- 1Department of Pediatric Cardiology and Critical Care, Hannover Medical School, Hanover, Germany
- 2Institute of Clinical Chemistry, Hannover Medical School, Hanover, Germany
Objectives: Interleukin-7 (IL-7) secures B cell maturation, regulatory T and natural killer (NK) cell survival, and homeostasis, all of which are important for beneficial immunomodulation in pulmonary arterial hypertension (PAH). However, the role and potential impact of IL-7, VEGF-C and the vascular injury markers ICAM-1, and VCAM-1 on the pathobiology and severity of PAH is unknown.
Methods: EDTA blood was collected during cardiac catheterization from the superior vena cava (SVC), pulmonary artery (PA), and ascending aorta (AAO) in children with pulmonary hypertension (PH) [n = 10; 9.1 (3.9–18.5) years] and non-PH controls [n = 10; 10.5 (2.0–17.3) years]. Compartment-specific plasma concentrations of IL-7, VEGF-C, aldosterone, ICAM-1, and VCAM-1 were determined using Meso Scale Discovery's multi array technology and the LIAISON Aldosterone Assay.
Results: Children with PH had approximately 50% lower IL-7 (p < 0.01) and 59% lower VEGF-C plasma levels (p < 0.001) in the SVC, PA, and AAO versus non-PH controls. IL-7 and VEGF-C concentrations negatively correlated with the pulmonary vascular resistance (PVR)/systemic vascular resistance (SVR) ratio (rho = −0.51 and r = −0.62, respectively). Central-venous IL-7 strongly positively correlated with VEGF-C (r = 0.81). Most patients had a step down in ICAM-1 and VCAM-1 plasma concentrations across the pulmonary circulation and both ICAM-1 and VCAM-1 transpulmonary gradients negatively correlated with invasive hemodynamics.
Conclusion: This manuscript is the first report on decreased circulating IL-7 and VEGF-C plasma concentrations in human PAH and their inverse correlations with invasive surrogates of PAH severity. Additional and larger studies are needed to explore the role of the immune-modulatory IL-7 and VEGF-C in pediatric and adult PAH.
Introduction
The inflammatory response and its resolution, delivered by the innate and adaptive immune systems, are important for development, disease severity, and responses to therapy, in many patients with pulmonary arterial hypertension (PAH) (1, 2). The pro-inflammatory phenotype of pulmonary endothelial cells in PAH is characterized by increased surface expression of intercellular adhesion molecule 1 (ICAM-1), vascular cell adhesion molecule 1 (VCAM-1), and E-selectin, and accompanied by an excessive release of cytokines and chemokines (3). The sequelae include impairment of angiogenesis and cardiovascular repair mechanisms, and promotion of pulmonary vascular remodeling. Emerging evidence suggests that natural killer (NK) cells (4–6) and regulatory T cells (Tregs, CD4+) (7) play major, protective immune-modulatory roles in both preclinical and human PAH, and associated right ventricular dysfunction (RVD). Additional protective factors include hepatocyte growth factor (HGF) that reduces PAH severity and inflammation by attenuation of NF-kB signaling (8), and suppresses vascular medial hyperplasia and extracellular matrix accumulation (9) in the monocrotaline PAH rat model. Interleukin-7 (IL-7) forms a heterodimer with HGF and secures B cell maturation, T and NK cell survival, and homeostasis (10). However, in contrast to HGF, the role and potential impact of IL-7 on the pathobiology and severity of PAH is unknown. In addition, VEGF-C, aldosterone, and the aforementioned vascular injury markers ICAM-1 and VCAM-1 have not been studied in children with PAH, and have not been correlated with invasive hemodynamics.
Here, we report on the compartment-specific plasma concentrations of IL-7, VEGF-C, aldosterone, ICAM-1 and VCAM-1 in the superior vena cava (SVC), pulmonary artery (PA), and ascending aorta (AAO). We investigated 10 well-phenotyped children with moderate PH (WHO functional class 2–3) and 10 age-matched, non-healthy controls with left ventricular outflow tract obstruction (LVOTO; n = 9) and s/p reconstruction of a double aortic arch (n = 1), as previously described (11–13).
Methods
A detailed description of the methods, including cardiac catheterization, simultaneous-compartmental blood draws, and enzyme-linked immunosorbent assays (ELISA) on EDTA plasma, can be found below:
Study Population
10 PH patients and 10 non-PH controls underwent combined right and left heart catheterization. Non-PH controls had left ventricular outflow tract obstruction (LVOTO, n = 9) or s/p reconstruction of a double aortic arch (n = 1). EDTA blood samples, pressure recordings, and blood gas analysis (SpO2) were obtained near-simultaneously at three sites: superior vena cava (SVC), pulmonary artery (PA), and ascending aorta (AAO). To ensure compartment-specific analysis, none of the PH patients, and non-PH controls had intra- or extracardiac shunts. The legal caregivers of each study subject gave written informed consent. Inclusion criteria were defined according to the World Symposium on Pulmonary Hypertension in Nice (2018): mean pulmonary artery pressure (mPAP) > 20 mmHg (14, 15). Detailed information on PH-patients and non-PH controls including demographic data, functional status, and hemodynamics can be found in Table 1. The study has been approved by Hannover Medical School (#2200).
Biomarker Assays
EDTA whole blood samples obtained during cardiac catheterization were immediately centrifuged for 10 min at 1300g. Aldosterone plasma concentrations were measured using the LIAISON Aldosterone Assay (REF 310450, DiaSorin Inc., Stillwater, MN, USA), a chemiluminescent immunoassay (CLIA) in routine clinical use. One sample had to be diluted 1:10 because the initial measured aldosterone concentration was above the detection limit of 1000 pg/ml. IL-7, VEGF-C, ICAM-1 and VCAM-1 plasma concentrations were determined using Meso Scale Discovery's Multi Array technology (MSD, Rockville, MD, USA) according to the manufacturer's instructions. IL-7 (dilution 1:2) plasma concentrations were determined within the Cytokine Panel, VEGF-C (dilution 1:2) within the Angiogenesis Panel and both ICAM-1 and VCAM-1 (dilution 1:1000) within the Vascular Injury Panel. Briefly, the 96-well plate for the Angiogenesis Panel was blocked with 150 μL/well of Blocker A solution and incubated at room temperature with gentle shaking for 1 hour. After blocking, the Angiogenesis Panel follows the same instructions as the Vascular Injury Panel and the Cytokine Panel. All plates were washed with 150 μL/well of wash buffer and 50 μL (Cytokine Panel and Angiogenesis Panel)/25 μL (Vascular Injury Panel) of diluted samples, calibrators or controls were added per well and incubated at 4°C overnight. The next day, the plates were washed three times with wash buffer and 25 μL of detection antibody was added to each well and incubated with gentle shaking for 2 h (Cytokine Panel and Angiogenesis Panel) / for 1 h (Vascular Injury Panel) at room temperature. After the incubation, the plate was again washed three times and 150 μL of Read Buffer was added per well. Signal intensities were determined with the MESO QuickPlex SQ 120 instrument (MSD, Rockville, MD, USA) and analysis was performed using the Discovery Workbench software 4.0 (MSD, Rockville, MD, USA), according to the manufacturer's instructions.
Statistical Analysis
Statistical analyses were performed with the GraphPad Prism 6 software and R. Data are expressed as mean ± standard error (SEM) and were tested for normal distribution with D'Agostino-Pearson omnibus, Shapiro-Wilk, and Kolmogorov-Smirnov tests. If the data passed all three normality tests, unpaired t-test with Welch's correction was performed. If the data were not normally distributed, Mann-Whitney U was used for group comparisons. P-values < 0.05 were considered significant. For the gradient analysis, we used Wald χ2 test implemented using mixed effects models with log2 of fold change (FC) between two catheterization sites (AAO vs. PA for the transpulmonary gradient) as the dependent variable, groups (PAH or control) as an independent variable, and each patient as a random effect [log2(FC)~Group, random = ~1|Patient]. Outliers were removed using the Hampel filter. To remove the most improbable values, we set the parameter sigma to 10. Samples with an outlier in at least one of the three sites were removed. For correlation analysis, normal distribution was tested with Shapiro-Wilk test and either Pearson or Spearman correlation test was used.
Results
Compartment-Specific Plasma Concentrations of IL-7, VEGF-C and Aldosterone in Pediatric PH Patients vs. Non-PH Controls
First, we determined the plasma concentrations of immune-modulatory IL-7: Children with PAH had approximately 50% lower IL-7 concentrations in the SVC (6.61 ± 1.04 vs. 13.25 ± 1.10 pg/mL, p < 0.001), PA (6.77 ± 0.93 vs. 13.63 ± 1.55 pg/mL, p < 0.01) and AAO (6.62 ± 0.82 vs. 13.97 ± 1.58 pg/mL, p < 0.01) vs. in the corresponding sites of controls (Figure 1A). The SVC IL-7 concentrations correlated negatively with the pulmonary vascular resistance (PVR)/systemic vascular resistance (SVR) ratio, an accepted indicator of PAH severity (rho = −0.51, p = 0.026; Figure 1A, right panel). In contrast to our two previous studies on miRNA (11) and lipid metabolites (12), we did not find any trans-RV (PA vs. SVC) or transpulmonary (AAO vs. PA) IL-7 gradients (data not shown).
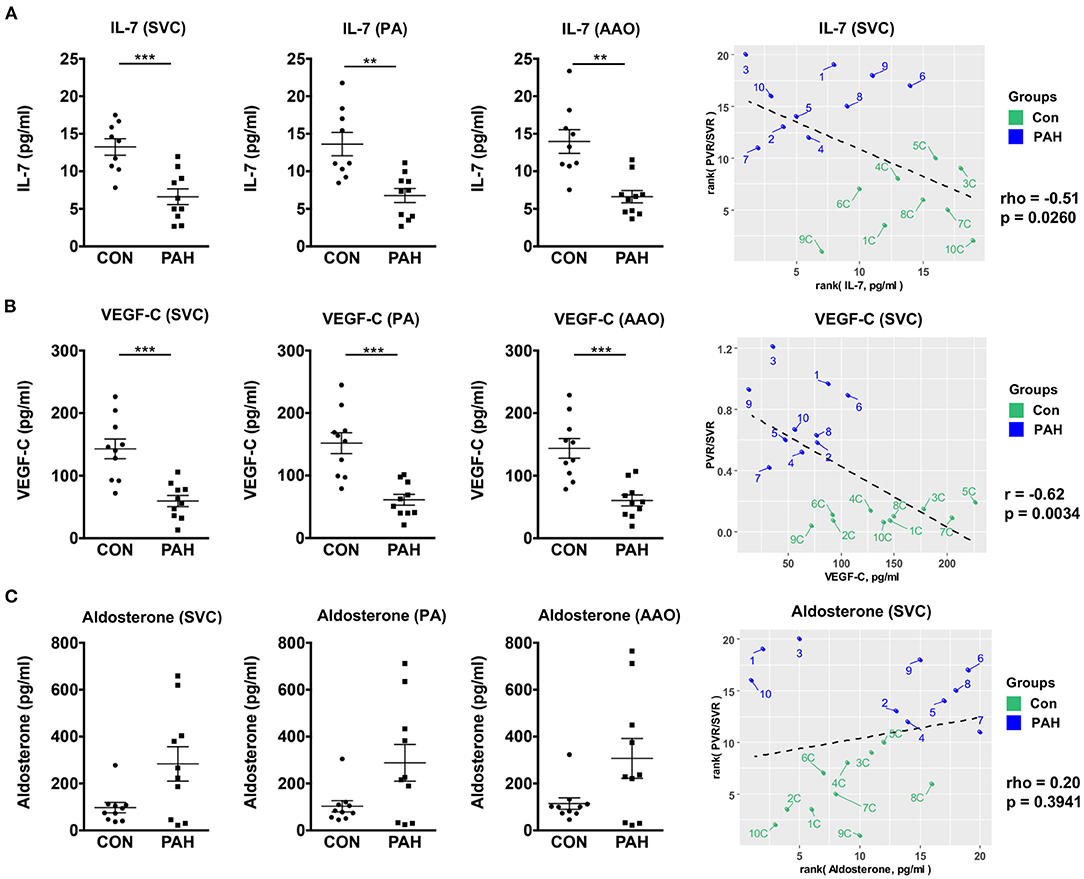
Figure 1. Compartment-specific plasma concentrations of IL-7, VEGF-C and aldosterone in pediatric PH patients vs. non-PH controls. For group comparisons (A–C) mean ± SEM are shown. Statistical test: Unpaired t-test with Welch's correction or Mann-Whitney U test. **p < 0.01, ***p < 0.001. (A) Plasma levels of IL-7 were significantly downregulated in PH in all three sites (SVC, PA, and AAO). (B) Plasma levels of VEGF-C were also significantly downregulated in PAH in all three sites. IL-7 (A) and VEGF-C (B) had moderate (negative) correlation with hemodynamic variables (PVR/SVR ratio). (C) No significant differences in plasma aldosterone concentrations were detected, however, the concentrations tended to be higher in PAH in all three sites. Aldosterone only weakly correlated with the measured hemodynamics (PVR/SVR ratio). For correlation analysis, we used either Spearman's rho (A,C) or Pearson's r (B), as appropriate. AAO, ascending aorta; IL-7, interleukin-7; PA, pulmonary artery; PH, pulmonary hypertension; PVR, pulmonary vascular resistance; SVC, superior vena cava; SVR, systemic vascular resistance; VEGF-C, vascular endothelial growth factor C.
In order to test whether pro-angiogenic factors are decreased in pediatric PAH, we measured plasma VEGF-C concentrations, and found – similarly to IL-7–approximately 59% lower circulating VEGF-C levels in pediatric PAH vs. controls (SVC, PA, AAO; p < 0.001) that correlated negatively with the PVR/SVR ratio (r = −0.62, p = 0.0034; Figure 1B). Central-venous IL-7 strongly positively correlated with VEGF-C (r = 0.81, p < 0.0001; Figure 2), suggesting a possible shared upstream pathway negatively affecting IL-7 mediated immunomodulation and VEGF-C induced angiogenesis in pediatric PAH. In addition, there was a trend toward higher aldosterone levels in PAH vs. controls in children, but there was no correlation between plasma aldosterone and PAH severity (PVR/SVR ratio; Figure 1C right panel). We had previously studied 41 adult patients with IPAH, and 8 age-matched, unrelated controls (16). Aldosterone plasma concentrations were similar in the PAH children under study (SVC, mean 283.6 ± 73.0 pg/ml; Figure 1C) and those of adults with IPAH (mean 248.5 ± 38.8 pg/ml) (16).
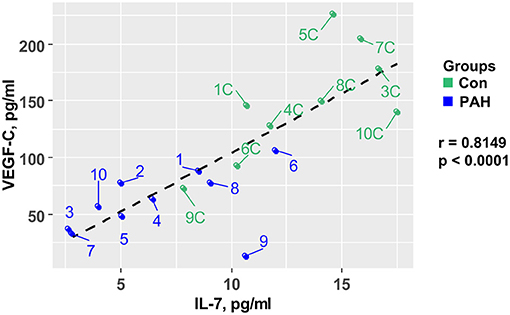
Figure 2. Central-venous IL-7 in pediatric samples strongly positively correlates with VEGF-C. IL-7 plasma concentrations correlate with VEGF-C plasma concentrations measured in the superior vena cava (SVC) of 10 PH patients and 10 non-PH controls. Statistical test: Pearson. IL-7, interleukin-7; PH, pulmonary hypertension; VEGF-C, vascular endothelial growth factor C.
Transpulmonary Decrease in the Vascular Injury Markers ICAM-1 and VCAM-1 in Pediatric PH Patients vs. Non-PH Controls
Next, we investigated the vascular injury markers ICAM-1 and VCAM-1 in pediatric PH. Most patients had a step down in ICAM-1 (Figure 3A) and VCAM-1 (Figure 3B) plasma concentrations across the pulmonary circulation (AAO vs. PA gradients) with the following fold changes (FC): FCPAH_ICAM−1 = 0.86, FCControl_ICAM−1 = 1.21, FCPAH_VCAM−1 = 0.85, and FCControl_ICAM−1 = 1.28. Both ICAM-1 and VCAM-1 transpulmonary gradients had moderate negative correlation with surrogates of PAH severity: ICAM-1 (AAO vs. PA) vs. mPAP/mSAP ratio (rho = −0.47, p = 0.044; Figure 3A) and VCAM-1 (AAO vs. PA) vs. dPAP/dSAP ratio (rho = −0.42, p = 0.079; Figure 3B). The decrease in transpulmonary ICAM-1 and VCAM-1 concentrations in PAH children (fold change 0.86 and 0.85, respectively; Figures 3A,B) indicates either intrapulmonary uptake or increased intrapulmonary degradation of these vascular injury mediators that drive endothelial dysfunction (1, 2).
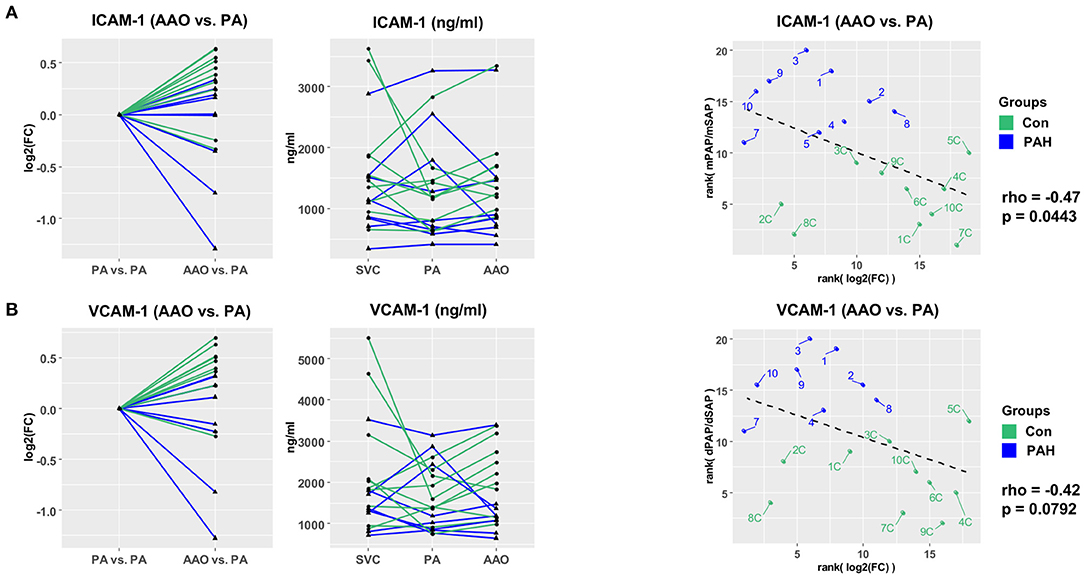
Figure 3. Vascular injury markers ICAM-1 and VCAM-1 have differential transpulmonary gradients in pediatric PH vs. non-PH controls. (A) Step-down in PAH (FC = 0.86) and step-up in controls (FC = 1.21) for ICAM-1 levels. (B) Step-down in PAH (FC = 0.85) and step-up in controls (FC = 1.28) for VCAM-1. For transpulmonary gradient analysis, we used Wald χ2 test (linear mixed effects models). For correlation analysis, we used Spearman's rho (A,B). ICAM-1 and VCAM-1 had moderate negative correlation with hemodynamic variables (mPAP/mSAP; dPAP/dSAP). AAO, ascending aorta; dPAP, diastolic pulmonary arterial pressure; dSAP, diastolic systemic arterial pressure; ICAM-1, intercellular adhesion molecule 1; mPAP, mean pulmonary arterial pressure; mSAP, mean systemic arterial pressure; PA, pulmonary artery; PH, pulmonary hypertension; SVC, superior vena cava; VCAM-1, vascular cell adhesion molecule 1.
Discussion
Here, we report for the first time invasive, compartment-specific levels of circulating IL-7, VEGF-C, aldosterone, ICAM-1 and VCAM-1 in children with moderate PH. We found a very pronounced decrease in plasma IL-7 concentrations in pediatric PH patients vs. non-PH controls, which inversely correlated with precapillary PH severity, as assessed by the ratio of PVR/SVR. Similarly to IL-7, we found approximately 59% lower circulating VEGF-C levels in pediatric PAH vs. controls that correlated negatively with the PVR/SVR ratio. Central-venous IL-7 strongly positively correlated with VEGF-C. Additionally, there was a trend toward higher aldosterone levels in pediatric PH patients vs. controls, with levels similar to those previously measured in adults (16). Transpulmonary gradient analysis revealed that most patients had a step down in ICAM-1 and VCAM-1 plasma concentrations across the pulmonary circulation, indicating either intrapulmonary uptake or increased intrapulmonary degradation of these vascular injury markers.
The rationale to investigate circulating IL-7 in (pediatric) PAH was its aforementioned role in NK and Treg cell survival (4–7), both of which are thought to be crucial for the adaptations necessary in PAH and RVD. NK cells are the innate immune equivalent of cytotoxic T cells that spot stressed or damaged cells and induce targeted cell death. NK cells are mainly circulating in the blood stream, but resident populations have also been found in lung, liver, and lymph nodes. NK cells are reduced in number and function in peripheral blood of adults with PAH, compared to control subjects, releasing less cytotoxic granules (4). Dysregulated immunity resulting from deficient Treg activity in athymic rats contributes to increased inflammation, leading to macrophage activation and rapid progressive PAH and RV failure (7).
Consistent with our finding of the greatly decreased IL-7 concentrations in the systemic and pulmonary circulation of children with PAH (Figure 1), others had demonstrated reduced expression of IL-7 receptor (IL-7R) in circulating PBMC and CD4+ T-cells from adult scleroderma (SSc) patients with PAH vs. those without PAH (17).
According to the aforementioned SSc-PAH study and our current findings, we postulate that blood cell IL-7/IL7R signaling is decreased in adult and pediatric PAH. However, increased immunoreactivity for IL-7 was found in tertiary (ectopic) lymphoid tissues, i.e., highly organized perivascular follicles, in adult idiopathic PAH (IPAH) lungs, suggesting specific immune-adaptive mechanisms in the pathophysiology of the disease, at least in adults (18). So far, it is unclear whether intrapulmonary IL-7 signaling is a detrimental driver or counterregulatory protector in pulmonary lymphoid neogenesis (18), and other hallmarks of PAH pathobiology.
Additionally, we revealed for the first time decreased levels of VEGF-C in pediatric PAH patients. VEGF-C primarily signals through vascular endothelial growth factor receptor 3 (VEGFR3) which is expressed in endothelial cells and regulates cardiovascular development and lymphangiogenesis (19, 20). VEGFR3 has been found to be a positive regulator of bone morphogenetic protein receptor 2 (BMPR2) signaling in PAH and knockout of VEGFR3 in endothelial cells caused exacerbation of hypoxia-induced PH in mice (21). Consistent with our finding of significantly decreased VEGF-C plasma levels in PH children, the expression of VEGFR3 (both VEGFR3 mRNA and protein) was found to be decreased in pulmonary arterial endothelial cells isolated from adult PAH patients vs. controls (21).
In our previous human biomarker study, ICAM-1 was increased in adult IPAH and CTD-PAH vs. controls, whereas VCAM-1 and pro-inflammatory, anti-angiogenic interleukin 12 (IL-12) were significantly elevated in CTD-PAH only (16). In the same study, we showed for the first time that a clinically relevant aldosterone/galectin-3 axis exists in adult PAH and associates with clinical symptoms (WHO functional class) (16).
Conclusion
The limitations of our study are common in biomarker studies on a rare disease, and include small sample size (children), non-healthy controls (invasive diagnostics), and a wide age range. Nevertheless, this article is the first report on decreased circulating IL-7 and VEGF-C plasma concentrations in human PAH and their inverse correlations with invasive surrogates of PAH severity. Additional and larger studies are warranted to explore the role of the immune-modulatory IL-7 and VEGF-C at multiple locations, cells and tissues (circulation, pulmonary vascular/lymph vessels, RV myocardium) in pediatric and adult PAH.
Data Availability Statement
The raw data supporting the conclusions of this article will be made available by the authors, without undue reservation.
Ethics Statement
The studies involving human participants were reviewed and approved by Hannover Medical School (#2200). Written informed consent to participate in this study was provided by the participants' legal guardian/next of kin.
Author Contributions
FD provided clinical data, performed experiments and statistical analysis, produced display items, and wrote parts of the manuscript. EL supervised, performed experiments and statistical analysis, and produced display items. PC performed advanced data analysis and produced display items. HB, CH, and GH collected blood samples during pediatric cardiac catheterizations. GH conceptualized, designed and supervised the study, performed experiments, and wrote the manuscript. All authors reviewed and revised the manuscript for important intellectual content.
Funding
This study was supported by the German Research Foundation (DFG; HA4348/2-2 and KFO311 HA4348/6-2 to GH) and the European Pediatric Pulmonary Vascular Disease Network (www.pvdnetwork.org). GH receives additional funding from the Federal Ministry of Education and Research (BMBF ViP+ program 03VP08053; BMBF 01KC2001B).
Conflict of Interest
The authors declare that the research was conducted in the absence of any commercial or financial relationships that could be construed as a potential conflict of interest.
Publisher's Note
All claims expressed in this article are solely those of the authors and do not necessarily represent those of their affiliated organizations, or those of the publisher, the editors and the reviewers. Any product that may be evaluated in this article, or claim that may be made by its manufacturer, is not guaranteed or endorsed by the publisher.
Acknowledgments
We thank the staff of the pediatric cardiac catheterization laboratory and Dr. Jana Sarkander (Mesoscale Discovery), for assistance with blood sample analysis.
Abbreviations
AAO, ascending aorta; BSA, body surface area; BMPR2, bone morphogenetic protein receptor 2; dPAP, diastolic pulmonary arterial pressure; dTPG, diastolic transpulmonary pressure gradient; dSAP, diastolic systemic arterial pressure; HGF, hepatocyte growth factor; HPAH, heritable pulmonary arterial hypertension; ICAM-1, intercellular adhesion molecule 1; IL-7, interleukin-7; IPAH, idiopathic pulmonary arterial hypertension; LV, left ventricle; LVEDP, left ventricular end-diastolic pressure; LVEF, left ventricular ejection fraction; LVOTO, left ventricular outflow tract obstruction; mPAP, mean pulmonary arterial pressure; mRAP, mean right atrial pressure; mSAP, mean systemic arterial pressure; mTPG, mean transpulmonary pressure gradient; NK cells, natural killer cells; NTproBNP, N-terminal pro b-type natriuretic peptide; PA, pulmonary artery; PAAT, pulmonary artery acceleration time; PAWP, pulmonary arterial wedge pressure; PAH, pulmonary arterial hypertension; PH, pulmonary hypertension; PSAX, parasternal short axis; PVRi, pulmonary vascular resistance index (indexed to body surface area); Qpi, pulmonary flow index; Qsi, systemic flow index; RV, right ventricle; RVAWD, right ventricular anterior wall diameter; RVEDD, right ventricular end-diastolic diameter; sPAP, systolic pulmonary arterial pressure; sSAP, systolic systemic arterial pressure; SVC, superior vena cava; SVR, systemic vascular resistance; TAPSE, tricuspid annular plane systolic excursion; VEGF-C, vascular endothelial growth factor C; VEGFR3, vascular endothelial growth factor receptor 3; VCAM-1, vascular cell adhesion molecule 1; WHO, world health organization.
References
1. Rabinovitch M, Guignabert C, Humbert M, Nicolls MR. Inflammation and immunity in the pathogenesis of pulmonary arterial hypertension. Circ Res. (2014) 115:165–75. doi: 10.1161/CIRCRESAHA.113.301141
2. Humbert M, Guignabert C, Bonnet S, Dorfmuller P, Klinger JR, Nicolls MR, et al. Pathology and pathobiology of pulmonary hypertension: state of the art and research perspectives. Eur Respir J. (2019) 53:1801887. doi: 10.1183/13993003.01887-2018
3. Le Hiress M, Tu L, Ricard N, Phan C, Thuillet R, Fadel E, et al. Proinflammatory signature of the dysfunctional endothelium in pulmonary hypertension. role of the macrophage migration inhibitory factor/CD74 complex. Am J Respir Crit Care Med. (2015) 192:983–97. doi: 10.1164/rccm.201402-0322OC
4. Ormiston ML, Chang C, Long LL, Soon E, Jones D, Machado R., et al. Impaired natural killer cell phenotype and function in idiopathic and heritable pulmonary arterial hypertension. Circulation. (2012) 126:1099–109. doi: 10.1161/CIRCULATIONAHA.112.110619
5. Ratsep MT, Moore SD, Jafri S, Mitchell M, Brady HJM, Mandelboim O., et al. Spontaneous pulmonary hypertension in genetic mouse models of natural killer cell deficiency. Am J Physiol Lung Cell Mol Physiol. (2018) 315:L977–90. doi: 10.1152/ajplung.00477.2017
6. Suen CM, Chaudhary KR, Deng Y, Jiang B, Stewart DJ. Fischer rats exhibit maladaptive structural and molecular right ventricular remodelling in severe pulmonary hypertension: a genetically prone model for right heart failure. Cardiovasc Res. (2019) 115:788–99. doi: 10.1093/cvr/cvy258
7. Tian W, Jiang X, Tamosiuniene R, Sung YK, Qian J, Dhillon G, et al. Blocking macrophage leukotriene b4 prevents endothelial injury and reverses pulmonary hypertension. Sci Transl Med. (2013) 5:200ra117. doi: 10.1126/scitranslmed.3006674
8. Pang Y, Liang MT, Gong Y, Yang Y, Bu PL, Zhang M., et al. HGF reduces disease severity and inflammation by attenuating the NF-kappaB signaling in a rat model of pulmonary artery hypertension. Inflammation. (2018) 41:924–31. doi: 10.1007/s10753-018-0747-1
9. Ono M, Sawa Y, Mizuno S, Fukushima N, Ichikawa H, Bessho K., et al. Hepatocyte growth factor suppresses vascular medial hyperplasia and matrix accumulation in advanced pulmonary hypertension of rats. Circulation. (2004) 110:2896–902. doi: 10.1161/01.CIR.0000146342.30470.30
10. Barata JT, Durum SK, Seddon B. Flip the coin: IL-7 and IL-7R in health and disease. Nat Immunol. (2019) 20:1584–93. doi: 10.1038/s41590-019-0479-x
11. Chouvarine P, Geldner J, Giagnorio R, Legchenko E, Bertram H, Hansmann G. Trans-right-ventricle and transpulmonary MicroRNA gradients in human pulmonary arterial hypertension. Pediatr Crit Care Med. (2020) 21:340–9. doi: 10.1097/PCC.0000000000002207
12. Chouvarine P, Giera M, Kastenmuller G, Artati A, Adamski J, Bertram H., et al. Trans-right ventricle and transpulmonary metabolite gradients in human pulmonary arterial hypertension. Heart. (2020) 106:1332–41. doi: 10.1136/heartjnl-2019-315900
13. Diekmann F, Chouvarine P, Sallmon H, Meyer-Kobbe L, Kieslich M, Plouffe BD, et al. Soluble receptor for advanced glycation end products (sRAGE) is a sensitive biomarker in human pulmonary arterial hypertension. Int J Mol Sci. (2021) 22:8591. doi: 10.3390/ijms22168591
14. Simonneau G, Montani D, Celermajer DS, Denton CP, Gatzoulis MA, Krowka M, et al. Haemodynamic definitions and updated clinical classification of pulmonary hypertension. Eur Respir J. (2019) 53:1801913. doi: 10.1183/13993003.01913-2018
15. Rosenzweig EB, Abman SH, Adatia I, Beghetti M, Bonnet D, Haworth S, et al. Paediatric pulmonary arterial hypertension: updates on definition, classification, diagnostics and management. Eur Respir J. (2019) 53:1801916. doi: 10.1183/13993003.01916-2018
16. Calvier L, Legchenko E, Grimm L, Sallmon H, Hatch A, Plouffe BD, et al. Galectin-3 and aldosterone as potential tandem biomarkers in pulmonary arterial hypertension. Heart. (2016) 102:390–6. doi: 10.1136/heartjnl-2015-308365
17. Risbano MG, Meadows CA, Coldren CD, Jenkins TJ, Edwards MG, Collier D., et al. Altered immune phenotype in peripheral blood cells of patients with scleroderma-associated pulmonary hypertension. Clin Transl Sci. (2010) 3:210–8. doi: 10.1111/j.1752-8062.2010.00218.x
18. Perros F, Dorfmuller P, Montani D, Hammad H, Waelput W, Girerd B, et al. Pulmonary lymphoid neogenesis in idiopathic pulmonary arterial hypertension. Am J Respir Crit Care Med. (2012) 185:311–21. doi: 10.1164/rccm.201105-0927OC
19. Olsson AK, Dimberg A, Kreuger J, Claesson-Welsh L. VEGF receptor signalling - in control of vascular function. Nat Rev Mol Cell Biol. (2006) 7:359–71. doi: 10.1038/nrm1911
20. Ma Z, Yu YR, Badea CT, Kovacs JJ, Xiong X, Comhair S, et al. Vascular endothelial growth factor receptor 3 regulates endothelial function through beta-arrestin 1. Circulation. (2019) 139:1629–42. doi: 10.1161/CIRCULATIONAHA.118.034961
21. Hwangbo C, Lee HW, Kang H, Ju H, Wiley DS, Papangeli I, et al. Modulation of endothelial bone morphogenetic protein receptor type 2 activity by vascular endothelial growth factor receptor 3 in pulmonary arterial hypertension. Circulation. (2017) 135:2288–98. doi: 10.1161/CIRCULATIONAHA.116.025390
Keywords: IL-7, VEGF-C, ICAM-1, VCAM-1, innate and adaptive immunity, pulmonary arterial hypertension, transpulmonary pressure gradient, vascular injury
Citation: Diekmann F, Legchenko E, Chouvarine P, Lichtinghagen R, Bertram H, Happel CM and Hansmann G (2021) Circulating Interleukin-7 in Human Pulmonary Arterial Hypertension. Front. Cardiovasc. Med. 8:794549. doi: 10.3389/fcvm.2021.794549
Received: 13 October 2021; Accepted: 08 November 2021;
Published: 08 December 2021.
Edited by:
Hannes Sallmon, Deutsches Herzzentrum Berlin, GermanyReviewed by:
Martin Koestenberger, Medical University of Graz, AustriaHirotsugu Tsuchimochi, National Cerebral and Cardiovascular Center, Japan
Copyright © 2021 Diekmann, Legchenko, Chouvarine, Lichtinghagen, Bertram, Happel and Hansmann. This is an open-access article distributed under the terms of the Creative Commons Attribution License (CC BY). The use, distribution or reproduction in other forums is permitted, provided the original author(s) and the copyright owner(s) are credited and that the original publication in this journal is cited, in accordance with accepted academic practice. No use, distribution or reproduction is permitted which does not comply with these terms.
*Correspondence: Georg Hansmann, georg.hansmann@gmail.com
†These authors have contributed equally to this work and share first authorship