- 1Bristol Heart Institute, University of Bristol, Bristol, United Kingdom
- 2William Harvey Research Institute, Queen Mary University of London, London, United Kingdom
- 3Bristol Trials Centre, Bristol Medical School, University of Bristol, Bristol, United Kingdom
- 4Royal Brompton and Harefield Hospitals, Guys’ and St.Thomas NHS Hospitals and School of Biomedical Engineering & Imaging Sciences, King’s College London, London, United Kingdom
Background: Myocardial infarction induces elevation of progenitor cells in the circulation, a reparative response inhibited by type-2 diabetes.
Objectives: Determine if myocardial infarct severity and diabetes interactively influence the migratory activity of CD34+/CXCR4+ progenitor cells and if the migratory test predicts cardiac outcomes.
Materials and methods: A longitudinal study was conducted on patients with or without diabetes with a STEMI or NSTEMI. CD34+/CXCR4+ cells were measured in the peripheral blood using flow cytometry, and migratory activity was tested in vitro on cells isolated from samples collected on days 0 and 4 post-infarct. Cardiac function was assessed at three months using cardiac MRI.
Results: Of 1,149 patients screened, 71 (6.3%) were eligible and consented. Fifty had STEMI (16 with diabetes) and 21 NSTEMI (8 with diabetes). The proportion of CD34+/CXCR4+ cells within blood mononuclear cells was 1.96 times higher after STEMI compared with NSTEMI (GMR = 1.96, 95% CI 0.87, 4.37) and 1.55 times higher in patients with diabetes compared to patients without diabetes (GMR = 1.55, 95% CI 0.77, 3.13). In the latter, STEMI was associated with a 2.42-times higher proportion of migrated CD34 + /CXCR4 + cells compared with NSTEMI (GMR = 2.42, 95% CI 0.66, 8.81). In patients with diabetes, the association was the opposite, with a 55% reduction in the proportion of migrated CD34+/CXCR4+ cells. No statistically significant associations were observed between the frequency in peripheral blood or in vitro migration capacity of CD34+/CXCR4+ cells and MRI outcomes.
Conclusion: We document the interaction between infarct and diabetes on the migratory activity of CD34+/CXCR4+ cells. The test did not predict functional outcomes in the studied cohort.
Background
Tissue repair after myocardial infarction (MI) comprises a phase dominated by the recruitment of neutrophils and proinflammatory macrophages and a subsequent resolution stage orchestrated by reparative macrophages (1–3). Progenitor cells (PCs) are also released from the bone marrow (BM) into the circulation and recruited to the injured myocardium through chemokine-orchestrated homing. Flow cytometry and in vitro migratory assays make it possible to define specific sub-populations of circulating PCs (CPCs) in relation to their functional properties (4).
The proportion of CPCs increases during the first week after the onset of acute MI (5–7), with an intensity not necessarily proportional to the increase in leukocyte counts (5). This finding suggests that the liberation of CPCs is a critical, autonomous determinant of post-MI healing. We and others have shown that type-2 diabetes mellitus (DM) and cardiovascular risk factors can impair the migratory and functional capacities of CPCs by negatively influencing the BM microenvironment (8–15), and the molecular crosstalk between mobilized cells and peripheral vasculature (16–18). CPC deficits have been claimed to represent excellent long-term predictors of cardiovascular outcomes in patients with type 2 DM (19–21). Moreover, new anti-diabetic drugs, such as glucagon-like peptide-1 receptor agonists and DPP-4 inhibitors, which reduce the risk of cardiovascular death in patients with DM (22, 23), increase the number and function of CPCs in peripheral blood (24–27). Therefore, assessing the state of CPCs could have prognostic and therapeutic implications.
It remains, however, unknown whether the negative effect of DM on CPC mobilization is influenced by the amount of damage to the heart after an acute MI and whether this interaction affects the post-MI outcome. To explore these questions, we have conducted a longitudinal study on patients with or without DM who have had an ST-segment Elevation MI (STEMI) or Non-ST-segment Elevation MI (NSTEMI). The first objective was to determine the interactive influence between DM and type of MI on (i) the relative abundance of CD34+/CXCR4+CPCs in peripheral blood (PB) and (ii) the in vitro migratory response of CPCs. The second objective was to investigate whether CPC abundance and migratory activity predicted cardiac magnetic resonance imaging (MRI) outcomes three months post-MI.
Materials and methods
Study design
ProMIS was a single center longitudinal, observational study conducted in Bristol, comprising four cohorts: patients with and without DM who have had a STEMI or a NSTEMI, defined according to international guidelines (28, 29). The study schema is illustrated in Supplementary Figure 1 and the study protocol in Supplementary Appendix 1. The study protocol and subsequent amendments received a favorable opinion from the Wiltshire Research Ethics Committee (ref 09/H0104/58) and were performed according to the declaration of Helsinki. The University Hospitals Bristol NHS Foundation Trust (UHBristol) was the sponsor. This study was overseen by the Cardiovascular Research Board of UHBristol and the University of Bristol.
Objectives
The specific study objectives were to determine whether: (1a) the number of CPCs differs after STEMI compared to NSTEMI; (1b) the migratory capacity of CPCs varies in patients with or without DM; (1c) the number and migratory ability of CPCs are associated with covariables characterizing the severity of the initial STEMI or NSTEMI (e.g., troponin I, high sensitivity C-reactive protein (hs-CRP) or the quality of glucose control (HbA1c); and (2) the number and migratory capacity of CPCs after a STEMI or NSTEMI influence the size of the myocardial scar and myocardial contractility three months after the initial cardiac event.
Participants
Patients aged 40–75 at admission with STEMI or NSTEMI presenting to a Bristol Heart Institute cardiologist within 24 h after the onset of symptoms and willing to participate for the duration of the study were eligible for inclusion. Patients with anemia (i.e., hemoglobin < 10 mg/dl), cardiogenic shock on presentation, renal impairment (eGFR < 50 ml), haemodynamic instability, contraindications to having the MRI scan (e.g., metallic implant, pacemakers, screws, claustrophobia, etc.), a previous coronary event within the last 12 weeks, participation in another clinical study, unable or unwilling to return for follow-up by the study schedule after three months or heightened anxiety during recruitment were excluded.
Study procedures and follow-up
Patients with STEMI underwent immediate emergency intervention in the catheterization laboratory. Patients with NSTEMI with no ongoing symptoms or signs of ischemia underwent diagnostic angiography the following working day.
Patients were approached for enrollment into the study on day 0, at the time of PCI or diagnostic angiography. Blood samples were obtained within 12 h of presentation. A research MRI scan was scheduled before discharge, usually between days 2 and 4. A second MRI scan was scheduled at three months. A summary of time-points of data collection is provided in Supplementary Table 1.
Laboratory measurements
The standardized protocol for counting CPCs and assessing their migratory activity has been described by us earlier (4).
Cardiac magnetic resonance imaging scans
All MRI scans were performed on a 1.5T scanner (Magnetom Avanto; Siemens; Elangen, Germany), with a surface phased array received coil and retrospective electrographic triggering. Myocardial perfusion and previous infarction were assessed using adenosine and gadolinium-DTPA first-pass data acquisition and late-enhancement imaging.
Regional LV thickening of the “affected” segments was determined from end-systolic and end-diastolic images. Secondary measures of wall thickening included standard wall score index (WMSI); wall motion for each segment was rated from cine MRI images on a 5-point scale (0, normal; 1, mild hypokinesia; 2, severe hypokinesia; 3, akinesia; 4, dyskinesia).
LV regional myocardial viability was measured using gadolinium contrast images. Infarct size was measured as a planimeter area (and volume derived by multiplying the area by slice thickness) and volume fraction of enhanced myocardium. For myocardial viability analysis, delayed-enhancement images for each segment were scored for function analysis, and quantified by computer-assisted planimetry on short-axis images. Segments were graded in transmural extent on a 5-point scale (0, no HE; 1, hyper-enhancement extending from 1 to 25%; 2, 26 to 50%; 3, 51 to 75%; 4, hyperenhancement >75% of LV wall thickness for that segment).
End systolic volume, stroke volume and ejection fraction were measured according to the validated MRI laboratory standards, using contiguous short axis slices obtained by cine MRI with correction for long axis motion (Argus 4D Software or Brompton). End-diastolic and end-systolic endocardial traces were used to determine end-diastolic and end-systolic LV volumes and total ejection fraction.
Statistical analysis
Baseline characteristics are summarized by exposure (STEMI and NSTEMI, without DM/DM) for all participants in the analysis population. Continuous variables are summarized using the mean and standard deviation (SD) or median and inter-quartile range (IQR) if distributions are skewed, and categorical data are summarized as a number and percentage.
The effect of the STEMI/NSTEMI and DM on CPC migration was estimated using a continuous longitudinal mixed effects methodology. All models incorporated random intercept and slope components where appropriate and used restricted maximum likelihood (REML) estimation and Kenward-Roger estimators to adjust the bias of estimating standard errors in small samples. Model fit was assessed using standard methods, such as residual plots and normality tests. If the assumptions were not met, outcomes were analyzed on a logarithmic scale with results presented as geometric mean ratios.
Two exposures of interest were considered for assessing the predictive value of CPCs: (1) the proportion of CD34+/CXCR4+ measured in the PB (within-person mean of replicates on day 0 and day 4) and (2) the ratio of migrated CD34+/CXCR4+ measured after STEMI or NSTEMI. Continuous outcomes were compared using linear regression and binary outcomes using logistic regression, adjusted for age, sex, time to MRI and baseline value of outcomes being modeled. For hypothesis tests, a two-tailed significance level of 5% was used, except for interactions. A 10% significance level was used to test the interactions between STEMI and DM, STEMI and timepoint, DM and timepoint, STEMI and chemo-attractant and DM and chemo-attractant. All analyses were performed in Stata version 17⋅0 (StataCorp, LP, College Station, TX, USA).
Results
Recruitment
Between February 2010 and September 2013, 1,149 patients were screened for inclusion. Of these, 807 (70.2%) were ineligible, 230 (20.0%) were eligible but not approached and 111 (9.7%) were eligible and approached for consent. Of the 111 patients approached for consent, 72 consented (6.3% of screened patients), of which one was identified as ineligible post-consent. Of the 71 consented participants, 50 were STEMI (16 with DM, 34 without DM) and 21 were NSTEMI (8 with DM, 13 without DM). The CONSORT diagram is presented in full in Figure 1.
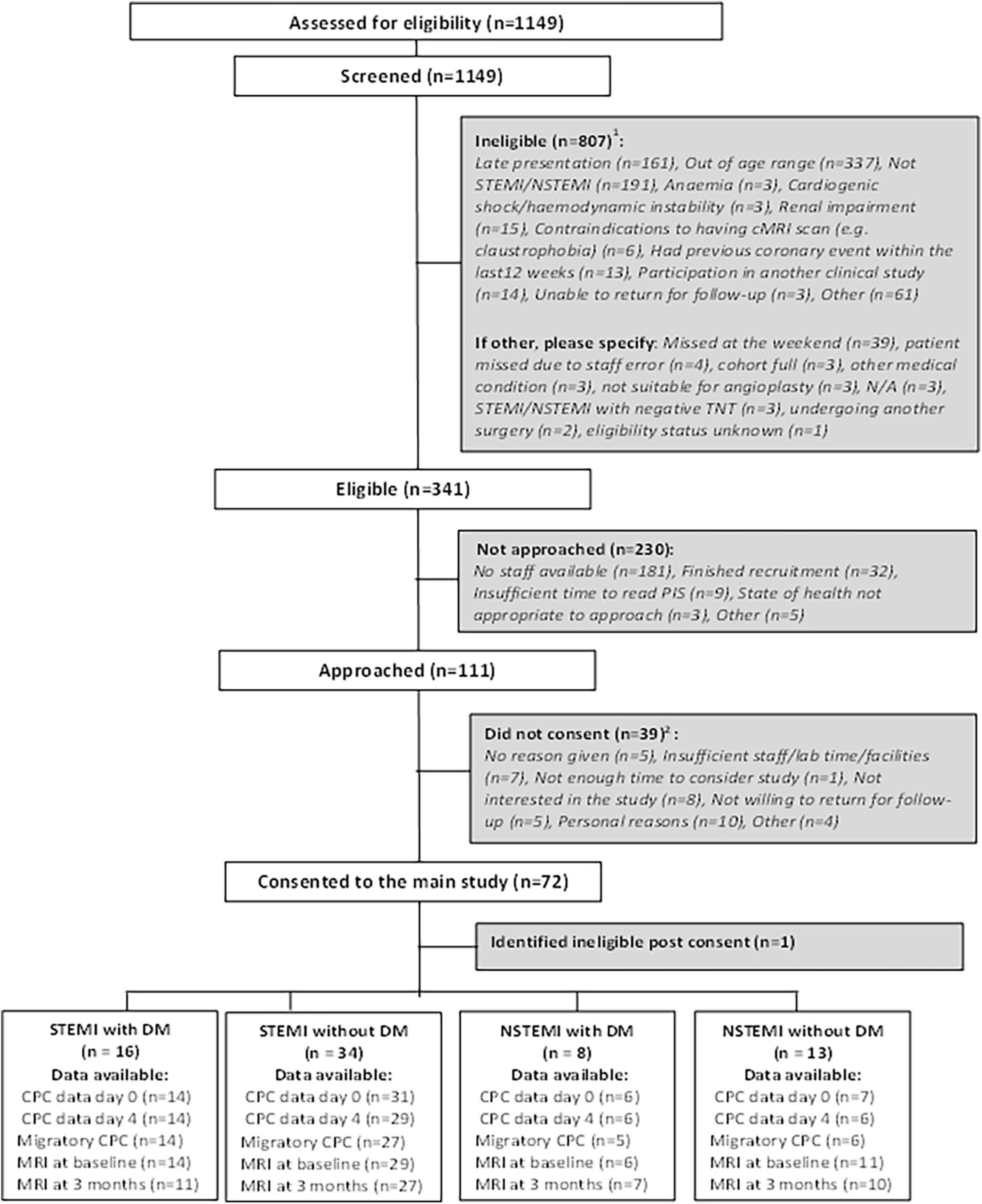
Figure 1. CONSORT diagram showing the flow of participants. Some patients may be ineligible for more than one reason. Some patients may have not consented for more than one reason.
Patient withdrawals
Two participants withdrew from the study (1 STEMI without DM, 1 NSTEMI without DM). Details of the withdrawals are given in Supplementary Table 2.
Protocol deviations
There were 32 protocol deviations (6 STEMI with DM, 9 STEMI without DM, 6 NSTEMI with DM, and 11 STEMI without DM). A summary of the protocol deviations is presented in Supplementary Table 3.
Baseline characteristics
All 71 consented participants had baseline data available and had a preoperative angiographic assessment. Patient characteristics are presented in Table 1. The median age of participants was 61.0 years (IQR 52.7, 66.6). The median age was highest in the group of participants with NSTEMI and DM (68.0 years, IQR 57.9, 72.1) and similar across the remaining groups.
Operative details and perioperative laboratory measurements
As shown in Table 2, PCI was performed in 64/71 (90.1%) participants. The average procedure time was 45 min (IQR 38 to 65.5 min). Aspirin was given pre-procedure in all participants and clopidogrel was given in under 50% of participants. Approximately 50% of participants had bystander disease in one or more vessels. Perioperative laboratory measurements on day 0 are given in Supplementary Table 4.
Medications
Medications given at hospital discharge are reported in Supplementary Table 5. All patients were prescribed aspirin and a lipid-lowering agent at discharge.
Cardiac magnetic resonance imaging assessments and circulating progenitor cell measurements
Sixty of the 71 participants had a cardiac MRI at baseline (14 STEMI with DM, 29 STEMI without DM, 6 NSTEMI with DM, 11 NSTEMI without DM), and 55 had a cardiac MRI at three months (11 STEMI with DM, 27 STEMI without DM, 7 NSTEMI with DM, 10 NSTEMI without DM). Of the latter 55, 52 had both baseline and 3-month MRI (11 STEMI with DM, 26 STEMI without DM, 5 NSTEMI with DM, 10 NSTEMI without DM). The number of patients with CPC laboratory and MRI data available is given in Supplementary Table 6.
Objective 1: Proportion and migratory capacity of circulating progenitor cells
Data on the abundance of CPCs in PB at day 0 and day 4 days post-MI is shown in Supplementary Table 7. The associations between STEMI and DM and the percentage of CPCs for the various cell markers are given in Figure 2. In summary, there were no statistically significant differences in the rate of CPCs between patients with/without STEMI and with/without DM for any of the cell markers. For the primary outcome, the proportion of CD34+/CXCR4+ cells was on average 1.96 times higher in STEMI than NSTEMI (GMR = 1.96, 95% CI 0.87–4.37). This difference was not statistically significant. Likewise, the proportion of CD34+/CXCR4+ cells tended to be higher in patients with DM compared to patients without DM (GMR = 1.55, 95% CI 0.77, 3.13). There was no evidence of an interaction between STEMI ± and DM (p = 0.30).
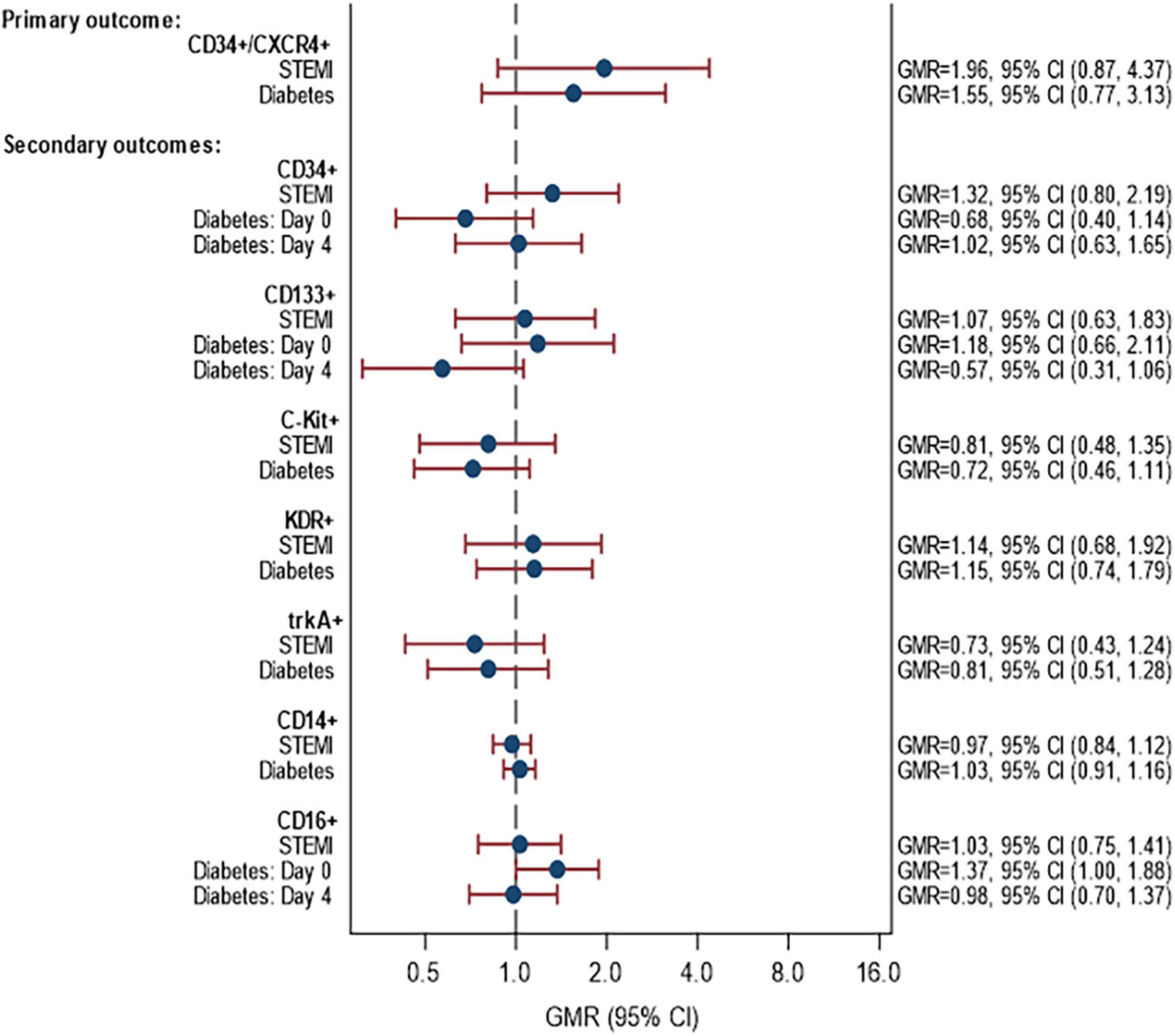
Figure 2. Associations between STEMI and DM with proportion of CPCs. Models fitted to participants with lab data available on day 0 and/or day 4: 58 participants (14 STEMI with DM, 31 STEMI without DM, 6 NSTEMI with DM, 7 NSTEMI without DM). Estimates are adjusted for age, sex and time since onset of symptoms. p-Values for interaction STEMI × diabetes: CD34+/CXCR4+ (p = 0.2967), CD34+ (p = 0.3257), CD133+ (p = 0.1023), C-Kit (p = 0.8526), KDR+ (p = 0.6713), trkA+ (p = 0.1884), CD14+ (p = 0.9978), CD16+ (p = 0.7661). p-Values for interaction STEMI × time: CD34+/CXCR4+ (p = 0.7517), CD34+ (p = 0.1756), CD133+ (p = 0.9222), C-Kit (p = 0.5820), KDR+ (p = 0.1839), trkA+ (p = 0.7548), CD14+ (p = 0.4178), CD16+ (p = 0.1561). p-Values for interaction diabetes × time: CD34+/CXCR4+ (p = 0.8349), CD34+ (p = 0.095), CD133+ (p = 0.0649), C-Kit (p = 0.660), KDR+ (p = 0.4274), trkA+ (p = 0.8302), CD14+ (p = 0.5026), CD16+ (p = 0.0551) STEMI, ST-elevation myocardial infarction; NSTEMI, non-ST-elevation myocardial infarction.
When looking at the change in the percentage of CD34+/CXCR4+ cells from day 0 to day 4, the results show no association between STEMI or DM and change in CD34/CXCR4 cells (Supplementary Figure 2).
A summary of CPC migration data is shown in Supplementary Table 8. The associations between STEMI and DM and the percentage of migrated cell markers on day 4 are given in Figure 3. There were no statistically significant differences in the proportion of migrated CPCs between patients with STEMI vs NSTEMI and DM vs non-DM for any of the cell markers. The relationship between STEMI/NSTEMI and CD34+/CXCR4+ cells was different in patients with DM than in those without DM. In patients without DM, STEMI was associated with over double the proportion of migrated CD34+/CXCR4+ cells compared to NSTEMI (GMR 2.42, 95% CI 0.66, 8.81). In patients with DM, the association was in the opposite direction, with a 55% reduction in migrated CD34+/CXCR4+ cells (GMR = 0.45, 95% CI 0.10, 2.07). However, it is essential to note that there are small numbers of patients within the subgroups (see footnote to Figure 3 for numbers) and, although the interaction was included (p = 0.098), the association of STEMI/NSTEMI with migrated CD34+/CXCR4+ cells was not statistically significant in either group.
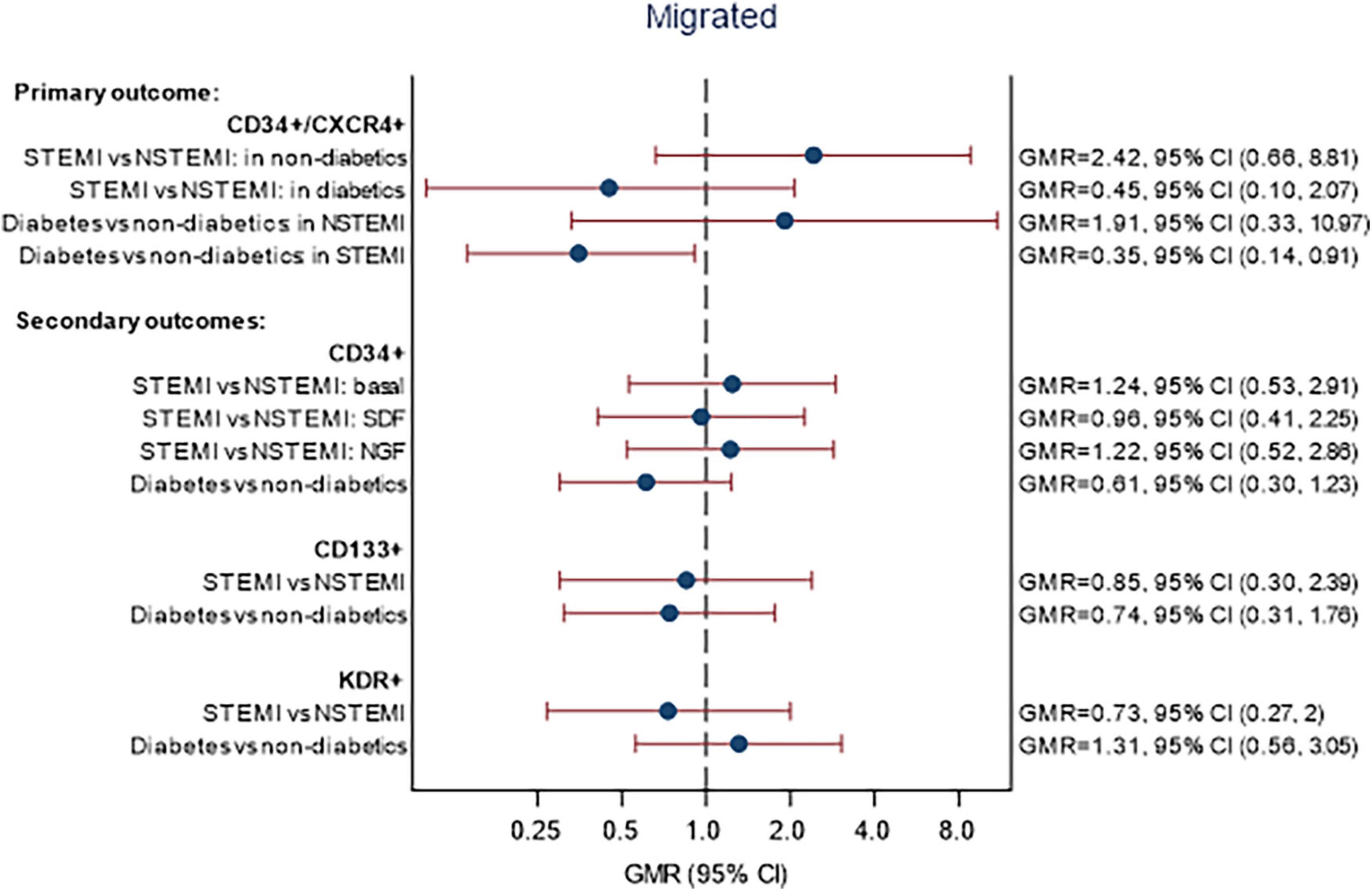
Figure 3. Associations between STEMI and DM with migration of CPCs. Models fitted to participants with migratory lab data available on day 4: 52 participants (14 STEMI with DM, 27 STEMI without DM, 5 NSTEMI with DM, 6 NSTEMI without DM). Estimates are adjusted for age, sex, and time since onset of symptoms. Migrated—p-values for interaction STEMI × DM: CD34+/CXCR4+ (p = 0.0983), CD34+ (p = 0.3235), CD133+ (p = 0.7571), KDR+ (p = 0.1383). p-Values for interaction STEMI × chemo-attractant: CD34+/CXCR4+ (p = 0.1217), CD34+ (p = 0.0908), CD133+ (p = 0.9977), KDR+ (p = 0.5262).
Finally, there was no significant association between peak troponin T or CRP on day 0 or nearest and the percentage of CPCs for the various cell markers (Supplementary Figure 3).
Objective 2: Magnetic resonance imaging and clinical outcomes
The MRI data are shown in Supplementary Table 9 for the assessment at baseline and in Supplementary Table 10 for the 3-month follow-up. The association between (a) the percentage of CD34+/CXCR4+ cells and (b) the percentage of migrated CD34+/CXCR4+ cells, with MRI outcomes are given in Figures 4, 5, respectively. No statistically significant associations were observed between the proportion of CD34+/CXCR4+ cells and MRI outcomes. There were also no statistically significant associations between migrated CD34+/CXCR4+ and the MRI outcomes, although effect sizes were stronger. For each unit increase in the proportion of migrated CD34+/CXCR4+, the longitudinal strain was on average 1.44 units lower (MD = −1.44, 95% CI −3.13, 0.25) and LVEF was 1.84 units higher (MD = 1.84, 95% CI −0.31, 3.99).
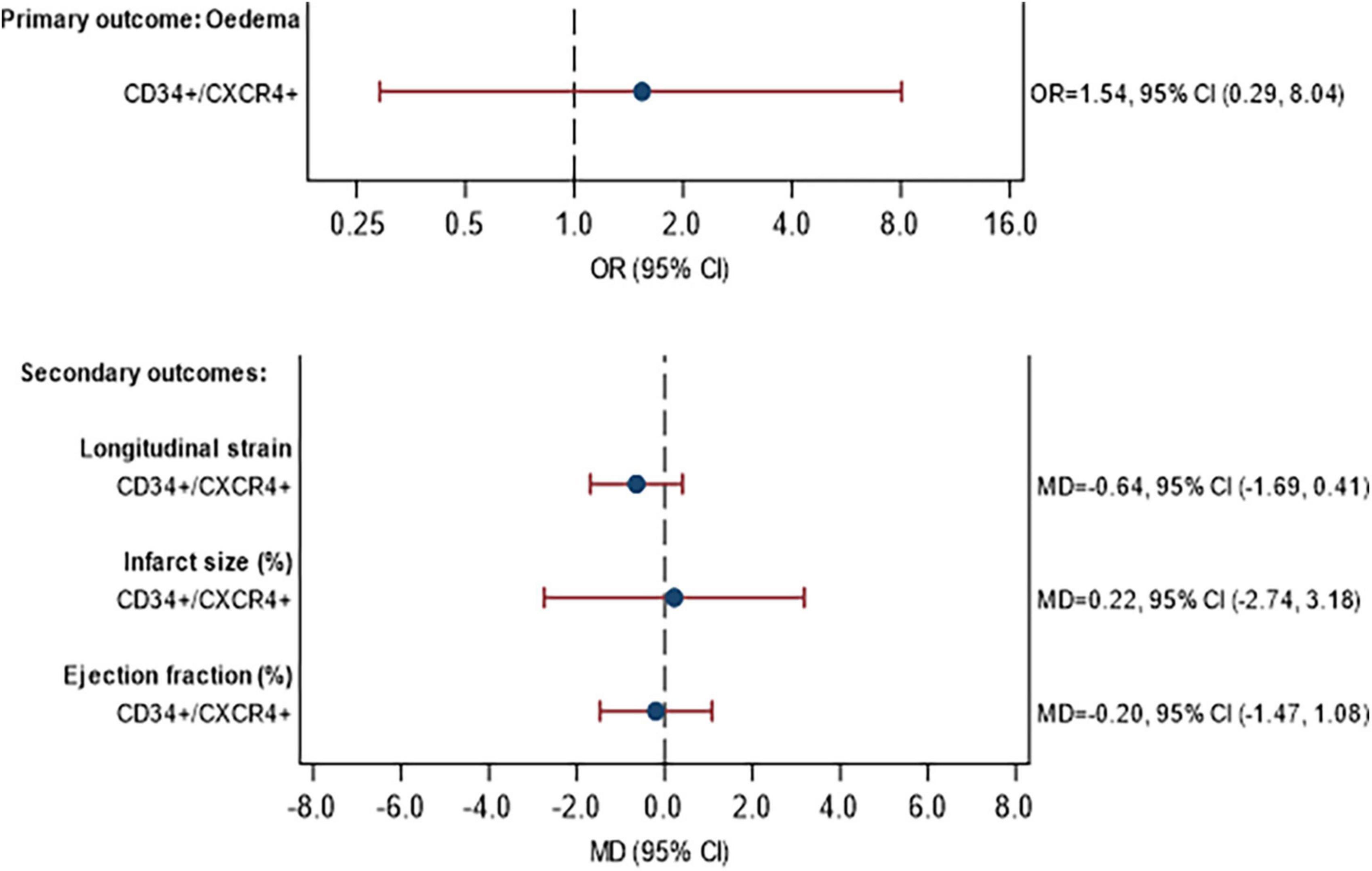
Figure 4. Association between CD34+/CXCR4+ frequency and primary and secondary MRI outcomes at 3 months. Model fitted to participants with MRI and CPC data availabl43 participants (11 STEMI with DM, 24 STEMI without DM, 3 NSTEMI with DM, 5 NSTEMI without DM), excluding participants with missing data (see below). Edema: excluding 2 participants with missing edema data at baseline or 3 months. Longitudinal strain: excluding 3 participants with missing Longitudinal strain data at baseline or 3 months. Infarct size: excluding 3 participants with missing infarct size data at baseline or 3 months. Ejection fraction: excluding 0 participants with missing ejection fraction data at baseline or 3 months. Estimates are adjusted for STEMI, diabetes, age, sex, time since onset of symptoms and baseline value of outcome being analyzed. CI, confidence interval; GMR, geometric mean ratio; OR, odds ratio; MD, mean difference.
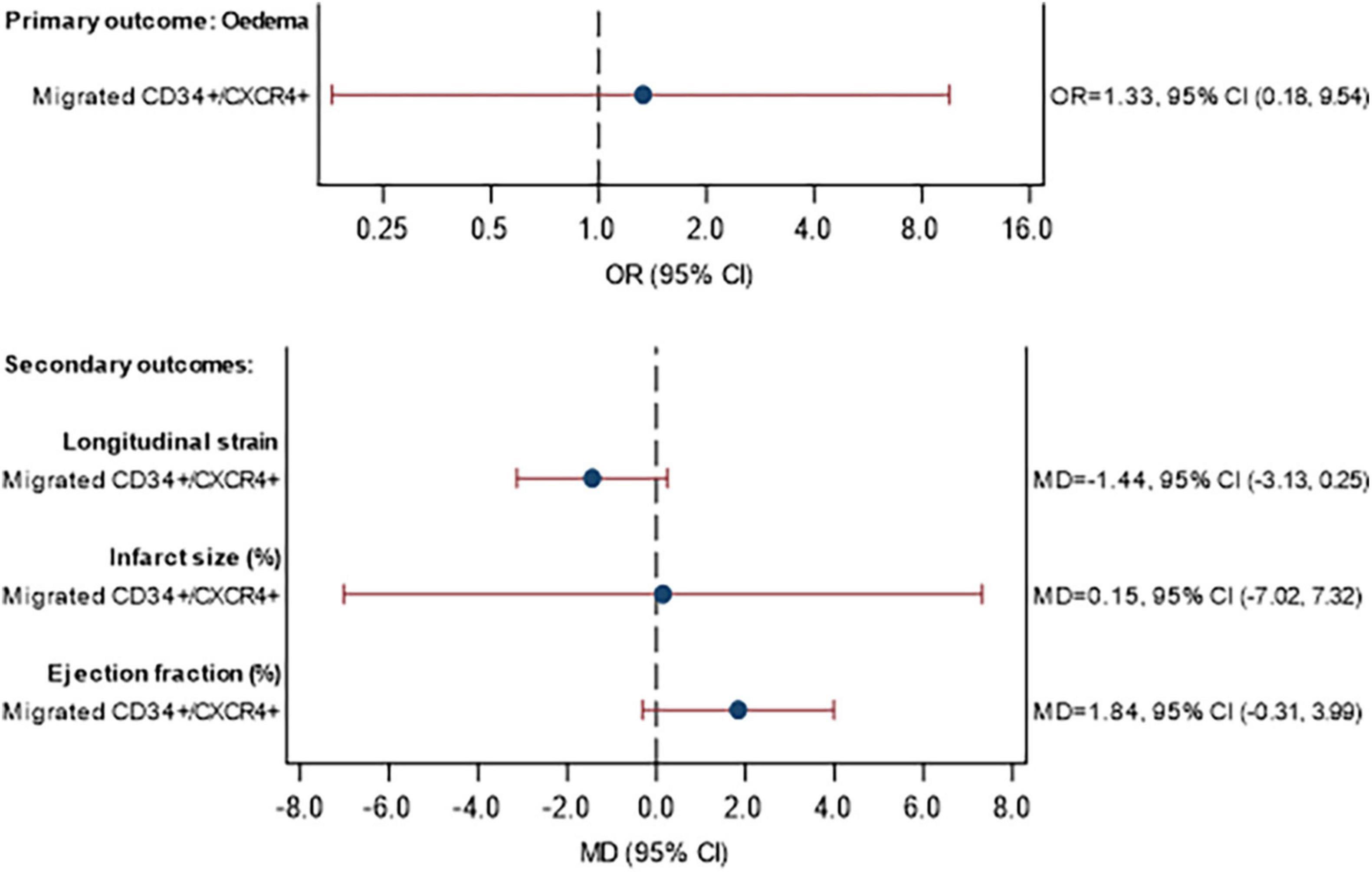
Figure 5. Association between CD34+/CXCR4+ migration and primary and secondary MRI outcomes at 3 months. Model fitted to participants with MRI and migrated CPC data available: 40 participants (11 STEMI with DM, 21 STEMI without DM, 3 NSTEMI with DM, 5 NSTEMI without DM), excluding participants with missing data (see below). Eedema: excluding 1 participant with missing eedema data at baseline or 3 months. Longitudinal strain: excluding 1 participant with missing Longitudinal strain data at baseline or 3 months. Infarct size: excluding 2 participants with missing infarct size data at baseline or 3 months Ejection fraction: excluding 0 participants with missing ejection fraction data at baseline or 3 months. Estimates are adjusted for STEMI, diabetes, age, sex, time since onset of symptoms and baseline value of outcome being analyzed. CI, confidence interval; GMR, geometric mean ratio; OR, odds ratio; MD, mean difference.
Clinical outcomes are summarized at each time point by STEMI and DM status in Table 3. Outcomes were not formally compared, as specified a priori in the study protocol. Overall, 12.7% of participants (9/71) experienced a major adverse cardiac-related event (MACE) during follow-up.
Supplementary Table 11 presents all adverse events (AEs) and SAEs reported during the study (in-hospital and during follow-up). Further details of unexpected SAEs are given in Supplementary Table 12.
Discussion
Cardiac repair after MI depends on the balance between the magnitude of ischemic injury and a regulated inflammatory and wound-healing response: larger infarcts and depressed reparative mechanisms increase the risk for developing adverse ventricular remodeling and heart failure (30). Accumulating evidence indicates that several cell types are mobilized from distant niches and reach the ischemic heart guided by a chemokine gradient. The cell migratory response has been modeled using an in vitro assay to generate useful prognostic and therapeutic insights (17). The medical value of the approach may be implemented by studying sub-categories of patients classified according to the severity of tissue injury and associated risk factors.
The present study indicates that DM impacts the relationship between myocardial damage and progenitor cell migratory response. CPC migratory activity was higher in STEMI vs. NSTEMI. However, in patients without DM, the effect of STEMI vs NSTEMI was GMR = 2.42. In patients with DM, the effect of STEMI vs. NSTEMI was GMR = 0.45; hence, the property of STEMI vs. NSTEMI was reversed in the presence of DM. In addition, there was a trend for a positive association between CPC migratory activity and LVEF at 3 months follow-up, following adjustment for STEMI, DM, age, sex, time since onset of symptoms and baseline LVEF. This suggests that the outcome after MI is advantaged in patients who have CPCs endowed with efficient migratory activity.
Shintani et al. were the first to describe the rapid mobilization of CD34+ cells in acute MI (31). The abundance of circulating CPCs peaked 7 days after the onset of MI and paralleled the significant increase in blood levels of angiogenic factors and chemokines. Moreover, nociceptive signaling to the BM can contribute to the regulation of CPC liberation from the BM (15). Massa et al. showed that hematopoietic CPCs follow the mobilization kinetics of endothelial progenitor cells; (5) however, there are discrepancies between reports regarding the time for CPCs to reach the highest levels in the PB (6, 7). A meta-analysis by Rigato et al. showed that the numerical reduction of circulating CPCs, in particular CD34+/CD133+ hematopoietic cells, was associated with a 2-fold increased risk of future cardiovascular events and cardiovascular death in patients with suspected coronary artery disease, acute coronary syndrome, previous stroke, or in patients without acute events but with cardiovascular risk factors (32). The quantitative assessment of this cell population was capable of predicting long-term adverse cardiovascular outcomes and multi-organ damage in DM patients (19, 33).
A novel aspect investigated here is the change occurring in CPC quantity during the first few days after an MI; with the expected result being that the infarct severity would influence the number of CPCs in the peripheral blood. To this aim, we measured the relative abundance of CD34+/CXCR4 CPCs on two occasions, during the first 24 h and between 84 and 132 h after MI. Results indicate that STEMI was more potent than NSTEMI in determining an increase in circulating CPCs. Intriguingly, the relative abundance of CD34+/CXCR4 CPCs in PB tended to be higher in the PB of patients with DM independent of a STEMI or NSTEMI. This result apparently contradicts the notion of a defective mobilization, alias mobilopathy, initially reported by Di Persio in people with DM (10). The sampling schedule adopted in the present study might not have been able to capture such mobilopathy. This may also explain the difference between our data and previous reports suggesting that the CPC abundance can predict the late outcome post-MI (19). In the present study, no significant association was observed between CD34+/CXCR4+ cell abundance and MRI outcomes.
We have next investigated whether the in vitro migratory activity of CPCs may reflect an intrinsic defect in the repair response with higher fidelity. This possibility is supported by the observation that migration in vitro was (1) directly proportional to the extent of myocardial damage in the comparison between STEMI vs. NSTEMI, (2) reversed in the presence of DM, and (3) predictive of a better LVEF as assessed by cardiac MRI at the three months follow up. This observation extends our previous report about the predictive value of cell migration in patients with critical limb ischemia, where a multivariable regression analysis showed that CD34+ cell migration forecasted cardiovascular mortality during a 6-year follow-up (17). This was interpreted as the consequence of altered paracrine signaling, involving the TUG1 sponge/miRNA-21/PDCD4 axis, which conveys antiangiogenic and proapoptotic features from CD34+ cells to the endothelium (17). Further research is needed to determine whether a similar mechanism is operative in CPCs mobilized after an acute MI and influenced by DM and cardiovascular risk factors.
Strengths and limitations
Despite attempts to reduce bias, this hypothesis-generating, observational study has limitations intrinsic to the small sample size. In particular, the recruitment reached the initial goal for STEMI but not for NSTEMI or cardiac MRI. In addition, no formal adjustment was made for multiple testing due to the hypothesis-generating nature of the study. Moreover, the migration assay is an in vitro test that does not reflect cell extrinsic determinants of mobilization, such as the state of the bone marrow niche, neurogenic and neurotrophic influences, and metabolic status (14, 34–40). Regardless of these limitations, the data collected for the study are, we believe, the only ones that exist regarding an interaction between infarct and diabetes on the CPC migratory activity—hence the importance of reporting the findings. The data reinforce the concept that cell migration especially if combined with an assessment of the molecular profile of migrated cells may provide useful clinical information in ischemic cardiovascular disease.
Data availability statement
The original contributions presented in this study are included in the article/Supplementary material, further inquiries can be directed to PM, bWRwcm1AYnJpc3RvbC5hYy51aw==.
Ethics statement
The studies involving human participants were reviewed and approved by Wiltshire Research 98 Ethics Committee. The patients/participants provided their written informed consent to participate in this study.
Author contributions
PM was responsible as corresponding author for the final writing and the data presented. All authors conceived and designed the article and read and approved the final manuscript.
Funding
This study was supported by a grant from the National Institute for Health Research (NIHR) Bristol Biomedical Research Unit in Cardiovascular Medicine. This work was also supported by grant number: CH/1992027/7163 to British Heart Foundation Chair Professor Gianni Angelini.
Acknowledgments
We thank Laura Collett and Eleanor Gidman for technical assistance in statistical analysis.
Conflict of interest
The authors declare that the research was conducted in the absence of any commercial or financial relationships that could be construed as a potential conflict of interest.
Publisher’s note
All claims expressed in this article are solely those of the authors and do not necessarily represent those of their affiliated organizations, or those of the publisher, the editors and the reviewers. Any product that may be evaluated in this article, or claim that may be made by its manufacturer, is not guaranteed or endorsed by the publisher.
Supplementary material
The Supplementary Material for this article can be found online at: https://www.frontiersin.org/articles/10.3389/fcvm.2022.1011140/full#supplementary-material
References
1. Hilgendorf I, Gerhardt LM, Tan TC, Winter C, Holderried TA, Chousterman BG, et al. Ly-6Chigh monocytes depend on Nr4a1 to balance both inflammatory and reparative phases in the infarcted myocardium. Circ Res. (2014) 114:1611–22. doi: 10.1161/CIRCRESAHA.114.303204
2. Horckmans M, Ring L, Duchene J, Santovito D, Schloss MJ, Drechsler M, et al. Neutrophils orchestrate post-myocardial infarction healing by polarizing macrophages towards a reparative phenotype. Eur Heart J. (2017) 38:187–97. doi: 10.1093/eurheartj/ehw002
3. Wojakowski W, Landmesser U, Bachowski R, Jadczyk T, Tendera M. Mobilization of stem and progenitor cells in cardiovascular diseases. Leukemia. (2012) 26:23–33. doi: 10.1038/leu.2011.184
4. Cui YX, Johnson T, Baumbach A, Reeves BC, Rogers CA, Angelini GD, et al. Stepwise optimization of the procedure for assessment of circulating progenitor cells in patients with myocardial infarction. PLoS One. (2012) 7:e30389. doi: 10.1371/journal.pone.0030389
5. Massa M, Rosti V, Ferrario M, Campanelli R, Ramajoli I, Rosso R, et al. Increased circulating hematopoietic and endothelial progenitor cells in the early phase of acute myocardial infarction. Blood. (2005) 105:199–206. doi: 10.1182/blood-2004-05-1831
6. Wojakowski W, Tendera M, Michałowska A, Majka M, Kucia M, Maślankiewicz K, et al. Mobilization of CD34/CXCR4+, CD34/CD117+, c-met+ stem cells, and mononuclear cells expressing early cardiac, muscle, and endothelial markers into peripheral blood in patients with acute myocardial infarction. Circulation. (2004) 110:3213–20. doi: 10.1161/01.CIR.0000147609.39780.02
7. Leone AM, Rutella S, Bonanno G, Abbate A, Rebuzzi AG, Giovannini S, et al. Mobilization of bone marrow-derived stem cells after myocardial infarction and left ventricular function. Eur Heart J. (2005) 26:1196–204. doi: 10.1093/eurheartj/ehi164
8. Fadini GP, Albiero M, Vigili de Kreutzenberg S, Boscaro E, Cappellari R, Marescotti M, et al. Diabetes impairs stem cell and proangiogenic cell mobilization in humans. Diabetes Care. (2013) 36:943–9. doi: 10.2337/dc12-1084
9. Fadini GP, DiPersio JF. Diabetes mellitus as a poor mobilizer condition. Blood Rev. (2018) 32:184–91. doi: 10.1016/j.blre.2017.11.002
10. DiPersio JF. Diabetic stem-cell “mobilopathy”. N Engl J Med. (2011) 365:2536–8. doi: 10.1056/NEJMcibr1112347
11. Ferland-McCollough D, Maselli D, Spinetti G, Sambataro M, Sullivan N, Blom A, et al. MCP-1 feedback loop between adipocytes and mesenchymal stromal cells causes fat accumulation and contributes to hematopoietic stem cell rarefaction in the bone marrow of patients with diabetes. Diabetes. (2018) 67:1380–94. doi: 10.2337/db18-0044
12. Dang Z, Maselli D, Spinetti G, Sangalli E, Carnelli F, Rosa F, et al. Sensory neuropathy hampers nociception-mediated bone marrow stem cell release in mice and patients with diabetes. Diabetologia. (2015) 58:2653–62. doi: 10.1007/s00125-015-3735-0
13. Spinetti G, Cordella D, Fortunato O, Sangalli E, Losa S, Gotti A, et al. Global remodeling of the vascular stem cell niche in bone marrow of diabetic patients: implication of the microRNA-155/FOXO3a signaling pathway. Circ Res. (2013) 112:510–22. doi: 10.1161/CIRCRESAHA.112.300598
14. Oikawa A, Siragusa M, Quaini F, Mangialardi G, Katare RG, Caporali A, et al. Diabetes mellitus induces bone marrow microangiopathy. Arterioscler Thromb Vasc Biol. (2010) 30:498–508. doi: 10.1161/ATVBAHA.109.200154
15. Amadesi S, Reni C, Katare R, Meloni M, Oikawa A, Beltrami AP, et al. Role for substance p-based nociceptive signaling in progenitor cell activation and angiogenesis during ischemia in mice and in human subjects. Circulation. (2012) 125:1774–86; S1–19. doi: 10.1161/CIRCULATIONAHA.111.089763
16. Sangalli E, Tagliabue E, Sala L, Prattichizzo F, Uccellatore A, Spada D, et al. Circulating MicroRNA-15a associates with retinal damage in patients with early stage type 2 diabetes. Front Endocrinol (Lausanne). (2020) 11:254. doi: 10.3389/fendo.2020.00254
17. Spinetti G, Sangalli E, Tagliabue E, Maselli D, Colpani O, Ferland-McCollough D, et al. MicroRNA-21/PDCD4 proapoptotic signaling from circulating CD34(+) cells to vascular endothelial cells: a potential contributor to adverse cardiovascular outcomes in patients with critical limb ischemia. Diabetes Care. (2020) 43:1520–9. doi: 10.2337/dc19-2227
18. Spinetti G, Fortunato O, Caporali A, Shantikumar S, Marchetti M, Meloni M, et al. MicroRNA-15a and microRNA-16 impair human circulating proangiogenic cell functions and are increased in the proangiogenic cells and serum of patients with critical limb ischemia. Circ Res. (2013) 112:335–46. doi: 10.1161/CIRCRESAHA.111.300418
19. Fadini GP, Rigato M, Cappellari R, Bonora BM, Avogaro A. Long-term prediction of cardiovascular outcomes by circulating CD34+ and CD34+CD133+ stem cells in patients with type 2 diabetes. Diabetes Care. (2017) 40:125–31. doi: 10.2337/dc16-1755
20. Spinetti G, Specchia C, Fortunato O, Sangalli E, Clerici G, Caminiti M, et al. Migratory activity of circulating mononuclear cells is associated with cardiovascular mortality in type 2 diabetic patients with critical limb ischemia. Diabetes Care. (2014) 37:1410–7. doi: 10.2337/dc13-2084
21. Fortunato O, Spinetti G, Specchia C, Cangiano E, Valgimigli M, Madeddu P. Migratory activity of circulating progenitor cells and serum SDF-1alpha predict adverse events in patients with myocardial infarction. Cardiovasc Res. (2013) 100:192–200. doi: 10.1093/cvr/cvt153
22. Gerstein HC, Colhoun HM, Dagenais GR, Diaz R, Lakshmanan M, Pais P, et al. Dulaglutide and cardiovascular outcomes in type 2 diabetes (REWIND): a double-blind, randomised placebo-controlled trial. Lancet. (2019) 394:121–30.
23. Ferrannini G, Gerstein H, Colhoun HM, Dagenais GR, Diaz R, Dyal L, et al. Similar cardiovascular outcomes in patients with diabetes and established or high risk for coronary vascular disease treated with dulaglutide with and without baseline metformin. Eur Heart J. (2021) 42:2565–73. doi: 10.1093/eurheartj/ehaa777
24. Xie D, Li Y, Xu M, Zhao X, Chen M. Effects of dulaglutide on endothelial progenitor cells and arterial elasticity in patients with type 2 diabetes mellitus. Cardiovasc Diabetol. (2022) 21:200. doi: 10.1186/s12933-022-01634-1
25. Nakamura K, Oe H, Kihara H, Shimada K, Fukuda S, Watanabe K, et al. DPP-4 inhibitor and alpha-glucosidase inhibitor equally improve endothelial function in patients with type 2 diabetes: EDGE study. Cardiovasc Diabetol. (2014) 13:110. doi: 10.1186/s12933-014-0110-2
26. Vinci MC, Gambini E, Bassetti B, Genovese S, Pompilio G. When good guys turn bad: bone marrow’s and hematopoietic stem cells’ role in the pathobiology of diabetic complications. Int J Mol Sci. (2020) 21:3864. doi: 10.3390/ijms21113864
27. Tedesco S, Ciciliot S, Menegazzo L, D’Anna M, Scattolini V, Cappellari R, et al. Pharmacologic PPAR-gamma activation reprograms bone marrow macrophages and partially rescues HSPC mobilization in human and murine diabetes. Diabetes. (2020) 69:1562–72. doi: 10.2337/db19-0640
28. Sandoval Y, Thygesen K, Jaffe AS. The universal definition of myocardial infarction: present and future. Circulation. (2020) 141:1434–6. doi: 10.1161/CIRCULATIONAHA.120.045708
29. Cosentino F, Grant PJ, Aboyans V, Bailey CJ, Ceriello A, Delgado V, et al. 2019 ESC guidelines on diabetes, pre-diabetes, and cardiovascular diseases developed in collaboration with the EASD. Eur Heart J. (2020) 41:255–323.
30. Prabhu SD, Frangogiannis NG. The biological basis for cardiac repair after myocardial infarction: from inflammation to fibrosis. Circ Res. (2016) 119:91–112. doi: 10.1161/CIRCRESAHA.116.303577
31. Shintani S, Murohara T, Ikeda H, Ueno T, Honma T, Katoh A, et al. Mobilization of endothelial progenitor cells in patients with acute myocardial infarction. Circulation. (2001) 103:2776–9. doi: 10.1161/hc2301.092122
32. Rigato M, Avogaro A, Fadini GP. Levels of circulating progenitor cells, cardiovascular outcomes and death: a meta-analysis of prospective observational studies. Circ Res. (2016) 118:1930–9. doi: 10.1161/CIRCRESAHA.116.308366
33. Fadini GP, Albiero M. Impaired hematopoietic stem/progenitor cell traffic and multi-organ damage in diabetes. Stem Cells. (2022) 40:716–23. doi: 10.1093/stmcls/sxac035
34. Garcia-Garcia A, Korn C, Garcia-Fernandez M, Dominques O, Villadiego J, Martin-Perez D, et al. Dual cholinergic signals regulate daily migration of hematopoietic stem cells and leukocytes. Blood. (2019) 133:224–36.
35. Boscari F, D’Anna M, Bonora BM, Tresso S, Cappellari R, Avogaro A, et al. Effects of glucose variability on hematopoietic stem/progenitor cells in patients with type 1 diabetes. J Endocrinol Invest. (2021) 44:119–26. doi: 10.1007/s40618-020-01278-6
36. Albiero M, Tedesco S, Amendolagine FI, D’Anna M, Migliozzi L, Zuccolotto G, et al. Inhibition of SGLT2 rescues bone marrow cell traffic for vascular repair: role of glucose control and ketogenesis. Diabetes. (2021) 70:1767–79. doi: 10.2337/db20-1045
37. Dang Z, Avolio E, Albertario A, Sala-Newby GB, Thomas AC, Wang N, et al. Nerve growth factor gene therapy improves bone marrow sensory innervation and nociceptor-mediated stem cell release in a mouse model of type 1 diabetes with limb ischaemia. Diabetologia. (2019) 62:1297–311. doi: 10.1007/s00125-019-4860-y
38. Hoyer FF, Zhang X, Coppin E, Vasamsetti SB, Modugu G, Schloss MJ, et al. Bone marrow endothelial cells regulate myelopoiesis in diabetes mellitus. Circulation. (2020) 142:244–58. doi: 10.1161/CIRCULATIONAHA.120.046038
39. Albiero M, D’Anna M, Bonora BM, Zuccolotto G, Rosato A, Giorgio M, et al. Hematopoietic and nonhematopoietic p66Shc differentially regulates stem cell traffic and vascular response to ischemia in diabetes. Antioxid Redox Signal. (2022) 36:593–607. doi: 10.1089/ars.2021.0097
Keywords: progenitor cells, migration, myocardial infarction, cardiac MRI, diabetes mellitus
Citation: Baumbach A, Cui Y-X, Evans RN, Culliford L, Johnson T, Rogers CA, Reeves BC, Bucciarelli-Ducci C, Harris J, Hamilton M and Madeddu P (2022) A cohort study of circulating progenitor cells after ST-segment elevation and non-ST segment elevation myocardial infarction in non-diabetic and diabetic patients. Front. Cardiovasc. Med. 9:1011140. doi: 10.3389/fcvm.2022.1011140
Received: 03 August 2022; Accepted: 03 November 2022;
Published: 17 November 2022.
Edited by:
Antonino Bruno, University of Insubria, ItalyReviewed by:
Chunying Li, Georgia State University, United StatesChunying Jiang, Xuzhou Central Hospital, China
Copyright © 2022 Baumbach, Cui, Evans, Culliford, Johnson, Rogers, Reeves, Bucciarelli-Ducci, Harris, Hamilton and Madeddu. This is an open-access article distributed under the terms of the Creative Commons Attribution License (CC BY). The use, distribution or reproduction in other forums is permitted, provided the original author(s) and the copyright owner(s) are credited and that the original publication in this journal is cited, in accordance with accepted academic practice. No use, distribution or reproduction is permitted which does not comply with these terms.
*Correspondence: Andreas Baumbach, YS5iYXVtYmFjaEBxbXVsLmFjLnVr; Paolo Madeddu, bWRwcm1AYnJpc3RvbC5hYy51aw==