- 1Cedars-Sinai Medical Center, Smidt Heart Institute, Los Angeles, CA, United States
- 2Bruce Rappaport Faculty of Medicine, Technion Israel Institute of Technology, Haifa, Israel
- 3Internal Medicine, Kaiser Permanente Los Angeles Medical Center, Los Angeles, CA, United States
- 4Internal Medicine, University Hospitals Cleveland Medical Center, Case Western Reserve University School of Medicine, Cleveland, OH, United States
- 5School of Medicine, University of Illinois Chicago, Chicago, IL, United States
- 6Sackler School of Medicine, Tel Aviv University, Tel Aviv, Israel
- 7Department of Cardiology, Gunma University Hospital, Gunma, Japan
- 8Heart Valve Center, NYU Langone Health, New York City, NY, United States
Background: There is growing evidence of the safety of same-day discharge for low-risk conscious sedated TAVR patients. However, the evidence supporting the safety of early discharge following GA-TAVR with routine transesophageal echocardiography (TEE) is limited.
Aims: To assess the safety of early discharge following transcatheter aortic valve replacement (TAVR) using General Anesthesia (GA-TAVR) and identify predictors for patient selection.
Materials and methods: We used data from 2,447 TEE-guided GA-TAVR patients performed at Cedars-Sinai between 2016 and 2021. Patients were categorized into three groups based on the discharge time from admission: 24 h, 24–48 h, and >48 h. Predictors for 30-day outcomes (cumulative adverse events and death) were validated on a matched cohort of 24 h vs. >24 h using the bootstrap model.
Results: The >48 h group had significantly worse baseline cardiovascular profile, higher surgical risk, low functional status, and higher procedural complications than the 24 h and the 24–48 h groups. The rate of 30-day outcomes was significantly lower in the 24 h than the >48 h but did not differ from the 24–48 h (11.3 vs. 15.5 vs. 11.7%, p = 0.003 and p = 0.71, respectively). Independent poor prognostic factors of 30-day outcomes had a high STS risk of ≥8 (OR 1.90, 95% CI 1.30–2.77, E-value = 3.2, P < 0.001), low left ventricle ejection fraction of <30% (OR 6.0, 95% CI 3.96–9.10, E-value = 11.5, P < 0.001), and life-threatening procedural complications (OR 2.65, 95% CI 1.20–5.89, E-value = 4.7, P = 0.04). Our formulated predictors showed a good discrimination ability for patient selection (AUC: 0.78, 95% CI 0.75–0.81).
Conclusion: Discharge within 24 h following GA-TAVR using TEE is safe for selected patients using our proposed validated predictors.
Introduction
Transcatheter aortic valve replacement (TAVR) has become the most common procedure in the United States for symptomatic aortic stenosis (AS) patients across all risk profiles (1–3). Technological advancements and utilization of pre-procedural imaging for planning continues to improve TAVR outcomes. The operator’s familiarity aids in minimizing procedural complications and improves patient care (4, 5).
Despite the maturity of TAVR, only minimal changes in the length of hospitalization (LOS) have been noted over the years, with a wide variation among national and international centers (6–8). Unplanned 30-day hospital readmissions were directly associated with longer LOS and adversely impacted patient outcomes and health care costs (9, 10). However, these studies were not randomized or used propensity matched comparisons and may be subject to bias.
Numerous clinical and multimodal pathways were designed over the years to identify true low-risk patients for earlier and safe discharge, addressing known late complications such as bleeding, conduction disturbance, and acute kidney injury (7, 11, 12). In recent times, studies have shown that same-day discharge following TAVR, with conscious sedation, can be safe in select patient populations (13).
We hypothesized that discharging transfemoral-TAVR (TF-TAVR) patients performed under general anesthesia (GA-TAVR) using transesophageal echocardiography within 24 h of admission is safe and feasible and doesn’t pose a higher risk for major adverse events.
The study aims to assess the safety of early discharge following GA-TAVR and proposes risk-assessment predictors that would optimize patient selection for GA-TAVR.
Materials and methods
Study cohort and patients’ selection
We conducted a single-center retrospective study of 2,736 consecutive patients, with severe symptomatic AS, who underwent TAVR at Cedars-Sinai Medical Center between January 2016 and December 2021. A consensus decision of the multidisciplinary cardiac team determined the indication of TAVR for all patients and the interventional team and attending physicians determined the time of discharge.
Exclusion criteria included death during hospitalization, canceled or aborted procedure, utilization of non-transfemoral access sites, incomplete medical records, and if lost to follow up. Patients discharged to a rehabilitation center, skilled nurse facilities, and hospice were not excluded from the study to ensure real-life data. We used the data of 2,447 eligible patients to formulate a predictors equation and validated it on the entire cohort (Figure 1).
The study was approved by the Cedars-Sinai medical center institutional review board (IRB), which also waived the requirement to obtain informed consent due to the study’s retrospective nature.
Definitions
Acute kidney injury, bleeding, vascular complications, and procedure-related complications were defined according to the updated Valve Academic Research Consortium-3 consensus document (VARC-3) (14). We defined procedure-related life-threatening complications to include any of the following adverse events: aortic valve annulus rupture, aortic dissection, cardiac arrest, cardiac tamponade, coronary obstruction, valve migration, conversion to open- heart surgery, major bleeding, and major vascular complications. We followed the valve academic research consortium-3 for the clinical outcome to include composite events of all-cause non-cardiac readmission, heart failure readmission, valve re-intervention, THV thrombosis, myocardial infarction, and unplanned percutaneous coronary intervention (PCI), cerebrovascular accident (CVA)/transient ischemic attack (TIA), new-onset atrioventricular block and atrial arrhythmias, pacemaker implantation, major bleeding, and vascular complications, acute renal failure, infective endocarditis, and death (14).
Data collection
Demographic, procedural, and follow-up data were entered retrospectively by a dedicated team and extracted using the Cedars-Sinai electronic records systems (CS-link).
Statistical analysis
The data is presented as a number of patients and percentage (%) for categorical variables and a median (IQR, interquartile range) for continuous variables. Patient characteristics were compared among discharge times (<24 h, 24–48 h and >48 h) using a Kruskal-Wallis test, chi-square test or Fisher’s exact test as appropriate. Pairwise comparisons were further carried out to compare discharge <24 h with 24–48 h and >48 h, and family-wise error rate was adjusted for inflation due to multiple comparisons using the Bonferroni correction method. Outcomes were reported as those occurring during the index hospitalization (“in-hospital”) and 30 days post discharge (“30-day”) as a means to prevent data analysis bias and emphasize each component of the outcome.
Univariate and multivariable analyses of discharge times (<24 h vs. 24–48 h, and < 24 h vs. >48) and 30-day outcomes were performed using a logistic regression model. Covariates considered in multivariable analyses were chosen a priori including age, sex, body mass index (BMI), Society of Thoracic Surgeons (STS) risk score, Kansas City Cardiomyopathy Questionnaire (KCCQ), left ventricle ejection fraction (LVEF), severe mitral and tricuspid regurgitation, urgent procedure, bicuspid aortic valve, use of intra-aortic balloon pump (IABP), in-hospital complications, procedure-related life-threatening complications, the use of contrast volume, and hours of stay in the intensive cardiac care unit.
Variable selection was performed using a stepwise variable selection procedure based on Akaike Information Criterion (AIC) (15). The model with the minimum AIC was chosen for analysis in order to minimize the loss of information. A forward stepwise analysis was used and a probability entry threshold value of p < 0.001 was set for all variables. In multivariable analyses, multicollinearity was assessed by the variance inflation factor. The performance of the formulated predictors of 30-day outcomes was assessed with measures of discrimination and calibration (16). Discrimination was assessed with a receiver operating characteristic (ROC) curve along with the area under the ROC curve (AUC, c-statistic). The calibration of the formulated predictors was evaluated with calibration-in-the-large, and the calibration slope proposed by Cox (17). Internal validation was performed by estimating and correcting possible overfitting and optimism in the predictors performance estimates (e.g., optimism-corrected c-statistic) using the bootstrap method with 1,000 replicates (18–20).
To address confounding caused by differing baseline patient characteristics, we adopted the inverse propensity treatment weighting (IPTW) using propensity score–based matching (PSM) and a greedy matching strategy with full matching in a one-to-one ratio including statistically significant variables from the logistic regression model and non-significant variables that might be related to unrecorded selections factors adopted from a parsimonious model (21, 22). The covariates included in the IPTW were age, sex, STS score, KCCQ performance and LVEF.
All statistical analyses were performed using SAS 9.4 (SAS Institute, Inc., Cary, North Carolina) and R package version 4.0.5 (23) with two-sided tests at a significance level of 0.05.
Results
Study population
We used the data of 2,736 TAVR patients at Cedars-Sinai medical center from 2016 to December 2021. Patients were eligible for the study if they were 18 years of age and older, had TAVR using general anesthesia, and used a new generation of commercial balloon-expanding and self-expandable valves.
We excluded 32 patients due to incomplete records, 4 patients due to procedure cancelation or abortion, and 24 patients who were lost to follow-up at their 30-day postoperative visit. We also excluded 35 (1.2%) patients who died during hospitalization and 194 patients who underwent non-transfemoral TAVR. TAVR was guided by transesophageal echocardiography (TEE) under general anesthesia in all patients.
Among 2,447 eligible patients, 837 patients were discharged within 24 h of admission (“24 h” group), 713 patients were discharged within 24–48 h (“24–48 h” group), and 897 patients were discharged after 48 h (“>48 h” group). Data from eligible patients was used to formulate predictors for patients selection and further validated using the bootstrap technique (Figures 1, 2).
Patients baseline characteristics
24 h group vs. >48 h group
Complete baseline characteristics are shown in Table 1. The 24 h group was significantly younger (79 vs. 82 years, p < 0.001), compromised of fewer females (34 vs. 40%, p = 0.005), more commonly demonstrated bicuspid aortic valve morphology (15.8 vs. 5.6%, p < 0.001), and had a lower rate of comorbidities including hypertension, hyperlipidemia, diabetes mellitus, peripheral artery disease (PAD), anemia, chronic kidney disease, chronic lung disease, coronary artery disease, CVA, and TIA (significant p-value for all).
The >48 h group comprised of more patients with high surgical risk (STS ≥ 8), low KCCQ (KCCQ < 50), low mean LVEF (57 vs. 62%, p < 0.001), and severe mitral and tricuspid regurgitation (p < 0.001 for both). Furthermore, the >48 h group more often required the use of an intra-aortic balloon pump (IABP) (1.3 vs. 0.3%, p = 0.04), exhibited longer procedure and fluoroscopy times, and used more contrast volume (p < 0.001, p = 0.08, p < 0.001, respectively).
The 24–48 h group shares baseline characteristics of both groups and includes older patients, including more females, higher rates of comorbidities, higher surgical risk, and lower KCCQ score than the 24 h group, however less pronounced when compared to >48 h group (Table 1).
Procedure-related outcome
In-hospital complications and procedure-related life-threatening complications occurred more in the >48 h group (15.0 vs. 7.0%, and 4.2 vs. 1.4%, p < 0.001 and p = 0.001) than in the 24 h group contributing mostly to a higher rate of unplanned percutaneous coronary intervention (PCI) (1.4 vs. 0%, p = 0.001), CVA or TIA (3.0 vs. 0.1%, p = 0.001), complete atrioventricular heart block (CAVB) (3.3 vs. 0.2%, p < 0.001), pacemaker implantation (7.3 vs. 4.9%, p = 0.04) and major vascular complications (2.0 vs. 0.1%, p < 0.001) (Table 2).
Thirty-day outcome (death and 30-day cumulative adverse events)
Despite the higher risk profile and in-hospital complication rates within the 24–48 h group, the 30-day outcomes did not differ significantly when compared to the 24 h group (11.7 vs. 11.3%, p = 0.9) (Table 2). Young males with bicuspid valves who had minimal use of contrast volume and no procedural-related complications were more likely to be discharged within 24 h than 24–48 h (Table 3). The >48 h had a significantly higher rate of 30-day death (2.1 vs. 0.1%, p < 0.001) and cumulative adverse events when compared to the 24 h (15.4 vs. 11.3%, p = 0.03). Non-bicuspid patients with low BMI, high surgical risk (STS ≥ 8), a low KCCQ score (<50), low LVEF, and severe mitral regurgitation that had procedure-related complications, were more likely to be discharged more than 48 h after the procedure (Table 4).
Predictors for outcome
Our study shows that independent poor prognostic factors of 30-day outcomes were discharge time (OR 0.26, 95% CI 0.16–0.43, E-value = 7.15, P = < 0.001 for discharge within 24 h), high surgical risk ≥8 (OR 1.90, 95% CI 1.30–2.77, E-value = 3.21, P < 0.001), low LVEF < 30 (OR 6.00, 95% CI 3.96–9.10, E-value = 11.48, P < 0.001), and procedure-related life-threatening complications (OR 2.65, 95% CI 1.20–5.89, E-value = 4.74, P = 0.04). Although the associations were not statistically significant, the female gender shows an association with a 30-day outcome (OR 0.72, 95% CI 0.50–1.02, P = 0.06) (Table 5 and Figure 3). A probability formula with a 30-day outcome using the significant predictors demonstrated a good ability to discriminate between those with and without a 30-day outcome (apparent area under the curve: 0.80; 95% CI: 0.77–0.83) (Figure 3). The internal validation by the bootstrapping method on entire cohort study showed an AUC (optimism-corrected AUC) of 0.78 (95% CI 0.75–0.81), which is slightly lower than the apparent AUC as expected and is well calibrated (optimism-corrected calibration-in-the-large of −0.05; the slope of 0.97) (Table 6).
Inverse probability treatment weighting (IPTW)
We balanced age, sex, STS score, KCCQ performance score and LVEF in a full inverse probability treatment weighting (IPTW) using propensity matching (Figure 4). The matched cohort included 1,428 patients, 714 patients discharged within 24 h, and 714 after 24 h. A total of 1,017 patients were unmatched or discarded from the analysis. We excluded procedural complications from the logistics regression analysis to address the possibility of selection bias for early discharge and to adhere as much as possible to a prospective methodology reflecting real-life.
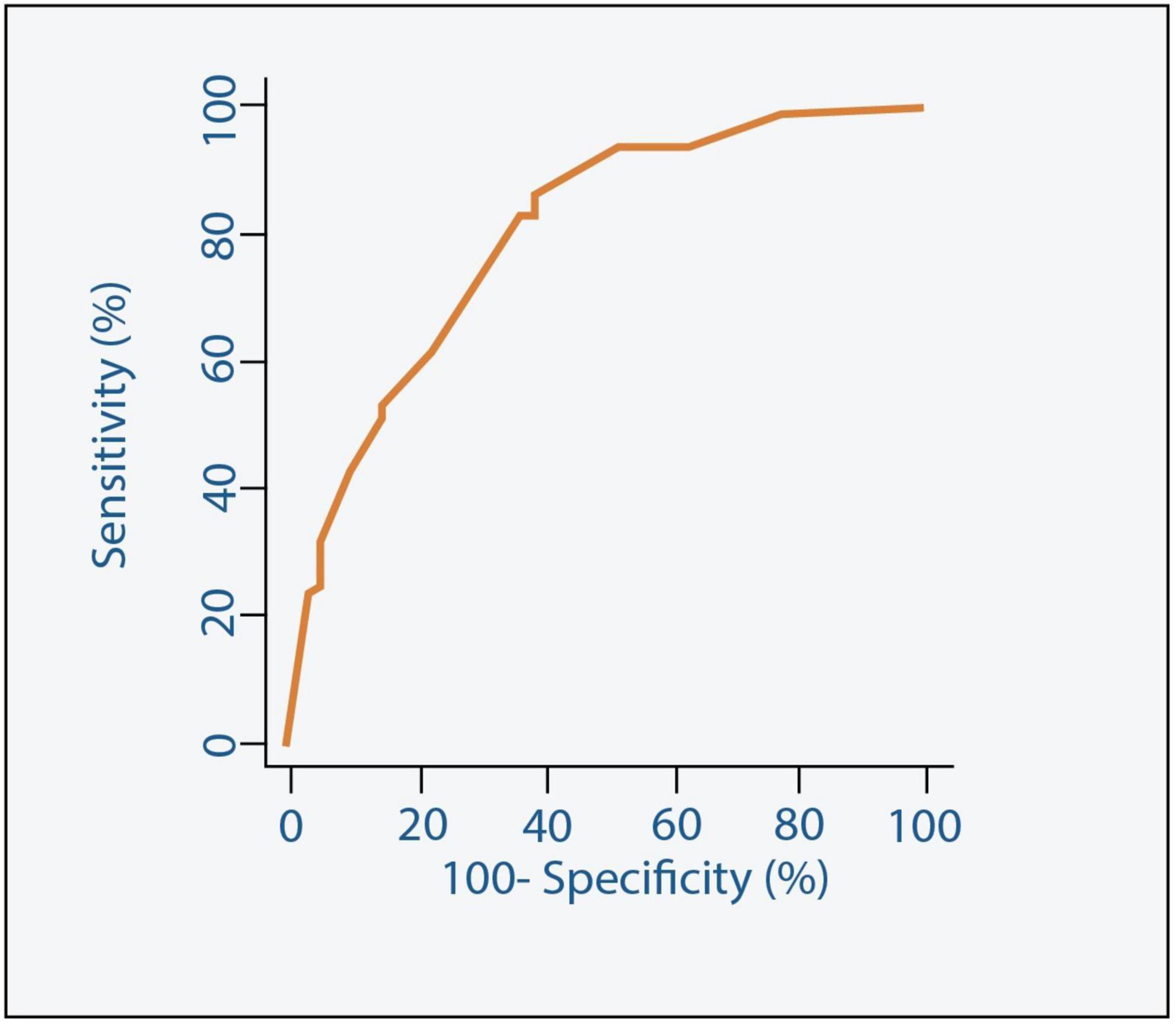
Figure 4. Receiver Operating Characteristic (ROC) curve along with the Area Under the ROC curve (AUC) for the model predicting 30-day outcome.
In a matched population, an STS score of ≥8, LVEF of <30%, and KCCQ of >50 remain statistically significant poor prognostic predictors for 30-day cumulative outcomes of adverse events and death regardless of the discharge time (OR 2.18, OR 8.80, OR 1.74, p = 0.012, p < 0.001, p = 0.02, respectively). While age was not correlated with 30-day outcomes, females showed a non-significant strong association (OR 1.15, 95% CI 0.69–1.92, P = 0.05) (Table 7).
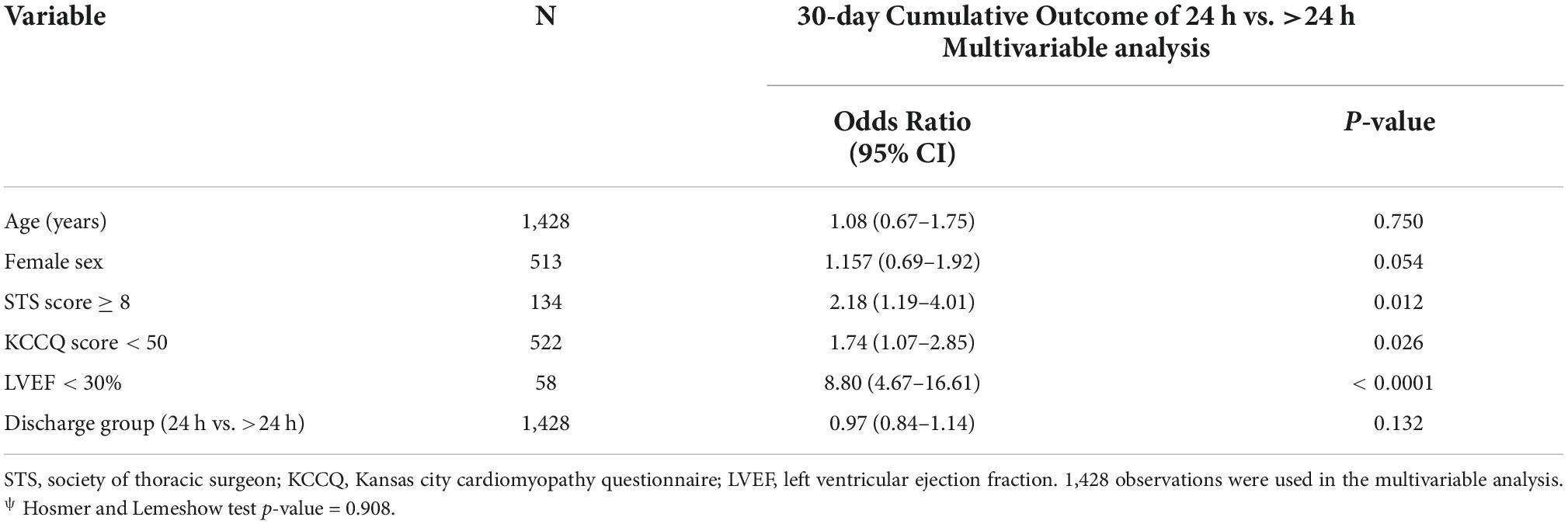
Table 7. Multivariable logistic regression of 30-day cumulative outcome after IPTW adjustment for patients discharge within 24 h and more than 24 h.
Discussion
Our study is the largest scale study known to address the safety and the feasibility of early discharge for TAVR patients under general anesthesia. We compared the outcomes of 837 patients discharged within 24 h of admission to 713 patients discharged within 24–48 h to 897 patients discharged after 48 h. The day of discharge is intuitive and involves numerous factors, both subjective, such as patient’s frailty and social support, and objective. Our study offers a validated tool for patient selection in borderline cases and illustrates that select patients can be discharged within 24 h of GA-TAVR without increasing the risk of adverse events.
The length of hospitalization following TAVR has dramatically evolved over the years (Figure 5) primarily due to increased operator ability as well as technological improvements of valves and delivery systems (7). Efficient hospital turnover is critical in today’s healthcare environment, especially in light of the healthcare burdens that have risen from the COVID-19 pandemic (24, 25).
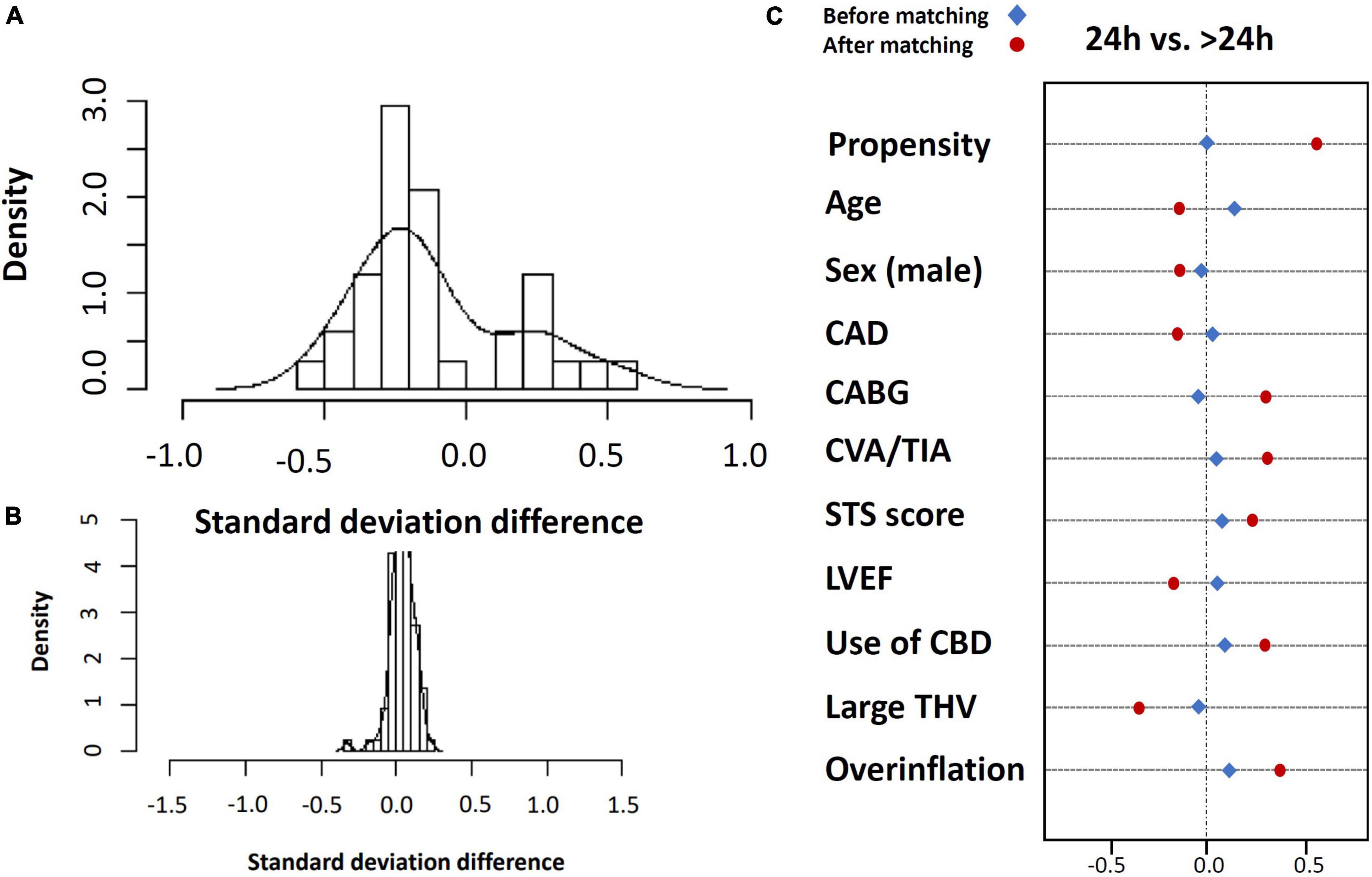
Figure 5. Standard deviation before (A) and after (B) inverse propensity treatment weighting (IPTW) using propensity matching and covariate balance for entire matched population (C).
General anesthesia vs. conscious sedation
The majority of TAVR procedures were initially performed using general anesthesia to aid in intraprocedural TEE for accurate assessment of annular dimensions, valve deployment, and paravalvular leak (PVL), minimizing patient’s movement and immediate response following life-threatening complications such as cardiac tamponade, annulus rupture, aortic dissection, and valve migrations (26–28). Recent data from TAVR registries indicate that CS shares a similar outcome of all-cause mortality, stroke, myocardial infarction, infection, and acute kidney injury at 30 days with an advantage of shorter procedure time, shorter hospital stay, and lower costs (29, 30). Despite the ongoing trend, the use of GA is still performed in 33–63% of all TAVRs, with a wide variation across North America and Europe (31). In a French registry study from 2011, GA was used in 41% of patients, and in a German registry, the use of CS was reported to vary from 26 to 68% of patients, depending on the center’s experience (32–34).
Our medical center uses general anesthesia and TEE as routine practice for all TAVR patients. TAVR is primarily performed in a hybrid operating room with a dedicated team of anesthesiologists and echocardiologists. The patients are intubated close to the time of the procedure and sedated using short-acting medication. After an uncomplicated TAVR, the patients are transferred directly to the post-anesthesia cardiac unit (PACU) for early extubating and 4–6 h of complete bed rest followed by mobilization 2–4 h later. Fully recovered and functioning patients with non-major complicated TAVR and no postprocedural adverse events such as atrioventricular block, atrial arrhythmias, hemodynamic instability, or chest pain, stay for overnight observation and are discharged early the following day after they undergo routine post-operative testing which includes transthoracic echocardiography study, resting ECG, renal function tests and complete blood count. The patients are monitored during their entire stay in the hospital.
Thirty-day outcome predictors and general anesthesia
The use of general anesthesia may be associated with a higher rate of adverse events and mortality in patients with comorbidities such as sleep apnea, chronic lung disease, illicit drug abuse, the use of supplementary home oxygen, and low cardiac ejection fraction (35, 36), which is incorporated in the STS score. To overcome it, we analyzed each component separately and found no interaction with the use of general anesthesia. Our study’s incidence of procedural-related life-threatening complications was not higher than reported in conscious sedation cohorts. We believe that these complications will probably result in longer LOH regardless of general anesthesia (36).
Same-day discharge
There is growing evidence that selected low-risk TAVR patients can be safely discharged as early as the same day (37–41). In a recent publication by Krishnaswamy et al., regarding the feasibility and safety of same-day discharge, the time of initiating the TAVR had the highest odds ratio for the outcome (40). GA-TAVR patients require a careful pre-anesthesia evaluation and a relative prolonged postprocedural care than CS patients (41).
Study limitation
The study is retrospective and based on data collections of patients’ electronic records and the 30-day outcome measures. The decision on exact discharge time may be affected by subjective factors such as social support and the patients frailty, which could not be thoroughly assessed using our study methodology. However, this was addressed by accounting for objective variables such as baseline comorbidities, related procedural characteristics, lab results, and the KCCQ, which is a performance scale model that, which integrates subjective assessment and subjective Variables as the Katz index of independence in activities of daily living which was validated in TAVR patients (42, 43). Moreover, we used a large sample size, three different comparison groups, and a multivariable strong statistical analysis and matching methods to avoid major bias in patient selection.
TAVR has changed dramatically over the past decade. With multiple commercially available valves and improvement in the delivery systems profiles, which have reduced procedural length and complications rates, patient safety has been enhanced (44–48). Our study included patients who underwent TAVR with new generation valves and delivery systems to reflect current day practices. However, our medical center utilizes balloon-expandable valves at a higher rate than self-expanding devices, which may decrease the external validity of our study (44, 45).
Length of stay (LOH) was affected during the late 2019 COVID outbreak and therefore necessitated rapid turnover and early discharge. This may impact our results, as other studies have previously reported (24, 25). We addressed it by including data from patients who underwent TAVR before and after the pandemic, as well as by including, and an intermediate discharge group of 24–48 h. This help to avoiding overlapping results. Furthermore, we demonstrate that the trend of minimizing LOS was initiated much before the pandemic, as we demonstrate.
We used propensity-score matching to compare patient population discharged at time points< and >24 h. It should be noted, however, that there were differences, particularly with the cohort discharged >48 h, that may have affected of our data. This included the baseline characteristics and higher rate of peri-procedural complications during the index hospitalization of those discharged at later time points.
Nevertheless procedural complications was not included in the logistics regression analysis to address the possibility of selection bias for early discharge and to adhere as much as possible to a prospective methodology reflecting real-life.
Our medical records are exposed to patients hospitalized only at Cedar’s networks and may therefore underestimate the true rehospitalization rate. However, the vast majority of patients (98%) attended the 30-day clinical visit and were interviewed by dedicated physicians and nurses to minimize the risk of missing information.
Conclusion
Our study shows that independent poor predictors for a 30-day outcome with correlation to discharge time were high surgical risk, low KCCQ score, low LVEF, and life-threatening in-hospital complications.
Our formulated predictors were tested and validated using the entire study population and they demonstrated an ability to identify patients at risk of 30-day adverse events.
The trend of an optimized length of stay should cover all treatment protocols using general anesthesia and balance the need for a proper and safe post-procedural care such as a complete echocardiography study, a blood test for renal function, an ECG, and a clinical follow-up following re-mobilization.
Data availability statement
The raw data supporting the conclusions of this article will be made available by the authors, without undue reservation.
Author contributions
OK, VP, HJ, and RM conceptual the hypothesis, validated the results, and supervised all processes of the manuscript. EN, RN, TN, AS, SN, SK, ZA, AB, and AD assist in data collection, statistical analysis, and the final writing. MN and WC provided a detailed review and helped in the last draft. All authors contributed to the article and approved the submitted version.
Funding
This study was supported in part by the California Chapter of the American College of Cardiology through the Save a Heart Foundation.
Conflict of interest
RM received grant support from Edwards Lifesciences Corporation; consulted for Abbott Vascular, Cordis, and Medtronic; and holds equity in Entourage Medical.
The remaining authors declare that the research was conducted in the absence of any commercial or financial relationships that could be construed as a potential conflict of interest.
Publisher’s note
All claims expressed in this article are solely those of the authors and do not necessarily represent those of their affiliated organizations, or those of the publisher, the editors and the reviewers. Any product that may be evaluated in this article, or claim that may be made by its manufacturer, is not guaranteed or endorsed by the publisher.
Abbreviations
AS, Aortic Stenosis; CS, conscious sedation; GA, general anesthesia; LVEF, Left ventricle ejection fraction; KCCQ, Kansas city cardiomyopathy questionnaire; LOS, Length of stay; PVL, paravalvular leakage; STS, Society of thoracic surgeon; TAVR, Transcatheter aortic valve replacement; TEE, Transesophageal echocardiography; THV, Transcatheter aortic valve.
References
1. Smith CR, Leon MB, Mack MJ, Miller DC, Moses JW, Svensson LG, et al. PARTNER Trial Investigators. Transcatheter versus surgical aortic-valve replacement in high-risk patients. N Engl J Med. (2011) 364:2187–98.
2. Leon MB, Smith CR, Mack MJ, Makkar RR, Svensson LG, Kodali SK, et al. PARTNER 2 investigators. transcatheter or surgical aortic-valve replacement in intermediate-risk patients. N Engl J Med. (2016) 374: 1609–20.
3. Mack MJ, Leon MB, Thourani VH, Makkar R, Kodali SK, Russo M, et al. PARTNER 3 investigators. transcatheter aortic-valve replacement with a balloon-expandable valve in low-risk patients. N Engl J Med. (2019) 380:1695–705. doi: 10.1056/NEJMoa1814052
4. Holmes DR Jr, Brennan JM, Rumsfeld JS, Dai D, O’Brien SM, Vemulapalli S, et al. STS/ACC TVT Registry. Clinical outcomes at 1 year following transcatheter aortic valve replacement. JAMA. (2015) 313:1019–28. doi: 10.1001/jama.2015.1474
5. Makkar RR, Thourani VH, Mack MJ, Kodali SK, Kapadia S, Webb JG, et al. PARTNER 2 investigators. five-year outcomes of transcatheter or surgical aortic-valve replacement. N Engl J Med. (2020) 382:799–809. doi: 10.1056/NEJMoa1910555
6. Arora S, Strassle PD, Kolte D, Ramm CJ, Falk K, Jack G, et al. Length of stay and discharge disposition after transcatheter versus surgical aortic valve replacement in the United States. Circ Cardiovasc Interv. (2018) 11:e006929. doi: 10.1161/CIRCINTERVENTIONS.118.006929
7. Wayangankar SA, Elgendy IY, Xiang Q, Jneid H, Vemulapalli S, Khachatryan T, et al. Length of stay after transfemoral transcatheter aortic valve replacement: an analysis of the society of thoracic surgeons/American college of cardiology transcatheter valve therapy registry. JACC Cardiovasc Interv. (2019) 12:422–30. doi: 10.1016/j.jcin.2018.11.015
8. Kolte D, Kennedy K, Wasfy JH, Jena AB, Elmariah S. Hospital variation in 30-day readmissions following transcatheter aortic valve replacement. J Am Heart Assoc. (2021) 10:e021350. doi: 10.1161/JAHA.120.021350
9. Kolte D, Khera S, Sardar MR, Gheewala N, Gupta T, Chatterjee S, et al. Thirty-day readmissions after transcatheter aortic valve replacement in the united states: insights from the nationwide readmissions database. Circ Cardiovasc Interv. (2017) 10:e004472. doi: 10.1161/CIRCINTERVENTIONS.116.004472
10. Goldsweig A, Aronow H. Identifying patients likely to be readmitted after transcatheter aortic valve replacement. Heart. (2019) 106:256–60. doi: 10.1136/heartjnl-2019-315381
11. Szerlip M, Tabachnick D, Hamandi M, Caras L, Lanfear AT, Squiers JJ, et al. Safe implementation of enhanced recovery after surgery protocol in transfemoral transcatheter aortic valve replacement. Proceedings. (2020) 34:5–10. doi: 10.1080/08998280.2020.1810198
12. Lauck SB, Wood DA, Baumbusch J, Kwon JY, Stub D, Achtem L, et al. Vancouver transcatheter aortic valve replacement clinical pathway: minimalist approach, standardized care, and discharge criteria to reduce length of stay. Circ Cardiovasc Qual Outcomes. (2016) 9:312–21. doi: 10.1161/CIRCOUTCOMES.115.002541
13. Costa G, Barbanti M, Picci A, Todaro D, La Spina K, Di Simone E, et al. Predictors and safety of next-day discharge in patients undergoing transfemoral transcatheter aortic valve implantation. EuroIntervention. (2020) 16:e494–501. doi: 10.4244/EIJ-D-19-01080
14. VARC-3 WRITING COMMITTEE, Généreux P, Piazza N, Alu MC, Nazif T, Hahn RT, et al. Valve Academic Research Consortium 3: updated endpoint definitions for aortic valve clinical research. Eur Heart J. (2021) 42:1825–57. doi: 10.1093/eurheartj/ehaa799
15. Yamashita T, Yamashita K, Kamimura R. A Stepwise AIC method for variable selection in linear regression. Commun Stat Theory Methods. (2007) 36:2395–403. doi: 10.1080/03610920701215639
16. Steyerberg E, Vickers A, Cook N, Gerds T, Gonen M, Obuchowski N, et al. Assessing the performance of prediction models: a framework for some traditional and novel measures. Epidemiology. (2010) 21:128–38. doi: 10.1097/EDE.0b013e3181c30fb2
17. Cox DR. Two further applications of a model for binary regression. Biometrika. (1958) 45:562–5. doi: 10.1093/biomet/45.3-4.562
18. Harrell F. Regression Modeling Strategies: With Applications To Linear Models, Logistic Regression, And Survival Analysis. New York, NY: Springer (2001). doi: 10.1007/978-1-4757-3462-1
19. Harrell FE Jr, Lee KL, Mark DB. Multivariable prognostic models: issues in developing models, evaluating assumptions and adequacy, and measuring and reducing errors. Stat Med. (1996) 15:361–87. doi: 10.1002/(SICI)1097-0258(19960229)15:4<361::AID-SIM168>3.0.CO;2-4
20. Steyerberg EW, Harrell FE Jr, Borsboom GJ, Eijkemans MJ, Vergouwe Y, Habbema JD. Internal validation of predictive models: efficiency of some procedures for logistic regression analysis. J Clin Epidemiol. (2001) 54:774–81. doi: 10.1016/S0895-4356(01)00341-9
21. Bergstralh EJ, Konsanke JL. Computerized Matching of Cases to Controls. Technical Report Series, No. 56. Rochester, MN: Mayo Clinic Department of Health Sciences Research (1995).
22. Blackstone EH. Comparing apples and oranges. J Thorac Cardiovasc Surg. (2002) 123:8–15. doi: 10.1067/mtc.2002.120329
23. R Core Team. R: A Language And Environment For Statistical Computing. Vienna: R Foundation for Statistical Computing (2020).
24. Barker M, Sathananthan J, Perdoncin E, Devireddy C, Keegan P, Grubb K, et al. Same-Day discharge post-transcatheter aortic valve replacement during the COVID-19 pandemic: the multicenter PROTECT TAVR study. JACC Cardiovasc Interv. (2022) 15:590–8.
25. Perdoncin E, Greenbaum AB, Grubb KJ, Babaliaros VC, Keegan P, Ceretto-Clark B, et al. Safety of same-day discharge after uncomplicated, minimalist transcatheter aortic valve replacement in the COVID-19 era. Catheter Cardiovasc Interv. (2021) 97:940–7. doi: 10.1002/ccd.29453
26. Thiele H, Kurz T, Feistritzer HJ, Stachel G, Hartung P, Lurz P, et al. SOLVE-TAVI investigators. general versus local anesthesia with conscious sedation in transcatheter aortic valve implantation: the randomized SOLVE-TAVI Trial. Circulation. (2020) 142:1437–47. doi: 10.1161/CIRCULATIONAHA.120.046451
27. Sato K, Jones PM. Sedation versus general anesthesia for transcatheter aortic valve replacement. J Thorac Dis. (2018):10:S3588–94. doi: 10.21037/jtd.2018.08.89
28. Schoechlin S, Brennemann T, Allali A, Ruile P, Jander N, Allgeier M, et al. Hemodynamic classification of paravalvular leakage after transcatheter aortic valve implantation compared with angiographic or echocardiographic classification for prediction of 1-year mortality. Catheter Cardiovasc Interv. (2018) 91:E56–63. doi: 10.1002/ccd.27384
29. Villablanca PA, Mohananey D, Nikolic K, Bangalore S, Slovut DP, Mathew V, et al. Comparison of local versus general anesthesia in patients undergoing transcatheter aortic valve replacement: a meta-analysis. Catheter Cardiovasc Interv. (2018) 91:330–42. doi: 10.1002/ccd.27207
30. Hyman MC, Vemulapalli S, Szeto WY, Stebbins A, Patel PA, Matsouaka RA, et al. Conscious sedation versus general anesthesia for transcatheter aortic valve replacement: insights from the national cardiovascular data registry society of thoracic surgeons/american college of cardiology transcatheter valve therapy registry. Circulation. (2017) 136:2132–40. doi: 10.1161/CIRCULATIONAHA.116.026656
31. Butala NM, Chung M, Secemsky EA, Manandhar P, Marquis-Gravel G, Kosinski AS, et al. Conscious sedation versus general anesthesia for transcatheter aortic valve replacement: variation in practice and outcomes. JACC Cardiovasc Interv. (2020) 13:1277–87. doi: 10.1016/j.jcin.2020.03.008
32. Oguri A, Yamamoto M, Mouillet G, Gilard M, Laskar M, Eltchaninoff H, et al. FRANCE 2 registry investigators. clinical outcomes and safety of transfemoral aortic valve implantation under general versus local anesthesia: subanalysis of the french aortic national corevalve and edwards 2 registry. Circ Cardiovasc Interv. (2014) 7:602–10. doi: 10.1161/CIRCINTERVENTIONS.113.000403
33. Husser O, Fujita B, Hengstenberg C, Frerker C, Beckmann A, Möllmann H, et al. GARY executive board. conscious sedation versus general anesthesia in transcatheter aortic valve replacement: the german aortic valve registry. JACC Cardiovasc Interv. (2018) 11:567–78. doi: 10.1016/j.jcin.2017.12.019
34. Tan C, Dean LS. Conscious sedation for TAVR: a wave of the future? Catheter Cardiovasc Interv. (2018) 91:343–4. doi: 10.1002/ccd.27501
35. Edrich T, Sadovnikoff N. Anesthesia for patients with severe chronic obstructive pulmonary disease. Curr Opin Anaesthesiol. (2010) 23:18–24. doi: 10.1097/ACO.0b013e328331ea5b
36. Rezq A, Basavarajaiah S, Latib A, Takagi K, Hasegawa T, Figini F, et al. Incidence, management, and outcomes of cardiac tamponade during transcatheter aortic valve implantation: a single-center study. JACC Cardiovasc Interv. (2012) 5:1264–72. doi: 10.1016/j.jcin.2012.08.012
37. Barbanti M, Baan J, Spence MS, Iacovelli F, Martinelli GL, Saia F, et al. Feasibility and safety of early discharge after transfemoral transcatheter aortic valve implantation - rationale and design of the FAST-TAVI registry. BMC Cardiovasc Disord. (2017) 17:259. doi: 10.1186/s12872-017-0693-0
38. Barbanti M. Early discharge after transcatheter aortic valve replacement: mature times. JACC Cardiovasc Interv. (2019) 12:431–2. doi: 10.1016/j.jcin.2018.12.017
39. Inayat A, Abbas S, Salman F. Predictors of mortality in patients with transcatheter aortic valve implantation: a national inpatient sample database analysis. Cureus. (2021) 13:e14344. doi: 10.7759/cureus.14344
40. Krishnaswamy A, Isogai T, Agrawal A, Shekhar S, Puri R, Reed GW, et al. Feasibility and safety of same-day discharge following transfemoral transcatheter aortic valve replacement. JACC Cardiovasc Interv. (2022) 15:575–89. doi: 10.1016/j.jcin.2022.01.013
41. Wenaweser P, Stortecky S. Early discharge after transcatheter aortic valve replacement: is early too soon? JACC Cardiovasc Interv. (2019) 12:470–2. doi: 10.1016/j.jcin.2019.01.225
42. Shelkey M, Wallace M. Katz index of independence in activities of daily living. J Gerontol Nurs. (1999) 25:8–9. doi: 10.3928/0098-9134-19990301-05
43. Puls M, Sobisiak B, Bleckmann A, Jacobshagen C, Danner BC, Hünlich M, et al. Impact of frailty on short- and long-term morbidity and mortality after transcatheter aortic valve implantation: risk assessment by Katz Index of activities of daily living. EuroIntervention. (2014) 10:609–19. doi: 10.4244/EIJY14M08_03
44. Seeger J, Gonska B, Rottbauer W, Wöhrle J. New generation devices for transfemoral transcatheter aortic valve replacement are superior compared with last generation devices with respect to VARC-2 outcome. Cardiovasc Interv Ther. (2018) 33:247–55. doi: 10.1007/s12928-017-0477-6
45. Tchetche D, Van Mieghem NM. New-generation TAVI devices: description and specifications. EuroIntervention. (2014) 10:U90–100. doi: 10.4244/EIJV10SUA13
46. Santangelo G, Ielasi A, Pellicano M, Latib A, Tespili M, Donatelli F. An update on new generation transcatheter aortic valves and delivery systems. J Clin Med. (2022) 11:499. doi: 10.3390/jcm11030499
47. Pilgrim T, Lee JKT, O’Sullivan CJ, Stortecky S, Ariotti S, Franzone A, et al. Early versus newer generation devices for transcatheter aortic valve implantation in routine clinical practice: a propensity score matched analysis. Open Heart. (2018) 5:e000695. doi: 10.1136/openhrt-2017-000695
Keywords: transcatheter aortic valve replacement, general anesthesia, conscious sedation, length of stay, early discharge, safety discharge, mortality, predictors
Citation: Koren O, Patel V, Kohan S, Naami R, Naami E, Allison Z, Natanzon SS, Shechter A, Nagasaka T, Al Badri A, Devanabanda AR, Nakamura M, Cheng W, Jilaihawi H and Makkar RR (2022) The safety of early discharge following transfemoral transcatheter aortic valve replacement under general anesthesia. Front. Cardiovasc. Med. 9:1022018. doi: 10.3389/fcvm.2022.1022018
Received: 18 August 2022; Accepted: 04 October 2022;
Published: 21 October 2022.
Edited by:
Hendrik Ruge, Technical University Munich, GermanyReviewed by:
Alberto Alperi, Central University Hospital of Asturias, SpainXiangbin Pan, Chinese Academy of Medical Sciences and Peking Union Medical College, China
Bo Mei, Affiliated Hospital of North Sichuan Medical College, China
Copyright © 2022 Koren, Patel, Kohan, Naami, Naami, Allison, Natanzon, Shechter, Nagasaka, Al Badri, Devanabanda, Nakamura, Cheng, Jilaihawi and Makkar. This is an open-access article distributed under the terms of the Creative Commons Attribution License (CC BY). The use, distribution or reproduction in other forums is permitted, provided the original author(s) and the copyright owner(s) are credited and that the original publication in this journal is cited, in accordance with accepted academic practice. No use, distribution or reproduction is permitted which does not comply with these terms.
*Correspondence: Raj R. Makkar, Raj.Makkar@cshs.org
†These authors have contributed equally to this work