- 1Department of Physical Education and Sport Sciences, Faculty of Humanities, University of Kashan, Kashan, Iran
- 2Department of Exercise Physiology, Faculty of Sport Sciences, University of Guilan, Guilan, Iran
- 3Academic Unit of Population and Lifespan Sciences, Centre for Perinatal Research, School of Medicine, University of Nottingham, Nottingham, United Kingdom
- 4Department of Kinesiology and Nutrition Sciences, University of Nevada, Las Vegas, Las Vegas, NV, United States
Aim: We performed a systematic review and meta-analysis to investigate the effects of high-intensity interval training (HIIT) compared with moderate-intensity continuous training (MICT) or with no exercise (CON) on vascular function in adults who were free of cardiometabolic diseases and those with cardiometabolic diseases.
Methods: A search across three electronic databases including Scopus, PubMed, and Web of Science was conducted through February 2022 to identify the randomized trials evaluating HIIT vs. MICT and/or CON on vascular function as measured using brachial artery flow-mediated dilation (FMD) in adults. Separate analyses were conducted for HIIT vs. MICT and/or CON to calculate weighted mean differences (WMD) and 95% confidence intervals (95% CIs) using random or fixed models.
Results: A total of 36 studies involving 1,437 participants who were either free of cardiometabolic diseases or had cardiometabolic diseases were included in the meta-analysis. HIIT effectively increased FMD when compared with MICT [1.59% (95% CI 0.87–2.31), p = 0.001] or CON [3.80% (95% CI 2.58–5.01), p = 0.001]. Subgroup analysis showed that HIIT increased FMD in participants with cardiovascular and metabolic diseases, but not in participants who were free of cardiometabolic diseases. In addition, HIIT effectively increased FMD regardless of age and body mass index.
Conclusion: We confirm that HIIT is effective for improving vascular function in individuals with metabolic disorders and cardiovascular diseases and has a superior effect compared to MICT, demonstrating time efficiency.
Systematic review registration: [https://www.crd.york.ac.uk/prospero], identifier [CRD42022320863].
Introduction
Vascular function, determined by brachial artery flow-mediated dilation (FMD), plays a key role in circulating cardiovascular homeostasis, which is critical for cardiovascular health (1, 2). Dysfunction of vascular endothelium determines the pathogenesis of atherosclerosis and contributes to the development of clinical cardiovascular diseases (3–5). Endothelial dysfunction is characterized by an imbalance between vasodilation and vasoconstriction and promoting inflammation, oxidative stress, and reduced production of nitric oxide (NO) (2). FMD is the gold standard technique to assess vascular function and is widely used to measure endothelial health (6), and is an independent predictor of cardiovascular disease (7, 8). It is an important therapeutic and preventive target in the management of cardiovascular disease.
There is growing evidence showing the beneficial effects of exercise training in mitigating some adverse effects of cardiovascular disease, with both aerobic and strength training (9–11). These include the modulation of inflammatory markers (12–14), lipid profiles (15), visceral fat mass (16, 17), markers of glycemia (18, 19), and vascular function (20, 21) in both healthy populations and those with chronic disease. Moderate-intensity continuous training (MICT) is often recommended to improve cardiometabolic health, with current physical activity guidelines recommending a minimum 150 min of moderate-intensity (∼40–60% VO2 max) or 75 min of vigorous-intensity (∼60–85% VO2 max) physical activity per week (22–24) or longer to prevent excess weight gain or reduce body weight (25). Despite the beneficial effects of continuous training, lack of time, poor adherence, and low motivation can limit engagement. Higher intensity exercise is associated with greater benefits, although it can be difficult to maintain for some people. Enjoyment of exercise training and adherence are important for effectiveness in health care-based interventions (26, 27). As such, high-intensity interval training (HIIT) characterized by alternating short bouts of high-intensity exercise with active or passive recovery periods, has been proposed as an alternative time-efficient method of training (28, 29). Emerging evidence from systematic reviews and meta-analyses demonstrate beneficial effects of HIIT on cardiometabolic risk factors (30). As such, HIIT improves body composition (31), visceral (17), and liver (32) fat mass, some inflammatory markers (13), glycemic markers (33–35), and several chronic pain conditions (36). In addition, HIIT may be an effective approach to improve vascular function. In this regard, a 2015 meta-analysis that included seven randomized trials with 182 patients with cardiovascular and metabolic diseases, indicated a significant increase in FMD compared with MICT (37). However, the effect of HIIT on vascular function compared with non-exercise controls (CON) was not investigated. Many new studies have been published that enable the influence of health status (free of cardiometabolic diseases vs. with cardiometabolic diseases), body mass index (BMI) and age, as well as type, duration, and volume of HIIT to be examined. Therefore, the current systematic review and meta-analysis investigated the effect of HIIT on FMD as compared with either MICT or CON. In addition, we compared subgroup analyses to investigate whether the health status (free of cardiometabolic diseases, metabolic disorders, and cardiovascular diseases), BMI, age, interval types, intervention duration, and volume of intense bouts of HIIT influenced the FMD.
Methods
Trial registration
The current meta-analysis was conducted according to the 27-item PRISMA guidelines (Preferred Reporting Items for Systematic Reviews and Meta Analyses) and the Cochrane Handbook of Systematic Reviews of Interventions. The systematic review and meta-analysis was registered prospectively (ID: CRD42022320863).
Search strategy
The search was conducted across three electronic databases including Scopus, PubMed, and Web of Science. Two independent reviewers (MK and MHS) identified published articles until February 2022. Records were searched using two sets of keywords. The operator “AND” was used to link terms, and synonyms were joined with the operator “R.” The search strategy for identifying studies with HIIT included “high intensity interval training,” “high intensity interval exercise,” “high intensity intermittent exercise,” “aerobic interval training,” “aerobic interval exercise,” “interval training,” “sprint interval training,” and “sprint interval exercise.” In addition, the search strategy for identification of studies with FMD included “brachial artery,” “brachial artery dilation,” “flow mediated dilation,” “endothelial function,” “endothelium,” “artery blood flow,” “artery dilation,” “flow-mediated,” “flow mediated,” “vascular,” “vascular endothelium,” “vascular reactivity,” and “vasodilation.” There were no limitations on publication dates, but the search was limited to English language articles, human research, and article type. Search term combinations used in each database are presented in Supplementary Table 1.
Eligibility criteria and study selection
Following removal of duplicate publications, studies were screened by title and abstract and then the full texts of potentially eligible studies were reviewed by two independent reviewers (MK and MHS) and any disagreements were resolved by discussion with another author (FK). Studies were included if they met the following criteria: (1) English language articles, (2) peer-reviewed, full-text articles, (3) studies with human participants, ages ≥18 years, (4) studies that included HIIT and MICT and/or control with randomized designs, (5) studies with intervention durations of at least 2 weeks, and (6) studies that included assessments of FMD at pre- and post-intervention or change scores. HIIT refers to repeated high-intensity exercise intervals performed at 80–100% of HRpeak, interspersed with recovery periods of complete rest or light exercise (38). Sprint interval training (SIT) was included as HIIT if exercise was characterized by “all-out” or “supramaximal” efforts (≥100% of maximal work rate or VO2 max) that was interspersed with recovery periods (39). For the HIIT intervention category, HIIT and/or SIT were included. Therefore, HIIT and SIT for subgroup analysis was categorized separately as longer-interval HIIT (LI-HIIE) and SIT. For MICT, aerobic-based exercise, including low-to-moderate intensity intervals or continuous training, were included. For exercise mode, running, walking, cycling, and elliptical exercise were included. For vascular function, studies were included where vascular function was measured using FMD with units of measurement expressed as percentages (%). Exclusion criteria included non-English language publications, non-original research, research letters, conference proceedings, case reports, short reports, and reviews. The flow diagram of the systematic search process is presented in Figure 1.
Data extraction and synthesis
Data extraction was conducted by two independent authors (FK and MHS) and any disagreements were resolved through discussion with another author (MK). Data extracted from each study included the following: (a) study characteristics including first author names, year of publication, study design, and sample size, (b) participant characteristics including age, sex, BMI, and health status, (c) intervention characteristics including exercise mode and classification (HIIT, SIT, or MICT), duration, frequency, and total time of volume of exercise a week, and (d) vascular function outcome assessment methodology. For each outcome of interest, pre- and post-intervention values (means and SDs) or mean differences and associated SDs were extracted. When required, means and SDs were calculated from standard errors, 95% confidence intervals (95% CIs), medians, ranges, or interquartile ranges (40–42). In addition, when required, data extraction from figures was performed using Getdata Graph Digitizer software. In addition, to compare the total minutes of HIIT vs. MICT per week (min), weekly total exercise time was calculated, including the warm-up and cool-down time. Also, volume of intense bouts of HIIT per session (bout duration × repetitions) and week (bout duration × repetitions × sessions per week) were calculated and categorized as HIIT volume (weekly total time of ≤30 min vs. >30 min).
Quality assessment and sensitivity analyses
The methodological quality of included studies was assessed using the Physiotherapy Evidence Database (PEDro) tool. We excluded 2 items (for non-blinding of participants and intervention) from the original 11-item scale, because participants and intervention providers could not be blind to the assigned exercise conditions during studies. Therefore, study quality was assessed based on 9 items (eligibility criteria, random allocation of participants, assessed outcomes in 85% of participants, baseline comparison, allocation concealment, intention-to-treat analysis, reporting of statistical comparisons between groups, and point estimates and variability statistics). PEDro scores were determined by one reviewer (MK) and verified by another (FK) (Supplementary Table 2). Sensitivity analyses were performed by omitting each study individually to determine whether results changed.
Statistical analyses
Meta-analyses were conducted using version 2.0 of the Comprehensive Meta-analysis (CMA) software (Biostat Inc., NJ, USA). Two separate analyses were performed for comparing the effects of (1) HIIT vs. MICT, and (2) HIIT vs. CON on FMD. Analyses were conducted through weighted mean differences (WMD) and 95% CIs using fixed or random effects models. Several subgroup analyses were conducted as follows: health status (free of cardiometabolic diseases, metabolic disorders, and cardiovascular diseases), mean BMI (BMI <30 kg/m2 vs. ≥30 kg/m2), mean age (age <0 years vs. ≥50 years), interval types (HIIT vs. SIT), intervention duration (intervention <12 weeks-short-term vs. ≥12 weeks-medium-term), and volume of intense bouts of HIIT (weekly total time of ≤30 min vs. >30 min). In addition, a subgroup analysis was performed based on type of HIIT (LI-HIIT and SIT), matching of work performed for HIIE vs. MICT (matched work vs. un-matched work). Significance was set at p < 0.05. The I2 statistic was used to determine the heterogeneity, and I2 values were defined as follows: 25, 50, and 75% indicated low, moderate, and high heterogeneity, respectively. Based on I2 values, fixed models were used when I2 values were lower than 25%, and random effects models were used when I2 values were higher than 25%. Finally, publication bias was assessed using visual interpretation of funnel plots with Egger’s tests performed as secondary assessments were significant publication bias was considered at p < 0.1 (43).
Results
Included studies
Our initial search strategy revealed 454 records from Scopus, 314 records from PubMed, and 386 records from Web of Science. After eliminating duplicates and screening titles and abstracts, 129 articles were included in the full-text analysis based on inclusion and exclusion criteria. After reviewing the full-texts, 93 studies were excluded according to the reasons presented in Figure 1. Finally, 36 studies met all eligibility criteria and were included in the meta-analysis, of which 18 studies compared HIIT vs. MICT (44–61), 10 studies compared HIIT vs. CON (62–71), and 8 studies compared HIIT vs. CON and MICT (72–79). In addition, only one included study used a crossover design (57). A majority of studies used cycling and others used running, walking, jogging and elliptical training.
Participant characteristics
A total of 1437 adults were included with the range of sample sizes being 11 (57) to 200 (47). The mean age of participants ranged from 21 (65) to 75 years (79) and the mean BMI of participants ranged from 21 (65) to 37 kg/m2 (66). Both males and females were included in the majority of studies (44–48, 50–53, 55, 57–61, 64, 65, 68–77, 79), females only in five studies (49, 62, 63, 66, 67), and males only in two studies (54, 78). In the meta-analysis, participant health status varied regarding health and disease status, and included overweight and obesity, metabolic syndrome, prediabetes, type 2 diabetes, type 1 diabetes, polycystic ovarian syndrome, hypertension, heart failure, coronary artery disease, myocardial infarction, repaired tetralogy of Fallot (open heart surgery), and heart transplant. In addition, in subgroup analyses, obesity, type 2 diabetes, polycystic ovarian syndrome, type 1 diabetes, prediabetes, and the metabolic syndrome were included together as metabolic disorders (45, 48, 50, 54, 58, 59, 62, 63, 66, 67, 69, 70, 72–74, 77), and coronary artery disease, myocardial infarction, repaired tetralogy of Fallot, hypertension, heart failure, and heart transplant were included as cardiovascular diseases (44, 46, 47, 51, 52, 57, 60, 61, 68, 71, 75, 76, 79). If participants did not have any chronic disorders, they were included as free of cardiometabolic diseases (49, 53, 55, 56, 64, 65, 78) and full details of participant characteristics are shown in Table 1.
Intervention characteristics
The intervention characteristics are described in Table 1. Briefly, most studies used cycling, and treadmill walking and running exercise modalities. The most frequent protocols for included HIIT studies comprised four sets of 4-min at 80–95% HRmax or HRR interspersed with 3- or 4-min active recovery periods of low–moderate intensity. One study used two types of HIIT (45). In addition, most included studies used HIIT, whereas SIT was used in four studies (49, 53–55) where high-intensity bouts were performed at 100% of peak power output, 170% of maximal workload, or Wingate tests were performed. Also, one study used both HIIT (2 sessions per week) and SIT (1 session per week) (63). Intervention duration ranged from two (49, 50, 57, 66) to 16 weeks (77), with 12 weeks being the most common. Frequency of training ranged from 2 to 6 sessions pre week, with 3 sessions per week being the most common. Of the 26 studies that directly compared HIIT vs. MICT (44–61, 72–79), 17 studies clearly reported that HIIT protocols were matched with MICT protocols based on energy, time, duration, and/or total work performed (45, 47, 48, 50, 52, 53, 56, 57, 59, 61, 73–79). MICT protocols included MICT at 50–75% HRmax, HRR, or VO2 peck/max with durations ranging from 15 to 60 min. For CON groups, maintaining sedentary lifestyles, maintaining usual activities of daily living, nutrition advice, and light-intensity exercise with short durations were used.
Meta-analysis
High-intensity interval training vs. moderate-intensity continuous training
Based on 27 intervention arms, HIIT effectively increased FMD [1.59% (95% CI 0.87–2.31), p = 0.001] when compared with MICT (Figure 2). There was significant heterogeneity amongst included studies (I2 = 61.11%, p = 0.001). Visual interpretation of funnel plots and Egger’s test results (p = 0.19) did not suggest publication bias.
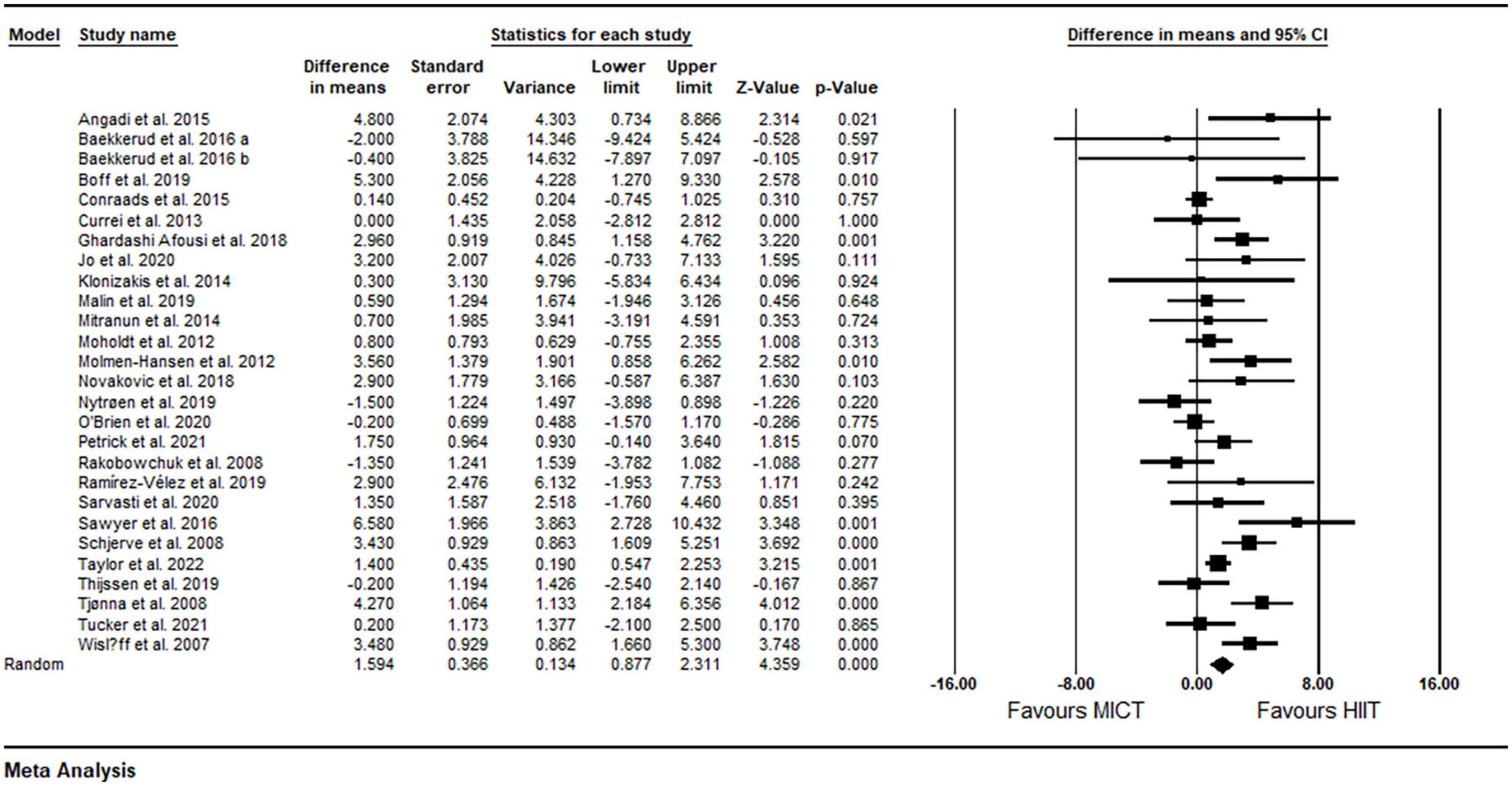
Figure 2. Forest plot of the effects of HIIT vs. MICT on FMD. Data are reported as WMD (95% confidence limits). FMD, brachial artery flow-mediated dilation; WMD, weighted mean differences.
Subgroup and sensitivity analyses
Subgroup analysis by health status revealed a significant increase in FMD in participants with metabolic disorders (WMD: 2.84%, p = 0.001) and cardiovascular disease (WMD: 1.24%, p = 0.008), but not in participants who were free of cardiometabolic diseases (WMD: −0.17%, p = 0.74) (Table 2). Subgroup analysis by participant BMI revealed a significant increase in FMD in participants with BMI <30 kg m2 (WMD: 1.24%, p = 0.001) and those with BMI ≥30 kg m2 (WMD: 2.72%, p = 0.001) (Table 2). Subgroup analysis based on age revealed a significant increase in FMD in participants aged <50 years (WMD: 1.65%, p = 0.02) and those aged ≥50 years (WMD: 1.59%, p = 0.001) (Table 2). Subgroup analysis based on intervention duration revealed a significant increase in FMD in both short-term (WMD: 1.36%, p = 0.008) and medium-term (WMD: 1.76%, p = 0.001) interventions (Table 2). Subgroup analysis based on the type of HIIT revealed a significant increase in FMD for LI-HIIT (WMD: 1.85 p = 0.001), but not for SIT (WMD: 0.16%, p = 0.80) (Table 2). Subgroup analysis based on volume of intense bouts of HIIT revealed a significant increase in FMD in both weekly total volume of ≤30 min (WMD: 1.58%, p = 0.03) and weekly total volume of >30 min (WMD: 1.62%, p = 0.001) (Table 2). In addition, subgroup analysis based on matching of work performed across protocols revealed a significant increase in FMD in both matched (WMD: 1.58%, p = 0.001) and un-matched (WMD: 1.63%, p = 0.008) protocols (Table 2). In addition, sensitivity analysis by omitting individual studies did not alter the significance or direction of overall results.
High-intensity interval training vs. CON
Based on 18 intervention arms, HIIT effectively increased FMD [3.80% (95% CI 2.58–5.01), p = 0.001] when compared with CON (Figure 3). There was significant heterogeneity amongst included studies (I2 = 79.49%, p = 0.001). Both visual interpretation of funnel plots and Egger’s test results (p = 0.004) suggested publication bias. After trim and fill correction, four studies required adjustment, with overall changes of WMD and CIs being 2.73 (95% CI 1.46–3.99).
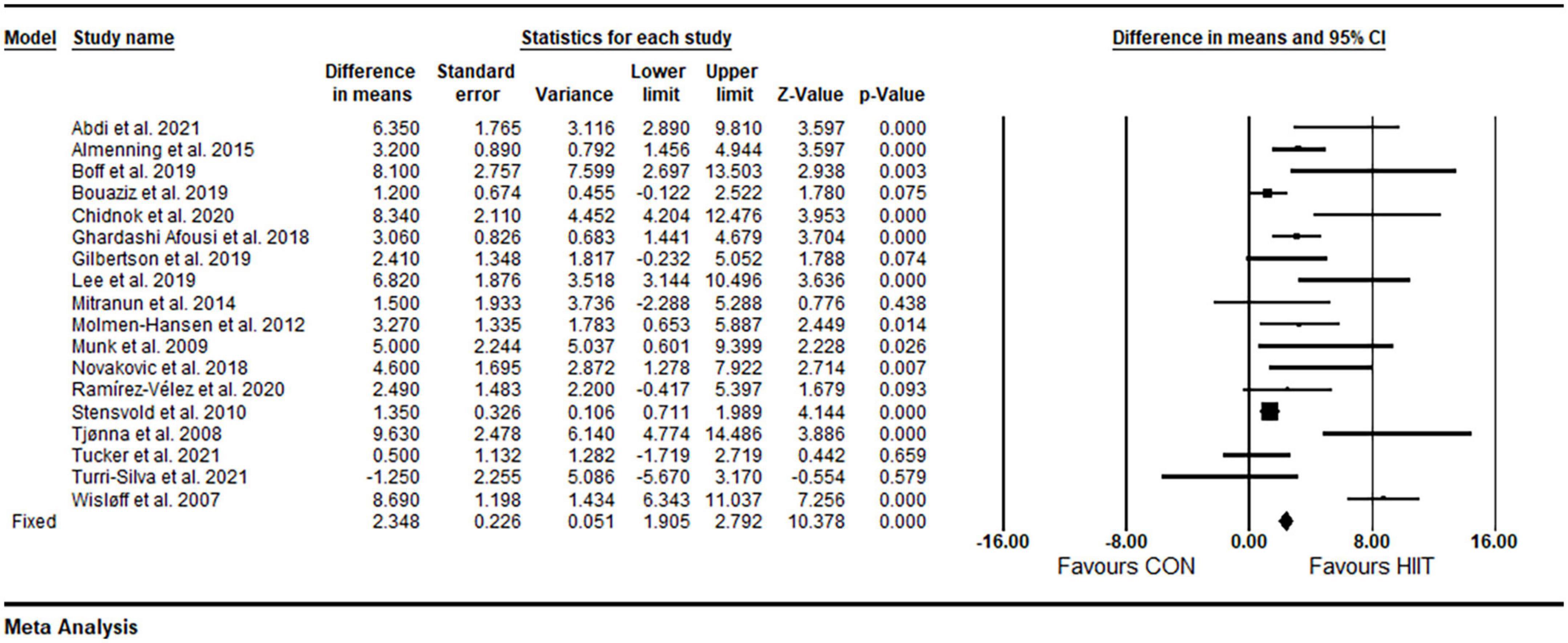
Figure 3. Forest plot of the effects of HIIT vs. CON on FMD. Data are reported as WMD (95% confidence limits). FMD, brachial artery flow-mediated dilation; WMD,: weighted mean differences.
Subgroup and sensitivity analyses
Subgroup analysis by health status revealed a significant increase in FMD in participants with metabolic disorders (WMD: 3.44%, p = 0.001) and cardiovascular diseases (WMD: 4.29%, p = 0.008), but not in participants who were free of cardiometabolic diseases (WMD: 2.73%, p = 0.09) (Table 2). Subgroup analysis by participant BMIs revealed a significant increase in FMD in participants with BMIs <30 kg m2 (WMD: 3.88%, p = 0.002) and those with BMIs ≥30 kg m2 (WMD: 3.83%, p = 0.001) (Table 2). Subgroup analysis based on age revealed a significant increase in FMD in participants ages <50 years (WMD: 3.78%, p = 0.001) and those with age ≥50 years (WMD: 3.79%, p = 0.001) (Table 2). Subgroup analysis based on intervention duration revealed a significant increase in FMD in both short-term (WMD: 3.62%, p = 0.001) and medium-term (WMD: 3.95%, p = 0.001) interventions (Table 2). Subgroup analysis based on volume of intense bouts HIIT revealed a significant increase in FMD for weekly total times of ≤30 min (WMD: 5.63%, p = 0.001) and weekly total times of >30 min (WMD: 3.24%, p = 0.001) (Table 2). Because of the small number of studies for SIT, subgroup analysis was not conducted by a type of HIIT. In addition, sensitivity analysis by omitting individual studies did not alter the significance or direction of overall results.
Quality assessment
The methodological quality of individual studies was assessed using the PEDro tool with scores ranging from 5–8 out of a maximum of 9 points. These data are summarized in Supplementary Table 2.
Discussion
Results from current systematic review and meta-analysis show that HIIT is effective for increasing FMD by 3.80%. In addition, when compared with MICT, HIIT increased FMD by 1.59% more than MICT. From a clinical perspective, these findings have important implications for the promotion of therapeutic strategies including HIIT given the efficacy and time-efficiency of exercise training type for improving vascular function.
It is well established that exercise training is associated with beneficial cardiometabolic health effects, that are mediated by improved vascular function (80). Previous systematic reviews involving healthy participants, as well as individuals with chronic diseases, indicated that exercise training including aerobic, resistance, and combined training increase FMD (81–84), with 1% change being associated with a 13% reduction in adverse cardiovascular events (7). Our finding of 3.8% increase in FMD following HIIT is thus predicted to be clinically important, and are in accord with HIIT improving brachial artery FMD by 4.31% in patients cardiovascular and metabolic diseases (37). However, the previous meta-analysis included interventions involving HIIT vs. MICT, and the HIIT effects were assessed using paired analysis (pre- and post-intervention analysis), not compared with a control group (37). This approach (within group) did not allow the appropriate evaluation of the effects of HIIT to be elucidated, especially since most of the participants were also taking medications that are known to improve vascular function (37).
The potential mechanisms underlying the increase in FMD may be explain by greater NO bioavailability, antioxidant capacity, anti-inflammatory effects, and increased abundance of endothelial progenitor cells (84, 85). Endothelial dysfunction is characterized by decreased NO bioavailability, and HIIT enhances blood flow and shear stress, thereby increasing endothelial NO synthase activity and NO quenching, leading to improvements in NO bioavailability and endothelium-dependent vasodilation (74, 86). In addition, HIIT is associated with increases in anti-inflammatory cytokines and antioxidant enzymes, reductions in pro-inflammatory cytokines and oxidative enzymes (13, 74, 87, 88), and mobilization and functionality of endothelial progenitor cells (89), which may enable improved endothelial function.
High-intensity interval training has been considered a time-efficient mode of exercise training for several physiological adaptations such as inflammation, glycemia, fat loss and weight management, with superior or similar effects, yet less overall total exercise time (13, 17, 32, 33, 35, 37, 38, 90). The second part of the current meta-analysis investigated the effect of HIIT vs. MICT showing superior effects for HIIT on FMD by 1.59%. This is consistent with previous systematic reviews and meta-analysis showing improvements in FMD of 2.26% compared with MICT. A possible mechanism for superior effects for HIIT, relative to MICT, is a combination of direct and indirect effects of HIIT on NO bioavailability and endothelial function. HIIT showed superior effects on shear stress as compared with MICT (37, 75, 79), and shear stress is strongly correlated with NO bioavailability. In addition, greater improvements in inflammatory cytokines, antioxidants status, insulin sensitivity, and lipid profiles following HIIT relative to MICT, are among the other possible mechanisms underlying the superior effects of HIIT for increasing FMD (37). Whilst not our primary research question, we examined the effects of MICT vs. CON in the trials where HIIT, MICT, and CON were all included. Meta-analysis indicated that MICT effectively increased FMD as compared with CON [1.65% (95% CI 0.13–3.17), p = 03], suggesting that, despite the greater effects of HIIT, MICT exercise can also be an effective mode of training. In addition, it should be noted that comparisons between training protocols based on energy expenditure may have resulted in risk for bias given the lack of consideration of internal training load. For example, it is possible that a greater training load occurred in the HIIT protocols in comparison to MICT (91). Therefore, when interpreting the current findings, these issues should be considered.
High-intensity interval training has been considered a potent and safe intervention for achieving beneficial health outcomes by central and peripheral adaptations in healthy populations, patients with chronic cardiometabolic disorders, and those with risk for cardiometabolic disease (92–94). However, there has been uncertainty regarding the effect HIIT may have on vascular function in healthy individuals vs. clinical populations. Ramos and colleagues reported that HIIT increased FMD in patients with metabolic disorders or cardiovascular diseases (37). The current analysis extends the previous meta-analysis (37) suggesting that HIIT, relative to both MICT and CON, increases FMD in participants with both metabolic disorders and cardiovascular diseases, but not in those who were free of cardiometabolic diseases. These observations are clinically significant, as vascular dysfunction is associated with an increased risk for cardiovascular diseases, for example heart failure or coronary artery disease. In addition, vascular dysfunction is associated with increased risk for metabolic disorders such as obesity and T2D.
The current meta-analysis suggests that HIIT is effective for increasing FMD regardless of age and BMI, when compared to both MICT and CON. Aging and obesity are associated with impaired vascular endothelial function which contributes to atherosclerosis (95–97). Several mechanisms, such as reductions in NO bioavailability, increased oxidative stress, development of low-grade inflammation, and increased activity of vasoconstrictors, are involved in vascular dysfunction that occurs with aging and obesity (95–97). Taken together, our novel findings suggest that HIIT favorably increases FMD and can be considered as a strategy for mitigating vascular dysfunction. HIIT duration and volume were also considered as an important moderator that may influence HIIT- induced adaptations (13, 30, 98, 99), but it is not clear whether these factors influence improvements in vascular function. We found that HIIT interventions of both medium and short-term duration, as well as with weekly total exercise times of ≤ and >30 min, relative to MICT and CON, are effective for increasing FMD. These results indicate that it is not necessary to engage in high volumes of HIIT to derive beneficial effects for vascular function, and further, these adaptations seem to occur rapidly once engaging in HIIT training.
As with any study, the current systematic review and meta-analysis had several limitations that should be considered when interpreting the results. There was significant heterogeneity in the results. To overcome this limitation, we performed several subgroup analyses to assess the sources of heterogeneity and found that the health status of participants may be an important contributor to differential results. Finally, we were not able to examine the effects of SIT as compared to the more common types of HIIT due to the lack of studies using SIT.
Conclusion
The current meta-analysis demonstrated that HIIT is an effective mode of exercise training for improving vascular function, particularly in those with metabolic disorders and cardiovascular diseases and is superior to MICT, suggesting HIIT is a time-efficient intervention for improving vascular function. These results hold true for low weekly volume exercise, occur relatively rapidly, and seem to be consistent across ages and BMI status.
Perspective
High-intensity interval training is an effective mode of exercise training which can improve vascular function in adults. These benefits were observed in both older adults with metabolic disorders and cardiovascular diseases. Our results highlight that HIIT is a time-efficient for improving FMD and therefore, HIIT should be considered a viable strategy for improvement of vascular function.
Data availability statement
The original contributions presented in this study are included in the article/Supplementary material, further inquiries can be directed to the corresponding author.
Author contributions
MK, MHS, FK, MES, and SR conceived and designed the study. MK, MHS, and FK carried out the screenings and reviews. MK and MHS analyzed the manuscript. MK drafted the manuscript. MES and SR revised the manuscript. All authors read and approved the final manuscript.
Funding
The publication fees for this article were supported by the UNLV University Libraries Open Article Fund.
Conflict of interest
The authors declare that the research was conducted in the absence of any commercial or financial relationships that could be construed as a potential conflict of interest.
Publisher’s note
All claims expressed in this article are solely those of the authors and do not necessarily represent those of their affiliated organizations, or those of the publisher, the editors and the reviewers. Any product that may be evaluated in this article, or claim that may be made by its manufacturer, is not guaranteed or endorsed by the publisher.
Supplementary material
The Supplementary Material for this article can be found online at: https://www.frontiersin.org/articles/10.3389/fcvm.2022.1046560/full#supplementary-material
References
1. Sandoo A, van Zanten JJ, Metsios GS, Carroll D, Kitas GD. The endothelium and its role in regulating vascular tone. Open Cardiovasc Med J. (2010) 4:302–12.
2. Sun HJ, Wu ZY, Nie XW, Bian JS. Role of endothelial dysfunction in cardiovascular diseases: the link between inflammation and hydrogen sulfide. Front Pharmacol. (2020) 10:1568. doi: 10.3389/fphar.2019.01568
3. Mudau M, Genis A, Lochner A, Strijdom H. Endothelial dysfunction: the early predictor of atherosclerosis. Cardiovasc J Afr. (2012) 23:222–31.
4. Davignon J, Ganz P. Role of endothelial dysfunction in atherosclerosis. Circulation. (2004) 109:III27–32.
5. Heitzer T, Schlinzig T, Krohn K, Meinertz T, Münzel T. Endothelial dysfunction, oxidative stress, and risk of cardiovascular events in patients with coronary artery disease. Circulation. (2001) 104:2673–8.
6. Flammer AJ, Anderson T, Celermajer DS, Creager MA, Deanfield J, Ganz P, et al. The assessment of endothelial function: from research into clinical practice. Circulation. (2012) 126:753–67.
7. Inaba Y, Chen JA, Bergmann SR. Prediction of future cardiovascular outcomes by flow-mediated vasodilatation of brachial artery: a meta-analysis. Int J Cardiovasc Imaging. (2010) 26:631–40.
8. Yeboah J, Folsom AR, Burke GL, Johnson C, Polak JF, Post W, et al. Predictive value of brachial flow-mediated dilation for incident cardiovascular events in a population-based study: the multi-ethnic study of atherosclerosis. Circulation. (2009) 120:502–9. doi: 10.1161/CIRCULATIONAHA.109.864801
9. Pedersen BK. Exercise-induced myokines and their role in chronic diseases. Brain Behav Immun. (2011) 25:811–6.
10. Pedersen BK, Saltin B. Exercise as medicine–evidence for prescribing exercise as therapy in 26 different chronic diseases. Scand J Med Sci Sports. (2015) 25(Suppl. 3):1–72. doi: 10.1111/sms.12581
11. Palermi S, Bragazzi NL, Cular D, Ardigò LP, Padulo J. How chest press-based exercises can alleviate the burden of cardiovascular diseases. Hum Mov. (2021) 22:88–98. doi: 10.5114/hm.2021.106911
12. Khalafi M, Symonds ME, Akbari A. The impact of exercise training versus caloric restriction on inflammation markers: a systemic review and meta-analysis. Crit Rev Food Sci Nutr. (2021) 62:1–16. doi: 10.1080/10408398.2021.1873732
13. Khalafi M, Symonds ME. The impact of high-intensity interval training on inflammatory markers in metabolic disorders: a meta-analysis. Scand J Med Sci Sports. (2020) 30:2020–36. doi: 10.1111/sms.13754
14. Khalafi M, Malandish A, Rosenkranz SK. The impact of exercise training on inflammatory markers in postmenopausal women: a systemic review and meta-analysis. Exp Gerontol. (2021) 150:111398. doi: 10.1016/j.exger.2021.111398
15. Halbert JA, Silagy CA, Finucane P, Withers RT, Hamdorf PA. Exercise training and blood lipids in hyperlipidemic and normolipidemic adults: a meta-analysis of randomized, controlled trials. Eur J Clin Nutr. (1999) 53:514–22. doi: 10.1038/sj.ejcn.1600784
16. Khalafi M, Malandish A, Rosenkranz SK, Ravasi AA. Effect of resistance training with and without caloric restriction on visceral fat: a systemic review and meta-analysis. Obes Rev. (2021) 22:e13275. doi: 10.1111/obr.13275
17. Maillard F, Pereira B, Boisseau N. Effect of high-intensity interval training on total, abdominal and visceral fat mass: a meta-analysis. Sports Med. (2018) 48:269–88.
18. Jorge MLMP, de Oliveira VN, Resende NM, Paraiso LF, Calixto A, Diniz AL, et al. The effects of aerobic, resistance, and combined exercise on metabolic control, inflammatory markers, adipocytokines, and muscle insulin signaling in patients with type 2 diabetes mellitus. Metabolism. (2011) 60:1244–52. doi: 10.1016/j.metabol.2011.01.006
19. Chudyk A, Petrella RJ. Effects of exercise on cardiovascular risk factors in type 2 diabetes: a meta-analysis. Diabetes Care. (2011) 34:1228–37.
20. Maiorana A, O”Driscoll G, Dembo L, Cheetham C, Goodman C, Taylor R. Effect of aerobic and resistance exercise training on vascular function in heart failure. Am J Physiol Heart Circ Physiol. (2000) 279:H1999–2005.
21. Seals DR, Nagy EE, Moreau KL. Aerobic exercise training and vascular function with ageing in healthy men and women. J Physiol. (2019) 597:4901–14.
22. American College of Sports Medicine [ACSM]. ACSM’s Guidelines for Exercise Testing and Prescription. Philadelphia, PA: Lippincott Williams & Wilkins (2013).
23. World Health Organization [WHO]. Global Recommendations on Physical Activity for Health. Geneva: World Health Organization (2010).
24. Colberg SR, Sigal RJ, Fernhall B, Regensteiner JG, Blissmer BJ, Rubin RR, et al. Exercise and type 2 diabetes: the American college of sports medicine and the American diabetes association: joint position statement. Diabetes Care. (2010) 33:e147–67.
25. Saris W, Blair SN, van Baak MA, Eaton SB, Davies PS, Di Pietro L, et al. How much physical activity is enough to prevent unhealthy weight gain? outcome of the IASO 1st stock conference and consensus statement. Obes Rev. (2003) 4:101–14. doi: 10.1046/j.1467-789x.2003.00101.x
26. Hagberg L, Lindahl B, Nyberg L, Hellénius ML. Importance of enjoyment when promoting physical exercise. Scand J Med Sci Sports. (2009) 19:740–7.
27. Collado-Mateo D, Lavín-Pérez AM, Peñacoba C, Del Coso J, Leyton-Román M, Luque-Casado A, et al. Key factors associated with adherence to physical exercise in patients with chronic diseases and older adults: an umbrella review. Int J Environ Res Public Health. (2021) 18:2023. doi: 10.3390/ijerph18042023
28. Buchheit M, Laursen PB. High-intensity interval training, solutions to the programming puzzle. Sports Med. (2013) 43:927–54.
29. Bartlett JD, Close GL, MacLaren DP, Gregson W, Drust B, Morton JP, et al. High-intensity interval running is perceived to be more enjoyable than moderate-intensity continuous exercise: implications for exercise adherence. J Sports Sci. (2011) 29:547–53. doi: 10.1080/02640414.2010.545427
30. Batacan RB, Duncan MJ, Dalbo VJ, Tucker PS, Fenning AS. Effects of high-intensity interval training on cardiometabolic health: a systematic review and meta-analysis of intervention studies. Br J Sports Med. (2017) 51:494–503. doi: 10.1136/bjsports-2015-095841
31. Wewege M, van den Berg R, Ward RE, Keech A. The effects of high-intensity interval training vs. moderate-intensity continuous training on body composition in overweight and obese adults: a systematic review and meta-analysis. Obes Rev. (2017) 18:635–46.
32. Khalafi M, Symonds ME. The impact of high intensity interval training on liver fat content in overweight or obese adults: a meta-analysis. Physiol Behav. (2021) 236:113416.
33. Khalafi M, Ravasi AA, Malandish A, Rosenkranz SK. The impact of high-intensity interval training on postprandial glucose and insulin: a systematic review and meta-analysis. Diabetes Res Clin Pract. (2022) 186:109815. doi: 10.1016/j.diabres.2022.109815
34. Jelleyman C, Yates T, O’Donovan G, Gray LJ, King JA, Khunti K, et al. The effects of high-intensity interval training on glucose regulation and insulin resistance: a meta-analysis. Obes Rev. (2015) 16:942–61. doi: 10.1111/obr.12317
35. Khalafi M, Mojtahedi S, Ostovar A, Rosenkranz SK, Korivi M. High-intensity interval exercise versus moderate-intensity continuous exercise on postprandial glucose and insulin responses: a systematic review and meta-analysis. Obes Rev. (2022) 23:e13459. doi: 10.1111/obr.13459
36. Botta RM, Palermi S, Tarantino D. High-intensity interval training for chronic pain conditions: a narrative review. J Exerc Rehabil. (2022) 18:10.
37. Ramos JS, Dalleck LC, Tjonna AE, Beetham KS, Coombes JS. The impact of high-intensity interval training versus moderate-intensity continuous training on vascular function: a systematic review and meta-analysis. Sports Med. (2015) 45:679–92. doi: 10.1007/s40279-015-0321-z
38. Costa EC, Hay JL, Kehler DS, Boreskie KF, Arora RC, Umpierre D, et al. Effects of high-intensity interval training versus moderate-intensity continuous training on blood pressure in adults with pre-to established hypertension: a systematic review and meta-analysis of randomized trials. Sports Med. (2018) 48:2127–42. doi: 10.1007/s40279-018-0944-y
39. Sultana RN, Sabag A, Keating SE, Johnson NA. The effect of low-volume high-intensity interval training on body composition and cardiorespiratory fitness: a systematic review and meta-analysis. Sports Med. (2019) 49:1687–721.
40. Wan X, Wang W, Liu J, Tong T. Estimating the sample mean and standard deviation from the sample size, median, range and/or interquartile range. BMC Med Res Methodol. (2014) 14:135. doi: 10.1186/1471-2288-14-135
41. Hozo SP, Djulbegovic B, Hozo I. Estimating the mean and variance from the median, range, and the size of a sample. BMC Med Res Methodol. (2005) 5:13. doi: 10.1186/1471-2288-5-13
42. Higgins JP, Thomas J, Chandler J, Cumpston M, Li T, Page MJ, et al. editors. Cochrane Handbook for Systematic Reviews of Interventions. New York, NY: John Wiley & Sons (2019).
43. Egger M, Davey Smith G, Schneider M, Minder C. Bias in meta-analysis detected by a simple, graphical test. BMJ. (1997) 315:629–34.
44. Angadi SS, Mookadam F, Lee CD, Tucker WJ, Haykowsky MJ, Gaesser GA. High-intensity interval training vs. moderate-intensity continuous exercise training in heart failure with preserved ejection fraction: a pilot study. J Appl Physiol. (2015) 119:753–8. doi: 10.1152/japplphysiol.00518.2014
45. Baekkerud FH, Solberg F, Leinan IM, Wisløff U, Karlsen T, Rognmo Ø. Comparison of three popular exercise modalities on VO2max in overweight and obese. Med Sci Sports Exerc. (2016) 48:491–8. doi: 10.1249/MSS.0000000000000777
46. Currie KD, Dubberley JB, McKelvie RS, MacDonald MJ. Low-volume, high-intensity interval training in patients with CAD. Med Sci Sports Exerc. (2013) 45:1436–42.
47. Conraads VM, Pattyn N, De Maeyer C, Beckers PJ, Coeckelberghs E, Cornelissen VA, et al. Aerobic interval training and continuous training equally improve aerobic exercise capacity in patients with coronary artery disease: the SAINTEX-CAD study. Int J Cardiol. (2015) 179:203–10. doi: 10.1016/j.ijcard.2014.10.155
48. Jo EA, Cho KI, Park JJ, Im DS, Choi JH, Kim BJ, et al. Effects of high-intensity interval training versus moderate-intensity continuous training on epicardial fat thickness and endothelial function in hypertensive metabolic syndrome. Metab Syndr Relat Disord. (2020) 18:96–102. doi: 10.1089/met.2018.0128
49. Klonizakis M, Moss J, Gilbert S, Broom D, Foster J, Tew GA, et al. Low-volume high-intensity interval training rapidly improves cardiopulmonary function in postmenopausal women. Menopause. (2014) 21:1099–105. doi: 10.1097/GME.0000000000000208
50. Malin SK, Gilbertson NM, Eichner NZM, Heiston E, Miller S, Weltman A, et al. Impact of short-term continuous and interval exercise training on endothelial function and glucose metabolism in prediabetes. J Diabetes Res. (2019) 2019:4912174. doi: 10.1155/2019/4912174
51. Moholdt T, Aamot IL, Granøien I, Gjerde L, Myklebust G, Walderhaug L, et al. Aerobic interval training increases peak oxygen uptake more than usual care exercise training in myocardial infarction patients: a randomized controlled study. Clin Rehabil. (2012) 26:33–44. doi: 10.1177/0269215511405229
52. Nytrøen K, Rolid K, Andreassen AK, Yardley M, Gude E, Dahle DO, et al. Effect of high-intensity interval training in de novo heart transplant recipients in Scandinavia. Circulation. (2019) 139:2198–211.
53. O’Brien MW, Johns JA, Robinson SA, Bungay A, Mekary S, Kimmerly DS, et al. Impact of high-intensity interval training, moderate-intensity continuous training, and resistance training on endothelial function in older adults. Med Sci Sports Exerc. (2020) 52:1057–67.
54. Petrick HL, King TJ, Pignanelli C, Vanderlinde TE, Cohen JN, Holloway GP, et al. Endurance and sprint training improve glycemia and (V) over dotO(2peak) but only frequent endurance benefits blood pressure and lipidemia. Med Sci Sports Exerc. (2021) 53:1194–205. doi: 10.1249/MSS.0000000000002582
55. Rakobowchuk M, Tanguay S, Burgomaster KA, Howarth KR, Gibala MJ, MacDonald MJ, et al. Sprint interval and traditional endurance training induce similar improvements in peripheral arterial stiffness and flow-mediated dilation in healthy humans. Am J Physiol Regul Integr Comp Physiol. (2008) 295:R236–42. doi: 10.1152/ajpregu.00069.2008
56. Ramírez-Vélez R, Hernández-Quiñones PA, Tordecilla-Sanders A, Álvarez C, Ramírez-Campillo R. Effectiveness of HIIT compared to moderate continuous training in improving vascular parameters in inactive adults. Lipids Health Dis. (2019) 18:42. doi: 10.1186/s12944-019-0981-z
57. Sarvasti D, Lalenoh I, Oepangat E, Purwowiyoto BS, Santoso A, Romdoni R, et al. Cardiovascular protection variables based on exercise intensity in stable coronary heart disease patients after coronary stenting: a comparative study. Vasc Health Risk Manag. (2020) 16:257–70. doi: 10.2147/VHRM.S259190
58. Sawyer BJ, Tucker WJ, Bhammar DM, Ryder JR, Sweazea KL, Gaesser GA, et al. Effects of high-intensity interval training and moderate-intensity continuous training on endothelial function and cardiometabolic risk markers in obese adults. J Appl Physiol. (2016) 121:279–88.
59. Schjerve IE, Tyldum GA, Tjønna AE, Stølen T, Loennechen JP, Hansen HE, et al. Both aerobic endurance and strength training programmes improve cardiovascular health in obese adults. Clin Sci. (2008) 115:283–93.
60. Taylor JL, Keating SE, Holland DJ, Green DJ, Coombes JS, Bailey TG, et al. Comparison of high intensity interval training with standard cardiac rehabilitation on vascular function. Scand J Med Sci Sports. (2022) 32:512–20.
61. Thijssen DHJ, Benda NMM, Kerstens TP, Seeger JPH, van Dijk APJ, Hopman MTE, et al. 12-week exercise training, independent of the type of exercise, attenuates endothelial ischaemia-reperfusion injury in heart failure patients. Front Physiol. (2019) 10:264. doi: 10.3389/fphys.2019.00264
62. Abdi S, Tadibi V, Sheikholeslami-Vatani D. Effect of high-intensity interval training on endothelial function in type 2 diabetic females. Asian J Sports Med. (2021) 12:e113566.
63. Almenning I, Rieber-Mohn A, Lundgren KM, Shetelig Løvvik T, Garnćs KK, Moholdt T, et al. Effects of high intensity interval training and strength training on metabolic, cardiovascular and hormonal outcomes in women with polycystic ovary syndrome: a pilot study. PLoS One. (2015) 10:e0138793. doi: 10.1371/journal.pone.0138793
64. Bouaziz W, Lang PO, Schmitt E, Leprêtre PM, Lefebvre F, Momas C, et al. Effects of a short-term interval aerobic training program with recovery bouts on vascular function in sedentary aged 70 or over: a randomized controlled trial. Arch Gerontol Geriatr. (2019) 82:217–25. doi: 10.1016/j.archger.2019.02.017
65. Chidnok W, Wadthaisong M, Iamsongkham P, Mheonprayoon W, Wirajalarbha W, Thitiwuthikiat P, et al. Effects of high-intensity interval training on vascular function and maximum oxygen uptake in young sedentary females. Int J Health Sci. (2020) 14:3–8.
66. Gilbertson NM, Miller SL, Eichner NZM, Malin SK. Endothelial function following interval exercise plus low-calorie diet treatment in obese females. Physiol Rep. (2019) 7:e14239. doi: 10.14814/phy2.14239
67. Lee K, Kang I, Mack WJ, Mortimer J, Sattler F, Salem G, et al. Effects of high-intensity interval training on vascular endothelial function and vascular wall thickness in breast cancer patients receiving anthracycline-based chemotherapy: a randomized pilot study. Breast Cancer Res Treat. (2019) 177:477–85. doi: 10.1007/s10549-019-05332-7
68. Munk PS, Staal EM, Butt N, Isaksen K, Larsen AI. High-intensity interval training may reduce in-stent restenosis following percutaneous coronary intervention with stent implantation a randomized controlled trial evaluating the relationship to endothelial function and inflammation. Am Heart J. (2009) 158:734–41.
69. Ramírez-Vélez R, Castro-Astudillo K, Correa-Bautista JE, González-Ruíz K, Izquierdo M, García-Hermoso A, et al. The effect of 12 weeks of different exercise training modalities or nutritional guidance on cardiometabolic risk factors, vascular parameters, and physical fitness in overweight adults: cardiometabolic high-intensity interval training-resistance training randomized controlled study. J Strength Cond Res. (2020) 34:2178–88.
70. Stensvold D, Tjønna AE, Skaug EA, Aspenes S, Stølen T, Wisløff U, et al. Strength training versus aerobic interval training to modify risk factors of metabolic syndrome. J Appl Physiol. (2010) 108:804–10. doi: 10.1152/japplphysiol.00996.2009
71. Turri-Silva N, Vale-Lira A, Verboven K, Quaglioti Durigan JL, Hansen D, Cipriano G Jr, et al. High-intensity interval training versus progressive high-intensity circuit resistance training on endothelial function and cardiorespiratory fitness in heart failure: a preliminary randomized controlled trial. PLoS One. (2021) 16:e0257607. doi: 10.1371/journal.pone.0257607
72. Boff W, da Silva AM, Farinha JB, Rodrigues-Krause J, Reischak-Oliveira A, Tschiedel B, et al. Superior effects of high-intensity interval vs. moderate-intensity continuous training on endothelial function and cardiorespiratory fitness in patients with type 1 diabetes: a randomized controlled trial. Front Physiol. (2019) 10:450. doi: 10.3389/fphys.2019.00450
73. Ghardashi Afousi A, Izadi MR, Rakhshan K, Mafi F, Biglari S, Gandomkar Bagheri H, et al. Improved brachial artery shear patterns and increased flow-mediated dilatation after low-volume high-intensity interval training in type 2 diabetes. Exp Physiol. (2018) 103:1264–76. doi: 10.1113/EP087005
74. Mitranun W, Deerochanawong C, Tanaka H, Suksom D. Continuous vs interval training on glycemic control and macro- and microvascular reactivity in type 2 diabetic patients. Scand J Med Sci Sports. (2014) 24:e69–76.
75. Molmen-Hansen HE, Stolen T, Tjonna AE, Aamot IL, Ekeberg IS, Tyldum GA, et al. Aerobic interval training reduces blood pressure and improves myocardial function in hypertensive patients. Eur J Prev Cardiol. (2012) 19:151–60.
76. Novaković M, Prokšelj K, Rajkovič U, Vižintin Cuderman T, Janša Trontelj K, Fras Z. Exercise training in adults with repaired tetralogy of fallot: a randomized controlled pilot study of continuous versus interval training. Int J Cardiol. (2018) 255:37–44. doi: 10.1016/j.ijcard.2017.12.105
77. Tjønna AE, Lee SJ, Rognmo Ø, Stølen TO, Bye A, Haram PM, et al. Aerobic interval training versus continuous moderate exercise as a treatment for the metabolic syndrome: a pilot study. Circulation. (2008) 118:346–54.
78. Tucker WJ, Jarrett CL, D’Lugos AC, Angadi SS, Gaesser GA. Effects of indulgent food snacking, with and without exercise training, on body weight, fat mass, and cardiometabolic risk markers in overweight and obese men. Physiol Rep. (2021) 9:e15118. doi: 10.14814/phy2.15118
79. Wisløff U, Støylen A, Loennechen JP, Bruvold M, Rognmo Ø, Haram PM, et al. Superior cardiovascular effect of aerobic interval training versus moderate continuous training in heart failure patients: a randomized study. Circulation. (2007) 115:3086–94. doi: 10.1161/CIRCULATIONAHA.106.675041
80. Fiuza-Luces C, Santos-Lozano A, Joyner M, Carrera-Bastos P, Picazo O, Zugaza JL, et al. Exercise benefits in cardiovascular disease: beyond attenuation of traditional risk factors. Nat Rev Cardiol. (2018) 15:731–43.
81. Qiu S, Cai X, Yin H, Sun Z, Zügel M, Steinacker JM, et al. Exercise training and endothelial function in patients with type 2 diabetes: a meta-analysis. Cardiovasc Diabetol. (2018) 17:64.
82. Pedralli ML, Eibel B, Waclawovsky G, Schaun MI, Nisa-Castro-Neto W, Umpierre D, et al. Effects of exercise training on endothelial function in individuals with hypertension: a systematic review with meta-analysis. J Am Soc Hypertens. (2018) 12:e65–75.
83. Silva JKTNF, Menêses AL, Parmenter BJ, Ritti-Dias RM, Farah BQ. Effects of resistance training on endothelial function: a systematic review and meta-analysis. Atherosclerosis. (2021) 333:91–9.
84. Ashor AW, Lara J, Siervo M, Celis-Morales C, Oggioni C, Jakovljevic DG, et al. Exercise modalities and endothelial function: a systematic review and dose–response meta-analysis of randomized controlled trials. Sports Med. (2015) 45:279–96. doi: 10.1007/s40279-014-0272-9
85. Ribeiro F, Alves AJ, Duarte JA, Oliveira J. Is exercise training an effective therapy targeting endothelial dysfunction and vascular wall inflammation? Int J Cardiol. (2010) 141:214–21.
86. Scott SN, Shepherd SO, Hopkins N, Dawson EA, Strauss JA, Wright DJ, et al. Home-hit improves muscle capillarisation and eNOS/NAD (P) Hoxidase protein ratio in obese individuals with elevated cardiovascular disease risk. J Physiol. (2019) 597:4203–25. doi: 10.1113/JP278062
87. Steckling FM, Farinha JB, Santos DL, Bresciani G, Mortari JA, Stefanello ST, et al. High intensity interval training reduces the levels of serum inflammatory cytokine on women with metabolic syndrome. Exp Clin Endocrinol Diabetes. (2016) 124:597–601.
88. Hooshmand Moghadam B, Golestani F, Bagheri R, Cheraghloo N, Eskandari M, Wong A, et al. The effects of high-intensity interval training vs. moderate-intensity continuous training on inflammatory markers, body composition, and physical fitness in overweight/obese survivors of breast cancer: a randomized controlled clinical trial. Cancers. (2021) 13:4386. doi: 10.3390/cancers13174386
89. Tsai HH, Lin CP, Lin YH, Hsu CC, Wang JS. High-intensity Interval training enhances mobilization/functionality of endothelial progenitor cells and depressed shedding of vascular endothelial cells undergoing hypoxia. Eur J Appl Physiol. (2016) 116:2375–88. doi: 10.1007/s00421-016-3490-z
90. Costigan SA, Eather N, Plotnikoff RC, Taaffe DR, Lubans DR. High-intensity interval training for improving health-related fitness in adolescents: a systematic review and meta-analysis. Br J Sports Med. (2015) 49:1253–61.
91. Iellamo F, Manzi V, Caminiti G, Vitale C, Castagna C, Massaro M, et al. Matched dose interval and continuous exercise training induce similar cardiorespiratory and metabolic adaptations in patients with heart failure. Int J Cardiol. (2013) 167:2561–5. doi: 10.1016/j.ijcard.2012.06.057
92. Kessler HS, Sisson SB, Short KR. The potential for high-intensity interval training to reduce cardiometabolic disease risk. Sports Med. (2012) 42:489–509.
93. Weston M, Taylor KL, Batterham AM, Hopkins WG. Effects of low-volume high-intensity interval training (HIT) on fitness in adults: a meta-analysis of controlled and non-controlled trials. Sports Med. (2014) 44:1005–17. doi: 10.1007/s40279-014-0180-z
94. Gibala MJ, Little JP, Macdonald MJ, Hawley JA. Physiological adaptations to low-volume, high-intensity interval training in health and disease. J Physiol. (2012) 590:1077–84.
96. El Assar M, Angulo J, Vallejo S, Peiró C, Sánchez-Ferrer CF, Rodríguez-Mañas L. Mechanisms involved in the aging-induced vascular dysfunction. Front Physiol. (2012) 3:132. doi: 10.3389/fphys.2012.00132
97. Stapleton PA, James ME, Goodwill AG, Frisbee JC. Obesity and vascular dysfunction. Pathophysiology. (2008) 15:79–89.
98. Wen D, Utesch T, Wu J, Robertson S, Liu J, Hu G, et al. Effects of different protocols of high intensity interval training for VO2max improvements in adults: a meta-analysis of randomised controlled trials. J Sci Med Sport. (2019) 22:941–7.
Keywords: high-intensity interval training, moderate-intensity continuous training, vascular function, cardiovascular diseases, metabolic disorders
Citation: Khalafi M, Sakhaei MH, Kazeminasab F, Symonds ME and Rosenkranz SK (2022) The impact of high-intensity interval training on vascular function in adults: A systematic review and meta-analysis. Front. Cardiovasc. Med. 9:1046560. doi: 10.3389/fcvm.2022.1046560
Received: 16 September 2022; Accepted: 04 November 2022;
Published: 17 November 2022.
Edited by:
Otto Alexander Sanchez, University of Minnesota Twin Cities, United StatesReviewed by:
Stefano Palermi, University of Naples Federico II, ItalyFerdinando Iellamo, University of Rome Tor Vergata, Italy
Copyright © 2022 Khalafi, Sakhaei, Kazeminasab, Symonds and Rosenkranz. This is an open-access article distributed under the terms of the Creative Commons Attribution License (CC BY). The use, distribution or reproduction in other forums is permitted, provided the original author(s) and the copyright owner(s) are credited and that the original publication in this journal is cited, in accordance with accepted academic practice. No use, distribution or reproduction is permitted which does not comply with these terms.
*Correspondence: Sara K. Rosenkranz, c2FyYS5yb3NlbmtyYW56QHVubHYuZWR1