- Department of Cardiology, Dushu Lake Hospital Affiliated to Soochow University, Suzhou, China
Introduction: Heart failure with preserved ejection fraction (HFpEF) is associated with disrupted intestinal epithelial function, resulting from intestinal congestion. Intestinal congestion changes the morphology and permeability of the intestinal wall, and it becomes easy for the gut microbiota to change and transfer. Intervention on gut microbiota may become a new target for HFpEF treatment. However, the characteristics of gut microbiota in patients with HFpEF remain unknown. This preliminary report aims to detect the structure of gut microbiota in HFpEF patients so as to explore their characteristic changes, thereby providing a theoretical basis for future research.
Methods: This research recruited 30 patients diagnosed with HFpEF and 30 healthy individuals. Stool specimens of research subjects were collected separately, and the microarray analyses of gut microbiota were conducted by Illumina high-throughput DNA sequencing. The differences in gut microbiota composition, alpha diversity, and beta diversity between the two groups were finally obtained.
Results: The composition of gut microbiota was significantly different between the two groups. At the phylum classification level, the abundance of Synergistetes tended to be higher in the HFpEF group (P = 0.012). At genus classification level, the abundance of Butyricicoccus (P < 0.001), Sutterella (P = 0.004), Lachnospira (P = 0.003), and Ruminiclostridium (P = 0.009) in the HFpEF group were lower, while the abundance of Enterococcus (P < 0.001) and Lactobacillus (P = 0.005) were higher. According to the Chao index of alpha diversity analysis, HFpEF patients showed a nominally significant lower species richness when compared with controls (P = 0.046). However, there was no statistical difference in the Shannon index (P = 0.159) and Simpson index (P = 0.495), indicating that there was no difference in species diversity between the two groups. Beta diversity analysis revealed a highly significant separation of HFpEF patients and controls.
Conclusions: An imbalance in the gut microbiota of HFpEF patients was observed. Patients with HFpEF have an increased abundance of microbiota associated with inflammation and a decreased abundance of microbiota associated with anti-inflammatory effects in the gut environment. In line with that, the species richness of gut microbiota in HFpEF patients tended to be lower.
Introduction
Heart failure with preserved ejection fraction (HFpEF) is a common cardiovascular disease with a poor prognosis (1). In spite of current medical treatment approaches, mortality of HFpEF remains high and novel treatment concepts are thus urgently required (2). A theory proposed recently showed a possible impact of gut microbiota on the incidence and progression of HFpEF (3).
The gut microbiota resides in the intestine of hosts and participates in various physiological processes of hosts. According to the classification method of biological research, gut microbiota can be classified into several types with different characteristics at seven levels, which are called a kingdom, phylum, class, order, family, genus, and species. At the phylum classification level, the gut microbiota in the human body can be divided into six main subtypes, including Bacteroidetes, Firmicutes, Proteobacteria, Actinobacteria, Fusobacteria, and Verrucomicrobia. Among them, Firmicutes and Bacteroidetes are the dominant microbiota in the healthy human body. The gut microbiota remains relatively stable. However, the changes in physiological functions can cause an imbalance of gut microbiota, which in turn can further cause a series of pathological changes in the human body. Dysbiosis of gut microbiota has been proposed to be related to several diseases including diabetes, hypertension, atherosclerosis, thrombotic events, and infectious diseases (such as tuberculosis) (4–6). For example, Luo et al. found that specific gut microbiota may be associated with the host's immune status and affect the prognosis and outcome of tuberculosis patients (7).
The gut hypothesis of heart failure (HF) is a hot spot in the field of cardiovascular research. It has been proposed that HF is associated with disrupted intestinal epithelial function, likely as a consequence of reduced intestinal perfusion. HF patients are also accompanied by intestinal congestion. Intestinal ischemia and congestion change the morphology and permeability of the intestinal wall, and the gut microbiota is prone to change and shift. The imbalance and displacement of gut microbiota further induce or aggravate systemic inflammation, which affects the progression of HF (8–12). The increase in intestinal wall permeability also makes it easier for endotoxin (such as lipopolysaccharide, LPS) to translocate to the systemic circulation. LPS levels in the blood directly correlate with systemic inflammation in HF patients (13). In addition, researchers found that there was a significant imbalance of protective metabolites and harmful metabolites in HF patients (9, 14).
Heart failure with preserved ejection fraction is a kind of heart failure characterized by diastolic dysfunction. HFpEF is associated with disrupted intestinal epithelial function, likely as a consequence of intestinal congestion, which also changes the morphology and permeability of the intestinal wall, and the gut microbiota is prone to change and shift (15–19).
However, recently published research investigating this topic is all aimed at patients diagnosed with heart failure with reduced ejection fraction (HFrEF). Studies on the gut microbiota in HFrEF patients have identified a reduction in gut microbial diversity (10). Whether the gut microbiota of HFpEF patients is different and how it contributes to the development of HFpEF are still unknown. This study aims to detect the structure of gut microbiota in HFpEF patients through high-throughput sequencing and to explore the characteristic changes of gut microbiota in HFpEF patients. We hope that the results from our research will be clinically beneficial for the treatment of HFpEF.
Materials and Methods
Study Population
This study has been approved by the Ethics Review Board of the First Affiliated Hospital of Soochow University and Dushu Lake Hospital Affiliated to Soochow University and is a preliminary report of study registered in the Chinese Clinical Trial Registry (ChiCTR2100050121). Research subjects were assessed for eligibility and written informed consent.
This research recruited 30 patients diagnosed with HFpEF between August 2020 and October 2021 and 30 people who underwent physical examination in the same hospital. HFpEF patients were approached during their regular clinic visits.
The inclusion criteria of the HFpEF group required compliance with the HFpEF diagnostic criteria in the “2021 ESC Guidelines for the Heart Failure” (20). The inclusion criteria of the control group included people with no cardiovascular diseases such as high blood pressure, coronary heart disease, cardiomyopathy, pericardial disease, and valvular heart disease. At the same time, according to the diagnostic criteria of heart failure, heart failure should be excluded based on clinical assessment and NT-proBNP serum levels. The exclusion criteria for research subjects were as follows: (1) patients with acute heart failure, acute pericarditis, acute myocarditis, and congenital heart disease; (2) patients who take antibiotics orally or intravenously within three months; (3) patients using antibiotics on the skin within one month; (4) patients who ingest probiotics within three months; (5) patients with intestinal diseases (such as inflammatory bowel diseases) or patients with a history of bowel surgery and (6) patients with other non-cardiovascular diseases that have been known to change the gut microbiota.
Sample Collection and Data Recording
Research subjects emptied their stool in special stool collection containers. After the defecation was completed, research subjects scooped up 5 g deep stool and placed it in the professional stool DNA preservation solution. The stool samples were stored at −80°C until further processing.
Baseline descriptive data such as age, gender, body mass index (BMI), New York Heart Association (NYHA) classification, main diagnosis, and past medical history of the two groups were available at the inception of the study. Laboratory tests such as blood routine, liver function, kidney function, and blood lipids were carried out within 24 h. Echocardiographic data of HFpEF patients such as left ventricular ejection fraction (LVEF) were also recorded. Clinical characteristics are presented in Table 1.
Sample Detection
The DNA of gut microbiota was extracted by using the QIAamp DNA stool kit. We designed the target region and fusion primers according to the requirements of the sequencing platform and used a two-step method for PCR amplification. Variable regions V4–V5 of the 16S rRNA gene were amplified. The PCR amplification product obtained in the previous step was recovered by using 2% agarose gel. We used the AxyPrep DNA Gel Extraction Kit to purify the amplified PCR product. Finally, we used the Illumina sequencing platform to perform high-throughput sequencing on the samples that we collected.
Data Analysis
The differences in gut microbiota between the two groups were mainly presented in three aspects, which included the composition of gut microbiota, alpha diversity, and beta diversity. The composition of gut microbiota between the two groups was presented at the classification level of phylum and genus. Wilcoxon rank sum test was used to compare the abundance of each microbiota when the value distribution deviated from normality, while two samples t-test was used when there was no deviation. Rank-abundance distribution curves and VEEN picture based on an operational taxonomic unit (OTU) were also used to compare the composition of gut microbiota between the two groups. When testing the differences in alpha diversity, the Wilcoxon rank sum test was used to compare three indexes (Chao index, Shannon index, and Simpson index) between the two groups when the value distribution deviated from normality. The principal co-ordinates analysis (PCoA) chart based on Bray-Curtis was used to present the results of beta diversity analysis, and Anosim analysis was carried out further. We also performed a subgroup analysis of the HFpEF group according to their etiologies. Alpha diversity analysis and beta diversity analysis were calculated by using R.
Results
Composition of gut Microbiota in HFpEF Patients and Controls
This study mainly analyzed the composition of gut microbiota at the classification levels of phylum and genus and compared the differences in gut microbiota composition between the two groups.
Relative abundance refers to the proportion of one microbiota in the total microbiota (i.e., the sequences number of one microbiota divided by the total sequences number). The result indicated that the HFpEF group mainly included Bacteroides, Firmicutes, Proteobacteria, Actinobacteria, Fusobacteria, Tenerictes, Verrucomicrobia, Synergetes, Lentisphaerae, Cyanobacteria, Spirochaeta, and Unclassified microbiota. On the other hand, the control group consisted of Bacteroides, Firmicutes, Proteobacteria, Actinobacteria, Fusobacteria, Tenerictes, Verrucomicrobia, Synergistetes, Lentisphaerae, and Unclassified. The microbiota with the highest abundance in HFpEF patients and controls were Bacteroides, Firmicutes, and Proteobacteria. The microbiota with a statistically significant difference at the phylum classification level was Synergistetes (P = 0.012). In other words, the abundance of Synergistetes tended to be higher in the HFpEF group when compared with the control group (Figure 1A).
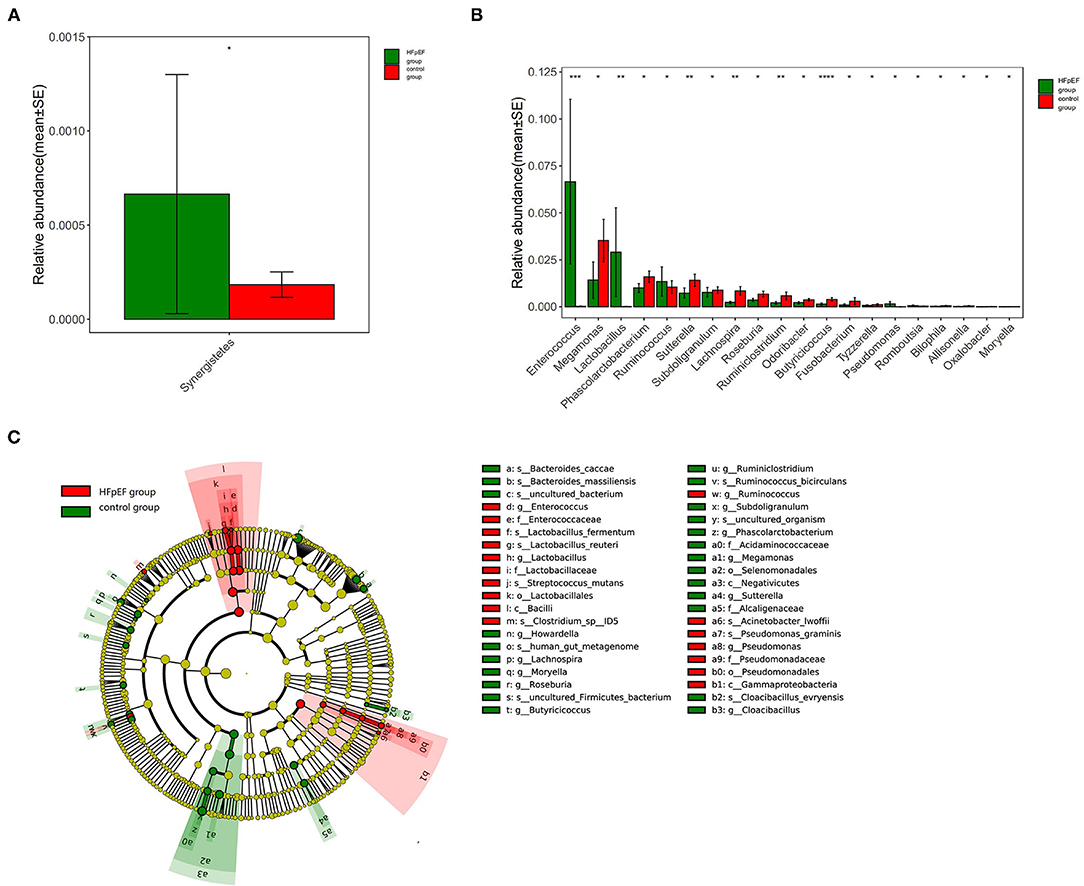
Figure 1. Differences in gut microbiota composition in patients with Heart failure with preserved ejection fraction (HFpEF) and controls. (A) Differences in gut microbiota composition at phylum classification level. (B) Differences in gut microbiota composition at genus classification level. (C) The results of linear discriminant analysis effect size (LEfSe) analysis. *0.01 < p < 0.05, **0.001 < p < 0.01, ***0.0001 < p < 0.001, ***p < 0.0001.
At the genus classification level, Bacteroides and Prevotella were two kinds of microbiota with the highest abundance. The abundance of Butyricicoccus (P < 0.001), Sutterella (P = 0.004), Lachnospira (P = 0.003), and Ruminiclostridium (P=0.009) in the HFpEF group were lower than that in the control group, while the abundance of Enterococcus (P < 0.001) and Lactobacillus (P = 0.005) were higher (Figure 1B).
The results of linear discriminant analysis effect size (LEfSe) analysis showed that the characteristic microbiota in the HFpEF group was Enterococcus, Lactobacillus, et al., while in the control group were Sutterella, Lachnospira, Ruminiclostridium, Butyricicoccus, et al. (Figure 1C).
Rank-Abundance Distribution Curves and VEEN Picture Based on OTUs
The detected sequences in Illumina high-throughput DNA sequencing can be classified according to a certain similarity. Operational taxonomic unit (OTU) refers to the sequences grouped by a certain similarity. We set 97% as the critical value, that is, if the sequence similarity ≥ 97%, it can be divided into the same OTU.
We drew Rank-abundance distribution curves and VEEN picture based on OTUs. The ordinate of Rank-abundance distribution curves represents the relative abundance of each OTU and the abscissa is the OTU arranged from high to low. The width of the curve reflects the species richness, while the shape of the curve can be used to explain the species evenness. As shown in Figure 2A, the species richness and evenness of the HFpEF group were lower than that of the control group. According to Figure 2B, the total number of OTUs in the HFpEF group was lower.
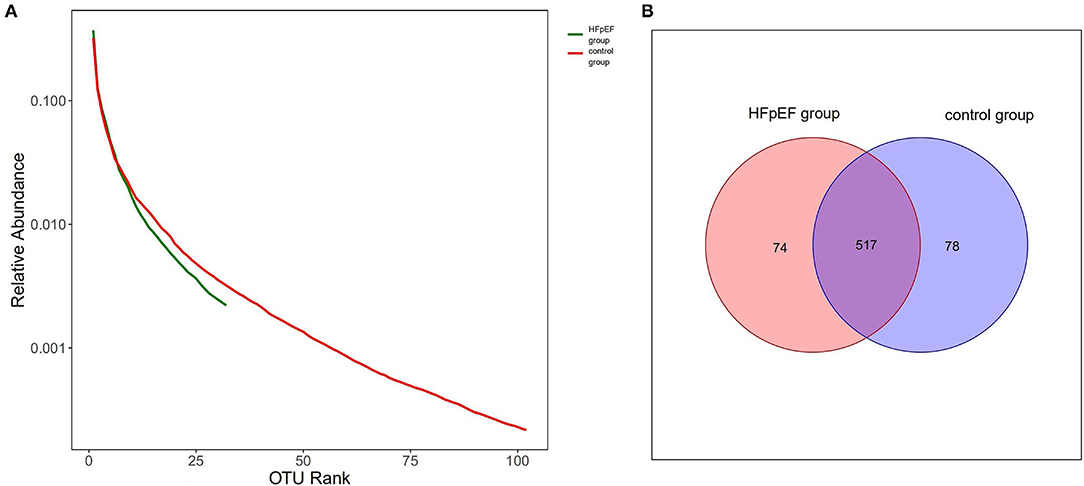
Figure 2. Rank-abundance distribution curves and VEEN picture based on operational taxonomic units (OTUs). (A) Rank-abundance distribution curves based on OTUs. (B) VEEN picture based on OTUs.
Differences Between HFpEF Patients and Controls With Regard to Alpha and Beta Diversity
With regard to alpha diversity, which reflected intra-individual variance, three indexes (Chao index, Shannon index, and Simpson index) were evaluated. There was a difference in the Chao index between the two groups (P = 0.046), which meant that the species richness of gut microbiota in the HFpEF group was lower than that in the control group. However, there was no statistical difference in the Shannon index (P = 0.159) and Simpson index (P = 0.495), indicating that there was no difference in the species diversity between the two groups (Figure 3).
Assessment of beta diversity, a parameter that represents inter-individual variances, showed a significant separation of HFpEF patients and controls (P < 0.05). The results provided evidence for a shift in the composition of gut microbiota induced by HFpEF. The PcoA chart based on Bray-Curtis was used to present the results of beta diversity analysis (Figure 4). Anosim analysis of this study showed that the differences between the groups were greater than the differences within the groups (R = 0.051, P = 0.028).
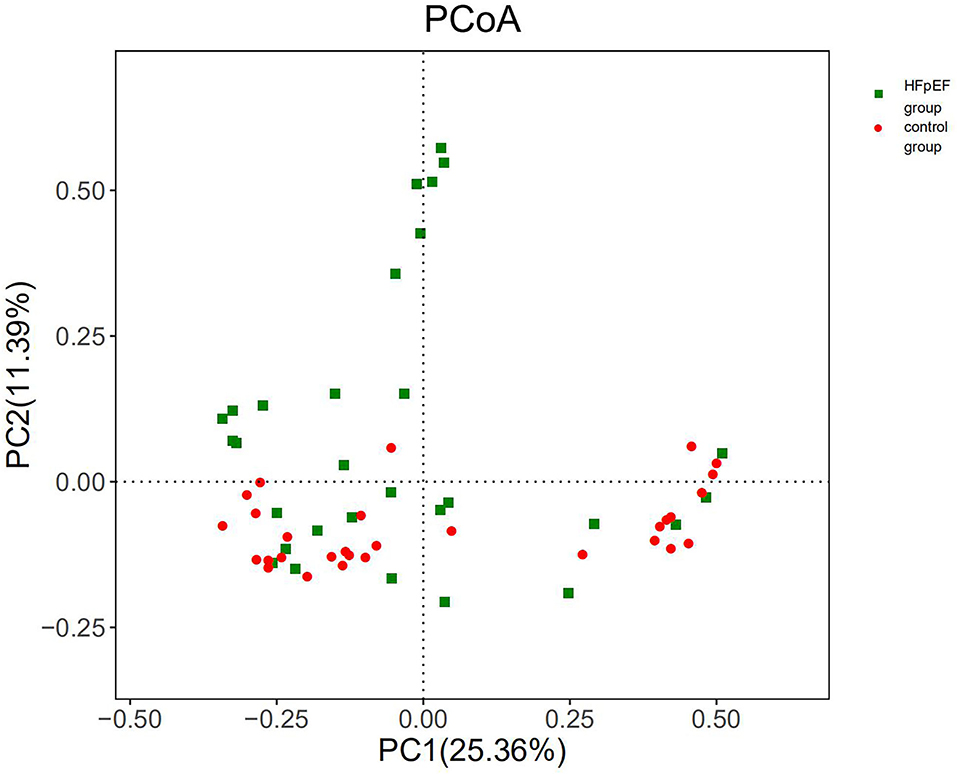
Figure 4. Beta diversity differed significantly in patients with HFpEF and controls. PC1, principal component 1; PC2, principal component 2.
Differences Between HFpEF Patients With Different Etiologies
We divided 30 HFpEF patients into three subgroups according to their etiologies: hypertensive heart disease group, coronary heart disease (CHD) group, and hypertrophic cardiomyopathy group. At the phylum classification level, there was no significant difference in the abundance of each microbiota. However, at the genus classification level, the abundance of 10 microbiota, such as Weissella (P = 0.008), Selenomonas (P = 0.006), and Dialister (P = 0.007), was statistically different between HFpEF patients, which was caused by different etiologies. In alpha diversity analysis, the Shannon index (P = 0.025) and Simpson index (P = 0.016) were statistically different, which indicated that the species diversity of gut microbiota in the CHD group was higher than that in the hypertensive heart disease group and hypertrophic cardiomyopathy group (P = 0.036, P = 0.011). To sum up, the composition and species diversity of gut microbiota was also different between HFpEF patients, which was caused by different etiologies.
Discussion
In this research, we determined the differences in gut microbiota between HFpEF patients and controls. At the phylum classification level, the abundance of Synergistetes in the HFpEF group tended to be higher. At the genus classification level, the abundance of Butyricicoccus, Sutterella, Lachnospira, and Ruminiclostridium in the HFpEF group was lower than that in the control group, while the abundance of Enterococcus and Lactobacillus was higher. Enterococcus belongs to a class of conditional pathogenic microbiota in the human gut. In vitro studies confirmed that Enterococcus can generate a kind of medium related to the inflammatory response, namely polymorphonuclear leukocyte chemotactic factor (PCF). In addition, clinical research demonstrated that Enterococcus is associated with endocarditis and urinary tract infections (21–23). Lactobacillus can decompose glucose and other sugars into lactic acid. An animal experiment found that Lactobacillus can aggravate the systemic inflammatory response in animals (24, 25). At the same time, patients with HFpEF had a depletion of microbiota known to be associated with anti-inflammatory effects. Butyricicoccus, Sutterella, Lachnospira, and Ruminiclostridium were all found to be related to anti-inflammatory effects in vitro studies or animal studies. For instance, Butyricicoccus can change the energy source of intestinal cells from glycolysis to fatty acid metabolism. A large amount of butyric acid will be produced in the process of fatty acid metabolism, which can downregulate the concentration of proinflammatory mediators and reduce inflammation in the human body (26–29). To sum up, the changes in gut microbiota in HFpEF patients included an increase in the abundance of microbiota associated with inflammation and a decrease in the abundance of microbiota associated with anti-inflammatory effects. This prolonged inflammatory state induced or aggravated by the gut microbiota imbalance may have a bad impact on the prognosis of patients with HFpEF. More research should focus on the mechanisms involving altered inflammatory pathways induced by gut microbiota imbalance. At the same time, maintaining the stability of gut microbiota in HFpEF patients through diet adjustment, antibiotic use, probiotic preparation use, fecal bacteria transplantation, and other methods is expected to become a new target of HFpEF treatment (30–32).
With regard to alpha diversity, our data pointed out that the species richness of gut microbiota in the HFpEF group was lower than that in the control group. Consistent with this, the results of Rank-abundance distribution curves based on OTU indicated that the species richness and species evenness of gut microbiota in the HFpEF group tended to be lower. Patients with HFpEF had a depletion of microbiota associated with anti-inflammatory effects, which was a driver for the reduction in gut microbial richness. Studies on the gut microbiota in HFrEF patients have also identified a reduction in gut microbial richness. The decrease in species richness had a bad impact on the prognosis of HFrEF patients (33–35). Therefore, we speculate that the reduction in species richness may also have an adverse effect on HFpEF patients. Beta diversity showed a separation of HFpEF patients and controls. Anosim analysis showed that the difference between the HFpEF group and the control group was greater than that within the group.
The general opinion favors the concept that gut microbial imbalance arises as a consequence of cardiac dysfunction. However, an impaired gut microbiota as a disease marker for the progress of HFpEF seems to be conceivable as well. Future studies could assess if alterations in disease status during the progression of HFpEF are mirrored by alterations in gut microbial composition (36–38).
This study provided valuable information on the characteristic changes of gut microbiota in HFpEF patients. Our data showed that an altered intestinal microbiome might be a potential player in the pathogenesis and progression of HFpEF. Moreover, we hope that the results from this research will be clinically beneficial for the specific treatment of HFpEF.
Conclusion
There was an imbalance of gut microbiota in HFpEF patients. Patients with HFpEF have an increased abundance of microbiota associated with inflammation and a decreased abundance of microbiota associated with anti-inflammatory effects in the gut environment. Moreover, the species richness of gut microbiota in HFpEF patients tended to be lower. We hope that the findings of this study will provide new directions for the clinical treatment of HFpEF.
Data Availability Statement
The datasets presented in this study can be found in online repositories. The names of the repository/repositories and accession number(s) can be found below: NCBI with BioProject ID PRJNA786060 (https://dataview.ncbi.nlm.nih.gov/object/PRJNA786060?reviewer=ibvi0m4rj6766p3qbdsspnl567).
Ethics Statement
The studies involving human participants were reviewed and approved by Suzhou Dushu Lake Hospital Ethics Board. The patients/participants provided their written informed consent to participate in this study. Written informed consent was obtained from the individual(s) for the publication of any potentially identifiable images or data included in this article.
Author Contributions
ZH, YJ, and YZ designed the study. ZH and XM wrote the first draft of this report. YZ, TC, and YJ helped to write the final version. All authors read and met the criteria for authorship and agree with the results and conclusions of the report.
Funding
This work was supported by grants from the National Natural Science Foundation of China (81873486), Suzhou Promoting Health through Science and Education Youth Science and Technology Project (KJXW2020001), Medical research project of Jiangsu Provincial Health Commission (Z2021011), Biomedical translational medicine innovation and applied research project (SZM2021020) and Project of Suzhou Science and Technology Development Program (Applied Basic Research, SKJYD2021045).
Conflict of Interest
The authors declare that the research was conducted in the absence of any commercial or financial relationships that could be construed as a potential conflict of interest.
Publisher's Note
All claims expressed in this article are solely those of the authors and do not necessarily represent those of their affiliated organizations, or those of the publisher, the editors and the reviewers. Any product that may be evaluated in this article, or claim that may be made by its manufacturer, is not guaranteed or endorsed by the publisher.
References
1. Sharma K, Kass DA. Heart failure with preserved ejection fraction. Circ Res. (2014) 115:79–96. doi: 10.1161/CIRCRESAHA.115.302922
2. Gladden JD, Chaanine AH, Redfield MM. Heart failure with preserved ejection fraction. Annu Rev Med. (2018) 69:65–79. doi: 10.1146/annurev-med-041316-090654
3. O'Donovan AN, Herisson FM, Fouhy F, Ryan PM, Whelan D, Johnson CN, et al. Gut microbiome of a porcine model of metabolic syndrome and HF-pEF. Am J Physiol Heart Circ Physiol. (2020) 318:H590–603. doi: 10.1152/ajpheart.00512.2019
4. Harikrishnan S. Diet, the Gut Microbiome and Heart Failure. Card Fail Rev. (2019) 5:119–22. doi: 10.15420/cfr.2018.39.2
5. Becattini S, Taur Y, Pamer EG. Antibiotic-induced changes in the intestinal microbiota and disease. Trends Mol Med. (2016) 22:458–78. doi: 10.1016/j.molmed.2016.04.003
6. Su D, Nie Y, Zhu A, Chen Z, Wu P, Zhang L, et al. Vitamin D Signaling through induction of paneth cell defensins maintains gut microbiota and improves metabolic disorders and hepatic steatosis in animal models. Front Physiol. (2016) 7:498. doi: 10.3389/fphys.2016.00498
7. Luo M, Liu Y, Wu P, Luo DX, Sun Q, Zheng H, et al. Alternation of gut microbiota in patients with pulmonary tuberculosis. Front Physiol. (2017) 8:822. doi: 10.3389/fphys.2017.00822
8. Lim GB. Heart failure: gut flora–pathogenic role in chronic heart failure. Nat Rev Cardiol. (2016) 13:61. doi: 10.1038/nrcardio.2015.200
9. Hayashi T, Yamashita T, Watanabe H, Kami K, Yoshida N, Tabata T, et al. Gut microbiome and plasma microbiome-related metabolites in patients with decompensated and compensated heart failure. Circ J. (2018) 83:182–92. doi: 10.1253/circj.CJ-18-0468
10. Pasini E, Aquilani R, Testa C, Baiardi P, Angioletti S, Boschi F, et al. Pathogenic gut flora in patients with chronic heart failure. JACC Heart Fail. (2016) 4:220–7. doi: 10.1016/j.jchf.2015.10.009
11. Luedde M, Winkler T, Heinsen FA, Rühlemann MC, Spehlmann ME, Bajrovic A, et al. Heart failure is associated with depletion of core intestinal microbiota. ESC Heart Fail. (2017) 4:282–90. doi: 10.1002/ehf2.12155
12. Costanza AC, Moscavitch SD, Faria Neto HC, Mesquita ET. Probiotic therapy with saccharomyces boulardii for heart failure patients: a randomized, double-blind, placebo-controlled pilot trial. Int J Cardiol. (2015) 179:348–50. doi: 10.1016/j.ijcard.2014.11.034
13. Sandek A, Bjarnason I, Volk HD, Crane R, Meddings JB, Niebauer J, et al. Studies on bacterial endotoxin and intestinal absorption function in patients with chronic heart failure. Int J Cardiol. (2012) 157:80–5. doi: 10.1016/j.ijcard.2010.12.016
14. Wang Z, Klipfell E, Bennett BJ, Koeth R, Levison BS, Dugar B, et al. Gut flora metabolism of phosphatidylcholine promotes cardiovascular disease. Nature. (2011) 472:57–63. doi: 10.1038/nature09922
15. Floras JS, Ponikowski P. The sympathetic/parasympathetic imbalance in heart failure with reduced ejection fraction. Eur Heart J. (2015) 36:1974–82. doi: 10.1093/eurheartj/ehv087
16. Packer M, Lam CSP, Lund LH, Maurer MS, Borlaug BA. Characterization of the inflammatory-metabolic phenotype of heart failure with a preserved ejection fraction: a hypothesis to explain influence of sex on the evolution and potential treatment of the disease. Eur J Heart Fail. (2020) 22:1551–67. doi: 10.1002/ejhf.1902
17. Franssen C, Chen S, Unger A, Korkmaz HI, De Keulenaer GW, Tschöpe C, et al. Myocardial microvascular inflammatory endothelial activation in heart failure with preserved ejection fraction. JACC Heart Fail. (2016) 4:312–24. doi: 10.1016/j.jchf.2015.10.007
18. Bhatia RS, Tu JV, Lee DS, Austin PC, Fang J, Haouzi A, et al. Outcome of heart failure with preserved ejection fraction in a population-based study. N Engl J Med. (2006) 355:260–9. doi: 10.1056/NEJMoa051530
19. Segers VF, De Keulenaer GW. Pathophysiology of diastolic dysfunction in chronic heart failure. Future Cardiol. (2013) 9:711–20. doi: 10.2217/fca.13.53
20. McDonagh TA, Metra M, Adamo M, Gardner RS, Baumbach A, Böhm M, et al. 2021 ESC Guidelines for the diagnosis and treatment of acute and chronic heart failure: Developed by the Task Force for the diagnosis and treatment of acute and chronic heart failure of the European Society of Cardiology (ESC) With the special contribution of the Heart Failure Association (HFA) of the ESC. Eur Heart J. (2021) 42:3599–726. doi: 10.1093/eurheartj/ehab670
21. Dias T, de Almeida J, Santos A, Santos RM, Carvalho A. Enterococcus gallinarum causing native valve endocarditis. Eur J Case Rep Intern Med. (2019) 6:001054. doi: 10.12890/2019_001054
22. Pandey R, Sam R. Infective endocarditis in hemodialysis patients. Int J Artif Organs. (2007) 30:334–7. doi: 10.1177/039139880703000409
23. Chatterjee I, Iredell JR, Woods M, Lipman J. The implications of enterococci for the intensive care unit. Crit Care Resusc. (2007) 9:69–75.
24. Gan XT, Ettinger G, Huang CX, Burton JP, Haist JV, Rajapurohitam V, et al. Probiotic administration attenuates myocardial hypertrophy and heart failure after myocardial infarction in the rat. Circ Heart Fail. (2014) 7:491–9. doi: 10.1161/CIRCHEARTFAILURE.113.000978
25. Zhou X, Li J, Guo J, Geng B, Ji W, Zhao Q, et al. Gut-dependent microbial translocation induces inflammation and cardiovascular events after ST-elevation myocardial infarction. Microbiome. (2018) 6:66. doi: 10.1186/s40168-018-0441-4
26. Chang PV, Hao L, Offermanns S, et al. The microbial metabolite butyrate regulates intestinal macrophage function via histone deacetylase inhibition. Proc Natl Acad Sci USA. (2014) 111:2247–52. doi: 10.1073/pnas.1322269111
27. Furusawa Y, Obata Y, Fukuda S, Medzhitov R. Commensal microbe-derived butyrate induces the differentiation of colonic regulatory T cells. Nature. (2013) 504:446–50. doi: 10.1038/nature12721
28. Trachsel J, Humphrey S, Allen HK. Butyricicoccus porcorum sp. nov, a butyrate-producing bacterium from swine intestinal tract. Int J Syst Evol Microbiol. (2018) 68:1737–42. doi: 10.1099/ijsem.0.002738
29. Takada T, Watanabe K, Makino H, Kushiro A. Reclassification of Eubacterium desmolans as Butyricicoccus desmolans comb. nov, and description of Butyricicoccus faecihominis sp nov, a butyrate-producing bacterium from human faces. Int J Syst Evol Microbiol. (2016) 66:4125–31. doi: 10.1099/ijsem.0.001323
30. Jia Q, Li H, Zhou H, Zhang X, Zhang A, Xie Y, et al. Role and Effective Therapeutic Target of Gut Microbiota in Heart Failure. Cardiovasc Ther. (2019) 5164298. doi: 10.1155/2019/5164298
31. Chen X, Li HY, Hu XM, Zhang Y, Zhang SY. Current understanding of gut microbiota alterations and related therapeutic intervention strategies in heart failure. Chin Med J. (2019) 132:1843–55. doi: 10.1097/CM9.0000000000000330
32. Marques FZ, Nelson E, Chu PY, Horlock D, Fiedler A, Ziemann M, et al. High-fiber diet and acetate supplementation change the gut microbiota and prevent the development of hypertension and heart failure in hypertensive mice. Circulation. (2017) 135:964–77. doi: 10.1161/CIRCULATIONAHA.116.024545
33. Hamilton MK, Boudry G, Lemay DG, Raybould HE. Changes in intestinal barrier function and gut microbiota in high-fat diet-fed rats are dynamic and region dependent. Am J Physiol Gastrointest Liver Physiol. (2015) 308:840–51. doi: 10.1152/ajpgi.00029.2015
34. Zheng A, Yi H, Li F, Han L, Yu J, Cheng X, et al. Changes in Gut Microbiome Structure and Function of Rats with Isoproterenol-Induced Heart Failure. Int Heart J. (2019) 60:1176–83. doi: 10.1536/ihj.18-194
35. Kamo T, Akazawa H, Suda W, Saga-Kamo A, Shimizu Y, Yagi H, et al. Dysbiosis and compositional alterations with aging in the gut microbiota of patients with heart failure. PLoS ONE. (2017) 12:e0174099. doi: 10.1371/journal.pone.0174099
36. Nagatomo Y, Tang WH. Intersections between microbiome and heart failure: revisiting the gut hypothesis. J Card Fail. (2015) 21:973–80. doi: 10.1016/j.cardfail.2015.09.017
37. Kain V, Van Der Pol W, Mariappan N, Ahmad A, Eipers P, Gibson D L, et al. Obesogenic diet in aging mice disrupts gut microbe composition and alters neutrophil: lymphocyte ratio, leading to inflamed milieu in acute heart failure. FASEB J. (2019) 33:6456–69. doi: 10.1096/fj.201802477R
Keywords: heart failure with preserved ejection fraction, gut microbiota, alpha diversity, beta diversity, HFpEF-heart failure with preserved ejection fraction
Citation: Huang Z, Mei X, Jiang Y, Chen T and Zhou Y (2022) Gut Microbiota in Heart Failure Patients With Preserved Ejection Fraction (GUMPTION Study). Front. Cardiovasc. Med. 8:803744. doi: 10.3389/fcvm.2021.803744
Received: 10 November 2021; Accepted: 06 December 2021;
Published: 06 January 2022.
Edited by:
Carlo Gabriele Tocchetti, University of Naples Federico II, ItalyReviewed by:
Richard Hu, University of California, Los Angeles, United StatesTongtong Li, Zhejiang University of Technology, China
Copyright © 2022 Huang, Mei, Jiang, Chen and Zhou. This is an open-access article distributed under the terms of the Creative Commons Attribution License (CC BY). The use, distribution or reproduction in other forums is permitted, provided the original author(s) and the copyright owner(s) are credited and that the original publication in this journal is cited, in accordance with accepted academic practice. No use, distribution or reproduction is permitted which does not comply with these terms.
*Correspondence: Yafeng Zhou, zhouyafeng73@126.com; Yufeng Jiang, yufeng_jiang@hotmail.com
†These authors share first authorship