- 1School of Traditional Chinese and Western Medicine, Gansu University of Chinese Medicine, Lanzhou, China
- 2Key Laboratory of Prevention and Treatment for Chronic Diseases by Traditional Chinese Medicine, University Hospital of Gansu Traditional Chinese Medicine, Lanzhou, China
- 3Affiliated Hospital of Gansu University of Chinese Medicine, Lanzhou, China
- 4The First Hospital of Lanzhou University, Lanzhou, China
- 5Lanzhou University Second Hospital, Lanzhou, China
Cardiotoxicity is a serious complication of cancer therapy. It is the second leading cause of morbidity and mortality in cancer survivors and is associated with a variety of factors, including oxidative stress, inflammation, apoptosis, autophagy, endoplasmic reticulum stress, and abnormal myocardial energy metabolism. A number of studies have shown that traditional Chinese medicine (TCM) can mitigate chemoradiotherapy-associated cardiotoxicity via these pathways. Therefore, this study reviews the effects and molecular mechanisms of TCM on chemoradiotherapy-related cardiotoxicity. In this study, we searched PubMed for basic studies on the anti-cardiotoxicity of TCM in the past 5 years and summarized their results. Angelica Sinensis, Astragalus membranaceus Bunge, Danshinone IIA sulfonate sodium (STS), Astragaloside (AS), Resveratrol, Ginsenoside, Quercetin, Danggui Buxue Decoction (DBD), Shengxian decoction (SXT), Compound Danshen Dripping Pill (CDDP), Qishen Huanwu Capsule (QSHWC), Angelica Sinensis and Astragalus membranaceus Bunge Ultrafiltration Extract (AS-AM),Shenmai injection (SMI), Xinmailong (XML), and nearly 60 other herbs, herbal monomers, herbal soups and herbal compound preparations were found to be effective as complementary or alternative treatments. These preparations reduced chemoradiotherapy-induced cardiotoxicity through various pathways such as anti-oxidative stress, anti-inflammation, alleviating endoplasmic reticulum stress, regulation of apoptosis and autophagy, and improvement of myocardial energy metabolism. However, few clinical trials have been conducted on these therapies, and these trials can provide stronger evidence-based support for TCM.
Introduction
Chemoradiotherapy has improved survival in patients with cancer; however, the resulting cardiotoxicity is a major cause of morbidity and mortality in the oncology population (1–3). In the United States, women have a significantly increased risk of death from cardiotoxicity, which exceeds the risk of death from cancer or recurrence, and it is the leading cause of death in patients over 50 years old with breast cancer (4). The cumulative incidence of chronic heart failure (CHF) 10 years after systemic therapy in Dutch patients with early-stage breast cancer was 4.8% (5). Anthracycline use in first-line lymphoma treatment is associated with a significantly increased incidence of CHF in Danish patients with lymphoma (6). The incidence of childhood cancer cardiotoxicity after anthracycline therapy in a multi-ethnic Asian population was 7%, of which 37.5% had CHF (7). Radiotherapy also causes cardiotoxicity, which has a 4–16% relative risk of heart disease and major cardiac events per Gray of the average cardiac radiation dose (8). Radiotherapy induces oxidative stress (OS) and matrix remodeling, which alters the cardiac microvascular and macrovascular environment and induces coronary artery disease, myocardial fibrosis, and cardiomyopathy, valvular disease, pericardial disease, and arrhythmias (9, 10); chemotherapies can cause cardiotoxicity through OS, lipid peroxidation, and inhibition of topoisomerase IIβ (Top2β), leading to cardiomyocytes (CMs) damage (11).
Cardiac oncology clinical practice guidelines define cardiotoxicity as (1) a relative decrease in overall longitudinal echocardiographic strain of >15% or a new increase in cardiac biomarkers in individuals with left ventricular ejection fraction (LVEF) ≥50%, (2) a decrease in LVEF to 40–49% (accompanied by a relative decrease in overall longitudinal echocardiographic strain of >15% or a decrease in LVEF of <10% and a new increase in new cardiac biomarkers), or (3) a decrease in LVEF to <40% (12). However, chemoradiotherapy not only affects resting LVEF but also has a wide range of effects on the entire cardiovascular system, including direct effects on cardiac structure (e.g., fibrosis), diastolic function, cardiac conduction and arrhythmias, systemic and pulmonary vascular function and hemodynamics, hemostasis and thrombosis, and cardiac response to injury and stress (13). Statins, angiotensin-converting enzyme inhibitors, angiotensin receptor blockers, beta-blockers, and dexrazoxane are currently used clinically to prevent or reduce radiotherapy-related cardiotoxicity (14–21). However, these drugs do not significantly reduce the risk of cardiotoxicity; instead, they interfere with the antitumor properties and prognostic benefits of anthracyclines, increasing the incidence of secondary malignancies (11, 14, 18). Therefore, there is an urgent need to explore safer and more effective options.
TCM has been used in China for thousands of years to treat human diseases and has attracted widespread attention from other countries owing to its unique healing properties (22). In recent years, TCM has made contributions to global public health, such as artemisinin for treating malaria (23), arsenic trioxide for acute promyelocytic leukemia (24, 25), and played an important role in treating pneumonia associated with novel coronavirus disease 2019 (COVID-19) (26–30). In 2019, the World Health Organization included TCM as an accepted form of treatment in its International Statistical Classification of Diseases (ICD-11) for the first time in the 72nd World Health Assembly, reflecting the contribution of TCM to global healthcare (31). Cancer and cardiovascular diseases are two major maladies that pose a serious threat to human health, and cardiovascular toxicity caused by these cancer treatments poses a serious and specific threat to the health and survival of patients with cancer. Recent studies have shown that TCM can combat chemoradiotherapy-related cardiotoxicity without affecting the antitumor activity of the treatment (32). However, the mechanism of action of TCM for the treatment of chemoradiotherapy-related cardiotoxicity is not fully understood. Therefore, we summarize recent studies on the prevention and treatment of chemoradiotherapy-related cardiotoxicity by TCM and explain its mechanism to provide a basis for the prevention and treatment of chemoradiotherapy-related cardiotoxicity by TCM.
Molecular mechanisms of chemoradiotherapy-related cardiotoxicity and the therapeutic effects of traditional Chinese medicine
The pathogenesis of chemoradiotherapy cardiotoxicity is associated with multiple molecular pathways, with OS and inflammation being the most important pathways, along with apoptosis, autophagy, endoplasmic reticulum stress, and abnormal myocardial energy metabolism (Table 1, Figure 1).
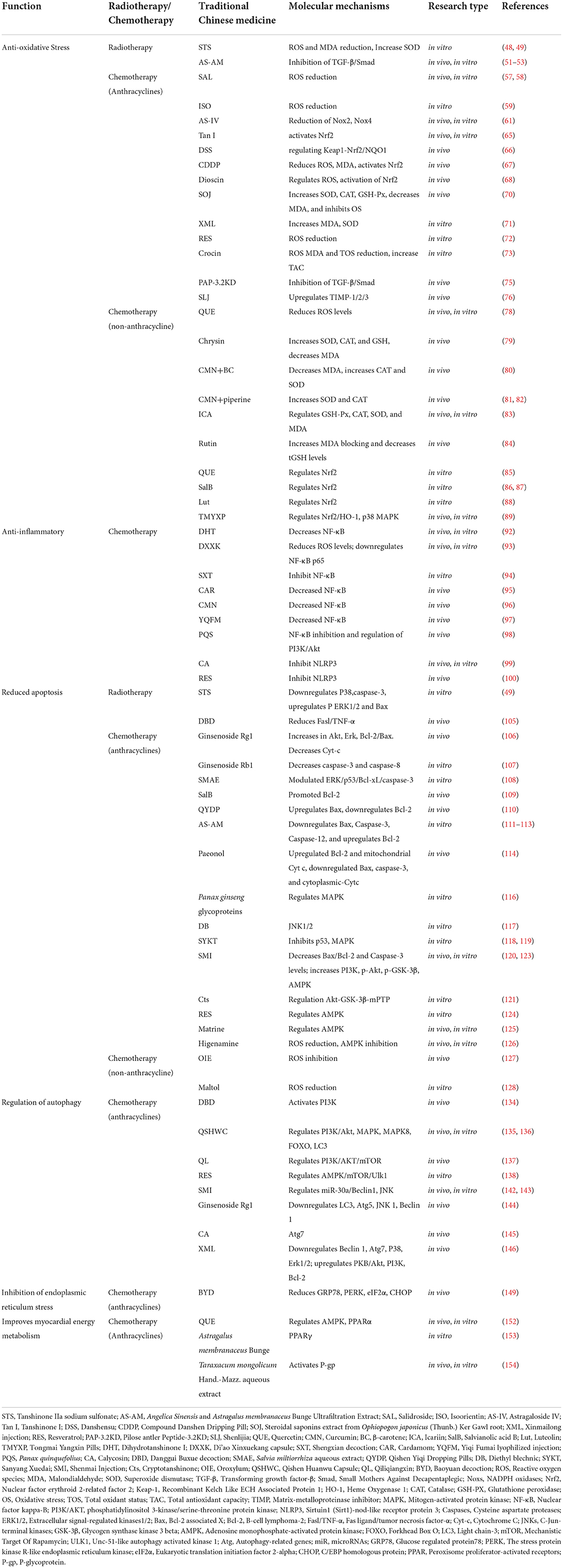
Table 1. Protective effects and mechanisms of traditional Chinese medicine against chemoradiotherapy-related cardiotoxicity.
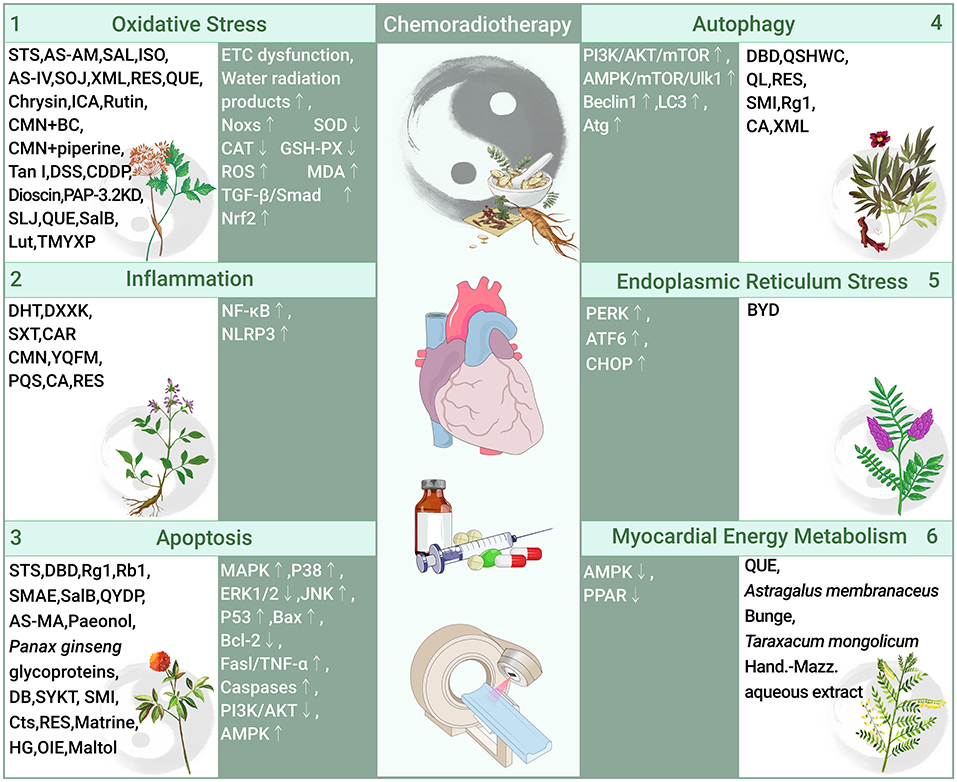
Figure 1. Role and molecular mechanism of traditional Chinese medicine in preventing cardiotoxicity associated with chemoradiotherapy (traditional Chinese medicine alleviates chemoradiotherapy-related cardiotoxicity by inhibiting oxidative stress, anti-inflammatory, regulating apoptosis and autophagy, inhibition of endoplasmic reticulum stress, and improves myocardial energy metabolism. OS, Oxidative stress; Noxs, NADPH oxidases; SOD, Superoxide dismutase; CAT, Catalase; GSH-PX, Glutathione; ROS, Reactive oxygen species; MDA, Malondialdehyde; TGF-β, Transforming growth factor-β; Smad, Small Mothers Against Decapentaplegic; Nrf2, Nuclear factor erythroid 2-related factor 2; STS, Tanshinone IIa sodium sulfonate; AS-AM, Angelica Sinensis and Astragalus membranaceus Bunge Ultrafiltration Extract; SAL, Salidroside; ISO, Isoorientin; AS-IV, Astragaloside IV; SOJ, Steroidal saponins extract from Ophiopogon japonicus (SOJ) root; XML, Xinmailong injection; RES, Resveratrol; QUE, Quercetin; ICA, Icariin; CMN, Curcumin; BC, β-carotene; Tan I, Tanshinone I; DSS, Danshensu; CDDP, Compound Danshen Dripping Pill; PAP-3.2KD, Pilose antler Peptide-3.2KD; SLJ, Shenlijia; SalB, Salvianolic acid B; Lut, Luteolin; TMYXP, Tongmai Yangxin Pills; NF-κB, Nuclear factor kappa-B; NLRP3, Sirtuin1 (Sirt1)-nod-like receptor protein 3; DHT, Dihydrotanshinone I; DXXK, Di'ao Xinxuekang capsule; SXT, Shengxian decoction; CAR, Cardamom; YQFM, Yiqi Fumai lyophilized injection; PQS, Panax quinquefolius; CA, Calycosin; RES, Resveratrol; MAPK, Mitogen-activated protein kinase; ERK, Extracellular signal-regulated kinases; JNK, C-Jun-terminal kinases; Bax, Bcl-2 associated X; Bcl-2, B-cell lymphoma-2; Fasl/TNF-α, Fas ligand/tumor necrosis factor-α; Caspases, Cysteine aspartate proteases; PI3K/AKT, phosphatidylinositol 3-kinase/serine-threonine protein kinase; AMPK, adenosine monophosphate-activated protein kinase; DBD, Danggui Buxue decoction; Rg1, ginsenoside Rg1; Rb1, ginsenoside Rb1; SMAE, Salvia miltiorrhiza aqueous extract; QYDP, Qishen Yiqi Dropping Pills; DB, Diethyl blechnic; SYKT, Sanyang Xuedai; SMI, Shenmai Injection; Cts, Cryptotanshinone; HG, Higenamine; OIE, Oroxylum; mTOR, Mechanistic Target Of Rapamycin; LC3, Light chain-3; Atg, Autophagy-related genes; QSHWC, Qishen Huanwu Capsule; QL, Qiliqiangxin; PERK, The stress protein kinase R-like endoplasmic reticulum kinase; ATF6, Activating transcription factor 6; CHOP:C/EBP homologous protein; BYD, Baoyuan decoction; PPAR, peroxisome proliferator-activated receptors).
Oxidative stress
OS refers to the imbalance of pro-oxidants and antioxidants and the disruption of redox signaling and control (33). The mitochondrial respiratory chain and nicotinamide adenine dinucleotide phosphate (NADPH) are the main cellular sources of reactive oxygen species (ROS) (34). NADPH oxidases (Noxs) are a group of plasma membrane-associated enzymes that are among the most important sources of ROS, Nox2 and Nox4 are the major cardiac isoforms (35), overexpression of Nox2 and Nox4 induces the production of ROS (36). Nuclear factor erythroid 2-related factor 2 (Nrf2) controls gene expression of endogenous antioxidant synthesis and ROS-eliminating enzymes in response to various electrophilic compounds, inactivates the negative regulator Kelch-like ECH-associated protein 1 (Keap1), and activates Nrf2 by overexpression of mitochondrial ROS (mtROS) and Nox2 and Nox4 (37). The role of antioxidant enzyme systems [superoxide dismutases (SODs), catalases (CAT), glutathione peroxidases (GPxs), and paraoxonases (PONs)] is to scavenge ROS (38), and oxidative damage occurs when ROS production exceeds the buffering capacity of ROS scavengers or when the antioxidant defense system is defective (39). Increased ROS also caused the development of myocardial fibrosis (MF) (40). Transforming growth factor-β (TGF-β) is a key factor in MF, and ROS is an immediate activator of TGF-β1.
Radiotherapy-induced oxidative stress
Oxidative stress and ROS may be the main cause of ionizing radiation (IR)-induced cardiotoxicity (41). IR leads to mitochondrial electron transport chain (ETC) dysfunction and ROS overproduction, causing DNA damage and protein and lipid peroxidation, the latter of which leads to the production of malondialdehyde (MDA) (39, 41–43); IR activates Noxs and inhibits SOD expression for ROS production and accumulation (44). Tissues and cells, which are 80% water, rapidly undergo OS responses after being targeted by ionizing radiation (45), forming water radiolysis products rich in ROS and releasing ROS (46). The late effects of radiotherapy are due to IR and IR-induced production of chronic free radicals from water molecules in the surrounding environment (47). STS significantly inhibits the increase of ROS and MDA content in H9C2 cells and cardiac fibroblasts (CFS) under X-ray radiation and increased the level of SOD (48, 49).
Myocardial fibrosis is a late manifestation of radiation-induced heart disease (RIHD) (50). The current studies showed that AS-AM, a DBD-derivative, downregulated TGF-β/Smad and COL-I expression in an X-ray-induced rat CFs fibrotic injury model (51–53) (Figure 2).
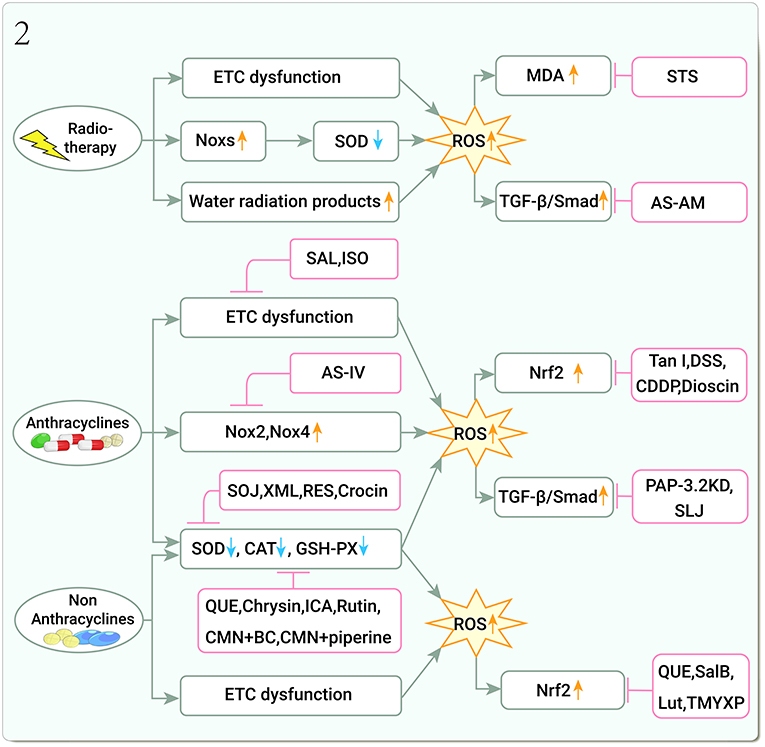
Figure 2. Traditional Chinese medicine alleviates cardiotoxicity associated with chemoradiotherapy by inhibiting oxidative stress (Noxs, NADPH oxidases; SOD, Superoxide dismutase; CAT, Catalase; GSH-PX, Glutathione peroxidase; ROS, Reactive oxygen species; MDA, Malondialdehyde; TGF-β, Transforming growth factor-β; Smad, Small Mothers Against Decapentaplegic; Nrf2, Nuclear factor erythroid 2-related factor 2; STS, Tanshinone IIa sodium sulfonate; AS-AM, Angelica Sinensis and Astragalus membranaceus Bunge Ultrafiltration Extract; SAL, Salidroside; ISO, Isoorientin; AS-IV, Astragaloside IV; SOJ, Steroidal saponins extract from Ophiopogon japonicus (SOJ) root; XML, Xinmailong injection; RES, Resveratrol; QUE, Quercetin; ICA, Icariin; CMN, Curcumin; BC, β-carotene; Tan I, Tanshinone I; DSS, Danshensu; CDDP, Compound Danshen Dripping Pill; PAP-3.2KD, Pilose antler Peptide-3.2KD; SLJ, Shenlijia; SalB, Salvianolic acid B; Lut, Luteolin; TMYXP, Tongmai Yangxin Pills).
Oxidative stress induced by anthracycline-based chemotherapeutic agents
Doxorubicin (DOX)-induced OS is thought to be a major cause of cardiotoxicity (54, 55). DOX alters myocardial ETC gene expression and translation in vivo, reducing the redox cycle of the ETC complex I, and generates large amounts of ROS (56). Disrupting this process, the salidroside (SAL) attenuates DOX-induced cardiac insufficiency by reducing ROS production and improving mitochondrial function (57, 58). Another TCM, isoorientin (3′,4′,5,7-tetrahydroxy-6-C-glucopyranosyl flavone) is a natural C-glycosyl flavonoid with strong free radical scavenging activity that reduces ROS, maintains mitochondrial function, and attenuates DOX-induced H9C2 CMs damage (59).
DOX induces Noxs activation, which leads to increased ROS production (60). The compound AS-IV attenuates DOX-induced Nox2 and Nox4 expression, OS, and cardiomyopathy in CMs (61). Nrf2 deficiency exacerbates DOX-induced cardiotoxicity and cardiac insufficiency (62–64). Playing a role in these pathways, Tanshinone I (Tan I) upregulated key proteins in the Nrf2 pathway to improve cardiac function and protect against both in vivo and in vitro DOX-induced myocardial structural damage in mice (65). Danshensu (DSS) effectively exerted anti-oxidative stress, anti-inflammatory, and anti-apoptotic therapeutic effects against DOX-induced cardiotoxicity by regulating the expression of Keap1-Nrf2/NQO1 (66). The CDDP activates Nrf2 expression to reduce the levels of ROS, MDA, and cardiac damage in mice (67). Dioscin, an extract from the rhizome of Dioscorea punctata, also inhibits myocardial oxidative damage by activating the Nrf2 pathway, lowers Keap1 expression, and attenuates cardiotoxicity (68).
DOX significantly reduces antioxidant enzyme levels, leading to redox imbalances and increased OS, but these effects can be treated with TCMs (69). Steroidal saponins extract from Ophiopogon japonicus (Thunb.) Ker Gawl root (SOJ) increased SOD, CAT, and GSH-Px activities and decreased MDA in rat myocardial tissue by inhibiting OS (70). In H9C2 cells, XML decrease DOX-induced MDA content, enhance SOD activity, increase ROS scavenging, and attenuate cardiotoxicity (71); RES reduced DOX-induced ROS content and improved cell survival, the effect of RES against DOX cardiotoxicity was comparable to that of dexrazoxane and carvedilol (72); additionally, crocin can reduce ROS, MDA and total oxidant status (TOS) levels, increase total antioxidant capacity (TAC), mitigation of DNA damage (73).
DOX both activated the TGF-β and P-Smad3 signaling pathways and enhanced collagen deposition in CMs (74), inducing MF development. Pilose antler peptide-3.2KD (PAP-3.2KD) has multiple biological activities in cardiomyopathy and reverses histological changes in cardiac tissue by decreasing TGF-β1, Smad2/3/4, and P-Smad2/3 levels, elevating Smad7 protein levels, thereby regulating pathological changes in the TGF-β/Smad signaling pathway, such as myofascial disorders, MF, and diffuse CMs edema (75). Shenlijia (SLJ) can improve cardiac function and inhibit MF progression. It improves cardiac function and ultrastructure, and inhibits MF development in DOX-induced CHF rats by upregulating extracellular matrix-metalloproteinase inhibitor (TIMP) expression (76) (Figure 2).
Oxidative stress induced by non-anthracycline chemotherapeutic agents
Aside from DOX, non-anthracycline chemotherapeutic agents, such as cyclophosphamide (CyC) and cisplatin (CP), cause cardiotoxicity by inducing mitochondrial dysfunction, leading to ETC damage, oxidative phosphorylation, decreased antioxidant enzyme levels and antioxidant capacity, increased ROS, activate Nrf2 and induce apoptosis (77).
CyC, an alkylating agent, combined with DOX is the most commonly used regimen for triple-negative breast cancer chemotherapy. CyC cardiotoxicity is caused by OS. Quercetin (QUE) has strong antioxidant activity and attenuates CyC-induced cardiotoxicity by inhibiting ROS accumulation in CMs; interestingly, it also enhances the antitumor activity of CyC (78). Another TCM that combats CyC-effects, chrysin increases enzyme levels that combat ROS and decreases levels of lipid peroxidation products in CyC-injured hearts, all of which protect against cardiotoxicity (79).
CP is another alkylating agent. Curcumin (CMN) has strong antioxidant effects, and when combined with β-carotene (BC), reduces lipid peroxidase product and increases anti-OS enzyme activities in CP-treated rat heart tissue (80). An additional CMN combination with piperine significantly increases anti-OS enzyme levels in the cardiac tissue of CP-treated rats (81, 82). Icariin (ICA) and rutin, attenuated CP-induced myocardial injury by increasing anti-OS enzyme and decreasing lipid peroxidase product levels (83, 84). QUE, salvianolic acid B (SalB), and luteolin (Lut) significantly reduced CP-induced OS by regulating Nrft2 signaling pathways (85–88). The 11-herbs combination treatment, Tongmai Yangxin Pills (TMYXP), nourish Qi and Yin, promoting blood circulation, relieving pain, and can improve the anti-OS ability of CP chemotherapy CMs by regulating Nrf2/HO-1 pathway and p38 MAPK pathway (89) (Figure 2).
OS is an important cause of disease, and this is no exception in cardiotoxicity due to chemoradiotherapy. Radiotherapy leads to increased ROS and MDA levels, decreased SOD levels, and upregulated TGF-β1 expression leading to MF, and STS and AS-AM can reverse these damages; chemotherapy leads to increased endogenous ROS production and decreased antioxidant enzyme expression, and the above-mentioned chemotherapy drugs counteract DOX-induced cardiotoxicity by reducing ROS production, inhibiting Nox2 and Nox4 overexpression, regulating Nrf2 function, and the above TCM counteracted DOX-induced cardiotoxicity by reducing ROS production, inhibiting Nox2 and Nox4 overexpression, regulating Nrf2 function, and increasing antioxidant enzyme content. However, there are few studies on the mechanism of radiotherapy-related cardiotoxicity in TCM, and there is a lack of studies on important factors and pathways such as Noxs and Nrf2. In the studies on chemotherapy-related cardiotoxicity, there are no studies on Top2β, which is a key factor leading to cardiotoxicity. In the future, more and more in-depth studies on key targets are needed.
Inflammation
Inflammation is another important factor in chemotherapy drug-related cardiotoxicity and is related to OS. Nuclear factor kappa-B (NF-κB) is a key transcription factor in the inflammatory response, sirtuin1 (Sirt1)-nod-like receptor protein 3 (NLRP3) inflammatory vesicles are protein complexes that activate the secretion of the pro-inflammatory cytokine interleukin (IL)-1β in a cysteine aspartate proteases (caspases)-1-dependent manner and are involved in inflammatory regulation. DOX activates NF-κB and NLRP3 inflammatory vesicles, causing cardiotoxicity (90, 91).
Dihydrotanshinone I (DHT) upregulates transcription factor EB (TFEB) nuclear expression and decreases p-IKKα/β and p-NF-κB expression, and is used for anti-inflammatory management of DOX induced cardiotoxicity via the mammalian target of rapamycin (mTOR)-TFEB-NF-κB signaling pathway (92). The main component of Di'ao Xinxuekang capsule (DXXK) is diosgenin, protects against cardiotoxicity by reducing ROS and downregulating NF-κB p65 (93). Shengxian decoction (SXT) inhibits NF-κB activity, thus preventing cardiotoxicity from DOX treatment (94). Cardamom (CAR) decreased cardiac NF-κB levels ameliorating DOX-induced cardiotoxicity in rats (95). In DOX-induced cardiotoxicity in rats, CMN showed anti-inflammatory potential by reducing IFN-γ levels and immune expression of iNOS, NF-κB, and tumor necrosis factor-α(TNF-α) (96). Yiqi Fumai lyophilized injection (YQFM) pretreatment of DOX-intoxicated rats significantly inhibited the expression of NF-κB, TNF-α, and cyclooxygenase-2 (97). Saponins from the leaves of Panax quinquefolius (PQS) inhibit NF-κB activity and disrupt the phosphatidylinositol 3 kinase (PI3K) /protein kinaseB(AKT) (PI3K/Akt)apoptotic pathway, thus preventing cardiotoxicity from CP treatment (98).
In DOX-treated cells and mouse hearts, levels of NLRP3 and related proteins were elevated, and calycosin (CA) ameliorated cardiotoxicity via the NLRP3 pathway (99). Resveratrol (RES) inhibition of NLRP3 inflammatory vesicle activation significantly reduced systemic inflammation and contributed to the improvement of DOX-induced myocardial injury and late-onset hypertension-induced cardiomyopathy in young mice (100) (Figure 3).
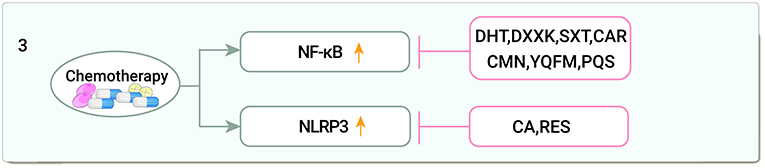
Figure 3. Traditional Chinese medicine alleviates cardiotoxicity associated with chemoradiotherapy by anti-inflammatory (NF-κB, Nuclear factor kappa-B; NLRP3, Sirtuin1 (Sirt1)-nod-like receptor protein 3; DHT, Dihydrotanshinone I; DXXK, Di'ao Xinxuekang capsule; SXT, Shengxian decoction; CAR, Cardamom; CMN, Curcumin; YQFM, Yiqi Fumai lyophilized injection; PQS, Panax quinquefolius; CA, Calycosin; RES, Resveratrol).
Among the inflammatory mechanisms, TCM for cardiotoxicity involves only two pathways, NF-κB and NLRP3, but other inflammatory pathways such as STAT1 and STAT3 also play important roles in the development of cardiotoxicity and should be of interest to investigators.
Apoptosis
Radiotherapy-induced apoptosis
The mitogen-activated protein kinase (MAPK) pathway is a common signaling pathway that transmits extracellular signals to downstream effector molecules and is involved in physiological processes such as cell proliferation, differentiation, and apoptosis (101), which consists of three branches: MAPK, extracellular signal-regulated kinases (ERKs) and c-Jun-terminal kinases (JNKs) (102). The p53 pathway is another critical pro-apoptotic pathway. P53 upregulates the pro-apoptosis B-cell lymphoma-2(Bcl-2) associated X(Bax) protein, downregulates the anti-apoptosis protein Bcl-2, and activates the transcription of Fas and other death receptor genes (103). Additionally, IR-induced DNA damage initiates apoptosis through a p53-dependent mechanism that activates downstream caspases (104). STS can disrupt the p53 pathway in CFs by decreasing the phosphorylation levels of p38, caspases 3 expression, and increasing the levels of Bax and phosphorylated ERK1/2 (49). DBD can reduce Fas ligand (Fasl) and TNF-α expression, block apoptotic signaling pathways, and attenuate radiological myocardial injury in CMs (105) (Figure 4).
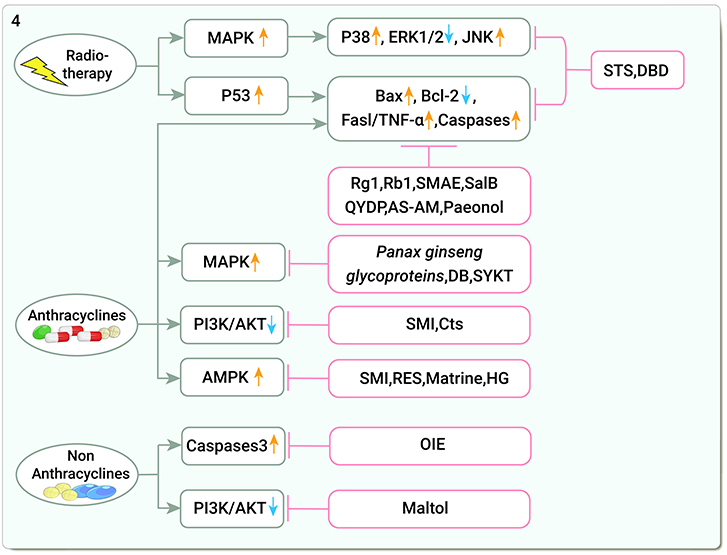
Figure 4. Traditional Chinese medicine alleviates cardiotoxicity associated with chemoradiotherapy by reducing apoptosis (MAPK, Mitogen-activated protein kinase; ERK1/2, Extracellular signal-regulated kinases1/2; JNKs, C-Jun-terminal kinases; Bax, Bcl-2 associated X; Bcl-2, B-cell lymphoma-2; Fasl/TNF-α, FasLigand/tumor necrosis factor-α; Caspases, Cysteine aspartate proteases; PI3K/AKT, Phosphatidylinositol 3-kinase/serine-threonine protein kinase; AMPK, Adenosine monophosphate-activated protein kinase; STS, Tanshinone IIa sodium sulfonate; DBD, Danggui Buxue decoction; Rg1, ginsenoside Rg1; Rb1, ginsenoside Rb1; SMAE, Salvia miltiorrhiza aqueous extract; SalB, Salvianolic acid B; QYDP, Qishen Yiqi Dropping Pills; AS-AM, Angelica Sinensis and Astragalus membranaceus Bunge Ultrafiltration Extract; DB, Diethyl blechnic; SYKT, Sanyang Xuedai; SMI, Shenmai Injection; Cts, Cryptotanshinone; RES, Resveratrol; HG, Higenamine; OIE, Oroxylum).
Anthracycline-based chemotherapy-induced apoptosis
DOX activates endogenous pathways and exogenous pathways of apoptosis (60). DOX downregulates the Akt pathway, induces caspases activity, and upregulates cell death receptors, all leading to CMs apoptosis (74). Blocking that impact, ginsenoside Rg1 increased Akt and ERK pathway phosphorylation, the ratio of Bcl-2 and Bax, and reduced Cytochrome C (Cyt-c) release from the mitochondria, thus disrupting DOX-induced CMs apoptosis (106). Additionally, ginsenoside Rb1 decreased caspase-3 and caspase-8 activity and blocked apoptosis in H9C2 cells (107). Salvia miltiorrhiza aqueous extract (SMAE) modulated ERK/p53/Bcl-xL/caspase-3 signaling pathway and improved mitochondrial dysfunction, significantly alleviating DOX-induced cardiomyopathy and apoptosis, and simultaneous administration of DOX and SMAE significantly inhibited the growth of breast cancer cells (108). SalB promoted Bcl-2 expression and attenuated DOX-induced apoptotic damage in cardiac tissue (109). Qishen Yiqi Dropping Pills (QYDP) increased vascular endothelial growth factor levels, myocardial microvascular density, and Bax expression, while it downregulated Bcl-2 and caspase 3 and attenuated MF in DOX-treated mice (110). AS-AM downregulated Bax, caspase 3, and caspase 12 and upregulated Bcl-2 expression. It also decreased apoptosis by inhibiting the intrinsic apoptotic pathway (111–113). Paeonol increased the viability and mitochondrial membrane potential (MMP) of DOX-induced CMs, upregulated the expression of Bcl-2 and mitochondrial Cyt c, downregulated the expression of Bax, caspase-3, and cytoplasmic-Cytc, and reduced apoptosis and ROS (114).
Members of the MAPK superfamily and PI3K are specifically involved in the induction of apoptosis and impairment of contractile function (102, 115). Panax ginseng glycoprotein protected against myocardial injury by inhibiting CMs apoptosis by upregulating the MAPK pathway (116). Diethyl blechnic (DB) activated the JNK1/2 pathway to protect CMs from cytotoxicity (117). Sanyang Xuedai (SYKT) has antioxidant properties and attenuates cardiotoxicity by inhibiting p53 and MAPK-induced apoptosis (118, 119). In a network pharmacology study, SMI increased PI3KCA and AKT1 expression, thus preventing CMs apoptosis (120). SMI reduced DOX-induced Bax/Bcl-2 and Caspase-3 levels and increased PI3K, p-Akt, and phosphorylated glycogen synthase kinase 3 beta (p-GSK-3β) levels in C57BL/6 mice. Similarly, cryptotanshinone (Cts) attenuated apoptosis via the Akt-GSK-3β-mPTP pathway (121).
AMP-activated protein kinase (AMPK) is at the center of DOX-induced cardiotoxicity. DOX has an inhibitory effect on cardiac AMPK, which increases cardiotoxicity (122). SMI increased AMPK phosphorylation levels, preventing DOX-induced excessive mitochondrial ROS generation, decreasing mitochondrial membrane potential, and reducing DOX-injured H9C2 cells from apoptosis (123). RES and matrine attenuated CMs apoptosis via the AMPK pathway (124, 125). Higenamine, the main active component of the TCM Wu-Tou, also attenuates DOX-induced cardiac remodeling and myocyte apoptosis by suppressing AMPK activation (126) (Figure 4).
Non-anthracycline-based chemotherapy-induced apoptosis
Oroxylum indicum extract (OIE) significantly reduces caspase-3 and protease activity in the hearts of DOX- and CP-treated C57BL/6 J mice (127). Maltol (produced by heating Panax ginseng) enhanced PI3K/Akt expression levels and reduced CP-induced apoptosis in H9C2 cardiomyocytes during cisplatin treatment (128) (Figure 4).
Radiotherapy induced the activation of MAPK and P53-dependent apoptotic pathways, and STS and DBD inhibited apoptosis through P53 and Fas/TNF-α pathways; studies on the inhibition of chemotherapy-induced cardiomyocyte apoptosis by TCM mainly focused on apoptosis-related genes such as Bax, Bcl-2, and MAPK and AMPK pathways. Other apoptotic pathways, such as the mitochondrial apoptosis pathway, have not been as thoroughly investigated, which may suggest a direction for future research.
Autophagy
Autophagy is a major regulator of homeostasis and heart function (129, 130). DOX regulates upstream regulatory processes of autophagy, such as mTOR and AMPK, and PI3K is also hyperactivated in a rat model of DOX cardiotoxicity (131). PI3K CI activates AKT, and activated AKT1 further activates mTORCl; mTORC2 is a bidirectional regulator of autophagy. mTORC2 indirectly inhibits autophagy through the AKT1/FOXO3a axis, and activated AKT1 leads to translocation of FOXO3 from the nucleus, thereby inhibiting autophagy-associated genes microtubule-associated protein light chain-3 (LC3) transcription (132). Activated AMPK directly promotes autophagy by phosphorylating mTORC1, ULK1, and autophagy-associated proteins in the PIK3C3/VPS34 complex (133).
TCMs can disrupt the ability of DOX to induce autophagy. DBD activated the PI3K pathway to inhibit CM autophagy in mice (134). QSHWC consists of 19 TCMs. Network pharmacological studies revealed that QSHWC contains 35 major active ingredients that can reduce the cardiotoxicity of anthracyclines by regulating PI3K/Akt, MAPK, FOXO, and other signaling pathways to regulate cellular autophagy and reduce the cardiotoxicity of anthracyclines (135), QSHWC downregulated pirarubicin-induced LC3, and played a cardioprotective role by inducing PI3K, AKT, and mTOR phosphorylation and pathway activation (136). Qiliqiangxin (QL), a compound used in TCM, protected against cardiotoxicity by deactivating the PI3K/AKT/mTOR pathway to inhibit autophagy (137). The protective effect of RES against DOX cardiotoxicity is largely dependent on its ability to regulate autophagy through the AMPK/mTOR/Ulk1 signaling pathway (138).
Beclin 1 was identified as a Bcl-2-interacting protein that is essential for autophagy (139). DOX-induced mitochondrial autophagy was evidenced by increased Beclin 1, LC3, decreased p62, and co-localization of LC3 in mitochondria (140). LC3 is associated with the development and maturation of autophagic vesicles (141). SMI inhibited excessive myocardial autophagy by downregulating Beclin1 expression and attenuated DOX-induced myocardial injury (142), in an in vitro model of DOX-induced cardiotoxicity and also attenuated myocardial cell damage by deactivating the JNK signaling pathway and blocking autophagy formation (143). Ginsenoside Rg1 reduced DOX-induced LC3, autophagy-related genes (Atg) 5, and Beclin 1 expression and improved cardiac insufficiency (144). CA exerted cardioprotective effects through Atg7 by promoting autophagic vesicle formation in a DOX-induced zebrafish embryonic heart injury model (145). XML reduced Beclin 1 and Atg7 accumulation, upregulated protein kinase B (PKB)/Akt, PI3K, and Bcl-2 levels, and inhibited autophagy to alleviate cardiomyopathy (146) (Figure 5).
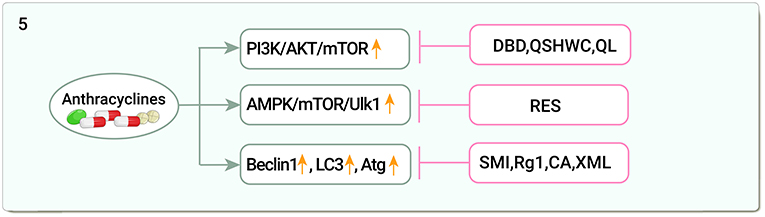
Figure 5. Traditional Chinese medicine alleviates cardiotoxicity associated with chemoradiotherapy by regulating autophagy (PI3K/AKT/mTOR, phosphatidylinositol 3-kinase/serine-threonine protein kinasem/Mechanistic Target Of Rapamycin; AMPK/mTOR/Ulk1, Adenosine monophosphate-activated protein kinase/Mechanistic Target Of Rapamycin /Unc-51-like autophagy activated kinase; LC3, Light chain-3; Atg, Autophagy-related genes; DBD, Danggui Buxue decoction; QSHWC, Qishen Huanwu Capsule; QL, Qiliqiangxin; RES, Resveratrol; SMI, Shenmai Injection; Rg1, Ginsenoside Rg1; CA, Calycosin; XML, Xinmailong injection).
DOX induces autophagy through activation of PI3K/AKT/mTOR, and AMPK/mTOR key pathways as well as autophagy-related genes such as Beclin 1, LC3, and Atg. TCM regulates autophagy through these pathways and alleviates DOX-induced cardiotoxicity. In addition to these mechanisms, whether non-coding RNAs regulate autophagy, epigenetics of autophagy, protein modification and autophagy activation, and other important transcription factors such as FOXO, E2F, and TFEB, which are involved in the regulation of autophagy, can become new targets of anti-DOX cardiotoxicity in TCM, needs to be confirmed by a large number of studies, which also provides us with a direction for future research.
Endoplasmic reticulum stress
DOX can cause marked endoplasmic reticulum (ER) expansion in the human heart (147), upregulating the stress protein kinase R-like endoplasmic reticulum kinase (PERK), C/EBP homologous protein (CHOP), and activating transcription factor 6 (ATF6) in cardiac tissue (148). Baoyuan decoction reduced glucose regulated protein78 (GRP78), PERK, eukaryotic translation initiation factor 2-alpha (eIF2α), and CHOP protein and mRNA expression and ameliorated DOX-induced myocardial injury by inhibiting CMs apoptosis by downregulating the endoplasmic reticulum stress (ERS) apoptotic pathway (149) (Figure 6).
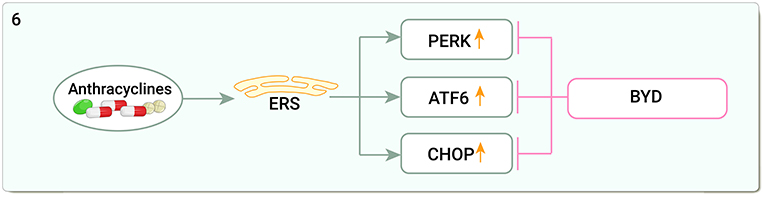
Figure 6. Traditional Chinese medicine alleviates cardiotoxicity associated with chemoradiotherapy by inhibiting endoplasmic reticulum stress (ERS, Endoplasmic reticulum stress; PERK, The stress protein kinase R-like endoplasmic reticulum kinase; ATF6, Activating transcription factor 6; CHOP:C/EBP homologous protein; BYD, Baoyuan decoction).
Studies on the mitigation of cardiotoxicity by TCM through ERS are limited and restricted to three pathways of ERS itself. In fact, many cellular processes including inflammation, apoptosis, and autophagy are regulated by the ERS pathway, and the importance of ER and its signaling pathways in inflammation, apoptosis, and autophagy in DOX-induced cardiotoxicity suggests that it may be a key factor in reducing DOX-induced cardiotoxicity (148).
Myocardial energy metabolism
DOX impairs most of the processes of myocardial energy metabolism through oxidative phosphorylation, the mitochondrial respiratory chain, and the AMPK signaling pathway, leading to significant downregulation of AMPKα2, peroxisome proliferator-activated receptors α (PPARα), and the peroxisome proliferator-activated receptor γ-coactivator 1α (PGC-1α) expression and affecting cardiac function (150, 151). QUE regulates the AMPK signaling pathway by promoting AMPKα2, PPARα, and PGC-1α expression to improve myocardial energy metabolism and prevent DOX-induced cardiac damage in rats (152). Astragalus membranaceus Bunge promotes fatty acid metabolism and activates PPARγ in DOX-induced heart failure in mice to maintain fatty acid homeostasis in H9C2 cells, thereby alleviating myocardial injury (153). Taraxacum mongolicum Hand.-Mazz. aqueous extract can activate P-glycoprotein in the cardiac tissue of triple-negative breast cancer patients and ameliorate DOX-induced cardiotoxicity (154) (Figure 7).

Figure 7. Traditional Chinese medicine alleviates cardiotoxicity associated with chemoradiotherapy by regulating myocardial energy metabolism (AMPK, adenosine monophosphate-activated protein kinase; PPAR, peroxisome proliferator-activated receptors; QUE, Quercetin).
Although the oxidation of mitochondrial fatty acids and carbohydrates is the main source of ATP production in the heart, the oxidation of other energy substrates, such as ketones and branched-chain amino acids, also contributes to energy production (155), and the use of TCM to improve myocardial energy metabolism to alleviate cardiotoxicity may be a promising research direction.
Discussion
The field of oncological cardiology formed due to the realization that cancer treatment-related cardiovascular disease is a major challenge for both cardiologists and oncologists (156). Aging populations and advances in diagnosis and treatment have improved survival rates for patients with cancer (157) but have also increased the incidence of cancer treatment-related cardiotoxicity (158). Cardiotoxicity is the result of a combination of mechanisms, and there are no effective western drugs that can reverse this damage. TCMs are unique in the treatment of chemoradiotherapy-related cardiotoxicity because of their single-target superposition, multi-target synergy, toxicity dispersion effects in many potent forms, and their ability to weaken their own toxicity (159). This paper summarizes six important mechanisms of TCM in the treatment of chemoradiotherapy-related cardiotoxicity: anti-OS and inflammation, regulation of apoptosis and autophagy, alleviation of ERS, and improvement of myocardial energy metabolism. The molecules and pathways involved include ROS, Noxs, Nrf2, TGF-β/Smad, NF-κB, NLRP3, P53, PI3K/AKT, MAPK, AMPK, PI3K/AKT/mTOR, AMPK/mTOR/Ulk1, Beclin1, LC3, Atg, ERS Pathway, ATP, ADP and PPAR, showing that the broad role and good effect of TCM in the treatment of chemoradiotherapy-related cardiotoxicity.
Among the many anti-cardiotoxic TCMs summarized in this paper, some drugs have attracted our attention, including various extracts and active ingredients of Salvia miltiorrhiza: STS, Tan I, DSS, SalB, DHT, danshensu, Cts, DB, CDDP; Astragalus membranaceus Bunge and its extracts AS-IV and CA; active ingredients of Panax ginseng: Ginsenoside Rg1, Ginsenoside Rb1, Panax ginseng glycoproteins, and Maltol. Soup containing Angelica sinensis DBD and AS-AM. Salvia miltiorrhiza is a well-known herb with a wide range of cardiovascular protective effects. Previous studies have shown that the lipophilic components (tanshinone I, tanshinone IIa, tanshinone IIb, cryptotanshinone, dihydrotanshinone, etc.) and the hydrophilic components (danshensu, salvianolic acid A and B, protocatechuic aldehyde, etc.) are involved in the cardioprotective effects of Salvia miltiorrhiza (160). Tanshinone IIA (Tan IIa) is a lipid-soluble compound isolated from the traditional Chinese medicine Salvia miltiorrhiza (161), and STS is a water-soluble derivative of Tan IIa (162), which can effectively inhibit the interaction between DNA and intracellular lipid peroxidation products (163) and can alleviate cardiotoxicity by anti-OS and reducing apoptosis. Astragalus membranaceus Bunge is the holy medicine of supplement Qi, which has the effect of supplement Qi, raising Yang, nourishing the Wei Qi, and fixing the surface. Eight key components in Astragalus membranaceus Bunge, including hederagenin, quercetin, calycosin, formononetin, jaranol, isorhamnetin, astragaloside III, and 9,10-dimethoxypterocarpan-3-O-β-D-glucoside, are involved in lipid metabolism, programmed cell death, fatty acid metabolism, which produce the ability to regulate the body's immune function, strengthen the heart, protect CM, improve substance metabolism (164), AS-IV is a cyclic aromatic triterpene glycoside compound, which is one of the main active components of Astragalus membranaceus Bunge and has good antioxidant activity (165), and can alleviate cardiotoxicity by anti-OS and improving myocardial energy metabolism. Panax ginseng is a widely used herb in the world, containing more polysaccharides and amino acids, with better protective effects against cardiovascular diseases, neurological diseases, cancer, and diabetes (166). Ginsenosides are the main active components of ginseng, which can reduce cardiotoxicity through anti-OS, reduce apoptosis and regulate autophagy. Angelica sinensis is one of the most popular traditional TCM, which has long been used as a blood tonic and blood activator, pain reliever, laxative, and treatment of female menstrual disorders and amenorrhea. It contains polysaccharides, ligustrolactone, ferulic acid, and other bioactive components, with antioxidant, anti-inflammatory, anti-fibrotic, and cardiocerebrovascular protective effects (167). DBD is a classical formula in TCM to supplement Qi and replenish blood. It is composed of Astragalus membranaceus Bunge and Angelica sinensis in a 5:1 ratio, and DBD and its extract AS-AM alleviate cardiotoxicity through various pathways such as anti-OS, inhibition of apoptosis and TGF-β overexpression, and reduction of autophagy. In conclusion, Salvia miltiorrhiza, Astragalus membranaceus Bunge, Panax ginseng, and Angelica sinensis are important TCMs against cardiotoxicity and should be given more attention.
Although significant progress has been made in exploring the molecular mechanisms of TCM against chemoradiotherapy-related cardiotoxicity, research on TCM against cardiotoxicity is still facing some problems and shortcomings. (1) The studies on the anti- chemoradiotherapy-related cardiotoxicity of TCM are limited to OS, inflammation, apoptosis, autophagy, ERS, and myocardial energy metabolism, but other important mechanisms such as Top2β have not been addressed, and many investigations into TCM have only studied one of these mechanisms, which is not conducive to our comprehensive understanding of the mechanisms of anti-cardiotoxicity of TCM. (2) Most of the studies were focus on chemotherapy-induced cardiotoxicity (particularly anthracyclines), and fewer studies were done on radiotherapy and non-anthracyclines. Among the six mechanisms summarized in this paper, only two mechanisms of OS and apoptosis were involved in radiotherapy, and even fewer in non-anthracyclines. However, the cardiotoxicity caused by radiotherapy and non-anthracycline drugs is worthy of attention, such as myocarditis caused by immune checkpoint inhibitors and the decrease of LVEF caused by arsenic trioxide, which may be alleviated by TCM, and this also broadens the idea of research on the effects of TCM on anti-radiotherapy cardiotoxicity. (3) Most of the studies on anti-cardiotoxicity of TCM are limited to the cellular level and animal trials, and till date, only a few clinical control studies have been conducted, with small sample size and irregular design, and the reproducibility of many therapies and prescriptions is poor. (4) The specific medicinal components and active parts of some herbal monomers, extracts, and compounds are not clear, and their targets are unknown, so high-performance liquid chromatography and mass spectrometry may be needed to identify the drug components and lay a clearer material basis for pharmacological research. (5) There is a lack of knowledge about the safety of TCM and their interactions with western drugs, which also limits the applications of TCM. (6) TCM emphasizes both a holistic view of the body and evidence-based treatment; patients with similar symptoms may be treated with different drugs because they suffer from different conditions, which requires the establishment of a systematic set of diagnostic and therapeutic criteria for better clinical treatment and research. (7) This paper only includes the studies on the cardiotoxicity caused by radiotherapy, anthracycline chemotherapy and two non-anthracycline chemotherapy drugs, CP and cyc, but not the studies on the cardiotoxicity caused by other drugs, such as immune checkpoint inhibitors (ICIs), arsenic trioxide and targeted chemotherapy drugs, which may make this study less comprehensive.
In addition to cardiotoxicity caused by radiotherapy and classical chemotherapeutic agents, ICIs, arsenic trioxide, and other antineoplastic agents may cause cardiotoxicity. ICIs, a unique antibody-based therapeutic strategy that has revolutionized the treatment landscape for solid and hematologic cancers, has been shown in a growing number of preclinical studies to trigger myocardial inflammation, and the incidence of cardiotoxicity in ICIs therapy may be underestimated (168). Cardiac immune-associated adverse events are rare but potentially fatal complications of immunotherapy with various potential risk factors, such as combinations of different ICIs (169). No studies related to the cardiotoxicity of TCM on ICIs have been retrieved, but this may be a neglected area of study. For TCM treatment of arsenic trioxide-induced cardiotoxicity, relatively few studies were found. One such studies indicated that crocin ameliorates arsenic trioxide-induced cardiotoxicity by reducing OS, inflammation, and apoptosis (170, 171), magnesium isoglycyrrhizinate attenuates via Nrf2 and TLR4/NF-κB signaling pathways arsenic trioxide-induced cardiotoxicity (172). In addition, microRNAs and non-coding RNAs are also involved in the pathogenesis of radiotherapy-associated cardiotoxicity (173–175), mitochondrial fusion (176), and cellular scorching (177). It is also a hot topic of current research that TCM can exert anti-cardiotoxic effects through these pathways, and we look forward to seeing more of these findings.
Conclusion
Cardiotoxicity development is the result of a combination of mechanisms. In recent years, the benefits of TCM in chemoradiotherapy-related cardiotoxicity have become evident. Herbal monomers, such as AS-IV and STS, herbal decoction, such as DBD and SXT, or compound preparations, such as SMI and QSHWC, can protect CMs through antioxidation, anti-inflammation, regulating autophagy and apoptosis, inhibiting ERS, and improving myocardial energy metabolism, and play a role in reducing anti-radiotherapy-related cardiotoxicity. Moreover, TCM is a promising drug for treating chemoradiotherapy-related cardiotoxicity, both guided by TCM theory and supported by modern research. However, our conclusions are based on numerous basic, smaller experiments and lack the results of large-scale clinical trials. We look forward to more relevant randomized controlled trials to show the benefits of TCM on chemoradiotherapy-related cardiotoxicity.
Author contributions
X-FL, R-QW, Y-DL, KL, and X-KZ designed the study. X-FL, XG, XW, and X-DZ acquired and researched the data for the article. C-LP, C-ZR, and Q-LC discussed its content. X-FL wrote the manuscript. C-LP, W-JL, and T-YB revised the manuscript. All authors read and approved the final manuscript.
Funding
This work was partly supported by the National Natural Science Foundation of China (No. 81873132), the Science and Technology Innovation Project of Gansu Provincial Education Department (No. 2021jyjbgs-03), and the Gansu Provincial Scientific Research Project on Prevention and Treatment of Major Diseases in Traditional Chinese Medicine (No. GZKZD-2018-02).
Acknowledgments
We thank all those who are committed to TCM and chemoradiotherapy-related cardiotoxicity.
Conflict of interest
The authors declare that the research was conducted in the absence of any commercial or financial relationships that could be construed as a potential conflict of interest.
Publisher's note
All claims expressed in this article are solely those of the authors and do not necessarily represent those of their affiliated organizations, or those of the publisher, the editors and the reviewers. Any product that may be evaluated in this article, or claim that may be made by its manufacturer, is not guaranteed or endorsed by the publisher.
References
1. Zamorano JL, Lancellotti P, Rodriguez Muñoz D, Aboyans V, Asteggiano R, Galderisi M, et al. 2016 ESC position paper on cancer treatments and cardiovascular toxicity developed under the auspices of the ESC committee for practice guidelines: the task force for cancer treatments and cardiovascular toxicity of the European society of cardiology (ESC). Eur Heart J. (2016) 37:2768–801. doi: 10.1093/eurheartj/ehw211
2. de Boer RA, Aboumsallem JP, Bracun V, Leedy D, Cheng R, Patel S, et al. A new classification of cardio-oncology syndromes. Cardiooncology. (2021) 7:24. doi: 10.1186/s40959-021-00110-1
3. Kostakou PM, Kouris NT, Kostopoulos VS, Damaskos DS, Olympios CD. Cardio-oncology: a new and developing sector of research and therapy in the field of cardiology. Heart Fail Rev. (2019) 24:91–100. doi: 10.1007/s10741-018-9731-y
4. Curigliano G, Cardinale D, Dent S, Criscitiello C, Aseyev O, Lenihan D, et al. Cardiotoxicity of anticancer treatments: epidemiology, detection, and management. CA Cancer J Clin. (2016) 66:309–25. doi: 10.3322/caac.21341
5. Jacobse JN, Schaapveld M, Boekel NB, Hooning MJ, Jager A, Baaijens MHA, et al. Risk of heart failure after systemic treatment for early breast cancer: results of a cohort study. Breast Cancer Res Treat. (2021) 185:205–14. doi: 10.1007/s10549-020-05930-w
6. Baech J, Hansen SM, Lund PE, Soegaard P, Brown PN, Haaber J, et al. Cumulative anthracycline exposure and risk of cardiotoxicity; a Danish nationwide cohort study of 2440 lymphoma patients treated with or without anthracyclines. Br J Haematol. (2018) 183:717–26. doi: 10.1111/bjh.15603
7. Tan VZZ, Chan NM, Ang WL, Mya SN, Chan MY, Chen CK. Cardiotoxicity after anthracycline chemotherapy for childhood cancer in a multiethnic Asian population. Front Pediatr. (2021) 9:639603. doi: 10.3389/fped.2021.639603
8. Bergom C, Bradley JA, Ng AK, Samson P, Robinson C, Lopez-Mattei J, et al. Past, present, and future of radiation-induced cardiotoxicity: refinements in targeting, surveillance, and risk stratification. JACC CardioOncol. (2021) 3:343–59. doi: 10.1016/j.jaccao.2021.06.007
9. Quintero-Martinez JA, Cordova-Madera SN, Villarraga HR. Radiation-induced heart disease. J Clin Med. (2021) 11:146. doi: 10.3390/jcm11010146
10. Kirova Y, Tallet A, Aznar MC, Loap P, Bouali A, Bourgier C. Radio-induced cardiotoxicity: from physiopathology and risk factors to adaptation of radiotherapy treatment planning and recommended cardiac follow-up. Cancer Radiother. (2020) 24:576–85. doi: 10.1016/j.canrad.2020.07.001
11. Saleh Y, Abdelkarim O, Herzallah K, Abela GS. Anthracycline-induced cardiotoxicity: mechanisms of action, incidence, risk factors, prevention, and treatment. Heart Fail Rev. (2021) 26:1159–73. doi: 10.1007/s10741-020-09968-2
12. Leong DP, Lenihan DJ. Clinical practice guidelines in cardio-oncology. Heart Fail Clin. (2022) 18:489–501. doi: 10.1016/j.hfc.2022.02.002
13. Lenneman CG, Sawyer DB. Cardio-oncology: an update on cardiotoxicity of cancer-related treatment. Circ Res. (2016) 118:1008–20. doi: 10.1161/CIRCRESAHA.115.303633
14. Obasi M, Abovich A, Vo JB, Gao Y, Papatheodorou SI, Nohria A, et al. Correction to: statins to mitigate cardiotoxicity in cancer patients treated with anthracyclines and/or trastuzumab: a systematic review and meta-analysis. Cancer Causes Control. (2021) 32:1407–9. doi: 10.1007/s10552-021-01495-1
15. Obasi M, Abovich A, Vo JB, Gao Y, Papatheodorou SI, Nohria A, et al. Statins to mitigate cardiotoxicity in cancer patients treated with anthracyclines and/or trastuzumab: a systematic review and meta-analysis. Cancer Causes Control. (2021) 32:1395–405. doi: 10.1007/s10552-021-01487-1
16. Atkins KM, Bitterman DS, Chaunzwa TL, Williams CL, Rahman R, Kozono DE, et al. Statin use, heart radiation dose, and survival in locally advanced lung cancer. Pract Radiat Oncol. (2021) 11:e459–67. doi: 10.1016/j.prro.2020.12.006
17. Cardinale D, Ciceri F, Latini R, Franzosi MG, Sandri MT, Civelli M, et al. Anthracycline-induced cardiotoxicity: a multicenter randomised trial comparing two strategies for guiding prevention with enalapril: the international cardiooncology society-one trial. Eur J Cancer. (2018) 94:126–37. doi: 10.1016/j.ejca.2018.02.005
18. Avila MS, Ayub-Ferreira SM, de Barros Wanderley MR Jr, das Dores Cruz F, Gonçalves Brandão SM, et al. Carvedilol for prevention of chemotherapy-related cardiotoxicity: the CECCY trial. J Am Coll Cardiol. (2018) 71:2281–90. doi: 10.1016/j.jacc.2018.02.049
19. Wang Y, Lu C, Li H, Liu K, Yu M, Zhang P. 3D-STI evaluation of the effect of dexrazoxane on the mechanical properties of right ventricular myocardium in breast cancer patients treated with pirarubicin. Ann Palliat Med. (2020) 9:1187–97. doi: 10.21037/apm-20-1074
20. Chow EJ, Aplenc R, Vrooman LM, Doody DR, Huang YV, Aggarwal S, et al. Late health outcomes after dexrazoxane treatment: a report from the children's oncology group. Cancer. (2022) 128:788–96. doi: 10.1002/cncr.33974
21. Ellahham S, Khalouf A, Elkhazendar M, Dababo N, Manla Y. An overview of radiation-induced heart disease. Radiat Oncol J. (2022) 40:89–102. doi: 10.3857/roj.2021.00766
22. Qu L, Zou W, Wang Y, Wang M. European regulation model for herbal medicine: the assessment of the EU monograph and the safety and efficacy evaluation in marketing authorization or registration in member states. Phytomedicine. (2018) 42:219–25. doi: 10.1016/j.phymed.2018.03.048
23. Tu Y. Artemisinin-a gift from traditional chinese medicine to the world (nobel lecture). Angew Chem Int Ed Engl. (2016) 55:10210–26. doi: 10.1002/anie.201601967
24. Kayser S, Schlenk RF, Lebon D, Carre M, Götze KS, Stölzel F, et al. Characteristics and outcome of patients with low-/intermediate-risk acute promyelocytic leukemia treated with arsenic trioxide: an international collaborative study. Haematologica. (2021) 106:3100–6. doi: 10.3324/haematol.2021.278722
25. Chen L, Zhu HM, Li Y, Liu QF, Hu Y, Zhou JF, et al. Arsenic trioxide replacing or reducing chemotherapy in consolidation therapy for acute promyelocytic leukemia (APL2012 trial). Proc Natl Acad Sci U S A. (2021) 118:e2020382118. doi: 10.1073/pnas.2020382118
26. Wang Z, Yang L. Chinese herbal medicine: fighting SARS-CoV-2 infection on all fronts. J Ethnopharmacol. (2021) 270:113869. doi: 10.1016/j.jep.2021.113869
27. Lee DYW, Li QY, Liu J, Efferth T. Traditional Chinese herbal medicine at the forefront battle against COVID-19: clinical experience and scientific basis. Phytomedicine. (2021) 80:153337. doi: 10.1016/j.phymed.2020.153337
28. Leung EL, Pan HD, Huang YF, Fan XX, Wang WY, He F, et al. The scientific foundation of Chinese herbal medicine against COVID-19. Engineering. (2020) 6:1099–107. doi: 10.1016/j.eng.2020.08.009
29. Wu XQ, Zhang WN, Hao MZ, Liu XP, Xiao J, Wang TF, et al. How Chinese herbal medicine prevents epidemics: from ancient pestilences to COVID-19 pandemic. Am J Chin Med. (2021) 49:1017–44. doi: 10.1142/S0192415X2150049X
30. Zhang SQ, Li JC. An introduction to traditional Chinese medicine, including acupuncture. Anat Rec. (2021) 304:2359–64. doi: 10.1002/ar.24782
31. Lam WC, Lyu A, Bian Z. ICD-11: impact on traditional chinese medicine and world healthcare systems. Pharmaceut Med. (2019) 33:373–7. doi: 10.1007/s40290-019-00295-y
32. Liu N, Li X, Yang Y, Wang X, Li L, et al. A review on the effect of traditional chinese medicine against anthracycline-induced cardiac toxicity. Front Pharmacol. (2018) 9:444. doi: 10.3389/fphar.2018.00444
33. Sies H, Berndt C, Jones DP. Oxidative stress. Annu Rev Biochem. (2017) 86:715–48. doi: 10.1146/annurev-biochem-061516-045037
34. Sarniak A, Lipińska J, Tytman K, Lipińska S. Endogenous mechanisms of reactive oxygen species (ROS) generation. Postepy Hig Med Dosw. (2016) 70:1150–65. doi: 10.5604/17322693.1224259
35. Narezkina A, Narayan HK, Zemljic-Harpf AE. Molecular mechanisms of anthracycline cardiovascular toxicity. Clin Sci. (2021) 135:1311–32. doi: 10.1042/CS20200301
36. Vermot A, Petit-Härtlein I, Smith SME, Fieschi F. NADPH oxidases (NOX): an overview from discovery, molecular mechanisms to physiology and pathology. Antioxidants. (2021) 10:890. doi: 10.3390/antiox10060890
37. Kasai S, Shimizu S, Tatara Y, Mimura J, Itoh K. Regulation of Nrf2 by mitochondrial reactive oxygen species in physiology and pathology. Biomolecules. (2020) 10:320. doi: 10.3390/biom10020320
38. Pignatelli P, Menichelli D, Pastori D, Violi F. Oxidative stress and cardiovascular disease: new insights. Kardiol Pol. (2018) 76:713–22. doi: 10.5603/KP.a2018.0071
39. Incalza MA, D'Oria R, Natalicchio A, Perrini S, Laviola L, Giorgino F. Oxidative stress and reactive oxygen species in endothelial dysfunction associated with cardiovascular and metabolic diseases. Vascul Pharmacol. (2018) 100:1–19. doi: 10.1016/j.vph.2017.05.005
40. Tang Z, Wang P, Dong C, Zhang J, Wang X, Pei H. Oxidative stress signaling mediated pathogenesis of diabetic cardiomyopathy. Oxid Med Cell Longev. (2022) 2022:5913374. doi: 10.1155/2022/5913374
41. Ping Z, Peng Y, Lang H, Xinyong C, Zhiyi Z, Xiaocheng W, et al. Oxidative stress in radiation-induced cardiotoxicity. Oxid Med Cell Longev. (2020) 2020:3579143. doi: 10.1155/2020/3579143
42. Jakubczyk K, Dec K, Kałduńska J, Kawczuga D, Kochman J, Janda K. Reactive oxygen species - sources, functions, oxidative damage. Pol Merkur Lekarski. (2020) 48:124–7. Available online at: http://medpress.com.pl/shop
43. Tsikas D. Assessment of lipid peroxidation by measuring malondialdehyde (MDA) and relatives in biological samples: analytical and biological challenges. Anal Biochem. (2017) 524:13–30. doi: 10.1016/j.ab.2016.10.021
44. Lassègue B, Griendling KK. NADPH oxidases: functions and pathologies in the vasculature. Arterioscler Thromb Vasc Biol. (2010) 30:653–61. doi: 10.1161/ATVBAHA.108.181610
45. Azzam EI, Jay-Gerin JP, Pain D. Ionizing radiation-induced metabolic oxidative stress and prolonged cell injury. Cancer Lett. (2012) 327:48–60. doi: 10.1016/j.canlet.2011.12.012
46. Yamamori T, Yasui H, Yamazumi M, Wada Y, Nakamura Y, Nakamura H, et al. Ionizing radiation induces mitochondrial reactive oxygen species production accompanied by upregulation of mitochondrial electron transport chain function and mitochondrial content under control of the cell cycle checkpoint. Free Radic Biol Med. (2012) 53:260–70. doi: 10.1016/j.freeradbiomed.2012.04.033
47. Okwuosa TM, Anzevino S, Rao R. Cardiovascular disease in cancer survivors. Postgrad Med J. (2017) 93:82–90. doi: 10.1136/postgradmedj-2016-134417
48. Zhang W, Li Y, Li R, Wang Y, Zhu M, Wang B, et al. Sodium tanshinone IIA sulfonate prevents radiation-induced toxicity in H9c2 cardiomyocytes. Evid Based Complement Alternat Med. (2017) 2017:4537974. doi: 10.1155/2017/4537974
49. Zhou D, Yang WK, Zhang YF, Xie JH, Ma L, Yang HD, et al. Sodium tanshinone IIA sulfonate prevents radiation-induced damage in primary rat cardiac fibroblasts. Chin J Nat Med. (2020) 18:436–45. doi: 10.1016/S1875-5364(20)30051-0
50. Ejaz A, Greenberger JS, Rubin PJ. Understanding the mechanism of radiation induced fibrosis and therapy options. Pharmacol Ther. (2019) 204:107399. doi: 10.1016/j.pharmthera.2019.107399
51. Ma C, Zhao X, Chang J, Guo H, Wei H, Fu Z, et al. Radix angelica sinensis and radix hedysari ultrafiltration extract protects against X-irradiation-induced cardiac fibrosis in rats. Evid Based Complement Alternat Med. (2020) 2020:4675851. doi: 10.1155/2020/4675851
52. Ma C, Fu Z, Guo H, Wei H, Zhao X, Li Y. The effects of radix angelica sinensis and radix hedysari ultrafiltration extract on X-irradiation-induced myocardial fibrosis in rats. Biomed Pharmacother. (2019) 112:108596. doi: 10.1016/j.biopha.2019.01.057
53. Chang J, Ma C, Guo H, Ran H, Chen G, Li Y. Ultrafiltration extract of radix angelica sinensis and radix hedysari attenuates risk of low-dose x-ray radiation-induced myocardial fibrosis in vitro. Evid Based Complement Alternat Med. (2021) 2021:5580828. doi: 10.1155/2021/5580828
54. Kong CY, Guo Z, Song P, Zhang X, Yuan YP, Teng T, et al. Underlying the mechanisms of doxorubicin-induced acute cardiotoxicity: oxidative stress and cell death. Int J Biol Sci. (2022) 18:760–70. doi: 10.7150/ijbs.65258
55. Songbo M, Lang H, Xinyong C, Bin X, Ping Z, Liang S. Oxidative stress injury in doxorubicin-induced cardiotoxicity. Toxicol Lett. (2019) 307:41–8. doi: 10.1016/j.toxlet.2019.02.013
56. Wenningmann N, Knapp M, Ande A, Vaidya TR, Ait-Oudhia S. Insights into doxorubicin-induced cardiotoxicity: molecular mechanisms, preventive strategies, and early monitoring. Mol Pharmacol. (2019) 96:219–32. doi: 10.1124/mol.119.115725
57. Yan F, Liu R, Zhuang X, Li R, Shi H, Gao X. Salidroside attenuates doxorubicin-induced cardiac dysfunction partially through activation of QKI/FoxO1 pathway. J Cardiovasc Transl Res. (2021) 14:355–64. doi: 10.1007/s12265-020-10056-x
58. Chen H, Zhu J, Le Y, Pan J, Liu Y, Liu Z, et al. Salidroside inhibits doxorubicin-induced cardiomyopathy by modulating a ferroptosis-dependent pathway. Phytomedicine. (2022) 99:153964. doi: 10.1016/j.phymed.2022.153964
59. Li S, Liu H, Lin Z, Li Z, Chen Y, Chen B, et al. Isoorientin attenuates doxorubicin-induced cardiac injury via the activation of MAPK, Akt, and caspase-dependent signaling pathways. Phytomedicine. (2022) 101:154105. doi: 10.1016/j.phymed.2022.154105
60. Ghigo A, Li M, Hirsch E. New signal transduction paradigms in anthracycline-induced cardiotoxicity. Biochim Biophys Acta. (2016) 1863:1916–25. doi: 10.1016/j.bbamcr.2016.01.021
61. Lin J, Fang L, Li H, Li Z, Lyu L, Wang H, et al. Astragaloside IV alleviates doxorubicin induced cardiomyopathy by inhibiting NADPH oxidase derived oxidative stress. Eur J Pharmacol. (2019) 859:172490. doi: 10.1016/j.ejphar.2019.172490
62. Bellezza I, Giambanco I, Minelli A, Donato R. Nrf2-Keap1 signaling in oxidative and reductive stress. Biochim Biophys Acta Mol Cell Res. (2018) 1865:721–33. doi: 10.1016/j.bbamcr.2018.02.010
63. Dodson M, Castro-Portuguez R, Zhang DD. NRF2 plays a critical role in mitigating lipid peroxidation and ferroptosis. Redox Biol. (2019) 23:101107. doi: 10.1016/j.redox.2019.101107
64. Wallace KB, Sardão VA, Oliveira PJ. Mitochondrial determinants of doxorubicin-induced cardiomyopathy. Circ Res. (2020) 126:926–41. doi: 10.1161/CIRCRESAHA.119.314681
65. Jiang Q, Chen X, Tian X, Zhang J, Xue S, Jiang Y, et al. Tanshinone I inhibits doxorubicin-induced cardiotoxicity by regulating Nrf2 signaling pathway. Phytomedicine. (2022) 106:154439. doi: 10.1016/j.phymed.2022.154439
66. Qi JY, Yang YK, Jiang C, Zhao Y, Wu YC, Han X, et al. Exploring the mechanism of danshensu in the treatment of doxorubicin-induced cardiotoxicity based on network pharmacology and experimental evaluation. Front Cardiovasc Med. (2022) 9:827975. doi: 10.3389/fcvm.2022.827975
67. Feng K, Liu Y, Sun J, Zhao C, Duan Y, Wang W, et al. Compound danshen dripping pill inhibits doxorubicin or isoproterenol-induced cardiotoxicity. Biomed Pharmacother. (2021) 138:111531. doi: 10.1016/j.biopha.2021.111531
68. Zhao L, Tao X, Qi Y, Xu L, Yin L, Peng J. Protective effect of dioscin against doxorubicin-induced cardiotoxicity via adjusting microRNA-140-5p-mediated myocardial oxidative stress. Redox Biol. (2018) 16:189–98. doi: 10.1016/j.redox.2018.02.026
69. Liguori I, Russo G, Curcio F, Bulli G, Aran L, Della-Morte D, et al. Oxidative stress, aging, and diseases. Clin Interv Aging. (2018) 13:757–72. doi: 10.2147/CIA.S158513
70. Wu Z, Zhao X, Miyamoto A, Zhao S, Liu C, Zheng W, et al. Effects of steroidal saponins extract from ophiopogon japonicus root ameliorates doxorubicin-induced chronic heart failure by inhibiting oxidative stress and inflammatory response. Pharm Biol. (2019) 57:176–83. doi: 10.1080/13880209.2019.1577467
71. Jiang Y, Liu Y, Xiao W, Zhang D, Liu X, Xiao H, et al. Xinmailong attenuates doxorubicin-induced lysosomal dysfunction and oxidative stress in H9c2 Cells via HO-1. Oxid Med Cell Longev. (2021) 2021:5896931. doi: 10.1155/2021/5896931
72. Monahan DS, Flaherty E, Hameed A, Duffy GP. Resveratrol significantly improves cell survival in comparison to dexrazoxane and carvedilol in a h9c2 model of doxorubicin induced cardiotoxicity. Biomed Pharmacother. (2021) 140:111702. doi: 10.1016/j.biopha.2021.111702
73. Motlagh PE, Novin AG, Ghahari F, Nikzad A, Khoshandam M, Mardani S, et al. Evaluation of the effect of crocin on doxorubicin-induced cardiotoxicity. Adv Exp Med Biol. (2021) 1328:143–53. doi: 10.1007/978-3-030-73234-9_10
74. Rawat PS, Jaiswal A, Khurana A, Bhatti JS, Navik U. Doxorubicin-induced cardiotoxicity: an update on the molecular mechanism and novel therapeutic strategies for effective management. Biomed Pharmacother. (2021) 139:111708. doi: 10.1016/j.biopha.2021.111708
75. Xu Y, Qu X, Zhou J, Lv G, Han D, Liu J, et al. Pilose antler peptide-3.2KD ameliorates adriamycin-induced myocardial injury through TGF-β/SMAD signaling pathway. Front Cardiovasc Med. (2021) 8:659643. doi: 10.3389/fcvm.2021.659643
76. Sun X, Song Y, Xie Y, Han J, Chen F, Sun Y, et al. Shenlijia attenuates doxorubicin-induced chronic heart failure by inhibiting cardiac fibrosis. Evid Based Complement Alternat Med. (2021) 2021:6659676. doi: 10.1155/2021/6659676
77. Madeddu C, Deidda M, Piras A, Cadeddu C, Demurtas L, Puzzoni M, et al. Pathophysiology of cardiotoxicity induced by nonanthracycline chemotherapy. J Cardiovasc Med. (2016) 17 Suppl 1:S12–18. doi: 10.2459/JCM.0000000000000376
78. Zhang P, Zhang J, Zhao L, Li S, Li K. Quercetin attenuates the cardiotoxicity of doxorubicin-cyclophosphamide regimen and potentiates its chemotherapeutic effect against triple-negative breast cancer. Phytother Res. (2022) 36:551–61. doi: 10.1002/ptr.7342
79. Ye B, Ling W, Wang Y, Jaisi A, Olatunji OJ. Protective effects of chrysin against cyclophosphamide-induced cardiotoxicity in rats: a biochemical and histopathological approach. Chem Biodivers. (2022) 19:e202100886. doi: 10.1002/cbdv.202100886
80. Bahadir A, Ceyhan A, Öz Gergin Ö, Yalçin B, Ülger M, Özyazgan TM, et al. Protective effects of curcumin and beta-carotene on cisplatin-induced cardiotoxicity: an experimental rat model. Anatol J Cardiol. (2018) 19:213–21. doi: 10.14744/AnatolJCardiol.2018.53059
81. Chakraborty M, Bhattacharjee A, Kamath JV. Cardioprotective effect of curcumin and piperine combination against cyclophosphamide-induced cardiotoxicity. Indian J Pharmacol. (2017) 49:65–70. doi: 10.53879/id.53.11.10769
82. Avci H, Epikmen ET, Ipek E, Tunca R, Birincioglu SS, Akşit H. Protective effects of silymarin and curcumin on cyclophosphamide-induced cardiotoxicity. Exp Toxicol Pathol. (2017) 69:317–27. doi: 10.1016/j.etp.2017.02.002
83. Xia J, Hu JN, Zhang RB, Liu W, Zhang H, Wang Z, et al. Icariin exhibits protective effects on cisplatin-induced cardiotoxicity via ROS-mediated oxidative stress injury in vivo and in vitro. Phytomedicine. (2022) 104:154331. doi: 10.1016/j.phymed.2022.154331
84. Topal I, Özbek Bilgin A, Keskin Çimen F, Kurt N, Süleyman Z, Bilgin Y, et al. The effect of rutin on cisplatin-induced oxidative cardiac damage in rats. Anatol J Cardiol. (2018) 20:136–42. doi: 10.14744/AnatolJCardiol.2018.32708
85. Wang SH, Tsai KL, Chou WC, Cheng HC, Huang YT, Ou HC, et al. Quercetin mitigates cisplatin-induced oxidative damage and apoptosis in cardiomyocytes through Nrf2/HO-1 signaling pathway. Am J Chin Med. (2022) 50:1281–98. doi: 10.1142/S0192415X22500537
86. Xiao Z, Liu W, Mu YP, Zhang H, Wang XN, Zhao CQ, et al. Pharmacological effects of salvianolic acid B against oxidative damage. Front Pharmacol. (2020) 11:572373. doi: 10.3389/fphar.2020.572373
87. Lin Z, Bao Y, Hong B, Wang Y, Zhang X, Wu Y. Salvianolic acid B attenuated cisplatin-induced cardiac injury and oxidative stress via modulating Nrf2 signal pathway. J Toxicol Sci. (2021) 46:199–207. doi: 10.2131/jts.46.199
88. Qi Y, Fu S, Pei D, Fang Q, Xin W, Yuan X, et al. Luteolin attenuated cisplatin-induced cardiac dysfunction and oxidative stress via modulation of Keap1/Nrf2 signaling pathway. Free Radic Res. (2022) 56:209–21. doi: 10.1080/10715762.2022.2067042
89. Cui Y, Li C, Zeng C, Li J, Zhu Z, Chen W, et al. Tongmai yangxin pills anti-oxidative stress alleviates cisplatin-induced cardiotoxicity: network pharmacology analysis and experimental evidence. Biomed Pharmacother. (2018) 108:1081–9. doi: 10.1016/j.biopha.2018.09.095
90. Behranvand N, Nasri F, Zolfaghari Emameh R, Khani P, Hosseini A, Garssen J, et al. Chemotherapy: a double-edged sword in cancer treatment. Cancer Immunol Immunother. (2022) 71:507–26. doi: 10.1007/s00262-021-03013-3
91. Li W, Cao T, Luo C, Cai J, Zhou X, Xiao X, et al. Crosstalk between ER stress, NLRP3 inflammasome, and inflammation. Appl Microbiol Biotechnol. (2020) 104:6129–40. doi: 10.1007/s00253-020-10614-y
92. Wang X, Wang Q, Li W, Zhang Q, Jiang Y, Guo D, et al. TFEB-NF-κB inflammatory signaling axis: a novel therapeutic pathway of Dihydrotanshinone I in doxorubicin-induced cardiotoxicity. J Exp Clin Cancer Res. (2020) 39:93. doi: 10.1186/s13046-020-01595-x
93. Li X, Liang J, Qin A, Wang T, Liu S, Li W, et al. Protective effect of Di'ao Xinxuekang capsule against doxorubicin-induced chronic cardiotoxicity. J Ethnopharmacol. (2022) 287:114943. doi: 10.1016/j.jep.2021.114943
94. Yao L, Gui M, Li J, Lu B, Wang J, Zhou X, et al. Shengxian decoction decreases doxorubicin-induced cardiac apoptosis by regulating the TREM1/NF-κB signaling pathway. Mol Med Rep. (2021) 23:219. doi: 10.3892/mmr.2021.11858
95. Abu Gazia M, El-Magd MA. Ameliorative effect of cardamom aqueous extract on doxorubicin-induced cardiotoxicity in rats. Cells Tissues Organs. (2018) 206:62–72. doi: 10.1159/000496109
96. Ibrahim Fouad G, Ahmed KA. Curcumin ameliorates doxorubicin-induced cardiotoxicity and hepatotoxicity via suppressing oxidative stress and modulating iNOS, NF-κB, and TNF-α in rats. Cardiovasc Toxicol. (2022) 22:152–66. doi: 10.1007/s12012-021-09710-w
97. Gu Y, Ju A, Jiang B, Zhang J, Man S, Liu C, et al. Yiqi Fumai lyophilized injection attenuates doxorubicin-induced cardiotoxicity, hepatotoxicity and nephrotoxicity in rats by inhibition of oxidative stress, inflammation and apoptosis. RSC Adv. (2018) 8:40894–911. doi: 10.1039/C8RA07163B
98. Xing JJ, Hou JG, Liu Y, Zhang RB, Jiang S, Ren S, et al. Supplementation of saponins from leaves of panax quinquefolius mitigates cisplatin-evoked cardiotoxicity via inhibiting oxidative stress-associated inflammation and apoptosis in mice. Antioxidants. (2019) 8:347. doi: 10.3390/antiox8090347
99. Zhai J, Tao L, Zhang S, Gao H, Zhang Y, Sun J, et al. Calycosin ameliorates doxorubicin-induced cardiotoxicity by suppressing oxidative stress and inflammation via the sirtuin 1-NOD-like receptor protein 3 pathway. Phytother Res. (2020) 34:649–59. doi: 10.1002/ptr.6557
100. Maayah ZH, Alam AS, Takahara S, Soni S, Ferdaoussi M, Matsumura N, et al. Resveratrol reduces cardiac NLRP3-inflammasome activation and systemic inflammation to lessen doxorubicin-induced cardiotoxicity in juvenile mice. FEBS Lett. (2021) 595:1681–95. doi: 10.1002/1873-3468.14091
101. Zhao YY, Chen LH, Huang L, Li YZ, Yang C, Zhu Y, et al. Cardiovascular protective effects of GLP-1: a focus on the MAPK signaling pathway. Biochem Cell Biol. (2022) 100:9–16. doi: 10.1139/bcb-2021-0365
102. Yue J, López JM. Understanding MAPK signaling pathways in apoptosis. Int J Mol Sci. (2020) 21:2346. doi: 10.3390/ijms21072346
103. Aubrey BJ, Kelly GL, Janic A, Herold MJ, Strasser A. How does p53 induce apoptosis and how does this relate to p53-mediated tumour suppression? Cell Death Differ. (2018) 25:104–13. doi: 10.1038/cdd.2017.169
104. Verheij M, Bartelink H. Radiation-induced apoptosis. Cell Tissue Res. (2000) 301:133–42. doi: 10.1007/s004410000188
105. Zhao XK, Qiu YY, Li YD, Jiang HG, Fei GJ, et al. Exploration of the effect of FasL/TNF-α signaling pathway-based angelica tonic blood soup on apoptotic response to radiation myocardial injury. Lishizhen Med Materia Medica Res. (2020) 31:1312–4. doi: 10.3969/j.issn.1008-0805.2020.06.009
106. Zhu C, Wang Y, Liu H, Mu H, Lu Y, Zhang J, et al. Oral administration of ginsenoside Rg1 prevents cardiac toxicity induced by doxorubicin in mice through anti-apoptosis. Oncotarget. (2017) 8:83792–801. doi: 10.18632/oncotarget.19698
107. Zhang Y, Wang Y, Ma Z, Liang Q, Tang X, Tan H, et al. Ginsenoside Rb1 inhibits doxorubicin-triggered H9C2 cell apoptosis via aryl hydrocarbon receptor. Biomol Ther. (2017) 25:202–12. doi: 10.4062/biomolther.2016.066
108. Hung YC, Wang PW, Lin TY, Yang PM, You JS, Pan TL. Functional redox proteomics reveal that salvia miltiorrhiza aqueous extract alleviates adriamycin-induced cardiomyopathy via inhibiting ROS-dependent apoptosis. Oxid Med Cell Longev. (2020) 2020:5136934. doi: 10.1155/2020/5136934
109. Chen R, Sun G, Yang L, Wang J, Sun X. Salvianolic acid B protects against doxorubicin induced cardiac dysfunction via inhibition of ER stress mediated cardiomyocyte apoptosis. Toxicol Res. (2016) 5:1335–45. doi: 10.1039/C6TX00111D
110. Wang L, Wang L, Zhou X, Ruan G, Yang G. Qishen yiqi dropping pills ameliorates doxorubicin-induced cardiotoxicity in mice via enhancement of cardiac angiogenesis. Med Sci Monit. (2019) 25:2435–44. doi: 10.12659/MSM.915194
111. Li YD, Ma YH, Zhao JX, Zhao XK. Protection of ultra-filtration extract from Danggui buxue decoction on oxidative damage in cardiomyocytes of neonatal rats and its mechanism. Chin J Integr Med. (2011) 17:854–9. doi: 10.1007/s11655-011-0897-6
112. Liu K, Xu RR, Sun SB, Zhu BB, Gao Y, Li YD. Effects of angelica sinensis blood tonic soup ultrafiltrate on mitochondrial apoptosis pathway in rat cardiomyocytes. Chin J Gerontol. (2016) 36:3115–8. doi: 10.3969/j.issn.1005-9202.2016.13.010
113. RR X. Protection of ultra-filtration extract from the mixture of anglica sinensis and hedysarum polybotrys on adriamycin-induced myocardial cell damage. Lanzhou: Lanzhou University (2013) 61.
114. Chen C, Liu S, Cao G, Hu Y, Wang R, Wu M, et al. Cardioprotective effect of paeonol on chronic heart failure induced by doxorubicin via regulating the miR-21-5p/S-phase kinase-associated protein 2 axis. Front Cardiovasc Med. (2022) 9:695004. doi: 10.3389/fcvm.2022.695004
115. Brosinsky P, Bornbaum J, Warga B, Schulz L, Schlüter KD, Ghigo A, et al. PI3K as mediator of apoptosis and contractile dysfunction in TGFβ(1)-stimulated cardiomyocytes. Biology. (2021) 10:670. doi: 10.3390/biology10070670
116. Wang L, Luo HM, Qiu ZD, Liu D. Protective effect and mechanism of ginseng glycoproteins on cardiotoxicity caused by adriamycin. Chin Tradit Herbal Drugs. (2021) 52:1965–73. doi: 10.7501/j.issn.0253-2670.2021.07.013
117. Yu J, Gao H, Wu C, Xu QM, Lu JJ, Chen X. Diethyl blechnic, a novel natural product isolated from salvia miltiorrhiza bunge, inhibits doxorubicin-induced apoptosis by inhibiting ROS and activating JNK1/2. Int J Mol Sci. (2018) 19:1809. doi: 10.3390/ijms19061809
118. Chen T, Shen HM, Deng ZY, Yang ZZ, Zhao RL, Wang L, et al. A herbal formula, SYKT, reverses doxorubicin-induced myelosuppression and cardiotoxicity by inhibiting ROS-mediated apoptosis. Mol Med Rep. (2017) 15:2057–66. doi: 10.3892/mmr.2017.6272
119. Chen T, Deng Z, Zhao R, Shen H, Li W. Alleviates doxorubicin-induced cardiotoxicity via modulating ROS-mediated p53 and MAPK signal pathways. Evid Based Complement Alternat Med. (2018) 2018:2581031. doi: 10.1155/2018/2581031
120. Li L, Yang D, Li J, Niu L, Chen Y, Zhao X, et al. Investigation of cardiovascular protective effect of Shenmai injection by network pharmacology and pharmacological evaluation. BMC Complement Med Ther. (2020) 20:112. doi: 10.1186/s12906-020-02905-8
121. Wang X, Sun Q, Jiang Q, Jiang Y, Zhang Y, Cao J, et al. Cryptotanshinone ameliorates doxorubicin-induced cardiotoxicity by targeting Akt-GSK-3β-mPTP pathway in vitro. Molecules. (2021) 26:1460. doi: 10.3390/molecules26051460
122. Timm KN, Tyler DJ. The role of AMPK activation for cardioprotection in doxorubicin-induced cardiotoxicity. Cardiovasc Drugs Ther. (2020) 34:255–69. doi: 10.1007/s10557-020-06941-x
123. Li L, Li J, Wang Q, Zhao X, Yang D, Niu L, et al. Shenmai injection protects against doxorubicin-induced cardiotoxicity via maintaining mitochondrial homeostasis. Front Pharmacol. (2020) 11:815. doi: 10.3389/fphar.2020.00815
124. Liu MH, Lin XL, Guo DM, Zhang Y, Yuan C, Tan TP, et al. Resveratrol protects cardiomyocytes from doxorubicin-induced apoptosis through the AMPK/P53 pathway. Mol Med Rep. (2016) 13:1281–6. doi: 10.3892/mmr.2015.4665
125. Hu C, Zhang X, Wei W, Zhang N, Wu H, Ma Z, et al. Matrine attenuates oxidative stress and cardiomyocyte apoptosis in doxorubicin-induced cardiotoxicity via maintaining AMPKα/UCP2 pathway. Acta Pharm Sin B. (2019) 9:690–701. doi: 10.1016/j.apsb.2019.03.003
126. Jin C, Chai Y, Hu Z, Tian W, Ling W, Li J, et al. Higenamine attenuates doxorubicin-induced cardiac remodeling and myocyte apoptosis by suppressing AMPK activation. Front Cell Dev Biol. (2022) 10:809996. doi: 10.3389/fcell.2022.809996
127. Pondugula SR, Harshan A, Ramesh S, Govindarajulu M, Almaghrabi M, Majrashi M, et al. Cardioprotective effects of oroxylum indicum extract against doxorubicin and cyclophosphamide-induced cardiotoxicity. Cardiovasc Toxicol. (2022) 22:67–77. doi: 10.1007/s12012-021-09701-x
128. Xing JJ, Mi XJ, Hou JG, Cai EB, Zheng SW, Wang SH, et al. Maltol mitigates cisplatin-evoked cardiotoxicity via inhibiting the PI3K/Akt signaling pathway in rodents in vivo and in vitro. Phytother Res. (2022) 36:1724–35. doi: 10.1002/ptr.7405
129. Sciarretta S, Maejima Y, Zablocki D, Sadoshima J. The role of autophagy in the heart. Annu Rev Physiol. (2018) 80:1–26. doi: 10.1146/annurev-physiol-021317-121427
130. Dikic I, Elazar Z. Mechanism and medical implications of mammalian autophagy. Nat Rev Mol Cell Biol. (2018) 19:349–64. doi: 10.1038/s41580-018-0003-4
131. Bartlett JJ, Trivedi PC, Pulinilkunnil T. Autophagic dysregulation in doxorubicin cardiomyopathy. J Mol Cell Cardiol. (2017) 104:1–8. doi: 10.1016/j.yjmcc.2017.01.007
132. Wang Y, Zhang H. Regulation of autophagy by mTOR signaling pathway. Adv Exp Med Biol. (2019) 1206:67–83. doi: 10.1007/978-981-15-0602-4_3
133. Li Y, Chen Y. AMPK and autophagy. Adv Exp Med Biol. (2019) 1206:85–108. doi: 10.1007/978-981-15-0602-4_4
134. Mao QY, Wang JK, Cao KD, Zhang YW, Gao PR, Yang XM, et al. Protective effect of danggui buxue decoction on myocardial injury induced by adriamycin in mice and its effect on autophagy of cardiomyocytes. Chin J Tradit Chin Med Pharm. (2021) 36:2515–20.
135. Wang F, Wang L, Liu F, Meng L, Zhao N, Zhai X, et al. Investigation of the mechanism of the reduction of anthracycline-induced cardiotoxicity by Qishen Huanwu Capsule based on network pharmacology. Ann Palliat Med. (2021) 10:16–28. doi: 10.21037/apm-20-2204
136. Wang F, Wang L, Jiao Y, Wang Z. Qishen Huanwu capsule reduces pirarubicin-induced cardiotoxicity in rats by activating the PI3K/Akt/mTOR pathway. Ann Palliat Med. (2020) 9:3453–61. doi: 10.21037/apm-20-1746
137. Qin Y, Lv C, Zhang X, Ruan W, Xu X, Chen C, et al. Protective effect of qiliqiangxin against doxorubicin-induced cardiomyopathy by suppressing excessive autophagy and apoptosis. Cardiovasc Ther. (2022) 2022:9926635. doi: 10.1155/2022/9926635
138. Gu J, Hu W, Song ZP, Chen YG, Zhang DD, Wang CQ. Resveratrol-induced autophagy promotes survival and attenuates doxorubicin-induced cardiotoxicity. Int Immunopharmacol. (2016) 32:1–7. doi: 10.1016/j.intimp.2016.01.002
139. Xu HD, Qin ZH. Beclin 1, Bcl-2 and autophagy. Adv Exp Med Biol. (2019) 1206:109–26. doi: 10.1007/978-981-15-0602-4_5
140. Yin J, Guo J, Zhang Q, Cui L, Zhang L, Zhang T, et al. Doxorubicin-induced mitophagy and mitochondrial damage is associated with dysregulation of the PINK1/parkin pathway. Toxicol In Vitro. (2018) 51:1–10. doi: 10.1016/j.tiv.2018.05.001
141. Schaaf MB, Keulers TG, Vooijs MA, Rouschop KM. LC3/GABARAP family proteins: autophagy-(un)related functions. FASEB J. (2016) 30:3961–78. doi: 10.1096/fj.201600698R
142. Zhang X, Lv S, Zhang W, Jia Q, Wang L, Ding Y, et al. Shenmai injection improves doxorubicin cardiotoxicity via miR-30a/Beclin 1. Biomed Pharmacother. (2021) 139:111582. doi: 10.1016/j.biopha.2021.111582
143. Wu YP, Zhang S, Xin YF, Gu LQ, Xu XZ, Zhang CD, et al. Evidences for the mechanism of Shenmai injection antagonizing doxorubicin-induced cardiotoxicity. Phytomedicine. (2021) 88:153597. doi: 10.1016/j.phymed.2021.153597
144. Xu ZM, Li CB, Liu QL, Li P, Yang H. Ginsenoside Rg1 prevents doxorubicin-induced cardiotoxicity through the inhibition of autophagy and endoplasmic reticulum stress in mice. Int J Mol Sci. (2018) 19:3658. doi: 10.3390/ijms19113658
145. Lu X, Lu L, Gao L, Wang Y, Wang W. Calycosin attenuates doxorubicin-induced cardiotoxicity via autophagy regulation in zebrafish models. Biomed Pharmacother. (2021) 137:111375. doi: 10.1016/j.biopha.2021.111375
146. Li H, Mao Y, Zhang Q, Han Q, Man Z, Zhang J, et al. Xinmailong mitigated epirubicin-induced cardiotoxicity via inhibiting autophagy. J Ethnopharmacol. (2016) 192:459–70. doi: 10.1016/j.jep.2016.08.031
147. Fu HY, Sanada S, Matsuzaki T, Liao Y, Okuda K, Yamato M, et al. Chemical endoplasmic reticulum chaperone alleviates doxorubicin-induced cardiac dysfunction. Circ Res. (2016) 118:798–809. doi: 10.1161/CIRCRESAHA.115.307604
148. Yarmohammadi F, Rezaee R, Haye AW, Karimi G. Endoplasmic reticulum stress in doxorubicin-induced cardiotoxicity may be therapeutically targeted by natural and chemical compounds: a review. Pharmacol Res. (2021) 164:105383. doi: 10.1016/j.phrs.2020.105383
149. Yuan XY. Improving effect of baoyuan decoction on myocardial injury and effects of PERK/eIF2α/CHOP signaling pathway in adriamycin indcued rats. Chin J Trad Chin Med Pharm. (2022) 37:3549–53.
150. Wu R, Wang HL, Yu HL, Cui XH, Xu MT, Xu X, et al. Doxorubicin toxicity changes myocardial energy metabolism in rats. Chem Biol Interact. (2016) 244:149–58. doi: 10.1016/j.cbi.2015.12.010
151. Yang Y, Zhang H, Li X, Yang T, Jiang Q. Effects of PPARα/PGC-1α on the myocardial energy metabolism during heart failure in the doxorubicin induced dilated cardiomyopathy in mice. Int J Clin Exp Med. (2014) 7:2435–42. Available online at: www.ijcem.com/ISSN:1940-5901/IJCEM0001739
152. Zakaria N, Khalil SR, Awad A, Khairy GM. Quercetin reverses altered energy metabolism in the heart of rats receiving adriamycin chemotherapy. Cardiovasc Toxicol. (2018) 18:109–19. doi: 10.1007/s12012-017-9420-4
153. Han Z, Guo L, Yu X, Guo H, Deng X, Yu J, et al. Network-driven targeted analysis reveals that astragali radix alleviates doxorubicin-induced cardiotoxicity by maintaining fatty acid homeostasis. J Ethnopharmacol. (2022) 287:114967. doi: 10.1016/j.jep.2022.114967
154. Qu J, Ke F, Yang X, Wang Y, Xu H, Li Q, et al. Induction of P-glycoprotein expression by dandelion in tumor and heart tissues: impact on the anti-tumor activity and cardiotoxicity of doxorubicin. Phytomedicine. (2022) 104:154275. doi: 10.1016/j.phymed.2022.154275
155. Lopaschuk GD, Ussher JR. Evolving concepts of myocardial energy metabolism: more than just fats and carbohydrates. Circ Res. (2016) 119:1173–6. doi: 10.1161/CIRCRESAHA.116.310078
156. Alexandre J, Cautela J, Ederhy S, Damaj GL, Salem JE, Barlesi F, et al. Cardiovascular toxicity related to cancer treatment: a pragmatic approach to the American and European cardio-oncology guidelines. J Am Heart Assoc. (2020) 9:e018403. doi: 10.1161/JAHA.120.018403
157. Miller KD, Siegel RL, Lin CC, Mariotto AB, Kramer JL, Rowland JH, et al. Cancer treatment and survivorship statistics, 2016. CA Cancer J Clin. (2016) 66:271–89. doi: 10.3322/caac.21349
158. Adhikari BB, Shi S, Dimond EP, Shelburne N, Desvigne-Nickens P, Minasian LM. Spectrum of national institutes of health-funded research in cardio-oncology: a basic, clinical, and observational science perspective. Heart Fail Clin. (2022) 18:515–28. doi: 10.1016/j.hfc.2022.01.001
159. Cai SQ, Shang MY, Xu F, Liu GX. Efficacy theory may help to explain characteristic advantages of traditional Chinese medicines. Chin J Chin Materia Medica. (2015) 40:3435–43.
160. Li ZM, Xu SW, Liu PQ. Salvia miltiorrhiza Burge (Danshen): a golden herbal medicine in cardiovascular therapeutics. Acta Pharmacol Sin. (2018) 39:802–24. doi: 10.1038/aps.2017.193
161. Wang X, Yang Y, Liu X, Gao X. Pharmacological properties of tanshinones, the natural products from Salvia miltiorrhiza. Adv Pharmacol. (2020) 87:43–70. doi: 10.1016/bs.apha.2019.10.001
162. Ren J, Fu L, Nile SH, Zhang J, Kai G. Salvia miltiorrhiza in treating cardiovascular diseases: a review on its pharmacological and clinical applications. Front Pharmacol. (2019) 10:753. doi: 10.3389/fphar.2019.00753
163. Tian XH, Wu JH. Tanshinone derivatives: a patent review (January 2006 - September 2012). Expert Opin Ther Pat. (2013) 23:19–29. doi: 10.1517/13543776.2013.736494
164. Bian YQ, Li J, Peng S, Lyu TY, Zhang YL, Qiao YJ. [Exploration of potential efficacy markers of Astragali Radix for invigorating Qi based on systematic traditional Chinese medicine]. Zhongguo Zhong Yao Za Zhi. (2020) 45:3266–74.
165. Zhang J, Wu C, Gao L, Du G, Qin X. Astragaloside IV derived from astragalus membranaceus: a research review on the pharmacological effects. Adv Pharmacol. (2020) 87:89–112. doi: 10.1016/bs.apha.2019.08.002
166. Liu H, Lu X, Hu Y, Fan X. Chemical constituents of Panax ginseng and Panax notoginseng explain why they differ in therapeutic efficacy. Pharmacol Res. (2020) 161:105263. doi: 10.1016/j.phrs.2020.105263
167. Wei WL, Zeng R, Gu CM, Qu Y, Huang LF. Angelica sinensis in China-a review of botanical profile, ethnopharmacology, phytochemistry and chemical analysis. J Ethnopharmacol. (2016) 190:116–41. doi: 10.1016/j.jep.2016.05.023
168. Sun JY, Qu Q, Lou YX, Hua Y, Sun GZ, Sun W, et al. Cardiotoxicity in cancer immune-checkpoint therapy: mechanisms, clinical evidence, and management strategies. Int J Cardiol. (2021) 344:170–8. doi: 10.1016/j.ijcard.2021.09.041
169. Liu Y, Wu W. Cardiovascular immune-related adverse events: evaluation, diagnosis and management. Asia Pac J Clin Oncol. (2020) 16:232–40. doi: 10.1111/ajco.13326
170. Zhao Z, Li J, Zheng B, Liang Y, Shi J, Zhang J, et al. Ameliorative effects and mechanism of crocetin in arsenic trioxide-induced cardiotoxicity in rats. Mol Med Rep. (2020) 22:5271–81. doi: 10.3892/mmr.2020.11587
171. Liang Y, Zheng B, Li J, Shi J, Chu L, Han X, et al. Crocin ameliorates arsenic trioxide-induced cardiotoxicity via Keap1-Nrf2/HO-1 pathway: reducing oxidative stress, inflammation, and apoptosis. Biomed Pharmacother. (2020) 131:110713. doi: 10.1016/j.biopha.2020.110713
172. Zheng B, Yang Y, Li J, Li J, Zuo S, Chu X, et al. Magnesium isoglycyrrhizinate alleviates arsenic trioxide-induced cardiotoxicity: contribution of Nrf2 and TLR4/NF-κB signaling pathway. Drug Des Devel Ther. (2021) 15:543–56. doi: 10.2147/DDDT.S296405
173. Piegari E, Cozzolino A, Ciuffreda LP, Cappetta D, De Angelis A, Urbanek K, et al. Cardioprotective effects of miR-34a silencing in a rat model of doxorubicin toxicity. Sci Rep. (2020) 10:12250. doi: 10.1038/s41598-020-69038-3
174. Fu Q, Pan H, Tang Y, Rong J, Zheng Z. MiR-200a-3p aggravates DOX-induced cardiotoxicity by targeting PEG3 through SIRT1/NF-κB signal pathway. Cardiovasc Toxicol. (2021) 21:302–13. doi: 10.1007/s12012-020-09620-3
175. Sun W, Xu J, Wang L, Jiang Y, Cui J, Su X, et al. Non-coding RNAs in cancer therapy-induced cardiotoxicity: mechanisms, biomarkers, and treatments. Front Cardiovasc Med. (2022) 9:946137. doi: 10.3389/fcvm.2022.946137
176. Maneechote C, Khuanjing T, Ongnok B, Arinno A, Prathumsap N, Chunchai T, et al. Promoting mitochondrial fusion in doxorubicin-induced cardiotoxicity: a novel therapeutic target for cardioprotection. Clin Sci. (2022) 136:841–60. doi: 10.1042/CS20220074
Keywords: cardio-oncology, cardiotoxicity, chemoradiotherapy, traditional Chinese medicine, pharmacology, alternative treatments
Citation: Lv X-F, Wen R-Q, Liu K, Zhao X-K, Pan C-L, Gao X, Wu X, Zhi X-D, Ren C-Z, Chen Q-L, Lu W-J, Bai T-Y and Li Y-D (2022) Role and molecular mechanism of traditional Chinese medicine in preventing cardiotoxicity associated with chemoradiotherapy. Front. Cardiovasc. Med. 9:1047700. doi: 10.3389/fcvm.2022.1047700
Received: 18 September 2022; Accepted: 20 October 2022;
Published: 07 November 2022.
Edited by:
Hongcai Shang, Beijing University of Chinese Medicine, ChinaReviewed by:
Chu Xianming, The Affiliated Hospital of Qingdao University, ChinaWeijun Kong, Capital Medical University, China
Copyright © 2022 Lv, Wen, Liu, Zhao, Pan, Gao, Wu, Zhi, Ren, Chen, Lu, Bai and Li. This is an open-access article distributed under the terms of the Creative Commons Attribution License (CC BY). The use, distribution or reproduction in other forums is permitted, provided the original author(s) and the copyright owner(s) are credited and that the original publication in this journal is cited, in accordance with accepted academic practice. No use, distribution or reproduction is permitted which does not comply with these terms.
*Correspondence: Ying-Dong Li, bHlkajQxMiYjeDAwMDQwOzE2My5jb20=