- 1Department of Advanced Medical and Surgical Sciences, University of Campania “Luigi Vanvitelli”, Naples, Italy
- 2Department of Experimental Medicine, Section of Pharmacology “L. Donatelli”, University of Campania “Luigi Vanvitelli”, Naples, Italy
- 3Mediterranea Cardiocentro, Napoli, Italy
Atherosclerosis is a progressive inflammatory disease leading to mortality and morbidity in the civilized world. Atherosclerosis manifests as an accumulation of plaques in the intimal layer of the arterial wall that, by its subsequent erosion or rupture, triggers cardiovascular diseases. Diabetes mellitus is a well-known risk factor for atherosclerosis. Indeed, Type 2 diabetes mellitus patients have an increased risk of atherosclerosis and its associated-cardiovascular complications than non-diabetic patients. Sodium-glucose co-transport 2 inhibitors (SGLT2i), a novel anti-diabetic drugs, have a surprising advantage in cardiovascular effects, such as reducing cardiovascular death in a patient with or without diabetes. Numerous studies have shown that atherosclerosis is due to a significant inflammatory burden and that SGLT2i may play a role in inflammation. In fact, several experiment results have demonstrated that SGLT2i, with suppression of inflammatory mechanism, slows the progression of atherosclerosis. Therefore, SGLT2i may have a double benefit in terms of glycemic control and control of the atherosclerotic process at a myocardial and vascular level. This review elaborates on the anti-inflammatory effects of sodium-glucose co-transporter 2 inhibitors on atherosclerosis.
Introduction
Atherosclerosis is a widespread chronic inflammatory disorder of large- and medium-caliber artery walls with a complex biochemical and cellular etiology (1). It is characterized by the accumulation of immune-system and endothelial cells, lipid particles, and extracellular matrix components in the sub-endothelial layer (2). In the final stage, atherosclerosis exhibits the development of plaques at the intimal layer of the arterial wall, triggering cardiovascular diseases (CVDs) caused by subsequent erosion or rupture of the atherosclerotic plaques (3).
Therefore, to date, it still represents the major potential risk factor of most CVDs, including myocardial infarction (MI), heart failure (HF), stroke, and peripheral arterial disease, causing disability and mortality worldwide (4).
Cardiovascular diseases are the leading cause of death globally. Over 17.9 million people died yearly from CVDs in 2019, representing 32% of all deaths worldwide. 85% of these deaths were due to heart attack and stroke (5). Globally, over three-quarters of CVDs deaths occur in low- and middle-income countries because individuals with CVDs have limited healthcare access, delaying CVDs detection and increasing mortality from CVDs. CVDs lead to 18% of disability-adjusted life years lost in high-income countries and 10% in low-income and middle-income countries, placing a heavy burden on the economies of developing countries (5, 6). CVDs account for 62% of European healthcare costs of 169 billion euros annually (6), and most countries cannot maintain such costs. Adopting a healthy lifestyle such as reducing tobacco and alcohol, avoiding an unhealthy diet, and performing physical activity should markedly reduce the atherosclerosis burden and its complications. However, it is essential to detect cardiovascular diseases as soon as possible to begin managing them with counseling and medicines (7).
Atherosclerosis results from predisposing genetic factors and exposure to oxidative and inflammatory damage mediators. In addition, modifiable and non-modifiable risk factors, which include age, sex, cigarette smoking, unhealthy diet, physical inactivity, dyslipidemia, diabetes mellitus, hypertension, and obesity, contribute to its beginning and development (8). Diabetes mellitus is a well-known risk factor for atherosclerosis. Subjects affected by type 2 diabetes mellitus (T2DM) have an increased risk of atherosclerosis and its associated-cardiovascular complications than non-diabetic patients (9). To date, a new class of anti-hyperglycemic drugs, sodium-glucose co-transport 2 inhibitors (SGLT2i), represent a therapeutic novelty essential for their pleiotropic effects. In addition to glycemic control, SGLT2i is known for the cardio and nephron-protective role and, more recently, for the anti-inflammatory effect. Several experimental results have shown that SGLT2i, with suppression of inflammation, slows the progression of atherosclerosis (10).
This review reports experimental and clinical evidence to clarify the anti-inflammatory mechanisms underlying the anti-atherosclerotic effect of SGLT2i.
Methods
A literature search was conducted by searching updated and relevant publications on SGLT2i and atherosclerosis in databases, including PubMed and Google Scholar. During this research, keywords such as “SGLT2 inhibitor, empagliflozin, dapagliflozin, canagliflozin, ertugliflozin, ipragliflozin, luseogliflozin, sotagliflozin, atherosclerosis, atherosclerosis cardiovascular diseases, inflammation, coronary heart disease, stroke, angina pectoris, myocardial infarction and peripheral artery disease, major adverse cardiac events (MACE), cardiovascular (CV) mortality” were used. In vitro and animal studies, clinical trials, reviews, meta-analyses, and guidelines were reviewed. We evaluated the anti-inflammatory effects of SGLT2i from experimental evidence (in vitro and animal models) and clinical trials. For the clinical trials, we considered the cardiovascular outcomes, including myocardial infarction, stroke, peripheral artery disease, cardiovascular death, or hospitalization for heart failure. Articles not in the English language or meeting abstracts were excluded.
Atherosclerosis: From inflammation to complications
Atherosclerosis disease can be schematized in three stages, starting from the endothelium activation/dysfunction and resulting in plaque formation (1).
In the initial stages of the lesion, endothelial dysfunction occurs under harmful stimuli such as hypertension, dyslipidemia, and disturbed shear stress resulting in a chronic inflammatory state. Parallel changes in endothelial permeability promote oxidation of low-density lipoproteins (LDL), followed by infiltration of monocytes in the intimal layer (11). Oxidized-LDL (ox-LDL) promotes damage-associated molecular patterns (DAMPs) secretion that initialize an innate immune response by Toll-like receptors (TLR). Ox-LDL accumulation induces the expression of adhesion molecules as vascular cell adhesion molecule 1 (VCAM-1) by endothelial cells, which recall other monocytes and leukocytes (12). In turn, monocytes transform themselves into activated macrophages altering the ratio between M1 and M2 macrophages. Specifically, based on the macrophage activation process, M1 macrophages are activated in response to TLR ligands, interferons, lipopolysaccharides (LPS), and lipoproteins and express the main pro-inflammatory molecules IL (interleukins)-1β, IL-6, and TNF-α (tumor necrosis factor-α) (13). Therefore, M1 macrophages play significant roles in maintaining chronic inflammation, forming foam cells, and plaque initiation and progression (14).
Inversely, M2 macrophages are associated with an anti-inflammatory phenotype being polarized in response to IL-4 and IL-13 and producing anti-inflammatory factors such as the IL-1 receptor agonist, transforming growth factor beta (TGF-β), and IL-10 (13, 15). Consequently, the imbalance of these polarized macrophages may be responsible for plaque development or regression (16) (Figure 1).
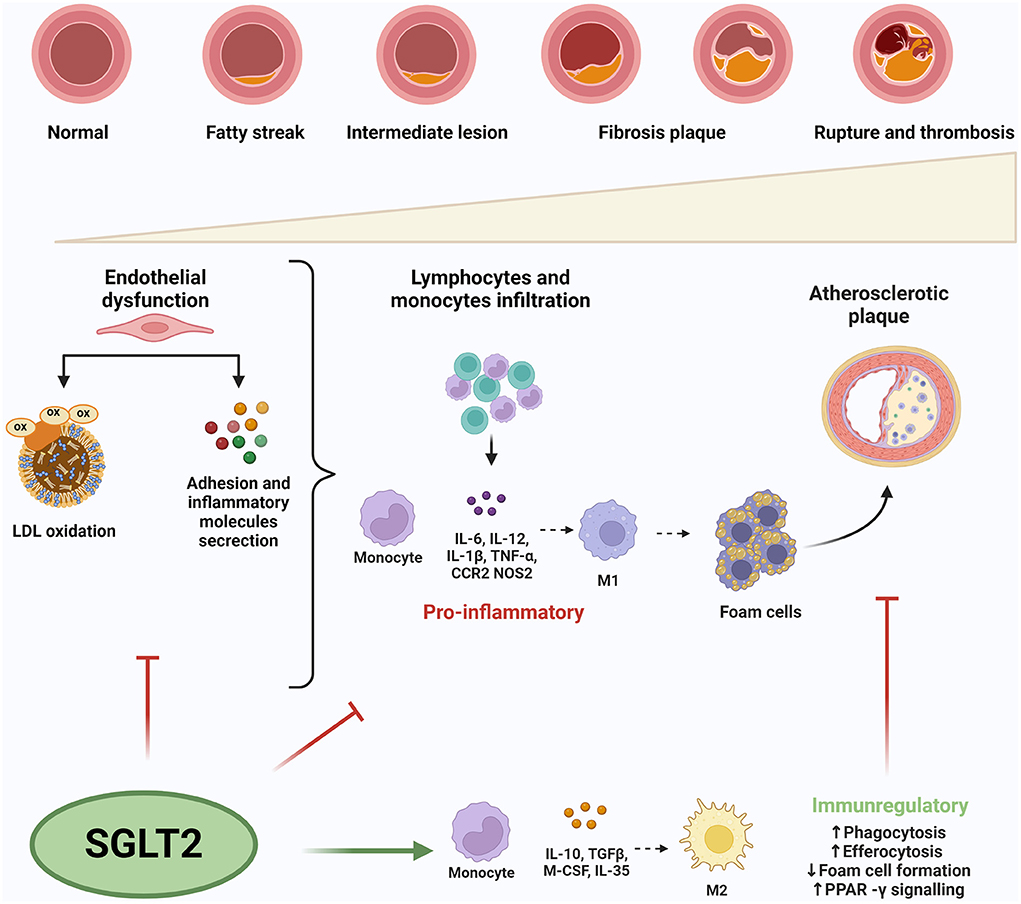
Figure 1. Sodium-glucose co-transport 2 inhibitors' effects on inflammation in atherosclerosis. SGLT2i inhibit endothelial dysfunction reducing the expression of circulating inflammatory molecules and LDL-oxidation. Moreover, SGLT2i attenuate macrophages infiltration, M1 polarization and foam cell formation. M1 macrophages express the main pro-inflammatory molecules playing role in maintaining chronic inflammation, forming foam cells, and plaque initiation and progression Inversely, M2 macrophages are associated with an anti-inflammatory phenotype producing anti-inflammatory factors. The imbalance of these,polarized macrophages may be responsible for plaque development or regression. ox-LDL, oxidized-LDL; ILs, interleukins; TNF-α, tumor necrosis factor-α; CCR2, C-C chemokine receptor type 2; NOS 2, nitric oxidase synthase 2; TGF-β, transforming growth factor beta; M-CSF, macrophage colony-stimulating factor.
In addition, the lipids accumulation in macrophages, with associated foam cell formation, results in the activation of the nucleotide-binding domain-like receptor protein 3 (NLRP3) inflammasome complex, which promotes the release of pro-inflammatory cytokines contributing to the development and progression of atherosclerosis (17). Indeed, inflammasome activation causes the amplification of the inflammatory response by promoting the expression of adhesion molecules, a proliferation of vascular smooth muscle cells, and the activation of macrophages (18).
Moreover, in the atherogenesis process, oxidative stress and cellular senescence contribute to the maintenance of inflammation and endothelial dysfunction. Indeed, reactive oxygen species (ROS) induce both the synthesis of pro-inflammatory cytokines and stimulate the expression of adhesion molecules, thus allowing monocytes to transmigrate into the vessel wall (19). In addition, ROS can promote the expression of scavenger receptors on vascular smooth muscle cells, promoting lipid accumulation and transformation into foamy cells (17).
Senescence-inducing stress can result from various cardiovascular risk factors (20). Indeed, senescent cells exhibit a specific senescence-associated secretory phenotype (SASP), which consists of inflammatory cytokines, chemokines, growth factors, and proteases (21). The accumulation of senescent cells progressively results in chronic low-grade inflammation, termed “inflammaging” (21). Senescent endothelial cells show altered permeability and nitric oxide (NO) production at the vascular level, inducing endothelial dysfunction. Senescent vascular smooth muscle cells, on the other hand, show reduced proliferation and an increased tendency to apoptosis. Therefore, the accumulation of senescent cells in atherosclerotic lesions may promote plaque progression (22).
In the second stage, characterized by plaque progression, smooth muscle cells produce extracellular matrix components contributing to plaque thickening and progressive growth in the vessel's lumen. In addition, macrophages and smooth muscle cells undergo apoptosis by going on to form a central lipid-rich core. Therefore, in the late stage, the plaque consists of a central lipid core and a fibrous cap (23). Pro-inflammatory cytokines, such as IL-1, IL-6, Interferons (IFN) gamma, metalloproteases-2 and 9 (MMP-2 and MMP-9), and metallopeptidase inhibitors (TIMP-1, TIMP-2), are responsible for plaque erosion/rupture. Specifically, IL-6 plays a crucial role in inducing a prothrombotic state by positive regulation of plasminogen activator inhibitor type 1 (PAI-1) during the acute inflammation phase response and negative regulation of antithrombin and protein S (24). Moreover, IL-6 induces the up-regulation of adhesion molecules in the endothelial cells and increases vascular permeability and cellular dysfunction (25).
Therefore, it is possible to distinguish two types of plaques:
- Stable plaques, which possess a thick fibrous cap. It can regress with the proper lifestyle and/or drug therapy or may progress by occluding the vessel lumen.
- Vulnerable or unstable plaques are rich in macrophages and possess a thick lipid core and a thin fibrous cap. It is more likely to undergo rupture and complications (26).
As a result, the cardiac complications of atherosclerotic disease are related to (I) reduced blood flow due to an insufficient oxygen supply, such as angina pectoris; (II) plaque can occlude the vessel leading to ischemia of downstream tissues, for example, in myocardial infarction or stroke; (III) detachment of part of the thrombus, termed an embolus can result in occlusions of distal arteries as pulmonary embolism (7).
SGLT2 inhibitors
The SGLT2i are a new category of anti-diabetic drugs, approved by the Food and Drug Administration (FDA), used to treat patients with type 2 diabetes (27). SGLT2i family includes dapagliflozin, empagliflozin, luseogliflozin, ipragliflozin, phlorizin, and canagliflozin (28).
Sodium-glucose co-transport 2 inhibitors, targeting the major glucose transporter SGLT2 in the kidney, block glucose reabsorption in the proximal renal tubule, increasing glycosuria independently of insulin sensitivity and secretion (29). The SGLT2 inhibition reduces glucose reabsorption, promotes urinary glucose secretion, and causes a negative caloric balance (30).
Phlorizin was the first natural SGLT2i isolated from the root bark of apple trees. Phlorizin, binding the extracellular surface of SGLT1 and SGLT2 in the presence of Na+, inhibits proteins in a reversible, competitive way (31). However, caused of its poor solubility in water and low absorption in the gastrointestinal tract, several molecules with a similar structure were subsequently developed (32).
Many compounds with increased stability, bioavailability, and high selectivity for SGLT2 over SGLT1 have been identified. Currently, only four molecules are licensed by the European Medicines Agency (EMA) and the FDA: canagliflozin, dapagliflozin, and empagliflozin (33).
In addition to structural differences, these compounds present variable selectivity to SGLT1 and SGLT2. Empagliflozin has the greatest selectivity for SGLT2 (>2,500-fold) compared to SGLT1. The binding affinity of dapagliflozin for SGLT2 is 100-fold higher than that for SGLT1, and Canagliflozin shows an SGLT2 selectivity of 250-fold (34).
Despite different selectivity, SGLT2i share similar pharmaco-kinetic characteristics, such as rapid oral absorption, a long half-life, extensive hepatic metabolism, low renal elimination, and the absence of drug-drug interactions (28).
Besides their effects on blood glucose, preclinical and clinical studies have demonstrated that SGLT2i therapy provides extra-glycemic effects and clinical advantages in patients with diabetes (35), such as improved metabolic control and cardiac and renal protection. In particular, being renal proximal, the mainly SGLT2i target, these drugs alleviate albuminuria and reduce the glomerular filtration rate (eGFR) and the plasma urate levels (36).
In addition, SGLT2 inhibitors have been convincingly demonstrated to reduce main adverse cardiovascular events and the occurrence of heart failure with reduced ejection fraction (HFrEF), independently of glycemic status (37). Interestingly, these cardio-protective effects appear independent of glucose-control efficacy and attributable to their direct and indirect action on organs (38). In particular, cardiovascular protection is related to hemodynamic mechanisms and the metabolic and anti-inflammatory effects. Indeed, SGLT2i improves lipid profile, reduces blood pressure, uricemia, visceral fat, body weight, and inflammatory markers, and reduces cardiovascular events and mortality (39–41).
Several studies have been conducted to establish underlying mechanisms of SGLT2i cardio-protective effects. In particular, studies conducted in animal models and patients with diabetes demonstrated the anti-atherosclerotic action of SGLT2i, revealing some potential mechanisms.
Nevertheless, the molecular mechanisms are still unclear (42). This gap is mainly due to the absence of evidence showing SGLT2 expression in monocytes/macrophages and endothelial cells.
The mechanisms underlying the SGLT2 benefit on atherosclerosis could be multifactorial and due to attenuation of secretion of inflammatory molecules, reduction of macrophage infiltration, improvement of autophagy impairment, and inhibition of endothelial dysfunction (43) out of their glucose-lowering effects (44).
Therefore, considering the clinically beneficial effects of SGLT2 on atherosclerosis, we provide an insightful overview focusing on mechanisms by which SGLT2i may act on inflammation in atherosclerosis and atherosclerosis cardiovascular diseases.
Effects of SGLT2 inhibitors on inflammation in atherosclerosis
As described before, inflammation is a significant factor in vascular cell dysfunction, causing the development and progression of atherosclerosis in diabetes (45).
The atherosclerosis treatment from an inflammatory perspective could be a valid therapeutic strategy. Clinical trials have demonstrated that modulation of inflammation can prevent atherosclerosis and its complications.
Recent studies have shown an improvement in inflammatory and oxidative status in subjects with T2DM treated with SGLT2i regardless of glycemic control (46). These data support the hypothesis that SGLT2i show cardio-protective effects action on inflammation.
Therefore, inflammation may be considered a mechanism by which SGLT2i can exert their protective effects against atherosclerosis. This role can be discussed regarding the impact of SGLT-2i on systemic inflammation and immune signaling pathways, including changes in local atherosclerotic tissues.
Experimental evidence
Experimental evidence revealed that SGLT2i reduced the expression of circulating inflammatory molecules, such as TNF-α, monocyte chemoattractant protein 1 (MCP-1), platelet endothelial cell adhesion molecule-1 (PECAM-1), VCAM-1, intercellular adhesion molecule 1 (ICAM-1), IL-1β, and IL-6 (47–50). Several studies have described potential mechanisms by which SGLT2i inhibit the expression of inflammatory molecules. In cultured human endothelial cells, canagliflozin impeded the release of IL-6 and MCP-1 induced by IL-1β in an 5' adenosine monophosphate-activated protein kinase (AMPK)-dependent manner (51).
Human umbilical vein endothelial cells (HUVECs) and macrophages exposed to dapagliflozin and LPS showed attenuate levels of LPS-induced TLR-4 expression, NF-κB p65 phosphorylation, and miR-155 and elevated levels of miR-146a. Moreover, dapagliflozin shifted from inflammatory M1 macrophages toward M2-dominant macrophages (52).
In LPS-stimulated RAW 264.7 macrophages, empagliflozin in association with gemigliptin and Dipeptidyl peptidase-4 (DPP-IV) inhibitor reduced gene expression and pro-inflammatory cytokine and chemokine release through the IKK/NF-κB/JAK2 - STAT1/3, and MKK4/7- JNK pathways (53).
Similar results were also obtained in mouse models. In nicotinamide and streptozotocin (NA/STZ)-treated ApoE KO mice, the treatment with luseogliflozin reduces the expression of inflammation-related genes, including F4/80, TNFα, IL-1β, IL-6, ICAM-1, PECAM-1, MMP2 and MMP9 (47).
In STZ-treated ApoE–/– mice, dapagliflozin inhibited IL-1β and IL-18 secretion, blocking the ROS-NLRP3-caspase-1 pathway showing that NLRP3 inflammasome complex could be considered an SGLT2i target (49, 54).
Two mechanisms by which empagliflozin inhibited NLRP3 activation were identified, one is dependent on β-hydroxybutyrate (BHB), and the other is Ca+ dependent. Specifically, ex vivo experiments with macrophages demonstrated that empagliflozin inhibited NLRP3, increasing BHB levels and reducing glucose, uric acid, and insulin (55). Empagliflozin reduced Ca+ levels by attenuating Na+ intracellular accumulation, causing the NLRP3 inhibition and improving functional recovery (56).
Moreover, it was demonstrated that also dapagliflozin reduced the production of NLRP3 protein by activating mTOR Complex 2 (mTORC2), leading to the activation of AMPK and Forkhead box 3 (FOXO3) (57, 58).
Considerable evidence showed that SGLT2i ameliorated endothelial dysfunction and improved endothelium-dependent vasodilation. In particular, in diabetic ApoE –/– mice, empagliflozin improved endothelial function by reducing CD68, MCP-1, ICAM-1, and TNF-α, suppressing the development and progression of atherosclerotic lesions (59). In a High-fat diet (HFD)-induced obese C577BL/6J MICE, empagliflozin increased plasma levels of Fibroblast Growth Factor 21 (FGF21), protecting the cells from damage caused by atherosclerosis-associated oxidative stress (60). Ipragliflozin reduced oxidative stress markers, thiobarbituric acid reactive substances (TBARS), and inflammation proteins (CRP, TNF-α, IL-6, and MCP-1) in streptozotocin-nicotinamide-induced diabetic mice (61). In abdominal aortic aneurysm induced by Angiotensin II infusion in apolipoprotein mice, the treatment with empagliflozin inhibited leukocyte-endothelial cell interactions, macrophages infiltration, and secretion of pro-inflammatory markers such as chemokine (C-C motif) ligand 2 (CCL-2), CCL-5, Vascular Endothelial Growth Factor (VEGF), MMP-2, MMP-9, p38 mitogen-activated protein kinase (MAPK), and NF-kB (62).
Evidence revealed that SGLT2i attenuates macrophage infiltration and inflammation, foam cell formation, and M1 polarization, crucial steps in the development of atherosclerosis. It was demonstrated that empagliflozin reduced macrophage infiltration and CD36 gene expression resulting in the loss of macrophage capability to scavenger ox-LDL and foam cell formation in db/db mice (63). A similar result was obtained in the streptozotocin (STZ)-induced diabetic model, where the macrophages proliferation and leukocyte adhesion were significantly decreased in empagliflozin and dapagliflozin -treated mice with a subsequent reduction in the plaque size (51, 64).
Moreover, dapagliflozin reduced macrophage infiltration and induced M2 polarization with a concomitant decrease in M1 through a mechanism depending on a RONS-dependent STAT3 pathway. These data demonstrated that dapagliflozin induced the production of anti-inflammatory factors participating in inflammation prevention and tissue repair (65).
Similar results were demonstrated in white rabbits, where dapagliflozin increased M2 macrophages and inhibited the expression of TLR4 and NF-κB (66).
However, the exact mechanism by which SGLT2i can induce M2 polarization and reduce macrophage infiltration is not fully elucidated, and many possible hypotheses are formulated. Indeed, reducing glucose levels, the principal energy source of macrophages, might have a role (35) (Figure 1; Table 1).
Clinical evidence
Several clinical studies have been conducted to evaluate the changes in the main inflammatory and oxidative stress biomarkers to evaluate the potential role of SGLT2i in protecting against atherosclerosis. The inflammatory markers implicated and therefore assessed in the atherosclerosis process are CRP, IL-6, and TNF-α.
In a single-center, open-label, randomized, prospective study, the administration of empagliflozin (10 mg/day for 12 months) to 51 diabetic patients induced a significant reduction of blood hs-CRP levels associated compared with baseline and placebo (−74.4% vs. placebo and −55.6% vs. baseline) (67).
Similarly, the CANOSSA trial, a prospective and open-label study that enrolled 35 patients with diabetes mellitus and stable chronic heart, demonstrated that the canagliflozin administration (100 mg/day for 12 months) induced a significant decrease in hs-CRP after 3, 6, and 12 months compared with baseline (3 months: p = 0.002, 6 months: p = 0.001, 12 months: p = 0.007) (68).
A comparative efficacy study between canagliflozin and empagliflozin was also conducted on 32 diabetic patients to evaluate the effect on inflammatory cytokines. The results suggest that treatment with empagliflozin 10 mg/day for 6 months is more effective in reducing inflammatory cytokines IL-6 (p = 0.002 vs. p = 0.27) and TNF-alpha (p = 0.002 vs. p = 0.29), while canagliflozin was more effective in reducing HbA1c (69).
DEFENSE STUDY, a prospective, randomized, open-label, blinded-endpoint, parallel-group, evaluated dapagliflozin's effectiveness on vascular endothelial function and glycemic control in subjects affected by T2DM early-stage. Dapagliflozin, in association with metformin for 16 weeks, improved endothelial function and significantly decreased urine 8-OHdG/creatinine, a marker of oxidative stress, compared to the only metformin group (p < 0.001) (70).
Moreover, atherosclerotic plaques of diabetic patients treated with canagliflozin displayed increased SIRT 6 expression and lower oxidative stress and inflammation markers (71) (Table 2).
Effects of SGLT2i on atherosclerosis cardiovascular diseases and cardiovascular mortality
Atherosclerotic cardiovascular diseases (ASCVD) represent a very important cause of death and disability, especially in subjects affected by T2DM. The main manifestations of ASCVD are coronary heart disease, ischemic stroke, peripheral artery disease, and heart failure (73).
Coronary artery disease
Coronary artery disease (CAD) is the most common atherosclerotic vascular disease, and it includes two main clinical phenotypes: stable/unstable angina and acute MI (AMI). Data regarding MI in patients treated with SGLT2i are conflicting. In the EMPA-REG OUTCOME study and CANVAS study, no significant difference in MI incidence was observed between the treated and placebo group (RR 0.87; 95% CI 0.70–1.09 and RR 0.85; 95% CI 0.69–1.05, respectively) (74, 75). However, the sub-analysis of the CVD-REAL study and the CVD-REAL2 study showed a lower risk of MI associated with SGLT2i therapy (RR 0.85; 95% CI 0.72–1.00) (RR 0.81; 95% CI 0.74–0.88) (76, 77). A meta-analysis, including 40 trials and ~60,000 participants, found a 14% reduction in the incidence of MI in SGLT2i users compared to controls (78). Nevertheless, no reduction was found in the occurrence of angina pectoris (79).
The effects of early SGLT2i treatment in patients with recent AMI were not well-studied.
Early SGLT2i treatment might improve cardiovascular outcomes through its beneficial effects on endothelial function, neurohormonal activation, cardiomyocyte necrosis, and reperfusion injury (80). Three studies are evaluating the efficacy and safety of SGLT2i in patients with AMI (80):
- EMMY (Impact of EMpagliflozin on Cardiac Function and Biomarkers of Heart Failure in Patients With Acute Myocardial Infarction) trial (NCT03087773)
- DAPA-MI (Dapagliflozin Effects on Cardiovascular Events in Patients With an Acute Heart Attack) (NCT04564742)
- EMPACT-MI (A Streamlined, Multicenter, Randomized, Parallel Group, Double-blind Placebo-controlled Superiority Trial to Evaluate the Effect of EMPAgliflozin on Hospitalization for Heart Failure and Mortality in Patients With Acute Myocardial Infarction) trial (NCT04509674).
Heart failure
The recent European clinical guidelines on HF have approved SGLT2 inhibitors for treating HFrEF and preventing HF hospitalizations independently of diabetes (37, 72, 81–83). In particular, EMPA-REG OUTCOME and the CANVAS study showed a significant reduction in hospitalizations for HF in patients treated with empagliflozin and canagliflozin, respectively, vs. placebo (RR 0.65; 95% CI 0.50–0.85) (RR 0.67; 95% CI 0.52–0.87) (75, 84). DECLARE-TIMI 58 showed a reduced hospitalization rate for HF compared to the placebo group (RR 0.73; 95% CI 0.61–0.88) (85).
However, in the VERTIS-CV study, the reduction of HF hospitalization was not statistically significant because the endpoint was a composite of cardiovascular death and hospitalization for HF (86).
Other studies, such as DAPA-HF and EMPEROR-Reduced, also provide convincing evidence of the beneficial effects of SGLT2i on HF (72, 87). A meta-analysis of these studies confirmed the important role of empagliflozin and dapagliflozin for reducing all-cause and cardiovascular mortality and HF hospitalizations and slowing the progression of renal disease (88). A meta-analysis, including 17 studies with ~51,348 participants, suggests a 32% reduction of HF risk (RR, 0.68; 95%CI, 0.63–0.73) in SGLT2-i users (78).
Stroke
Data regarding the risk of stroke in patients treated with SGLT2i are conflicting. The EMPAREG OUTCOME study did not show a significant reduction in the incidence of stroke in patients treated with empagliflozin compared to the placebo group (RR 1.18; 95% CI 0.89–1.56) (89). The same result was found in CANVAS and DECLARE-TIMI 58 studies (RR 0.9; 95% CI 0.71–1.15 and RR 1.01; 95% CI 0.84–1.21, respectively) (75, 85).
However, the CVD-REAL and the CVD-REAL2 studies showed a lower risk of stroke associated with SGLT2i therapy (RR 0.83; 95% CI 0.71–0.97) (RR 0.68; 95% CI 0.55–0.84) (76, 77).
However, the analysis conducted by these studies did not consider the SGLT2i effect on different types of stroke (fatal, non-fatal, ischemic, hemorrhagic, and TIA), making the data conflicting.
Therefore, a systematic review and meta-analysis were performed to evaluate the stroke subtypes. Considering five studies, CANAVAS, CREDENCE, DECLARE-TIMI, EMPAREG AND VERTIS- CV, they found that SGLT2i reduced the incidence of hemorrhagic stroke and Atrial Fibrillation (AF) by 0.5%. This could be related to the natriuretic effect and subsequent reduction in blood pressure. However, Further studies aimed at evaluating the impact of SGLT2 on this pathology will be needed (90).
Peripheral artery disease
Peripheral artery disease (PAD) is a manifestation of systemic atherosclerosis, characterized by progressive narrowing to occlusion of arterial vessels in the limbs, resulting in ulceration and subsequent gangrene and amputation.
Peripheral artery disease increases the risk of coronary artery and cerebrovascular disease and is an independent predictor of CVD death (91). The EMPAREG study, although it did not consider PAD as a primary and secondary endpoint, revealed that Empaglifozin resulted in significant benefits in subjects with PAD, which no reported amputations.
However, the CANAVAS and CANAVAS-R studies reported that amputation risk was twice in the treated group compared to the placebo group (6.3 cases per 1,000 patients per year) (75). Although the molecular mechanisms responsible for this have not yet been elucidated, the EMA has reported the potential increased risk of lower limb amputation (affecting mainly the toes) in patients taking SGLT2 inhibitors.
Major adverse cardiac events
The most important cardiovascular trials for SGLT2 evaluated the incidence of major adverse cardiac events (MACEs), such as cardiovascular death, non-fatal myocardial infarction, and non-fatal stroke. A meta-analysis of 6 placebo-controlled clinical outcomes trials (EMPA-REG OUTCOME, CANVAS, DECLARE-TIMI 58, CREDENCE, VERTIS CV) (85, 86, 92, 93), including a total of 46 969 patients (66.2% with prevalent ASCVD) suggests that SGLT2 inhibitors significantly reduced the risk of MACE (HR, 0.90; 95% CI, 0.85–0.95;); the presence or absence of ASCVD did not modify the treatment outcome on MACE (HR, 0.89; 95% CI, 0.83–1.07) (94).
Cardiovascular mortality
Four large cardiovascular outcome studies have recently been completed: EMPAREG OUTCOME, CANVAS, DECLARE-TIMI, and VERTIS CV (85, 86, 92, 93). These studies showed a reduction in overall and cardiovascular mortality (CV Mortality), which was significant in the EMPA-REG and CANVAS studies but not in DECLARE-TIMI 58. Specifically, the CV mortality rate was lower in the EMPA-REG OUTCOME study. in patients treated with SGLT2i compared to placebo (RR 0.62; 95% CI 0.49–0.77) (89). CV mortality rate was not significantly reduced in CANVAS, DECLARETIMI 58 and VERTIS-CV (RR 0.87; 95% CI 0.72–1.06 and RR 0.98; 95% CI 0.82–1.17, HR 0.89 95% CI 0.73–1.05, respectively) (75, 95). A recent meta-analysis showed no significant effect on CV mortality associated with SGLT2i treatment (except Empagliflozin) compared to placebo (OR 0.87; 95% CI 0.63–1.21) (96) (Table 3).
From clinical impact to future prospective
Sodium-glucose co-transport 2 inhibitors exert anti-atherosclerotic properties attenuating inflammatory factors, alleviating inflammation, mitigating insulin resistance, and reducing stress on vessels, inhibiting atherosclerosis development and progression (46). Recent clinical trials have analyzed the landmark cardiovascular outcomes, showing that SGLT2i may reduce MI, heart failure and HF hospitalization, MACE, and cardiovascular death in subjects affected by T2DM (81, 86–89). Nevertheless, data regarding MI, stroke, and PAD are conflicting, probably because the analyzed population is heterogeneous, the different stroke subtypes were not considered, different molecules were analyzed, and the follow-up time is short. Therefore, observational studies of high quality, with an adequate number of events and follow-up times, should be conducted to examine the potential role of SGLT2i in subclinical atherosclerosis and ASCVD events in subjects affected and not by T2DM. Despite conflicting data, SGLT2i represents a promising drug class; important innovation is reported in European guidelines on diagnosing and treating heart failure. SGLT2i is used as a class I recommendation in treating HFrEF (72).
Moreover, cardiovascular safety studies conducted with SGLT2i suggested that subjects affected by T2DM could benefit from SGLT2i treatments with positive effects on cardiovascular events (97). Unfortunately, only a small number of subjects affected by T2DM are treated with SGLT2i, whereas their widespread use would reduce deaths and hospitalizations each year. We think using these drugs will lead to a global evaluation of patients regarding glycemic control and cardiac function. Moreover, its use will have a substantial cost reduction and hospitalization advantage.
Conclusions
Atherosclerosis is a chronic inflammatory disorder representing the major potential risk factor of CVDs. The progress in managing the atherosclerosis complications has extended life, but many individuals still present impaired cardiac function, straining healthcare systems and resources (7). In this review, we emphasize SGLT2i by providing updated information about its implication in atherosclerosis and ASCVD. SGLT2i exerts anti-atherosclerotic properties attenuating inflammatory factors and reducing MI, heart failure MACE in subjects affected by T2DM.
Therefore, in conclusion, SGLT2 inhibitors can represent up-and-coming therapeutic drugs with pleiotropic effects in terms of metabolic control and reduction of associated cardiovascular complications.
Author contributions
LSc, VC, and MB: concept and design. LSc, VC, FT, RF, APe, PG, MF, APu, AD, LSp, RM, and MB: drafting of the manuscript. All authors have read and agreed to the published version of the manuscript.
Funding
This study was funded by PON Ricerca e Innovazione 2014–2020 ARS01_01270.
Conflict of interest
The authors declare that the research was conducted in the absence of any commercial or financial relationships that could be construed as a potential conflict of interest.
Publisher's note
All claims expressed in this article are solely those of the authors and do not necessarily represent those of their affiliated organizations, or those of the publisher, the editors and the reviewers. Any product that may be evaluated in this article, or claim that may be made by its manufacturer, is not guaranteed or endorsed by the publisher.
Abbreviations
8-OHdG, 8-hydroxy-2'-deoxyguanosine; AMI, acute MI; AMPK, 5’ adenosine monophosphate-activated protein kinase; ASCVD, atherosclerotic cardiovascular diseases; BHB, β-hydroxybutyrate; CAD, coronary artery desease; CCL, chemokine (C-C motif) ligand 2; CCR2, C-C chemokine receptor type 2; CD68, cluster of differentiation 68; CRP, C-reactive protein; CVD, cardiovascular disease; CXCL, chemokines ligand; DAMPs, damage-associated molecular patterns; DPP-IV, dipeptidyl peptidase-4; eGFR, glomerular filtration rate; EMA, European Medicines Agency; FDA, Food and Drug Administration; FGF21, fibroblast growth factor 21; FOXO 3, forkhead box; HF, heart failure; HFD, high-fat diet; HFrEF, heart failure with reduced ejection fraction; ICAM-1, intercellular adhesion molecule 1; IFN, interferons; IKK, IkappaB kinase; IL-1R1, interleukin-1 Receptor 1; ILs, interleukins; JAK2, Janus kinase; JNK, c-Jun N-terminal kinases; LDL, low density lipoprotein; LPS, lipopolysaccharide; MACE, major adverse cardiac event; MAPK, mitogen-activated protein kinase; MCP-1, monocyte chemoattractant protein 1; MI, myocardial infarction; MKK, protein kinase kinase; MMPs, metalloproteases; mTORC2, mTOR complex 2; NA/STZ, nicotinamide and streptozotocin; NF-kB, nuclear factor kappa-light-chain-enhancer of activated B cells; NLRP3, nucleotide binding domain-like receptor protein 3; NO, nitric oxide; NOS 2, nitric oxidase synthase 2; ox-LDL, oxidized-LDL; PAD, peripheral artery disease; PAI-1, plasminogen activator inhibitor type 1; PECAM-1, platelet endothelial cell adhesion molecule-1; ROS, reactive oxygen species; SASP, senescence-associated secretory phenotype; SGLT2i, sodium-glucose co-transport 2 inhibitors; SIRT, sirtuin; STAT, signal transducer and activator of transcription; STZ, streptozotocin; T2DM, type 2 diabetes mellitus; TBARS, thiobarbituric acid reactive substances; TGF-ß, transforming growth factor beta; TIMPs, metallopeptidase inhibitor 1; TLR, toll-like receptors; TNF-α, tumor necrosis factor- α; VCAM-1, vascular cell adhesion molecule 1; VEGF, vascular endothelial growth factor.
References
1. Jebari-Benslaiman S, Galicia-Garcia U, Larrea-Sebal A, Olaetxea JR, Alloza I, Vandenbroeck K, et al. Pathophysiology of atherosclerosis. Int J Mol Sci. (2022) 23:3346. doi: 10.3390/ijms23063346
2. Emini Veseli B, Perrotta P, De Meyer GRA, Roth L, Van der Donckt C, Martinet W, et al. Animal models of atherosclerosis. Eur J Pharmacol. (2017) 816:3–13. doi: 10.1016/j.ejphar.2017.05.010
3. Poznyak A, Grechko AV, Poggio P, Myasoedova VA, Alfieri V, Orekhov AN. The diabetes mellitus-atherosclerosis connection: the role of lipid and glucose metabolism and chronic inflammation. Int J Mol Sci. (2020) 21:1835. doi: 10.3390/ijms21051835
4. Crowther MA. Pathogenesis of atherosclerosis. Hematology Am Soc Hematol Educ Program. (2005) 436–41. doi: 10.1182/asheducation-2005.1.436
5. Roth GA, Mensah GA, Johnson CO, Addolorato G, Ammirati E, Baddour LM, et al. Global burden of cardiovascular diseases and risk factors, 1990–2019: update from the GBD 2019 study. J Am Coll Cardiol. (2020) 76:2982–3021. doi: 10.1016/j.jacc.2020.11.010
6. Timmis A, Townsend N, Gale CP, Torbica A, Lettino M, Petersen SE, et al. European society of cardiology: cardiovascular disease statistics 2019. Eur Heart J. (2020) 41:12–85. doi: 10.1093/eurheartj/ehz859
7. Libby P, Buring JE, Badimon L, Hansson GK, Deanfield J, Bittencourt MS, et al. Atherosclerosis. Nat Rev Dis Primers. (2019) 5:56. doi: 10.1038/s41572-019-0106-z
8. Boren J, Chapman MJ, Krauss RM, Packard CJ, Bentzon JF, Binder CJ, et al. Low-density lipoproteins cause atherosclerotic cardiovascular disease: pathophysiological, genetic, and therapeutic insights: a consensus statement from the European atherosclerosis society consensus panel. Eur Heart J. (2020) 41:2313–30. doi: 10.1093/eurheartj/ehz962
9. Ma CX, Ma XN, Guan CH, Li YD, Mauricio D, Fu SB. Cardiovascular disease in type 2 diabetes mellitus: progress toward personalized management. Cardiovasc Diabetol. (2022) 21:74. doi: 10.1186/s12933-022-01516-6
10. Liu Z, Ma X, Ilyas I, Zheng X, Luo S, Little PJ, et al. Impact of sodium glucose co-transporter 2 (SGLT2) inhibitors on atherosclerosis: from pharmacology to pre-clinical and clinical therapeutics. Theranostics. (2021) 11:4502–15. doi: 10.7150/thno.54498
11. Navab M, Ananthramaiah GM, Reddy ST, Van Lenten BJ, Ansell BJ, Fonarow GC, et al. The oxidation hypothesis of atherogenesis: the role of oxidized phospholipids and HDL. J Lipid Res. (2004) 45:993–1007. doi: 10.1194/jlr.R400001-JLR200
12. Lundberg AM, Hansson GK. Innate immune signals in atherosclerosis. Clin Immunol. (2010) 134:5–24. doi: 10.1016/j.clim.2009.07.016
13. Mosser DM, Edwards JP. Exploring the full spectrum of macrophage activation. Nat Rev Immunol. (2008) 8:958–69. doi: 10.1038/nri2448
14. Rocha VZ, Libby P. Obesity, inflammation, and atherosclerosis. Nat Rev Cardiol. (2009) 6:399–409. doi: 10.1038/nrcardio.2009.55
15. De Paoli F, Staels B, Chinetti-Gbaguidi G. Macrophage phenotypes and their modulation in atherosclerosis. Circ J. (2014) 78:1775–81. doi: 10.1253/circj.CJ-14-0621
16. Barrett TJ. Macrophages in atherosclerosis regression. Arterioscler Thromb Vasc Biol. (2020) 40:20–33. doi: 10.1161/ATVBAHA.119.312802
17. Forstermann U. Oxidative stress in vascular disease: causes, defense mechanisms and potential therapies. Nat Clin Pract Cardiovasc Med. (2008) 5:338–49. doi: 10.1038/ncpcardio1211
18. Dinarello CA. Immunological and inflammatory functions of the interleukin-1 family. Annu Rev Immunol. (2009) 27:519–50. doi: 10.1146/annurev.immunol.021908.132612
19. Marchio P, Guerra-Ojeda S, Vila JM, Aldasoro M, Victor VM, Mauricio MD. Targeting early atherosclerosis: a focus on oxidative stress and inflammation. Oxid Med Cell Longev. (2019) 2019:8563845. doi: 10.1155/2019/8563845
20. Munoz-Espin D, Serrano M. Cellular senescence: from physiology to pathology. Nat Rev Mol Cell Biol. (2014) 15:482–96. doi: 10.1038/nrm3823
21. Coppe JP, Desprez PY, Krtolica A, Campisi J. The senescence-associated secretory phenotype: the dark side of tumor suppression. Annu Rev Pathol. (2010) 5:99–118. doi: 10.1146/annurev-pathol-121808-102144
22. Minamino T, Komuro I. Vascular cell senescence: contribution to atherosclerosis. Circ Res. (2007) 100:15–26. doi: 10.1161/01.RES.0000256837.40544.4a
23. Bennett MR, Sinha S, Owens GK. Vascular smooth muscle cells in atherosclerosis. Circ Res. (2016) 118:692–702. doi: 10.1161/CIRCRESAHA.115.306361
24. Schlommer C, Brandtner A, Bachler M. Antithrombin and its role in host defense and inflammation. Int J Mol Sci. (2021) 22:4283. doi: 10.3390/ijms22084283
25. Alsaffar H, Martino N, Garrett JP, Adam AP. Interleukin-6 promotes a sustained loss of endothelial barrier function via Janus kinase-mediated STAT3 phosphorylation and de novo protein synthesis. Am J Physiol Cell Physiol. (2018) 314:C589–602. doi: 10.1152/ajpcell.00235.2017
26. Tomaniak M, Katagiri Y, Modolo R, de Silva R, Khamis RY, Bourantas CV, et al. Vulnerable plaques and patients: state-of-the-art. Eur Heart J. (2020) 41:2997–3004. doi: 10.1093/eurheartj/ehaa227
27. Andreadou I, Bell RM, Botker HE, Zuurbier CJ. SGLT2 inhibitors reduce infarct size in reperfused ischemic heart and improve cardiac function during ischemic episodes in pre-clinical models. Biochim Biophys Acta Mol Basis Dis. (2020) 1866:165770. doi: 10.1016/j.bbadis.2020.165770
28. Shaffner J, Chen B, Malhotra DK, Dworkin LD, Gong R. Therapeutic targeting of SGLT2: a new era in the treatment of diabetes and diabetic kidney disease. Front Endocrinol. (2021) 12:749010. doi: 10.3389/fendo.2021.749010
29. Wilcox CS. Antihypertensive and renal mechanisms of SGLT2 (Sodium-Glucose Linked Transporter 2) inhibitors. Hypertension. (2020) 75:894–901. doi: 10.1161/HYPERTENSIONAHA.119.11684
30. Vallon V, Thomson SC. Targeting renal glucose reabsorption to treat hyperglycaemia: the pleiotropic effects of SGLT2 inhibition. Diabetologia. (2017) 60:215–25. doi: 10.1007/s00125-016-4157-3
31. Wright EM. SGLT2 inhibitors: physiology and pharmacology. Kidney360. (2021) 2:2027–37. doi: 10.34067/KID.0002772021
32. Kshirsagar RP, Kulkarni AA, Chouthe RS, Pathan SK, Une HD, Reddy GB, et al. SGLT inhibitors as anti-diabetic agents: a comprehensive review. RSC Adv. (2020) 10:1733–56. doi: 10.1039/C9RA08706K
33. Cowie MR, Fisher M. SGLT2 inhibitors: mechanisms of cardiovascular benefit beyond glycaemic control. Nat Rev Cardiol. (2020) 17:761–72. doi: 10.1038/s41569-020-0406-8
34. Cinti F, Moffa S, Impronta F, Cefalo CM, Sun VA, Sorice GP, et al. Spotlight on ertugliflozin and its potential in the treatment of type 2 diabetes: evidence to date. Drug Des Devel Ther. (2017) 11:2905–19. doi: 10.2147/DDDT.S114932
35. Bonora BM, Avogaro A, Fadini GP. Extraglycemic effects of SGLT2 inhibitors: a review of the evidence. Diabetes Metab Syndr Obes. (2020) 13:161–74. doi: 10.2147/DMSO.S233538
36. Provenzano M, Pelle MC, Zaffina I, Tassone B, Pujia R, Ricchio M, et al. Sodium-glucose co-transporter-2 inhibitors and nephroprotection in diabetic patients: more than a challenge. Front Med. (2021) 8:654557. doi: 10.3389/fmed.2021.654557
37. McDonagh TA, Metra M, Adamo M, Gardner RS, Baumbach A, Bohm M, et al. 2021 ESC guidelines for the diagnosis and treatment of acute and chronic heart failure. Eur Heart J. (2021) 42:3599–726. doi: 10.1093/eurheartj/ehab368
38. Lopaschuk GD, Verma S. Mechanisms of cardiovascular benefits of sodium glucose co-transporter 2 (SGLT2) inhibitors: a state-of-the-art review. JACC Basic Transl Sci. (2020) 5:632–44. doi: 10.1016/j.jacbts.2020.02.004
39. Tamargo J. Sodium-glucose co-transporter 2 inhibitors in heart failure: potential mechanisms of action, adverse effects and future developments. Eur Cardiol. (2019) 14:23–32. doi: 10.15420/ecr.2018.34.2
40. Paolisso P, Bergamaschi L, Santulli G, Gallinoro E, Cesaro A, Gragnano F, et al. Infarct size, inflammatory burden, and admission hyperglycemia in diabetic patients with acute myocardial infarction treated with SGLT2-inhibitors: a multicenter international registry. Cardiovasc Diabetol. (2022) 21:77. doi: 10.1186/s12933-022-01506-8
41. Sardu C, Barbieri M, Santamaria M, Giordano V, Sacra C, Paolisso P, et al. Multipolar pacing by cardiac resynchronization therapy with a defibrillators treatment in type 2 diabetes mellitus failing heart patients: impact on responders rate, and clinical outcomes. Cardiovasc Diabetol. (2017) 16:75. doi: 10.1186/s12933-017-0554-2
42. Hodrea J, Saeed A, Molnar A, Fintha A, Barczi A, Wagner LJ, et al. SGLT2 inhibitor dapagliflozin prevents atherosclerotic and cardiac complications in experimental type 1 diabetes. PLoS ONE. (2022) 17:e0263285. doi: 10.1371/journal.pone.0263285
43. Salvatore T, Galiero R, Caturano A, Rinaldi L, Di Martino A, Albanese G, et al. An overview of the cardiorenal protective mechanisms of SGLT2 inhibitors. Int J Mol Sci. (2022) 23:3651. doi: 10.3390/ijms23073651
44. Tsai KF, Chen YL, Chiou TT, Chu TH, Li LC, Ng HY, et al. Emergence of SGLT2 inhibitors as powerful antioxidants in human diseases. Antioxidants. (2021) 10:1166. doi: 10.3390/antiox10081166
45. Nedosugova LV, Markina YV, Bochkareva LA, Kuzina IA, Petunina NA, Yudina IY, et al. Inflammatory mechanisms of diabetes and its vascular complications. Biomedicines. (2022) 10:1168. doi: 10.3390/biomedicines10051168
46. Oguntibeju OO. Type 2 diabetes mellitus, oxidative stress and inflammation: examining the links. Int J Physiol Pathophysiol Pharmacol. (2019) 11:45–63.
47. Nakatsu Y, Kokubo H, Bumdelger B, Yoshizumi M, Yamamotoya T, Matsunaga Y, et al. The SGLT2 inhibitor luseogliflozin rapidly normalizes aortic mRNA levels of inflammation-related but not lipid-metabolism-related genes and suppresses atherosclerosis in diabetic ApoE KO mice. Int J Mol Sci. (2017) 18:1704. doi: 10.3390/ijms18081704
48. Dimitriadis GK, Nasiri-Ansari N, Agrogiannis G, Kostakis ID, Randeva MS, Nikiteas N, et al. Empagliflozin improves primary haemodynamic parameters and attenuates the development of atherosclerosis in high fat diet fed APOE knockout mice. Mol Cell Endocrinol. (2019) 494:110487. doi: 10.1016/j.mce.2019.110487
49. Leng W, Ouyang X, Lei X, Wu M, Chen L, Wu Q, et al. The SGLT-2 inhibitor dapagliflozin has a therapeutic effect on atherosclerosis in diabetic ApoE(-/-) mice. Mediators Inflamm. (2016) 2016:6305735. doi: 10.1155/2016/6305735
50. Lee DM, Battson ML, Jarrell DK, Hou S, Ecton KE, Weir TL, et al. SGLT2 inhibition via dapagliflozin improves generalized vascular dysfunction and alters the gut microbiota in type 2 diabetic mice. Cardiovasc Diabetol. (2018) 17:62. doi: 10.1186/s12933-018-0708-x
51. Pennig J, Scherrer P, Gissler MC, Anto-Michel N, Hoppe N, Funer L, et al. Glucose lowering by SGLT2-inhibitor empagliflozin accelerates atherosclerosis regression in hyperglycemic STZ-diabetic mice. Sci Rep. (2019) 9:17937. doi: 10.1038/s41598-019-54224-9
52. Abdollahi E, Keyhanfar F, Delbandi AA, Falak R, Hajimiresmaiel SJ, Shafiei M. Dapagliflozin exerts anti-inflammatory effects via inhibition of LPS-induced TLR-4 overexpression and NF-kappaB activation in human endothelial cells and differentiated macrophages. Eur J Pharmacol. (2022) 918:174715. doi: 10.1016/j.ejphar.2021.174715
53. Lee N, Heo YJ, Choi SE, Jeon JY, Han SJ, Kim DJ, et al. Anti-inflammatory effects of empagliflozin and gemigliptin on LPS-stimulated macrophage via the IKK/NF-kappaB, MKK7/JNK, and JAK2/STAT1 signalling pathways. J Immunol Res. (2021) 2021:9944880. doi: 10.1155/2021/9944880
54. Karasawa T, Takahashi M. Role of NLRP3 inflammasomes in atherosclerosis. J Atheroscler Thromb. (2017) 24:443–51. doi: 10.5551/jat.RV17001
55. Kim SR, Lee SG, Kim SH, Kim JH, Choi E, Cho W, et al. SGLT2 inhibition modulates NLRP3 inflammasome activity via ketones and insulin in diabetes with cardiovascular disease. Nat Commun. (2020) 11:2127. doi: 10.1038/s41467-020-15983-6
56. Gronda E, Lopaschuk GD, Arduini A, Santoro A, Benincasa G, Palazzuoli A, et al. Mechanisms of action of SGLT2 inhibitors and their beneficial effects on the cardiorenal axis. Can J Physiol Pharmacol. (2022) 100:93–106. doi: 10.1139/cjpp-2021-0399
57. Ye Y, Bajaj M, Yang HC, Perez-Polo JR, Birnbaum Y. SGLT-2 inhibition with dapagliflozin reduces the activation of the Nlrp3/ASC inflammasome and attenuates the development of diabetic cardiomyopathy in mice with type 2 diabetes. Further augmentation of the effects with saxagliptin, a DPP4 inhibitor. Cardiovasc Drugs Ther. (2017) 31:119–32. doi: 10.1007/s10557-017-6725-2
58. Chen H, Tran D, Yang HC, Nylander S, Birnbaum Y, Ye Y. Dapagliflozin and ticagrelor have additive effects on the attenuation of the activation of the NLRP3 inflammasome and the progression of diabetic cardiomyopathy: an AMPK-mTOR interplay. Cardiovasc Drugs Ther. (2020) 34:443–61. doi: 10.1007/s10557-020-06978-y
59. Ganbaatar B, Fukuda D, Shinohara M, Yagi S, Kusunose K, Yamada H, et al. Empagliflozin ameliorates endothelial dysfunction and suppresses atherogenesis in diabetic apolipoprotein E-deficient mice. Eur J Pharmacol. (2020) 875:173040. doi: 10.1016/j.ejphar.2020.173040
60. Xu L, Nagata N, Nagashimada M, Zhuge F, Ni Y, Chen G, et al. SGLT2 inhibition by empagliflozin promotes fat utilization and browning and attenuates inflammation and insulin resistance by polarizing M2 macrophages in diet-induced obese mice. EBioMedicine. (2017) 20:137–49. doi: 10.1016/j.ebiom.2017.05.028
61. Tahara A, Kurosaki E, Yokono M, Yamajuku D, Kihara R, Hayashizaki Y, et al. Effects of SGLT2 selective inhibitor ipragliflozin on hyperglycemia, hyperlipidemia, hepatic steatosis, oxidative stress, inflammation, and obesity in type 2 diabetic mice. Eur J Pharmacol. (2013) 715:246–55. doi: 10.1016/j.ejphar.2013.05.014
62. Ortega R, Collado A, Selles F, Gonzalez-Navarro H, Sanz MJ, Real JT, et al. SGLT-2 (Sodium-Glucose Co-transporter 2) inhibition reduces Ang II (Angiotensin II)-induced dissecting abdominal aortic aneurysm in ApoE (Apolipoprotein E) knockout mice. Arterioscler Thromb Vasc Biol. (2019) 39:1614–28. doi: 10.1161/ATVBAHA.119.312659
63. Terasaki M, Hiromura M, Mori Y, Kohashi K, Nagashima M, Kushima H, et al. Amelioration of hyperglycemia with a sodium-glucose co-transporter 2 inhibitor prevents macrophage-driven atherosclerosis through macrophage foam cell formation suppression in type 1 and type 2 diabetic mice. PLoS ONE. (2015) 10:e0143396. doi: 10.1371/journal.pone.0143396
64. Lin B, Koibuchi N, Hasegawa Y, Sueta D, Toyama K, Uekawa K, et al. Glycemic control with empagliflozin, a novel selective SGLT2 inhibitor, ameliorates cardiovascular injury and cognitive dysfunction in obese and type 2 diabetic mice. Cardiovasc Diabetol. (2014) 13:148. doi: 10.1186/s12933-014-0148-1
65. Lee TM, Chang NC, Lin SZ. Dapagliflozin, a selective SGLT2 inhibitor, attenuated cardiac fibrosis by regulating the macrophage polarization via STAT3 signaling in infarcted rat hearts. Free Radic Biol Med. (2017) 104:298–310. doi: 10.1016/j.freeradbiomed.2017.01.035
66. Lee SG, Lee SJ, Lee JJ, Kim JS, Lee OH, Kim CK, et al. Anti-inflammatory effect for atherosclerosis progression by sodium-glucose co-transporter 2 (SGLT-2) inhibitor in a normoglycemic rabbit model. Korean Circ J. (2020) 50:443–57. doi: 10.4070/kcj.2019.0296
67. Hattori S. Anti-inflammatory effects of empagliflozin in patients with type 2 diabetes and insulin resistance. Diabetol Metab Syndr. (2018) 10:93. doi: 10.1186/s13098-018-0395-5
68. Real J, Vlacho B, Ortega E, Valles JA, Mata-Cases M, Castelblanco E, et al. Cardiovascular and mortality benefits of sodium-glucose co-transporter-2 inhibitors in patients with type 2 diabetes mellitus: CVD-real catalonia. Cardiovasc Diabetol. (2021) 20:139. doi: 10.1186/s12933-021-01323-5
69. Alshnbari A, Idris I. Can sodium-glucose co-transporter-2 (SGLT-2) inhibitor reduce the risk of adverse complications due to COVID-19? - Targeting hyperinflammation. Curr Med Res Opin. (2022) 38:357–64. doi: 10.1080/03007995.2022.2027141
70. Shigiyama F, Kumashiro N, Miyagi M, Ikehara K, Kanda E, Uchino H, et al. Effectiveness of dapagliflozin on vascular endothelial function and glycemic control in patients with early-stage type 2 diabetes mellitus: DEFENCE study. Cardiovasc Diabetol. (2017) 16:84. doi: 10.1186/s12933-017-0564-0
71. D'Onofrio N, Sardu C, Trotta MC, Scisciola L, Turriziani F, Ferraraccio F, et al. Sodium-glucose co-transporter2 expression and inflammatory activity in diabetic atherosclerotic plaques: effects of sodium-glucose co-transporter2 inhibitor treatment. Mol Metab. (2021) 54:101337. doi: 10.1016/j.molmet.2021.101337
72. McMurray JJV, Solomon SD, Inzucchi SE, Kober L, Kosiborod MN, Martinez FA, et al. Dapagliflozin in patients with heart failure and reduced ejection fraction. N Engl J Med. (2019) 381:1995–2008. doi: 10.1056/NEJMoa1911303
73. Beckman JA, Paneni F, Cosentino F, Creager MA. Diabetes and vascular disease: pathophysiology, clinical consequences, and medical therapy: part II. Eur Heart J. (2013) 34:2444–52. doi: 10.1093/eurheartj/eht142
74. Zinman B, Wanner C, Lachin JM, Fitchett D, Bluhmki E, Hantel S, et al. empagliflozin, cardiovascular outcomes, and mortality in type 2 diabetes. N Engl J Med. (2015) 373:2117–28. doi: 10.1056/NEJMoa1504720
75. Neal B, Perkovic V, Matthews DR, Mahaffey KW, Fulcher G, Meininger G, et al. Rationale, design and baseline characteristics of the CANagliflozin cardiovascular assessment study-renal (CANVAS-R): a randomized, placebo-controlled trial. Diabetes Obes Metab. (2017) 19:387–93. doi: 10.1111/dom.12829
76. Kosiborod M, Birkeland KI, Cavender MA, Fu AZ, Wilding JP, Khunti K, et al. Rates of myocardial infarction and stroke in patients initiating treatment with SGLT2-inhibitors versus other glucose-lowering agents in real-world clinical practice: results from the CVD-REAL study. Diabetes Obes Metab. (2018) 20:1983–7. doi: 10.1111/dom.13299
77. Kosiborod M, Lam CSP, Kohsaka S, Kim DJ, Karasik A, Shaw J, et al. Cardiovascular events associated with SGLT-2 inhibitors versus other glucose-lowering drugs: the CVD-REAL 2 study. J Am Coll Cardiol. (2018) 71:2628–39. doi: 10.1016/j.jacc.2018.03.009
78. Zhu J, Yu X, Zheng Y, Li J, Wang Y, Lin Y, et al. Association of glucose-lowering medications with cardiovascular outcomes: an umbrella review and evidence map. Lancet Diabetes Endocrinol. (2020) 8:192–205. doi: 10.1016/S2213-8587(19)30422-X
79. Pahud de Mortanges A, Salvador D Jr, Laimer M, Muka T, Wilhelm M, Bano A. The role of SGLT2 inhibitors in atherosclerosis: a narrative mini-review. Front Pharmacol. (2021) 12:751214. doi: 10.3389/fphar.2021.751214
80. Udell JA, Jones WS, Petrie MC, Harrington J, Anker SD, Bhatt DL, et al. Sodium glucose co-transporter-2 inhibition for acute myocardial infarction: JACC review topic of the week. J Am Coll Cardiol. (2022) 79:2058–68. doi: 10.1016/j.jacc.2022.03.353
81. Packer M, Anker SD, Butler J, Filippatos G, Pocock SJ, Carson P, et al. Cardiovascular and renal outcomes with empagliflozin in heart failure. N Engl J Med. (2020) 383:1413–24. doi: 10.1056/NEJMoa2022190
82. Gallinoro E, Paolisso P, Candreva A, Bermpeis K, Fabbricatore D, Esposito G, et al. Microvascular dysfunction in patients with type II diabetes mellitus: invasive assessment of absolute coronary blood flow and microvascular resistance reserve. Front Cardiovasc Med. (2021) 8:765071. doi: 10.3389/fcvm.2021.765071
83. Paolisso P, Foa A, Bergamaschi L, Angeli F, Fabrizio M, Donati F, et al. Impact of admission hyperglycemia on short and long-term prognosis in acute myocardial infarction: MINOCA versus MIOCA. Cardiovasc Diabetol. (2021) 20:192. doi: 10.1186/s12933-021-01384-6
84. Zhang XL, Zhu QQ, Chen YH, Li XL, Chen F, Huang JA, et al. Cardiovascular safety, long-term non-cardiovascular safety, and efficacy of sodium-glucose co-transporter 2 inhibitors in patients with type 2 diabetes mellitus: a systemic review and meta-analysis with trial sequential analysis. J Am Heart Assoc. (2018) 7:e007165. doi: 10.1161/JAHA.117.007165
85. Wiviott SD, Raz I, Bonaca MP, Mosenzon O, Kato ET, Cahn A, et al. Dapagliflozin and cardiovascular outcomes in type 2 diabetes. N Engl J Med. (2019) 380:347–57. doi: 10.1056/NEJMoa1812389
86. Cannon CP, Pratley R, Dagogo-Jack S, Mancuso J, Huyck S, Masiukiewicz U, et al. Cardiovascular outcomes with ertugliflozin in type 2 diabetes. N Engl J Med. (2020) 383:1425–35. doi: 10.1056/NEJMoa2004967
87. Packer M, Anker SD, Butler J, Filippatos G, Ferreira JP, Pocock SJ, et al. Empagliflozin in patients with heart failure, reduced ejection fraction, and volume overload: EMPEROR-reduced trial. J Am Coll Cardiol. (2021) 77:1381–92. doi: 10.1016/j.jacc.2021.01.033
88. Zannad F, Ferreira JP, Pocock SJ, Anker SD, Butler J, Filippatos G, et al. SGLT2 inhibitors in patients with heart failure with reduced ejection fraction: a meta-analysis of the EMPEROR-reduced and DAPA-HF trials. Lancet. (2020) 396:819–29. doi: 10.1016/S0140-6736(20)31824-9
89. Zinman B, Lachin JM, Inzucchi SE. Empagliflozin, cardiovascular outcomes, and mortality in type 2 diabetes. N Engl J Med. (2016) 374:1094. doi: 10.1056/NEJMc1600827
90. Tsai WH, Chuang SM, Liu SC, Lee CC, Chien MN, Leung CH, et al. Effects of SGLT2 inhibitors on stroke and its subtypes in patients with type 2 diabetes: a systematic review and meta-analysis. Sci Rep. (2021) 11:15364. doi: 10.1038/s41598-021-94945-4
91. Mohler ER 3rd. Peripheral arterial disease: identification and implications. Arch Intern Med. (2003) 163:2306–14. doi: 10.1001/archinte.163.19.2306
92. Scheen AJ. [EMPA-REG OUTCOME: empagliflozin reduces mortality in patients with type 2 diabetes at high cardiovascular risk]. Rev Med Liege. (2015) 70:583–9.
93. Neal B, Perkovic V, Mahaffey KW, de Zeeuw D, Fulcher G, Erondu N, et al. Canagliflozin and cardiovascular and renal events in type 2 diabetes. N Engl J Med. (2017) 377:644–57. doi: 10.1056/NEJMoa1611925
94. McGuire DK, Shih WJ, Cosentino F, Charbonnel B, Cherney DZI, Dagogo-Jack S, et al. Association of SGLT2 inhibitors with cardiovascular and kidney outcomes in patients with type 2 diabetes: a meta-analysis. JAMA Cardiol. (2021) 6:148–58. doi: 10.1001/jamacardio.2020.4511
95. Cosentino F, Cannon CP, Cherney DZI, Masiukiewicz U, Pratley R, Dagogo-Jack S, et al. Efficacy of ertugliflozin on heart failure-related events in patients with type 2 diabetes mellitus and established atherosclerotic cardiovascular disease: results of the VERTIS CV trial. Circulation. (2020) 142:2205–15. doi: 10.1161/CIRCULATIONAHA.120.050255
96. Sinha B, Ghosal S. Meta-analyses of the effects of DPP-4 inhibitors, SGLT2 inhibitors and GLP1 receptor analogues on cardiovascular death, myocardial infarction, stroke and hospitalization for heart failure. Diabetes Res Clin Pract. (2019) 150:8–16. doi: 10.1016/j.diabres.2019.02.014
Keywords: SGLT2 inhibitors (SGLT2i), SGLT2, atherosclerosis, atherosclerosis cardiovascular diseases, inflammation
Citation: Scisciola L, Cataldo V, Taktaz F, Fontanella RA, Pesapane A, Ghosh P, Franzese M, Puocci A, De Angelis A, Sportiello L, Marfella R and Barbieri M (2022) Anti-inflammatory role of SGLT2 inhibitors as part of their anti-atherosclerotic activity: Data from basic science and clinical trials. Front. Cardiovasc. Med. 9:1008922. doi: 10.3389/fcvm.2022.1008922
Received: 01 August 2022; Accepted: 16 August 2022;
Published: 06 September 2022.
Edited by:
Emilio Clementi, University of Milan, ItalyReviewed by:
Graziamaria Corbi, University of Naples Federico II, ItalyVirginia Boccardi, University of Perugia, Italy
Copyright © 2022 Scisciola, Cataldo, Taktaz, Fontanella, Pesapane, Ghosh, Franzese, Puocci, De Angelis, Sportiello, Marfella and Barbieri. This is an open-access article distributed under the terms of the Creative Commons Attribution License (CC BY). The use, distribution or reproduction in other forums is permitted, provided the original author(s) and the copyright owner(s) are credited and that the original publication in this journal is cited, in accordance with accepted academic practice. No use, distribution or reproduction is permitted which does not comply with these terms.
*Correspondence: Lucia Scisciola, bHVjaWEuc2Npc2Npb2xhJiN4MDAwNDA7dW5pY2FtcGFuaWEuaXQ=
†These authors share first authorship