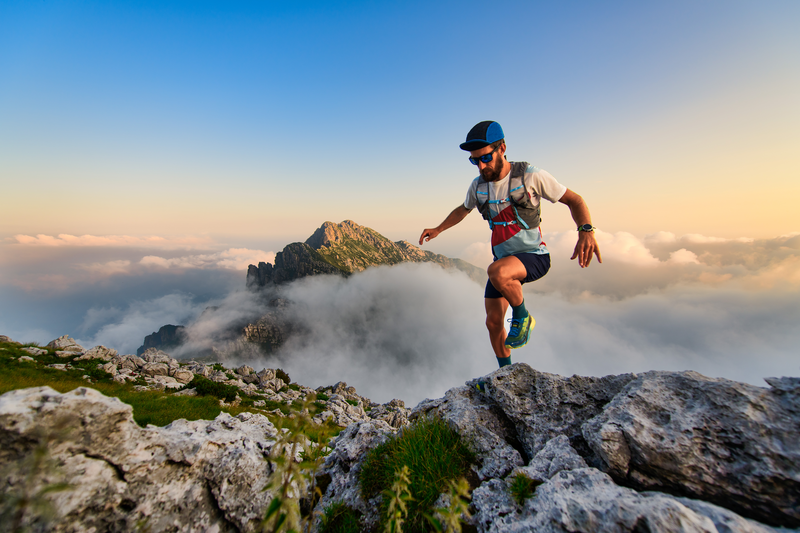
94% of researchers rate our articles as excellent or good
Learn more about the work of our research integrity team to safeguard the quality of each article we publish.
Find out more
ORIGINAL RESEARCH article
Front. Cardiovasc. Med. , 23 November 2022
Sec. Hypertension
Volume 9 - 2022 | https://doi.org/10.3389/fcvm.2022.1048507
Background: Non-invasive estimation of central blood pressure (BP) may have better prognostic value than brachial BP. The accuracy of central BP is limited in certain populations, such as in females and the elderly. This study aims to examine whether statistical modeling of central BP for clinical and hemodynamic parameters results in enhanced accuracy.
Methods: This study is a cross-sectional analysis of 500 patients who underwent cardiac catheterization. Non-invasive brachial cuff and central BP were measured simultaneously to invasive aortic systolic BP (AoSBP). Central BP was calibrated for brachial systolic (SBP) and diastolic BP (Type I calibration; C1SBP) or brachial mean and diastolic BP (Type II calibration; C2SBP). Differences between central SBP and the corresponding AoSBP were assessed with linear regression models using clinical and hemodynamic parameters. These parameters were then added to C1SBP and C2SBP in adjusted models to predict AoSBP. Accuracy and precision were computed in the overall population and per age or sex strata.
Results: C1SBP underestimated AoSBP by 11.2 mmHg (±13.5) and C2SBP overestimated it by 6.2 mmHg (±14.8). Estimated SBP amplification and heart rate were the greatest predictors of C1- and C2-AoSBP accuracies, respectively. Statistical modeling improved both accuracy (0.0 mmHg) and precision (±11.4) but more importantly, eliminated the differences of accuracy seen in different sex and age groups.
Conclusion: Statistical modeling greatly enhances the accuracy of central BP measurements and abolishes sex- and age-based differences. Such factors could easily be implemented in central BP devices to improve their accuracy.
Hypertension is the leading risk factor for global disease burden worldwide (1). It has been associated with numerous adverse health outcomes such as cardiovascular disease, dementia, and chronic kidney disease (2, 3), and is responsible for annual health costs of more than 130 billion dollars in the United States alone (4). Brachial cuff measurement of blood pressure (BP) has been used for more than a century to diagnose and guide the management of hypertension. While brachial BP is clinically convenient and was shown in a meta-analysis to closely approximate the true (invasively measured) aortic BP at the populational level (mean difference of 0.3 mmHg), it remains imprecise at the individual level (mean absolute differences of 8 mmHg) (5).
Since direct measurement of the true aortic BP is not feasible on a routine basis, non-invasive methods to estimate central BP have been developed. Non-invasive aortic BP (herein referred to as “central BP”) estimating devices use tonometry or cuff-based methods to capture a peripheral waveform that can be transformed into an estimated central waveform after calibration with either systolic BP (SBP) and diastolic BP (DBP; Type I calibration) or mean BP (MAP) and DBP (Type II calibration) (6, 7). Cuff-based methods can also be extended to 24 h monitoring to provide nocturnal and diurnal patterns of central BP (8, 9). While these non-invasive measurements of central BP have been found to be superior predictors of cardiovascular risk and end-organ damage compared to brachial BP (10–16), they are limited by a dependency from accurate brachial cuff BP measures which limits their routine clinical use. Both radial tonometry and brachial-cuff devices have indeed been shown to underestimate the true aortic SBP (AoSBP) by 5–8 mmHg when using Type I calibration (17, 18). Since this underestimation is not seen when invasive measurements of brachial BP are used for calibration (17), it was hypothesized that the use of brachial cuff SBP (which is known to underestimate the true brachial SBP) was partly responsible for the inaccuracy between central SBP and AoSBP (5). Type II calibration of central SBP, which does not rely on SBP but on MAP, was instead shown to be more accurate while slightly overestimating AoSBP (19).
Although the inaccuracy between central BP and the true aortic SBP was traditionally attributed to errors in the measurement of brachial cuff BP, recent studies conducted by our group have shown that several clinical characteristics (notably female sex and height) influenced the accuracy between non-invasive and invasive brachial BP (20, 21). Since central BP measurements are highly dependent on an accurate brachial cuff BP for calibration, we hypothesized that adjusting for such clinical parameters could enhance the performance of central BP devices. Therefore, using a cohort of patients with true aortic BP measurements made during cardiac catheterization, we aimed to (1) identify which clinical parameters are associated with the inaccuracy between central BP and true aortic BP measurements; (2) evaluate the performance of models based on these clinical parameters in the prediction of true aortic BP measurements; and (3) assess the impact of these clinical parameters on age and sex-associated differences in accuracy.
Data from this study was derived from a prospective cohort of patients undergoing clinically indicated cardiac catheterization (for either suspected coronary artery disease or acute coronary syndromes) at the Hôpital du Sacré-Coeur de Montréal (Canada) between January and December 2019 (20). Patients were included unless: (1) they had previously documented severe aortic stenosis or atrial fibrillation (since their pulse wave characteristics may differ from the general population); (2) pre-catheterization inter-arm SBP/DBP difference was elevated (>10 mmHg); or (3) invasive and non-invasive BP measures could not be taken simultaneously for safety, time or technical reasons (notably if a patient urgently required coronary angiography due to its clinical condition). Clinical data was obtained by medical chart review. Written consent was obtained before inclusion in the study. Our study was conducted following the ARTERY Society task force consensus statement on protocol standardization (22) and was approved by the institutional ethics review board of the CIUSSS du Nord-de-l’île-de-Montréal. All investigators adhered to the Declaration of Helsinki.
True aortic BPs were measured in hemodynamically stable conditions at the end of the cardiac catheterization procedure by the cardiologist responsible for the intervention. Intra-arterial vasodilators were given after radial artery cannulation, but no vasoactive or contrast agent was given at least 5 min prior to measurements of all invasive BP. A fluid-filled catheter (5F or 6F) was connected to the Xper Flex Cardio Physiomonitoring System (Phillips, Amsterdam, Netherlands) with the manifold position maintained at heart level on the angiography table. Following the cardiac procedure, the catheter tip was positioned using fluoroscopy within 3 cm of the aortic valve in the aorta. Blood was then aspirated to remove bubbles and the catheter was flushed with 10 ml of 0.9% sodium chloride solution. The monitoring system was zeroed and calibrated before each measure. After the calibration of the system and visual confirmation of the correct waveform, BP measures were recorded for every heartbeat during 20 s and then averaged.
Non-invasive BP was measured in the arm contralateral to the arterial access using the Mobil-O-Graph NG device (I.E.M., Stolberg, Germany) with an appropriately sized cuff (7, 23). Trained research personnel were responsible for non-invasive BP measurements. The Mobil-O-Graph device first measures brachial cuff BP using a validated oscillometric procedure with stepwise deflation of the cuff. Then, it reinflates at a pressure below the brachial cuff DBP for 10 s to capture and generate a brachial pulse waveform. This waveform is then converted into an aortic waveform using a generalized transfer function. This conversion can either be calibrated for brachial SBP and DBP (Type I calibration; C1SBP) or mean BP and DBP (Type II calibration; C2SBP). Other aortic parameters {augmentation index corrected for 75 bpm [100 × (pulse pressure − incident pressure wave height) ÷ pulse pressure], reflection magnitude (backward ÷ forward component of the waveform), estimated aortic pulse wave velocity, SBP amplification (brachial cuff SBP − C1SBP)} can be derived using this waveform. The inflation sequence was timed such that invasive aortic and non-invasive BP measurements coincided.
Analyses were conducted with R 4.1.0 (R Project for Statistical Computing). p-Values under 0.05 were considered significant. Categorical descriptive characteristics were presented as counts (percentages) and continuous characteristics as either means (±SD) or medians (interquartile range) according to their distribution. Accuracy was calculated as the mean difference between a non-invasive/estimated BP and the corresponding invasive/true BP and was assessed in both the overall population and in age and sex-strata (22). Differences between AoSBP and central SBP were modelized using linear regression models with pre-specified clinical characteristics (demographics, comorbidities, and hemodynamic parameters) as predictors and either C1SBP or C2SBP as outcome. The role of each predictor was assessed by computing its effect on the mean difference (the beta coefficient in the linear regression model), its p-value and its coefficient of determination (partial R2; computed by the rsq package). Global R2 and mean differences were also computed. Absence of multicollinearity was verified using variance inflation factors, which were all below 5.
The performance of central SBP either alone or with the addition of clinical and non-invasive hemodynamic parameters was assessed using linear regression models with AoSBP as an outcome. Successively tested models included C1SBP alone, C2SBP alone, C1SBP-C2SBP combined, and then four adjusted models including C1SBP and C2SBP plus clinical parameters: (1) a demographics model, using age, and sex; (2) a pulse wave analysis (PWA) model using the demographics model plus heart rate and PWA parameters (SBP amplification, augmentation index, reflection magnitude, and estimated aortic pulse wave velocity); (3) a full model using the parameters of the PWA model plus height, weight, eGFR, active smoking, diabetes, anti-hypertensive medications, statin use, and aspirin use; and (4) a model with parameters contained in the full but selected using a least absolute shrinkage and selection operator (LASSO) procedure. Performance was assessed using accuracy, precision (SD of the accuracy), goodness-of-fit (R2) and the percentage of patients with non-invasive SBP within ±10 mmHg of the corresponding AoSBP measurement. Models were also assessed after a bootstrap resampling procedure of 1,000 iterations. As sensitivity analysis, height and weight were replaced by body mass index in the fully adjusted model. The impact of adjustment for clinical parameters was also assessed by building modified Bland–Altman plots for both C1SBP and C2SBP either before or after adjustment (22). Finally, the impact of adjustment on age- (40–60, 60–80, and ≥80 years old) and sex-specific differences was calculated.
Characteristics of the 500 participants included in this study are displayed in Table 1. The mean age was 65.7 ± 10.0 years and 71% were men. Most patients used at least one anti-hypertensive medication (79.8%), aspirin (70.8%), or a statin (69.6%). Mean brachial cuff SBP was 124.4 mmHg (±16.8) and mean brachial cuff DBP was 76.6 mmHg (±10.8). The mean true aortic SBP was 126.5 mmHg (±21.7) while mean C1SBP was 115.4 mmHg (±16.2) and C2SBP was 132.7 mmHg (±18.1). The distribution of C1SBP and C2SBP according to AoSBP are displayed in Figure 1.
Figure 1. Scatter plots of brachial SBP/estimated central SBP with invasive aortic SBP. Each gray dot represents the relationship between invasive aortic SBP and brachial SBP/estimated central SBP for a given individual. Correlation coefficients, regression slopes and mean accuracy for aortic SBP are displayed with their 95th confidence interval. (A) Brachial SBP; (B) C1SBP; (C) C2SBP; and (D) adjusted central SBP (full model). SBP, systolic blood pressure.
As displayed in Table 2, brachial cuff SBP was 2.1 mmHg lower than AoSBP (with precision, or SD, of ±12.1) while C1SBP underestimated AoSBP by 11.2 mmHg (±13.5) and C2SBP overestimated AoSBP by 6.2 mmHg (±14.8). When these differences were stratified by age and sex (Table 2 and Figures 2, 3), the underestimation of AoSBP by brachial SBP and C1SBP was more pronounced in older patients and in women. Similarly, the overestimation of AoSBP by C2SBP was more pronounced in younger patients and in men. These differences were statistically significant for age (brachial SBP and C1SBP) and for sex (brachial SBP, C1SBP, and C2SBP).
Figure 2. Differences between brachial SBP/estimated central SBP and invasive aortic SBP by age before and after adjustment. The distribution of differences by age strata are displayed by boxplots before and adjustment (full model). The horizontal black line in the middle of each box indicates the median, the square extremities indicate the interquartile range, and the vertical black lines end at either the minimum (first quartile minus 1.5 times the interquartile range) or the maximum (third quartile plus 1.5 times the interquartile range). Gray dots indicate outliers. The full models include the age, sex, heart rate, estimated SBP amplification, pulse wave velocity, augmentation index at 75 bpm, reflection magnitude, height, weight, eGFR, active smoking, diabetes, and antihypertensive treatment. C1SBP, Type I calibrated central SBP; C2SBP, Type II calibrated central SBP; eGFR, estimated glomerular filtration rate; SBP, systolic blood pressure.
Figure 3. Differences between brachial SBP/estimated central SBP and invasive aortic SBP by sex before and after adjustment. The distribution of differences by sex strata are displayed by boxplots before and adjustment (full model). The horizontal black line in the middle of each box indicates the median, the square extremities indicate the interquartile range, and the vertical black lines end at either the minimum (first quartile minus 1.5 times the interquartile range) or the maximum (third quartile plus 1.5 times the interquartile range). Gray dots indicate outliers. The full models include the age, sex, heart rate, estimated SBP amplification, pulse wave velocity, augmentation index at 75 bpm, reflection magnitude, height, weight, eGFR, active smoking, diabetes, antihypertensive treatment. C1SBP, Type I calibrated central SBP; C2SBP, Type II calibrated central SBP; eGFR, estimated glomerular filtration rate; SBP, Systolic blood pressure.
As shown in Supplementary Table 1, 27.9% of the mean difference between C1SBP and AoSBP could be explained by clinical parameters contained in a linear regression model. Higher SBP amplification significantly increased the underestimation of AoSBP by C1SBP and was the factor with the most elevated partial R2 in the model (10.1%). Other parameters significantly associated with mean differences between C1SBP and AoSBP were height (partial R2 = 1.8%), age (1.1%) and augmentation index at 75 bpm (0.9%).
A slightly smaller share of the mean differences between C2SBP and AoSBP (25.2%) was explained by clinical parameters contained in a linear regression model (Supplementary Table 2). Patients with lower heart rate had higher levels of overestimation (+4.7 mmHg per 10 bpm decrease in heart rate). Besides heart rate (partial R2 = 11.8%), augmentation index at 75 bpm (1.4%), age (1.1%) and estimated SBP amplification (0.8%) were significantly associated with mean differences between C2SBP and AoSBP.
Table 3 displays the impact of various levels of adjustment on the prediction of AoSBP by estimated central SBP. By design, all models yielded accuracies of 0.0 mmHg. Compared to either C1SBP or C2SBP alone, combining both increased precision, model R2 and the percentage of patients with predicted SBP between ±10 mmHg of the AoSBP. Similarly, successively adding clinical characteristics also increased predictive performance. The full model, adjusting for all clinical and hemodynamic characteristics with both C1SBP and C2SBP, resulted in the best enhancement of central BP with a precision of 11.4 mmHg, a model R2 of 69.6 and 66.6% of patients within 10 mmHg of the reference invasive value. This performance was higher than the one observed for brachial SBP. A simpler model with characteristics chosen by a LASSO procedure (C1SBP, C2SBP, age, sex, estimated SBP amplification, augmentation index at 75 bpm, and height) resulted in similar predictive characteristics while containing a much smaller number of predictors. Interestingly, most characteristics identified by the LASSO procedure were the ones significantly associated with mean differences between crude central SBP estimates and AoSBP. Similar findings concerning accuracy, precision, R2 and patients within 10 mmHg were obtained after a bootstrap procedure using 1,000 resamples. Sensitivity analyses replacing height and weight by body mass index in the fully adjusted model yielded similar accuracy (difference of 0 mmHg), precision (11.5 mmHg), R2 (69.0) and percentage of patients within 10 mmHg of reference value (67.2%).
The impact of adjustment for clinical characteristics was also assessed by building modified Bland–Altman plots for brachial SBP, C1SBP, C2SBP, and full model-adjusted central SBP. As shown in Figure 4, the underestimation of AoSBP by crude non-invasive SBP (brachial SBP, C1SBP, and C2SBP) was more pronounced at higher values, yielding negative Bland–Altman slopes. Adjustment for previously stated characteristics led to the smallest slope of Bland–Altman plots (slope = −0.30 [−0.34, −0.26]) among the four non-invasive parameters evaluated.
Figure 4. Bland–Altman plots for aortic SBP before and after adjustment. Modified Bland–Altman plots show the reference intra-arterial measurements (aortic SBP) on the X-axis instead of the mean of both measurements. Left to right panels show in order the agreement of brachial cuff SBP, C1SBP, and C2SBP with aortic SBP. All values are in mmHg. Red dashed lines and red solid lines represent mean differences (bias) and limits of agreement (bias ± 2 SDs) respectively. C1SBP, Type I central systolic blood pressure; C2SBP, Type II systolic blood pressure; SBP, systolic blood pressure.
Finally, adjusting for clinical characteristics eliminated the age- and sex-associated differences in accuracy between CSBP and AoSBP, as shown in Table 2 and Figures 2, 3. Resulting p-values for age and sex differences were respectively of 0.859 (age) and 1.000 (sex).
In our cohort of 500 patients with simultaneous invasive and non-invasive BP measurements, we identified several parameters that contributed to the accuracy and precision of central BP measurements toward the true (invasive) aortic SBP. We then showed that by adjusting central SBP measurements with clinical and non-invasive hemodynamical characteristics, the predictive performance of central BP can be significantly enhanced and that inaccuracies inherent to age and sex can be eliminated.
In this study, we first observed that Type I calibration central BP underestimated AoSBP while Type II calibration central BP overestimated it. These findings were comparable to previous studies (17–19), but the underestimation of AoSBP by C1SBP in our study was larger in magnitude than the one observed in a previous study validating the Mobil-O-Graph device. Nevertheless, this degree of underestimation is in line with the range of differences observed in a previous meta-analysis of studies using tonometer-based devices, in which underestimations as high as 15 mmHg have been reported. Although the gap between true aortic and central SBP has been traditionally attributed to imprecisions in the brachial cuff BPs used for calibration, we aimed to evaluate whether clinical characteristics might be linked to accuracy. We observed that elevated estimated SBP amplification was the strongest determinant of the underestimation of AoSBP by C1SBP. Since this parameter is computed by subtracting C1SBP from brachial cuff SBP, it was expected that patients with lower C1SBP (and therefore higher estimated SBP amplification) would have greater levels of underestimation (24). Nevertheless, we observe that a parameter solely derived from estimated values could identify patients with a greater inaccuracy between estimated and invasive AoSBP. In contrast, we observed that low heart rate was the strongest determinant of the overestimation of AoSBP by C2SBP. Again, this finding can be explained by the changes in arterial waveform shape at lower heart rates (notably an earlier reflected wave) which decreases the peripheric to central BP ratio and could lead to overestimation of aortic SBP (25). In addition to these two parameters, we showed that higher age and augmentation index were associated with increased underestimation of AoSBP by both C1SBP and C2SBP. This relationship may reflect the greater loss of peripheral pulse pressure amplification seen in patients with high aortic stiffness (associated with age and the augmentation index) that is not fully accounted for by the generalized transfer function (26–29).
According to the ARTERY Society guidelines (22), an accurate central BP device should have an overall accuracy within 5 mmHg and a precision within 8 mmHg compared to reference invasive measurements. These thresholds of accuracy and precision have nevertheless not been met in most previous validation studies using tonometry or cuff-based devices, which still limits the clinical application of central BP (17–19). In our study, neither the two calibrations of central SBP nor usual brachial SBP did fulfill the ARTERY standards relative to invasive aortic SBP. Hence, adjusted models using clinical and hemodynamic parameters were tested. Compared to unadjusted models, models adding PWA and clinical parameters to central SBP led to a mean accuracy of 0 mmHg in the global and in the age and sex-stratified populations. While this finding was expected with statistical modeling, a similar accuracy was observed even after bootstrap validation, thus reinforcing our findings concerning the role of adjustment on central SBP accuracy. Furthermore, adjustment for these parameters also increased the goodness-of-fit (as demonstrated by the increase of the calculated R2), the precision (or SD) and the percentage of patients within 10 mmHg of the invasive aortic SBP in the overall population. These results are in line with the recent work of Esposito et al., who studied 93 patients undergoing cardiac catheterization and reported that the 6.4 mmHg inaccuracy (6.4 mmHg) and precision (17.4 mmHg) between CSBP and AoSBP were improved after the use of a linear-fit function (30). However, in contrast with our study, these authors did not include any clinical or hemodynamical parameters in their equation (only a slope and an intercept), did not study C1SBP and C2SBP separately, used SphygmoCor rather than the Mobil-O-Graph, and had a smaller sample size than ours.
It has previously been shown that at similar brachial SBPs, women appear to have higher CVD risk (31, 32). Hypotheses such as smaller vessel caliber or more pronounced aging-related loss of compliance have been proposed to explain this association (33, 34). As such, our team has recently shown that female sex is associated with a greater inaccuracy of brachial cuff, C1SBP for AoSBP while male sex is associated with greater inaccuracy of C2SBP for predicting AoSBP (20, 21). As expected, we obtained similar findings in this study using the same cohort. However, we expanded this previous work by showing that differences in accuracy were also observed for age in both brachial SBP, C1SBP, and C2SBP. These differences were easily eliminated by adding clinical and hemodynamical confounders to C1SBP and C2SBP. In short, statistical modeling erased the overall inaccuracy of both Type I and Type II central SBP, removed age- and sex-related differences in accuracy, and slightly improved other predictive parameters.
Interestingly, we also showed that a model containing only variables selected by LASSO led to an improvement similar to our fully adjusted model, but with much less complexity. This simplified model includes easily obtained characteristics (age, sex, and height) and variables automatically calculated by the Mobil-O-Graph (Type I and II central SBPs, estimated SBP amplification and augmentation index at 75 bpm) which could facilitate its implementation. Nevertheless, while all predictive parameters were improved by adjustment, none of the obtained models had a precision ≤ 8 mmHg when compared to invasive measurements, hence not adhering to the precision criteria as per ARTERY guidelines (22). This observation is coherent with the fact that only 25–28% of the invasive-estimated inaccuracy was explained by clinical parameters, thus leading to only a relatively small improvement in precision after adjustment. Our findings thus reinforce the major role of inter-individual differences in pulse waveforms across the arterial tree in central BP estimation.
Our study has several strengths. To our knowledge, it is the first study to evaluate the effect of statistical modeling on the predictive performance of non-invasive central BP estimates. Second, we used a robust methodology following the ARTERY guidelines to obtain invasive BP measurements (22). Third, non-invasive measurements were standardized by research personnel and taken simultaneously to the invasive aortic BP measurements. Furthermore, several clinical and hemodynamic parameters were used for model adjustment. Lastly, the effect of adjusted models was assessed using several statistical and graphical methods. However, some limitations are worth considering. Our results are based on the Mobil-O-Graph device and as such, may not be generalizable to other central BP devices. Also, as all patients required cardiac catheterization for a clinical indication, it remains to be determined if our findings are generalizable to patients without suspected cardiac disease, although a large-scale invasive BP study on other populations is not ethically feasible. The exclusion of participants with inter-arm SBP differences above 10 mmHg also limits the external validity of our results. Finally, external validation will be required before the models developed in this study could be adopted at a larger scale.
Using a large cohort of patients with simultaneously measured invasive and non-invasive BP, we showed that both brachial SBP and non-invasive central SBP have sex- and age-associated differences in accuracy. We identified easily accessible clinical factors associated with non-invasive SBP accuracy and showed that adjusting central SBP measurements with these parameters improves the accuracy and precision toward the true aortic SBP. More so, we succeeded in removing the inaccuracy bias inherent to advancing age and female sex. These findings pave the way to an enhancement of central BP measurements that could potentially minimize under- and overtreatment of hypertension. Integration of such parameters into the estimating algorithms of central BP may not only improve accuracy, but also constitutes a first step toward a personalized approach for hypertension diagnosis and treatment targets.
The raw data supporting the conclusions of this article will be made available by the authors, without undue reservation.
The studies involving human participants were reviewed and approved by the Comité d’Éthique de la Recherche du CIUSSS du Nord-de-l’île-de-Montréal. The patients/participants provided their written informed consent to participate in this study.
RG was responsible for the conception and design of the study, and oversaw all aspect of the study and manuscript. L-CD and NG performed the statistical analysis. NG wrote the first draft of the manuscript. L-CD wrote the second draft of the manuscript. CF, AB-J, and MA provided critical appraisal of the study conception, statistical analysis plan, and manuscript. All authors contributed to manuscript revision, read, and approved the submitted version.
RG held a research scholarship from the Fonds de recherche du Québec – Santé and was a recipient of the Société québécoise d’hypertension artérielle – Bourse Jacques-de-Champlain scholarship.
We are thankful to all the cardiologists, fellows and research staff who performed the invasive and non-invasive BP measurements for this study.
The authors declare that the research was conducted in the absence of any commercial or financial relationships that could be construed as a potential conflict of interest.
All claims expressed in this article are solely those of the authors and do not necessarily represent those of their affiliated organizations, or those of the publisher, the editors and the reviewers. Any product that may be evaluated in this article, or claim that may be made by its manufacturer, is not guaranteed or endorsed by the publisher.
The Supplementary Material for this article can be found online at: https://www.frontiersin.org/articles/10.3389/fcvm.2022.1048507/full#supplementary-material
1. Murray CJL, Aravkin AY, Zheng P, Abbafati C, Abbas KM, Abbasi-Kangevari M, et al. Global burden of 87 risk factors in 204 countries and territories, 1990–2019: a systematic analysis for the Global Burden of Disease Study 2019. Lancet. (2020) 396:1223–49. doi: 10.1016/s0140-6736(20)30752-2
2. Forouzanfar MH, Liu P, Roth GA, Ng M, Biryukov S, Marczak L, et al. Global burden of hypertension and systolic blood pressure of at least 110 to 115 Mm Hg, 1990-2015. JAMA. (2017) 317:165–82. doi: 10.1001/jama.2016.19043
3. Hughes D, Judge C, Murphy R, Loughlin E, Costello M, Whiteley W, et al. Association of blood pressure lowering with incident dementia or cognitive impairment: a systematic review and meta-analysis. JAMA. (2020) 323:1934–44. doi: 10.1001/jama.2020.4249
4. Kirkland EB, Heincelman M, Bishu KG, Schumann SO, Schreiner A, Axon RN, et al. Trends in healthcare expenditures among US adults with hypertension: National Estimates, 2003-2014. J Am Heart Assoc. (2018) 7:008731. doi: 10.1161/JAHA.118.008731
5. Picone DS, Schultz MG, Otahal P, Aakhus S, Al-Jumaily AM, Black JA, et al. Accuracy of cuff-measured blood pressure: systematic reviews and meta-analyses. J Am Coll Cardiol. (2017) 70:572–86. doi: 10.1016/j.jacc.2017.05.064
6. Chen CH, Nevo E, Fetics B, Pak PH, Yin FC, Maughan WL, et al. Estimation of central aortic pressure waveform by mathematical transformation of radial tonometry pressure. Validation of generalized transfer function. Circulation. (1997) 95:1827–36. doi: 10.1161/01.cir.95.7.1827
7. Weber T, Wassertheurer S, Rammer M, Maurer E, Hametner B, Mayer CC, et al. Validation of a brachial cuff-based method for estimating central systolic blood pressure. Hypertension. (2011) 58:825–32. doi: 10.1161/HYPERTENSIONAHA.111.176313
8. Weber T, Wassertheurer S, Schmidt-Trucksass A, Rodilla E, Ablasser C, Jankowski P, et al. Relationship between 24-hour ambulatory central systolic blood pressure and left ventricular mass: a prospective multicenter study. Hypertension. (2017) 70:1157–64. doi: 10.1161/HYPERTENSIONAHA.117.09917
9. Weber T, Protogerou AD, Agharazii M, Argyris A, Aoun Bahous S, Banegas JR, et al. Twenty-four-hour central (aortic) systolic blood pressure: reference values and dipping patterns in untreated individuals. Hypertension. (2022) 79:251–60. doi: 10.1161/HYPERTENSIONAHA.121.17765
10. Vlachopoulos C, Aznaouridis K, O’Rourke MF, Safar ME, Baou K, Stefanadis C. Prediction of cardiovascular events and all-cause mortality with central haemodynamics: a systematic review and meta-analysis. Eur Heart J. (2010) 31:1865–71.
11. Roman MJ, Devereux RB, Kizer JR, Okin PM, Lee ET, Wang W, et al. High central pulse pressure is independently associated with adverse cardiovascular outcome: the strong heart study. J Am Coll Cardiol. (2009) 54:1730–4.
12. Roman MJ, Devereux RB, Kizer JR, Lee ET, Galloway JM, Ali T, et al. Central pressure more strongly relates to vascular disease and outcome than does brachial pressure: the strong heart study. Hypertension. (2007) 50:197–203. doi: 10.1161/HYPERTENSIONAHA.107.089078
13. Pini R, Cavallini MC, Palmieri V, Marchionni N, Di Bari M, Devereux RB, et al. Central but not brachial blood pressure predicts cardiovascular events in an unselected geriatric population: the ICARe dicomano study. J Am Coll Cardiol. (2008) 51:2432–9.
14. Kollias A, Lagou S, Zeniodi ME, Boubouchairopoulou N, Stergiou GS. Association of central versus brachial blood pressure with target-organ damage: systematic review and meta-analysis. Hypertension. (2016) 67:183–90. doi: 10.1161/HYPERTENSIONAHA.115.06066
15. Dong Y, Jiang L, Wang X, Chen Z, Zhang L, Zhang Z, et al. Central rather than brachial pressures are stronger predictors of cardiovascular outcomes: a longitudinal prospective study in a Chinese population. J Clin Hypertens. (2020) 22:623–30. doi: 10.1111/jch.13838
16. Lamarche F, Agharazii M, Madore F, Goupil R. Prediction of cardiovascular events by type i central systolic blood pressure: a prospective study. Hypertension. (2021) 77:319–27. doi: 10.1161/HYPERTENSIONAHA.120.16163
17. Cheng HM, Lang D, Tufanaru C, Pearson A. Measurement accuracy of non-invasively obtained central blood pressure by applanation tonometry: a systematic review and meta-analysis. Int J Cardiol. (2013) 167:1867–76. doi: 10.1016/j.ijcard.2012.04.155
18. Gotzmann M, Hogeweg M, Seibert FS, Rohn BJ, Bergbauer M, Babel N, et al. Accuracy of fully automated oscillometric central aortic blood pressure measurement techniques. J Hypertens. (2020) 38:235–42. doi: 10.1097/HJH.0000000000002237
19. Gotzmann M, Hogeweg M, Bauer F, Seibert FS, Rohn BJ, Mugge A, et al. The impact of calibration approaches on the accuracy of oscillometric central aortic blood pressure measurement. J Hypertens. (2020) 38:2154–60. doi: 10.1097/HJH.0000000000002563
20. Kowalski C, Yang K, Charron T, Doucet M, Hatem R, Kouz R, et al. Inaccuracy of brachial blood pressure and its potential impact on treatment and aortic blood pressure estimation. J Hypertens. (2021) 39:2370–8. doi: 10.1097/HJH.0000000000002943
21. Abbaoui Y, Fortier C, Desbiens LC, Kowalski C, Lamarche F, Nadeau-Fredette AC, et al. Accuracy difference of noninvasive blood pressure measurements by sex and height. JAMA Netw Open. (2022) 5:e2215513. doi: 10.1001/jamanetworkopen.2022.15513
22. Sharman JE, Avolio AP, Baulmann J, Benetos A, Blacher J, Blizzard CL, et al. Validation of non-invasive central blood pressure devices: artery society task force consensus statement on protocol standardization. Eur Heart J. (2017) 38:2805–12. doi: 10.1093/eurheartj/ehw632
23. Jones CR, Taylor K, Chowienczyk P, Poston L, Shennan AH. A validation of the mobil o graph (version 12) ambulatory blood pressure monitor. Blood Press Monit. (2000) 5:233–8. doi: 10.1097/00126097-200008000-00007
24. Schultz MG, Picone DS, Armstrong MK, Black JA, Dwyer N, Roberts-Thomson P, et al. The influence of Sbp amplification on the accuracy of form-factor-derived mean arterial pressure. J Hypertens. (2020) 38:1033–9. doi: 10.1097/HJH.0000000000002385
25. Wilkinson IB, MacCallum H, Flint L, Cockcroft JR, Newby DE, Webb DJ. The influence of heart rate on augmentation index and central arterial pressure in humans. J Physiol. (2000) 525(Pt. 1):263–70. doi: 10.1111/j.1469-7793.2000.t01-1-00263.x
26. Lam CS, Xanthakis V, Sullivan LM, Lieb W, Aragam J, Redfield MM, et al. Aortic root remodeling over the adult life course: longitudinal data from the framingham heart study. Circulation. (2010) 122:884–90. doi: 10.1161/CIRCULATIONAHA.110.937839
27. Torjesen AA, Wang N, Larson MG, Hamburg NM, Vita JA, Levy D, et al. Forward and backward wave morphology and central pressure augmentation in men and women in the framingham heart study. Hypertension. (2014) 64:259–65. doi: 10.1161/HYPERTENSIONAHA.114.03371
28. McEniery CM, Yasmin, Hall IR, Qasem A, Wilkinson IB, Cockcroft JR, et al. Normal vascular aging: differential effects on wave reflection and aortic pulse wave velocity: the Anglo-Cardiff Collaborative Trial (ACCT). J Am Coll Cardiol. (2005) 46:1753–60. doi: 10.1016/j.jacc.2005.07.037
29. Kelly R, Hayward C, Avolio A, O’Rourke M. Noninvasive determination of age-related changes in the human arterial pulse. Circulation. (1989) 80:1652–9. doi: 10.1161/01.cir.80.6.1652
30. Esposito C, Machado P, Cohen IS, Mehrotra P, Savage M, Fischman D, et al. Comparing central aortic pressures obtained using a sphygmocor device to pressures obtained using a pressure catheter. Am J Hypertens. (2022) 35:397–406. doi: 10.1093/ajh/hpac010
31. Roush GC, Fagard RH, Salles GF, Pierdomenico SD, Reboldi G, Verdecchia P, et al. Prognostic impact of sex-ambulatory blood pressure interactions in 10 cohorts of 17 312 patients diagnosed with hypertension: systematic review and meta-analysis. J Hypertens. (2015) 33:212–20. doi: 10.1097/HJH.0000000000000435
32. Wei YC, George NI, Chang CW, Hicks KA. Assessing sex differences in the risk of cardiovascular disease and mortality per increment in systolic blood pressure: a systematic review and meta-analysis of follow-up studies in the United States. PLoS One. (2017) 12:e0170218. doi: 10.1371/journal.pone.0170218
33. Dickerson JA, Nagaraja HN, Raman SV. Gender-related differences in coronary artery dimensions: a volumetric analysis. Clin Cardiol. (2010) 33:E44–9. doi: 10.1002/clc.20509
Keywords: central blood pressure, aortic blood pressure, invasive blood pressure, accuracy, brachial cuff-based, oscillometric devices for measurement of central BP, statistical modeling
Citation: Desbiens L-C, Goulamhoussen N, Fortier C, Bernier-Jean A, Agharazii M and Goupil R (2022) Enhancing central blood pressure accuracy through statistical modeling: A proof-of-concept study. Front. Cardiovasc. Med. 9:1048507. doi: 10.3389/fcvm.2022.1048507
Received: 19 September 2022; Accepted: 28 October 2022;
Published: 23 November 2022.
Edited by:
Tlili Barhoumi, King Abdullah International Medical Research Center (KAIMRC), Saudi ArabiaReviewed by:
Alessandro Maloberti, University of Milano-Bicocca, ItalyCopyright © 2022 Desbiens, Goulamhoussen, Fortier, Bernier-Jean, Agharazii and Goupil. This is an open-access article distributed under the terms of the Creative Commons Attribution License (CC BY). The use, distribution or reproduction in other forums is permitted, provided the original author(s) and the copyright owner(s) are credited and that the original publication in this journal is cited, in accordance with accepted academic practice. No use, distribution or reproduction is permitted which does not comply with these terms.
*Correspondence: Rémi Goupil, cmVtaS5nb3VwaWxAdW1vbnRyZWFsLmNh
†These authors share first authorship
Disclaimer: All claims expressed in this article are solely those of the authors and do not necessarily represent those of their affiliated organizations, or those of the publisher, the editors and the reviewers. Any product that may be evaluated in this article or claim that may be made by its manufacturer is not guaranteed or endorsed by the publisher.
Research integrity at Frontiers
Learn more about the work of our research integrity team to safeguard the quality of each article we publish.