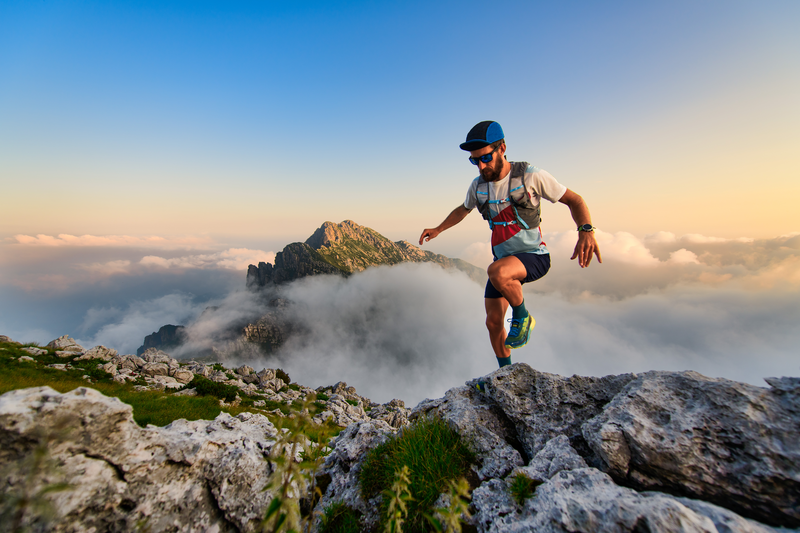
95% of researchers rate our articles as excellent or good
Learn more about the work of our research integrity team to safeguard the quality of each article we publish.
Find out more
ORIGINAL RESEARCH article
Front. Cardiovasc. Med. , 03 November 2022
Sec. Heart Failure and Transplantation
Volume 9 - 2022 | https://doi.org/10.3389/fcvm.2022.1039348
Objective: Empagliflozin (EMPA), a sodium-glucose cotransporter 2 inhibitor (SGLT2i), is recommended for all patients with Heart failure (HF) to reduce the risk of Cardiovascular death, hospitalization, and HF exacerbation. Qualitative and quantitative evaluation was conducted by searching relevant literatures of EMPA for Heart Failure from 2013 to 2022, and visual analysis in this field was conducted.
Methods: The data were from the Web of Science Core Collection database (WOSCC). The bibliometric tools, CiteSpace and VOSviewer, were used for econometric analysis to probe the evolvement of disciplines and research hotspots in the field of EMPA for Heart Failure.
Results: A total of 1461 literatures with 43861 references about EMPA for Heart Failure in the decade were extracted from WOSCC, and the number of manuscripts were on a rise. In the terms of co-authorship, USA leads the field in research maturity and exerts a crucial role in the field of EMPA for Heart Failure. Multidisciplinary research is conducive to future development. With regards to literatures, we obtained 9 hot paper, 93 highly cited literatures, and 10 co-cited references. The current research focuses on the following three aspects: EMPA improves left ventricular remodeling, exert renal protection, and increases heart rate variability.
Conclusion: Based on methods such as bibliometrics, citation analysis and knowledge graph, this study analyzed the current situation and trend of EMPA for Heart Failure, sorted out the knowledge context in this field, and provided reference for current and future prevention and scientific research.
Empagliflozin (EMPA), a sodium-glucose cotransporter 2 inhibitor (SGLT2i), is recommended for all patients with Heart failure (HF) who have been treated with ACE-I/ARNI, β-blockers, and MRA, regardless of diabetes, to reduce the risk of Cardiovascular (CV) death, hospitalization, and HF exacerbation in HF patients (1–4). The EMPEROR-Reduced trial found that EMPA reduced the composite primary end point of CV death or HF hospitalization by 25% in patients with NYHA class II-IV symptoms (1) and reduced eGFR decline in individuals. Similarly, EMPA reduced the combined risk of CV death or hospitalization due to HF with a preserved ejection fraction, regardless of diabetes (4). It is also associated with improved quality of life (5, 6). The natriuretic/diuretic properties of EMPA may provide additional benefits regarding reduce congestion and the need for circulatory diuresis (7, 8). In patients with HF with reduced ejection fraction (EF), EMPA reduced the risk and total number of inpatient and outpatient exacerbations of HF events, ameliorate renal endpoints (9), and decrease CV and all-cause death (10), with benefits seen early after treatment initiation and sustained for the duration of double-blind therapy (11).
Changes in cardiac metabolism and ion homeostasis are precursors and drivers of cardiac remodeling and the development of HF. EMPA exerts direct and acute effects on cardiac ion homeostasis by inhibiting the cardiac Na+/H+ exchanger (NHE) and reducing intracellular Na+ and Ca2+ (12, 13). Subsequent studies showed for the first time that EMPA had acute specific metabolic effects in isolated type II diabetic db/db mouse hearts, namely, decreased lactate production of labeled glucose and increased α-ketoglutarate synthesis of labeled palmitate, which appeared to be mediated by NHE-1 inhibition (14).
In addition to cardiovascular benefits, EMPA slowed the estimated rate of decline in GFR in double-blind treatment, and the risk of a composite renal outcome was lower in the EMPA group than in the placebo group. Comparing measurements taken at the beginning and end of the trial after discontinuation of EMPA and placebo, the estimated decrease in GFR was greater in the placebo group than in the EMPA group. These observations are consistent with the benefits observed with SGLT2 inhibitors in type 2 diabetic patients who are largely free of HF. Thus, the positive effect of EMPA on renal function in patients with diabetes, HF, and patients with both conditions is evident (1).
Bibliometrics analysis can systematically deal with the original manuscripts of relevant research fields, track current research hotspots and general trends, reveal landmark literatures in this field and obtain clearer and more intuitive results (15–17). CiteSpace and VOSviewer, series of user-friendly bibliometrics analysis software, make the analyzer easy to operate. In this paper, using literature metrology method combing these documents, determine the influential countries, institutions, scholars, journals, and landmark theory, is to summarize the basic situation, provide references for study in the field of EMPA for HF.
The following category was used to retrieve literature from WOSCC: (Topic = Empagliflozin and Heart Failure). There were no language, publication date, and document type restrictions in the selection of the articles. To avoid data bias, the plain text files containing full record and cited references were exported in the text as download_***.txt in under a day (August 10, 2022). In ClinicalTrials.gov1, a database of privately and publicly funded clinical studies conducted around the world, we performed a combination search of “Empagliflozin” and “Heart Failure” for clinical trials focused on intervention therapy. Then the retrieval results were analyzed comprehensively according to the stage of the experiment.
Figure 1A shows a flowchart of the scientometric analysis. We used the WOSCC database for most of the analyses including Publications, Citations, Web of Science Categories, Journal, Country/Region, Affiliations, Authors, hot papers, and highly cited literatures.
Figure 1. (A) The flow diagram of scientometric analysis. (B) The trend of Publications and Citations. (C) The document types of all literatures. (D) Global distribution of clinical trials about EMPA for HF (https://clinicaltrials.gov/ct2/home).
VOSviewer (Version 1.6.17) was utilized for the analysis of countries, organizations, and authors. Based on network visualization, VOSviewer divides countries, organizations, or authors into diverse clusters and colors them in the light of the temporal progression of their occurrence, superimposing time on the network of co-occurrence countries, organizations, or authors. The data about country cooperation was converted into GML format by VOSviewer and imported into Scimago Graphica Beta software (Version 1.0.18) to demonstrate geographical distribution and country cluster.
The software CiteSpace (Version 5.8.R3 64-bit) was used for the analysis of co-cited reference and keywords. In the co-occurrence graph, the circle size represents the number of co-citations, and the centrality and burstiness was strikingly marked by the purple and red in the outer ring of the node, respectively. Key points in CiteSpace are nodes with a centrality of more than 0.1. Long-likelihood ration algorithm was used for keywords cluster analysis based on co-occurrence. In the cluster map, the ones surrounded by boxed represent the same cluster. Cluster names are displayed based on the cluster scale.
It can be seen from Figure 1B that in the decade, the amount of literature published in the field of EMPA for HF showed a rise and peaked in 2021. At present, just 8 months into 2022, the analysis of posts is not included yet. This citation analysis of the 1461 papers on EMPA for HF resulted in a grand total of 43861 times cited in the past 10 years, with a mean of 30.02 citations per paper and h-index of 84. H-index is an important indicator of a researchers’ scientific output (18). The times of citations increased year by year from 1 in 2013 to 14754 in 2021 (Based on the uptrend, citations are likely to continue to break through in 2022). The initial database search yielded 1461 articles, which comprise nine different document types (Figure 1C) including Articles (724 papers, 49.555%), Review Articles (450 papers, 30.801%), Meeting Abstracts (133 papers, 9.103%), Editorial Materials (100 papers, 6.845%), Early Access (47 papers, 3.217%), etc.
To date, EMPA has 42 clinical trials for HF worldwide and the exact distribution is shown in Figure 1D. Of the 42 trials, 21 have been completed, 11 are recruiting patients, 7 have not yet started recruitment, 2 have been terminated by slow enrollment (ClinicalTrials.gov Identifier: NCT03152552) and outbreak involvement (ClinicalTrials.gov Identifier: NCT03554200), and 1 has unknown information (ClinicalTrials.gov Identifier: NCT03271879). Of the 21 completed trials, 10 submitted final findings and all of them had positive effects, as detailed in Table 1. The number of enrolled patients ranged from 23 to 5988, all of whom were over 18 years old and of both sexes. EMPA was used in two specifications: 10 and 25 mg, with 10 mg being the majority and placebo being used as the control drug. The main outcome measures were NT-proBNP, Left Ventricle-End Systolic/Diastolic Volume (LV-EDV/ESV), 6-Min-Walking-Test (6MWT), Kansas City Cardiomyopathy Questionnaire (KCCQ) score, CV death, hospitalization rate, all-cause mortality, and renal function related indicators. The KCCQ, a self-administered questionnaire designed to evaluate physical limitations, symptoms (frequency, severity, and changes over time), social limitations, self-efficacy, and quality of life in patients with HF, incorporates the following domains: symptom burden, symptom frequency, and physical limitation (19). The KCCQ questionnaire has 23 items and 12 items. The KCCQ-12 is a shorter version of the original 23-item test tool, and its credibility has been verified in clinical trials (20).
A series of clinical trials have demonstrated that EMPA improves hemodynamics in patients with HF, rapidly reducing pulmonary artery pressure that is amplified over time and appears to be independent of loop diuresis management (21–23). In the EMPA-REG OUTCOME trial, EMPA reduced CV risk events and influenced mortality and hospitalization in patients with HF independent of its hypoglycemic effect. In addition, the effect of EMPA on exercise capacity in patients with HF did not differ according to LVEF (24–28). In the EMPULSE trial (29), the initiation of EMPA in hospitalized patients with acute HF produced clinical benefits, improved KCCQ scores regardless of the extent of symptom impairment at baseline, and improved symptoms, physical limitations, and quality of life, with benefits seen as early as 15 days and maintained up to 90 days. EMPA resulted in significant reductions in body weight and serum urate, and when combined with loop diuretics, a significant increase in 24-h urine volume but no increase in urinary sodium. It is hypothesized that EMPA’s role as an osmotic diuretic and its effect on natriuretic may underlie the CV and renal benefits (30, 31).
Table 2 shows the top 15 disciplines. The top three subjects covered by all included literature are Cardiac Cardiovascular Systems (713 papers, 48.802%), Endocrinology Metabolism (352 papers, 24.093%), and Pharmacology Pharmacy (175 papers, 11.978%). The remaining subjects are in order Medicine General Internal (141 papers, 9.651%), Peripheral Vascular Disease (134 papers, 9.172%), Urology Nephrology (54 papers, 3.696%), Medicine Research Experimental (44 papers, 3.012%), Biochemistry Molecular Biology (38 papers, 2.601%), Cell Biology (18 papers, 1.232%), Multidisciplinary Sciences (17 papers, 1.164%), Health Care Sciences Services (16 papers, 1.095%), Toxicology (14 papers, 0.958%), Chemistry Multidisciplinary (12 papers, 0.821%), Chemistry Medicinal (10 papers, 0.684%), and Geriatrics Gerontology (10 papers, 0.684%).
Regarding the number of published papers, Circulation is the most published journal with 86 articles, with IF of 39.918 and JCR of Q1, followed by Cardiovascular Diabetology (71 articles, IF2021 = 8.949, Q1), European Journal Of Heart Failure (67 articles, IF2021 = 17.349, Q1), Diabetes Obesity Metabolism (54 articles, IF2021 = 6.408, Q1), European Heart Journal (39 articles, IF2021 = 35.855, Q1), Journal Of The American College Of Cardiology (35 articles, IF2021 = 27.203, Q1), Esc Heart Failure (31 articles, IF2021 = 3.612, Q2), Diabetologia (27 articles, IF2021 = 10.46, Q1), Heart Failure Reviews (25 articles, IF2021 = 4.654, Q2) and New England Journal Of Medicine (24 articles, IF2021 = 176.079, Q1) (Table 3).
In terms of publication numbers, USA is the country with the highest number of publications, accounting for 37 percent of the total, followed by the Germany (286 papers, 19.576%), Canada (213 papers, 14.579%), England (208 papers, 14.237%), Japan (135 papers, 9.24%), China (133 papers, 9.103%), Italy (121 papers, 8.282%), Greece (114 papers, 7.803%), France (110 papers, 7.529%), and Netherlands (107 papers, 7.324%) (Table 4 and Figure 2A). Figure 2B shows geographical distribution of all contributing countries. Of these top 15 countries/regions, ten from Europe, two are from Asia, two from North America and one from Oceania. The total citations, total link strength and h-index ranked first in the USA, which were 28,404, 1,194, and 70 respectively, suggesting that the USA is relatively mature in the field of EMPA for HF. As shown in Figure 2C, 51 countries met the threshold, and 3 clusters were generated, among which the largest clusters were 21 countries, which are represented by red, green, and blue. In Figure 2D, the more purple the color, the earlier the study, and the redder the later the study. It shows that the USA, Canada, and Germany were studied earlier. China, Mexico, and Turkey appear later, around 2021.
Figure 2. The analysis of country/region. (A) The destiny of all contributing countries. (B) The cluster of all contributing countries by Scimago. (C) The occurrence of all contributing countries. (D) The time overlay of all contributing countries.
The three institutions with the largest number of publications were from University of Toronto, Boehringer Ingelheim and Saint Michaels Hospital Toronto, accounting for 11.567, 10.678, and 8.966% of the total reports respectively, will in Table 5 and Figure 3A. Six of the top 15 published research institutions are in the USA, five are in Germany, while two are in Canada. University of Toronto is the research institution with the most total citations, with an API of 134.19 and an h-index of 44, followed by Harvard University and Saint Michaels Hospital Toronto. Figure 3B shows the connections between institutions. Nodes represent the number of publications issued by institutions, and lines represent the connections between institutions. Three clusters are formed with red, blue, and green respectively. As can be seen from the time superposition chart of institutions (Figure 3C), the study of University of Toronto started earlier, while Charite Universitatsmedizin Berlin appeared later, around the end of 2020.
Figure 3. The analysis of institutions and authors. (A) The destiny of all contributing institutions. (B) The occurrence of all contributing institutions. (C) The time overlay of all contributing institutions. (D) The destiny of all contributing authors. (E) The occurrence of all contributing authors. (F) The time overlay of all contributing authors.
With regards to authors, Butler J from the University of Mississippi was the most prolific, followed by Anker SD from Charite Universitatsmedizin Berlin and Inzucchi SE from Yale University. Of the top 15 authors, eight are from the USA and Germany, evenly split. Inzucchi SE from Yale University have the most citations of 1,0007 and the highest h-index of 26 on the field of EMPA for HF (Table 6 and Figure 3D). Figure 3E shows the connections between authors. Nodes represent the number of publications issued by authors, and lines represent the connections between authors. Three clusters are formed with red, blue, and green respectively. The clustering formed by Zinman B, Fitchett D, and Mcguire DK appeared earliest. Since then, many scholars have conducted in-depth research (Figure 3F).
Hot papers were those published in the past 2 years and received enough citations in November/December 2021 to be in the top 0.1% of papers in an academic field in a subject area. There were nine hot papers (1, 4, 10, 32–37) in total, and all of them were highly cited (Table 7). The types of documents are all articles, and all of them are published in the journal with JCR of Q1, most of which were New England Journal of Medicine (IF2021 = 176.079, Q1). The above nine hot papers included two meta-analyses, and the results showed that SGLT2 inhibitors were associated with a reduced risk of major adverse CV events and renal outcomes (36), as well as a reduced combined risk of CV death or hospitalization due to HF in patients with or without diabetes (4). The results were confirmed in another study (10). Three other trials (1, 32, 35), all of which looked at the effects of SGLT2i (EMPA, dapagliflozin, and sotagliflozin) on the kidneys, were also positive.
As of November/December 2021, these highly cited literatures received enough citations to place it in the top 1% of the academic field based on a highly cited threshold for the field and publication year. A total of 93 highly cited literatures were detected in the database in this research field, with the highest citation frequency of 4753. Among them, the above nine hot papers were all among the highly cited articles. Therefore, the top 15 highly cited literatures excluding the hot papers are shown in Table 8. With regards to the document type, 12 were articles and 3 were review. The publication time of highly cited literatures was generally from 2015 to 2019. Among the top 15 highly cited literatures, only one described the underlying mechanism of EMPA in the treatment of HF, involving in vivo experiments in animals (12). Elevated cardiac cytoplasmic Na+([Na+]c) and Ca2+([Ca2+]c) concentrations and decreased mitochondrial Ca2+([Ca2+]m) concentrations are driving factors for HF and cardiac death (38, 39). Previous studies (40, 41) have shown that chronic NHE inhibition prevents or attenuates HF in animal models. Ventricular myocytes of rabbits and rats were acutely isolated, and the activities of [Na+]c, [Ca2+]c, [Ca2+]m and NHE were measured by fluorimetric assay. The results showed that EMPA had a direct effect on the heart by injuring myocardial NHE flux, reducing myocardial [Na+]c and [Ca2+]c, and enhancing [Ca2+]m, independent of SGLT2 activity (12). Thus, it is hypothesized that the beneficial CV effects of EMPA are at least partially attributable to NHE inhibition, and it is appropriate to complement the mechanistic studies of EMPA in the treatment of HF.
The analysis of co-cited references reveals that there are two references in the list of the third citation article, and then the two references form a co-citation relationship. Figures 4A–D respectively represents the citations, centrality, and burst intensity of co-cited literature. The blue line in the figure represents the time axis of the existence of included literatures, and the red line represents the period of the strongest emergence of corresponding literatures. In the co-occurrence diagram of co-cited literatures, the line between nodes means that two literatures appear in the reference of the same article. The more the line is, the articles have certain similarity in content. Nodes represent the citations of literature, purple outside nodes represents the centrality of literature, and red represents the intensity of highlighting. According to the intensity of burst, the top 10 literatures are shown in Table 9.
Figure 4. The analysis of co-cited references. (A) The occurrence of co-cited references by citations. (B) The occurrence of co-cited references by centrality. (C) The occurrence of co-cited references by burst intensity. (D) The emergence of co-cited references.
The first article named “Empagliflozin, Cardiovascular Outcomes, and Mortality in Type 2 Diabetes,” which written by Zinman B and published in the New England Journal of Medicine (IF2021 = 176.079) in 2015, is the first study to provide evidence that antidiabetic agents reduce CV events (42). In the EMPA-REG OUTCOME trials, a total of 7020 patients with T2DM were randomized to receive either 10 or 25 mg of EMPA daily or placebo. Compared with placebo, EMPA significantly reduced the risk of death from CV causes, non-fatal myocardial infarction, or non-fatal stroke. The results were surprising; In contrast to other CV risk reducing interventions, such as lowering LDL-C (43) and Blood Pressure (44), CV death was significantly reduced by 38% (P = 0.001). The separation of Kaplan–Meier curves for CV mortality and HF hospitalization observed during the first 3 months of the trial suggests that the treatment mechanism for EMPA has an early and profound effect on the risk of death or HF (42). Another article titled “Heart Failure Outcomes with Empagliflozin in patients with Type 2 Diabetes at High Cardiovascular Risk: Results of the EMPA-Reg OUTCOME® Trial,” written by Fitchett D, published in the European Heart Journal (IF2021 = 35.855) in 2016, highlighting the strength 13.9, also reported these findings from the same randomized controlled trial (ClinicalTrials.gov Identifier: NCT01131676).
Our analysis of the co-occurrence diagram was used to see which were the main directions and hotspots of EMPA for HF research, as well as to understand how its themes and topics developed and changed over time. The keyword co-occurrence diagram of 386 nodes and 768 links was obtained by running CiteSpace (Figure 5A). The node size represents the frequency of keywords. The centrality of keywords was calculated by “Node-compute Node Centrality” in CiteSpace, and the top 10 keywords in frequency and centrality were summarized.
Figure 5. Keywords analysis and visualization. (A) The occurrence of keywords. (B) The cluster of keywords.
As previous studies (45), based on keyword co-occurrence graph, the keyword clustering analysis was carried out (Figure 5B). The evaluation diagram was mainly judged from the clustering effect and reliability. Modularity Q = 0.7799, the closer Q value was to 1, the better the clustering result of the network was, and Q value > 0.3 indicated that the clustering structure was significant. Silhouette S = 0.91, which measured network homogeneity. When Silhouette was more closely to 1, the more homogenous the Silhouette would be. An S value > 0.5 was considered reasonable and an S value > 0.7 was considered convincing (46). The cluster information is exported in “Summary Table/Whitelists” on the cluster menu bar of CiteSpace, and the key information was displayed in Table 10. A total of 16 clusters were formed in this study. The smaller the number, the larger the cluster size was. #0 sglt2 inhibitor was the largest cluster, followed by #1 lv remodeling, #2 diabetes mellitus, #3 cardiorenal syndrome, #4 chronic heart failure, #5 clinical trial, #6 cardiac function, #7 heart failure, #8 dpp-4 inhibitors, #9 mellitus, #10 preserved ejection fraction, #11 empagliflozin, #12 sodium-glucose co-transporter 2 inhibitors, #13 heart rate variability, #14 cardiomyocyte relaxation and contraction, and #15 glomerular hyperfiltration.
Different from traditional review, bibliometrics analysis can systematically deal with the original manuscripts of relevant research fields, track current research hotspots and general trends, reveal landmark literatures in this field, and obtain clearer and more intuitive results. CiteSpace and VOSviewer, series of user-friendly bibliometrics analysis software, make the analyzer easy to operate. In addition, we use other graphics software, such as Scimago Graphica, to make the data more visible.
The WOSCC system is the most famous database of scientific publications regarding many investigations. According to the literature screening strategy mentioned earlier, 1461 literatures about EMPA for HF in the decade were extracted from WOSCC, with a total of 43861 references, and the number of manuscripts was on a rise. Compared with the h-index, the mean number of citations per paper is a superior indicator of scientific quality, in terms of both accuracy and precision (47). As shown in the geographical distribution map of all contributing countries, most of the research comes from developed countries, and the Americas, Asia and Europe are heavily published and closely linked. Africa has few publications and few contacts with other countries, which suggests that countries should further break regional barriers for academic exchanges.
In the WOSCC database, there are 45 disciplines involved in the field of EMPA for HF and the top 15 are summarized in Table 2. Among them, multidisciplinary research shows an outbreak trend in the current research stage, which is conducive to future development. Most of the JCR in the top 15 journals are Q1/Q2, and seven articles had an IF (an index of the quality of articles included in the journal) of more than 10, with the highest score of 176.079, and four of them scored 5–10, suggesting that the quality of articles written by scholars in this field is high and can provide better guidance for new researchers.
Based on keywords co-occurrence, clustering and burstiness studies, the current research focuses on the following three aspects: EMPA improves left ventricular remodeling, exert renal protection, and increases heart rate variability (HRV).
Acute or chronic myocardial injury results in metabolic disorders followed by cardiomyocyte death. The remaining viable myocardium copes with cell loss through the development of cardiomyocyte hypertrophy and fibrosis in the interstitial space (48, 49). A reduction in contractile force activates neurohormonal compensatory mechanisms designed to maintain systemic perfusion, such as the sympathetic and renal angiotensin systems. Structural changes in the left ventricular (LV) occurred over time, including an increase in ventricular volume, a shift to the right of the end-diastolic pressure-volume relationship (EDPVR), and a development of sphericity (50–52). These anatomical alterations are accompanied by alterations in gene expression, metabolic substrate preference and utilization, and extracellular matrix composition (53–55). These structural, biochemical, and molecular changes are collectively known as ventricular remodeling.
Ventricular remodeling, first described in animal models of LV stress and injury, occurs progressively after large myocardial infarction and in patients with dilated cardiomyopathy. Ventricular remodeling refers to changes in ventricular structure, associated with increased volume and changes in ventricular structure, which are driven by pathological cardiomyocyte hypertrophy, cardiomyocyte apoptosis, myofibroblast proliferation, and interstitial fibrosis at the histological level (56–58). LVEF, the most used clinical measure of cardiac function, is more affected by the degree of LV remodeling than any other factor. Other more precise remodeling measures, such as LV volume and mass, have received greater attention in clinical trials than in clinical practice, but these measures are more closely associated with prognostic and therapeutic effects than LVEF (59). Numerous studies have shown that LV volume measurements at a single time point and over time are of great value in predicting clinical outcomes in patients with HF. A recent meta-analysis of HF trials showed that LV volume stood out among surrogate measures and was strongly associated with the impact of specific drug or device treatment on patient survival. These findings confirm the importance of ventricular remodeling in advancing the pathophysiology of HF and support the role of ventricular remodeling measures in clinical studies of new treatments for HF (60).
EMPATROPISM trial, a pioneer trial to be published first, demonstrated a reduction in LV volumes, regression in LV mass, improvement in LVEF, amelioration in LV sphericity (61). Moreover, EMPA also improved peak oxygen consumption (62), the gold-standard parameter evaluating exercise capacity. EMPA also reduced LV interstitial fibrosis and aortic stiffness (63). The pioneering results of EMPATROPISM have been subsequently confirmed by other independent trials such as SUGAR-DM (64) and eMPIRE-HF (62). In the EMPA-HEART trial (61), the mean LV mass in the regression of body surface area after 6 months was 2.6 g/m2 in the EMPA group and 0.01 g/m2 in the placebo group (P = 0.01), with a significant reduction in the EMPA group, which is presumed to have a positive effect on CV outcomes.
The results were verified in animal experiments. Researchers used LAD ligation to establish a model of HF after MI in female Yorkshire pigs and randomly assigned them to either EMPA or placebo for 2 months. Myocardial injury was subsequently detected using cardiac magnetic resonance and three-dimensional (3D) Echocardiography. The LV end-systolic and end-diastolic diameters of EMPA treated pigs were significantly reduced, indicating a significant mitigation in LV dilatation. LV mass was significantly reduced in EMPA treated pigs and the degree of sphericity of the heart was lower than in control pigs, as indicated by the lower 3D-LV sphericity index, thus indicating reduced structural changes in the treated arm. Further CMR results showed that the improvement of LV remodeling in the EMPA group was associated with an increase in LVEF 2 months after treatment (65). Santos-Gallego demonstrated that the improvement in LVEF is due to a switch in myocardial metabolism. EMPA induces a shift in myocardial metabolism away from glucose-inefficient glucose toward the consumption of free fatty acid and ketone bodies, which enhances cardiac energetics (66, 67). The circumference of porcine cardiomyocytes treated with EMPA was smaller than that in the control group, indicating that there was less compensatory hypertrophy at the cellular level in the EMPA group (68). Increased interstitial myocardial fibrosis (IMF) causes diastolic dysfunction. Collagen deposition was reduced in EMPA-treated pigs versus controls, and levels of hydroxyproline, an essential amino acid found only in collagen and thus an indirect marker of IMF, were lower in the EMPA group. IMF was reduced as the treated group exhibited stiffer Type-1 collagen and reduced expression of the pro-fibrotic cytokine TGF-B gene, as well as reduced TGF-B activity (68). This suggests that EMPA can ameliorate IMF, thereby improving ventricular remodeling.
In the EMPEROR-Reduced Trial, EMPA slowed the progression of eGFR reduction compared with placebo (69, 70). EMPA resulted in a similar placebo-adjusted reduction in the urinary albumin-to-creatinine ratio (UACR) over a mean period of 3.1 years, regardless of Obstructive Sleep Apnea status at baseline (71). EMPA has moderate diuretic and natriuretic effects that ameliorate OSA symptoms by reducing fluid retention in the pleural cavity (72) and rostral fluid transfer to the legs (73), as well as affecting kidney sodium handling (74). Moreover, EMPA induces natriuresis, which enhances the diuretic effect of loop diuretics (8), but does seems only to be maintained in the acute phase (30). It is this acute natriuretic effect which explains the quick benefits of EMPA in acute heart failure within the first days (75).
Brenner (76) proposed that glomerular hyperfiltration with glomerular capillary hypertension is the pathogenesis of renal disease, among which glomerular hyperfiltration has been considered as a potential risk factor for Diabetic nephropathy (DN) (77). SGLT2i reduce glomerular hyperfiltration, thereby preventing the progression of DN, which may in turn reduce CV risk, including HF. Although EMPA have shown significant renal and CV benefits in humans in clinical trials (42, 78), the mechanism of action is not fully understood but is attributable, at least in part, to a reduction in intraglomerular hypertension and hyperfiltration. Single-nephron GFR (SNGFR) in spontaneously diabetic Ins2+/Akita mice was significantly higher than in control groups, reflecting glomerular hyperfiltration, which was ameliorated in EMPA treatment (79).
Hyperuricemia is common in HF and is an independent predictor of advanced disease severity and increased mortality. In the EMPEROR-reduced trial, EMPA induced a rapid and sustained reduction of serum uric acid levels and of clinical events related to hyperuricemia and gout (80). Diabetes mellitus is a risk factor for nephrolithiasis. In an observational study involving 24,290 individuals with T2DM taking SGLT2 inhibitors, Kristensen et al. reported that SGLT2 inhibitor use was associated with a 49% lower risk of nephrolithiasis compared with GLP-1 receptor agonists (81). In EMPA-REG OUTCOME trial, compared with placebo, EMPA therapy was associated with an approximate 40% reduced risk of urinary tract stone events. The underlying mechanisms are unknown but may involve altered lithogenic profile of the urine (82).
Progressive decline in renal function often leads to chronic cardiac dysfunction and CV events. HF is a common complication in patients with chronic kidney disease (CKD), and this process is defined as type 4 cardiorenal syndrome. Trials have shown that SGLT2i treatment significantly improves the outcome of HF, reducing the length of hospitalization for this complication in CKD patients by 30–40% (83). Indeed, treatment with EMPA in diabetic adipose rats improved systemic endothelial function (84). These effects are thought to be mediated probably through increased endothelial viability, reduced senescence, and inflammation, and reduced oxidative damage in the nitric oxide (NO)/cycloguanosine monophosphate signaling cascade, rather than upregulation of endothelial NO synthase expression (84).
Juni et al. (85) provide an interesting mechanistic insight into how uremia impinges on the interaction between endothelial cells and cardiomyocytes and highlight the critical role of cardiac microvascular endothelium in the cardiac benefits of EMPA treatment (Figure 6). The researchers used a co-culture model of human cardiac microvascular endothelial cells (CMECs) and rat ventricular cardiomyocytes. The results showed that CMECs positively affected the relaxation and contraction of cardiomyocytes mainly through endoderm-derived NO. They demonstrated that uremic serum impairs endothelium mediated enhancement of cardiomyocyte function and that EMPA counteracts the deleterious effects of uremic serum on CMECs, leading to restoration of cardiomyocyte function (86).
Arterial stiffness, a surrogate marker of renal and CV clinical outcomes, is influenced by activation of the renin angiotensin aldosterone system (RAAS) and sympathetic nervous system (SNS) and inhibition of NO (87, 88), increases under the influence of ambient hyperglycemia and can be ameliorated by strict glycemic control (89–91), which may help improve BP control and reduce the risk of CV complications (92–94). Traditional oral hypoglycemic agents (liraglutide or glibenclamide) do not improve arterial stiffness parameters, and a significant proportion of patients develop hypertension as the duration of diabetes increases (95, 96). In an 8-week, open-label, prospective clinical trial (NCT01392560) (97), the novel oral hypoglycemic agents, EMPA, could significantly reduce the parameters of vascular sclerosis, including radial augmentation Index, various sclerosis Index and aortic augmentation index. They suggest that the physiological mechanism of reduced arterial stiffness may be related to a variety of factors, including BP lowering, diuretic effects, altered neurohormone activation, improved glycemic control, and weight loss (Figure 6) (98–103).
Heart rate variability is a non-invasive ECG parameter that assesses sympathetic vagal balance at the sinoatrial level, but its physiological interpretation is still under discussion (104–106). In patients with HF, when short-term or 24-h recordings were analyzed, reduced HRV was consistently observed and explained primarily because of sympathetic and reduced vagal modulation of sinus node (104). For the time domain, the following indexes were calculated according to the recommended values (104): mean n-n interval (SDNN), root mean square difference of successive N–N intervals (RMSSD), Standard deviation of all 5-min mean normal RR intervals (SDANN), Mean RR interval for 24 h (mean NN) and proportion of differences between adjacent N–N intervals of > 50 ms (PNN50). In an 8-week, open-label, prospective clinical trial (NCT01392560) (97), EMPA increased RMSSD and SDNN, but not statistically significant. In the EMBODY trial (107), SDNN (101.1S vs. 112.8S, P < 0.01), SDANN (81.0S vs. 92.7S, P = 0.02), and RMSSD (34.2S vs. 40.7S, P = 0.01) were significantly higher after 24 weeks of EMPA versus baseline.
There are some limitations in this paper. We analyze all the articles on EMPA for HF from the WOSCC database, but due to differences in text quality, the credibility of the analysis may be low. With the continuous updating of WOSCC literature, the number of literatures obtained is different from the actual number of literatures, and the cited times and H-index will also change accordingly.
The research progress, hotspots, and frontier in this field in the decade were elucidated by us through information visualization technology. The publications of manuscripts were on a rise. With regards to country, USA leads the field in research maturity. The scholars, institutions, and representative literature that play an important role in this area have been identified. The current research focuses on the following three aspects: EMPA improves LV remodeling, exert renal protection, and increases HRV.
The original contributions presented in this study are included in the article/supplementary material, further inquiries can be directed to the corresponding author.
XZ and YZ designed the study and co-wrote the manuscript. XZ re-examined and analyzed the data. YH reviewed and revised the manuscript. All authors contributed to the article, approved the submitted version, and agreed to be held responsible for all aspects of the work.
This work was supported by National Natural Science Foundation of China (No. 82074409).
The authors declare that the research was conducted in the absence of any commercial or financial relationships that could be construed as a potential conflict of interest.
All claims expressed in this article are solely those of the authors and do not necessarily represent those of their affiliated organizations, or those of the publisher, the editors and the reviewers. Any product that may be evaluated in this article, or claim that may be made by its manufacturer, is not guaranteed or endorsed by the publisher.
1. Packer M, Anker SD, Butler J, Filippatos G, Pocock SJ, Carson P, et al. Cardiovascular and renal outcomes with empagliflozin in heart failure. N Engl J Med. (2020) 383:1413–24.
2. McDonagh TA, Metra M, Adamo M, Gardner RS, Baumbach A, Böhm M, et al. 2021 ESC Guidelines for the diagnosis and treatment of acute and chronic heart failure. Eur Heart J. (2021) 42:3599–726.
3. Writing Committee Members, ACC/AHA Joint Committee Members. 2022 AHA/ACC/HFSA Guideline for the management of heart failure. J Card Fail. (2022) 28:e1–167. doi: 10.1016/j.cardfail.2022.03.346
4. Anker SD, Butler J, Filippatos G, Ferreira JP, Bocchi E, Böhm M, et al. Empagliflozin in heart failure with a preserved ejection fraction. N Engl J Med. (2021) 385:1451–61.
5. Butler J, Anker SD, Filippatos G, Khan MS, Ferreira JP, Pocock SJ, et al. Empagliflozin and health-related quality of life outcomes in patients with heart failure with reduced ejection fraction: the EMPEROR-Reduced trial. Eur Heart J. (2021) 42:1203–12. doi: 10.1093/eurheartj/ehaa1007
6. Requena-Ibanez JA, Santos-Gallego CG, Rodriguez-Cordero A, Vargas-Delgado AP, Badimon JJ. Empagliflozin improves quality of life in nondiabetic HFrEF patients. Sub-analysis of the EMPATROPISM trial. Diabetes Metab Syndr. (2022) 16:102417. doi: 10.1016/j.dsx.2022.102417
7. Jackson AM, Dewan P, Anand IS, Bělohlávek J, Bengtsson O, de Boer RA, et al. Dapagliflozin and diuretic use in patients with heart failure and reduced ejection fraction in DAPA-HF. Circulation. (2020) 142:1040–54. doi: 10.1161/CIRCULATIONAHA.120.047077
8. Griffin M, Rao VS, Ivey-Miranda J, Fleming J, Mahoney D, Maulion C, et al. Empagliflozin in heart failure: diuretic and cardiorenal effects. Circulation. (2020) 142:1028–39. doi: 10.1161/CIRCULATIONAHA.120.045691
9. Santos-Gallego CG, Van Spall HGC. In HFrEF, adding empagliflozin to medical therapy reduced a composite outcome, regardless of CKD status. Ann Intern Med. (2021) 174:JC68. doi: 10.7326/ACPJ202106150-068
10. Zannad F, Ferreira JP, Pocock SJ, Anker SD, Butler J, Filippatos G, et al. SGLT2 inhibitors in patients with heart failure with reduced ejection fraction: a meta-analysis of the EMPEROR-Reduced and DAPA-HF trials. Lancet. (2020) 396:819–29. doi: 10.1016/S0140-6736(20)31824-9
11. Packer M, Anker SD, Butler J, Filippatos G, Ferreira JP, Pocock SJ, et al. Effect of empagliflozin on the clinical stability of patients with heart failure and a reduced ejection fraction: the EMPEROR-reduced trial. Circulation. (2021) 143:326–36. doi: 10.1161/CIRCULATIONAHA.120.051783
12. Baartscheer A, Schumacher CA, Wust RC, Fiolet JW, Stienen GJ, Coronel R, et al. Empagliflozin decreases myocardial cytoplasmic Na(+) through inhibition of the cardiac Na(+)/H(+) exchanger in rats and rabbits. Diabetologia. (2017) 60:568–73. doi: 10.1007/s00125-016-4134-x
13. Uthman L, Baartscheer A, Bleijlevens B, Schumacher CA, Fiolet JWT, Koeman A, et al. Class effects of SGLT2 inhibitors in mouse cardiomyocytes and hearts: inhibition of Na(+)/H(+) exchanger, lowering of cytosolic Na(+) and vasodilation. Diabetologia. (2018) 61:722–6. doi: 10.1007/s00125-017-4509-7
14. Zhang H, Uthman L, Bakker D, Sari S, Chen S, Hollmann MW, et al. Empagliflozin decreases lactate generation in an NHE-1 dependent fashion and increases alpha-ketoglutarate synthesis from palmitate in type ii diabetic mouse hearts. Front Cardiovasc Med. (2020) 7:592233. doi: 10.3389/fcvm.2020.592233
15. Smith DR. Bibliometrics, dermatology and contact dermatitis. Contact Dermatitis. (2008) 59:133–6. doi: 10.1111/j.1600-0536.2008.01405.x
16. Chandra SP, Singh A, Goyal N, Laythalling RK, Singh M, Kale SS, et al. Analysis of changing paradigms of management in 179 patients with spinal tuberculosis over a 12-year period and proposal of a new management algorithm. World Neurosurg. (2013) 80:190–203. doi: 10.1016/j.wneu.2012.12.019
17. Pu QH, Lyu QJ, Su HY. Bibliometric analysis of scientific publications in transplantation journals from Mainland China, Japan, South Korea and Taiwan between 2006 and 2015. BMJ Open. (2016) 6:e011623. doi: 10.1136/bmjopen-2016-011623
18. Hirsch JE. An index to quantify an individual’s scientific research output. Proc Natl Acad Sci U.S.A. (2005) 102:16569–72. doi: 10.1073/pnas.0507655102
19. Baron SJ, Arnold SV, Wang K, Magnuson EA, Chinnakondepali K, Makkar R, et al. Health status benefits of transcatheter vs surgical aortic valve replacement in patients with severe aortic stenosis at intermediate surgical risk: results from the PARTNER 2 randomized clinical trial. JAMA Cardiol. (2017) 2:837–45. doi: 10.1001/jamacardio.2017.2039
20. Spertus JA, Jones PG. Development and validation of a short version of the kansas city cardiomyopathy questionnaire. Circ Cardiovasc Qual Outcomes. (2015) 8:469–76. doi: 10.1161/CIRCOUTCOMES.115.001958
21. Nassif ME, Qintar M, Windsor SL, Jermyn R, Shavelle DM, Tang F, et al. Empagliflozin effects on pulmonary artery pressure in patients with heart failure: results from the EMBRACE-HF trial. Circulation. (2021) 143:1673–86. doi: 10.1161/CIRCULATIONAHA.120.052503
22. Nassif ME, Spertus JA, Tang F, Windsor SL, Jones P, Thomas M, et al. Association between change in ambulatory hemodynamic pressures and symptoms of heart failure. Circ Heart Fail. (2021) 14:e008446. doi: 10.1161/CIRCHEARTFAILURE.121.008446
23. Nassif ME, Kosiborod M. Effects of sodium glucose cotransporter type 2 inhibitors on heart failure. Diabetes Obes Metab. (2019) 21(Suppl. 2):19–23. doi: 10.1111/dom.13678
24. Fitchett D, Zinman B, Wanner C, Lachin JM, Hantel S, Salsali A, et al. Heart failure outcomes with empagliflozin in patients with type 2 diabetes at high cardiovascular risk: results of the EMPA-REG OUTCOME(R) trial. Eur Heart J. (2016) 37:1526–34. doi: 10.1093/eurheartj/ehv728
25. Inzucchi SE, Zinman B, Fitchett D, Wanner C, Ferrannini E, Schumacher M, et al. How does empagliflozin reduce cardiovascular mortality? insights from a mediation analysis of the EMPA-REG OUTCOME trial. Diabetes Care. (2018) 41:356–63. doi: 10.2337/dc17-1096
26. Inzucchi SE, Kosiborod M, Fitchett D, Wanner C, Hehnke U, Kaspers S, et al. Improvement in cardiovascular outcomes with empagliflozin is independent of glycemic control. Circulation. (2018) 138:1904–7. doi: 10.1161/CIRCULATIONAHA.118.035759
27. Butler J, Hamo CE, Filippatos G, Pocock SJ, Bernstein RA, Brueckmann M, et al. The potential role and rationale for treatment of heart failure with sodium-glucose co-transporter 2 inhibitors. Eur J Heart Fail. (2017) 19:1390–400. doi: 10.1002/ejhf.933
28. Ferreira JP, Butler J, Zannad F, Filippatos G, Schueler E, Steubl D, et al. Mineralocorticoid receptor antagonists and empagliflozin in patients with heart failure and preserved ejection fraction. J Am Coll Cardiol. (2022) 79:1129–37. doi: 10.1016/j.jacc.2022.01.029
29. Kosiborod MN, Angermann CE, Collins SP, Teerlink JR, Ponikowski P, Biegus J, et al. Effects of empagliflozin on symptoms, physical limitations, and quality of life in patients hospitalized for acute heart failure: results from the EMPULSE trial. Circulation. (2022) 146:279–88. doi: 10.1161/CIRCULATIONAHA.122.059725
30. Mordi NA, Mordi IR, Singh JS, McCrimmon RJ, Struthers AD, Lang CC. Renal and cardiovascular effects of SGLT2 inhibition in combination with loop diuretics in patients with type 2 diabetes and chronic heart failure: the RECEDE-CHF trial. Circulation. (2020) 142:1713–24. doi: 10.1161/CIRCULATIONAHA.120.048739
31. Mordi NA, Mordi IR, Singh JS, Baig F, Choy AM, McCrimmon RJ, et al. Renal and cardiovascular effects of sodium-glucose cotransporter 2 (SGLT2) inhibition in combination with loop diuretics in diabetic patients with chronic heart failure (RECEDE-CHF): protocol for a randomised controlled double-blind cross-over trial. BMJ Open. (2017) 7:e018097. doi: 10.1136/bmjopen-2017-018097
32. Heerspink HJL, Stefansson BV, Correa-Rotter R, Chertow GM, Greene T, Hou FF, et al. Dapagliflozin in patients with chronic kidney disease. N Engl J Med. (2020) 383:1436–46. doi: 10.1056/NEJMoa2024816
33. Bhatt DL, Szarek M, Steg PG, Cannon CP, Leiter LA, McGuire DK, et al. Sotagliflozin in patients with diabetes and recent worsening heart failure. N Engl J Med. (2021) 384:117–28. doi: 10.1056/NEJMoa2030183
34. Cannon CP, Pratley R, Dagogo-Jack S, Mancuso J, Huyck S, Masiukiewicz U, et al. Cardiovascular Outcomes with ertugliflozin in type 2 diabetes. N Engl J Med. (2020) 383:1425–35. doi: 10.1056/NEJMoa2004967
35. Bhatt DL, Szarek M, Pitt B, Cannon CP, Leiter LA, McGuire DK, et al. Sotagliflozin in patients with diabetes and chronic kidney disease. N Engl J Med. (2021) 384:129–39. doi: 10.1056/NEJMoa2030186
36. McGuire DK, Shih WJ, Cosentino F, Charbonnel B, Cherney DZI, Dagogo-Jack S, et al. Association of SGLT2 inhibitors with cardiovascular and kidney outcomes in patients with type 2 diabetes: a meta-analysis. JAMA Cardiol. (2021) 6:148–58. doi: 10.1001/jamacardio.2020.4511
37. Packer M, Butler J, Zannad F, Filippatos G, Ferreira JP, Pocock SJ, et al. Effect of empagliflozin on worsening heart failure events in patients with heart failure and preserved ejection fraction: EMPEROR-preserved trial. Circulation. (2021) 144:1284–94. doi: 10.1161/CIRCULATIONAHA.121.056824
38. Pogwizd SM, Sipido KR, Verdonck F, Bers DM. Intracellular Na in animal models of hypertrophy and heart failure: contractile function and arrhythmogenesis. Cardiovasc Res. (2003) 57:887–96. doi: 10.1016/S0008-6363(02)00735-6
39. Liu T, O’Rourke B. Enhancing mitochondrial Ca2+ uptake in myocytes from failing hearts restores energy supply and demand matching. Circ Res. (2008) 103:279–88. doi: 10.1161/CIRCRESAHA.108.175919
40. Baartscheer A, Schumacher CA, van Borren MM, Belterman CN, Coronel R, Opthof T, et al. Chronic inhibition of Na+/H+-exchanger attenuates cardiac hypertrophy and prevents cellular remodeling in heart failure. Cardiovasc Res. (2005) 65:83–92. doi: 10.1016/j.cardiores.2004.09.024
41. Baartscheer A, Hardziyenka M, Schumacher CA, Belterman CN, van Borren MM, Verkerk AO, et al. Chronic inhibition of the Na+/H+ – exchanger causes regression of hypertrophy, heart failure, and ionic and electrophysiological remodelling. Br J Pharmacol. (2008) 154:1266–75. doi: 10.1038/bjp.2008.189
42. Zinman B, Wanner C, Lachin JM, Fitchett D, Bluhmki E, Hantel S, et al. Empagliflozin, cardiovascular outcomes, and mortality in type 2 diabetes. N Engl J Med. (2015) 373:2117–28. doi: 10.1056/NEJMoa1504720
43. Long-Term Intervention with Pravastatin in Ischaemic Disease (Lipid) Study Group. Prevention of cardiovascular events and death with pravastatin in patients with coronary heart disease and a broad range of initial cholesterol levels. N Engl J Med. (1998) 339:1349–57. doi: 10.1056/NEJM199811053391902
44. Patel A, Advance Collaborative Group, MacMahon S, Chalmers J, Neal B, Woodward M, et al. Effects of a fixed combination of perindopril and indapamide on macrovascular and microvascular outcomes in patients with type 2 diabetes mellitus (the ADVANCE trial): a randomised controlled trial. Lancet. (2007) 370:829–40. doi: 10.1016/S0140-6736(07)61303-8
45. Zhang X, Zhou Y, Wei N, Shou X, Fan S, You Y, et al. A bibliometric analysis of heart failure with preserved ejection fraction from 2000 to 2021. Curr Probl Cardiol. (2022) 47:101243. doi: 10.1016/j.cpcardiol.2022.101243
46. Chen C, Hu Z, Liu S, Tseng H. Emerging trends in regenerative medicine: a scientometric analysis in CiteSpace. Expert Opin Biol Ther. (2012) 12:593–608. doi: 10.1517/14712598.2012.674507
47. Lehmann S, Jackson AD, Lautrup BE. Measures for measures. Nature. (2006) 444:1003–4. doi: 10.1038/4441003a
48. Anversa P, Olivetti G, Capasso JM. Cellular basis of ventricular remodeling after myocardial infarction. Am J Cardiol. (1991) 68:7–16D. doi: 10.1016/0002-9149(91)90256-K
49. Weber KT, Pick R, Silver MA, Moe GW, Janicki JS, Zucker IH, et al. Fibrillar collagen and remodeling of dilated canine left ventricle. Circulation. (1990) 82:1387–401. doi: 10.1161/01.CIR.82.4.1387
50. Hochman JS, Bulkley BH. Expansion of acute myocardial infarction: an experimental study. Circulation. (1982) 65:1446–50. doi: 10.1161/01.CIR.65.7.1446
51. Mitchell GF, Lamas GA, Vaughan DE, Pfeffer MA. Left ventricular remodeling in the year after first anterior myocardial infarction: a quantitative analysis of contractile segment lengths and ventricular shape. J Am Coll Cardiol. (1992) 19:1136–44. doi: 10.1016/0735-1097(92)90314-D
52. Pfeffer JM, Pfeffer MA, Fletcher PJ, Braunwald E. Progressive ventricular remodeling in rat with myocardial infarction. Am J Physiol. (1991) 260(5 Pt. 2):H1406–14. doi: 10.1152/ajpheart.1991.260.5.H1406
53. Margulies KB, Matiwala S, Cornejo C, Olsen H, Craven WA, Bednarik D. Mixed messages: transcription patterns in failing and recovering human myocardium. Circ Res. (2005) 96:592–9. doi: 10.1161/01.RES.0000159390.03503.c3
54. Spinale FG, Coker ML, Heung LJ, Bond BR, Gunasinghe HR, Etoh T, et al. A matrix metalloproteinase induction/activation system exists in the human left ventricular myocardium and is upregulated in heart failure. Circulation. (2000) 102:1944–9. doi: 10.1161/01.CIR.102.16.1944
55. Kim GH, Uriel N, Burkhoff D. Reverse remodelling and myocardial recovery in heart failure. Nat Rev Cardiol. (2018) 15:83–96. doi: 10.1038/nrcardio.2017.139
56. Eaton LW, Weiss JL, Bulkley BH, Garrison JB, Weisfeldt ML. Regional cardiac dilatation after acute myocardial infarction: recognition by two-dimensional echocardiography. N Engl J Med. (1979) 300:57–62. doi: 10.1056/NEJM197901113000202
57. Erlebacher JA, Weiss JL, Eaton LW, Kallman C, Weisfeldt ML, Bulkley BH. Late effects of acute infarct dilation on heart size: a two dimensional echocardiographic study. Am J Cardiol. (1982) 49:1120–6. doi: 10.1016/0002-9149(82)90035-2
58. McKay RG, Pfeffer MA, Pasternak RC, Markis JE, Come PC, Nakao S, et al. Left ventricular remodeling after myocardial infarction: a corollary to infarct expansion. Circulation. (1986) 74:693–702. doi: 10.1161/01.CIR.74.4.693
59. Anand IS, Florea VG, Solomon SD, Konstam MA, Udelson JE. Noninvasive assessment of left ventricular remodeling: concepts, techniques, and implications for clinical trials. J Card Fail. (2002) 8(6 Suppl.):S452–64. doi: 10.1054/jcaf.2002.129286
60. Konstam MA, Kramer DG, Patel AR, Maron MS, Udelson JE. Left ventricular remodeling in heart failure: current concepts in clinical significance and assessment. JACC Cardiovasc Imaging. (2011) 4:98–108. doi: 10.1016/j.jcmg.2010.10.008
61. Verma S, Mazer CD, Yan AT, Mason T, Garg V, Teoh H, et al. Effect of empagliflozin on left ventricular mass in patients with type 2 diabetes mellitus and coronary artery disease: the EMPA-HEART cardiolink-6 randomized clinical trial. Circulation. (2019) 140:1693–702. doi: 10.1161/CIRCULATIONAHA.119.042375
62. Omar M, Jensen J, Ali M, Frederiksen PH, Kistorp C, Videbæk L, et al. Associations of empagliflozin with left ventricular volumes, mass, and function in patients with heart failure and reduced ejection fraction: a substudy of the empire hf randomized clinical trial. JAMA Cardiol. (2021) 6:836–40. doi: 10.1001/jamacardio.2020.6827
63. Requena-Ibanez JA, Santos-Gallego CG, Rodriguez-Cordero A, Vargas-Delgado AP, Mancini D, Sartori S, et al. Mechanistic insights of empagliflozin in nondiabetic patients with HFrEF: from the EMPA-TROPISM study. JACC Heart Fail. (2021) 9:578–89. doi: 10.1016/j.jchf.2021.04.014
64. Lee MMY, Brooksbank KJM, Wetherall K, Mangion K, Roditi G, Campbell RT, et al. Effect of empagliflozin on left ventricular volumes in patients with type 2 diabetes, or prediabetes, and heart failure with reduced ejection fraction (SUGAR-DM-HF). Circulation. (2021) 143:516–25. doi: 10.1161/CIRCULATIONAHA.121.055067
65. Santos-Gallego CG, Requena-Ibanez JA, San Antonio R, Ishikawa K, Watanabe S, Picatoste B, et al. Empagliflozin ameliorates adverse left ventricular remodeling in nondiabetic heart failure by enhancing myocardial energetics. J Am Coll Cardiol. (2019) 73:1931–44. doi: 10.1016/j.jacc.2019.01.056
66. Santos-Gallego CG, Mayr M, Badimon J. SGLT2 Inhibitors in heart failure: targeted metabolomics and energetic metabolism. Circulation. (2022) 146:819–21. doi: 10.1161/CIRCULATIONAHA.122.060805
67. Garcia-Ropero A, Vargas-Delgado AP, Santos-Gallego CG, Badimon JJ. Inhibition of sodium glucose cotransporters improves cardiac performance. Int J Mol Sci. (2019) 20:3289. doi: 10.3390/ijms20133289
68. Santos-Gallego CG, Requena-Ibanez JA, San Antonio R, Garcia-Ropero A, Ishikawa K, Watanabe S, et al. Empagliflozin ameliorates diastolic dysfunction and left ventricular fibrosis/stiffness in nondiabetic heart failure: a multimodality study. JACC Cardiovasc Imaging. (2021) 14:393–407. doi: 10.1016/j.jcmg.2020.07.042
69. Anker SD, Butler J, Filippatos G, Khan MS, Marx N, Lam CSP, et al. Effect of empagliflozin on cardiovascular and renal outcomes in patients with heart failure by baseline diabetes status: results from the EMPEROR-reduced trial. Circulation. (2021) 143:337–49. doi: 10.1161/CIRCULATIONAHA.120.051824
70. Bohm M, Anker SD, Butler J, Filippatos G, Ferreira JP, Pocock SJ, et al. Empagliflozin improves cardiovascular and renal outcomes in heart failure irrespective of systolic blood pressure. J Am Coll Cardiol. (2021) 78:1337–48. doi: 10.1016/j.jacc.2021.07.049
71. Neeland IJ, Eliasson B, Kasai T, Marx N, Zinman B, Inzucchi SE, et al. The impact of empagliflozin on obstructive sleep apnea and cardiovascular and renal outcomes: an exploratory analysis of the EMPA-REG OUTCOME trial. Diabetes Care. (2020) 43:3007–15. doi: 10.2337/dc20-1096
72. Pearse SG, Cowie MR. Sleep-disordered breathing in heart failure. Eur J Heart Fail. (2016) 18:353–61. doi: 10.1002/ejhf.492
73. Kasai T. Fluid retention and rostral fluid shift in sleep-disordered breathing. Curr Hypertens Rev. (2016) 12:32–42. doi: 10.2174/1573402112666160114093550
74. de Albuquerque Rocha N, Neeland IJ, McCullough PA, Toto RD, McGuire DK. Effects of sodium glucose co-transporter 2 inhibitors on the kidney. Diab Vasc Dis Res. (2018) 15:375–86. doi: 10.1177/1479164118783756
75. Voors AA, Angermann CE, Teerlink JR, Collins SP, Kosiborod M, Biegus J, et al. The SGLT2 inhibitor empagliflozin in patients hospitalized for acute heart failure: a multinational randomized trial. Nat Med. (2022) 28:568–74. doi: 10.1038/s41591-021-01659-1
76. Brenner BM. Hemodynamically mediated glomerular injury and the progressive nature of kidney disease. Kidney Int. (1983) 23:647–55. doi: 10.1038/ki.1983.72
77. Fioretto P, Zambon A, Rossato M, Busetto L, Vettor R. SGLT2 inhibitors and the diabetic kidney. Diabetes Care. (2016) 39(Suppl. 2):S165–71. doi: 10.2337/dcS15-3006
78. Wanner C, Inzucchi SE, Zinman B. Empagliflozin and progression of kidney disease in type 2 diabetes. N Engl J Med. (2016) 375:1801–2. doi: 10.1056/NEJMc1611290
79. Kidokoro K, Cherney DZI, Bozovic A, Nagasu H, Satoh M, Kanda E, et al. Evaluation of glomerular hemodynamic function by empagliflozin in diabetic mice using in vivo imaging. Circulation. (2019) 140:303–15. doi: 10.1161/CIRCULATIONAHA.118.037418
80. Doehner W, Anker SD, Butler J, Zannad F, Filippatos G, Ferreira JP, et al. Uric acid and sodium-glucose cotransporter-2 inhibition with empagliflozin in heart failure with reduced ejection fraction: the EMPEROR-reduced trial. Eur Heart J. (2022) 43:3435–46. doi: 10.1093/eurheartj/ehac320
81. Kristensen KB, Henriksen DP, Hallas J, Pottegard A, Lund LC. Sodium-glucose cotransporter 2 inhibitors and risk of nephrolithiasis. Diabetologia. (2021) 64:1563–71. doi: 10.1007/s00125-021-05424-4
82. Balasubramanian P, Wanner C, Ferreira JP, Ofstad AP, Elsaesser A, Zinman B, et al. Empagliflozin and decreased risk of nephrolithiasis: a potential new role for SGLT2 inhibition? J Clin Endocrinol Metab. (2022) 107:e3003–7. doi: 10.1210/clinem/dgac154
83. Salah HM, Al’Aref SJ, Khan MS, Al-Hawwas M, Vallurupalli S, Mehta JL, et al. Effect of sodium-glucose cotransporter 2 inhibitors on cardiovascular and kidney outcomes-Systematic review and meta-analysis of randomized placebo-controlled trials. Am Heart J. (2021) 232:10–22. doi: 10.1016/j.ahj.2020.10.064
84. Dou L, Jourde-Chiche N. Endothelial toxicity of high glucose and its by-products in diabetic kidney disease. Toxins. (2019) 11:578. doi: 10.3390/toxins11100578
85. Juni RP, Al-Shama R, Kuster DWD, van der Velden J, Hamer HM, Vervloet MG, et al. Empagliflozin restores chronic kidney disease-induced impairment of endothelial regulation of cardiomyocyte relaxation and contraction. Kidney Int. (2021) 99:1088–101. doi: 10.1016/j.kint.2020.12.013
86. Juni RP, Kuster DWD, Goebel M, Helmes M, Musters RJP, van der Velden J, et al. Cardiac microvascular endothelial enhancement of cardiomyocyte function is impaired by inflammation and restored by empagliflozin. JACC Basic Transl Sci. (2019) 4:575–91. doi: 10.1016/j.jacbts.2019.04.003
87. Bellien J, Favre J, Iacob M, Gao J, Thuillez C, Richard V, et al. Arterial stiffness is regulated by nitric oxide and endothelium-derived hyperpolarizing factor during changes in blood flow in humans. Hypertension. (2010) 55:674–80. doi: 10.1161/HYPERTENSIONAHA.109.142190
88. Sasson AN, Cherney DZ. Renal hyperfiltration related to diabetes mellitus and obesity in human disease. World J Diabetes. (2012) 3:1–6. doi: 10.4239/wjd.v3.i1.1
89. Gordin D, Ronnback M, Forsblom C, Makinen V, Saraheimo M, Groop PH. Glucose variability, blood pressure and arterial stiffness in type 1 diabetes. Diabetes Res Clin Pract. (2008) 80:e4–7. doi: 10.1016/j.diabres.2008.01.010
90. Gordin D, Ronnback M, Forsblom C, Heikkila O, Saraheimo M, Groop PH. Acute hyperglycaemia rapidly increases arterial stiffness in young patients with type 1 diabetes. Diabetologia. (2007) 50:1808–14. doi: 10.1007/s00125-007-0730-0
91. Manolis AJ, Iraklianou S, Pittaras A, Zaris M, Tsioufis K, Psaltiras G, et al. Arterial compliance changes in diabetic normotensive patients after angiotensin-converting enzyme inhibition therapy. Am J Hypertens. (2005) 18:18–22. doi: 10.1016/j.amjhyper.2004.08.014
92. Kim SG, Ryu OH, Kim HY, Lee KW, Seo JA, Kim NH, et al. Effect of rosiglitazone on plasma adiponectin levels and arterial stiffness in subjects with prediabetes or non-diabetic metabolic syndrome. Eur J Endocrinol. (2006) 154:433–40. doi: 10.1530/eje.1.02100
93. Agarwal N, Rice SP, Bolusani H, Luzio SD, Dunseath G, Ludgate M, et al. Metformin reduces arterial stiffness and improves endothelial function in young women with polycystic ovary syndrome: a randomized, placebo-controlled, crossover trial. J Clin Endocrinol Metab. (2010) 95:722–30. doi: 10.1210/jc.2009-1985
94. Kiyici S, Ersoy C, Kaderli A, Fazlioglu M, Budak F, Duran C, et al. Effect of rosiglitazone, metformin and medical nutrition treatment on arterial stiffness, serum MMP-9 and MCP-1 levels in drug naive type 2 diabetic patients. Diabetes Res Clin Pract. (2009) 86:44–50. doi: 10.1016/j.diabres.2009.07.004
95. Forst T, Michelson G, Ratter F, Weber MM, Anders S, Mitry M, et al. Addition of liraglutide in patients with Type 2 diabetes well controlled on metformin monotherapy improves several markers of vascular function. Diabet Med. (2012) 29:1115–8. doi: 10.1111/j.1464-5491.2012.03589.x
96. Koren S, Shemesh-Bar L, Tirosh A, Peleg RK, Berman S, Hamad RA, et al. The effect of sitagliptin versus glibenclamide on arterial stiffness, blood pressure, lipids, and inflammation in type 2 diabetes mellitus patients. Diabetes Technol Ther. (2012) 14:561–7. doi: 10.1089/dia.2011.0296
97. Cherney DZ, Perkins BA, Soleymanlou N, Har R, Fagan N, Johansen OE, et al. The effect of empagliflozin on arterial stiffness and heart rate variability in subjects with uncomplicated type 1 diabetes mellitus. Cardiovasc Diabetol. (2014) 13:28. doi: 10.1186/1475-2840-13-28
98. List JF, Whaley JM. Glucose dynamics and mechanistic implications of SGLT2 inhibitors in animals and humans. Kidney Int Suppl. (2011) 120:S20–7. doi: 10.1038/ki.2010.512
99. Rosenstock J, Jelaska A, Wang F, Kim G, Broedl U, Woerle HJ, et al. Empagliflozin as add on to basal insulin for 78 weeks improves glycemic control with weight loss in insulin-treated type 2 diabetes (T2DM). Can J Diabetes. (2013) 37S4(Suppl. S4):S32. doi: 10.1016/j.jcjd.2013.08.093
100. Perkins BA, Cherney DZ, Partridge H, Soleymanlou N, Tschirhart H, Zinman B, et al. The sodium glucose co-transporter-2 (SGLT2) inhibitor empagliflozin improves glycaemic control in patients with type 1 diabetes: a single-arm clinical trial. Diabetes Care. (2013) 37:1480–3. doi: 10.2337/dc13-2338
101. Mudaliar S, Armstrong DA, Mavian AA, O’Connor-Semmes R, Mydlow PK, Ye J, et al. Remogliflozin etabonate, a selective inhibitor of the sodium-glucose transporter 2, improves serum glucose profiles in type 1 diabetes. Diabetes Care. (2012) 35:2198–200. doi: 10.2337/dc12-0508
102. Cherney DZ, Perkins BA, Soleymanlou N, Maione M, Lai V, Lee A, et al. Renal hemodynamic effect of sodium-glucose cotransporter 2 inhibition in patients with type 1 diabetes mellitus. Circulation. (2014) 129:587–97. doi: 10.1161/CIRCULATIONAHA.113.005081
103. List JF, Woo V, Morales E, Tang W, Fiedorek FT. Sodium-glucose cotransport inhibition with dapagliflozin in type 2 diabetes. Diabetes Care. (2009) 32:650–7. doi: 10.2337/dc08-1863
105. Malik M, Hnatkova K, Huikuri HV, Lombardi F, Schmidt G, Zabel M. CrossTalk proposal: heart rate variability is a valid measure of cardiac autonomic responsiveness. J Physiol. (2019) 597:2595–8. doi: 10.1113/JP277500
106. Boyett M, Wang Y, D’Souza A. CrossTalk opposing view: heart rate variability as a measure of cardiac autonomic responsiveness is fundamentally flawed. J Physiol. (2019) 597:2599–601. doi: 10.1113/JP277501
107. Shimizu W, Kubota Y, Hoshika Y, Mozawa K, Tara S, Tokita Y, et al. Effects of empagliflozin versus placebo on cardiac sympathetic activity in acute myocardial infarction patients with type 2 diabetes mellitus: the EMBODY trial. Cardiovasc Diabetol. (2020) 19:148. doi: 10.21203/rs.3.rs-35207/v2
108. Scirica BM, Bhatt DL, Braunwald E, Steg PG, Davidson J, Hirshberg B, et al. Saxagliptin and cardiovascular outcomes in patients with type 2 diabetes mellitus. N Engl J Med. (2013) 369:1317–26. doi: 10.1056/NEJMoa1307684
109. White WB, Cannon CP, Heller SR, Nissen SE, Bergenstal RM, Bakris GL, et al. Alogliptin after acute coronary syndrome in patients with type 2 diabetes. N Engl J Med. (2013) 369:1327–35. doi: 10.1056/NEJMoa1305889
110. Green JB, Bethel MA, Armstrong PW, Buse JB, Engel SS, Garg J, et al. Effect of sitagliptin on cardiovascular outcomes in type 2 diabetes. N Engl J Med. (2015) 373:232–42. doi: 10.1056/NEJMoa1501352
111. Pfeffer MA, Claggett B, Diaz R, Dickstein K, Gerstein HC, Køber LV, et al. Lixisenatide in patients with type 2 diabetes and acute coronary syndrome. N Engl J Med. (2015) 373:2247–57. doi: 10.1056/NEJMoa1509225
112. Marx N, McGuire DK. Sodium-glucose cotransporter-2 inhibition for the reduction of cardiovascular events in high-risk patients with diabetes mellitus. Eur Heart J. (2016) 37:3192–200. doi: 10.1093/eurheartj/ehw110
Keywords: empagliflozin, heart failure, CiteSpace, VOSviewer, bibliometrics, visualization
Citation: Zhang X, Zhang Y and Hu Y (2022) Knowledge domain and emerging trends in empagliflozin for heart failure: A bibliometric and visualized analysis. Front. Cardiovasc. Med. 9:1039348. doi: 10.3389/fcvm.2022.1039348
Received: 08 September 2022; Accepted: 18 October 2022;
Published: 03 November 2022.
Edited by:
Germán Cediel, Germans Trias i Pujol Hospital, SpainReviewed by:
Juan Badimon, Icahn School of Medicine at Mount Sinai, United StatesCopyright © 2022 Zhang, Zhang and Hu. This is an open-access article distributed under the terms of the Creative Commons Attribution License (CC BY). The use, distribution or reproduction in other forums is permitted, provided the original author(s) and the copyright owner(s) are credited and that the original publication in this journal is cited, in accordance with accepted academic practice. No use, distribution or reproduction is permitted which does not comply with these terms.
*Correspondence: Yuanhui Hu, aHVpeXVodWk1NUBzb2h1LmNvbQ==
†These authors have contributed equally to this work and share first authorship
Disclaimer: All claims expressed in this article are solely those of the authors and do not necessarily represent those of their affiliated organizations, or those of the publisher, the editors and the reviewers. Any product that may be evaluated in this article or claim that may be made by its manufacturer is not guaranteed or endorsed by the publisher.
Research integrity at Frontiers
Learn more about the work of our research integrity team to safeguard the quality of each article we publish.