neutrophils promote the transcription of IL-6 and pro-IL-1β in macrophages, inducing Th17 cell differentiation and recruiting more inflammatory cells (81). However, IL-17, which is produced by the Th17 cell, and the lack of IL-17 receptor may increase Proteobacteria and Bacteroidetes phyla and reduce A. muciniphila, which lead to increased commensal bacterial translocation into the bloodstream (82). Another study showed a significant increase in plaques in LDLR(−/−) mice, together with increased neutrophil infiltration in the aortic root, and decreased concentrations of the anti-inflammatory SCFAs propionate, acetate, and butyrate in the cecum, suggesting that gut microbes can influence the level of neutrophils in the circulation and plaques to mediate plaque growth (83). Moreover, Pg is known to be involved in the pathogenesis of human AAA through neutrophil activation that is associated with neutrophil extracellular trap (NET) formation in the intraluminal thrombus (57). Neutrophil elastase and TNF-α levels were significantly elevated in aneurysm walls covered by thick layers of ILT, while neutrophil gelatinase-associated lipocalin, myeloperoxidase, and neutrophil elastase were positively correlated, and their mediators may infiltrate the thick AAA compartment and weaken the AAA wall (84).
Aortic resident macrophages, blood-derived monocytes, and inflammatory macrophages are significantly expanded in the elastase-induced model of AAA (85). Initiate IL-6 production to accumulate monocytes/macrophages, activate STAT3 and monocyte chemoattractant protein-1 (MCP-1), and ultimately promote the dilation of AAA. Macrophages secrete MMPs to promote the degradation of vascular wall structural fibrin and secrete various pro-inflammatory factors to accelerate migration. Moreover, M1 macrophages can be activated by stimuli such as LPS and IFN-γ, aggravating local inflammation and promoting aortic dilation and vascular remodeling (86). A previous study introduced a Notch receptor inhibitor that upregulates M2 macrophages and downregulates M1 macrophages into ApoE(−/−) mice with AAA, and the results showed significantly improved AAA progression (87). Gut microbiota also contributes to AAA development by regulating macrophage infiltration and inflammatory cytokine expression. Indeed, gut microbiome-dependent metabolites tryptophan and indole-3-acetate can inhibit fatty acids and LPS-stimulated secretion of pro-inflammatory cytokines in macrophages (88). Data have shown that antibiotic administration increases the gut microbiota Firmicutes/Bacteroidetes ratio and expression of CD68+ foam cells, with enhanced M1 polarization in plaques, thereby delaying inflammation within atherosclerotic plaque regression (89). More direct evidence suggests that B. fragilis supplementation in mice on an HFD reduces the abundance of Lactobacillus and increases the abundance of Desulfovibrio, resulting in increased macrophage accumulation in the small intestine and aortic tissue (90).
Shi Laboratory reported the occurrence and accumulation of EOS in AAA lesions, and the lack of EOS-aggravated AAA growth suggested that EOS plays a protective role in AAA (91). ECP has been shown to significantly alter the gut microbiota structure and promote the growth of probiotics in C57BL/6J mice (92). The same study also showed that ECP had different effects on male and female microbiota. In females, ECP increased the abundance of Bifidobacterium and Akkermansia muscaria, while in males, ECP increased the abundance of Lactobacillus spp. (92). Moreover, serum LPS-binding protein of gram-negative bacteria, a well-established biomarker for studying gut antigenic load, was shown to be significantly reduced by ECP to maintain normal gut homeostasis (92). ECP is a novel, food-based, anti-inflammatory agent that alleviates UC by modulating gut dysbiosis (93). Additionally, EOS deficiency leads to altered gut microbiota composition, which in turn may affect EOS function (94) and contribute to gut immune homeostasis.
A previous study showed that increased MC counts are observed in the outer media and adventitia of the patient's AAA tissues; MCs directly enhance the activity of MMP9 produced by monocytes and macrophages (95), suggesting that MCs play a critical role in the progression of AAA. Moreover, patients with AAA have elevated MC proteases, such as chymotrypsin and tryptase, which contribute to leukocyte adhesion and migration, VSMC apoptosis, foam cell formation, and expression of MMPs and cathepsins (96). Additionally, MCs release IL6, IFN-γ, and β-FGF upon activation, induce VSMCs and VECs to express tissue-destructive cathepsins, and promote angiogenesis, which plays harmful roles in AS and AAA (97, 98). Evidence shows that LPS from Rhodobacter sphaeroides leads to the activation of MCs, causing impairment of the intestinal barrier function (99). Despite few reports on the interaction between gut microbes and MCs in the cardiovascular field, the direct or indirect relationship between gut microbes and MCs has been found in inflammatory diseases, such as irritable bowel syndrome (99). It is speculated that the imbalance of the gut microbiome causes MC activation, which will promote the progress of AAA.
DCs were found in all inflammatory infiltrating and contacting lymphocytes in specimens from patients with AAA, suggesting that they are closely related to AAA (100). NET recruits plasmacytoid dendritic cells, induces IFNs and elastase activation, and promotes AAA development in neutrophil-derived dipeptidyl peptidase I -deficient mice (101). Conditional depletion of CD11C+ cells in the ApoE(−/−) mice model of AAA induced by infusion of AngII reduced the maximum diameter of AAA, suggesting that DCs contribute to the development of AAA (102). Previous studies have shown that oral administration of anti-CD3 antibodies or active vitamin D3 reduces AS in mice by recruiting tolerogenic DCs to gut-associated lymphoid tissues (103, 104).
Together, the gut microbiota mediated directly or indirectly damage to the abdominal aorta mainly through various inflammatory cells; the diagram for the mechanism is shown in Figures 2, 3.
Figure 2. Schematic of gut bacterial infection mediated direct injury to aorta. After entering the circulation, gut bacteria can be engulfed by immune cells or directly invade the wall of the aorta. Bacterial LPS induces DC, MC, and MΦ to overexpress TLR4 and activates them into endothelial cells, releasing chymase, tryptase, and tissue-damaging hist proteases, destroying the aortic wall. Injured vascular endothelial cells produce IL-6, IL-8, MCP-1, ICAM-1, activate circulating TC, BC, DC, MC, NK, NKT, and MΦ, and promote their local adhesion and penetration, leading to metastasis to lesions. LPS also induces Th0 differentiation into Th1 and Th2, further activating effector T cells and plasma cells that directly kill bacteria. NK cells are activated by cytokines, bind to infected vascular endothelial cells, release toxic particles, and induce apoptosis. Pg participates in the onset of AAA through neutrophil activation, and neutrophil activation is associated with the formation of NET in ILT. AAA is eventually led by mechanisms, such as extracellular matrix degradation, abnormalities of angiogenesis, proliferation, differentiation, apoptosis, and atherosclerosis. MCP-1, monocyte chemoattractant protein-1; ICAM-1, Intercellular adhesion molecule-1; Pg, Porphyrinum gingivalis; NET, neutrophil extracellular traps; ILT, intraluminal thrombosis.
Figure 3. Schematic of indirect damage to AAA caused by inflammatory response to the gut-aortic axis. Bacteroides fragilis PSA has been shown to impact Th1/Th2 ratio. NLRP3 inflammasomes, members of the NOD-like receptor (NLRs) family, are widely present in vascular endothelial cells and various immune cells. Activation of TLR and NLRP3 inflammasomes during initial immunity can exacerbate vascular wall damage through caspase-mediated apoptosis. MCs, MΦ, and NEU are activated by LPS and then release a variety of pro-inflammatory factors. EOS upregulation of IL4 regulates the polarization of MΦ and monocytes and blocks NF-κB activation in aortic inflammation and vascular wall cells. Notably, IL-6 can chemotact monocytes/macrophages and activate STAT3 and MCP-1 to promote the expansion of the abdominal aorta. Various inflammatory factors and enzymes lead to the formation, growth and rupture of AAA. PSA, polysaccharide A; NLR, NOD-like receptor; TLRs, toll-like receptors.
Gut dysbiosis leads to a decrease in SCFAs and exacerbated inflammation and pulmonary HTN (105). Normal SCFA-producing bacteria include Bacteroides, Prevotella, Iprevo, Ricinobacter butyricum, Eubacterium, Stinrobacter, and Clostridium IV (92). Bacteroides mainly produce acetate and propionate, while Firmicutes mainly produce butyrate (106) and propionate, promoting extrathoracic Treg differentiation (107). Butyrate can also cause gut macrophages and DCs to downregulate LPS-induced pro-inflammatory cytokine production (i.e., NO, IL-6, and IL-12), further supporting its role as an anti-inflammatory metabolite, forming the anti-inflammatory effect of SCFAs (108). Besides, SCFAs also increase the levels of glucagon-like peptide-1 to improve insulin resistance, and these factors are closely related to the pathogenesis of AAA (109).
The bacteria that produce TMA/TMAO are mainly Clostridium, Vibrio desulfuricus, Enterobacter, and Escherichia coli. A previous study found that the proportions of Klebsiella, Pseudomonas, Roche, Proctor, Clostridium, Staphylococcus, Streptococcus, Citrobacter, and Coriolis were significantly increased in patients with pulmonary arterial HTN, which was negatively related to the production of TMA/TMAO (110). TMAO has been shown to be closely related to CVD, and increased plasma TMAO can promote the formation of AS, leading to platelet hyperreactivity and foam cell formation in the aortic root, and can be related to future major adverse cardiovascular events (111). TMAO also promotes the proliferation and migration of VSMCs by upregulating the secretion of inflammatory factors by macrophages (112). Additionally, 3,3-dimethyl-1-butanol can reduce the production of TMAO by inhibiting distinct microbial TMA lyases and alleviating vascular remodeling (112, 113), which will help to inhibit the progression of AAA.
Indole is produced by various symbiotic gram-positive and gram-negative bacteria, such as Escherichia coli, Prevotella, and Bacteroides (110). Gut microbiome imbalance can lead to abnormal tryptophan metabolism, which increases the level of 3-hydroxy-o-aminobenzoic acid through the transcription factor NF-κB, which upregulates MMP2, resulting in the occurrence of AAA (114). Indoleamine 2-3 dioxygenase 1 (IDO) knockout can prevent VSMC apoptosis in AngII-treated LDLr(−/−) mice fed an HFD, indicating that IDO plays a harmful role in the formation of AAA and may be an important target (115). Tryptophan metabolites have both high inflammatory and anti-inflammatory effects, which can cause the development of AS and aneurysms; thus, targeting the tryptophan metabolic pathway will likely assist with AAA treatment (116).
GABA can exert its protective effect against vascular endothelial cells damaged. Oral GABA can inhibit the activity and proliferation of APCs and T cells to reduce the inflammatory response (117). GABA deficiency in humans causes not only mental disorders but also abnormal regulation of blood pressure, inflammatory reactions, and AAA (118, 119). Indeed, Afroz et al. (120) showed that the offspring of patients with HSDs had reduced levels of Lactobacillus, which may hinder the expression of GABA receptors in the male offspring of HSD parents, leading to neurodevelopmental disorders. Moreover, Topiramate, a GABA receptor agonist, can attenuate experimental AAA progression by promoting macrophage preservation and conversion of M1 to M2 macrophage phenotypes (118).
Briefly, gut bacteria and metabolites participate in endotoxemia, intestinal permeability change, insulin resistance, hormone environment, gene expression regulating adipogenesis, bile acid interaction, and inflammatory reaction, which play an important role in the pathogenesis of AAA (Figure 4).
Figure 4. The role of gut microbiota metabolites in the pathogenesis of AAA. Gut microbiome dysbiosis cause the decrease of GABA, Tryptophan, and SCFA produced by certain specific flora, while the increase of TAM and BAs production, which will lead to TC, DC, MAC activating and upregulation of many inflammatory factors (i.e., TNF-α, NF-κ B, IL-1, IL-6, IL-8,IL-12, and MPP-2) to promote AAA formation and progression. GABA, γ-aminobutyric acid; AMP, antimicrobial peptides; GPR, G protein-coupled receptor; ILC, innate lymphocytes; RAAS, renin-angiotensin-aldosterone system; FXR, farnesoid X receptor; TGR5, G-protein-coupled bile acid receptor-1; TMA, Trimethylamine.
Lactobacillus reuteri can decrease the serum levels of triglycerides, LDL, and HHcy, while Parabacteroides goldsteinii reduces the weight of HFD-fed mice, reduces inflammation, and improves insulin resistance (75, 121). Moreover, oral administration of Lactobacillus brevis OW38 to aging mice strengthens gut barrier junctions, reduces circulating LPS levels and pro-inflammatory cytokine expression, and inhibits NF-κB activation (122). Lactobacillus plantarum HAC01 improves metabolic disorder in HFD-induced diabetic mice through the regulation of the gut microbiota (61). Shewanella marinintestina MCCC1 A01703 isolated from the gut tract of marine animals can produce Eicosapentaenoic acid and prevents AAA formation and development by inhibiting the Tak-1-JNK-MMP9 pathway (123). Itaconic acid, which is decomposed by the filamentous fungus Aspergillus terreus (124), inhibits AAA formation by inhibiting vascular inflammation, and treatment with increased itaconic acid may help to prevent AAA formation (125). Recently, a new generation probiotic, A. muciniphila, was found to be significantly reduced in AAA mice, and it was found to improve AS and repair the damaged gut barrier in ApoE(−/−) mice AS model (126). A. muciniphila has also prominence in weight loss, lipid-lowering, blood glucose control, insulin resistance reduction, and anti-inflammatory effects (127). Moreover, genistein, an active isoflavone, alleviates insulin resistance and the inflammatory response by regulating the abundance of genera Bacteroides, Prevotella, Helicobacter, and Ruminococcus in HFD- and streptozotocin-induced T2DM mice (128).
Prebiotics and synbiotics have prominent advantages in improving gut microbiome imbalance and inflammatory state and have become safe options for next-generation therapeutics of chronic diseases and improvement of human health in recent years (129) and may provide prospects for AAA treatment. ECP stimulates the growth of SCFA-producing bacteria, increases the level of SCFAs in the gut, and for the first time, demonstrated that ECP is a novel prebiotic for health promotion and management of dissonance-related diseases (92). Synbiotics are mixtures of prebiotics and probiotics that significantly reduced the risk of cardiovascular and metabolic syndromes and insulin resistance in elderly patients (130), which not only increase the number of gut probiotics, Bifidobacterial and Lactobacillus, but also reduce the proportion of the Coliform group (131). Antibacterial peptides, mainly originating from bacitracin, gramicidin S, and polyxin E, can prevent LPS from binding to TLR4 and triggering inflammation and are expected to be used to treat AAA by inhibiting the vascular inflammatory response (132).
Doxycycline, a broad MMP inhibitor, can prevent aneurysm growth, and life-threatening aneurysm rupture, and reduce the need for expensive invasive therapy in patients with small AAA (133). An interesting report has shown that 2-weeks of doxycycline treatment before aneurysm repair surgery improved the proteolytic balance in AAA (134). DAV13, a powerful antibiotic adsorbent, decreased the free moxifloxacin fecal concentrations by 99% but largely preserved the richness and composition of the gut microbiota (70). A previous clinical trial has proposed that the administration of roxithromycin could limit the growth of AAA (135). Depletion of the gut microbiota was achieved via an oral antibiotic cocktail of vancomycin, metronidazole, ampicillin, and neomycin, to decrease macrophage infiltration and mRNA levels of inflammatory cytokines, which significantly reduced the incidence of aneurysms (136).
Rapamycin is a commonly used immunosuppressant in the clinic. After binding with FK binding protein, rapamycin can inhibit mTOR function. Rapamycin changes not only the host gene expression profile but also the gut subgenome in mice (137). Moreover, an HFD can upregulate the active expression of aortic macrophages, MCP-1, and MMPs, which can be inhibited by rapamycin (41). Resveratrol is a specific inhibitor of the mechanistic target of rapamycin complex 1, which can reduce Lactococcus, Clostridium XI, Oscillibacter, and Hydrogenoanaerobacterium, and can improve the glucose intolerance and insulin resistance of HFD-fed mice (138). Moreover, Sirtuin1 can effectively block NF-κB and MCP-1 from initiating an inflammatory response in VSMCs, representing an important target for preventing the formation of AAA (139). Besides, selectively blocking IL-6 signal transduction with sgp130 can improve the survival rate of AAA mice (140). Cilostazol could reduce macrophage accumulation, MMPs activation, and inflammatory gene expression in the aortic media, which may be a promising new therapeutic option for inhibiting the occurrence and growth of AAA (141). Montelukast, a cysteinyl leukotriene receptor 1 antagonist, also induces M2 macrophage polarization and suppresses gene expression of MMP2, MMP9, and IL-1β, which inhibits murine AAA formation (43). Calcitriol also significantly decreases macrophage infiltration, neovessel formation, and MMP2, MMP9, and vascular endothelial growth factor expression in the suprarenal aortic walls; thus, oral calcitriol can reduce dissecting AAA formation (142). Although the inhibition of MC activity may be a target of AAA therapy, the MC stabilizing drug of pemirolast is ineffective in limiting the progression of AAA (143). A recently discovered novel chemokine, FAM3D, was also found to be significantly upregulated in human AAA tissues, and the application of a FAM3D neutralizing antibody was shown to significantly inhibit the formation of AAA and the infiltration of neutrophils (144).
Nitric oxide (NO), which is produced by nitrate-reducing bacteria including Veillonella, Actinomyces, Haemophilus, and Neisseria (145), is involved in neurotransmission, nerve transmission, vasodilation, and gastrointestinal motility (146), and it has physiological functions in the vascular endothelium via NO synthases. Additionally, macrophages are stimulated by inflammatory cytokines, such as TNF-α, IL-1, and IFN-γ, which can produce NO (147). It has been found that local infection with or dissemination of bacteria causes impairment of NO bioavailability and low circulatory levels of NO, leading to endothelial-dependent vascular dysfunction and the formation of atherosclerotic plaques (148). Evidence has shown that restoring the oral flora and NO activity by utilizing probiotics may be beneficial in treating HTN (145). Therefore, how to increase NO activity is considered a potential therapeutic strategy to treat AAA.
Cholesterol-lowering drugs of the statin family are potent inhibitors of HMGCoA reductase and reduce serum CRP and IL-6 levels significantly (149). Although statins are effective in treating AS by lowering LDL, current cardiovascular strategies aimed at lowering LDL may not prevent AAAs (150). High-density lipoprotein, injected into P. gingivalis-induced AAA rats, led to a significant reduction in AAA diameter and neutrophil activation and effectively prevented AAA progression (151). Additionally, ezetimibe reduced proteolysis and inflammation through MMP9 and IL-6 in the aortic wall to inhibit the progression of AAA (152). Corydalis bungeana, a Chinese herbal medicine, not only has anti-obesity and lipid-lowering effects but also positively regulates the gut S microbiome (40).
Clinically, FMT is mainly used to treat bacterial infections (153) and other non-infectious diseases, such as obesity, diabetes, metabolic syndrome, cancer, and Parkinson's disease, most of which are AAA risk factors (154). Therefore, FMT may also play a role in treating AAA. Recently, capsule-based FMT has been proven to be a clinically effective method to restore the composition of gut microbiota, which provides a simple route of administration and flexibility for clinicians and patients (155).
In summary, the bacterial–gut–aortic axis provides a potential measure for targeting AAA. The full list of interventions is listed in Supplementary Table 2.
We summarized the evidence and risk factors of AAA related to the gut microbiome. The pathological mechanism is mainly based on the direct injury of the gut microbiome to the abdominal aortic wall and the indirect effect of the inflammatory reactions mediated by the gut microbiome imbalance on AAA. The outlined studies prove the importance of the gut microbiome in the pathogenesis of AAA and reveal new targets that can be used to treat AAA. Studying the role of pathophysiological factors related to the intestinal microbiome in the occurrence and development of AAA will provide a new direction for the pathogenesis, biomarkers, and drug R&D of AAA.
JG, XL, and TL conceived and designed the study. XL and WJ wrote the manuscript. XQ, SZ, and KS collected the literature. All authors read and approved the final manuscript.
This work was supported by grants from the Hainan Province Science and Technology special fund (ZDYF2020214 to JG and ZDYF2020122 to WJ), the National Natural Science Foundation of China (82170440 and U220A20270 to JG, 81860075 to TL, and 82260083 to WJ), the National Science Fund for Distinguished Young Scholars (JBGS202104 to JG), Hainan Provincial Nature Foundation (821QN0986 to XL), and the Cardiovascular Disease Research Science Innovation Group of Hainan Medical University.
We thank International Science Editing for editing this manuscript.
The authors declare that the research was conducted in the absence of any commercial or financial relationships that could be construed as a potential conflict of interest.
All claims expressed in this article are solely those of the authors and do not necessarily represent those of their affiliated organizations, or those of the publisher, the editors and the reviewers. Any product that may be evaluated in this article, or claim that may be made by its manufacturer, is not guaranteed or endorsed by the publisher.
The Supplementary Material for this article can be found online at: https://www.frontiersin.org/articles/10.3389/fcvm.2022.1063683/full#supplementary-material
1. Nordon IM, Hinchliffe RJ. Loftus IM, Thompson MM. Pathophysiology and epidemiology of abdominal aortic aneurysms. Nat Rev Cardiol. (2011) 8:92–102. doi: 10.1038/nrcardio.2010.180
3. Choong AM, Woo CC. Foo R, Sorokin V. Genetic and epigenetic mechanisms underlying vascular smooth muscle cell phenotypic modulation in abdominal aortic aneurysm. Int J Mol Sci. (2020) 21:6344. doi: 10.3390/ijms21176334
4. Sanchez-Infantes D, Nus M, Navas-Madronal M, Fite J, Perez B, Barros-Membrilla AJ, et al. Oxidative stress and inflammatory markers in abdominal aortic aneurysm. Antioxidants. (2021) 10:602. doi: 10.3390/antiox10040602
5. Machate DJ, Figueiredo PS., Marcelino G, Guimaraes RCA, Hiane PA, Bogo D, et al. Fatty acid diets: Regulation of gut microbiota composition and obesity and its related metabolic dysbiosis. Int J Mol Sci. (2020) 21:4093. doi: 10.3390/ijms21114093
6. Watanabe N, Koyama S, Tabira M, Matsuno J, Taji Y, Kobayashi K, et al. Infected aortic aneurysm caused by streptococcus pyogenes: a case report. J Infect Chemother. (2021) 27:647–9. doi: 10.1016/j.jiac.2020.11.008
7. Marques da. Silva R, Lingaas PS, Geiran O, Tronstad L, Olsen I. Multiple bacteria in aortic aneurysms. J Vasc Surg. (2003) 38:1384–9. doi: 10.1016/S0741-5214(03)00926-1
8. Kawabata S, Takagaki M, Nakamura H, Oki H, Motooka D, Nakamura S, et al. Dysbiosis of gut microbiome is associated with rupture of cerebral aneurysms. Stroke. (2022) 53:895–903. doi: 10.1161/STROKEAHA.121.034792
9. Xie J, Lu W, Zhong L, Hu Y, Li Q, Ding R, et al. Alterations in gut microbiota of abdominal aortic aneurysm mice. BMC Cardiovasc Disord. (2020) 20:32. doi: 10.1186/s12872-020-01334-2
10. Gu GC, Chen SL., Wang W, Ren JR, Li FD, et al. Differences of gut microbiota diversity between patients with abdominal aortic aneurysm and atherosclerosis. Zhongguo Yi Xue Ke Xue Yuan Xue Bao. (2021) 43:677–84. doi: 10.3881/j.issn.1000-503X.13443
11. Jablonska A, Neumayer C, Bolliger M, Gollackner B, Klinger M, Paradowska E, et al. Analysis of host toll-like receptor 3 and rig-i-like receptor gene expression in patients with abdominal aortic aneurysm. J Vasc Surg. (2018) 68:39S−46S. doi: 10.1016/j.jvs.2017.10.087
12. Haase S, Haghikia A, Wilck N, Muller DN, Linker RA. Impacts of microbiome metabolites on immune regulation and autoimmunity. Immunology. (2018) 154:230–8. doi: 10.1111/imm.12933
13. Lutsey PL, Pankow JS. Guan W. Association of carotid atherosclerosis and stiffness with abdominal aortic aneurysm: The atherosclerosis risk in communities (aric) study. Atherosclerosis. (2018) 270:110–6. doi: 10.1016/j.atherosclerosis.2018.01.044
14. Brady AR, Thompson SG. Fowkes FG, Greenhalgh RM, Powell JT. Participants UKSAT abdominal aortic aneurysm expansion: risk factors and time intervals for surveillance. Circulation. (2004) 110:16–21. doi: 10.1161/01.CIR.0000133279.07468.9F
15. Liu H, Chen X, Hu X, Niu H, Tian R, Wang H, et al. Alterations in the gut microbiome and metabolism with coronary artery disease severity. Microbiome. (2019) 7:68. doi: 10.1186/s40168-019-0683-9
16. Tindall AM, McLimans CJ. Petersen KS, Kris-Etherton PM, Lamendella R. Walnuts and vegetable oils containing oleic acid differentially affect the gut microbiota and associations with cardiovascular risk factors: Follow-up of a randomized, controlled, feeding trial in adults at risk for cardiovascular disease. J Nutr. (2020) 150:806–17. doi: 10.1093/jn/nxz289
17. Tsao CW, Aday AW, Almarzooq ZI, Alonso A, Beaton AZ, Bittencourt MS, et al. Heart disease and stroke statistics-2022 update: A report from the american heart association. Circulation. (2022) 145:e153–639. doi: 10.1161/CIR.0000000000001052
18. Kaneko H, Anzai T, Morisawa M, Kohno T, Nagai T, Anzai A, et al. Resveratrol prevents the development of abdominal aortic aneurysm through attenuation of inflammation, oxidative stress, and neovascularization. Atherosclerosis. (2011) 217:350–7. doi: 10.1016/j.atherosclerosis.2011.03.042
19. Youm YH, Grant RW., McCabe LR, Albarado DC, Nguyen KY, Ravussin A, et al. Canonical nlrp3 inflammasome links systemic low-grade inflammation to functional decline in aging. Cell Metab. (2013) 18:519–32. doi: 10.1016/j.cmet.2013.09.010
20. Zapata HJ, Quagliarello VJ. The microbiota and microbiome in aging: potential implications in health and age-related diseases. J Am Geriatr Soc. (2015) 63:776–81. doi: 10.1111/jgs.13310
21. Kim S, Jazwinski SM. The gut microbiota and healthy aging: a mini-review. Gerontology. (2018) 64:513–20. doi: 10.1159/000490615
22. Li L, Chen B, Zhu R, Li R, Tian Y, Liu C, et al. Fructus ligustri lucidi preserves bone quality through the regulation of gut microbiota diversity, oxidative stress, tmao and sirt6 levels in aging mice. Aging. (2019) 11:9348–68. doi: 10.18632/aging.102376
23. Lutsey PL, Steenson CC. Lifetime risk and risk factors for abdominal aortic aneurysm in a 24-year prospective study: the aric study (atherosclerosis risk in communities) Arterioscler Thromb Vasc Biol. (2016) 36:2468–77. doi: 10.1161/ATVBAHA.116.308147
24. Wang J, Thingholm LB, Skieceviciene J, Rausch P, Kummen M, Hov JR, et al. Genome-wide association analysis identifies variation in vitamin d receptor and other host factors influencing the gut microbiota. Nat Genet. (2016) 48:1396–406. doi: 10.1038/ng.3695
25. Walker MM, Talley NJ. Morrison M. Influence of cigarette smoking on the human duodenal mucosa-associated microbiota. Microbiome. (2018) 6:150. doi: 10.1186/s40168-018-0531-3
26. Huang C, Shi G. Smoking and microbiome in oral, airway, gut and some systemic diseases. J Transl Med. (2019) 17:225. doi: 10.1186/s12967-019-1971-7
27. Wang S, Zhang C, Zhang M, Liang B, Zhu H, Lee J, et al. Activation of amp-activated protein kinase alpha2 by nicotine instigates formation of abdominal aortic aneurysms in mice in vivo. Nat Med. (2012) 18:902–10. doi: 10.1038/nm.2711
28. Cronin O, Walker PJ, Golledge J. The association of obesity with abdominal aortic aneurysm presence and growth. Atherosclerosis. (2013) 226:321–7. doi: 10.1016/j.atherosclerosis.2012.10.041
29. Liu CL, Ren J, Wang Y, Zhang X, Sukhova GK, Liao M, et al. Adipocytes promote interleukin-18 binding to its receptors during abdominal aortic aneurysm formation in mice. Eur Heart J. (2020) 41:2456–68. doi: 10.1093/eurheartj/ehz856
30. Lange LA, Wagner BD. Lozupone CA. Gut microbiota phenotypes of obesity. NPJ Biofilms Microb. (2019) 5:18. doi: 10.1038/s41522-019-0091-8
31. Turnbaugh PJ, Ley RE. Mahowald MA, Magrini V, Mardis ER, Gordon JI. An obesity-associated gut microbiome with increased capacity for energy harvest. Nature. (2006) 444:1027–31. doi: 10.1038/nature05414
32. Cooper HA, Cicalese S, Preston KJ, Kawai T, Okuno K, Choi ET, et al. Targeting mitochondrial fission as a potential therapeutic for abdominal aortic aneurysm. Cardiovasc Res. (2021) 117:971–82. doi: 10.1093/cvr/cvaa133
33. Yang T, Santisteban MM, Rodriguez V, Li E, Ahmari N, Carvajal JM. gut dysbiosis is linked to hypertension. Hypertension. (2015) 65:10. doi: 10.1161/HYPERTENSIONAHA.115.05315
34. Li H, Liu B, Song J, An Z, Zeng X, Li J, et al. Characteristics of gut microbiota in patients with hypertension and/or hyperlipidemia: a cross-sectional study on rural residents in xinxiang county, Henan province. Microorganisms. (2019) 7:399. doi: 10.3390/microorganisms7100399
35. Li J, Zhao F, Wang Y, Chen J, Tao J, Tian G, et al. Gut microbiota dysbiosis contributes to the development of hypertension. Microbiome. (2017) 5:14. doi: 10.1186/s40168-016-0222-x
36. Kim YR, Lee KH., Heo ST. The first case of abdominal mycotic aneurysm caused by k1 hypervirulent klebsiella pneumoniae in a healthy adult. Acute Crit Care. (2021) 36:390–4. doi: 10.4266/acc.2021.00010
37. Kim S, Goel R, Kumar A, Qi Y, Lobaton G, Hosaka K, et al. Imbalance of gut microbiome and intestinal epithelial barrier dysfunction in patients with high blood pressure. Clin Sci. (2018) 132:701–18. doi: 10.1042/CS20180087
38. Wan X, Li T, Liu D, Chen Y, Liu Y, Liu B, et al. Effect of marine microalga chlorella pyrenoidosa ethanol extract on lipid metabolism and gut microbiota composition in high-fat diet-fed rats. Mar Drugs. (2018) 16:498. doi: 10.3390/md16120498
39. Horne RG Yu Y, Zhang R, Abdalqadir N, Rossi L, Surette M, et al. High fat-high fructose diet-induced changes in the gut microbiota associated with dyslipidemia in syrian hamsters. Nutrients. (2020) 12:3557. doi: 10.3390/nu12113557
40. Fu MH, Yu HZ., Bao TR, Ba GN. Anti-obesity and lipid-lowering mechanism of corydalis bungeanae herba: Based on intestinal microflora and metabolomic. Zhongguo Zhong Yao Za Zhi. (2022) 47:3049–58. doi: 10.19540/j.cnki.cjcmm.20211125.701
41. Boada C, Zinger A, Tsao C, Zhao P, Martinez JO, Hartman K, et al. Rapamycin-loaded biomimetic nanoparticles reverse vascular inflammation. Circ Res. (2020) 126:25–37. doi: 10.1161/CIRCRESAHA.119.315185
42. Tomasello G, Mazzola M, Leone A, Sinagra E, Zummo G, Farina F, et al. Nutrition, oxidative stress and intestinal dysbiosis: influence of diet on gut microbiota in inflammatory bowel diseases. Biomed Pap Med Fac Univ Palacky Olomouc. (2016) 160:461–6. doi: 10.5507/bp.2016.052
43. Di Gennaro A, Araujo AC, Busch A, Jin H, Wagsater D, Vorkapic E, et al. Cysteinyl leukotriene receptor 1 antagonism prevents experimental abdominal aortic aneurysm. Proc Natl Acad Sci U S A. (2018) 115:1907–12. doi: 10.1073/pnas.1717906115
44. Arrieta MC, Stiemsma LT, Dimitriu PA, Thorson L, Russell S, Yurist-Doutsch S, et al. Early infancy microbial and metabolic alterations affect risk of childhood asthma. Sci Transl Med. (2015) 7:307ra152. doi: 10.1126/scitranslmed.aab2271
45. Arrieta MC, Arevalo A, Stiemsma L, Dimitriu P, Chico ME, Loor S, et al. Associations between infant fungal and bacterial dysbiosis and childhood atopic wheeze in a nonindustrialized setting. J Allergy Clin Immunol. (2018) 142:424–34. doi: 10.1016/j.jaci.2017.08.041
46. Fujimura KE, Sitarik AR., Havstad S, Lin DL, Levan S, Fadrosh D, et al. Neonatal gut microbiota associates with childhood multisensitized atopy and t cell differentiation. Nat Med. (2016) 22:1187–91. doi: 10.1038/nm.4176
47. Guo JL, Zhang YY., Liu TX, Levy BD, Libby P, Shi GP, et al. Allergic asthma is a risk factor for human cardiovascular diseases. Nat Cardiovas Res. (2022) 1:417–30. doi: 10.1038/s44161-022-00067-z
48. Takagi H, Umemoto T, Group A. Association of chronic obstructive pulmonary, coronary artery, or peripheral artery disease with abdominal aortic aneurysm rupture. Int Angiol. (2017) 36:322–31. doi: 10.23736/S0392-9590.16.03762-7
49. Takagi H, Umemoto T. No association of chronic obstructive pulmonary disease with abdominal aortic aneurysm growth. Heart Vessels. (2016) 31:1806–16. doi: 10.1007/s00380-016-0795-0
50. Bowerman KL, Rehman SF., Vaughan A, Lachner N, Budden KF, Kim RY, et al. Disease-associated gut microbiome and metabolome changes in patients with chronic obstructive pulmonary disease. Nat Commun. (2020) 11:5886. doi: 10.1038/s41467-020-19701-0
51. Folsom AR, Yao L, Alonso A, Lutsey PL, Missov E, Lederle FA, et al. Circulating biomarkers and abdominal aortic aneurysm incidence: the atherosclerosis risk in communities (aric) study. Circulation. (2015) 132:578–85. doi: 10.1161/CIRCULATIONAHA.115.016537
52. Aranson NJ, Lancaster RT., Ergul EA, Conrad MF, LaMuraglia GM, Kwolek CJ, et al. Chronic kidney disease class predicts mortality after abdominal aortic aneurysm repair in propensity-matched cohorts from the medicare population. Ann Surg. (2016) 264:386–91. doi: 10.1097/SLA.0000000000001519
53. Vaziri ND, Wong J, Pahl M, Piceno YM, Yuan J, DeSantis TZ, et al. Chronic kidney disease alters intestinal microbial flora. Kidney Int. (2013) 83:308–15. doi: 10.1038/ki.2012.345
54. Wang IK, Lai HCYu CJ, Liang CC, Chang CT, Kuo HL, et al. Real-time pcr analysis of the intestinal microbiotas in peritoneal dialysis patients. Appl Environ Microbiol. (2012) 78:1107–12. doi: 10.1128/AEM.05605-11
55. Salhi L, Sakalihasan N, Okroglic AG, Labropoulos N, Seidel L, Albert A, et al. Further evidence on the relationship between abdominal aortic aneurysm and periodontitis: a cross-sectional study. J Periodontol. (2020) 91:1453–64. doi: 10.1002/JPER.19-0671
56. Figuero E, Lindahl C, Marin MJ, Renvert S, Herrera D, Ohlsson O, et al. Quantification of periodontal pathogens in vascular, blood, and subgingival samples from patients with peripheral arterial disease or abdominal aortic aneurysms. J Periodontol. (2014) 85:1182–93. doi: 10.1902/jop.2014.130604
57. Delbosc S, Alsac JM, Journe C, Louedec L, Castier Y, Bonnaure-Mallet M, et al. Porphyromonas gingivalis participates in pathogenesis of human abdominal aortic aneurysm by neutrophil activation. Proof concept in rats. PLoS ONE. (2011) 6:e18679. doi: 10.1371/journal.pone.0018679
58. Jones AW, Kirk RS. Bloor K. The association between aneurysm of the abdominal aorta and peptic ulceration. Gut. (1970) 11:679–84. doi: 10.1136/gut.11.8.679
59. Aria H, Kalani M, Hodjati H, Doroudchi M. Different cytokine patterns induced by helicobacter pylori and lactobacillus acidophilus extracts in pbmcs of patients with abdominal aortic aneurysm. Comp Immunol Microbiol Infect Dis. (2020) 70:101449. doi: 10.1016/j.cimid.2020.101449
60. Sweeting MJ, Thompson SG. Brown LC, Powell JT. Meta-analysis of individual patient data to examine factors affecting growth and rupture of small abdominal aortic aneurysms. Br J Surg. (2012) 99:655–65. doi: 10.1002/bjs.8707
61. Park GS, Ko SH, Park J, Lee YK. Lactobacillus plantarum hac01 ameliorates type 2 diabetes in high-fat diet and streptozotocin-induced diabetic mice in association with modulating the gut microbiota. Food Funct. (2021) 12:6363–73. doi: 10.1039/D1FO00698C
62. Mouries J, Brescia P, Silvestri A, Spadoni I, Sorribas M, Wiest R, et al. Microbiota-driven gut vascular barrier disruption is a prerequisite for non-alcoholic steatohepatitis development. J Hepatol. (2019) 71:1216–28. doi: 10.1016/j.jhep.2019.08.005
63. Lareyre F, Moratal C, Zereg E, Carboni J, Panaia-Ferrari P, Bayer P, et al. Association of abdominal aortic aneurysm diameter with insulin resistance index. Biochem Med. (2018) 28:030702. doi: 10.11613/BM.2018.030702
64. Chen PC, Chien YW, Yang SC. The alteration of gut microbiota in newly diagnosed type 2 diabetic patients. Nutrition. (2019) 64:51–6. doi: 10.1016/j.nut.2018.11.019
65. Tsai SH, Hsu LA., Tsai HY, Yeh YH, Lu CY, Chen PC, et al. Aldehyde dehydrogenase 2 protects against abdominal aortic aneurysm formation by reducing reactive oxygen species, vascular inflammation, and apoptosis of vascular smooth muscle cells. FASEB J. (2020) 34:9498–511. doi: 10.1096/fj.201902550RRR
66. Cabreiro F, Au C, Leung KY, Vergara-Irigaray N, Cocheme HM, Noori T, et al. Metformin retards aging in c. elegans by altering microbial folate and methionine metabolism. Cell. (2013) 153:228–39. doi: 10.1016/j.cell.2013.02.035
67. Weersma RK, Zhernakova A, Fu J. Interaction between drugs and the gut microbiome. Gut. (2020) 69:1510–9. doi: 10.1136/gutjnl-2019-320204
68. Javelle E, Mayet A, Million M, Levasseur A, Allodji RS, Marimoutou C, et al. Gut microbiota in military international travelers with doxycycline malaria prophylaxis: Towards the risk of a simpson paradox in the human microbiome field. Pathogens. (2021) 10:1063. doi: 10.3390/pathogens10081063
69. Dong YH, Chang CH. Wang JL, Wu LC, Lin JW, Toh S. Association of infections and use of fluoroquinolones with the risk of aortic aneurysm or aortic dissection. JAMA Intern Med. (2020) 180:1587–95. doi: 10.1001/jamainternmed.2020.4192
70. de Gunzburg J, Ghozlane A, Ducher A, Le Chatelier E, Duval X, Ruppe E, et al. Protection of the human gut microbiome from antibiotics. J Infect Dis. (2018) 217:628–36. doi: 10.1093/infdis/jix604
71. Denner DR, Sangwan N, Becker JB, Hogarth DK, Oldham J, Castillo J, et al. Corticosteroid therapy and airflow obstruction influence the bronchial microbiome, which is distinct from that of bronchoalveolar lavage in asthmatic airways. J Allergy Clin Immunol. (2016) 137:1398–1405. doi: 10.1016/j.jaci.2015.10.017
72. Taylor SL, Leong LEX., Mobegi FM, Choo JM, Wesselingh S, Yang IA, et al. Long-term azithromycin reduces haemophilus influenzae and increases antibiotic resistance in severe asthma. Am J Respir Crit Care Med. (2019) 200:309–17. doi: 10.1164/rccm.201809-1739OC
73. Baines KJ, Yang IA, Upham JW, Reynolds PN. Airway dysbiosis: Haemophilus influenzae and tropheryma in poorly controlled asthma. Eur Respir J. (2016) 47:792–800. doi: 10.1183/13993003.00405-2015
74. Xia N, Lu Y, Gu M, Li N, Liu M, Jiao J, et al. A unique population of regulatory t cells in heart potentiates cardiac protection from myocardial infarction. Circulation. (2020) 142:1956–73. doi: 10.1161/CIRCULATIONAHA.120.046789
75. George AK, Homme RP, Tyagi N, Singh M. Gut microbiota and the periodontal disease: Role of hyperhomocysteinemia. Can J Physiol Pharmacol. (2021) 99:9–17. doi: 10.1139/cjpp-2020-0215
76. Kalani M, Hodjati H, Ghoddusi Johari H, Doroudchi M. Memory t cells of patients with abdominal aortic aneurysm differentially expressed micro rnas 21, 92a, 146a, 155, 326 and 663 in response to helicobacter pylori and lactobacillus acidophilus. Mol Immunol. (2021) 130:77–84. doi: 10.1016/j.molimm.2020.11.007
77. Mazmanian SK, Liu CH, Tzianabos AO, Kasper DL. An immunomodulatory molecule of symbiotic bacteria directs maturation of the host immune system. Cell. (2005) 122:107–18.j.cell.2005.05.007 doi: 10.1016/j.cell.2005.05.007
78. Lee JY, Hall JA., Kroehling L, Wu L, Najar T, Nguyen HH, et al. Serum amyloid a proteins induce pathogenic th17 cells and promote inflammatory disease. Cell. (2020) 183:2036–9. doi: 10.1016/j.cell.2020.12.008
79. Lindholt JS, Sukhova GK., Shi MA, Xia M, Chen H, et al. Ige actions on cd4+ t cells, mast cells, and macrophages participate in the pathogenesis of experimental abdominal aortic aneurysms. EMBO Mol Med. (2014) 6:952–69. doi: 10.15252/emmm.201303811
80. Lopez-Sanz L, Bernal S, Jimenez-Castilla L, Prieto I, La Manna S, Gomez-Lopez S, et al. Fcgamma receptor activation mediates vascular inflammation and abdominal aortic aneurysm development. Clin Transl Med. (2021) 11:e463. doi: 10.1002/ctm2.463
81. An Z, Li J, Yu J, Wang X, Gao H, Zhang W, et al. Neutrophil extracellular traps induced by il-8 aggravate atherosclerosis via activation nf-kappab signaling in macrophages. Cell Cycle. (2019) 18:2928–38. doi: 10.1080/15384101.2019.1662678
82. Perez MM, Martins LMS., Dias MS, Pereira CA, Leite JA, Goncalves ECS, et al. Interleukin-17/interleukin-17 receptor axis elicits intestinal neutrophil migration, restrains gut dysbiosis and lipopolysaccharide translocation in high-fat diet-induced metabolic syndrome model. Immunology. (2019) 156:339–55. doi: 10.1111/imm.13028
83. Petriello MC, Brandon JA., Hoffman J, Wang C, Tripathi H, Abdel-Latif A, et al. Dioxin-like pcb 126 increases systemic inflammation and accelerates atherosclerosis in lean ldl receptor-deficient mice. Toxicol Sci. (2018) 162:548–58. doi: 10.1093/toxsci/kfx275
84. Siennicka A, Adamowicz M, Grzesch N, Klysz M, Wozniak J, Cnotliwy M, et al. Association of aneurysm tissue neutrophil mediator levels with intraluminal thrombus thickness in patients with abdominal aortic aneurysm. Biomolecules. (2022) 12:254. doi: 10.3390/biom12020254
85. Zhao G, Lu H, Chang Z, Zhao Y, Zhu T, Chang L, et al. Single-cell rna sequencing reveals the cellular heterogeneity of aneurysmal infrarenal abdominal aorta. Cardiovasc Res. (2021) 117:1402–16. doi: 10.1093/cvr/cvaa214
86. Funes SC, Rios M, Escobar-Vera J, Kalergis AM. Implications of macrophage polarization in autoimmunity. Immunology. (2018) 154:186–95. doi: 10.1111/imm.12910
87. Koenig SN, Kuivaniemi HS., Garg V, Hans CP. Pharmacological inhibitor of notch signaling stabilizes the progression of small abdominal aortic aneurysm in a mouse model. J Am Heart Assoc. (2014) 3:e001064. doi: 10.1161/JAHA.114.001064
88. Sridharan GV, Sherr DH, Yarmush ML, Alaniz RC, Jayaraman A, Lee K. Gut microbiota-derived tryptophan metabolites modulate inflammatory response in hepatocytes and macrophages. Cell Rep. (2019) 28:3285. doi: 10.1016/j.celrep.2019.08.080
89. Barrett TJ, Wu BG, Wu BG, Gao Z, Blaser MJ, Fisher EA. Reshaping of the gastrointestinal microbiome alters atherosclerotic plaque inflammation resolution in mice. Sci Rep. (2021) 11:8966. doi: 10.1038/s41598-021-88479-y
90. Shi G, Lin Y, Wu Y, Zhou J, Cao L, Chen J, et al. Bacteroides fragilis supplementation deteriorated metabolic dysfunction, inflammation, and aorta atherosclerosis by inducing gut microbiota dysbiosis in animal model. Nutrients. (2022) 14:2199. doi: 10.3390/nu14112199
91. Liu CL, Liu X, Zhang Y, Liu J, Yang C, Luo S, et al. Eosinophils protect mice from angiotensin-ii perfusion-induced abdominal aortic aneurysm. Circ Res. (2021) 128:188–202. doi: 10.1161/CIRCRESAHA.120.318182
92. Shang Q, Wang Y, Pan L, Niu Q, Li C, Jiang H, et al. Dietary polysaccharide from enteromorpha clathrata modulates gut microbiota and promotes the growth of akkermansia muciniphila, bifidobacterium spp. and lactobacillus spp. Mar Drugs. (2018) 16:167. doi: 10.3390/md16050167
93. Lu X, Jing Y, Zhang N, Cao Y. Eurotium cristatum, a probiotic fungus from fuzhuan brick tea, and its polysaccharides ameliorated dss-induced ulcerative colitis in mice by modulating the gut microbiota. J Agric Food Chem. (2022) 70:2957–67. doi: 10.1021/acs.jafc.1c08301
94. Chu VT, Beller A, Rausch S, Strandmark J, Zanker M, Arbach O, et al. Eosinophils promote generation and maintenance of immunoglobulin-a-expressing plasma cells and contribute to gut immune homeostasis. Immunity. (2014) 40:582–93. doi: 10.1016/j.immuni.2014.02.014
95. Tsuruda T, Kato J, Hatakeyama K, Kojima K, Yano M, Yano Y, et al. Adventitial mast cells contribute to pathogenesis in the progression of abdominal aortic aneurysm. Circ Res. (2008) 102:1368–77. doi: 10.1161/CIRCRESAHA.108.173682
96. Wang Y, Shi GP. Mast cell chymase and tryptase in abdominal aortic aneurysm formation. Trends Cardiovasc Med. (2012) 22:150–5. doi: 10.1016/j.tcm.2012.07.012
97. Wolters PJ, MacFarlane LA, Libby P. Mast cells modulate the pathogenesis of elastase-induced abdominal aortic aneurysms in mice. J Clin Invest. (2007) 117:3359–68. doi: 10.1172/JCI31311
98. Sukhova GK, Wolters PJ., Yang M, Kitamoto S, Libby P, et al. Mast cells promote atherosclerosis by releasing proinflammatory cytokines. Nat Med. (2007) 13:719–24. doi: 10.1038/nm1601
99. Gao J, Xiong T, Grabauskas G, Owyang C. Mucosal serotonin reuptake transporter expression in irritable bowel syndrome is modulated by gut microbiota via mast cell-prostaglandin e2. Gastroenterology. (2022) 162:13. doi: 10.1053/j.gastro.2022.02.016
100. Bobryshev YV, Lord RS. Vascular-associated lymphoid tissue (valt) involvement in aortic aneurysm. Atherosclerosis. (2001) 154:15–21. doi: 10.1016/S0021-9150(00)00441-X
101. Yan H, Zhou HF, Akk A, Hu Y, Springer LE, Ennis TL, et al. Neutrophil proteases promote experimental abdominal aortic aneurysm via extracellular trap release and plasmacytoid dendritic cell activation. Arterioscler Thromb Vasc Biol. (2016) 36:1660–9. doi: 10.1161/ATVBAHA.116.307786
102. Krishna SM, Moran CS. Jose RJ, Lazzaroni S, Huynh P, Golledge J. Depletion of cd11c+ dendritic cells in apolipoprotein e-deficient mice limits angiotensin II-induced abdominal aortic aneurysm formation and growth. Clin Sci. (2019) 133:2203–15. doi: 10.1042/CS20190924
103. Sasaki N, Yamashita T, Takeda M, Shinohara M, Nakajima K, Tawa H, et al. Oral anti-cd3 antibody treatment induces regulatory t cells and inhibits the development of atherosclerosis in mice. Circulation. (2009) 120:1996–2005. doi: 10.1161/CIRCULATIONAHA.109.863431
104. Takeda M, Yamashita T, Sasaki N, Nakajima K, Kita T, Shinohara M, et al. Oral administration of an active form of vitamin d3 (calcitriol) decreases atherosclerosis in mice by inducing regulatory t cells and immature dendritic cells with tolerogenic functions. Arterioscler Thromb Vasc Biol. (2010) 30:2495–503. doi: 10.1161/ATVBAHA.110.215459
105. Wu P, Zhu T, Tan Z, Chen S, Fang Z. Role of gut microbiota in pulmonary arterial hypertension. Front Cell Infect Microbiol. (2022) 12:812303. doi: 10.3389/fcimb.2022.812303
106. Den Besten G, van Eunen K, Groen AK, Venema K, Reijngoud DJ, Bakker BM. The role of short-chain fatty acids in the interplay between diet, gut microbiota, and host energy metabolism. J Lipid Res. (2013) 54:2325–40. doi: 10.1194/jlr.R036012
107. Smith PM, Howitt MR., Panikov N, Michaud M, Gallini CA, Bohlooly YM, et al. The microbial metabolites, short-chain fatty acids, regulate colonic treg cell homeostasis. Science. (2013) 341:569–73. doi: 10.1126/science.1241165
108. Chang PV, Hao L, Offermanns S, Medzhitov R. The microbial metabolite butyrate regulates intestinal macrophage function via histone deacetylase inhibition. Proc Natl Acad Sci U S A. (2014) 111:2247–52. doi: 10.1073/pnas.1322269111
109. Jie Yu KM, Wulan B. Zhenhai Yu. Glucagon-like peptide-1 prevented abdominal aortic aneurysm development in rats. Surg Today. (2016) 46:1099–107. doi: 10.1007/s00595-015-1287-z
110. Gazzana MB, Knorst MM., Richards EM, Pepine CJ, et al. Altered gut microbiome profile in patients with pulmonary arterial hypertension. Hypertension. (2020) 75:1063–71. doi: 10.1161/HYPERTENSIONAHA.119.14294
111. Gregory JC, Buffa JA., Org E, Wang Z, Levison BS, Zhu W, et al. Transmission of atherosclerosis susceptibility with gut microbial transplantation. J Biol Chem. (2015) 290:5647–60. doi: 10.1074/jbc.M114.618249
112. Huang Y, Lin F, Tang R, Bao C, Zhou Q, Ye K, et al. Gut microbial metabolite trimethylamine n-oxide aggravates pulmonary hypertension. Am J Respir Cell Mol Biol. (2022) 66:452–60. doi: 10.1165/rcmb.2021-0414OC
113. Roberts AB, Buffa JA., Levison BS, Zhu W, Org E, et al. Non-lethal inhibition of gut microbial trimethylamine production for the treatment of atherosclerosis. Cell. (2015) 163:1585–95. doi: 10.1016/j.cell.2015.11.055
114. Wang Q, Ding Y, Song P, Zhu H, Okon I, Ding YN, et al. Tryptophan-derived 3-hydroxyanthranilic acid contributes to angiotensin ii-induced abdominal aortic aneurysm formation in mice in vivo. Circulation. (2017) 136:2271–83. doi: 10.1161/CIRCULATIONAHA.117.030972
115. Metghalchi S, Vandestienne M, Haddad Y, Esposito B, Dairou J, Tedgui A, et al. Indoleamine 2 3-dioxygenase knockout limits angiotensin ii-induced aneurysm in low density lipoprotein receptor-deficient mice fed with high fat diet. PLoS One. (2018) 13:e0193737. doi: 10.1371/journal.pone.0193737
116. Ramprasath T, Han YM, Zhang D, Yu CJ, Zou MH. Tryptophan catabolism and inflammation: A novel therapeutic target for aortic diseases. Front Immunol. (2021) 12:731701. doi: 10.3389/fimmu.2021.731701
117. Tian J, Yong J, Dang H, Kaufman DL. Oral gaba treatment downregulates inflammatory responses in a mouse model of rheumatoid arthritis. Autoimmunity. (2011) 44:465–70. doi: 10.3109/08916934.2011.571223
118. Chen X, Li Y, Xiao J, Zhang H, Yang C, Wei Z, et al. Modulating neuro-immune-induced macrophage polarization with topiramate attenuates experimental abdominal aortic aneurysm. Front Pharmacol. (2020) 11:565461. doi: 10.3389/fphar.2020.565461
119. Sen S, Roy S, Bandyopadhyay G, Scott B, Xiao D, Ramadoss S, et al. Gamma-aminobutyric acid is synthesized and released by the endothelium: Potential implications. Circ Res. (2016) 119:621–34. doi: 10.1161/CIRCRESAHA.116.308645
120. Afroz KF, Reyes N, Young K, Parikh K, Misra V, Alvina K. Altered gut microbiome and autism like behavior are associated with parental high salt diet in male mice. Sci Rep. (2021) 11:8364. doi: 10.1038/s41598-021-87678-x
121. Wu TR, Lin CS, Chang CJ, Lin TL, Martel J, Ko YF, et al. Gut commensal parabacteroides goldsteinii plays a predominant role in the anti-obesity effects of polysaccharides isolated from hirsutella sinensis. Gut. (2019) 68:248–62. doi: 10.1136/gutjnl-2017-315458
122. Romano L, Gualtieri P, Nicoletti F, Merra G. Neurodegenerative disorders, gut human microbiome and diet: Future research for prevention and supportive therapies. Eur Rev Med Pharmacol Sci. (2018) 22:5771–2. doi: 10.26355/eurrev_201809_15901
123. Kamata R, Bumdelger B, Kokubo H, Fujii M, Yoshimura K, Ishida T, et al. Epa prevents the development of abdominal aortic aneurysms through gpr-120/ffar-4. PLoS ONE. (2016) 11:e0165132. doi: 10.1371/journal.pone.0165132
124. Sun W, Vila-Santa A, Liu N, Prozorov T, Xie D, Faria NT, et al. Metabolic engineering of an acid-tolerant yeast strain pichia kudriavzevii for itaconic acid production. Metab Eng Commun. (2020) 10:e00124. doi: 10.1016/j.mec.2020.e00124
125. Song H, Xu T, Feng X, Lai Y, Yang Y, Zheng H, et al. Itaconate prevents abdominal aortic aneurysm formation through inhibiting inflammation via activation of nrf2. EBioMedicine. (2020) 57:102832. doi: 10.1016/j.ebiom.2020.102832
126. Vanhoutte PM, Woo CW, Xu A. Akkermansia muciniphila protects against atherosclerosis by preventing metabolic endotoxemia-induced inflammation in apoe-/- mice. Circulation. (2016) 133:2434–46. doi: 10.1161/CIRCULATIONAHA.115.019645
127. Depommier C, Everard A, Druart C, Plovier H, Van Hul M, Vieira-Silva S. Supplementation with akkermansia muciniphila in overweight and obese human volunteers: a proof-of-concept exploratory study. Nat Med. (2019) 25:8. doi: 10.1038/s41591-019-0495-2
128. Yang R, Jia Q, Mehmood S, Ma S, Liu X. Genistein ameliorates inflammation and insulin resistance through mediation of gut microbiota composition in type 2 diabetic mice. Eur J Nutr. (2021) 60:2155–68. doi: 10.1007/s00394-020-02403-0
129. Yadav MK, Kumari I, Singh B, Sharma KK, Tiwari SK. Probiotics, prebiotics and synbiotics: Safe options for next-generation therapeutics. Appl Microbiol Biotechnol. (2022) 106:505–21. doi: 10.1007/s00253-021-11646-8
130. Cicero AFG, Fogacci F, Bove M, Giovannini M, Borghi C. Impact of a short-term synbiotic supplementation on metabolic syndrome and systemic inflammation in elderly patients: A randomized placebo-controlled clinical trial. Eur J Nutr. (2021) 60:655–63. doi: 10.1007/s00394-020-02271-8
131. Yang SC, Chen JY., Shang HF, Cheng TY, Tsou SC, Chen JR. Effect of synbiotics on intestinal microflora and digestive enzyme activities in rats. World J Gastroenterol. (2005) 11:7413–7. doi: 10.3748/wjg.v11.i47.7413
132. Lai Y, Gallo RL. Amped up immunity: How antimicrobial peptides have multiple roles in immune defense. Trends Immunol. (2009) 30:131–41. doi: 10.1016/j.it.2008.12.003
133. Khan TM, Patel NP. Chandrasekaran S, De Sousa JFM, Tsouklidis N. Doxycycline therapy for abdominal aortic aneurysm: Inhibitory effect on matrix metalloproteinases. Cureus. (2021) 13:e14966. doi: 10.7759/cureus.14966
134. Verheijen JH, van Bockel JH. Geelkerken RH, Lindeman JH. Doxycycline therapy for abdominal aneurysm: Improved proteolytic balance through reduced neutrophil content. J Vasc Surg. (2009) 49:741–9. doi: 10.1016/j.jvs.2008.09.055
135. Vammen S, Lindholt JS, Ostergaard L, Fasting H, Henneberg EW. Randomized double-blind controlled trial of roxithromycin for prevention of abdominal aortic aneurysm expansion. Br J Surg. (2001) 88:1066–72. doi: 10.1046/j.0007-1323.2001.01845.x
136. Shikata F, Shimada K, Sato H, Ikedo T, Kuwabara A, Furukawa H, et al. Potential influences of gut microbiota on the formation of intracranial aneurysm. Hypertension. (2019) 73:491–6. doi: 10.1161/HYPERTENSIONAHA.118.11804
137. Bitto A, Ito TK, Pineda VV, LeTexier NJ, Huang HZ, Sutlief E, et al. Transient rapamycin treatment can increase lifespan and healthspan in middle-aged mice. Elife. (2016) 5: e16351. doi: 10.7554/eLife.16351
138. Shin NR, Kim MS, Hyun DW, Yun JH. Chronic repression of mtor complex 2 induces changes in the gut microbiota of diet-induced obese mice. Sci Rep. (2016) 6:30887. doi: 10.1038/srep30887
139. Chen HZ, Wang F, Gao P, Pei JF, Liu Y, Xu TT, et al. Age-associated sirtuin 1 reduction in vascular smooth muscle links vascular senescence and inflammation to abdominal aortic aneurysm. Circ Res. (2016) 119:1076–88. doi: 10.1161/CIRCRESAHA.116.308895
140. Paige E, Clement M, Lareyre F, Sweeting M, Raffort J, Grenier C, et al. Interleukin-6 receptor signaling and abdominal aortic aneurysm growth rates. Circ Genom Precis Med. (2019) 12:e002413. doi: 10.1161/CIRCGEN.118.002413
141. Umebayashi R, Uchida HA, Kakio Y, Subramanian V, Daugherty A, Wada J. Cilostazol attenuates angiotensin II-induced abdominal aortic aneurysms but not atherosclerosis in apolipoprotein e-deficient mice. Arterioscler Thromb Vasc Biol. (2018) 38:903–12. doi: 10.1161/ATVBAHA.117.309707
142. Martorell S, Hueso L, Gonzalez-Navarro H, Collado A, Sanz MJ, Piqueras L. Vitamin d receptor activation reduces angiotensin-II-induced dissecting abdominal aortic aneurysm in apolipoprotein e-knockout mice. Arterioscler Thromb Vasc Biol. (2016) 36:1587–97. doi: 10.1161/ATVBAHA.116.307530
143. Sillesen H, Eldrup N, Hultgren R, Lindeman J, Bredahl K, Thompson M, et al. Randomized clinical trial of mast cell inhibition in patients with a medium-sized abdominal aortic aneurysm. Br J Surg. (2015) 102:894–901. doi: 10.1002/bjs.9824
144. He L, Fu Y, Deng J, Shen Y, Wang Y, Yu F, et al. Deficiency of fam3d (family with sequence similarity 3, membered), a novel chemokine, attenuates neutrophil recruitment and ameliorates abdominal aortic aneurysm development. Arterioscler Thromb Vasc Biol. (2018) 38:1616–31. doi: 10.1161/ATVBAHA.118.311289
145. Pignatelli P, Fabietti G, Ricci A, Piattelli A, Curia MC. How periodontal disease and presence of nitric oxide reducing oral bacteria can affect blood pressure. Int J Mol Sci. (2020) 21:7538. doi: 10.3390/ijms21207538
146. Walker MY, Pratap S, Southerland JH, Farmer-Dixon CM, Lakshmyya K, Gangula PR. Role of oral and gut microbiome in nitric oxide-mediated colon motility. Nitric Oxide. (2018) 73:81–8. doi: 10.1016/j.niox.2017.06.003
147. Poorsattar Bejeh-Mir A, Parsian H, Akbari Khoram M, Ghasemi N, Bijani A, Khosravi-Samani M. Diagnostic role of salivary and gcf nitrite, nitrate and nitric oxide to distinguish healthy periodontium from gingivitis and periodontitis. Int J Mol Cell Med. (2014) 3:138–45.
148. Velsko IM, Chukkapalli SS., Rivera MF, Lee JY, Chen H, Zheng D, et al. Active invasion of oral and aortic tissues by porphyromonas gingivalis in mice causally links periodontitis and atherosclerosis. PLoS ONE. (2014) 9:e97811. doi: 10.1371/journal.pone.0097811
149. Young RP, Hopkins R, Eaton TE. Pharmacological actions of statins: Potential utility in copd. Eur Respir Rev. (2009) 18:222–32. doi: 10.1183/09059180.00005309
150. Bonder MJ, Cenit MC., Tigchelaar EF, Maatman A, Dekens JA, et al. The gut microbiome contributes to a substantial proportion of the variation in blood lipids. Circ Res. (2015) 117:817–24. doi: 10.1161/CIRCRESAHA.115.306807
151. Delbosc S, Rouer M, Alsac JM, Louedec L, Al Shoukr F, Rouzet F, et al. High-density lipoprotein therapy inhibits porphyromonas gingivalis-induced abdominal aortic aneurysm progression. Thromb Haemost. (2016) 115:789–99. doi: 10.1160/TH15-05-0398
152. Loftus IM, Cockerill GW, Thompson MM. A randomised placebo-controlled double-blind trial to evaluate lipid-lowering pharmacotherapy on proteolysis and inflammation in abdominal aortic aneurysms. Eur J Vasc Endovasc Surg. (2011) 41:28–35. doi: 10.1016/j.ejvs.2010.08.023
153. Kim KO, Gluck M. Fecal microbiota transplantation: an update on clinical practice. Clin Endosc. (2019) 52:137–43. doi: 10.5946/ce.2019.009
154. Antushevich H. Fecal microbiota transplantation in disease therapy. Clin Chim Acta. (2020) 503:90–8. doi: 10.1016/j.cca.2019.12.010
Keywords: gut microbiome, abdominal aortic aneurysm, risk factors, pathogenesis, treatment
Citation: Ling X, Jie W, Qin X, Zhang S, Shi K, Li T and Guo J (2022) Gut microbiome sheds light on the development and treatment of abdominal aortic aneurysm. Front. Cardiovasc. Med. 9:1063683. doi: 10.3389/fcvm.2022.1063683
Received: 07 October 2022; Accepted: 03 November 2022;
Published: 25 November 2022.
Edited by:
Junjie Xiao, Shanghai University, ChinaReviewed by:
Zhi Xin Shan, Guangdong Provincial People's Hospital, ChinaCopyright © 2022 Ling, Jie, Qin, Zhang, Shi, Li and Guo. This is an open-access article distributed under the terms of the Creative Commons Attribution License (CC BY). The use, distribution or reproduction in other forums is permitted, provided the original author(s) and the copyright owner(s) are credited and that the original publication in this journal is cited, in accordance with accepted academic practice. No use, distribution or reproduction is permitted which does not comply with these terms.
*Correspondence: Junli Guo, R3VvamxAaGFpbm1jLmVkdS5jbg==
†These authors have contributed equally to this work
Disclaimer: All claims expressed in this article are solely those of the authors and do not necessarily represent those of their affiliated organizations, or those of the publisher, the editors and the reviewers. Any product that may be evaluated in this article or claim that may be made by its manufacturer is not guaranteed or endorsed by the publisher.
Research integrity at Frontiers
Learn more about the work of our research integrity team to safeguard the quality of each article we publish.