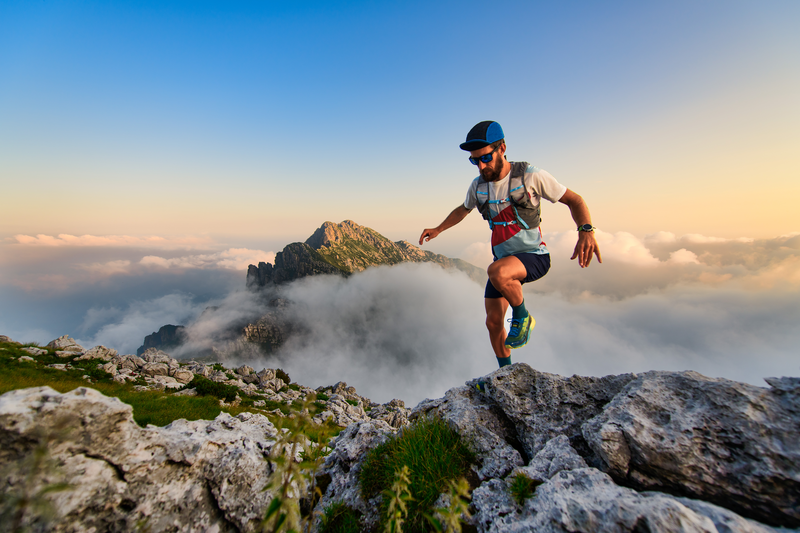
95% of researchers rate our articles as excellent or good
Learn more about the work of our research integrity team to safeguard the quality of each article we publish.
Find out more
MINI REVIEW article
Front. Cardiovasc. Med. , 24 October 2022
Sec. Thrombosis and Haemostasis
Volume 9 - 2022 | https://doi.org/10.3389/fcvm.2022.1040196
This article is part of the Research Topic COVID-19 and Thrombo-inflammatory Responses View all 12 articles
Coronavirus disease 2019 (COVID-19) is a viral respiratory infection caused by the severe acute respiratory syndrome virus (SARS-CoV-2). Vaccines that protect against SARS-CoV-2 infection have been widely employed to reduce the incidence of symptomatic and severe disease. However, adenovirus-based SARS-CoV-2 vaccines can cause a rare, thrombotic disorder termed vaccine-induced immune thrombotic thrombocytopenia (VITT). VITT often develops in the first 5 to 30 days following vaccination and is characterized by thrombocytopenia and thrombosis in unusual locations (e.g., cerebral venous sinus thrombosis). The diagnosis is confirmed by testing for anti-PF4 antibodies, as these antibodies are capable of platelet activation without any cofactor. It can be clinically challenging to differentiate VITT from a similar disorder called heparin-induced thrombocytopenia (HIT), since heparin is commonly used in hospitalized patients. VITT and HIT have similar pathobiology and clinical manifestations but important differences in testing including the need for PF4-enhanced functional assays and the poor reliability of rapid immunoassays for the detection of anti-platelet factor 4 (PF4) antibodies. In this review we summarize the epidemiology of VITT; highlight similarities and differences between HIT and VITT; and provide an update on the clinical diagnosis of VITT.
Vaccine-induced immune thrombotic thrombocytopenia (VITT) is a recently-described hematological disorder that emerged during the mass coronavirus disease 2019 (COVID-19) vaccination programs (1). The incidence of VITT ranges from 1 in 26,500 to 263,000 people after the administration of an adenoviral vector vaccine, with an overall incidence of ~1 in 100,000 (2). VITT is similar to heparin-induced thrombocytopenia (HIT), which is a side effect of heparin exposure, that is also characterized by thrombocytopenia and thrombosis (3, 4). VITT often presents with a severe clinical illness, yet the diagnosis can be missed since it is rare and clinical manifestations are non-specific. Laboratory testing is essential for VITT diagnosis and is based on similar concepts as HIT testing but with important differences. These differences include the type of screening immunoassay used and the need for specialized functional assays.
In this review we provide a pragmatic overview of VITT. We review the differences in clinical presentation between HIT and VITT; VITT pathophysiology; and unique aspects of laboratory investigations.
VITT is characterized by new-onset thrombocytopenia and thrombosis following vaccination with an adenoviral COVID-19 vaccine (1, 3). Examples of these include the ChAdOx1 nCoV-19 [AstraZeneca-Oxford] and Ad21.COV2.S [Johnson & Johnson/Janssen] vaccines. VITT patients have a medium time to symptom onset of 15 days post-vaccination (ranging from 7 to 61 days) based on a Canadian cohort of 43 patients (5). The median age of this cohort was 52 years old (ranging from 29 to 73 years old) with no apparent sex-related differences (5). Patients present with thrombocytopenia and the majority have concurrent thrombosis (85.7%) (5). Rarely, patients can present without thrombocytopenia, as occurred in 2/35 patients in the Canadian cohort. Cerebral venous sinus thrombosis (CVST) has been reported as the most common site of thrombosis (36.8%); other locations include deep-vein thrombosis of the lower extremity (23.7%); pulmonary embolism (31.6%); and splanchnic vein thrombosis (19%) (1, 3, 5, 6). Appropriate imaging based on symptom presentation (e.g., MRI venogram for severe headache) should be completed to confirm thrombosis.
A complete blood count, D-dimer, coagulation tests [international normalized ratio (INR), partial thromboplastin time (PTT)], and liver enzymes should be ordered in suspected VITT patients. The median platelet count in VITT ranges from 25,000 to 47,000 based on several reports (3, 7, 8). D-dimer levels are often markedly elevated and may worsen with disease progression (9). If the D-dimer level is <2,000 fibrinogen equivalent units (or four times the upper limit of normal), VITT is unlikely and alternate diagnoses should be considered (10). Coagulation testing (INR, aPTT, fibrinogen) are used to monitor for consumptive coagulopathy that can occur with severe or late presentations. Elevated liver enzymes (aspartate transaminase, alanine transaminase, and alkaline phosphatase) or liver function abnormalities may suggest underlying splanchnic vein thrombosis. While these initial investigations can support the diagnosis, specialized testing is ultimately required for confirmation (see Diagnostic Testing below).
A recent report described eleven VITT patients who presented to hospital with no thrombosis on initial assessment. All eleven patients had thrombocytopenia and severe headache following adenoviral vaccination (4). They also had biochemical features suggestive of VITT (thrombocytopenia, elevated D-dimer) with high anti-PF4/heparin IgG levels confirming the diagnosis. Two patients had CVST and seven did not develop thrombosis. This highlights the fact that VITT may present with isolated thrombocytopenia and, rarely, thrombosis with platelet count levels that are in the normal range. Treatment should not be delayed if clinical suspicion is high (e.g., strongly positive anti-PF4 testing with a high optical density).
A review of VITT pathobiology is essential to understand the clinical application of specialized screening and diagnostic tests. VITT is a prothrombotic, autoimmune disorder that is a result of pathologic anti-PF4 antibodies (11–13). These antibodies target the cationic protein, PF4, that is normally released from platelets during coagulation (14, 15). Anti-PF4 antibodies bind to PF4 on platelet surfaces to form immune complexes that subsequently activate platelets through the FcγRIIa receptor (16, 17). This results in significant release of intracellular molecules, including serotonin (see Serotonin Release Assay below), with resulting thrombocytopenia and thrombosis. It is postulated that monocytes and neutrophils are also activated, in a similar manner to HIT, but this has yet to be confirmed (18–20).
Similarly, HIT is mediated through anti-PF4 antibodies, but the antibody characteristics and mechanism of platelet activation are different than VITT. HIT occurs following heparin exposure and the immune complex is dependent on this hapten. Heparin, a negatively charged molecule, serves as a scaffold to bridge PF4 molecules. This facilitates anti-PF4/heparin antibody immune complex formation. Heparin binds to a unique site on the PF4 protein that has been well characterized through alanine scanning mutagenesis studies (21, 22). Anti-PF4 antibodies (VITT) bind directly to this heparin-binding site on PF4 and thus do not require heparin as a hapten. Indeed, heparin appears to inhibit VITT anti-PF4 antibody binding to PF4 in vitro since they both bind to the same region (22, 23). The trigger for anti-PF4 antibody production is unclear but several reports have speculated that the negatively charged surface of the adenovirus vector or other vaccine components bind PF4 to stimulate antibody production and activation, similar to heparin (13, 24). These distinct molecular differences, including heparin-dependence and anti-PF4 antibody specificity, have important implications for VITT testing.
Screening tests for VITT were initially adapted from HIT assays due to the similarities in pathobiology and antibody specificity. The two broad categories of testing include enzyme immunoassays (EIAs) and rapid assay (25). These immunoassays rely on antibody recognition of PF4, either alone or in complex with a negatively charged scaffold such as heparin (PF4/heparin) or polyvinylsulfate (PVS, PF4/PVS) (26–29). Rapid assays, as the name implies, offer faster turnaround time compared to EIAs and do not require sample batching. For HIT, both the EIA and rapid assays have a high sensitivity (90–99%) but low specificity (~50–70%) for “pathogenic” anti-PF4/heparin antibodies (25, 30–36). Reduced specificity is due to false positive results from patients with “non-pathogenic” anti-PF4/heparin antibodies. These “non-pathogenic” forms are unable to bind the appropriate site on PF4 required for immune complex formation and subsequent platelet activation and thus do not manifest with HIT (21, 35). Although rapid assays have similar efficacy in HIT testing, this does not hold true in the context of VITT, as will be discussed below.
EIAs quantify the optical density emitted from anti-PF4/heparin antibodies in patient serum when mixed with a secondary antibody. They consist of microtiter plates containing antigen complexes (PF4/heparin) bound to the well surface. Patient sera is added to the well and anti-PF4/heparin antibodies bind to the antigen. A secondary antibody (anti-human IgG) that can emit light is added and the degree of transmission is quantified. Commercially available EIAs for HIT detect either all immunoglobulin classes (polyspecific, i.e., IgG/A/M) or IgG-only antibodies. When used for VITT testing, commercial EIA has been reported to have a sensitivity of 100% and the specificity of commercial, in-house, and anti-P4F/heparin ranging between 95.6 and 97.4% (5). Various rapid assays are commercially available for the diagnosis of HIT including the LIA, chemiluminescence immunoassay (CLIA), particle gel immunoassay (PaGIA), and lateral flow assay (LFA). The LIA measures light transmission of a sample containing PF4/PVS-coated latex nanoparticles mixed with a HIT-mimicking antibody (30, 31). This antibody agglutinates the nanoparticles, thus resulting in low light transmission through the sample. When patient plasma containing anti-PF4/heparin antibodies is added, there is competition for binding to the nanoparticles. This disrupts the agglutination and results in higher light transmission. Therefore, the amount of patient anti-PF4/heparin antibodies is directly related to the degree of light transmission. The CLIA also utilizes PF4/PVS coated particles to capture anti-PF4/heparin antibodies and then measures the emitted light of an isoluminol-labeled anti-human IgG secondary antibody (25). Similarly, the ID-PaGIA measures the ability of patient sera to agglutinate beads coated with PF4/heparin complexes (32). Lastly, LFAs capture antibodies bound to PF4/PVS when as patient samples migrate with buffer across a test strip, appearing as a visible colored line when positive (37). Rapid assays thus represent an attractive option for HIT testing because of their ease of use, rapid turnaround time (<1 h), and superior performance compared to standard EIAs (slightly lower sensitivity, but greater specificity) (30, 33). However, rapid assays should not be used for VITT testing due to their inferior sensitivity (high false-positive rate) compared to EIA (12, 37).
Platton et al. (11) tested 23 suspected or probable VITT patients using six commercially available EIAs and four rapid assays. IgG-specific EIAs had comparable diagnostic performances to the polyspecific EIAs, with 5/6 tests showing >90% sensitivity. On the other hand, the rapid tests performed poorly in VITT: three of the four rapid assays had diagnostic sensitivities between 0 and 5.9% while the fourth had a sensitivity of 45.5% (13). Another study also found similarly poor detection of anti-PF4 antibodies in VITT patients with the following assays: ID-PaGIA (3/12, 25% detected), CLIA/HemosIL AcuStar HIT-IgG (8%), and LFA/Milenia QuickLine HIT-Test (0%) (37). Using the same samples, two commercial EIAs (ZYMUTEST HIA IgG-EIA and Immucor PF4 IgG-EIA) were shown to better identify anti-PF4 antibodies in 11/12 (92%) and 12/12 (100%) VITT patients. The superiority of EIAs was confirmed in another study of 9 VITT patients that showed reduced accuracy of rapid assays for VITT antibodies (12). All rapid assays tested in this study were negative for anti-PF4 antibodies whereas the three EIAs used showed variable results depending on the antigen target. Only the PF4-PVS EIA (Lifecodes PF4 IgG, Immucor) successfully identified high titres of anti-PF4 antibodies in 7/9 patients. The PF4/heparin EIA (Asserachrom HPIA, Stago) identified anti-PF4 antibodies in 5/9 suspected patients while the platelet-lysate/heparin EIA (Zymutest HIA IgG, Hyphen) only identified antibodies in 4/9 suspected patients. Finally, a multi-center UK study evaluating anti-PF4 EIAs and rapid assays showed rapid assays to have inferior performance compared to EIAs for identifying anti-PF4 VITT antibodies (38).
Therefore, while rapid assays are often used to rule-out the presence of anti-PF4/heparin antibodies in HIT, they perform poorly in VITT. EIAs are the preferred screening assays as they can accurately identify anti-PF4 VITT antibodies. The Lifecodes Immucor PF4-EIA has demonstrated the best performance in this regard. Reference laboratories should be able to perform more than one type of EIA, given that no single assay detects all VITT sera. Future work should look to evaluate the diagnostic capabilities of EIAs to further substantiate their usefulness as a diagnostic test for VITT due to the limited sample size of these studies. However, functional platelet activating assays remain the gold-standard test for laboratory confirmation of VITT.
Functional platelet activation assays are required to confirm the diagnosis of HIT after a positive screen for anti-PF4/heparin antibodies with the EIA or rapid assays (39). Functional assays thus reduce false positive results due to non-pathogenic antibodies (34, 40). Examples of HIT functional assays include the heparin-induced platelet aggregation assay (HIPA), heparin-induced multiple electrode aggregometry (HIMEA), PF4-dependent P-selectin expression assay (PEA), and the serotonin release assay (SRA) (39, 41–43). The HIPA, PEA and SRA rely on the use of fresh donor platelets that are mixed with patient serum, while the HIMEA is a whole blood-based assay (see Table 1). In the case of the HIPA, HIEMA and SRA, varying exogenous heparin doses are used to confirm heparin-dependence or independence. The assays mostly differ in their measured endpoints of platelet activation: HIPA measures platelet aggregation via turbidity change (assessed visually), while the HIMEA measures aggregation by electrical impedance; the PEA measures P-selectin expression on the platelet surface; and the SRA measures radioactive serotonin release from platelet granules (34, 39, 41–43, 47). These assays can also be used to identify HIT patients who may safely receive heparin products following treatment. For example, in a HIT patient treated with intravenous immunoglobulin, the SRA (functional) test will be negative despite a persistently positive EIA (48).
Functional HIT assays were quickly implemented for VITT testing once it was discovered that patients had anti-PF4 antibodies (45). However, standard functional assays often produced false-negative results in VITT. Therefore, a modification of the functional assay is needed by adding exogenous PF4 instead of heparin (1, 22, 44). In classic HIT, exogenous heparin serves as a scaffold to complex PF4 and induce a conformational change such that it can be recognized by anti-PF4/heparin HIT antibodies (40). This results in a “heparin-dependent” platelet activation, as evidenced in the SRA (39, 44). In contrast, heparin was found to reduce the sensitivity of platelet activation assays in VITT and produced a false-negative SRA. However, the addition of exogenous PF4 significantly increased the sensitivity of HIT assays and produced an activation pattern similar to HIT. The heparin-mediated inhibition (at pharmacological concentrations, i.e., 0.1 to 0.5 IU/mL heparin) of platelet activation in VITT can be explained by the recent finding that the majority of anti-PF4 VITT antibodies bind to the heparin-binding site on PF4 (22). Therefore, heparin should be avoided in VITT functional platelet testing and exogenous PF4 should be added (49). In one study, the addition of heparin to the PF4-enhanced HIPA assay reduced the sensitivity from 100 to 20% of clinically diagnosed VITT samples (5). In another study, sensitivity was reduced from 100 to 46.2% when heparin was added to the PF4-enhanced SRA (1). Interestingly, both the PEA and the PF4-induced flow cytometry–based platelet activation assay (PIFPA) already require PF4 and do not use heparin in their reactions (46). Both assays measure P-selectin expression via flow cytometry for their activation endpoints; the main differences between the PFIPA and PEA is the use of a whole blood-based assay compared to washed platelet-based assay, respectively.
PF4-enhanced functional assays can confirm whether anti-PF4 antibodies are capable of platelet activation and whether persistent anti-PF4 antibodies continue to be pathogenic. This was highlighted in a recent study of 69 patients with thrombocytopenia and thrombosis syndrome (TTS, an epidemiologic classification that includes VITT) (8). The sensitivity and specificity of functional platelet assays in this cohort were 96 and 78%, respectively. The two patients who yielded false negative platelet activation had receive intravenous immunoglobulin prior to blood collection, which is known to interfere with these assays (44). This suggests that functional assays may even approach 100% sensitivity if appropriate clinical context and pre-analytical variables are used, and with optimal assay reactivity. Rarely, PF4 supplementation may be required as demonstrated by “SRA-negative HIT” patient sera (47, 50). In one study, four of eight VITT patients (50%) with thrombocytopenia but without thrombosis also tested strongly positive for anti-PF4, platelet-activating antibodies. Functional assays can thus confirm the diagnosis in patients with atypical VITT presentation (e.g., thrombocytopenia alone on initial presentation). However, given that the excellent operating characteristics of the EIAs, functional assays are not required to confirm the diagnosis of VITT. This contrasts with HIT where the screening test has a low specificity and confirmatory functional assays are needed to confirm the diagnosis.
Functional assays can also potentially identify disease resolution in the context of persistent anti-PF4 antibodies following VITT. In a longitudinal study of 35 VITT patients, 32 (91%) continued to test positive on anti-PF4/heparin IgG EIA, albeit with significantly reduced optical density results (51). In those who had follow-up >12 weeks, the majority (14/15, 93%) became negative in the platelet-activation assays. The remaining patient exhibited recurrent thrombocytopenia with persistently high-titre anti-PF4/heparin IgG and positive platelet-activation. Therefore, platelet functional assays may be used to monitor disease activity longitudinally in patients with ongoing symptoms or evidence of disease recurrence. It is still unclear whether persistent EIA positivity indicates potential for recurrent disease.
VITT functional assays are thus a useful adjunct and diagnostic tool for patient cases that are atypical or require longitudinal monitoring. When testing well clinically defined VITT patient samples, VITT functional assays have reliable and accurate detection. Neither the PF4 serotonin release assay (PF4- SRA), the PF4-induced platelet activation assay (PIPA), or flow-cytometry based assay (PIFPA) missed any of the clinically diagnosed VITT samples, whereas the HEMA missed three samples in two different studies. Of these three samples, one was inconclusive while the other two were patients undergoing IVIG treatment (Table 1) (1, 8, 44–46). Given the relative novelty of VITT and rapidity at which these assays were developed, the majority of studies featured small, well-selected patient populations. No significant large-scale comparative studies have been done to compare the functional assays to each other as of this publication. It is because of this small sample population that the sensitivity and specificity of these tests has not been well defined; however, by all initial indications the assays appear to be strong diagnostic tools for VITT and should be considered when EIA testing is not available or additional supportive testing is required.
VITT is a prothrombotic disorder that has been characterized through application of existing knowledge related to HIT. Although these disorders share many similarities, clinicians should be aware of several important differences: First, HIT antibodies and VITT antibodies have different binding sites on PF4. Second, anti-PF4/heparin EIAs have high sensitivity and high specificity for the VITT diagnosis, whereas the specificity is low for HIT. Third, rapid diagnostic assays developed for HIT often yield false-negative results for VITT and should not be used as a diagnostic test for VITT.
SJ, DA, DM, NI, A-LB, and IN were all involved in the conceptualization, writing, and editing of the manuscript. All authors contributed to the article and approved the submitted version.
Funding was received from the Canadian Institutes of Health Research (CIHR 452655) awarded to IN.
The authors declare that the research was conducted in the absence of any commercial or financial relationships that could be construed as a potential conflict of interest.
All claims expressed in this article are solely those of the authors and do not necessarily represent those of their affiliated organizations, or those of the publisher, the editors and the reviewers. Any product that may be evaluated in this article, or claim that may be made by its manufacturer, is not guaranteed or endorsed by the publisher.
1. Greinacher A, Thiele T, Warkentin TE, Weisser K, Kyrle PA, Eichinger S. Thrombotic thrombocytopenia after ChAdOx1 nCov-19 vaccination. N Engl J Med. (2021) 384:2092–101. doi: 10.1056/NEJMoa2104840
2. Pai M. Epidemiology of VITT. Semin Hematol. (2022) 59:72–5. doi: 10.1053/j.seminhematol.2022.02.002
3. Pavord S, Scully M, Hunt BJ, Lester W, Bagot C, Craven B, et al. Clinical features of vaccine-induced immune thrombocytopenia and thrombosis. N Engl J Med. (2021) 385:1680–9. doi: 10.1056/NEJMoa2109908
4. Salih F, Schönborn L, Kohler S, Franke C, Möckel M, Dörner T, et al. Vaccine-induced thrombocytopenia with severe headache. N Engl J Med. (2021) 385:2103–5. doi: 10.1056/NEJMc2112974
5. Bissola AL, Daka M, Arnold DM, Smith JW, Moore JC, Clare R, et al. The clinical and laboratory diagnosis of vaccine-induced immune thrombotic thrombocytopenia. Blood Adv. (2022) 6:4228–35. doi: 10.1182/bloodadvances.2022007766
6. Klok FA, Pai M, Huisman MV, Makris M. Vaccine-induced immune thrombotic thrombocytopenia. Lancet Haematol. (2021) 9:E73–80. doi: 10.1016/S2352-3026(21)00306-9
7. Franchini M, Liumbruno GM, Pezzo M. COVID-19 vaccine-associated immune thrombosis and thrombocytopenia (VITT): diagnostic and therapeutic recommendations for a new syndrome. Eur J Haematol. (2021) 107:173–80. doi: 10.1111/ejh.13665
8. Thiele T, Weisser K, Schönborn L, Funk MB, Weber G, Greinacher A, et al. Laboratory confirmed vaccine-induced immune thrombotic thrombocytopenia: Retrospective analysis of reported cases after vaccination with ChAdOx-1 nCoV-19 in Germany. Lancet Reg Health - Eur. (2022) 12:100270. doi: 10.1016/j.lanepe.2021.100270
9. Favaloro EJ. Laboratory testing for suspected COVID-19 vaccine–induced (immune) thrombotic thrombocytopenia. Int J Lab Hematol. (2021) 43:559–70. doi: 10.1111/ijlh.13629
10. Greinacher A, Langer F, Makris M, Pai M, Pavord S, Tran H, et al. Vaccine-induced immune thrombotic thrombocytopenia (VITT): Update on diagnosis and management considering different resources. J Thromb Haemost. (2022) 20:149–56. doi: 10.1111/jth.15572
11. Platton S, Bartlett A, MacCallum P, Makris M, McDonald V, Singh D, et al. Evaluation of laboratory assays for anti-platelet factor 4 antibodies after ChAdOx1 nCOV-19 vaccination. J Thromb Haemost JTH. (2021) 19:2007–13. doi: 10.1111/jth.15362
12. Vayne C, Rollin J, Gruel Y, Pouplard C, Galinat H, Huet O, et al. PF4 Immunoassays in Vaccine-Induced Thrombotic Thrombocytopenia. N Engl J Med. (2021) 385:376–8. doi: 10.1056/NEJMc2106383
13. Greinacher A, Selleng K, Palankar R, Wesche J, Handtke S, Wolff M, et al. Insights in ChAdOx1 nCoV-19 vaccine-induced immune thrombotic thrombocytopenia. Blood. (2021) 138:2256–68. doi: 10.1182/blood.2021013231
14. Moore S, Pepper DS, Cash JD, Platelet antiheparin activity. The isolation and characterisation of platelet factor 4 released from thrombin-aggregated washed human platelets and its dissociation into subunits and the isolation of membrane-bound antiheparin activity. Biochim Biophys Acta. (1975) 379:370–84. doi: 10.1016/0005-2795(75)90143-9
15. Poncz M, Surrey S, LaRocco P, Weiss MJ, Rappaport EF, Conway TM, et al. Cloning and characterization of platelet factor 4 cDNA derived from a human erythroleukemic cell line. Blood. (1987) 69:219–23. doi: 10.1182/blood.V69.1.219.bloodjournal691219
16. Hughes M, Hayward CP, Warkentin TE, Horsewood P, Chorneyko KA, Kelton JG. Morphological analysis of microparticle generation in heparin-induced thrombocytopenia. Blood. (2000) 96:188–94. doi: 10.1182/blood.V96.1.188.013k12_188_194
17. Brandt S, Krauel K, Gottschalk KE, Renné T, Helm CA, Greinacher A, et al. Characterisation of the conformational changes in platelet factor 4 induced by polyanions: towards in vitro prediction of antigenicity. Thromb Haemost. (2014) 112:53–64. doi: 10.1160/TH13-08-0634
18. Perdomo J, Leung HHL, Ahmadi Z, Yan F, Chong JJH, Passam FH, et al. Neutrophil activation and NETosis are the major drivers of thrombosis in heparin-induced thrombocytopenia. Nat Commun. (2019) 10:1322. doi: 10.1038/s41467-019-09160-7
19. Tutwiler V, Madeeva D, Ahn HS, Andrianova I, Hayes V, Zheng XL, et al. Platelet transactivation by monocytes promotes thrombosis in heparin-induced thrombocytopenia. Blood. (2016) 127:464–72. doi: 10.1182/blood-2013-11-539262
20. Rauova L, Cines DB, Poncz M. Defining the role of monocytes in heparin-induced thrombocytopenia (HIT): insights from a murine HIT model. Blood. (2007) 110:280. doi: 10.1182/blood.V110.11.280.280
21. Huynh A, Arnold DM, Kelton JG, Smith JW, Horsewood P, Clare R, et al. Characterization of platelet factor 4 amino acids that bind pathogenic antibodies in heparin-induced thrombocytopenia. J Thromb Haemost JTH. (2019) 17:389–99. doi: 10.1111/jth.14369
22. Huynh A, Kelton JG, Arnold DM, Daka M, Nazy I. Antibody epitopes in vaccine-induced immune thrombotic thrombocytopaenia. Nature. (2021) 596:565–9. doi: 10.1038/s41586-021-03744-4
23. Huynh A, Arnold DM, Michael JV, Clare R, Smith JW, Daka M, et al. Characteristics of VITT antibodies in patients vaccinated with Ad26.COV2.S. Blood Adv. (2022). doi: 10.1182/bloodadvances.2022007336. [Epub ahead of print].
24. Baker AT, Boyd RJ, Sarkar D, Teijeira-Crespo A, Chan CK, Bates E, et al. ChAdOx1 interacts with CAR and PF4 with implications for thrombosis with thrombocytopenia syndrome. Sci Adv. (2021) 7:eabl8213. doi: 10.1126/sciadv.abl8213
25. Warkentin TE, Sheppard JAI, Smith JW, Li N, Moore JC, Arnold DM, et al. Combination of two complementary automated rapid assays for diagnosis of heparin-induced thrombocytopenia (HIT). J Thromb Haemost. (2020) 18:1435–46. doi: 10.1111/jth.14794
26. Visentin GP, Moghaddam M, Beery SE, McFarland JG, Aster RH. Heparin is not required for detection of antibodies associated with heparin-induced thrombocytopenia/thrombosis. J Lab Clin Med. (2001) 138:22–31. doi: 10.1067/mlc.2001.115525
27. Warkentin TE, Sheppard JI, Moore JC, Sigouin CS, Kelton JG. Quantitative interpretation of optical density measurements using PF4-dependent enzyme-immunoassays. J Thromb Haemost JTH. (2008) 6:1304–12. doi: 10.1111/j.1538-7836.2008.03025.x
28. Amiral J, Bridey F, Dreyfus M, Vissoc AM, Fressinaud E, Wolf M, et al. Platelet factor 4 complexed to heparin is the target for antibodies generated in heparin-induced thrombocytopenia. Thromb Haemost. (1992) 68:95–6. doi: 10.1055/s-0038-1656329
29. Newman PM, Chong BH. Further characterization of antibody and antigen in heparin-induced thrombocytopenia. Br J Haematol. (1999) 107:303–9. doi: 10.1046/j.1365-2141.1999.01717.x
30. Warkentin TE, Sheppard JAI, Linkins LA, Arnold DM, Nazy I. Performance characteristics of an automated latex immunoturbidimetric assay [HemosIL ® HIT-Ab (PF4-H)] for the diagnosis of immune heparin-induced thrombocytopenia. Thromb Res. (2017) 153:108–17. doi: 10.1016/j.thromres.2017.03.010
31. Refaai MA, Conley G, Ortel TL, Francis JL. Evaluation of a rapid and automated heparin-induced thrombocytopenia immunoassay. Int J Lab Hematol. (2019) 41:478–84. doi: 10.1111/ijlh.13029
32. Meyer O, Salama A, Pittet N, Schwind P. Rapid detection of heparin-induced platelet antibodies with particle gel immunoassay (ID-HPF4). Lancet. (1999) 354:1525–6. doi: 10.1016/S0140-6736(99)03625-9
33. Althaus K, Hron G, Strobel U, Abbate R, Rogolino A, Davidson S, et al. Evaluation of automated immunoassays in the diagnosis of heparin induced thrombocytopenia. Thromb Res. (2013) 131:e85–90. doi: 10.1016/j.thromres.2013.01.005
34. Warkentin TE. Laboratory diagnosis of heparin-induced thrombocytopenia. Int J Lab Hematol. (2019) 41:15–25. doi: 10.1111/ijlh.12993
35. Warkentin TE, Sheppard JAI, Horsewood P, Simpson PJ, Moore JC, Kelton JG. Impact of the patient population on the risk for heparin-induced thrombocytopenia. Blood. (2000) 96:1703–8. doi: 10.1182/blood.V96.5.1703.h8001703_1703_1708
36. Althaus K, Strobel U, Warkentin TE, Greinacher A. Combined use of the high heparin step and optical density to optimize diagnostic sensitivity and specificity of an anti-PF4/heparin enzyme-immunoassay. Thromb Res. (2011) 128:256–60. doi: 10.1016/j.thromres.2011.05.003
37. Sachs U, Hesberg J, Santoso S, Bein G, Bakchoul T. Evaluation of a new nanoparticle-based lateral-flow immunoassay for the exclusion of heparin-induced thrombocytopenia (HIT). Thromb Haemost. (2011) 106:1197–202. doi: 10.1160/TH11-06-0390
38. Reilly-Stitt C, Kitchen S, Jennings I, Horner K, Jones R, Makris M, et al. Anti-PF4 testing for vaccine-induced immune thrombocytopenia and thrombosis and heparin induced thrombocytopenia: results from a UK national external quality assessment scheme exercise april 2021. J Thromb Haemost JTH. (2021) 19:2263–7. doi: 10.1111/jth.15423
39. Sheridan D, Carter C, Kelton J. A diagnostic test for heparin-induced thrombocytopenia. Blood. (1986) 67:27–30. doi: 10.1182/blood.V67.1.27.27
40. Minet V, Dogné JM, Mullier F. Functional assays in the diagnosis of heparin-induced thrombocytopenia: a review. Molecules. (2017) 22:617. doi: 10.3390/molecules22040617
41. Galea V, Khaterchi A, Robert F, Gerotziafas G, Hatmi M, Elalamy I. Heparin-induced multiple electrode aggregometry is a promising and useful functional tool for heparin-induced thrombocytopenia diagnosis: confirmation in a prospective study. Platelets. (2013) 24:441–7. doi: 10.3109/09537104.2012.724736
42. Padmanabhan A, Jones CG, Curtis BR, Bougie DW, Sullivan MJ, Peswani N, et al. A novel PF4-dependent platelet activation assay identifies patients likely to have heparin-induced thrombocytopenia/thrombosis. Chest. (2016) 150:506–15. doi: 10.1016/j.chest.2016.02.641
43. Greinacher A, Michels I, Kiefel V, Mueller-Eckhardt C. A rapid and sensitive test for diagnosing heparin-associated thrombocytopenia. Thromb Haemost. (1991) 66:734–6. doi: 10.1055/s-0038-1646493
44. Bourguignon A, Arnold DM, Warkentin TE, Smith JW, Pannu T, Shrum JM, et al. Adjunct immune globulin for vaccine-induced immune thrombotic thrombocytopenia. N Engl J Med. (2021) 385:720–8. doi: 10.1056/NEJMoa2107051
45. Schultz NH, Sørvoll IH, Michelsen AE, Munthe LA, Lund-Johansen F, Ahlen MT, et al. Thrombosis and thrombocytopenia after ChAdOx1 nCoV-19 vaccination. N Engl J Med. (2021) 384:2124–30. doi: 10.1056/NEJMoa2104882
46. Handtke S, Wolff M, Zaninetti C, Wesche J, Schönborn L, Aurich K, et al. A flow cytometric assay to detect platelet-activating antibodies in VITT after ChAdOx1 nCov-19 vaccination. Blood. (2021) 137:3656–9. doi: 10.1182/blood.2021012064
47. Warkentin TE, Nazy I, Sheppard JAI, Smith JW, Kelton JG, Arnold DM. Serotonin-release assay-negative heparin-induced thrombocytopenia. Am J Hematol. (2020) 95:38–47. doi: 10.1002/ajh.25660
48. Warkentin TE, Climans TH, Morin PA. Intravenous Immune Globulin to Prevent Heparin-Induced Thrombocytopenia. N Engl J Med. (2018) 378:1845–8. doi: 10.1056/NEJMc1801799
49. Nazi I, Arnold DM, Warkentin TE, Smith JW, Staibano P, Kelton JG. Distinguishing between anti-platelet factor 4/heparin antibodies that can and cannot cause heparin-induced thrombocytopenia. J Thromb Haemost. (2015) 13:1900–7. doi: 10.1111/jth.13066
50. Pandya KA, Johnson EG, Davis GA, Padmanabhan A. Serotonin release assay (SRA)-negative HIT, a newly recognized entity: Implications for diagnosis and management. Thromb Res. (2018) 172:169–71. doi: 10.1016/j.thromres.2018.10.022
Keywords: COVID-19, platelet, antigen-antibody complex, immune complex, thrombosis, thrombocytopenia, heparin, vaccine
Citation: Jevtic SD, Arnold DM, Modi D, Ivetic N, Bissola A-L and Nazy I (2022) Vaccine-induced immune thrombotic thrombocytopenia: Updates in pathobiology and diagnosis. Front. Cardiovasc. Med. 9:1040196. doi: 10.3389/fcvm.2022.1040196
Received: 09 September 2022; Accepted: 04 October 2022;
Published: 24 October 2022.
Edited by:
Christoph Reinhardt, Johannes Gutenberg University Mainz, GermanyReviewed by:
Lukas Graf, Zentrum für Labormedizin (ZLM), SwitzerlandCopyright © 2022 Jevtic, Arnold, Modi, Ivetic, Bissola and Nazy. This is an open-access article distributed under the terms of the Creative Commons Attribution License (CC BY). The use, distribution or reproduction in other forums is permitted, provided the original author(s) and the copyright owner(s) are credited and that the original publication in this journal is cited, in accordance with accepted academic practice. No use, distribution or reproduction is permitted which does not comply with these terms.
*Correspondence: Ishac Nazy, bmF6eWlAbWNtYXN0ZXIuY2E=
Disclaimer: All claims expressed in this article are solely those of the authors and do not necessarily represent those of their affiliated organizations, or those of the publisher, the editors and the reviewers. Any product that may be evaluated in this article or claim that may be made by its manufacturer is not guaranteed or endorsed by the publisher.
Research integrity at Frontiers
Learn more about the work of our research integrity team to safeguard the quality of each article we publish.