- Department of Cardiac Surgery, The University of Tokyo Hospital, Tokyo, Japan
Moderate to severe aortic insufficiency (AI) in patients who underwent continuous-flow left ventricular assist device (CF-LVAD) implantation is a significant complication. According to the INTERMACS registry analysis, at least mild AI occurs in 55% of patients at 6 months after CF-LVAD implantation and moderate to severe AI is significantly associated with higher rates of re-hospitalization and mortality. The clinical implications of these data may underscore consideration of prophylactic aortic valve replacement, or repair, at the time of CF-LVAD implantation, particularly with expected longer duration of support and in patients with preexisting AI that is more than mild. More crucially, even if a native aortic valve is seemingly competent at the time of VAD implantation, we frequently find de novo AI as time goes by, potentially due to commissural fusion in the setting of inconsistent aortic valve opening or persistent valve closure caused by CF-LVAD support, that alters morphological and functional properties of innately competent aortic valves. Therefore, close monitoring of AI is mandatory, as the prognostic nature of its longitudinal progression is still unclear. Clearly, significant AI during VAD support warrants surgical intervention at the appropriate timing, especially in patients of destination therapy. Nonetheless, such an uncertainty in the progression of AI translates to a lack of consensus regarding the management of this untoward complication. In practice, proposed surgical options are aortic valve replacement, repair, closure, and more recently transcatheter aortic valve implantation or closure. Transcatheter approach is of course less invasive, however, its efficacy in terms of long-term outcome is limited. In this review, we summarize the recent evidence related to the pathophysiology and surgical treatment of AI associated with CF-LVAD implantation.
Introduction
Moderate to severe aortic insufficiency (AI) in patients who underwent continuous-flow left ventricular assist device (CF-LVAD) implantation is a significant complication affecting long-term outcomes (1–7). According to the INTERMACS registry analysis, at least mild AI occurs in 55% of patients at 6 months after CF-LVAD implantation and moderate to severe AI is significantly associated with higher rates of re-hospitalization and mortality (1). The clinical implications of these data may underscore consideration of prophylactic aortic valve replacement (AVR), or repair, at the time of CF-LVAD implantation, particularly with expected longer duration of support and in patients with preexisting AI that is more than mild (8). More crucially, even if a native aortic valve (AV) is seemingly competent at the time of VAD implantation, we frequently find de novo AI as time goes by, potentially due to commissural fusion in the setting of inconsistent aortic valve opening or persistent valve closure caused by CF-LVAD support, that alters morphological and functional properties of innately competent aortic valves (9–13). Therefore, close monitoring of AI is mandatory, as the prognostic nature of its longitudinal progression is still unclear. Clearly, significant AI during CF-LVAD support warrants surgical or percutaneous intervention at the appropriate timing (14–18), especially in patients of destination therapy. Nonetheless, such an uncertainty in the progression of AI translates to a lack of consensus regarding the management of this untoward complication. Additionally, before facing to the evaluation of AI during CF-LVAD support, even today, we have not yet established a reliable, or reproducible, method of quantifying the grade of AI in those patients. In the patients under CF-LVAD support, color doppler method might not be enough to measure the amount of actual AI regurgitant flow, as in most circumstances, significant CF-LVAD-associated AI is a continuous one, not a diastolic one, due to continuous suction by the devices. Such another uncertainty, or variability, in the evaluation of AI might have partly contributed to a current lack of consensus in this topic.
In practice, proposed surgical options are AVR (19, 20), AV repair (21–28), AV closure (29), and more recently transcatheter aortic valve implantation (TAVI) (30–33) or transcatheter device closure (34, 35). Briefly, AVR with bioprosthetic valve could be a gold standard treatment for AI in CF-LVAD patients, especially when the native AV contains structural problems. However, it necessitates longer ischemic time, posing a concern of further deterioration of biventricular function in these VAD patients particularly with reduced right ventricular function at baseline. AV repair, or what we call central AV closure (CAVC) or Park's stitch (21, 22), is more simple and technically possible with shorter ischemic time under limited AV exposure. The drawback of CAVC is a durability specifically when the patients' expected support duration is long, such as destination therapy. AV closure could be a last option to consider, as the clinical outcome is not satisfactory (29). Finally, transcatheter approach is of course less invasive, however, its efficacy in terms of long-term outcome is limited. In this review, we summarize the recent evidence related to the pathophysiology and surgical treatment of AI late after CF-LVAD implantation.
Pathophysiology of AI associated with CF-LVAD support
Although the true mechanisms of de novo AI under CF-LVAD support remain controversial, following three factors are likely to be associated with de novo AI: (1) continuous or intermittent AV closure due to the constant increase of aortic diastolic pressure with the decrease in LV end-diastolic pressure, (2) increased transvalvular gradient due to decompression of the LV, leading to stretching or partial prolapse of AV leaflets, and (3) pathologic changes or dilatations in the aortic sinus due to turbulent backflow with high blood velocity from a CF-LVAD outflow (11, 12). These factors could interact with one another, eventually yielding AV disorganization and/or commissural fusion, with a time-related manner. Historically, as diagnostic modalities were quite limited, the effect of CF-LVAD on aortic blood flow dynamics and kinetics as well as on AV physiology had not been fully elucidated. Today, computational fluid dynamic (CFD) studies have demonstrated that the blood stream from LVAD outflow could increase the shear stress on the aortic root and AV. Kasinpila et al. conducted a CFD study in 10 patients with de novo AI and 20 patients without AI after CF-LVAD implantation, and concluded that those who developed de novo AI had greater wall shear stress on the aortic root and their outflow grafts were placed closer to the aortic root than those patients without de novo AI (13). Similar CFD studies were reported by Yoshida et al. (9). They investigated the impact of non-physiological retrograde blood flow in the aortic root on de novo AI after CF-LVAD implantation by CFD analysis. Yoshida et al. demonstrated that those with de novo AI had a perpendicular outflow anastomosis at the ascending aorta, concluding the angle and position of LVAD outflow anastomosis might impact retrograde blood flow and de novo AI after CF-LVAD implantation (9). While higher wall shear stress on the aortic root could be associated with root or annular dilatation, eventually leading to AI progression, lower wall shear stress, as compared to physiological one, is known to be a cause of atherosclerosis (36). Based on the idea, Kainuma et al. proposed a different explanation on the AV degeneration during CF-LVAD support (10). They used an intraoperative epi-aortic echocardiography and calculated wall shear stress by vector flow mapping technology. This in-vivo study, not a computational simulation, demonstrated peak wall shear stress on the ascending aorta, aortic root, and ventricularis of AV was significantly reduced by CF-LVAD support, as compared to baseline (before LVAD). Kainuma et al. suggested such an altered mechanical stress on the AV could be associated with the structural, functional, and histological changes of the aorta and AV (10). Thus, we need more prospective studies to fully clarify the true mechanism of LVAD-induced AI.
Incidence and clinical significance of late AI during CF-LVAD support
In contrast to the pathophysiology, the incidence of late AI during CF-LVAD support has been well-documented (1, 37). A recent analysis on the INTERMACS registry revealed late AI as a progressive disease that develops during CF-LVAD support with well-over 50% developing mild disease at 6 months of support and 15% developing moderate to severe within 2 years (1). Predictors of worsening AI included older age, female sex, smaller body mass index, mild pre-implantation AI, and destination therapy. Significant AI was associated with higher rates of rehospitalization (32.1 vs. 26.6%, p = 0.015) and lower rates of survival (77.2 vs. 71.4%, p = 0.005) (1). There are a few other recent single-institutional studies focused on detrimental effects of AI after CF-LVAD (2–6). Auvil et al. reported that they found moderate or greater AI in 8.5% of patients who underwent CF-LVAD implantation, at 6 months after the implant, and demonstrated that moderate AI was significantly associated with 2-year mortality after the implant [Odds ratio (OR) 4.32, 95% CI 1.21–15.4, p = 0.024] (4). Imamura et al. reported that worsening of AI was observed 53.7% of CF-LVAD patients at 3 months after the implantation, which was significantly associated with higher hazard of death or heart-failure readmission (HR 3.24, 95% CI 1.02–18.5, p = 0.038) (2). Kagawa et al. reported that 13.3% of CF-LVAD patients progressed to significant AI during median follow-up of 469 days, and mortality during the follow-up was significantly higher in the significant AI group (59.5 vs. 37.2%, p = 0.006) (5).
In contrast, there are several studies proposing that the influence of late AI on mortality during CF-LVAD support is not significant. Patil et al. reported that mild AI developed in 51.6% of CF-LVAD patients over a median duration of 126 days and moderate one developed in 14.0% over a median duration of 493 days (37). Like other studies, independent predictors of AI were duration of support and persistently closed aortic valve, although they did not find any association between AI progression and survival outcomes. Holley et al. showed that significant de novo AI occurred in 15.2% of patients after CF-LVAD implantation and such a de novo AI was not significantly associated with mortality (38). Compatible to the prior studies, they concluded that the independent predictors of late AI were older age, female gender, longer duration of LVAD support, and destination therapy.
As for the predictors of late AI, the effect of device type is a matter of much account and still controversial (18, 37, 39, 40). Historically, the development of intermittent low-speed (ILS) algorithm, or its analog, to avoid persistent closure of the AV, was expected to decrease the rate of late de novo AI (41–45). However, the favorable evidence of its efficacy on late AI is still limited. Patil et al. compared 58 HeartMate II (Axial pump, Abbott, MN, USA) cases with 35 HeartWare HVAD (Centrifugal pump, Medtronic, MN, USA) cases, and reported that the incidence of mild or greater AI was 43.1% in HeartMate II vs. 65.7% in HeartWare HVAD (p = 0.035, without baseline adjustment), during median follow-up of 527 days (37). Malic et al. compared 270 HeartMate II cases with 121 HeartMate 3 (Abbott, MN, USA), and reported that the cumulative incidence of mild or greater AI was 11.3% in HeartMate II vs. 8.4% in HeartMate 3 (p = 0.68, with baseline adjustment), at 1 year after VAD implantation (39). Finally, Jimenez Contreras et al. compared 562 HeartMate II cases with 300 HeartMate 3 cases, and reported that the incidence of moderate or severe AI was 17.0% in HeartMate II vs. 9.9% in HeartMate 3 at 6 months after VAD implantation. The multivariable Cox regression analysis demonstrated that the adjusted HRs of moderate or severe AI in HeartMate 3, as compared to HeartMate II, was 0.624 (p = 0.0537, 95% CI 0.386–1.008) (40). More recently, Uriel et al. presented based on MOMENTUM 3 pivotal trial (42), that the incidence of moderate or severe AI was 11.5% in HeartMate II vs. 5.6% in HeartMate 3 at 2 years after VAD implantation and the HRs of moderate or severe AI in HeartMate 3, as compared to HeartMate II, was 0.35 (p = 0 < 0.01, 95% CI 0.20–0.59) in their randomized study (46), which could be promising.
Thus, there are several conflicting studies to each other, regarding the risk factors of significant AI and its effect on mortality. However, given the results shown in the registry analysis (1), it would be reasonable to consider AV intervention at the time of CF-LVAD implantation in a patient with significant, or greater than mild, AI.
Concomitant intervention on the aortic valve at CF-LVAD implantation
Should we intervene on the AV with mild AI at CF-LVAD implantation?
Given these clinical impacts of late AI on the prognosis, some clinicians would advocate concomitant intervention on the AV at the time of CF-LVAD implantation, especially in patients of destination therapy. According to the recent ISHLT guideline, greater than mild AI (assessed by echocardiography with appropriate afterload) should be addressed with either valve closure, repair, or replacement (8) (Class of recommendations: I, Level of evidence: B). However, there is no definite consensus on whether we should preventively intervene on a competent AV with mild or less AI at CF-LVAD implantation. The recent reports on the efficacy of concomitant AV interventions at the time of continuous-flow LVAD implantation were summarized in Table 1. Based on the IMACS registry analysis, Veenis et al. reported that, even after adjustment for other significant predictors, concomitant AVR remained an independent predictor for early (HR 1.23, 95% CI 1.04–1.45) and late (HR 1.48, 95% CI 1.15–1.89) mortality (47). It should be noted, however, that when they focused just on the patients with moderate to severe AI, concomitant AV intervention was not an independent predictor of mortality, indicating that this result would not preclude concomitant AVR or repair when the patient has significant AI at CF-LVAD implantation. Likewise, based on the IMACS registry analysis, Yalcin et al. demonstrated that concomitant AV surgery at CF-LVAD implantation was associated with increased risk of bleeding events (HR 1.158, 95% CI 1.018–1.317, p = 0.026), but not thromboembolic events (48). These findings may indicate that stringent criteria for a concomitant AV procedure at the time of VAD surgery may be warranted, especially in patients with only mild AI (47).
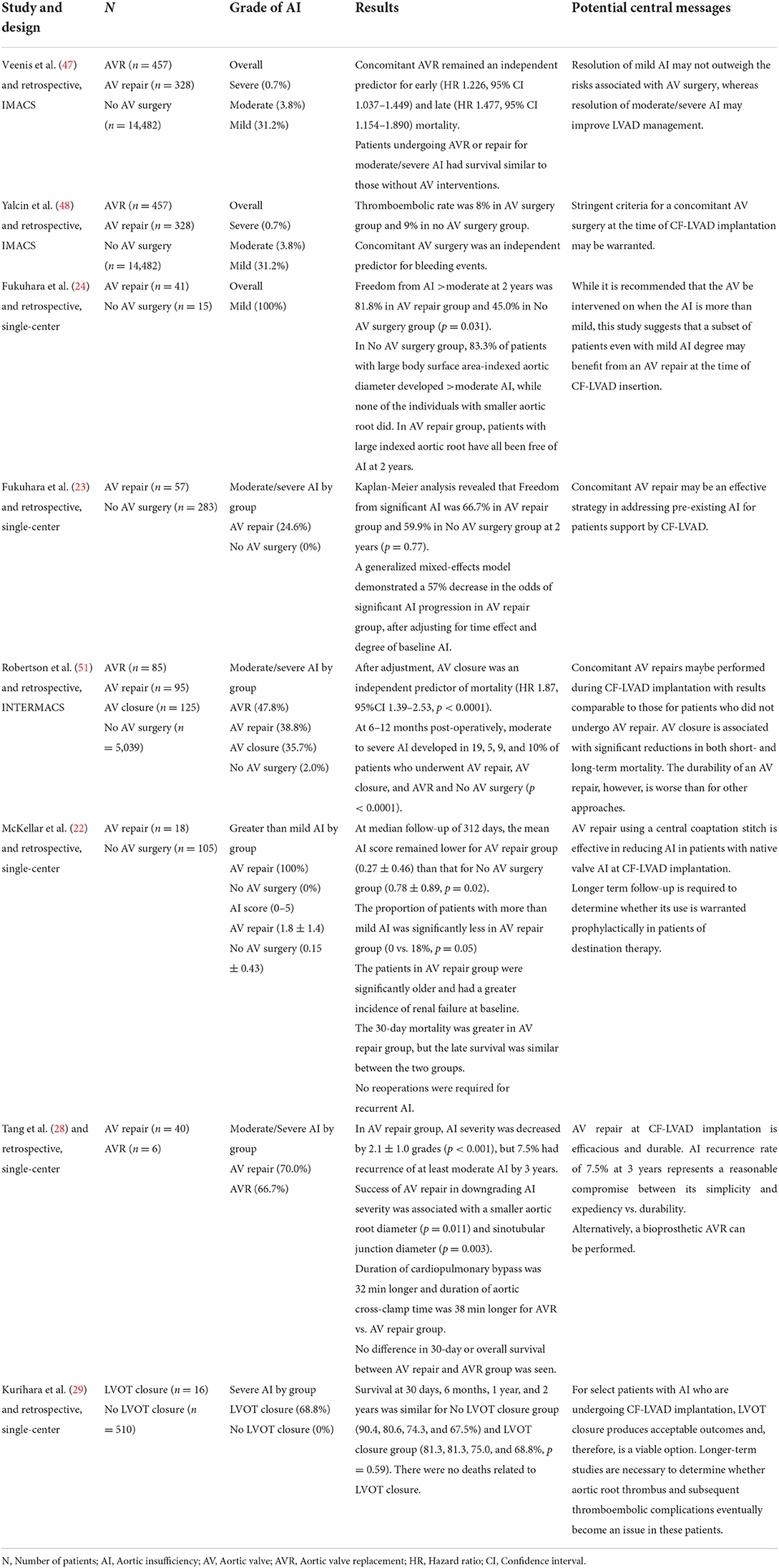
Table 1. Efficacy of concomitant aortic valve interventions at the time of continuous-flow LVAD implantation.
On the contrary, while there are several concerns for concomitant AV procedures, Tanaka et al. reported the detrimental impact of uncorrected mild AI at the time of CF-LVAD implantation on the non-survival outcomes (49). Although their analysis was a single-center one and did not demonstrate significant survival differences, after propensity-score matching, uncorrected mild AI was significantly associated with a higher risk of progression to moderate or greater AI (43.6% with the mean follow-up period of 2.3 ± 1.8 years) and worse NYHA functional class (p < 0.01). Notably, more CHF-related readmissions were observed in the mild AI group, as compared with no or trace AI (HR: 2.62, 95% CI 1.42–4.69) (49). Their results shed light on the need for proactive intervention on the mild AI at CF-LVAD implantation to improve the patients' quality of life in the future.
As for the surgical management of mild AI, Fukuhara et al. reported the efficacy of concomitant AV repair, or central AV closure as described later, at CF-LVAD implantation on the progression of AI (24). This study by Fukuhara is unique and worthwhile, in that they specifically focused on those with mild AI, to reveal whether we should intervene on the AV with mild AI simultaneously at VAD implant or not. In the AV repair group, freedom from AI greater than moderate at 2 years was 81.8% as compared to 45.0% in the AV non-repair group (p = 0.031), leading to no survival difference (24). Interestingly, their decision to perform a repair was made on the selected candidates with anticipated prolonged device support, such as destination therapy, bridge-to-transplant patients with large body size (body mass index >35) and bridge-to-transplant patients with blood type O (50). Given the recent refinements in surgical technique and expected longer waiting period in heart transplant candidates, the threshold of intervening on the AV would be gradually getting lower, especially in these selected candidates.
What is a desirable concomitant AV intervention at CF-LVAD implantation, AVR or AV repair?
An ideal AV procedure to treat AI, simultaneously with CF-LVAD implantation, is still controversial. Potential options could be AVR, AV repair, and AV closure. Based on the INTERMACS registry analysis, Robertson et al. reported that actuarial 1-year survival after CF-LVAD implantation was significantly worse in those who underwent concomitant AV closure (AV closure vs. AV repair vs. AVR, 63.2 vs. 76.8 vs. 71.8%, p = 0.0003) (51). As for the efficacy of AI treatment, they also demonstrated that AI recurrence rate (moderate to severe) at 6 to 12 months after the implantation was the highest in the AV repair group (AV closure vs. AV repair vs. AVR vs. No intervention, 5 vs. 19 vs. 9 vs. 10%, p < 0.0001) (51). Although Kurihara et al. reported the feasibility of AV (or left ventricular outlet) closure as a concomitant first-line procedure at CF-LVAD implantation, a disadvantage to close AV is that the patient will not be able to maintain hemodynamic stability if the device fails, and that bridge to recovery is no longer an option, as they admit (29). From these results, it would be reasonably safe to avoid AV closure from the first-line modalities.
While AVR with bioprosthetic valve contains a few issues, such as longer ischemic time, valve thrombosis, or commissural fusion (52), AV repair would be advantageous in terms of these issues, at the expense of potential recurrent AI in the future. As there are several other different techniques of AV repair in VAD-associated AI, for example aortic ring annuloplasty (25, 26), one of the most typical techniques among AV repair is a central AV closure (CAVC), or what we call Park's stitch, which was originally reported by Park et al. for a pulsatile LVAD in 2004 (21). This is basically a technique for central AI without any structural problems on the AV, by putting a simple coaptation stitch with a pledged supported 4-0 polypropylene sutures to approximate the fibrous nodules of Arantius. As compared to AV closure, the benefit of CAVC is that AV is still able to open for ejection, even though the effective orifice area is diminished. CAVC has the potential to be the ideal technique because it is inexpensive, quick, and simple to perform, and might not have the same degenerative potential as biologic valve prostheses. In 2014, Park's group first published its efficacy in CF-LVADs (22). They conducted a concomitant CAVC at the time of CF-LVAD implantation in 18 patients, those with greater than mild AI at baseline. Amazingly, among all the 18 patients, the grades of AI were mild or less at 2 years after CF-LVAD implantation (22).
A largest single-center experience of AV repair by central AV closure at VAD implantation is reported by Fukuhara and colleagues (23). They conducted concomitant central AV closures in 57 patients at the time of CF-LVAD implantation and its efficacy was compared with 283 patients those who underwent CF-LVAD implantation without central AV closures. Although Fukuhara et al. did not find any significant survival differences between the groups, their generalized mixed-effects model demonstrated a 57% decrease in the odds of significant AI progression among those who underwent the central AV closure as a concomitant procedure, after adjusting for time effect and degree of baseline pre-existing AI (23).
Thus, while CAVC could potentially be a first-line treatment of AI at the time of CF-LVAD implantation, one of its major drawbacks is a recurrence of AI during follow-up. Although there are few studies directly comparing CAVC with AVR, Tang et al. conducted a retrospective analysis on the concomitant CAVCs (n = 40) and AVRs (n = 6) (28). The CAVC group yielded shorter ischemic and cardiopulmonary bypass time, however, 7.5% of CAVC patients had recurrence of at least moderate AI by 3 years. Although they did not find any survival difference between the groups, such a decision of CAVC or AVR, as a concomitant procedure at CF-LVAD implantation, should depend on each clinical context.
The effect of impella—Another indispensable consideration
In 2018, the new United Network for Organ Sharing (UNOS) donor heart allocation system commenced giving a priority to patients supported with non-dischargeable mechanical circulatory support (MCS) devices while awaiting heart transplantation, prompting temporary MCS devices in heart transplant centers being more frequently used in the United States (US) (53). The Impella device (Abiomed Inc, Danvers, MA, USA) is an axial-flow percutaneous ventricular assist device used in cases in cardiogenic shock (54), and the use of Impella is gradually increasing especially in the US, reflecting the forementioned updates in the UNOS criteria. As for those who are eligible for heart transplantation, recently they could be directly bridged to transplant with the Impella in the US, as a new status 2 category. However, those who are ineligible for transplant might need to undergo CF-LVAD implantation as destination therapy to survive, and there are several reports on the adverse impact of the Impella on the AV in such patients (55–58). In fact, just on the Impella support, an increase in AI grade was observed in 17.2% of patients with an event per support days of 0.03 (55). Such a potentially iatrogenic damage on the AV could be associated with AI development even after VAD implants. Rao et al. compared the development of de novo AI after CF-LVAD implantation between those who were on the Impella support and those not, concluding that mild or moderate de novo AI was observed in 82% of patients in the Impella group, as compared 43% in the non-Impella group (p = 0.038) (56). The pathophysiology of AI due to the Impella support is still unclear. Oishi et al. reported two cases of de novo moderate AI due to the Impella, both of which required concomitant CAVCs at the VAD implantation (57). They speculated, like CF-LVAD support, that the AV is not opening by the Impella support, making the pressure load on the AV greater and causing disorganization and remodeling of the valve (57). Thus, especially when the Impella was placed before CF-LVAD implantation, careful intraoperative observation of the AV is mandatory at VAD implant, of course after the Impella removal, to avoid future progression of Impella-induced de novo AI.
Preventive strategies of de novo AI after CF-LVAD implantation
Up to this point, we summarized the current updates of AI associated with CF-LVAD, regarding its pathophysiology, incidence, clinical significance, and its concomitant surgical treatment at the time of CF-LVAD implantation. Henceforth, we moved on to the preventive strategies of de novo AI after VAD implants (14), followed by the options of late intervention on the AV.
Since continuous closure of the AV is reported as one of the major risk factors of de novo AI (17, 37, 59, 60), pump speed optimization to maintain AV opening would be one of the key aspects in the prevention of de novo AI (16). Jorde et al. demonstrated the efficacy of speed optimization study, or right heart catheter pressure study with transthoracic echocardiography with different pump speed before discharge, on the prevention of de novo AI (59). They conducted this optimization in 29 patients, and they found only 1 patient developed greater than mild AI during a median follow-up time of 205 days (59). In contrast, without this optimization study, 20 out of 62 patients developed greater than mild AI during a median follow-up time of 265 days. Jorde et al. concluded that their speed optimization study before discharge was significantly associated with reduced risk of de novo AI after CF-LVAD implantation (HR 11.2, 95% CI 4.6–27.4, p = 0.003) (59). Based on their report, such a speed optimization study is now routinely performed before discharge in some VAD-implant centers.
Another key aspect in the prevention of de novo AI could be afterload optimization. In fact, in non-VAD general populations, elevated systolic pressure is known to be associated with increased risk of valvular heart disease including AI (61, 62). Mechanistic evidence for the potential causal role of high blood pressure on de novo AI is unclear, although some speculate that high blood pressure causes abnormally high tensile stress on the AV, which can lead to endothelial injury or disruption (62). Like general populations, blood pressure management is an essential part of the routine care of CF-LVAD patients, especially for the prevention of thromboembolic events and de novo AI. Patil et al. reported that systolic blood pressure at 3 months after CF-LVAD implantation was an independent predictor of more than mild de novo AI, as well as aortic valve closure and longer support durations (60). However, in other studies, such a significant association between blood pressure in CF-LVAD patients and AI development was not observed (17, 59). Table 2 shows the summary of suggested medical and surgical interventions to treat AI in the patients support by CF-LVAD. Thus, so far there is no established strategies that can perfectly prevent the progression of AI. Once de novo AI in CF-LVAD patients becomes significant, next we need to consider when to intervene.
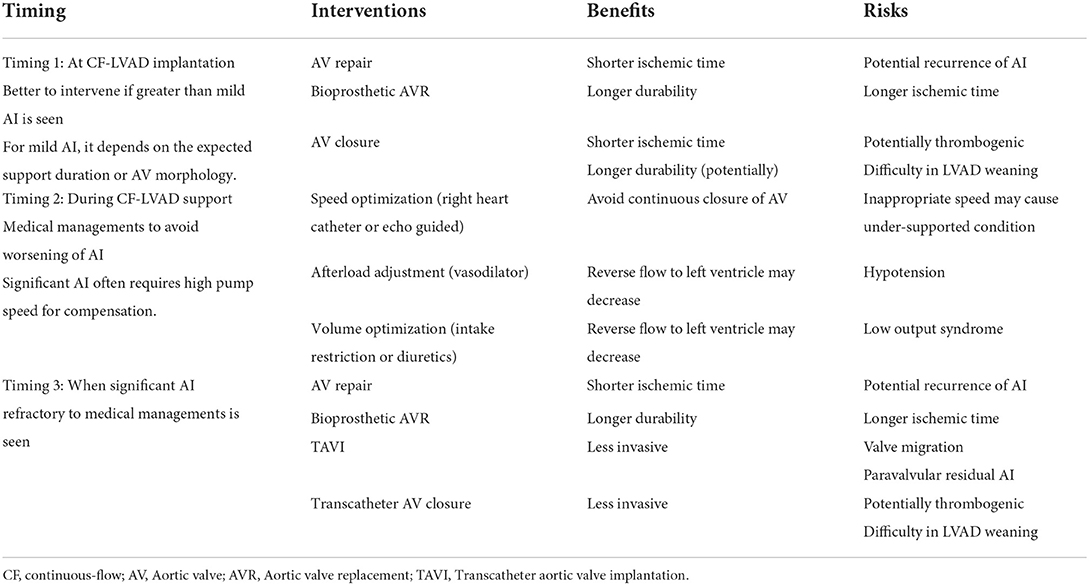
Table 2. Summary of suggested medical and surgical interventions to treat aortic insufficiency in patients support by continuous-flow LVAD.
Late intervention on the aortic valve
Even today, there is no definite consensus on when to intervene significant de novo AI after CF-LVAD implantation (16). Clinically, severe AI does not necessarily result in heart failure or elevated filling pressures. First-line medical treatment of de novo AI could be diuretics and vasodilators to decrease congestion and control blood pressure. However, once the patient becomes symptomatic because of significant AI, he or she surely needs to undergo right heart catheter study with simultaneous echocardiography for speed optimization (63). An increase in pump speed might be considered to improve cardiac output and end-organ perfusion, but this is at the expense of worsening AI. In general, this speed optimization for significant AI is only palliative and effective in the short term. Even today, there is no clear recommendation regarding the most pertinent surgical or interventional options to treat such patients. If the patient is eligible for heart transplantation, upgrading on the waiting list could be considered in some countries. Other potential treatment modalities are like the ones mentioned previously at the concomitant procedures with CF-LVAD implantation (15). Those are bioprosthetic AVR, CAVC, and surgical AV closure. In the future, total artificial heart could be another choice (64). Since these options requires redo-sternotomy in such a high-risk patient with elevated filling pressures due to significant AI, TAVI or trans-catheter device closure of AV can also be a reasonable select for de novo AI.
What is a desirable secondary AV intervention for late de novo AI during CF-LVAD support, AVR, AV repair, or else?
To the best of our knowledge, prospective studies on the efficacy of bioprosthetic AVR or AV repair for late de novo AI is quite limited, probably because of the following three reasons: (1) we currently tend to intervene on the AV more aggressively at the time of CF-LVAD implantation and the need of late AV intervention is decreasing, (2) for late de novo AI, less invasive procedures, such as TAVI or percutaneous closure, are more likely to be conducted instead of surgical interventions, and (3) In some countries, urgent heart transplantation is now becoming a feasible option to deal with de novo significant AI. For these reasons, there is no definite agreement on an ideal secondary AV intervention, and the decision should depend on each clinical scenario.
Nonetheless, AVR with bioprosthetic valve could be a gold standard therapy of de novo AI in VAD patients, especially when significant morphological change in the AV is observed. However, this procedure necessitates longer ischemic time and decent exposure of the aortic root, as compared to AV repair, which may raise a concern for postoperative right ventricular dysfunction in these high-risk candidates (19). We found two case series reports on the secondary AVR for late de novo AI (19, 20). Firstly, Atkins et al. reported that 6 out of 225 CF-LVAD patients developed de novo severe AI accompanied by heart failure, and for these 6 patients, they conducted 1 AVR with bioprosthetic valve, 1 Dacron patch closure, 2 aortic valve repair, and 2 TAVIs, one of which required revision by open surgery for AVR (20). Among these 6 patients, while 5 patients experienced significant improvement in functional capacity and symptom, 1 patient who underwent AVR unfortunately passed away postoperatively secondary to multiorgan failure and sepsis (20). Secondly, Gyoten et al. reported the similar case series of late AVR for de novo AI (19). They performed a total of 792 CF-LVAD implantations during the study period, and among them, 6 AVRs were performed for late severe AI, all of which were successfully done. However, 4 patients required temporary right ventricular assist devices, and 3 of them necessitated urgent heart transplantation to survive right heart failure. Judging from these two reports by Atkins and Gyoten (19, 20), secondary AVR for de novo AI in CF-LVAD patients is surely a procedure with considerable surgical risks.
A next question here would be whether AV repair can be a satisfactory alternative of AVR, with less risks and comparable outcomes. As far as our investigation, unfortunately, there is few reports on the efficacy of AV repair for late de novo AI. Our group previously published one case report of AV repair, or CAVC, for late de novo AI in a CF-LVAD patient, which was quite successful (27). Certainly, AV repair is less invasive as compared to AVR, in that it merely needs shorter ischemic time and less dissection around the aortic root, at the expense of potential AI recurrence in the future. Although we do not find the evidence on long-term outcomes after secondary AV repair for late de novo AI, based on the AI recurrence rate after concomitant AV repair at CF-LVAD implantation (23, 24, 51), clinical utility of AV repair could be similar to AVR.
Apart from the clinical case series above, there is a registry database analysis on the risk of AVR in CF-LVAD patients. Zaidi et al. reported the survival outcomes of AVR and TAVI late after CF-LVAD implantations, using the Nationwide Readmission Database in the US (7). Although they did not refer to the efficacy of AVR or TAVI in terms of controlling AI grade, they demonstrated in-hospital mortality was significantly higher in the AVR group than the TAVI group (42.3 vs. 6.4%, adjusted OR 10.4, 95% CI 1.37–79.5, p = 0.02), warranting a prudent judgement on the indication of surgical AVR for late de novo AI. Additionally, Doi et al. reported a case of commissural fusion after bioprosthetic AVR after CF-LVAD implantation, casting doubt on bioprosthetic AVR as a desirable option, as compared to AV repair (52). Right ventricular failure due to longer ischemic time is another non-negligible concern, as Gyoten et al. reported (19). Based on these data, the decision of AVR, AV repair, or other AV interventions should be tailored by case-by-case basis, considering surgical risks, right ventricular functions, AV morphologies, and expected support time.
TAVI is less invasive, but still not a promising option
Another option for treating AI could be TAVI. Although TAVI is not used routinely as a treatment option for severe AI, as of the year 2022, an international multicenter registry data already demonstrated its feasibility and efficacy in non-VAD patients, especially with new-generation devices (65). However, when it comes to the AI on CF-LVAD patients, only a few case series with very limited sample size (30, 32, 33, 66) or single case reports (67–72) are found. Yehya et al. conducted a TAVI in 9 CF-LVAD patients for severe AI (30). They reported all the 9 patients were discharged home and 8 patients were alive at 6 months. Five procedural complications were found, which are two valve migrations, one retroperitoneal hematoma, one groin hematoma, and one femoral pseudoaneurysm (30). As for two cases complicated with valve migrations, they used CoreValve 31 mm (Medtronic, MN, USA), one of the prior generation devices. Technically, most of TAVI devices are not initially designed to place on the dilated annulus in such AI patients. As Yehya et al. admits, in AI cases the lack of significant annular calcification to serve as an anchor for the valve can pose a technical challenge while increasing the risk of valve migration and lack of stability (30). In fact, even in the centers of excellence with new-generation devices, the second valve implantations were required in 12.7% of pure AI patients who underwent TAVI (65). Additionally, suction from the in-situ CF-LVAD may disturb prosthesis deployment and increase the risk of prosthesis migration and still there is no consensus on how to optimize pump speed to prevent valve migration while deploying the device (69). Hopefully, these issues may be partly addressed by the devices technically designed to place on the annulus without significant calcification (31, 73), such as JenaValve (JenaValve Technology, Munich, Germany) (70)and J-valve (JC Medical, Suzhou, China).
There are three other case series regarding TAVI for AI in CF-LVAD patients. First one is by Belkin et al., reporting 7 patients underwent 9 attempted TAVI procedures (33). Unfortunately, two patients expired within the first day for cardiogenic shock due to inadequate valve fixation and severe paravalvular leakage. Five patients out of 7 (71%) survived over median follow-up of 9 months. It is noteworthy that they demonstrated significant improvements in the right ventricular function, as well as the degree of AI (33). Second one is by Gondi et al., reporting 11 patients underwent TAVI. Like the report by Belkin et al., one died during the procedure from ventricular fibrillation associated with valve migration and one died 19 days after the procedure for persistent shock. Eight patients out of 11 (73%) were alive at 12 months, and all survivors had improvement in the grade of AI and NYHA class (66). Third one is by Dhillon et al., reporting 4 patients underwent TAVI (32). One valve migration occurred out of 4 cases, which required a rescue valve-in-valve procedure. Although all the 4 patients were once successfully discharged home, 3 patients (75%) expired at 10 days, 2 months, and 3 months after the procedure, by congestive heart failure, septic shock, and LVAD thrombosis, respectively (32). These data might indicate that their TAVI candidates could have been ineligible for redo surgical intervention, just because too sick at baseline, and accordingly their outcomes after TAVI, possibly a palliative option, was still quite poor. Thus, TAVI could be a reasonable option for the treatment of AI in selected CF-LVAD cases, however, prospective studies with larger sample size are needed to assess the durability and long-term efficacy of this procedure, in addition to its technical refinements.
Trans-catheter closure of the aortic valve; is a last option to consider?
As mentioned previously, concomitant AV closure at CF-LVAD implantation was associated with increased risk of mortality (51). Moreover, it also contains ineluctable drawbacks, such as risk of sudden death if the device fails, and difficulty in CF-LVAD weaning even when recovery is an option (29). In this sense, AV closure, surgical or trans-catheter one, could be a last option to consider. However, same as TAVI, for those who cannot tolerate invasive open surgery, such as old CF-LVAD patients of destination therapy, trans-catheter closure of the AV might be a palliative option to treat AI under selected circumstances. Retzer et al. reported the efficacy of trans-catheter AV closure using an Amplatzer Multi-Fenestrated Septal Occluder “Cribriform” device to close the AV of CF-LVAD patients (34). Notably, technical success was accomplished in 100% of patients. However, 6-month survival rate was only 30%, reflecting pre-procedural co-morbidities such as right ventricular failure. Phan et al. conducted a systematic review and meta-analysis on the outcomes after percutaneous trans-catheter interventions for AI in CF-LVAD patients (35). They included 8 cases of TAVI and 21 cases of trans-catheter AV closure, concluding that both procedures were effective in reducing the AI grade. Nonetheless, while 20 months survival was ~35% in the TAVI group, it was zero in the trans-catheter AV closure group. These data might indicate that survival outcomes after trans-catheter AV closure are unsatisfactory. Therefore, this option might not be the first-line treatment of VAD-associated AI, especially in young and healthy candidates. Furthermore, the ethical dilemma of AV closure with considerations of CF-LVAD withdrawal is another important consideration. Even on the appropriate level of sedation, sudden termination of pump rotation may lead to immediate death associated with acute pulmonary congestion in the patients with AV closure. In view of comfort care at terminal stage, we cannot overlook such an ethical drawback of AV closure.
Conclusions
The present review summarized current updates on CF-LVAD associated AI, in terms of its pathophysiology, incidence, clinical impacts on outcomes, prevention, and surgical or trans-catheter interventions. As a concomitant procedure with CF-LVAD implantation, current guidelines are recommending AV repair or AVR for greater than mild AI, which is well-supported (15). For mild or less AI at VAD implants, the decision to intervene on the AV should be tailored by case-by-case basis, considering patients' co-morbidities, surgical risks, right ventricular functions, AV morphologies, and expected support time. Correcting mild AI during CF-LVAD implantation may be reasonable in destination therapy patients. As for the managements of late de novo AI, still there is no clear consensus on the timing of intervention or the choice of treatment modality. Clearly, symptomatic severe AI in a CF-LVAD patient needs to be addressed, either surgically or percutaneously. Hopefully in the future, TAVI would become a first-line treatment of late de novo AI in CF-LVAD patients, after technical refinements and device improvements. Despite the scarcity of established evidence so far, our continuing efforts are imperative to develop new insights in the future, overcoming this scabrous clinical entity during CF-LVAD support.
Author contributions
Both authors listed have made a substantial, direct, and intellectual contribution to the work and approved it for publication.
Conflict of interest
The authors declare that the research was conducted in the absence of any commercial or financial relationships that could be construed as a potential conflict of interest.
Publisher's note
All claims expressed in this article are solely those of the authors and do not necessarily represent those of their affiliated organizations, or those of the publisher, the editors and the reviewers. Any product that may be evaluated in this article, or claim that may be made by its manufacturer, is not guaranteed or endorsed by the publisher.
Abbreviations
ISHLT, international society for heart and lung transplantation; INTERMACS, interagency registry for mechanically assisted circulatory support; AV, aortic valve; AVR, aortic valve replacement; HR, hazard ratio; OR, odds ratio; CI, confidence interval; NYHA, New York Heart Association; CHF, congestive heart failure; CAVC, central aortic valve closure.
References
1. Truby LK, Garan AR, Givens RC, Wayda B, Takeda K, Yuzefpolskaya M, et al. Aortic insufficiency during contemporary left ventricular assist device support: analysis of the INTERMACS registry. JACC Heart Fail. (2018) 6:951–60. doi: 10.1016/j.jchf.2018.07.012
2. Imamura T, Narang N, Kim G, Nitta D, Fujino T, Nguyen A, et al. Impact of worsening of aortic insufficiency during HeartMate 3 LVAD support. Artif Organs. (2021) 45:297–302. doi: 10.1111/aor.13825
3. Imamura T, Narang N, Kim G, Nitta D, Fujino T, Nguyen A, et al. Aortic insufficiency during HeartMate 3 left ventricular assist device support. J Card Fail. (2020) 26:863–9. doi: 10.1016/j.cardfail.2020.05.013
4. Auvil B, Chung J, Ameer A, Han J, Helmers M, Birati E, et al. Moderate aortic insufficiency with a left ventricular assist device portends a worse long-term survival. ASAIO J. (2020) 66:780–5. doi: 10.1097/MAT.0000000000001071
5. Kagawa H, Aranda-Michel E, Kormos RL, Keebler M, Hickey G, Wang Y, et al. Aortic insufficiency after left ventricular assist device implantation: predictors and outcomes. Ann Thorac Surg. (2020) 110:836–43. doi: 10.1016/j.athoracsur.2019.12.030
6. Toda K, Fujita T, Domae K, Shimahara Y, Kobayashi J, Nakatani T. Late aortic insufficiency related to poor prognosis during left ventricular assist device support. Ann Thorac Surg. (2011) 92:929–34. doi: 10.1016/j.athoracsur.2011.04.115
7. Zaidi SH, Minhas AMK, Sagheer S, ManeshGangwani K, Dani SS, Goel SS, et al. Clinical outcomes of transcatheter aortic valve replacement (TAVR) vs. Surgical aortic valve replacement (SAVR) in patients with durable left ventricular assist device (LVAD). Curr Probl Cardiol. (2022) 47:101313. doi: 10.1016/j.cpcardiol.2022.101313
8. Kirklin JK, Pagani FD, Goldstein DJ, John R, Rogers JG, Atluri P, et al. American association for thoracic surgery/international society for heart and lung transplantation guidelines on selected topics in mechanical circulatory support. J Thorac Cardiovasc Surg. (2020) 159:865–96. doi: 10.1016/j.jtcvs.2019.12.021
9. Yoshida S, Toda K, Miyagawa S, Yoshikawa Y, Hata H, Yoshioka D, et al. Impact of turbulent blood flow in the aortic root on de novo aortic insufficiency during continuous-flow left ventricular-assist device support. Artif Organs. (2020) 44:883–91. doi: 10.1111/aor.13671
10. Kainuma A, Itatani K, Hayashi H, Kaku Y, Wu IY, Colombo PC, et al. Left ventricular assist device support-induced alteration of mechanical stress on aortic valve and aortic wall. ASAIO J. (2022) 68:516–23. doi: 10.1097/MAT.0000000000001522
11. Nishida H, Song T, Onsager D, Nguyen A, Grinstein J, Chung B, et al. Proximal ascending aorta size is associated with the incidence of de novo aortic insufficiency with left ventricular assist device. Heart Vessels. (2022) 37:647–53. doi: 10.1007/s00380-021-01946-4
12. Fine NM, Park SJ, Stulak JM, Topilsky Y, Daly RC, Joyce LD, et al. Proximal thoracic aorta dimensions after continuous-flow left ventricular assist device implantation: longitudinal changes and relation to aortic valve insufficiency. J Heart Lung Transplant. (2016) 35:423–32. doi: 10.1016/j.healun.2015.10.029
13. Kasinpila P, Kong S, Fong R, Shad R, Kaiser AD, Marsden AL, et al. Use of patient-specific computational models for optimization of aortic insufficiency after implantation of left ventricular assist device. J Thorac Cardiovasc Surg. (2021) 162:1556–63. doi: 10.1016/j.jtcvs.2020.04.164
14. Cowger J, Rao V, Massey T, Sun B, May-Newman K, Jorde U, et al. Comprehensive review and suggested strategies for the detection and management of aortic insufficiency in patients with a continuous-flow left ventricular assist device. J Heart Lung Transplant. (2015) 34:149–57. doi: 10.1016/j.healun.2014.09.045
15. Goodwin ML, Bobba CM, Mokadam NA, Whitson BA, Essandoh M, Hasan A, et al. Continuous-flow left ventricular assist devices and the aortic valve: interactions, issues, and surgical therapy. Curr Heart Fail Rep. (2020) 17:97–105. doi: 10.1007/s11897-020-00464-0
16. Noly PE, Pagani FD, Noiseux N, Stulak JM, Khalpey Z, Carrier M, et al. Continuous-flow left ventricular assist devices and valvular heart disease: a comprehensive review. Can J Cardiol. (2020) 36:244–60. doi: 10.1016/j.cjca.2019.11.022
17. Gasparovic H, Kopjar T, Saeed D, Cikes M, Svetina L, Petricevic M, et al. Novo aortic regurgitation after continuous-flow left ventricular assist device implantation. Ann Thorac Surg. (2017) 104:704–11. doi: 10.1016/j.athoracsur.2017.01.114
18. Bouabdallaoui N, El-Hamamsy I, Pham M, Giraldeau G, Parent MC, Carrier M, et al. Aortic regurgitation in patients with a left ventricular assist device: a contemporary review. J Heart Lung Transplant. (2018) 37:1289–97. doi: 10.1016/j.healun.2018.07.002
19. Gyoten T, Morshuis M, Fox H, Deutsch MA, Hakim-Meibodi K, Schramm R, et al. Secondary aortic valve replacement in continuous flow left ventricular assist device therapy. Artif Organs. (2021) 45:736–41. doi: 10.1111/aor.13906
20. Atkins BZ, Hashmi ZA, Ganapathi AM, Harrison JK, Hughes GC, Rogers JG, et al. Surgical correction of aortic valve insufficiency after left ventricular assist device implantation. J Thorac Cardiovasc Surg. (2013) 146:1247–52. doi: 10.1016/j.jtcvs.2013.05.019
21. Park SJ, Liao KK, Segurola R, Madhu KP, Miller LW. Management of aortic insufficiency in patients with left ventricular assist devices: a simple coaptation stitch method (Park's stitch). J Thorac Cardiovasc Surg. (2004) 127:264–6. doi: 10.1016/s0022-5223(03)01301-1
22. McKellar SH, Deo S, Daly RC, Durham LA. 3rd, Joyce LD, Stulak JM, Park SJ. Durability of central aortic valve closure in patients with continuous flow left ventricular assist devices. J Thorac Cardiovasc Surg. (2014) 147:344–8. doi: 10.1016/j.jtcvs.2012.09.098
23. Fukuhara S, Takeda K, Chiuzan C, Han J, Polanco AR, Yuzefpolskaya M, et al. Concomitant aortic valve repair with continuous-flow left ventricular assist devices: results and implications. J Thorac Cardiovasc Surg. (2016) 151:201–9. doi: 10.1016/j.jtcvs.2015.09.128
24. Fukuhara S, Ikegami H, Polanco AR, Song JJ, Han J, Takeda K, et al. Concomitant repair for mild aortic insufficiency and continuous-flow left ventricular assist devices. Eur J Cardiothorac Surg. (2017) 52:1062–8. doi: 10.1093/ejcts/ezx150
25. Barac YD, Castleberry AW, Patel CB, McCartney SL, Schroder JN. Aortic valve ring annuloplasty is an option in left ventricular assist device patients. J Thorac Cardiovasc Surg. (2019) 157:e381–3. doi: 10.1016/j.jtcvs.2018.10.149
26. Han JJ, Atluri P. Commentary: when less is more: is valve repair the optimal intervention for aortic insufficiency at time of ventricular assist device implantation? J Thorac Cardiovasc Surg. (2019) 157:e385–6. doi: 10.1016/j.jtcvs.2018.11.044
27. Itoda Y, Nawata K, Yamauchi H, Kinoshita O, Kimura M, Ono M. Central aortic valve closure successfully treated aortic insufficiency of the patient with Jarvik 2000 continuous flow left ventricular assist device: a case report. J Artif Organs. (2017) 20:99–101. doi: 10.1007/s10047-016-0929-2
28. Tang PC, Sarsour N, Haft JW, Romano MA, Konerman M, Colvin M, et al. Aortic valve repair versus replacement associated with durable left ventricular assist devices. Ann Thorac Surg. (2020) 110:1259–64. doi: 10.1016/j.athoracsur.2020.01.015
29. Kurihara C, Cohn WE, Kawabori M, Sugiura T, Civitello AB, Morgan JA. Long-term continuous-flow left ventricular assist device support after left ventricular outflow tract closure. ASAIO J. (2019) 65:558–64. doi: 10.1097/MAT.0000000000000856
30. Yehya A, Rajagopal V, Meduri C, Kauten J, Brown M, Dean L, et al. Short-term results with transcatheter aortic valve replacement for treatment of left ventricular assist device patients with symptomatic aortic insufficiency. J Heart Lung Transplant. (2019) 38:920–6. doi: 10.1016/j.healun.2019.03.001
31. Fried JA, Nazif TM, Colombo PC. A new frontier for TAVR: aortic insufficiency in CF-LVAD patients. J Heart Lung Transplant. (2019) 38:927–9. doi: 10.1016/j.healun.2019.06.024
32. Dhillon AS, Jones BM, Hodson RW, Korngold EC. Transcatheter aortic valve replacement for severe aortic regurgitation in patients with a left ventricular assist device. J Invasive Cardiol. (2022) 34:E369–73.
33. Belkin MN, Imamura T, Fujino T, Kanelidis AJ, Holzhauser L, Ebong I, et al. Transcatheter aortic valve replacement in left ventricular assist device patients with aortic regurgitation. Figshare. (2020) 4:107–112. doi: 10.1080/24748706.2019.1706793
34. Retzer EM, Sayer GT, Fedson SE, Nathan S, Jeevanandam V, Friant J, et al. Predictors of survival following trans-catheter aortic valve closure for left ventricular assist device associated aortic insufficiency. Catheter Cardiovasc Interv. (2016) 87:971–9. doi: 10.1002/ccd.26280
35. Phan K, Haswell JM, Xu J, Assem Y, Mick SL, Kapadia SR, et al. Percutaneous transcatheter interventions for aortic insufficiency in continuous-flow left ventricular assist device patients: a systematic review and meta-analysis. ASAIO J. (2017) 63:117–22. doi: 10.1097/MAT.0000000000000447
36. Cecchi E, Giglioli C, Valente S, Lazzeri C, Gensini GF, Abbate R, et al. Role of hemodynamic shear stress in cardiovascular disease. Atherosclerosis. (2011) 214:249–56. doi: 10.1016/j.atherosclerosis.2010.09.008
37. Patil NP, Sabashnikov A, Mohite PN, Garcia D, Weymann A, Zych B, et al. De novo aortic regurgitation after continuous-flow left ventricular assist device implantation. Ann Thorac Surg. (2014) 98:850–7. doi: 10.1016/j.athoracsur.2014.05.030
38. Holley CT, Fitzpatrick M, Roy SS, Alraies MC, Cogswell R, Souslian L, et al. Aortic insufficiency in continuous-flow left ventricular assist device support patients is common but does not impact long-term mortality. J Heart Lung Transplant. (2017) 36:91–6. doi: 10.1016/j.healun.2016.07.018
39. Malick A, Ning Y, Kurlansky PA, Melehy A, Yuzefpolskaya M, Colombo PC, et al. Development of de novo aortic insufficiency in patients with HeartMate 3. Ann Thorac Surg. (2022) 114:450–6. doi: 10.1016/j.athoracsur.2021.08.074
40. Jimenez Contreras F, Mendiola Pla M, Schroder J, Bryner B, Agarwal R, Russell SD, et al. Progression of aortic valve insufficiency during centrifugal versus axial flow left ventricular assist device support. Eur J Cardiothorac Surg. (2022) 61:1188–96. doi: 10.1093/ejcts/ezac087
41. Rossi M, Serraino GF, Jiritano F, Renzulli A. Late de novo aortic regurgitation with the Jarvik 2000 Flowmaker(R) left ventricular assist device. Int J Artif Organs. (2012) 35:1080–2. doi: 10.5301/ijao.5000111
42. Mehra MR, Uriel N, Naka Y, Cleveland JC, Yuzefpolskaya M, Salerno CT, et al. A fully magnetically levitated left ventricular assist device - final report. N Engl J Med. (2019) 380:1618–1627. doi: 10.1056/NEJMoa1900486
43. Wieselthaler GM. G OD, Jansz P, Khaghani A, Strueber M, Investigators HC. Initial clinical experience with a novel left ventricular assist device with a magnetically levitated rotor in a multi-institutional trial. J Heart Lung Transplant. (2010) 29:1218–25. doi: 10.1016/j.healun.2010.05.016
44. Bhagra S, Bhagra C, Ozalp F, Butt T, Ramesh BC, Parry G, et al. Development of de novo aortic valve incompetence in patients with the continuous-flow HeartWare ventricular assist device. J Heart Lung Transplant. (2016) 35:312–9. doi: 10.1016/j.healun.2015.10.022
45. Saeed D, Westenfeld R, Maxhera B, Keymel S, Sherif A, Sadat N, et al. Prevalence of de novo aortic valve insufficiency in patients after heartware VAD implantation with an intermittent low-speed algorithm. ASAIO J. (2016) 62:565–70. doi: 10.1097/MAT.0000000000000391
46. Uriel N, Mehra MR. Incidence and clinical significance of aortic regurgitation in HeartMate 3 continuous flow left ventricular assist device recipients: an analysis from the momentum 3 trial portfolio. J Heart Lung Transplant. (2021) 40:S79.
47. Veenis JF, Yalcin YC, Brugts JJ, Constantinescu AA, Manintveld OC, Bekkers JA, et al. Survival following a concomitant aortic valve procedure during left ventricular assist device surgery: an ISHLT mechanically assisted circulatory support (IMACS) registry analysis. Eur J Heart Fail. (2020) 22:1878–87. doi: 10.1002/ejhf.1989
48. Yalcin YC, Veenis JF, Brugts JJ, Antonides CFJ, Veen KM, Muslem R, et al. Rate of thromboembolic and bleeding events in patients undergoing concomitant aortic valve surgery with left ventricular assist device implantation. Int J Cardiol. (2022) 359:39–45. doi: 10.1016/j.ijcard.2022.04.041
49. Tanaka Y, Nakajima T, Fischer I, Wan F, Kotkar K, Moon MR, et al. The impact of uncorrected mild aortic insufficiency at the time of left ventricular assist device implantation. J Thorac Cardiovasc Surg. (2020) 160:1490–500. doi: 10.1016/j.jtcvs.2020.02.144
50. Ando M, Takeda K, Kurlansky PA, Garan AR, Topkara VK, Yuzefpolskaya M, et al. Association between recipient blood type and heart transplantation outcomes in the United States. J Heart Lung Transplant. (2020) 39:363–70. doi: 10.1016/j.healun.2019.12.006
51. Robertson JO, Naftel DC, Myers SL, Prasad S, Mertz GD, Itoh A, et al. Concomitant aortic valve procedures in patients undergoing implantation of continuous-flow left ventricular assist devices: an INTERMACS database analysis. J Heart Lung Transplant. (2015) 34:797–805. doi: 10.1016/j.healun.2014.11.008
52. Doi A, Marasco SF, McGiffin DC. Is a bioprosthetic valve in the aortic position desirable with a continuous flow LVAD? J Card Surg. (2015) 30:466–8. doi: 10.1111/jocs.12541
53. Varshney AS, Berg DD, Katz JN, Baird-Zars VM, Bohula EA, Carnicelli AP, et al. Use of temporary mechanical circulatory support for management of cardiogenic shock before and after the united network for organ sharing donor heart allocation system changes. JAMA Cardiol. (2020) 5:703–8. doi: 10.1001/jamacardio.2020.0692
54. Panuccio G, Neri G, Macri LM, Salerno N, De Rosa S, Torella D. Use of impella device in cardiogenic shock and its clinical outcomes: a systematic review and meta-analysis. Int J Cardiol Heart Vasc. (2022) 40:101007. doi: 10.1016/j.ijcha.2022.101007
55. Hironaka CE, Ortoleva J, Zhan Y, Chen FY, Couper GS, Kapur NK, et al. The effects of percutaneous left ventricular assist device placement on native valve competency. ASAIO J. (2022) 68:541–6. doi: 10.1097/MAT.0000000000001529
56. Rao SD, Johnson B, Olia SE, Wald J, Medina V, Rame JE, et al. Treatment with impella increases the risk of de novo aortic insufficiency post left ventricular assist device implant. J Card Fail. (2020) 26:870–5. doi: 10.1016/j.cardfail.2020.06.014
57. Oishi H, Kondo T, Fujimoto K, Mutsuga M, Morimoto R, Hirano KI, et al. Aortic insufficiency associated with Impella that required surgical intervention upon implantation of the durable left ventricular assist device. J Artif Organs. (2020) 23:378–82. doi: 10.1007/s10047-020-01184-x
58. Ueda K, Yoshitani K, Hosotani S, Hayashi H, Fukushima S, Ohnishi Y. Aortic valve insufficiency after Impella device insertion that required aortic valve replacement after Heart Mate III left ventricular assist device implantation: a case report. J Surg Case Rep. (2021) 2021:rjab420. doi: 10.1093/jscr/rjab420
59. Jorde UP, Uriel N, Nahumi N, Bejar D, Gonzalez-Costello J, Thomas SS, et al. Prevalence, significance, and management of aortic insufficiency in continuous flow left ventricular assist device recipients. Circ Heart Fail. (2014) 7:310–9. doi: 10.1161/CIRCHEARTFAILURE.113.000878
60. Patil NP, Mohite PN, Sabashnikov A, Dhar D, Weymann A, Zeriouh M, et al. et al. Does postoperative blood pressure influence development of aortic regurgitation following continuous-flow left ventricular assist device implantation?dagger. Eur J Cardiothorac Surg. (2016) 49:788–94. doi: 10.1093/ejcts/ezv221
61. Nazarzadeh M, Pinho-Gomes AC, Smith Byrne K, Canoy D, Raimondi F, Ayala Solares JR, et al. Systolic blood pressure and risk of valvular heart disease: a mendelian randomization study. JAMA Cardiol. (2019) 4:788–95. doi: 10.1001/jamacardio.2019.2202
62. Rahimi K, Mohseni H, Kiran A, Tran J, Nazarzadeh M, Rahimian F, et al. Elevated blood pressure and risk of aortic valve disease: a cohort analysis of 54 million UK adults. Eur Heart J. (2018) 39:3596–603. doi: 10.1093/eurheartj/ehy486
63. Sujino Y, Kuroda K, Yoshitake K, Yagi N, Anegawa E, Mochizuki H, et al. Clinical potential of hemodynamic ramp test by simultaneous echocardiography and right heart catheterization for aortic insufficiency in a patient with continuous-flow left ventricular assist device. J Artif Organs. (2021) 24:265–8. doi: 10.1007/s10047-020-01210-y
64. Melton N, Soleimani B, Dowling R. Current role of the total artificial heart in the management of advanced heart failure. Curr Cardiol Rep. (2019) 21:142. doi: 10.1007/s11886-019-1242-5
65. Yoon SH, Schmidt T, Bleiziffer S, Schofer N, Fiorina C, Munoz-Garcia AJ, et al. Transcatheter aortic valve replacement in pure native aortic valve regurgitation. J Am Coll Cardiol. (2017) 70:2752–63. doi: 10.1016/j.jacc.2017.10.006
66. Gondi K, Tam M, Chetcuti SJ, Pagani FD, Grossman PM, Deeb GM, et al. Transcatheter aortic valve replacement for the treatment of left ventricular assist device-related aortic regurgitation. J Am Coll Cardiol. (2022) 79:707.
67. Bjelic M, Ayers B, Ling FS, Prasad SM, Gosev I. Transcatheter aortic valve replacement in left ventricular assist device patient-overcoming the complications with transapical approach and circulatory arrest. J Card Surg. (2021) 36:403–5. doi: 10.1111/jocs.15199
68. Rao SD, Jagasia D, Anwaruddin S, Birati EY. Transcatheter aortic valve replacement thrombosis in patient supported with durable left ventricular assist device. Catheter Cardiovasc Interv. (2020) 96:500–3. doi: 10.1002/ccd.28742
69. Chang HH, Chen PL, Chen YH, Leu HB, Kuo CC, Wu NY. Intraoperative ECMO assistance during TAVI for aortic insufficiency in an Asian patient with LVAD support. ESC Heart Fail. (2021) 8:3418–21. doi: 10.1002/ehf2.13426
70. Ranard LS, Kaple R, Khalique OK, Agarwal V, Bellumkonda L, Bonde P, et al. First transfemoral implantation of a novel transcatheter valve in an LVAD patient with aortic insufficiency. JACC Case Rep. (2021) 3:1806–10. doi: 10.1016/j.jaccas.2021.08.026
71. Rene AG, Desai N, Wald J, Rame JE, Frogel JK, Anwaruddin S. Transfemoral transcatheter aortic valve replacement with a self-expanding valve for severe aortic regurgitation in a patient with left ventricular assist device. J Card Surg. (2017) 32:741–5. doi: 10.1111/jocs.13238
72. Santini F, Forni A, Dandale R, Ribichini F, Rossi A, Franchi G, et al. First successful management of aortic valve insufficiency associated with HeartMate II left ventricular assist device support by transfemoral corevalve implantation: the columbus's egg? JACC Cardiovasc Interv. (2012) 5:114–5. doi: 10.1016/j.jcin.2011.10.009
Keywords: left ventricular assist device (LVAD), aortic insufficiency (AI), aortic valve replacement (AVR), aortic valve repair (AV repair), heart transplant (HTx)
Citation: Ando M and Ono M (2022) Concomitant or late aortic valve intervention and its efficacy for aortic insufficiency associated with continuous-flow left ventricular assist device implantation. Front. Cardiovasc. Med. 9:1029984. doi: 10.3389/fcvm.2022.1029984
Received: 28 August 2022; Accepted: 31 October 2022;
Published: 15 November 2022.
Edited by:
Paul C. Tang, University of Michigan, United StatesReviewed by:
Mahwash Kassi, Houston Methodist Hospital, United StatesSelim Krim, Ochsner Medical Center, United States
Copyright © 2022 Ando and Ono. This is an open-access article distributed under the terms of the Creative Commons Attribution License (CC BY). The use, distribution or reproduction in other forums is permitted, provided the original author(s) and the copyright owner(s) are credited and that the original publication in this journal is cited, in accordance with accepted academic practice. No use, distribution or reproduction is permitted which does not comply with these terms.
*Correspondence: Masahiko Ando, YW5kb21hLXN1ckBoLnUtdG9reW8uYWMuanA=