- 1Department of Cardiology, Dongzhimen Hospital Affiliated to Beijing University of Chinese Medicine, Beijing, China
- 2Department of Cardiology, Xiyuan Hospital, China Academy of Chinese Medical Sciences, Beijing, China
- 3Department of Cardiology, Dongfang Hospital Beijing University of Chinese Medicine, Beijing, China
Percutaneous coronary intervention (PCI) is one of the most effective reperfusion strategies for acute myocardial infarction (AMI) despite myocardial ischemia/reperfusion (I/R) injury, causing one of the causes of most cardiomyocyte injuries and deaths. The pathological processes of myocardial I/R injury include apoptosis, autophagy, and irreversible cell death caused by calcium overload, oxidative stress, and inflammation. Eventually, myocardial I/R injury causes a spike of further cardiomyocyte injury that contributes to final infarct size (IS) and bound with hospitalization of heart failure as well as all-cause mortality within the following 12 months. Therefore, the addition of adjuvant intervention to improve myocardial salvage and cardiac function calls for further investigation. Phytochemicals are non-nutritive bioactive secondary compounds abundantly found in Chinese herbal medicine. Great effort has been put into phytochemicals because they are often in line with the expectations to improve myocardial I/R injury without compromising the clinical efficacy or to even produce synergy. We summarized the previous efforts, briefly outlined the mechanism of myocardial I/R injury, and focused on exploring the cardioprotective effects and potential mechanisms of all phytochemical types that have been investigated under myocardial I/R injury. Phytochemicals deserve to be utilized as promising therapeutic candidates for further development and research on combating myocardial I/R injury. Nevertheless, more studies are needed to provide a better understanding of the mechanism of myocardial I/R injury treatment using phytochemicals and possible side effects associated with this approach.
Introduction
AMI remains the world's leading cause of morbidity and mortality (1). Of all the deaths, adverse acute ischemic events, such as ST-elevation myocardial infarction (STEMI), are the main triggers (2). In recent years, most of the endeavors in the processing of STEMI have been focused on guaranteeing the prompt coronary revascularization of the culprit artery and exploitation of pharmacological regimens for further preservation of the coronary blood flow (3, 4). Early primary percutaneous coronary intervention (pPCI; within 2 h since symptoms onset) has proved effective in reducing ischemia time to improve the outcomes of patients with STEMI (5); however, the cardiomyocytes begin to die having experienced a long-term ischemic environment. Even though reperfusion proves effective in limiting this process, it causes a spike of further cardiomyocyte injury (known as “reperfusion injury”) that contributes to final IS (6), which remains a crucial determinant of prognosis and is bound with hospitalization of heart failure as well as all-cause mortality within the following 12 months. While the ischemic injury increases with the severity and the duration of blood flow reduction, reperfusion injury achieves its maximum with a moderate amount of ischemic injury (7). Therefore, the addition of adjuvant intervention to limit cardiomyocyte death during myocardial I/R injury has become necessary.
The exact mechanisms of how the homeostasis of cardiac cells is impaired during myocardial I/R injury are not fully understood (8). Pathological changes, such as calcium overload, inflammation, apoptosis, neurohumoral activation, autophagy, and oxidative stress, are considered to be of equal contribution to I/R injury (9). Phytochemicals, the secondary metabolites and natural components of herbs, mainly composed of non-nutritive bioactive compounds, have long been recognized as promising therapeutic candidates for novel drugs (10). They are synthesized only in specific plant cells and do not take part in the energy metabolism nor the catabolic or anabolic ones (11). More than 10,000 phytochemicals have been discovered so far, including saponins, polyphenols, carotenoids, terpenes, and alkaloids, while many remain unknown (12). In recent years, they have attracted more attention as modulators of many cellular signaling pathways and by the ability of health improvement (13). For example, metabolic disorders, such as cardiovascular disease, cancer, and obesity, may benefit from many phytochemicals (14). Research and clinical studies have demonstrated the compounds' biological effects, such as antioxidant, anti-inflammatory, and cytotoxic activities, suggesting that these natural products may be potential to alleviate the myocardial I/R injury (15, 16).
This review provides a concise summary of the efforts of former researchers on how phytochemicals alleviate myocardial I/R injury and highlights the evidence of their cardioprotective-related mechanisms. We aim to present new insight into the development of potential treatments for myocardial I/R injury.
The Mechanism of Myocardial I/R Injury
Many pathways that induce cell death, also known as apoptosis, programmed necrosis, or necroptosis, are initiated by myocardial I/R injury, involving several signaling pathways (9). Therefore, it is necessary to find improved protective strategies to prevent myocardial I/R injury, of which the related mechanisms have been widely studied. A growing number of pieces of research, both in vitro and in vivo, have proved phytochemicals to be potently cardioprotective on myocardial I/R injury, mainly by restraining irreversible cell death caused by apoptosis, autophagy, and necrosis via preventing calcium overload, oxidative stress, and inflammation (17) (Figure 1).
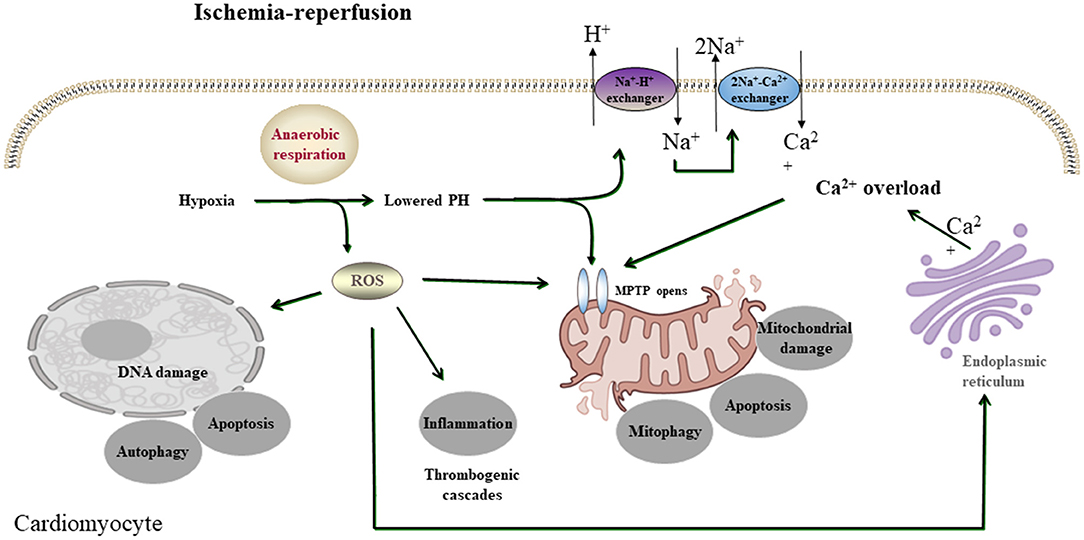
Figure 1. A simplified scheme of the mechanism of acute myocardial I/R injury. During acute myocardial ischemia, ischemic cardiomyocytes switch to anaerobic metabolism to provide ATP. However, this results in the Na+-H+ exchanger to extrude H+ and results in intracellular Na+ overload, which activates the 2Na+-Ca2+ exchanger to function in reverse to extrude Na+ and leads to intracellular Ca2+ overload. The endoplasmic reticulum also markedly reduces Ca2+ reuptake, which exacerbates intracellular Ca2+ overload. Ca2+ can also induce MPTP opening. During reperfusion, the influx of oxygen fuels production of ROS (oxygen paradox). Other sources of ROS include xanthine oxidase (endothelial cells) and NADPH oxidase (neutrophils). ROS can damage virtually every biomolecule found in cells, promote the opening of mPTPs, and activate inflammatory and thrombogenic cascades to exacerbate cell injury.
Apoptosis
Apoptosis exists in several cellular organisms. Stimulation of apoptotic pathways leads to cell death in ischemic heart cells (18). Generally, the high level of reactive oxygen species (ROS) can be lethal for I/R-injured cells and attributed to cardiomyocyte apoptosis (19). It is proved that during myocardial I/R injury, apoptosis-related genes, such as signal transducer and activator of transcription 3(STAT3), B-cell lymphoma-C(BCL-C), and B-cell lymphoma-xL(Bcl-xL) in the myocardial tissue, are rearranged (20). There is evidence that the increased expression of these genes produces protective proteins against apoptotic pathways and reduces physiotherapy (21). Ca2+ signaling, which can be modulated and synchronized by mitochondria, is an essential part of apoptosis. Accumulation of Ca2+ in mitochondria leads to apoptosis (22). In porcine models of chronic myocardial ischemia and hibernation, autophagy-enhanced cardiomyocytes were negative for apoptosis, while apoptotic cells were negative for autophagy, suggesting that autophagy plays a protective role against apoptosis in this model (23). mTORC1 can sense cellular nutrient status (24) and inhibits myocardial I/R injury. Growth factor receptor-bound protein 1(GRb1) treatment antagonizes the inhibitory effect of mTORC1 (25). B-cell lymphoma-2(Bcl-2) has the potential to inhibit apoptosis, mitochondria disruption, the following cytochrome c(Cyt c) release, and, finally, caspase activation (26). Pretreating with Eupatilin can increase Bcl-2 expression, decrease BCL2-associated X(Bax), and cleaved caspase-3 expression induced by hypoxia-reoxygenation (H/R) in H9c2 cells (27).
Autophagy
Autophagy is essential to maintain cellular homeostasis. But its effects on myocardial I/R injury are paradoxical (17). Autophagy is characterized by the formation of a cup-shaped pre-autophagosomal double-membrane structure, which surrounds cytoplasmic material and closes to form the autophagosome (19). Autophagosome clearance, which can cause autophagy acceleration and cardiomyocyte death, is damaged during I/R injury (28). The knocking out of Beclin1 heterozygous eliminates myocardial I/R-induced autophagosome formation, as well as reduces myocardial infarction and cell death (29). Likewise, 3-methyladenine (3-MA) reduces autophagy caused by I/R via prohibiting autophagy and increases survived cells (30). Hesperidin can inhibit excessive autophagy by triggering the PI3K/Akt/mammalian target of the rapamycin (mTOR) pathway. Hesperidin was found to be capable of reinforcing p-PI3K, p-Akt, and p-mTOR levels and downregulating LC3II and Beclin1, whereas its specific inhibitor, LY294002, obviously invalidated all the effects mentioned above (31).
On the contrary, autophagy has been widely reported to be beneficial to myocardial I/R injury. The recovery of myocardium function after I/R benefits from a high level of autophagy. However, the depletion of adenosine triphosphate (ATP) is possibly the reason for autophagosome-lysosome pathway impairment during ischemia, which correlates with permanent injury in contractile function (32). With enhanced autophagy, apoptosis decreases in cardiac myocytes, same as autophagy in apoptotic cells in the porcine model of chronic myocardial ischemia and hibernating myocardium. Therefore, there is a deduction that cells are protected by autophagy against apoptosis in this model (33). Also, glucose deprivation-mediated cell death can be promoted when autophagy is inhibited (29). It can be concluded that when autophagy is upregulated, cells are likely to survive during I/R. Resveratrol is found to alleviate I/R injury of the myocardium in diabetic patients by promoting programmed cell death and via upregulating Beclin 1 and LC3-II (34).
Ca2+ Overload
When there is myocardium hypoperfusion, affected cardiomyocytes switch to use less oxygen, leading to lactate, H+, and nicotinamide adenine dinucleotide (NADH+) accumulation and cytosolic pH decrease. To reestablish acid-based balance, the plasmalemma Na+/Ca2+ exchanger is activated. Then, the extracellular H+ ions accumulated during ischemia raise the proton gradient across the plasmalemma and further result in cytosolic Ca2+ increase (9). In addition, under physiological conditions, inactive calpains compete with their endogenous inhibitor calpastatin and are stored in cellular cytosol (35). Calpain is activated by the elevation of intracellular calcium levels, and its conformational changes promote the intracellular translocation of Ca2+, where phospholipids close Ca2+ channels, activating downstream proteins or diminishing the Ca2+ threshold for calpain activation (36). Myocardial ischemia/reperfusion injury is associated with a calcium homeostasis imbalance (37). In vivo studies showed an increment in intracellular Ca2+ concentration caused by ischemia/reperfusion in isolated perfused mammalian hearts (36). Reperfusion leads to rapid alterations in ion flux and alters the state of ion exchange, resulting in intracellular calcium overload (38). Increased calcium overload plays a key role in apoptosis, cell cycle, and differentiation, modifying cardiomyocyte function.
Oxidative Stress
Oxidative stress is the result of an imbalance between oxidants and anti-oxidants. When the blood supply in an ischemic area is reestablished, the influx of oxygen produces excessive ROS, which is harmful to the ischemic area. This phenomenon is called the oxygen paradox, meaning that reperfusion after ischemia can result in injury rather than protection. This is because ROS modifies the metabolism in cells and tissues, leading to dysfunction or even cell death (39). ROS is the reason why I/R is deleterious for cells and tissues (40). Thus, oxidative stress reduction may combat I/R injury, and further investigations are needed. NF-E2-related factor 2(Nrf2), a member of the NF-E2 family of nuclear basic leucine zipper transcription factors, promotes the detoxification of pro-oxidative stressors. The Nrf2 signaling pathway plays a critical role in protecting the ischemic myocardium from myocardial I/R injury. Nrf2 deficiency mice show increased oxidative stress as well as an aggravated cardiac injury during I/R (41).
Inflammation
Inflammation is a strong shield to protect the body, but dysfunctional inflammation has much to do with the pathogenesis of many diseases. Leukocyte infiltration can be activated in the infarcted myocardial region via a complex inflammatory pathway to protect unaffected regions (42). Evidence shows that, in a heart, reperfused areas can be harmed by an excessively activated inflammatory reaction (43). Nuclear factor kappa-light-chain enhancer of the activated B cells (NF-κB) signaling pathway is crucial to cardiac I-R injury. NF-κB interacts with the nucleus by regulating more than 200 genes, among which some produce inflammatory cytokines, which ultimately lead to excessive inflammation. H9C2 cells, which are damaged by hypoxia through BRCA1/ROS-regulated NLRP3 inflammasome/IL-1β and NF-κB/TNF-α/IL-6 pathways, can be improved by Paeonol (44). When intercellular macromolecular proteins aggregate, they are called inflammasomes, which promote the maturation of inflammatory cytokines (45). NLRP3 consists of NLRP3, ASC, and caspase-1 precursor (Pro-Casp-1) (46) and is the most widely studied inflammatory pathway for now. Artemisinin can reduce the oxidative stress reaction due to its NLRP3-regulating ability (47).
Cardioprotective Phytochemicals Attenuating Myocardial I/R Injury
We thoroughly illustrated the phytochemicals proved to possess protective effects in the heart against I/R injury. Because of their variety, phytochemicals have been classified into phenols, saponins, lignans, terpenes, alkaloids, quinones, polysaccharides, carotenoids, coumarin, and other compounds for a better summary in this review.
Phenols
Phenolic compounds constitute one of the most ubiquitous groups of plant metabolites and are an integral part of both human and animal diets (48). Among numerous natural phytochemicals used to prevent myocardial I/R injury, phenolic compounds are particularly important because of their unique properties. Although, these compounds were first known for their antioxidant properties, several studies over the years have shown that they can exert protective effects against myocardial I/R injury. The mechanisms underlying these potential benefits include the regulation of different cell signaling pathways and gene expression.
Paeonol
Paeonol (2′-hydroxy-4′-methoxyacetophenone), isolated from the plant Moutan Cortex, was found to possess broad pharmacological effects on treating atherosclerotic lesions. This is associated with alleviating endothelial injury, ameliorating inflammation and oxidative stress, repressing platelet activation and aggregation, inhibiting vascular smooth muscle cell (VSMC) proliferation and migration, as well as lowering blood lipids (49–53). Pretreating with paeonol can significantly improve the hypoxia-reoxygenation (H/R) damage and the BRCA1 expression of H9C2 cells through the BRCA1/ROS-regulated NLRP3 inflammasome/IL-1β and NF-κB/TNF-α/IL-6 pathways. It may be a candidate drug for treating myocardial I/R injury (44).
Oridonin
Oridonin is a wide-studied flavonoid compound extracted from Isodon rubescens (Hemsl.) H.Hara, and it has a multitargeting anticancer effect (54). Lu et al. demonstrated that it exerts cardioprotective effects by reducing I/R-induced inflammatory injury. Pretreating with oridonin also reduced oxidative stress and downregulated the NLRP3 inflammasome pathway. These recent findings have shown the molecular mechanism of its alleviating myocardial I/R injury. Applying oridonin could help prevent and treat myocardial I/R injury (55).
Baicalin
Baicalin is a flavonoid compound isolated from the roots of Scutellaria baicalensis Georgi. It proves effective in treating diseases like cancer, osteoarthritis, hepatitis, and nephritis (56–58). It was reported that baicalin exerted antioxidant, anti-apoptotic, and anti-inflammatory properties (59). Studies demonstrated that baicalin was protective for rat cardiomyocytes through downregulating H/R-induced injury (60). It was demonstrated by Kong et al. that baicalin reduced I/R damage in the heart by its antioxidant and paracrine effects (61). Luan et al. demonstrated that by regulating the Akt/NF-κB signaling pathway, baicalin downregulated myocardial apoptosis and inflammation (62). Liu et al. reported that LV functions were improved and myocardial apoptosis was suppressed by baicalin via suppressing the CaSR/ERK1/2 signaling pathway in myocardial I/R injury rats (63). Xu et al. reported the protective effect of baicalin, via the JAK/STAT pathway, on myocardial I/R injury. In addition, baicalin reduced cardiomyocytes damage, downregulated cell death caused by I/R, and inhibited inflammation response in the heart by interfering with macrophages (64).
Resveratrol
The natural compound resveratrol was mainly extracted in fruits, such as peanut, grape, and berry. It has been demonstrated that resveratrol downregulates the pathological progression in many disease models, such as cancer or diabetes mellitus (65–67). Currently, resveratrol has been demonstrated to carry a potentially cardioprotective property against myocardial I/R injury via regulating inflammatory, angiogenesis, energy metabolism, mitochondrial function, and cardiomyocyte apoptosis (68, 69). Compared with vehicles, resveratrol significantly reduced the size heart infarction area in small animal studies both in vivo and ex vivo. Neither the reperfusion time nor the route of administration affects the effects of resveratrol (70). Resveratrol also exerts protection on myocardial post-I/R damage through inhibiting stromal interaction molecule1 (STIM1)-mediated store-operated Ca2+ accumulation (71), upregulating of Beclin-1 and LC-3II expression to induce autophagy (34) and regulating phosphorylation levels of proteins relative to the PI3K/Akt/e-NOS pathway (72). Concluding from the available data, resveratrol presents a significant limiting effect against myocardial I/R damage.
Polydatin
Polydatin, isolated from Reynoutria japonica Houtt., is another monocrystalline compound like resveratrol. The difference between them is at position C-3, where polydatin has the substitution of a glucoside group instead of a hydroxy group (73, 74). Polydatin exerts several pharmacological properties, such as anti-inflammatory, antioxidant (75), and alleviation of cardiac remodeling induced by pressure overload (74). Ling et al. reported that this compound aggravated autophagy and inhibited cell death during I/R or H/R, and co-treatment with adenovirus carrying short hairpin RNA for Beclin 1 and 3-MA, an autophagic inhibitor, would reverse this effect. Polydatin-treated mice showed a significantly reduced IS in heart tissue and a better heart function, compared with vehicle-treated mice, whereas these effects could be partly antagonized by 3-methyladenine (3-MA). These findings showed that polydatin treatment after infarction lowered myocardial I/R damage by enhancing autophagy to clear impaired mitochondria and to downregulate ROS and apoptosis (76).
Salvianolic Acid B
Salvianolic acid B (Sal B), derived from Salvia miltiorrhiza Bunge., is a water-soluble compound (77). Sal B exerts multiple effects, such as reducing inflammatory factor expression, inhibiting cell death, and alleviating oxidative stress (78, 79). Former evidence has shown that Sal B can alleviate oxidative stress, modulate calcium overload, promote endothelial function, stabilize mitochondrial membrane potential, and upregulate microRNA-30 (80), making it protective against myocardial I/R injury. A recent study has revealed that Sal B could alleviate myocardial I/R damage dose-dependently, promote cardiac function, decrease myocardial infarction size, reduce myocardial injury marker expression, inhibit inflammatory responses, increase PI3K/Akt expression, and decrease high-mobility group box protein 1(HMGB1) expression. The mechanism is that Sal B ameliorated myocardial I/R damage by promoting PI3K/Akt and decreasing the release of HMGB1 in rats (81).
6-Gingerol
6-Gingerol (6-G), a main component of gingerols and a phenolic compound isolated from Curcuma longa L., exerts antioxidative, antiapoptotic, and anti-inflammatory effects (82). Sampath et al. demonstrated that 6-G could prevent atherosclerosis via reducing cell death caused by excess oxidative stress (83). El-Bakly et al. found 6-G significantly protected cardiomyocytes by inhibiting cell death via alleviating oxidative stress and doxorubicin-induced myocardial damage (84). Lv et al. reported that pretreating with 6-G remarkably promoted cardiac function and decreased IS and I/R-induced creatine kinase-MB levels. 6-G alleviates myocardial I/R injury by reducing I/R-induced cardiomyocyte cell death and upregulating the PI3K/Akt signaling pathway. This evidence proved that 6-G may be a candidate drug for alleviating myocardial I/R injury (85).
Oleuropein
Oleuropein, a glycoside compound, is of antispasmodic effects, and can also reverse arrhythmia. In the rabbit isolated heart, it increases the coronary blood flow by 50% (86). Oleuropein can also lower blood pressure as it strongly inhibits the angiotensin-converting enzyme, as a result of its highly reactive 2,3-dihydroxy glutaraldehyde structure. A study reported that oleuropein protected the heart from myocardial I/R injury. In a myocardial I/R rat model, oleuropein reduced CK-MB and lactate dehydrogenase (LDH) levels as well as infarction size in the heart. Oleuropein also inhibited the caspase-3 pathway and reduced p53, p-IκBα protein, p-extracellular signal-regulated protein kinase (ERK), and phosphorylated (p)-mitogen-activated protein kinase kinase (MEK) expression. This evidence proved that by regulating the MEK/ERK/STAT3 signaling pathway, oleuropein inhibits myocardial I/R in rats (86).
Calycosin-7-O-β-D-Glucoside
Calycosin-7-O-β-D-glucoside (CG) is a major isoflavone extracted from Astragalus mongholicus Bunge, which has been proved to exert anti-inflammatory (87) and antioxidant abilities (88). Studies demonstrated that CG could decrease the size of cerebral infarction in the process of cerebral I/R injury, maintain the stability of the blood-brain barrier, and reduce the I/R-induced neuronal injury (89). In vitro and in vivo experiments showed that CG activated the JAK2/STAT3 pathway and upregulated the secretion of IL-10, and, therefore, can protect cardiomyocytes from I/R-induced cell death (90).
Puerarin
Puerarin (7,4'-dihydroxy-8-C-glucosylisoflavone) is an isoflavone of broad pharmacological abilities (91), including treating cardiovascular and cerebrovascular diseases, which can be a potential drug in alleviating I/R injury (92). Puerarin lowers the lipid peroxidation level, and aldose reductase activity decreases superoxide ion radicals and protects endothelial cells (93). Studies showed that puerarin remarkably shrinks the myocardial infarction size and increased pressure in the left ventricular in rats with diabetes mellitus suffering from myocardial I/R. Puerarin significantly reduced oxidative stress, inflammation, and NF-κB protein expression. Furthermore, puerarin raised the levels of VEGFA and Ang-I, as well as increased nitric oxide (NO) production, caspase-3 activity, and phosphorylated-endothelial NO synthase protein expression. These findings illustrated that puerarin protected cardiomyocytes and served to reduce myocardial I/R damage (94).
Hesperidin
Widely found in citrus fruits, hesperidin is a flavanone glycoside with a molecular formula of C28H34O15 and a molecular weight of 610.57 Da (95). Hesperidin has been found to possess broad biological effects, including antioxidant, anti-cancer, radio-protective, anti-inflammatory, and anti-allergic, properties (96–99). Gandhi et al. reported that hesperidin reduced arrhythmias and apoptosis caused in myocardial I/R injury, also reduced inflammation and oxidative stress, decreased excessive autophagy, and promoted the PI3K/Akt/mTOR pathway (31, 100).
Luteolin
Luteolin is a flavone widely presented in several plants. Former experiments reported that Lut protected the cardiomyocytes from I/R damage by decreasing microRNA-208b-3p expression and inhibiting the PI3K/Akt pathway (101, 102), and partly reversing the low expression and activity of SERCA2a in the injured area (103). Other evidence demonstrated that it modulated SERCA2a by SUMOylation at lysine 585 (104). These studies demonstrated that luteolin prevents the heart from suffering from I/R injury.
Honokiol
Honokiol (HKL) is isolated from Magnolia Officinalis Rehder and E.H. Wilson, which has long been used as a herb in traditional Chinese medicine. It is known for its effect of treating various vascular diseases, including ischemia and infarction (105). HKL was reported to be able to alleviate cerebral I/R injury via relieving oxidative stress and downregulating inflammatory reaction (106). Early evidence also proved that HKL could limit the infarct area and reduce arrhythmia in rats with AMI (107), in which its antioxidative and antiapoptotic abilities played critical roles. What is more, HKL could also regulate the SIRT1/Nrf2 signaling pathway, which was also important for its cardioprotective effects (108). Tan et al. demonstrated that post-treating with HKL reduced myocardial I/R injury and promoted autophagic flux in C57BL/6 mice (109).
Tournefolic Acid B
Tournefolic acid B (TAB) is a newly discovered compound isolated from Clinopodium chinense (Benth.) Kuntze, a traditional Chinese herbal medicine with modern pharmacological effects, such as anti-inflammatory, antitumor, antiradiation, and lowering blood glucose. Yu et al. reported that TAB significantly prevented the heart from being damaged by I/R injuries by suppressing ER stress and oxidative stress through inhibiting PI3K/AKT pathways. In vitro and ex vivo experiments, both supported this conclusion, meaning that TAB likely inhibited cell apoptosis by resisting oxidation-endoplasmic reticulum stress via activating the PI3K/AKT pathway (110).
Orientin
Orientin, one of the major active flavonoids of Persicaria orientalis (L.) Spach, is a traditional Chinese herb. It was reported to exert broad pharmacological properties, including anti-oxidant, anti-inflammation, and anti-apoptosis (111). Former experiments have demonstrated that orientin protected myocardium from I/R damage probably by reducing cell death (112). Evidence showed that the protective effect of orientin against myocardial I/R damage is partly regulated through subtle induction of autophagy, which involves the AMPK-mTORC1 signaling pathway and the phosphorylation of Beclin 1/Bcl-2 interaction in ER (113).
Icariin
Icariin, a natural flavonoid glucoside, is of broad pharmacological properties (114). Studies proved that icariin had antioxidant, antidepressant, anti-inflammatory, neuroprotective, and male sexual function improvement effects in vitro (115–119). In congestive heart failure rats, icariin promoted left ventricular function and attenuated cardiac remodeling via down-regulating matrix metalloproteinase-2 and−9 activity and inhibited cardiomyocyte death (119). Previous experiments have proved that myocardial function was protected by icariin from myocardial I/R damage in rats. It reduced IS, decreased I/R injury, and inhibited its remodeling. These properties of icariin are associated with lower blood indicators CK, IMA, and LDH levels in the serum and upregulated PI3K/Akt/eNOS pathway in rats' ischemic tissue, making it a candidate drug for preventing and resisting I/R injury in the early stage (120).
Curcumin
Curcumin [1,7-bis(4-hydroxy-3-methoxyphenyl)-1,6-heptadiene-3,5-dione], a natural compound isolated from the roots of Curcuma longa L., exerts wide pharmacological activities, including antioxidant, anti-inflammatory, and anticarcinogenic abilities in several rodent models. Previous experiments have suggested that curcumin is protective against some cardiovascular pathological conditions leading to heart failure (121, 122). Curcumin can improve heart function and ameliorate heart damage because it reduces oxidative stress and cell death, specifically by activating the phosphorylation of JAK2 and STAT3, increasing the myocardium Bcl-2/Bax expression and inhibiting caspase-3 (123).
Salvianolic Acid A
Salvianolic acid A is a water-soluble compound of Salvia miltiorrhiza Bunge, which is known to exert broad effects, including antioxidant, anticarcinogenic, anti-fibrotic, anti-inflammatory, and anti-platelet aggregation (124, 125). A previous study reported that, in diabetic rats, the JNK/PI3K/Akt signaling pathway correlated with myocardial I/R injury, and Sal A improved the recovery of heart function and prevented cell death following I/R damage in this model. This study provided critical evidence of the molecular mechanisms relating to Sal A's cardioprotective effects on I/R-injured diabetic rats (126).
Astilbin
Astilbin, a flavonoid compound extracted from the roots of Smilax china L., which has been long used in the traditional Chinese medicine clinical practice, has been found to have anti-hepatic, anti-arthritic, and anti-renal injury effects (127–129). Researchers have reported that in the early stages of STZ-induced diabetes rats, Astilbin resulted in a better heart function recovery caused by myocardial I/R damage via constraining inflammation and reducing HMGB1, phosphorylating NF-κB in ischemic myocardial tissue (130).
Eupatilin
Eupatilin (5,7-dihydroxy-3′,4′,6-trimethoxyflavone), which comes from the species of Artemisia plants, is a flavonoid of bioactive properties. Increasing studies have demonstrated that eupatilin exerts anti-allergic, anti-oxidant, anti-tumor, and anti-inflammatory activities (131–133). Experiments proved that eupatilin alleviated myocardial I/R damage via decreasing ROS and cell death by activating the Akt/glycogen synthase kinase-3β(GSK-3β) signaling pathway. Eupatilin is of therapeutic usage in treating myocardial I/R injury (27).
Syringic Acid
Syringic acid (SA), a natural O-methylated trihydroxy benzoic acid isolated from Dendrobium nobile Lindl., possesses broad biological activities, such as anti-oxidant, anti-tumor, and anti-inflammatory properties (134, 135). SA was found to prevent I/R injury. Tokmak et al. (136) reported that pretreating with SA in the spinal cord could reduce oxidative stress and neuronal degeneration induced by I/R. SA also ameliorated renal I/R injury (122). Liu et al. verified that SA exerted cardioprotective activities against myocardial I/R damage via activating the PI3K/Akt/GSK-3β signaling pathway and inhibiting the mitochondria-induced cell death (137).
Epigallocatechin-3-Gallate
Epigallocatechin-3-gallate (EGCG), the most widely studied catechin extracted from green tea leaves, was reported to reduce cardiovascular risk (138) through anti-inflammation and antioxidant activities, lowering serum cholesterol levels and reducing atherosclerosis (139, 140). Also, EGCG pretreatment limits IS caused by ischemia in the rat heart (141). Studies verified that giving rats EGCG together with reperfusion protected their hearts from regional myocardial I/R damage by activating pro-survival kinases, involving PI3K-Akt/GSK-3β and inhibiting cell death pathway p38 and JNK but not involving the ERK pathway (142).
Icariin
Icariin was found in Epimedium brevicornu Maxim., and it is a major compound of the herb Yin Yang Huo in traditional Chinese medicine. Its effects include anti-inflammation, antidepression, and antineoplastic properties. It also improves male sexual function, enhances bone healing, and protects neurons (143). Icariin postconditioning could attenuate myocardial I/R injury in the experimental rat model by activating the PI3K/Akt pathway and reducing cell death (144).
Troxerutin
Troxerutin, also known as vitamin P4, a derived natural bioflavonoid, owns wide biological properties including anti-oxidation and anti-inflammation (145). Pretreatment with this flavonoid extracted from Sophora japonica and Dimorphandra gardneriana (146) could decrease the occurrence of arrhythmias induced by I/R in diabetic and healthy rat hearts. Studies proved that the possible mechanism of its cardioprotective abilities may be the downregulating of inflammatory cytokines and inflammatory reactions in the heart (147).
Tilianin
Tilianin proves effective in upregulating NO synthase expression and NO production. It also acts as an anti-inflammatory ingredient (148). A study demonstrated that Tilianin exerted a significant protective effect on myocardial I/R-injured rat hearts (149). Studies verified that Tilianin pretreatment ameliorated the myocardial infarction and I/R damage in rats via the preservation of mitochondrial functions. The underlying mechanisms of Tilianin's cardioprotective activities may be mitochondrial preservation and cell apoptosis inhibition (150).
Isoquercitrin
Isoquercitrin is a natural compound present in vegetables, herbs, and flowers (151). It has been discovered that isoquercitrin reduces inflammatory, allergic reactions, and oxidative stress. (152). Isoquercetin was reported to reserve mitochondrial function and inhibit Cyt release induced by I/R injury in H9C2 cells (153). These findings verified that isoquercetin can be a candidate drug with cardiovascular protective effects in the treatment of myocardial I/R injury.
Vitexin
Vitexin (apigenin-8-C-β-D-glucopyranoside) is a flavonoid derived from Acer rubrum L., Anthurium andraeanum, and Cucumis sativus L. (154). Early studies have shown the hypotensive property and anti-inflammatory ability of vitexin. Recent pieces of research demonstrated vitexin's potential application for treating diseases like cancer (155). In vivo studies verified that vitexin was protective against myocardial I/R damage in rat heart, of which the mechanism could be related to its antioxidation ability and lowering of the levels of inflammatory cytokines by inhibiting the expression of NF-κB and TNF-α, as well as the upregulating phospho-ERK and downregulating phospho-c-Jun expression (156).
Apigenin
As a member of the non-mutagenic flavone subclass, Apigenin, isolated from the leaves of Apium graveolens L., exhibits low levels of toxicity. Previous studies have revealed Api's broad bioactive effects, including antiviral, antibacterial, anti-carcinogenic, antioxidant, and anti-inflammatory (157). Studies showed that Api could inhibit the p38 MAPK signaling pathway to protect the myocardium from I/R damage (158).
Bauhinia Championii Flavone
Bauhinia championii flavone (BCF) is extracted from the stem of Phanera championii Benth., of which the extract promotes blood circulation, reduces inflammatory and oxidative stress, and prevents platelet aggregation (159). Jian et al. reported BCF's protective properties of myocardium suffering from I/R damage in rats. The underlying mechanisms may depend on its ability to inhibit lipid peroxidation and activate the anti-oxidative system, its anti-inflammatory property by downregulating inflammatory levels by inhibiting signaling pathways, such as TLR4/NF-κB. It could also inhibit Bax/Bcl-2 ratios and caspase-3 activation (160).
Gastrodin
The phenolic glycoside gastrodin (GAS) is a monomeric component derived from Gastrodia elata Blume and has a variety of properties. It has long been used in treating cerebrovascular and cardiovascular diseases (161). Previous studies have demonstrated that GAS could reduce oxidative stress, lower inflammatory levels, and elevate hypoxia tolerance (162). Neighboring mitochondria and cardiomyocytes could be protected by the pretreatment of GAS via promoting autophagic flux and eliminating dysfunctional mitochondria (163).
Pinocembrin
The flavonoid pinocembrin, mainly found in Propolis, possesses antibacterial, anti-oxidant, and anti-inflammatory properties (164, 165). Pretreating with pinocembrin reduced cardiac arrhythmia in I/R rats through the enhancement of Na+-K+-ATPase and Ca2+-Mg2+-ATPase and the upregulation of Cx43 and Kir2.1 protein expression levels. Via upregulating gap junction- or ion channel-related gene or protein expression, cellular gap junction connexin function and IK1 current were restored, and cardiac arrhythmia was suppressed by correcting the P-R intermittent period, QRS duration, intracellular transmission velocity (166).
Silibinin
Silibinin, a polyphenolic flavonoid, is the main active component extracted from the seed of silybum marianum (milk thistle) or artichoke (cynara scolymus) (167). Previous studies have reported silibinin confers protective advantage in improving both liver and cerebral function after I/R, which raises concern about the role of silibinin against reperfusion injury in other tissues, especially in myocardium (168, 169). Chen et al. demonstrated silibinin reduces cardiomyocytes apoptosis, attenuates mitochondrial impairment and endoplasmic reticulum (ER) stress, alleviates ROS generation, neutrophil infiltration, and cytokines release (170). Furthermore, silibinin plus BAY 11-7082 (a selected NF-κB inhibitor) do not provide incremental benefits in improving myocytes apoptosis, oxidative stress, and inflammation in comparison with NF-κB signaling inhibition only. Thus, silibinin could prevent myocardial I/R injury by inhibiting cardiomyocytes apoptosis, reducing ER stress and oxidative stress, and modulating inflammatory response via deactivation of the NF-κB signaling pathway.
Saponins
Saponins are known as surface-active compounds that are widely distributed in the plant kingdom (171). They comprise a non-polar aglycone or non-saccharide moiety, coupled with polar mono or oligosaccharides. Saponins mainly include four-ring triterpene saponins and five-ring triterpene saponins. In recent years, many studies have shown that the saponins extracted from herbal are great protective of myocardial I/R injury in vivo and in vitro. The mechanisms are diverse and mainly involve regulating energy metabolism and calcium homeostasis, and inhibiting oxidative stress and inflammation (172).
Polyphyllin I
Polyphyllin I (PPI) is a steroidal saponin extracted from the roots of Paris polyphylla. PPI's anti-cancer effect via inhibiting the proliferation and growth of tumor cells has been proved in previous studies, making it an anti-cancer drug candidate (173–175). A recent study has shown that PPI could prevent myocardial I/R injury, decrease myocardial death, and reduce the inflammation response and oxidative stress after I/R. Also, PPI activates NF-κB p65. Therefore, it can be deduced that PPI is protective of myocardial I/R injury in rats (176).
Ginsenoside Rb1
Ginseng (Panax ginseng C.A.Mey.) can improve the immune system (177). In many cases, Ginsenoside Rb1 (GRb1) represents ginseng saponins of Panax ginseng C. A. Mey. Recent animal studies have shown that GRb1 is protective of many myocardial diseases, including myocardial I/R injury (178). In vivo and ex vivo studies have demonstrated GRb1 reduced myocardial I/R injury, and the underlying mechanism is by reducing both CK-MB and Trop l levels after I/R. GRb1 improves cardiac function as well as increases Bcl-2 expression by activating the phosphorylation of mTOR, inhibiting apoptosis-related proteins Bax and cleaved-caspase 3 (25).
Gypenoside
Chinese doctors have been using G. pentaphyllum [Gynostemma pentaphyllum (Thunb.) Makino] to treat diseases for hundreds of years (179). The main component of G. pentaphyllum is gypenoside (GP), which is known for its antitumor, anti-inflammatory, and anti-oxidative effects (180, 181). To our interest, GP also exhibits protective property against I/R injury. Qi et al. proved that GP protected I/R-injured cerebral neuronal (182). Chang et al. reported the mechanism of this protective effect, which is by lowering the miR-143-3p level through the activation of the AMPK/Foxo1 pathway. The changes in signaling pathways eventually resulted in the improving condition of I/R-induced OGD/R in H9c2 cells in rat myocardial tissue. No studies before had unveiled gypenosides' effect on miR functions, which means this new finding could be used as a novel strategy for myocardial I/R injuries (183).
Astragaloside IV
As one of the main active components extracted from Astragalus membranaceus Bunge., the lanolin alcohol-shaped Astragaloside IV (AS-IV) is a tetracyclic triterpenoid saponin with high polarity, which has antiapoptotic effects. It has been demonstrated that AS-IV possessed anti-ischemic properties against cerebral I/R injury, pulmonary and cardiovascular disease, diabetic nephropathy, and liver fibrosis (184, 185). The pharmacologic effects of AS-IV include regulating the calcium balance, antioxidative stress, anti-inflammatory, anti-apoptosis, antifibrotic, anti-diabetes, immunoregulation, and cardioprotective effect via different signaling pathways (184–186). A review of AS-IV on animal studies demonstrated that AS-IV's cardioprotective effect of antioxidant, anti-apoptosis, and anti-inflammatory in acute myocardial I/R injury depended largely on improving the circulation and upregulation of angiogenesis (187). A previous study reported that AsIV treatment attenuated myocardial I/R injury via inhibition of Toll-like receptor 4- and nuclear factor-κB-mediated inflammatory responses and subsequent myocardial apoptosis in a rat model (188). Further study demonstrated that AsIV treatment attenuated myocardial injury, reduced cardiomyocyte apoptosis, decreased [Ca2+]i, inhibited CaSR expression, and increased ERK1/2 phosphorylation levels. These findings not only provided the underlying mechanisms of the cardioprotective effect of AsIV but also further demonstrated the pivotal role of CaSR in myocardial I/R injury (189).
Ginsenoside Rg3
Ginsenoside Rg3 can improve cardiac functions by mitochondria dynamic remodeling and increasing the number of mitochondria (190). It can also attenuate myocardial I/R injury by regulating Akt/endothelial NO synthase (191). Ginsenoside Rg3 exhibits anti-apoptosis and anti-inflammation properties, which is the underlying mechanism of heart function impairment induced by I/R (192).
Ginsenoside Rb3
Ginsenoside Rb3, a component isolated from Panax ginseng (Panax ginseng C. A. Meyer), is drawing increasing attention in the treatment of cardiovascular diseases, including myocardial I/R injury. It has been found out that ginsenoside Rb3 exhibited a protective effect on neurons on the I/R injury model in vitro by inhibiting cell death and inflammatory cytokines (193). It was found by Ma et al. that ginsenoside Rb3's protective effect partly depended on inhibiting the NF-κB pathway, meaning that ginsenoside Rb3 can be a potential treatment for myocardial I/R injury (194).
Platycodin D
Platycodin D is among the main saponins of Platycodon grandiflorus (195). It possesses a variety of effects, including antiinflammation, anti-atherosclerotic, and anti-oxidant (196). Studies showed that Platycodin D could protect the heart from H/R-induced, Akt/Nrf2/HO-1 pathway-mediated oxidative stress, cell damage, as well as cell apoptosis (197).
Lignans
Lignans are a large class of natural compounds comprising two phenyl propane units. Lignans have been found rich in fruits, seeds, and vegetables, and received widespread interest due to their various biological activities, including antioxidant, antitumor, antibacterial, antiviral, insecticidal, fungistatic, estrogenic, and antiestrogenic activities (198).
Isovaleroylbinankadsurin A
Isovaleroylbinankadsurin A (ISBA) is a dibenzocyclooctadiene lignan extracted from Schisandra Chinensis (Turcz.) Baill. (199). ISBA possesses more than anti-inflammatory, anti-oxidant, and anti-tumor abilities (200, 201). It was reported that ISBA protected I/R-injured cardiomyocytes in models both in vitro and in vivo. Apoptosis induced by H/R injury was significantly inhibited via the mitochondrial-dependent pathway by ISBA. ISBA's protective effect on cardiomyocytes was mainly by activating the reperfusion injury salvage kinase (RISK) pathway. What is more, ISBA remarkably promoted the cellular anti-oxidative capacity by activating the RISK pathway, and, therefore, reduced oxidative damage induced by I/R injury by inhibiting the ROS generation, which proved ISBA's potential to be as a candidate drug for cardiovascular diseases (202).
Schisandrin B
Schisandrin B (Sch B) is also derived from the fruit of Schisandra chinensis (Turcz.) Baill., a common herb of traditional Chinese medicine (203). Sch B exerts hepatoprotective, anticancer, antioxidant, and antiinflammatory abilities (204). Zhang et al. reported that the pretreatment of Sch B on cardiomyocytes could decrease the size of the infarct area, promote the antioxidant ability, inhibit the ERS-induced apoptosis, and protect the myocardium (205).
Sauchinone
Sauchinone is also extracted from Schisandra Chinensis (Turcz.) Baill. (206), which protects cardiomyocytes from I/R injury in rats. Previous studies have shown it can inhibit p38, JNK, and other cell apoptosis signaling pathways (207).
Terpenes
Terpenes represent one of the largest groups of plant secondary metabolites, with ~55,000 different structures (208). Depending on the number of linked isoprene units, the resulting terpenes are classified into hemi-, mono-, sesqui-, di-, sester-, tri-, sesquar-, tetra-, and polyterpenes. For many decades, it has been suggested that terpenes and terpenoids are potential chemopreventive and therapeutic agents for various diseases (209).
Glaucocalyxin A
Glaucocalyxin A (GLA) is derived from Isodon japonicus var. glaucocalyx (Maxim.). H.W.Li exerts wide bioactive effects, including inhibiting platelet aggregation (210), suppressing the immune system, protecting DNA damage, and cytotoxic activity (211). Previous studies have demonstrated that GLA restored heart function, decreased infarction size, and inhibited apoptosis signaling pathways in mice hearts injured by myocardial I/R. These abilities of GLA may associate with its anti-platelet effect and the reduction of microvascular thrombosis. With decreased bleeding risk, GLA may be a potential therapy for alleviating myocardial RI during cardiac revascularization (212). Another study demonstrated that by activating the Akt/Nrf2/HO-1 pathway, GLA protected H9c2 cells against H/R-induced damage. Therefore, GLA might be a candidate drug for preventing and treating MI (213).
Artemisinin
Artemisinin, isolated mainly from Artemisia annua L., is a sesquiterpene lactone compound with a peroxisome bridging group structure. Recent pieces of research have reported that it was not only anti-malaria but also an anti-tumor agent. Artemisinin can promote cell death, block cell cycle, prevent angiogenesis, and tumor metastasis (214). Its protective effect against I/R injury is mainly due to the activation of the NLRP3 inflammasome pathway, but preconditioning with artemisinin preconditioning could significantly suppress NLRP3 inflammasome activation. On the rat I/R injury model, artemisinin could reduce ROS and inflammation induced by I/R injury, therefore promoting myocardial recovery, including reducing the size of myocardial infarction and inhibiting cardiomyocyte apoptosis and autophagy (47).
Geniposide
Geniposide (C17H24O10, GP), one of the major components of the fruit of Gardenia jasminoides J. Ellis and is found in nearly 40 species of herbal plants, is also a well-studied iridoid glycoside (215). There have been a growing number of studies on the bioeffects of geniposide over the past few decades. It has been proved to be antidiabetic, antioxidant, antithrombotic, analgesic, hepatoprotective, neuroprotective, anti-inflammatory, antidepressant, cardioprotective, immune-regulatory, and antitumoral (216). It reduced the myocardial infarct area and apoptosis and promoted heart function. In vitro studies demonstrated, in H9c2 cells, GP enhanced the cell viability and prevented apoptosis during H/R. Both in vivo and in vitro experiments demonstrated that GP downregulated the expression of proteins related to autophagy and prevented autophagosome accumulation. Rapamycin administration could reverse these effects. In summary, GP protected cardiomyocytes from I/R damage and inhibited autophagy by activating AKT/mTOR signaling pathways (217).
Ginkgolide B
Isolated from the leaves of Ginkgo, Ginkgolide B (GB) is a diterpene lactone compound and has a strong effect on inhibiting platelet aggregation (218). Its anti-inflammatory, antioxidant, and anti-apoptotic properties have made it protective of stroke, both ischemic and hemorrhagic (219–221). In hydrogen peroxide-treated H9c2 cells, they pretreated with GB-activated PI3K/Akt/mTOR signaling pathway and upregulated the phosphorylation levels of Akt and mTOR and, therefore, inhibited cell apoptosis (222). Via activating the A20-dependent NF-κB signal pathway, GB could also ameliorate I/R-induced inflammatory damage both in vivo and in vitro (223). Thus, GB protected against myocardial I/R injury by inhibiting ER stress-induced apoptosis via the PI3K/AKT/mTOR signaling pathway. This finding suggests GB may be a promising therapy in treating I/R injury (224).
Araloside C
Araloside C is among the major triterpenoid compounds derived from A. elata and was found to significantly promote heart function (225). Araloside C was proved to protect the myocardium from I/R damage by inhibiting ROS generation as well as Ca2+ overload. It is demonstrated that such cardioprotective effect is due to its ability to combine with the Hsp90 protein and interact with the ATP/ADP-binding domain of Hsp90 (226).
Triptolide
Isolated from Tripterygium wilfordii Hook.f., triptolide possesses neuroprotective, anti-tumor, and anti-inflammatory abilities (227). Triptolide can alleviate cerebral and hepatic I/R injuries in experiments (228, 229), and it could promote heart condition and reduce inflammation and oxidative stress induced by I/R in rats. Such protective effects of the heart may relate to triptolide's influences on the Nrf2/HO-1 defense pathway (230).
Alkaloids
Alkaloids, a class of nitrogen-containing basic organic compounds found in nature, are varied and complex. Alkaloids are mainly plants, but some are also found in animals. Alkaloids have an extensive pharmacological function. These significant biological activities often play a therapeutic role in Chinese herbal medicine management.
Berberine
Derived from several medicinal plants, such as Coptis Chinensis Franch. and Berberis vulgaris L., berberine (BBR) is an alkaloid with broad bioactive effects and has been used for treating many diseases (231). It showed antiapoptotic and antiinflammatory abilities both in cell and animal experiments (232). Huang et al. reported it enhanced H/R-induced cell viability and reduced I/R-induced IS and autophagy in cardiomyocytes (233). Also, BBR decreased CK-MB, LDH, and cTnI serum levels by decreasing myocardial cell death and promoting mitochondrial functions (234). Besides, BBR protects neurons by modulating cell death (235). It protects the myocardium from myocardial I/R injury via promoting proliferation, attenuating apoptosis via the mitophagy-mediated HIF-1α/BNIP3 pathway (236).
Galanthamine
Galanthamine protects neurons via activating the cholinergic pathway in the heart to prevent ischemic injury (237) and is important in promoting heart fitness and limiting IS (238). A previous study reported that it reduced cardiac dysfunction and alleviated endoplasmic reticulum stress (ERS)-related cell death induced by I/R via downregulating the expression of CHOP, Cleaved caspase 12, and caspase 3, as well as upregulating the expression of CADD34 and BiP in rats. It could also mitigate I/R-induced myocardial fibrosis in rats by inhibiting the expression of α-SMA and Collagen I. It was demonstrated that the mechanism of its cardioprotective and apoptosis-inhibiting effects were suppressing AMPK/Nrf2 pathways (239).
Matrine
The quinolizidine alkaloid compound Matrine, extracted from Sophora flavescens Aiton, which has been used as a herb in China, possesses antivirus, antitumor, antiallergic, anti-inflammatory, and antifibrotic effects (240–243). It activates the JAK2/STAT3 pathway and the upregulation of HSP70 expression. Guo et al. reported that the compound remarkably increased cell viability suppressed by H/R by decreasing lactate dehydrogenase and inhibiting creatine kinase activity in vitro. Also, it was proven to reduce CK-MB and TnI levels in the blood and decrease the size of the infarcted area in the heart as well as the I/R-induced apoptotic index of cardiomyocytes in vivo. It alleviated myocardial I/R damage by increasing HSP70 expression via activating the JAK2/STAT3 signaling pathway (244).
Palmatine
Palmatine, a natural quaternary protoberberine in the class of isoquinoline alkaloids, possesses pharmacological effects, such as anti-inflammatory and antioxidant (245–247). A previous study demonstrated that it could protect cardiomyocytes from I/R damage in rats. Its possible mechanism is relieving oxidative stress and regulating inflammatory mediators (248).
Capsaicin
Capsaicin is extracted from capsicum plants, such as Capsicum annuum L. and is widely used in food, medicine, and pharmacy (249). Pretreating with this compound protects H9c2 cells from H/R injury by upregulating 14-3-3η expression, modulating Bcl-2 and Bax expression and activity, reducing ROS generation, limiting mPTP opening, inhibiting caspase-3 activity, and, ultimately, suppressing cardiomyocytes apoptosis (250).
Quinones
Quinones are mainly divided into four types: benzoquinone, naphthoquinone, phenanthraquinone, and anthraquinone. They naturally occur in bacteria, fungi, animals, and plants and have a variety of pharmacological effects. They are produced in organisms and are utilized as electron-transfer agents, pigments, and in defense mechanisms (251).
Sodium Tanshinone IIA Sulfonate
Among the derivatives of tanshinone, IIA is sodium tanshinone IIA sulfonate (STS), a major lipophilic constitute of Salvia miltiorrhiza Bge (252). Studies have shown that it was cardioprotective against several cardiovascular diseases and neuroprotective against neural dysfunction (253–255). Previous studies demonstrated that it also showed pharmacological actions including anti-oxidative stress and anti-inflammation (256, 257). The study provides some evidence that this compound was significantly protective in treating myocardial I/R injury in rats. Its antioxidant ability partly improves heart condition (258).
Shikonin
Shikonin is isolated from Lithospermum erythrorhizon Siebold and Zucc., which has been used for treating several inflammatories and infectious conditions (259). It has been reported that it exerted anti-inflammatory, antibacterial, antiviral, and antioxidant activities (260). The potential merits of pretreating it in H/R-induced cardiomyocyte apoptosis are partly regulated through activating the PI3K/Akt signaling pathway (261).
Polysaccharides
Polysaccharides are widely distributed as natural ingredients in vegetables and fruits. Several studies have shown that the polysaccharides improve cardiovascular diseases through various mechanisms, such as anti-oxidative stress, regulating metabolism, anti-inflammatory, anti-cancer, and immunity-booster properties (262).
Fucoidan
Fucoidan is a sulfated polysaccharide molecule that has been known for its anticancer abilities. It is isolated mainly from the cell wall of different species of brown algae (Phaeophyta), a varied group of organisms (263). Omata et al. reported that, in the rat myocardial I/R injury model, there is a limited myocardial-IS and inhibited neutrophil accumulation, and one of the possible mechanisms could be the blockade of P-selectin-mediated neutrophil rolling on the vessel wall (264). Li et al. reported fucoidan improved left ventricular systolic pressure (LVSP), left ventricular end-diastolic pressure (LVEDP), and the contractility index in the rat myocardial I/R injury model, and could regulate the inflammation response via HMGB1 and NF-κB inactivation in I/R-induced myocardial damage (265).
Carotenoids
Carotenoids are naturally found in the natural ingredients, particularly in fruits, vegetables, and algae. At present, more than 750 kinds of carotenoids have been identified, of which there are about 100 in edible foods. Carotenoids exhibit several biological and pharmaceutical benefits, such as anti-inflammatory, anti-cancer, and immunity-booster properties (266).
Lycopene
Lycopene is a natural compound whose antioxidant effects have been widely studied (267). Previous studies demonstrated that low levels of circulating lycopene are related to a higher risk of cardiovascular diseases (268, 269). It was proved that pretreatment with 1-μM lycopene before reoxygenation remarkably decreased cardiomyocytes apoptosis induced by H/R. Moreover, IV injection of 1-μM circulating lycopene significantly decreased the risk of MI during in vivo I/R in mice and effectively inhibited the oxidation of fatty acid and the activation of JNK signaling during reperfusion (270).
Retinol Palmitate
Retinol palmitate, an analog of vitamin A, exhibits effective peroxyl radical scavengers via suppressing peroxidation (271). It has been proved to exert the ability to promote neuronal differentiation, neural patterning, and axonal growth, making it potentially neuroprotective against cerebral I/R damage (272). It could also limit myocardial IS and inhibit cellular apoptosis via the downregulation of proapoptotic-related proteins expression and the upregulation of SOD-related proteins expression. Tao et al. suggested that pretreating with retinol palmitate effectively protected the heart from myocardial I/R injury through balancing intracellular oxidants and antioxidants (273).
Coumarin
Coumarin compounds represent an important type of naturally occurring and synthetic oxygen-containing heterocycles with a typical benzopyrone framework. This type of special benzopyrone structure enables its derivatives to readily interact with a diversity of enzymes and receptors in organisms through weak bond interactions, thereby exhibiting wide potentiality as medicinal drugs compounds, inclusive of analgesic, anticoagulant anti-inflammatory, antimicrobial, antineoplastic, antioxidant, and immunomodulatory effects (274).
Osthole
Osthole is a compound mainly derived from Cnidium monnieri (L.) Cusson and Angelica pubescens Maxim., and has been used as tonics and aphrodisiacs in clinical practice of traditional Chinese medicine for many years (275). Modern pharmacological studies demonstrated that it possessed antitumor, anti-hepatic, anti-allergic, anti-inflammatory, anti-apoptotic, and estrogen-like effects (276–278). Wang et al. reported that this compound was beneficial to functional recovery after myocardial I/R injury by increasing SOD, GPx, and CAT activities, and decreasing lipid peroxidation products, MDA, and 4-HNE in the damaged heart tissues. The mechanism behind these effects was related to decreasing the expression of the pro-inflammatory factors, increasing anti-inflammatory cytokines, as well as lowering HMGB1, phosphorylated IκB-α, and NF-κB proteins (279).
Esculetin
The natural coumarin compound Esculetin (6,7-dihydroxy coumarin) possesses antioxidant, anti-inflammatory, anti-nociceptive, and anti-tumor activities (280, 281). It also protects against I/R injury. In H/R-stimulated H9c2 cells, esculetin promotes cell viability and reduces lactate dehydrogenase (LDH) release. It also reduces ROS and cell death, following H/R injury through the JAK2/STAT3 pathway (282).
Others
Plantamajoside
Plantamajoside (PMS) is a phenylpropanoid glycoside extracted from Plantago Asiatica L. with a long history in food and medical application (283). PMS exhibits anti-inflammatory and antioxidant properties (284, 285). Because of its protective effects against cadmium-induced renal injury and its anti-inflammatory and antifibrotic effects, it has been used to treat many diseases (286, 287). In the in vitro I/R model, a previous study investigated the protective effects of PMS on H/R-stimulated oxidative stress, inflammation, and apoptosis in H9c2 cells. PMS attenuated myocardial I/R damage by reducing the inflammatory response, oxidative stress, and apoptosis through Akt/Nrf2/HO-1 and NF-κB signaling pathways (288).
Diallyl Trisulfide
Garlic (Allium sativum L.) has long before been recognized as beneficial for several diseases. One of its main bioactive compounds is diallyl trisulfide (DATS), also known as allitridin or 4,5,6-trithia-1,8-nonadiene. DATS is a natural, stable, and safe component that attracts H2S donors for in vivo studies with an eye to clinical relevance (289). Jeremic et al. reported that DATS consumption could improve heart functions and prevent the oxidative and histoarchitectural variation in the heart suffering from ex vivo induced I/R heart injury (290).
Eleutheroside E
Eleutheroside E (EE) is a bioactive component of Eleutherococcus senticosus (Rupr. and Maxim.) Maxim. It significantly alleviates physical fatigue and promotes endurance performance, protects against neuritic atrophy and neuron apoptosis, and inhibits inflammatory gene expression (291). Previous studies have shown that treating with EE remarkably limited H/R-induced damage in heart tissue by reducing oxidative stress, inactivating NF-κB, and modulating metabolic responses. Moreover, EE reprograms metabolic action. This evidence proved EE to be potentially valuable in treating H/R-injured heart tissue and emphasized the relationship between EE's protection and metabolic reprogramming (292).
Salidroside
Salidroside is a bioactive compound with anti-inflammatory, anti-cancer, anti-oxidant, and anti-fatigue effects (293, 294). A previous study demonstrated that the mechanism of Sal's protective effects against myocardial I/R damage was related to the inhibition of the TLR4/NF-κB signaling pathway, inflammatory response, and cardiomyocyte apoptosis (295).
Glycyrrhizin
Glycyrrhizin, one of the most effective ingredients of the root extraction of Glycyrrhiza glabra L., is consisted of glucuronic acid and glycyrrhetinic acid and possesses anti-allergic, anti-oxidant, anti-ulcer, anti-viral, anti-cancer, and immunomodulatory effects (296, 297). It also protects the liver and stabilizes the cell membrane. This compound has been broadly used in Europe and the Middle East (298). It was reported that, in rats, glycyrrhizin triggered HMGB1 and the blocked p38 and JNK pathways, ultimately reducing myocardial I/R damage by attenuating oxidative stress, iNOS, and inflammatory reactions in vivo (299).
Cornuside
Cornuside is a secoiridoid glucoside derived from the fruit of Cornus officinalis Siebold and Zucc., which has long been used for attenuating inflammation and promoting blood circulation. It has been found that the crude extract of this fruit had pharmacological effects including, anti-neoplasm, anti-sepsis, antiinflammatory, anti-diabetic nephropathy, and hepatoprotection effects (300, 301). It was reported that in rats suffering from myocardial I/R injury, cornuside decreased infarct volume, improved hemodynamics parameters, and alleviated myocardial injury via inhibiting PMN infiltration and MPO activity, decreased pro-inflammatory factors, and reduced phosphorylated IB- and NF-B proteins (302).
Phytochemicals and Signal Transduction Pathways
We systematically summarized the phytochemicals' characteristics for their cardioprotective mechanisms in preventing myocardial I/R injury from experimental studies (Table 1). Various internal mechanisms related to myocardial I/R injury that control the fate of cardiomyocytes by phytochemicals interventions are systematically summarized (Figure 2). Among several signal transduction pathways, NF-κB, PI3K/Akt, Nrf2/HO-1, JAK2/STAT, mTOR, and AMPK signaling pathways take an important position in the modulation of myocardial I/R injury by phytochemicals.
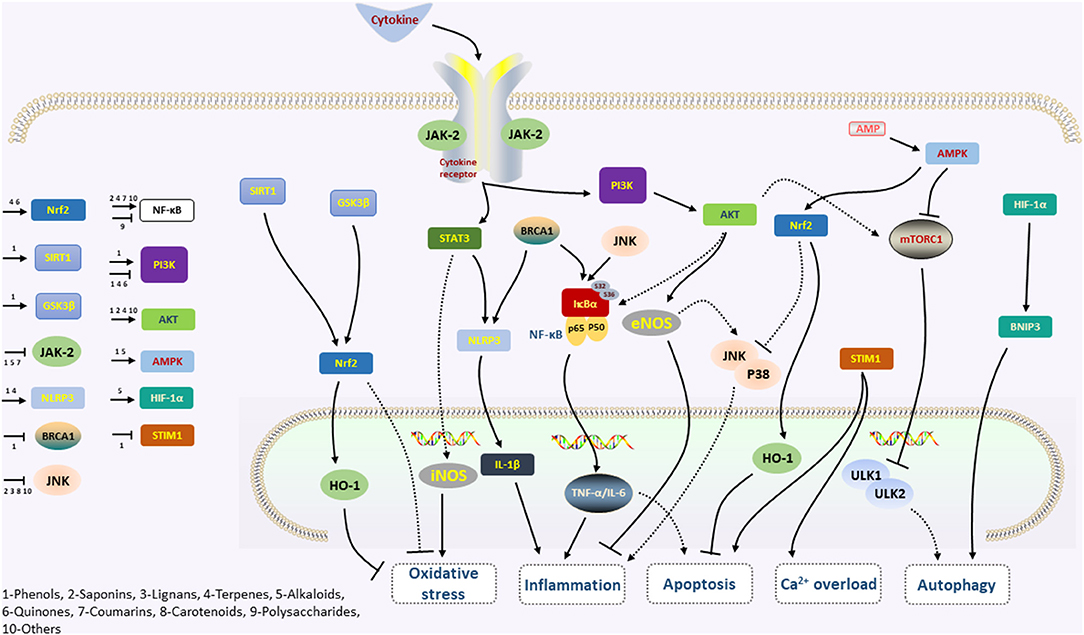
Figure 2. The simplified mechanism scheme of phytochemicals in cardiovascular disease. Phytochemicals reduce the phosphorylation of STAT3 by inhibiting JAK2, which is activated following the binding of cytokines and cognate receptors. Inhibition of the JAK/STAT pathway leads to decreasing of iNOS and NLRP3/IL-1β levels, and thus protects against oxidative stress and inflammation. Activation of the AMPK signaling pathway may also play a key role in the anti-inflammation, further acting on the mTOR and Nrf2 factors and participating in the actions of phytochemicals on oxidative stress, apoptosis, and autophagy. Moreover, the NF-κB signaling pathway, activated by the BRCA1, JNK, and AKT, promotes the expression of TNF-α and IL-6, which regulate inflammation and apoptosis. The phytochemicals are also against apoptosis and inhibit Ca2+ accumulation via the STIM1 pathway. The PI3K-AKT signaling pathway is activated by many types of cellular stimuli or toxic insults, activates downstream mTOR, eNOS, and NF-κB, and sequentially regulates the inflammation and apoptosis.
NF-κB Signaling Pathway
NF-κB (Nuclear Factor-kappa B) is composed of different transcription factors—the Rel family. The Rel/NF-κB family regulates immune and inflammatory responses. Activated NF-κB prevents ischemic injury and inhibits both inflammation and apoptosis (304). Paeonol significantly alleviates hypoxia and attenuates I/R injury in H9C2 cells through the BRCA1/ROS-regulated NF-κB/TNF-α/IL-6 pathways and NLRP3 inflammasome (44). Puerarin exerts a similar effect by suppressing NF-κB and upregulating VEGFA/Ang-1 in diabetic rats with myocardial I/R injury (94). Similarly, fisetin reduces ischemic injury and oxidative damage by inhibiting cytokines, such as IL-1β and TNF-α (305). Polyphenols modulate the immune system by inhibiting NF-κB (304).
PI3K/Akt Signaling Pathway
PI3K and the downstream target serine/threonine kinase Akt are crucial in various physiological processes. Activated PI3K/Akt signaling pathways is protective in myocardial I/R injury (306, 307). A study by Wang et al. suggests it is associated with H/R-induced cardiomyocyte apoptosis in Shikonin pretreated cells (261). Another study shows resveratrol inhibits I/R injury-induced cardiomyocyte apoptosis by regulating phosphorylation levels of PI3K/Akt/e-NOS pathway-related proteins (72). The PI3K/Akt signaling pathway regulates the life cycle of cardiomyocytes by regulating the morphology and function (308). 6-Gingerol possesses similar potent via this pathway (85).
Nrf2/HO-1 Signaling Pathway
Normally, Nrf2is a transcription factor that regulates the expression of several factors involved in the cellular defense against oxidative stress and inflammation, including heme oxygenase-1 (HO-1) (309). Once activated, it is stabilized and translocates to the nucleus, and binds antioxidant response element (ARE), which activates HO-1 (310). Numerous studies have shown the potential role of the Nrf2/HO-1 pathway in myocardial I/R injury (311). A study by Yu et al. found Nrf2 accumulated more in the nuclear due to triptolide in reperfused myocardium (230). Also, triptolide promoted the activity and expression of HO-1. This study proved triptolide was cardioprotective by activating the Nrf2/HO-1 defense pathway in treatments in I/R injuries (312). In addition, Zhou et al. demonstrated 160-nM triptolide pretreatment for a short period (<6 h) raised the levels of nuclear Nrf2 and HO-1 in H9c2 cardiomyocytes, but they are downregulated if pretreatment lasted for a longer period (> 9 h) (313). Glaucocalyxin A is also reported to increase cell viability and decrease oxidative stress in H9c2 cells, resulting in fewer cell death from H/R-stimulated oxidative damage. The protective effect of GLA is proved to be associated with the activation of the Akt/Nrf2/HO-1 signaling pathway (213).
JAK2/STAT Signaling Pathway
Several reports proposed that JAK/STAT signaling is associated with cardiac dysfunction in myocardial I/R injury (314). JAKs are rapidly recruited to the receptor and activated after the upstream receptor molecule, and then catalyze its tyrosine phosphorylation. This process supplies binding sites for the SH2 domain of STATs, ultimately leading to specific gene transcription. In particular, myocardial I/R injury activated JAK1, and JAK2, in turn, activates STAT1 and STAT3. STAT1 promotes apoptosis, while STAT3 protects cardiomyocyte (315). Ming Xu reported baicalin alleviated post-I/R myocardial injury and reduced inflammation via JAK/STAT pathway (64). CG pretreatment protected the myocardium against I/R injury by upregulating IL-10 expression (90). Matrine can attenuate myocardial I/R injury by upregulating HSP70, which can be activated by the JAK/STAT pathway (244).
MTOR Signaling Pathway
mTOR is a mammalian target of rapamycin (RAPA) and downregulates autophagy (316). Luo et al. found that GP upregulated p-mTORSer2448 expression and inhibited autophagy, but these effects were counteracted by RAPA. They also observed that RAPA enhanced p-AKTSer473 expression, which might be associated with the activation of upstream AKT by mTOR inhibition (217). However, RAPA's effects on activating autophagy were inconsistent in myocardial I/R injury. In myocardial I/R injury, GRb1's effects are also controversial. Some studies have shown that mTOR switched on I/R (317), whereas others tend to hold the opposite view. Li et al. proved p-mTOR to be in an inhibitory state in I/R injury. Remarkably, GRb1 treatment reversed the inhibitory state and activated it (25). P-mTOR changes are dynamic after myocardial cell injury, and this may account for the difference in the performance of mTOR in I/R across studies.
AMPK Signaling Pathway
AMPK regulates cell homeostasis and reprograms metabolism. Hou et al. reported Gal alleviated I/R-induced cardiac dysfunction, reduced ERS-related apoptosis, and inhibited myocardial fibrosis by suppressing AMPK/Nrf2 pathways (239). The relationship between the cardio-protective effect of GP depends on suppressing miR-143-3p via activating AMPK, which furthered the understanding by connecting their function with miRs (183).
Conclusion and Perspectives
To date, this review provides the most comprehensive overview of the current knowledge of phytochemicals that interfere with the myocardial I/R injury. Among the phytochemicals with potential anti-I/R injury ability, phenolic compounds take up the largest proportion (45.1%). Saponins, lignans, terpenes, alkaloids, quinones, coumarin, carotenoids, and other compounds make up the remainder, respectively. In addition, phytochemicals extensively modulated autophagy, oxidative stress, Ca2+ overload, apoptosis, inflammation, and key regulatory targets and proteases activities. From this point of view, phytochemicals may be a potential panacea for myocardial I/R injury treatment, and studies on their mechanisms rule out the possibility of applying a single molecule as a pathophysiological cause of myocardial I/R injury, while most natural products have more than one “target” and may affect multiple pathways.
Although phytochemicals found in natural products have made great progress in alleviating myocardial I/R injury, future studies focusing on human clinical trials of several potent phytochemicals and their combinations should be carried out. Theoretically, animal models help to explore the probable mechanism; however, there is still a huge anatomic and/or physiological gap between the different species, which may possibly be responsible for the inconsistency between preclinical studies and clinical studies currently (70). Therefore, more appropriate experimental models and precise pharmaceutical intervention studies are needed to simulate human heart physiology. Furthermore, phytochemicals must be investigated for the risk assessment and safety evaluation to observe any undesirable effects, which may hinder further use of phytochemicals as a cardioprotective adjuvant in the human body, as well as the enthusiasm for further pharmaceutical development. In addition, there may be a paradox that the cardio protection of phytochemicals is associated with inhibition of cell death, but it is an antineoplastic activity with the promotion of cell death (318). Cancer cells express different levels of apoptosis-promoting or inhibiting proteases compared to cardiomyocytes, which might partly explain these differences (318). Overall, phytochemicals may be a potential panacea for myocardial I/R injury treatment, but more research is needed to support this promising means of enhancing prognosis and, possibly, prevention.
Author Contributions
QL and JW contributed to study conception and design, contributed to final approval, and overall responsibility for this published work. CC, L-TY, and B-RC contributed to acquisition, analysis, and interpretation of data. J-LX, YC, J-LJ, R-LF, LX, X-YQ, DL, JL, YL, X-YC, J-JL, and KZ contributed to article revision. All the authors contributed to the article and approved the submitted version.
Funding
This work was supported by the Fundamental Research Funds for the Central Universities (No. 2019-JYB-TD-008), the National Natural Science Foundation of China (No. 81803906), and the special project of Research and Demonstration Application of Clinical Diagnosis and Treatment Technology of Beijing Science and Technology Plan (No. Z1910000006619070).
Conflict of Interest
The authors declare that the research was conducted in the absence of any commercial or financial relationships that could be construed as a potential conflict of interest.
Publisher's Note
All claims expressed in this article are solely those of the authors and do not necessarily represent those of their affiliated organizations, or those of the publisher, the editors and the reviewers. Any product that may be evaluated in this article, or claim that may be made by its manufacturer, is not guaranteed or endorsed by the publisher.
Abbreviations
AMI, acute myocardial infarction; STEMI, ST-elevation myocardial infarction; pPCI, primary percutaneous coronary intervention; IS, infarct size; I/R, ischemia-reperfusion; NADH+, nicotinamide adenine dinucleotide; ROS, reactive oxygen species; STAT3, signal transducer and activator of transcription 3; BCL-C, B-cell lymphoma-C; Bcl-xL, B-cell lymphoma-xL; mTOR, mammalian target of rapamycin; Cyt c, cytochrome c; Bcl-2, B-cell lymphoma-2; Bax, BCL2-associated X; H/R, hypoxia-reoxygenation; VSMC, vascular smooth muscle cell; 3-MA, 3-methyladenine; HMGB1, high-mobility group box protein 1; GSK-3β, glycogen synthase kinase-3β; PI3K, phosphatidylinositol-3-OH kinase; Akt, protein kinase b; ATP, adenosine triphosphate; NF-κB, nuclear factor kappa-light-chain-enhancer of activated B cells; BRCA1, breast cancer type 1 sensitive protein; NLRP3, nod-like receptor protein 3; TNF-α, tumor necrosis factor-α; IL-6, interleukin-6; Nrf2, nuclear factor-erythroid 2-related factor 2; STIM1, stromal interaction molecule1; Sal-B, salvianolatic acid B; 6-G, 6-Gingerol; CK-MB, creatine kinase-MB; LDH, lactate dehydrogenase; ERK, p-extracellular signal-regulated protein kinase; MEK, (p)-mitogen-activated protein kinase kinase; CG, Calycosin-7-O-β-D-glucoside; NO, nitric oxide; HKL, honokiol; TAB, tournefolic acid B; ER, endoplasmic reticulum; AMPK, adenosine monophosphate-activated protein kinase; JAK2, Janus kinase 2; SalA, salvinolic acid A; SA, salvinolic acids; EGCG, epigallocatechin-3-gallate; JNK, c-Jun N-terminal kinases; ERK, extracellular signal-regulated kinase; BCF, bauhinia championii flavone; GAS, gastrodin; AS-IV, astragaloside IV; ISBA, isovaleroylbinankadsurin A; RISK, reperfusion injury salvage kinase; GLA, glaucocalyxin A; GB, ginkgolide B; BBR, berberine; ERS, endoplasmic reticulum stress; SOD, superoxide dismutase; HSP70, heat shock proteins 70; PMS, plantamajoside; DATS, diallyl trisulfide; EE, eleutheroside E; TLR4, toll-like receptor 4; RAPA, rapamycin; SHR, spontaneous hypertension rat; TCM, traditional Chinese medicine.
References
1. Reed GW, Rossi JE, Cannon CP. Acute myocardial infarction. Lancet. (2017) 389:197–210. doi: 10.1016/S0140-6736(16)30677-8
2. Davidson SM, Adameová A, Barile L, Cabrera-Fuentes HA, Lazou A, Pagliaro P, et al. Mitochondrial and mitochondrial-independent pathways of myocardial cell death during ischaemia and reperfusion injury. J Cell Mol Med. (2020) 24:3795–806. doi: 10.1111/jcmm.15127
3. Szummer K, Wallentin L, Lindhagen L, Alfredsson J, Erlinge D, Held C, et al. Relations between implementation of new treatments and improved outcomes in patients with non-ST-elevation myocardial infarction during the last 20 years: experiences from SWEDEHEART registry 1995 to 2014. Eur Heart J. (2018) 39:3766–76. doi: 10.1093/eurheartj/ehy554
4. Montalescot G, van 't Hof AW, Lapostolle F, Silvain J, Lassen JF, Bolognese L, et al. Prehospital ticagrelor in ST-segment elevation myocardial infarction. N Engl J Med. (2014) 371:1016–27. doi: 10.1056/NEJMoa1407024
5. Bellis A, Di Gioia G, Mauro C, Mancusi C, Barbato E, Izzo R, et al. Reducing cardiac injury during ST-elevation myocardial infarction: a reasoned approach to a multitarget therapeutic strategy. J Clin Med. (2021) 10:2968. doi: 10.3390/jcm10132968
6. Davidson SM, Arjun S, Basalay MV, Bell RM, Bromage DI, Bøtker HE, et al. The 10th Biennial Hatter Cardiovascular Institute workshop: cellular protection-evaluating new directions in the setting of myocardial infarction, ischaemic stroke, and cardio-oncology. Basic Res Cardiol. (2018) 113:43. doi: 10.1007/s00395-018-0704-z
7. Heusch G. Myocardial ischaemia-reperfusion injury and cardioprotection in perspective. Nat Rev Cardiol. (2020) 17:773–89. doi: 10.1038/s41569-020-0403-y
8. Baines CP. How and when do myocytes die during ischemia and reperfusion: the late phase. J Cardiovasc Pharmacol Ther. (2011) 16:239–43. doi: 10.1177/1074248411407769
9. Kalogeris T, Baines CP, Krenz M, Korthuis RJ. Ischemia/reperfusion. Compr Physiol. (2016) 7:113–70. doi: 10.1002/cphy.c160006
10. Upadhyay S, Dixit M. Role of polyphenols and other phytochemicals on molecular signaling. Oxid Med Cell Longev. (2015) 2015:504253. doi: 10.1155/2015/504253
11. Probst YC, Guan VX, Kent K. Dietary phytochemical intake from foods and health outcomes: a systematic review protocol and preliminary scoping. BMJ Open. (2017) 7:e013337. doi: 10.1136/bmjopen-2016-013337
12. Zhang YJ, Gan RY, Li S, Zhou Y, Li AN, Xu DP, et al. Antioxidant phytochemicals for the prevention and treatment of chronic diseases. Molecules. (2015) 20:21138–56. doi: 10.3390/molecules201219753
13. González-Vallinas M, González-Castejón M, Rodríguez-Casado A, Ramírez de Molina A. Dietary phytochemicals in cancer prevention and therapy: a complementary approach with promising perspectives. Nutr Rev. (2013) 71:585–99. doi: 10.1111/nure.12051
14. Altemimi A, Lakhssassi N, Baharlouei A, Watson DG, Lightfoot DA. Phytochemicals: extraction, isolation, and identification of bioactive compounds from plant extracts. Plants. (2017) 6:42. doi: 10.3390/plants6040042
15. Ismaeel A, Greathouse KL, Newton N, Miserlis D, Papoutsi E, Smith RS, et al. Phytochemicals as therapeutic interventions in peripheral artery disease. Nutrients. (2021) 13:2143. doi: 10.3390/nu13072143
16. Hacioglu C, Kar F, Kara Y, Yucel E, Donmez DB, Sentürk H, et al. Comparative effects of metformin and Cistus laurifolius L. extract in streptozotocin-induced diabetic rat model: oxidative, inflammatory, apoptotic, and histopathological analyzes. Environ Sci Pollut Res Int. (2021) 28:57888–901. doi: 10.1007/s11356-021-14780-y
17. Mokhtari-Zaer A, Marefati N, Atkin SL, Butler AE, Sahebkar A. The protective role of curcumin in myocardial ischemia-reperfusion injury. J Cell Physiol. (2018) 234:214–22. doi: 10.1002/jcp.26848
18. Gunata M, Parlakpinar H. A review of myocardial ischaemia/reperfusion injury: Pathophysiology, experimental models, biomarkers, genetics and pharmacological treatment. Cell Biochem Funct. (2020) 39:190–217. doi: 10.1002/cbf.3587
19. Gustafsson AB, Gottlieb RA. Autophagy in ischemic heart disease. Circ Res. (2009) 104:150–8. doi: 10.1161/CIRCRESAHA.108.187427
20. Gu S, Li X. Regulation of Autophagy in Cardiovascular Diseases by Natural Products. Adv Exp Med Biol. (2020) 1207:731–6. doi: 10.1007/978-981-15-4272-5_55
21. Enomoto D, Obana M, Miyawaki A, Maeda M, Nakayama H, Fujio Y. Cardiac-specific ablation of the STAT3 gene in the subacute phase of myocardial infarction exacerbated cardiac remodeling. Am J Physiol Heart Circ Physiol. (2015) 309:H471–80. doi: 10.1152/ajpheart.00730.2014
22. Jeong SY, Seol DW. The role of mitochondria in apoptosis. BMB Rep. (2008) 41:11–22. doi: 10.5483/BMBRep.2008.41.1.011
23. Timmers L, Henriques JP, de Kleijn DP, Devries JH, Kemperman H, Steendijk P, et al. Exenatide reduces infarct size and improves cardiac function in a porcine model of ischemia and reperfusion injury. J Am Coll Cardiol. (2009) 53:501–10. doi: 10.1016/j.jacc.2008.10.033
24. Hietakangas V, Cohen SM. Regulation of tissue growth through nutrient sensing. Annu Rev Genet. (2009) 43:389–410. doi: 10.1146/annurev-genet-102108-134815
25. Li CY, Yang P, Jiang YL, Lin Z, Pu YW, Xie LQ, et al. Ginsenoside Rb1 attenuates cardiomyocyte apoptosis induced by myocardial ischemia reperfusion injury through mTOR signal pathway. Biomed Pharmacother. (2020) 125:109913. doi: 10.1016/j.biopha.2020.109913
26. Zhang W, Xing B, Yang L, Shi J, Zhou X. Icaritin Attenuates Myocardial Ischemia and Reperfusion Injury Via Anti-Inflammatory and Anti-Oxidative Stress Effects in Rats. Am J Chin Med. (2015) 43:1083–97. doi: 10.1142/S0192415X15500627
27. Qiao Z, Xu YW, Yang J. Eupatilin inhibits the apoptosis in H9c2 cardiomyocytes via the Akt/GSK-3β pathway following hypoxia/reoxygenation injury. Biomed Pharmacother. (2016) 82:373–8. doi: 10.1016/j.biopha.2016.05.026
28. Ma X, Liu H, Foyil SR, Godar RJ, Weinheimer CJ, Hill JA, et al. Impaired autophagosome clearance contributes to cardiomyocyte death in ischemia/reperfusion injury. Circulation. (2012) 125:3170–81. doi: 10.1161/CIRCULATIONAHA.111.041814
29. Matsui Y, Takagi H, Qu X, Abdellatif M, Sakoda H, Asano T, et al. Distinct roles of autophagy in the heart during ischemia and reperfusion: roles of AMP-activated protein kinase and Beclin 1 in mediating autophagy. Circ Res. (2007) 100:914–22. doi: 10.1161/01.RES.0000261924.76669.36
30. Valentim L, Laurence KM, Townsend PA, Carroll CJ, Soond S, Scarabelli TM, et al. Urocortin inhibits Beclin1-mediated autophagic cell death in cardiac myocytes exposed to ischaemia/reperfusion injury. J Mol Cell Cardiol. (2006) 40:846–52. doi: 10.1016/j.yjmcc.2006.03.428
31. Li X, Hu X, Wang J, Xu W, Yi C, Ma R, et al. Inhibition of autophagy via activation of PI3K/Akt/mTOR pathway contributes to the protection of hesperidin against myocardial ischemia/reperfusion injury. Int J Mol Med. (2018) 42:1917–24. doi: 10.3892/ijmm.2018.3794
32. Decker RS, Wildenthal K. Lysosomal alterations in hypoxic and reoxygenated hearts. I. Ultrastructural and cytochemical changes. Am J Pathol. (1980) 98:425–44.
33. Yan L, Vatner DE, Kim SJ, Ge H, Masurekar M, Massover WH, et al. Autophagy in chronically ischemic myocardium. Proc Natl Acad Sci USA. (2005) 102:13807–12. doi: 10.1073/pnas.0506843102
34. Qu X, Chen X, Shi Q, Wang X, Wang D, Yang L. Resveratrol alleviates ischemia/reperfusion injury of diabetic myocardium via inducing autophagy. Exp Ther Med. (2019) 18:2719–25. doi: 10.3892/etm.2019.7846
35. Neri M, Riezzo I, Pascale N, Pomara C, Turillazzi E. Ischemia/Reperfusion Injury following Acute Myocardial Infarction: A Critical Issue for Clinicians and Forensic Pathologists. Mediators Inflamm. (2017) 2017:7018393. doi: 10.1155/2017/7018393
36. Moldoveanu T, Hosfield CM, Lim D, Elce JS, Jia Z, Davies PL. A Ca(2+) switch aligns the active site of calpain. Cell. (2002) 108:649–60. doi: 10.1016/S0092-8674(02)00659-1
37. Neuhof C, Neuhof H. Calpain system and its involvement in myocardial ischemia and reperfusion injury. World J Cardiol. (2014) 6:638–52. doi: 10.4330/wjc.v6.i7.638
38. Seki S, Horikoshi K, Takeda H, Izumi T, Nagata A, Okumura H, et al. Effects of sustained low-flow ischemia and reperfusion on Ca2+ transients and contractility in perfused rat hearts. Mol Cell Biochem. (2001) 216:111–9. doi: 10.1023/A:1011067529272
39. Granger DN, Kvietys PR. Reperfusion injury and reactive oxygen species: The evolution of a concept. Redox Biol. (2015) 6:524–51. doi: 10.1016/j.redox.2015.08.020
40. Kubes P, Granger DN. Leukocyte-endothelial cell interactions evoked by mast cells. Cardiovasc Res. (1996) 32:699–708. doi: 10.1016/S0008-6363(96)00118-6
41. Calvert JW, Jha S, Gundewar S, Elrod JW, Ramachandran A, Pattillo CB, et al. Hydrogen sulfide mediates cardioprotection through Nrf2 signaling. Circ Res. (2009) 105:365–74. doi: 10.1161/CIRCRESAHA.109.199919
42. Nahrendorf M, Pittet MJ, Swirski FK. Monocytes: protagonists of infarct inflammation and repair after myocardial infarction. Circulation. (2010) 121:2437–45. doi: 10.1161/CIRCULATIONAHA.109.916346
43. Swirski FK, Nahrendorf M. Leukocyte behavior in atherosclerosis, myocardial infarction, and heart failure. Science. (2013) 339:161–6. doi: 10.1126/science.1230719
44. Zheng J, Mao Z, Zhang J, Jiang L, Wang N. Paeonol pretreatment attenuates anoxia-reoxygenation induced injury in cardiac myocytes via a BRCA1 dependent pathway. Chem Pharm Bull. (2020) 68:1163–9. doi: 10.1248/cpb.c20-00524
45. Bauernfeind F, Ablasser A, Bartok E, Kim S, Schmid-Burgk J, Cavlar T, et al. Inflammasomes: current understanding and open questions. Cell Mol Life Sci. (2011) 68:765–83. doi: 10.1007/s00018-010-0567-4
46. Martinon F, Gaide O, Pétrilli V, Mayor A, Tschopp J. NALP inflammasomes: a central role in innate immunity. Semin Immunopathol. (2007) 29:213–29. doi: 10.1007/s00281-007-0079-y
47. Wang F, Gao Q, Yang J, Wang C, Cao J, Sun J, et al. Artemisinin suppresses myocardial ischemia-reperfusion injury via NLRP3 inflammasome mechanism. Mol Cell Biochem. (2020) 474:171–80. doi: 10.1007/s11010-020-03842-3
48. Cheynier V. Polyphenols in foods are more complex than often thought. Am J Clin Nutr. (2005) 81(1 Suppl):223s–9. doi: 10.1093/ajcn/81.1.223S
49. Li C, Yang L, Wu H, Dai M. Paeonol inhibits oxidized low-density lipoprotein-induced vascular endothelial cells autophagy by upregulating the expression of miRNA-30a. Front Pharmacol. (2018) 9:95. doi: 10.3389/fphar.2018.00095
50. Wu H, Song A, Hu W, Dai M. The anti-atherosclerotic effect of paeonol against vascular smooth muscle cell proliferation by up-regulation of autophagy via the AMPK/mTOR signaling pathway. Front Pharmacol. (2017) 8:948. doi: 10.3389/fphar.2017.00948
51. Choy KW, Lau YS, Murugan D, Vanhoutte PM, Mustafa MR. Paeonol attenuates LPS-induced endothelial dysfunction and apoptosis by inhibiting BMP4 and TLR4 signaling simultaneously but independently. J Pharmacol Exp Ther. (2018) 364:420–32. doi: 10.1124/jpet.117.245217
52. Choy KW, Mustafa MR, Lau YS, Liu J, Murugan D, Lau CW, et al. Paeonol protects against endoplasmic reticulum stress-induced endothelial dysfunction via AMPK/PPARδ signaling pathway. Biochem Pharmacol. (2016) 116:51–62. doi: 10.1016/j.bcp.2016.07.013
53. Koo YK, Kim JM, Koo JY, Kang SS, Bae K, Kim YS, et al. Platelet anti-aggregatory and blood anti-coagulant effects of compounds isolated from Paeonia lactiflora and Paeonia suffruticosa. Pharmazie. (2010) 65:624–8.
54. Li D, Han T, Liao J, Hu X, Xu S, Tian K, et al. Oridonin, a promising ent-kaurane diterpenoid lead compound. Int J Mol Sci. (2016) 17:1395. doi: 10.3390/ijms17091395
55. Lu C, Chen C, Chen A, Wu Y, Wen J, Huang F, et al. Oridonin attenuates myocardial ischemia/reperfusion injury via downregulating oxidative stress and NLRP3 inflammasome pathway in mice. Evid Based Complement Alternat Med. (2020) 2020:7395187. doi: 10.1155/2020/7395187
56. R IE-G, Gaber SAA, Nasr M. Polymeric nanocapsular baicalin: Chemometric optimization, physicochemical characterization and mechanistic anticancer approaches on breast cancer cell lines. Sci Rep. (2019) 9:11064. doi: 10.1038/s41598-019-47586-7
57. Wang G, Liang J, Gao LR, Si ZP, Zhang XT, Liang G, et al. Baicalin administration attenuates hyperglycemia-induced malformation of cardiovascular system. Cell Death Dis. (2018) 9:234. doi: 10.1038/s41419-018-0318-2
58. Cai X, Li C, Du G, Cao Z. Protective effects of baicalin on ligature-induced periodontitis in rats. J Periodontal Res. (2008) 43:14–21. doi: 10.1111/j.1600-0765.2007.00989.x
59. Li S, Sun X, Xu L, Sun R, Ma Z, Deng X, et al. Baicalin attenuates in vivo and in vitro hyperglycemia-exacerbated ischemia/reperfusion injury by regulating mitochondrial function in a manner dependent on AMPK. Eur J Pharmacol. (2017) 815:118–26. doi: 10.1016/j.ejphar.2017.07.041
60. Lin L, Wu XD, Davey AK, Wang J. The anti-inflammatory effect of baicalin on hypoxia/reoxygenation and TNF-alpha induced injury in cultural rat cardiomyocytes. Phytother Res. (2010) 24:429–37. doi: 10.1002/ptr.3003
61. Kong F, Luan Y, Zhang ZH, Cheng GH, Qi TG, Sun C. Baicalin protects the myocardium from reperfusion-induced damage in isolated rat hearts via the antioxidant and paracrine effect. Exp Ther Med. (2014) 7:254–9. doi: 10.3892/etm.2013.1369
62. Luan Y, Sun C, Wang J, Jiang W, Xin Q, Zhang Z, et al. Baicalin attenuates myocardial ischemia-reperfusion injury through Akt/NF-κB pathway. J Cell Biochem. (2019) 120:3212–9. doi: 10.1002/jcb.27587
63. Liu X, Zhang S, Xu C, Sun Y, Sui S, Zhang Z, et al. The Protective of Baicalin on Myocardial Ischemia-Reperfusion Injury. Curr Pharm Biotechnol. (2020) 21:1386–93. doi: 10.2174/1389201021666200605104540
64. Xu M, Li X, Song L. Baicalin regulates macrophages polarization and alleviates myocardial ischaemia/reperfusion injury via inhibiting JAK/STAT pathway. Pharm Biol. (2020) 58:655–63. doi: 10.1080/13880209.2020.1779318
65. Gao F, Deng G, Liu W, Zhou K, Li M. Resveratrol suppresses human hepatocellular carcinoma via targeting HGF-c-Met signaling pathway. Oncol Rep. (2017) 37:1203–11. doi: 10.3892/or.2017.5347
66. Tian Z, Wang J, Xu M, Wang Y, Zhang M, Zhou Y. Resveratrol improves cognitive impairment by regulating apoptosis and synaptic plasticity in streptozotocin-induced diabetic rats. Cell Physiol Biochem. (2016) 40:1670–7. doi: 10.1159/000453216
67. Yang L, Zhang Y, Zhu M, Zhang Q, Wang X, Wang Y, et al. Resveratrol attenuates myocardial ischemia/reperfusion injury through up-regulation of vascular endothelial growth factor B. Free Radic Biol Med. (2016) 101:1–9. doi: 10.1016/j.freeradbiomed.2016.09.016
68. Dong W, Yang R, Yang J, Yang J, Ding J, Wu H, et al. Resveratrol pretreatment protects rat hearts from ischemia/reperfusion injury partly via a NALP3 inflammasome pathway. Int J Clin Exp Pathol. (2015) 8:8731–41.
69. Cheng L, Jin Z, Zhao R, Ren K, Deng C, Yu S. Resveratrol attenuates inflammation and oxidative stress induced by myocardial ischemia-reperfusion injury: role of Nrf2/ARE pathway. Int J Clin Exp Med. (2015) 8:10420–8.
70. Mao ZJ, Lin H, Hou JW, Zhou Q, Wang Q, Chen YH. A meta-analysis of resveratrol protects against myocardial ischemia/reperfusion injury: evidence from small animal studies and insight into molecular mechanisms. Oxid Med Cell Longev. (2019) 2019:5793867. doi: 10.1155/2019/5793867
71. Xu H, Cheng J, Wang X, Liu H, Wang S, Wu J, et al. Resveratrol pretreatment alleviates myocardial ischemia/reperfusion injury by inhibiting STIM1-mediated intracellular calcium accumulation. J Physiol Biochem. (2019) 75:607–18. doi: 10.1007/s13105-019-00704-5
72. Zhang X, Huang LF, Hua L, Feng HK, Shen B. Resveratrol protects myocardial apoptosis induced by ischemia-reperfusion in rats with acute myocardial infarction via blocking P13K/Akt/e-NOS pathway. Eur Rev Med Pharmacol Sci. (2019) 23:1789–96. doi: 10.26355/eurrev_201902_17142
73. Ravagnan G, De Filippis A, Cartenì M, De Maria S, Cozza V, Petrazzuolo M, et al. Polydatin, a natural precursor of resveratrol, induces β-defensin production and reduces inflammatory response. Inflammation. (2013) 36:26–34. doi: 10.1007/s10753-012-9516-8
74. Gao JP, Chen CX, Gu WL, Wu Q, Wang Y, Lü J. Effects of polydatin on attenuating ventricular remodeling in isoproterenol-induced mouse and pressure-overload rat models. Fitoterapia. (2010) 81:953–60. doi: 10.1016/j.fitote.2010.06.023
75. Ji H, Zhang X, Du Y, Liu H, Li S, Li L. Polydatin modulates inflammation by decreasing NF-κB activation and oxidative stress by increasing Gli1, Ptch1, SOD1 expression and ameliorates blood-brain barrier permeability for its neuroprotective effect in pMCAO rat brain. Brain Res Bull. (2012) 87:50–9. doi: 10.1016/j.brainresbull.2011.09.021
76. Ling Y, Chen G, Deng Y, Tang H, Ling L, Zhou X, et al. Polydatin post-treatment alleviates myocardial ischaemia/reperfusion injury by promoting autophagic flux. Clin Sci. (2016) 130:1641–53. doi: 10.1042/CS20160082
77. Lam FF, Yeung JH, Chan KM, Or PM. Relaxant effects of danshen aqueous extract and its constituent danshensu on rat coronary artery are mediated by inhibition of calcium channels. Vascul Pharmacol. (2007) 46:271–7. doi: 10.1016/j.vph.2006.10.011
78. Lv H, Wang L, Shen J, Hao S, Ming A, Wang X, et al. Salvianolic acid B attenuates apoptosis and inflammation via SIRT1 activation in experimental stroke rats. Brain Res Bull. (2015) 115:30–6. doi: 10.1016/j.brainresbull.2015.05.002
79. Zhao DH, Wu YJ, Liu ST, Liu RY. Salvianolic acid B attenuates lipopolysaccharide-induced acute lung injury in rats through inhibition of apoptosis, oxidative stress and inflammation. Exp Ther Med. (2017) 14:759–64. doi: 10.3892/etm.2017.4534
80. Li Y, Li J, Hou Z, Yu Y, Yu B. KLF5 overexpression attenuates cardiomyocyte inflammation induced by oxygen-glucose deprivation/reperfusion through the PPARγ/PGC-1α/TNF-α signaling pathway. Biomed Pharmacother. (2016) 84:940–6. doi: 10.1016/j.biopha.2016.09.100
81. Liu H, Liu W, Qiu H, Zou D, Cai H, Chen Q, et al. Salvianolic acid B protects against myocardial ischaemia-reperfusion injury in rats via inhibiting high mobility group box 1 protein expression through the PI3K/Akt signalling pathway. Naunyn Schmiedebergs Arch Pharmacol. (2020) 393:1527–39. doi: 10.1007/s00210-019-01755-7
82. Li Y, Xu B, Xu M, Chen D, Xiong Y, Lian M, et al. 6-Gingerol protects intestinal barrier from ischemia/reperfusion-induced damage via inhibition of p38 MAPK to NF-κB signalling. Pharmacol Res. (2017) 119:137–48. doi: 10.1016/j.phrs.2017.01.026
83. Sampath C, Sang S, Ahmedna M. In vitro and in vivo inhibition of aldose reductase and advanced glycation end products by phloretin, epigallocatechin 3-gallate and [6]-gingerol. Biomed Pharmacother. (2016) 84:502–13. doi: 10.1016/j.biopha.2016.09.073
84. El-Bakly WM, Louka ML, El-Halawany AM, Schaalan MF. 6-gingerol ameliorated doxorubicin-induced cardiotoxicity: role of nuclear factor kappa B and protein glycation. Cancer Chemother Pharmacol. (2012) 70:833–41. doi: 10.1007/s00280-012-1975-y
85. Lv X, Xu T, Wu Q, Zhou Y, Huang G, Xu Y, et al. 6-Gingerol activates PI3K/Akt and inhibits apoptosis to attenuate myocardial ischemia/reperfusion injury. Evid Based Complement Alternat Med. (2018) 2018:9024034. doi: 10.1155/2018/9024034
86. Impellizzeri D, Esposito E, Mazzon E, Paterniti I, Di Paola R, Bramanti P, et al. The effects of a polyphenol present in olive oil, oleuropein aglycone, in an experimental model of spinal cord injury in mice. Biochem Pharmacol. (2012) 83:1413–26. doi: 10.1016/j.bcp.2012.02.001
87. Cao J, Xie H, Sun Y, Zhu J, Ying M, Qiao S, et al. Sevoflurane post-conditioning reduces rat myocardial ischemia reperfusion injury through an increase in NOS and a decrease in phopshorylated NHE1 levels. Int J Mol Med. (2015) 36:1529–37. doi: 10.3892/ijmm.2015.2366
88. Liu XH, Zhao JB, Guo L, Yang YL, Hu F, Zhu RJ, et al. Simultaneous determination of calycosin-7-O-β-D-glucoside, ononin, calycosin, formononetin, astragaloside IV, and astragaloside II in rat plasma after oral administration of Radix Astragali extraction for their pharmacokinetic studies by ultra-pressure liquid chromatography with tandem mass spectrometry. Cell Biochem Biophys. (2014) 70:677–86. doi: 10.1007/s12013-014-9972-x
89. Fu S, Gu Y, Jiang JQ, Chen X, Xu M, Chen X, et al. Calycosin-7-O-β-D-glucoside regulates nitric oxide /caveolin-1/matrix metalloproteinases pathway and protects blood-brain barrier integrity in experimental cerebral ischemia-reperfusion injury. J Ethnopharmacol. (2014) 155:692–701. doi: 10.1016/j.jep.2014.06.015
90. Liu Y, Che G, Di Z, Sun W, Tian J, Ren M. Calycosin-7-O-β-D-glucoside attenuates myocardial ischemia-reperfusion injury by activating JAK2/STAT3 signaling pathway via the regulation of IL-10 secretion in mice. Mol Cell Biochem. (2020) 463:175–87. doi: 10.1007/s11010-019-03639-z
91. Li W, Zhao W, Wu Q, Lu Y, Shi J, Chen X. Puerarin improves diabetic aorta injury by inhibiting NADPH oxidase-derived oxidative stress in STZ-induced diabetic rats. J Diabetes Res. (2016) 2016:8541520. doi: 10.1155/2016/8541520
92. Shukla R, Pandey N, Banerjee S, Tripathi YB. Effect of extract of Pueraria tuberosa on expression of hypoxia inducible factor-1α and vascular endothelial growth factor in kidney of diabetic rats. Biomed Pharmacother. (2017) 93:276–85. doi: 10.1016/j.biopha.2017.06.045
93. Gao Y, Wang X, He C. An isoflavonoid-enriched extract from Pueraria lobata (kudzu) root protects human umbilical vein endothelial cells against oxidative stress induced apoptosis. J Ethnopharmacol. (2016) 193:524–30. doi: 10.1016/j.jep.2016.10.005
94. Guo BQ, Xu JB, Xiao M, Ding M, Duan LJ. Puerarin reduces ischemia/reperfusion-induced myocardial injury in diabetic rats via upregulation of vascular endothelial growth factor A/angiotensin-1 and suppression of apoptosis. Mol Med Rep. (2018) 17:7421–7. doi: 10.3892/mmr.2018.8754
95. Garg A, Garg S, Zaneveld LJ, Singla AK. Chemistry and pharmacology of the Citrus bioflavonoid hesperidin. Phytother Res. (2001) 15:655–69. doi: 10.1002/ptr.1074
96. Wei D, Ci X, Chu X, Wei M, Hua S, Deng X. Hesperidin suppresses ovalbumin-induced airway inflammation in a mouse allergic asthma model. Inflammation. (2012) 35:114–21. doi: 10.1007/s10753-011-9295-7
97. Yumnam S, Hong GE, Raha S, Saralamma VV, Lee HJ, Lee WS, et al. Mitochondrial dysfunction and Ca(2+) overload contributes to hesperidin induced paraptosis in hepatoblastoma cells, HepG2. J Cell Physiol. (2016) 231:1261–8. doi: 10.1002/jcp.25222
98. Petrova A, Davids LM, Rautenbach F, Marnewick JL. Photoprotection by honeybush extracts, hesperidin and mangiferin against UVB-induced skin damage in SKH-1 mice. J Photochem Photobiol B. (2011) 103:126–39. doi: 10.1016/j.jphotobiol.2011.02.020
99. Kim SH, Kim BK, Lee YC. Antiasthmatic effects of hesperidin, a potential Th2 cytokine antagonist, in a mouse model of allergic asthma. Mediators Inflamm. (2011) 2011:485402. doi: 10.1155/2011/485402
100. Gandhi C, Upaganalawar A, Balaraman R. Protection against in vivo focal myocardial ischemia/reperfusion injury-induced arrhythmias and apoptosis by hesperidin. Free Radic Res. (2009) 43:817–27. doi: 10.1080/10715760903071656
101. Bian C, Xu T, Zhu H, Pan D, Liu Y, Luo Y, et al. Luteolin Inhibits Ischemia/Reperfusion-Induced Myocardial Injury in Rats via Downregulation of microRNA-208b-3p. PLoS ONE. (2015) 10:e0144877. doi: 10.1371/journal.pone.0144877
102. Fang F, Li D, Pan H, Chen D, Qi L, Zhang R, et al. Luteolin inhibits apoptosis and improves cardiomyocyte contractile function through the PI3K/Akt pathway in simulated ischemia/reperfusion. Pharmacology. (2011) 88:149–58. doi: 10.1159/000330068
103. Nai C, Xuan H, Zhang Y, Shen M, Xu T, Pan D, et al. Luteolin exerts cardioprotective effects through improving sarcoplasmic reticulum Ca(2+)-ATPase activity in rats during ischemia/reperfusion in vivo. Evid Based Complement Alternat Med. (2015) 2015:365854. doi: 10.1155/2015/365854
104. Du Y, Liu P, Xu T, Pan D, Zhu H, Zhai N, et al. Luteolin modulates SERCA2a leading to attenuation of myocardial ischemia/ reperfusion injury via sumoylation at lysine 585 in mice. Cell Physiol Biochem. (2018) 45:883–98. doi: 10.1159/000487283
105. Liou KT, Lin SM, Huang SS, Chih CL, Tsai SK. Honokiol ameliorates cerebral infarction from ischemia-reperfusion injury in rats. Planta Med. (2003) 69:130–4. doi: 10.1055/s-2003-37707
106. Chen CM, Liu SH, Lin-Shiau SY. Honokiol, a neuroprotectant against mouse cerebral ischaemia, mediated by preserving Na+, K+-ATPase activity and mitochondrial functions. Basic Clin Pharmacol Toxicol. (2007) 101:108–16. doi: 10.1111/j.1742-7843.2007.00082.x
107. Tsai SK, Huang CH, Huang SS, Hung LM, Hong CY. Antiarrhythmic effect of magnolol and honokiol during acute phase of coronary occlusion in anesthetized rats: influence of L-NAME and aspirin. Pharmacology. (1999) 59:227–33. doi: 10.1159/000028324
108. Zhang B, Zhai M, Li B, Liu Z, Li K, Jiang L, et al. Honokiol ameliorates myocardial ischemia/reperfusion injury in type 1 diabetic rats by reducing oxidative stress and apoptosis through activating the SIRT1-Nrf2 signaling pathway. Oxid Med Cell Longev. (2018) 2018:3159801. doi: 10.1155/2018/3159801
109. Tan Z, Liu H, Song X, Ling Y, He S, Yan Y, et al. Honokiol post-treatment ameliorates myocardial ischemia/reperfusion injury by enhancing autophagic flux and reducing intracellular ROS production. Chemico-Biol Interact. (2019) 307:82–90. doi: 10.1016/j.cbi.2019.04.032
110. Yu Y, Xing N, Xu X, Zhu Y, Wang S, Sun G, et al. Tournefolic acid B, derived from Clinopodium chinense (Benth.) Kuntze, protects against myocardial ischemia/reperfusion injury by inhibiting endoplasmic reticulum stress-regulated apoptosis via PI3K/AKT pathways. Phytomedicine. (2019) 52:178–86. doi: 10.1016/j.phymed.2018.09.168
111. Ku SK, Kwak S, Bae JS. Orientin inhibits high glucose-induced vascular inflammation in vitro and in vivo. Inflammation. (2014) 37:2164–73. doi: 10.1007/s10753-014-9950-x
112. Fu XC, Wang MW, Li SP, Wang HL. Anti-apoptotic effect and the mechanism of orientin on ischaemic/reperfused myocardium. J Asian Nat Prod Res. (2006) 8:265–72. doi: 10.1080/10286020500207347
113. Liu L, Wu Y, Huang X. Orientin protects myocardial cells against hypoxia-reoxygenation injury through induction of autophagy. Eur J Pharmacol. (2016) 776:90–8. doi: 10.1016/j.ejphar.2016.02.037
114. Li S, Dong P, Wang J, Zhang J, Gu J, Wu X, et al. Icariin, a natural flavonol glycoside, induces apoptosis in human hepatoma SMMC-7721 cells via a ROS/JNK-dependent mitochondrial pathway. Cancer Lett. (2010) 298:222–30. doi: 10.1016/j.canlet.2010.07.009
115. Liu ZQ. Icariin: a special antioxidant to protect linoleic acid against free-radical-induced peroxidation in micelles. J Phys Chem A. (2006) 110:6372–8. doi: 10.1021/jp053998z
116. Pan Y, Wang FM, Qiang LQ, Zhang DM, Kong LD. Icariin attenuates chronic mild stress-induced dysregulation of the LHPA stress circuit in rats. Psychoneuroendocrinology. (2010) 35:272–83. doi: 10.1016/j.psyneuen.2009.06.020
117. Wu J, Zhou J, Chen X, Fortenbery N, Eksioglu EA, Wei S, et al. Attenuation of LPS-induced inflammation by ICT, a derivate of icariin, via inhibition of the CD14/TLR4 signaling pathway in human monocytes. Int Immunopharmacol. (2012) 12:74–9. doi: 10.1016/j.intimp.2011.10.015
118. Liu B, Zhang H, Xu C, Yang G, Tao J, Huang J, et al. Neuroprotective effects of icariin on corticosterone-induced apoptosis in primary cultured rat hippocampal neurons. Brain Res. (2011) 1375:59–67. doi: 10.1016/j.brainres.2010.12.053
119. Song YH, Cai H, Gu N, Qian CF, Cao SP, Zhao ZM. Icariin attenuates cardiac remodelling through down-regulating myocardial apoptosis and matrix metalloproteinase activity in rats with congestive heart failure. J Pharm Pharmacol. (2011) 63:541–9. doi: 10.1111/j.2042-7158.2010.01241.x
120. Zhai M, He L, Ju X, Shao L, Li G, Zhang Y, et al. Icariin acts as a potential agent for preventing cardiac ischemia/reperfusion injury. Cell Biochem Biophys. (2015) 72:589–97. doi: 10.1007/s12013-014-0506-3
121. Kumphune S, Surinkaew S, Chattipakorn SC, Chattipakorn N. Inhibition of p38 MAPK activation protects cardiac mitochondria from ischemia/reperfusion injury. Pharm Biol. (2015) 53:1831–41. doi: 10.3109/13880209.2015.1014569
122. Ahuja S, Kohli S, Krishnan S, Dogra D, Sharma D, Rani V. Curcumin: a potential therapeutic polyphenol, prevents noradrenaline-induced hypertrophy in rat cardiac myocytes. J Pharm Pharmacol. (2011) 63:1604–12. doi: 10.1111/j.2042-7158.2011.01363.x
123. Liu H, Wang C, Qiao Z, Xu Y. Protective effect of curcumin against myocardium injury in ischemia reperfusion rats. Pharm Biol. (2017) 55:1144–8. doi: 10.1080/13880209.2016.1214741
124. Xu H, Li Y, Che X, Tian H, Fan H, Liu K. Metabolism of salvianolic acid A and antioxidant activities of its methylated metabolites. Drug Metab Dispos. (2014) 42:274–81. doi: 10.1124/dmd.113.053694
125. Fan HY, Fu FH, Yang MY, Xu H, Zhang AH, Liu K. Antiplatelet and antithrombotic activities of salvianolic acid A. Thromb Res. (2010) 126:e17–22. doi: 10.1016/j.thromres.2010.04.006
126. Chen Q, Xu T, Li D, Pan D, Wu P, Luo Y, et al. JNK/PI3K/Akt signaling pathway is involved in myocardial ischemia/reperfusion injury in diabetic rats: effects of salvianolic acid A intervention. Am J Transl Res. (2016) 8:2534–48.
127. Chen L, Lan Z, Zhou Y, Li F, Zhang X, Zhang C, et al. Astilbin attenuates hyperuricemia and ameliorates nephropathy in fructose-induced hyperuricemic rats. Planta Med. (2011) 77:1769–73. doi: 10.1055/s-0030-1271135
128. Li GS, Jiang WL, Yue XD, Qu GW, Tian JW, Wu J, et al. Effect of astilbin on experimental diabetic nephropathy in vivo and in vitro. Planta Med. (2009) 75:1470–5. doi: 10.1055/s-0029-1185802
129. Wang J, Zhao Y, Xu Q. Astilbin prevents concanavalin A-induced liver injury by reducing TNF-alpha production and T lymphocytes adhesion. J Pharm Pharmacol. (2004) 56:495–502. doi: 10.1211/0022357023033
130. Diao H, Kang Z, Han F, Jiang W. Astilbin protects diabetic rat heart against ischemia-reperfusion injury via blockade of HMGB1-dependent NF-κB signaling pathway. Food Chem Toxicol. (2014) 63:104–10. doi: 10.1016/j.fct.2013.10.045
131. Choi EJ, Oh HM, Na BR, Ramesh TP, Lee HJ, Choi CS, et al. Eupatilin protects gastric epithelial cells from oxidative damage and down-regulates genes responsible for the cellular oxidative stress. Pharm Res. (2008) 25:1355–64. doi: 10.1007/s11095-008-9531-5
132. Min SW, Kim NJ, Baek NI, Kim DH. Inhibitory effect of eupatilin and jaceosidin isolated from Artemisia princeps on carrageenan-induced inflammation in mice. J Ethnopharmacol. (2009) 125:497–500. doi: 10.1016/j.jep.2009.06.001
133. Cheong JH, Hong SY, Zheng Y, Noh SH. Eupatilin inhibits gastric cancer cell growth by blocking STAT3-mediated VEGF expression. J Gastric Cancer. (2011) 11:16–22. doi: 10.5230/jgc.2011.11.1.16
134. Ham JR, Lee HI, Choi RY, Sim MO, Seo KI, Lee MK. Anti-steatotic and anti-inflammatory roles of syringic acid in high-fat diet-induced obese mice. Food Funct. (2016) 7:689–97. doi: 10.1039/C5FO01329A
135. Cikman O, Soylemez O, Ozkan OF, Kiraz HA, Sayar I, Ademoglu S, et al. Antioxidant activity of syringic acid prevents oxidative stress in l-arginine-induced acute pancreatitis: an experimental study on rats. Int Surg. (2015) 100:891–6. doi: 10.9738/INTSURG-D-14-00170.1
136. Tokmak M, Yuksel Y, Sehitoglu MH, Guven M, Akman T, Aras AB, et al. The neuroprotective effect of syringic acid on spinal cord ischemia/reperfusion injury in rats. Inflammation. (2015) 38:1969–78. doi: 10.1007/s10753-015-0177-2
137. Liu G, Zhang B-f, Hu Q, Liu X-p, Chen J. Syringic acid mitigates myocardial ischemia reperfusion injury by activating the PI3K/Akt/GSK-3β signaling pathway. Biochem Biophys Res Commun. (2020) 531:242–9. doi: 10.1016/j.bbrc.2020.07.047
138. Cabrera C, Artacho R, Giménez R. Beneficial effects of green tea–a review. J Am Coll Nutr. (2006) 25:79–99. doi: 10.1080/07315724.2006.10719518
139. Chyu KY, Babbidge SM, Zhao X, Dandillaya R, Rietveld AG, Yano J, et al. Differential effects of green tea-derived catechin on developing versus established atherosclerosis in apolipoprotein E-null mice. Circulation. (2004) 109:2448–53. doi: 10.1161/01.CIR.0000128034.70732.C2
140. Nagai K, Jiang MH, Hada J, Nagata T, Yajima Y, Yamamoto S, et al. (-)-Epigallocatechin gallate protects against NO stress-induced neuronal damage after ischemia by acting as an anti-oxidant. Brain Res. (2002). 956:319–22. doi: 10.1016/S0006-8993(02)03564-3
141. Song DK, Jang Y, Kim JH, Chun KJ, Lee D, Xu Z. Polyphenol (-)-epigallocatechin gallate during ischemia limits infarct size via mitochondrial K(ATP) channel activation in isolated rat hearts. J Korean Med Sci. (2010) 25:380–6. doi: 10.3346/jkms.2010.25.3.380
142. Kim SJ, Li M, Jeong CW, Bae HB, Kwak SH, Lee SH, et al. Epigallocatechin-3-gallate, a green tea catechin, protects the heart against regional ischemia-reperfusion injuries through activation of RISK survival pathways in rats. Arch Pharm Res. (2014) 37:1079–85. doi: 10.1007/s12272-013-0309-x
143. Chen Y, Huang JH, Ning Y, Shen ZY. [Icariin and its pharmaceutical efficacy: research progress of molecular mechanism]. Zhong Xi Yi Jie He Xue Bao. (2011) 9:1179–84. doi: 10.3736/jcim20111104
144. Meng X, Pei H, Lan C. Icariin exerts protective effect against myocardial ischemia/reperfusion injury in rats. Cell Biochem Biophys. (2015) 73:229–35. doi: 10.1007/s12013-015-0669-6
145. Fan SH, Zhang ZF, Zheng YL, Lu J, Wu DM, Shan Q, et al. Troxerutin protects the mouse kidney from d-galactose-caused injury through anti-inflammation and anti-oxidation. Int Immunopharmacol. (2009) 9:91–6. doi: 10.1016/j.intimp.2008.10.008
146. Kessler M, Ubeaud G, Walter T, Sturm F, Jung L. Free radical scavenging and skin penetration of troxerutin and vitamin derivatives. J Dermatolog Treat. (2002) 13:133–41. doi: 10.1080/09546630260199505
147. Najafi M, Noroozi E, Javadi A, Badalzadeh R. Anti-arrhythmogenic and anti-inflammatory effects of troxerutin in ischemia/reperfusion injury of diabetic myocardium. Biomed Pharmacother. (2018) 102:385–91. doi: 10.1016/j.biopha.2018.03.047
148. Nam KH, Choi JH, Seo YJ, Lee YM, Won YS, Lee MR, et al. Inhibitory effects of tilianin on the expression of inducible nitric oxide synthase in low density lipoprotein receptor deficiency mice. Exp Mol Med. (2006) 38:445–52. doi: 10.1038/emm.2006.52
149. Guo X, Cao W, Yao J, Yuan Y, Hong Y, Wang X, et al. Cardioprotective effects of tilianin in rat myocardial ischemia-reperfusion injury. Mol Med Rep. (2015) 11:2227–33. doi: 10.3892/mmr.2014.2954
150. Yuan Y, Cao W, Hong Y, Guo X, Wang Y, Wang Y, et al. Tilianin pretreatment prevents myocardial ischemia-reperfusion injury via preservation of mitochondrial function in rat heart. Phytomedicine. (2017) 34:106–14. doi: 10.1016/j.phymed.2017.08.007
151. Razavi SM, Zahri S, Zarrini G, Nazemiyeh H, Mohammadi S. Biological activity of quercetin-3-O-glucoside, a known plant flavonoid. Bioorg Khim. (2009) 35:414–6. doi: 10.1134/S1068162009030133
152. Liu Z, Zhang A, Guo Y, Dong C. Electrochemical sensor for ultrasensitive determination of isoquercitrin and baicalin based on DM-β-cyclodextrin functionalized graphene nanosheets. Biosens Bioelectron. (2014) 58:242–8. doi: 10.1016/j.bios.2014.02.051
153. Cao H, Xu H, Zhu G, Liu S. Isoquercetin ameliorated hypoxia/reoxygenation-induced H9C2 cardiomyocyte apoptosis via a mitochondrial-dependent pathway. Biomed Pharmacother. (2017) 95:938–43. doi: 10.1016/j.biopha.2017.08.128
154. Kim J, Lee I, Seo J, Jung M, Kim Y, Yim N, et al. Vitexin, orientin and other flavonoids from Spirodela polyrhiza inhibit adipogenesis in 3T3-L1 cells. Phytother Res. (2010) 24:1543–8. doi: 10.1002/ptr.3186
155. Lima LKF, Pereira SKS, Junior R, Santos F, Nascimento AS, Feitosa CM, et al. A brief review on the neuroprotective mechanisms of vitexin. Biomed Res Int. (2018) 2018:4785089. doi: 10.1155/2018/4785089
156. Dong LY, Li S, Zhen YL, Wang YN, Shao X, Luo ZG. Cardioprotection of vitexin on myocardial ischemia/reperfusion injury in rat via regulating inflammatory cytokines and MAPK pathway. Am J Chin Med. (2013) 41:1251–66. doi: 10.1142/S0192415X13500845
157. Salehi B, Venditti A, Sharifi-Rad M, Kregiel D, Sharifi-Rad J, Durazzo A, et al. The therapeutic potential of apigenin. Int J Mol Sci. (2019) 20:1305. doi: 10.3390/ijms20061305
158. Yang X, Yang J, Hu J, Li X, Zhang X, Li Z. Apigenin attenuates myocardial ischemia/reperfusion injury via the inactivation of p38 mitogen-activated protein kinase. Mol Med Rep. (2015) 12:6873–8. doi: 10.3892/mmr.2015.4293
159. Tesfaye R, Degu A, Abebe B, Ayalew H. Evaluation of analgesic and anti-inflammatory potential of 80% methanol leaf extract of otostegia integrifolia benth (Lamiaceae). J Inflamm Res. (2020) 13:1175–83. doi: 10.2147/JIR.S285932
160. Jian J, Xuan F, Qin F, Huang R. The antioxidant, anti-inflammatory and anti-apoptotic activities of the bauhinia championii flavone are connected with protection against myocardial ischemia/reperfusion injury. Cell Physiol Biochem. (2016) 38:1365–75. doi: 10.1159/000443080
161. Zhang Q, Yang YM, Yu GY. [Effects of gastrodin injection on blood pressure and vasoactive substances in treatment of old patients with refractory hypertension: a randomized controlled trial]. Zhong Xi Yi Jie He Xue Bao. (2008) 6:695–9. doi: 10.3736/jcim20080707
162. Peng Z, Wang S, Chen G, Cai M, Liu R, Deng J, et al. Gastrodin alleviates cerebral ischemic damage in mice by improving anti-oxidant and anti-inflammation activities and inhibiting apoptosis pathway. Neurochem Res. (2015) 40:661–73. doi: 10.1007/s11064-015-1513-5
163. Fu S, Chen L, Wu Y, Tang Y, Tang L, Zhong Y, et al. Gastrodin pretreatment alleviates myocardial ischemia/reperfusion injury through promoting autophagic flux. Biochem Biophys Res Commun. (2018) 503:2421–8. doi: 10.1016/j.bbrc.2018.06.171
164. Said MM, Azab SS, Saeed NM, El-Demerdash E. Antifibrotic mechanism of pinocembrin: impact on oxidative stress, inflammation and TGF-β/smad inhibition in rats. Ann Hepatol. (2018) 17:307–17. doi: 10.5604/01.3001.0010.8662
165. Celerino de Moraes Porto IC, Chaves Cardoso de Almeida D, Vasconcelos Calheiros de Oliveira Costa G, Sampaio Donato TS, Moreira Nunes L, Gomes do Nascimento T, et al. Mechanical and aesthetics compatibility of Brazilian red propolis micellar nanocomposite as a cavity cleaning agent. BMC Complement Altern Med. (2018) 18:219. doi: 10.1186/s12906-018-2281-y
166. Zhang P, Xu J, Hu W, Yu D, Bai X. Effects of pinocembrin pretreatment on connexin 43 (Cx43) protein expression after rat myocardial ischemia-reperfusion and cardiac arrhythmia. Med Sci Monit. (2018) 24:5008–14. doi: 10.12659/MSM.909162
167. Post-White J, Ladas EJ, Kelly KM. Advances in the use of milk thistle (Silybum marianum). Integr Cancer Ther. (2007) 6:104–9. doi: 10.1177/1534735407301632
168. Wang M, Li YJ, Ding Y, Zhang HN, Sun T, Zhang K, et al. Silibinin prevents autophagic cell death upon oxidative stress in cortical neurons and cerebral ischemia-reperfusion injury. Mol Neurobiol. (2016) 53:932–43. doi: 10.1007/s12035-014-9062-5
169. Tsaroucha AK, Valsami G, Kostomitsopoulos N, Lambropoulou M, Anagnostopoulos C, Christodoulou E, et al. Silibinin effect on Fas/FasL, HMGB1, and CD45 expressions in a rat model subjected to liver ischemia-reperfusion injury. J Invest Surg. (2018) 31:491–502. doi: 10.1080/08941939.2017.1360416
170. Chen YH, Lin H, Wang Q, Hou JW, Mao ZJ, Li YG. Protective role of silibinin against myocardial ischemia/reperfusion injury-induced cardiac dysfunction. Int J Biol Sci. (2020) 16:1972–88. doi: 10.7150/ijbs.39259
171. García-Pupo L, Van San E, Delgado-Hernández R, Vanden Berghe T, Vanden Berghe W. Emerging immune and cell death mechanisms in stroke: Saponins as therapeutic candidates. Brain Behav Immun Health. (2020) 9:100152. doi: 10.1016/j.bbih.2020.100152
172. Wang R, Wang M, Zhou J, Wu D, Ye J, Sun G, et al. Saponins in Chinese herbal medicine exerts protection in myocardial ischemia-reperfusion injury: possible mechanism and target analysis. Front Pharmacol. (2020) 11:570867. doi: 10.3389/fphar.2020.570867
173. Dong R, Guo J, Zhang Z, Zhou Y, Hua Y. Polyphyllin I inhibits gastric cancer cell proliferation by downregulating the expression of fibroblast activation protein alpha (FAP) and hepatocyte growth factor (HGF) in cancer-associated fibroblasts. Biochem Biophys Res Commun. (2018) 497:1129–34. doi: 10.1016/j.bbrc.2018.02.193
174. Zhang Y, Huang P, Liu X, Xiang Y, Zhang T, Wu Y, et al. Polyphyllin I inhibits growth and invasion of cisplatin-resistant gastric cancer cells by partially inhibiting CIP2A/PP2A/Akt signaling axis. J Pharmacol Sci. (2018) 137:305–12. doi: 10.1016/j.jphs.2018.07.008
175. Gu L, Feng J, Zheng Z, Xu H, Yu W. Polyphyllin I inhibits the growth of ovarian cancer cells in nude mice. Oncol Lett. (2016) 12:4969–74. doi: 10.3892/ol.2016.5348
176. Huang R, Shu J, Dai X, Liu Y, Yu F, Shi G. The protective effect of polyphyllin I on myocardial ischemia/reperfusion injury in rats. Ann Transl Med. (2020) 8:644. doi: 10.21037/atm-20-3371
177. Murthy HN, Dandin VS, Park SY, Paek KY. Quality, safety and efficacy profiling of ginseng adventitious roots produced in vitro. Appl Microbiol Biotechnol. (2018) 102:7309–17. doi: 10.1007/s00253-018-9188-x
178. Zheng Q, Bao XY, Zhu PC, Tong Q, Zheng GQ, Wang Y. Ginsenoside Rb1 for myocardial ischemia/reperfusion injury: preclinical evidence and possible mechanisms. Oxid Med Cell Longev. (2017) 2017:6313625. doi: 10.1155/2017/6313625
179. Quan Y, Yang Y, Wang H, Shu B, Gong QH, Qian M. Gypenosides attenuate cholesterol-induced DNA damage by inhibiting the production of reactive oxygen species in human umbilical vein endothelial cells. Mol Med Rep. (2015) 11:2845–51. doi: 10.3892/mmr.2014.3095
180. Li K, Du Y, Fan Q, Tang CY, He JF. Gypenosides might have neuroprotective and immunomodulatory effects on optic neuritis. Med Hypotheses. (2014) 82:636–8. doi: 10.1016/j.mehy.2014.02.030
181. Yan H, Wang X, Niu J, Wang Y, Wang P, Liu Q. Anti-cancer effect and the underlying mechanisms of gypenosides on human colorectal cancer SW-480 cells. PLoS ONE. (2014) 9:e95609. doi: 10.1371/journal.pone.0095609
182. Qi G, Zhang L, Xie WL, Chen XY, Li JS. Protective effect of gypenosides on DNA and RNA of rat neurons in cerebral ischemia-reperfusion injury. Acta Pharmacol Sin. (2000) 21:1193–6.
183. Chang L, Shi R, Wang X, Bao Y. Gypenoside A protects ischemia/reperfusion injuries by suppressing miR-143-3p level via the activation of AMPK/Foxo1 pathway. Biofactors. (2020) 46:432–40. doi: 10.1002/biof.1601
184. Ren S, Zhang H, Mu Y, Sun M, Liu P. Pharmacological effects of Astragaloside IV: a literature review. J Tradit Chin Med. (2013) 33:413–6. doi: 10.1016/S0254-6272(13)60189-2
185. Li L, Hou X, Xu R, Liu C, Tu M. Research review on the pharmacological effects of astragaloside IV. Fundam Clin Pharmacol. (2017) 31:17–36. doi: 10.1111/fcp.12232
186. Schmidt MR, Smerup M, Konstantinov IE, Shimizu M, Li J, Cheung M, et al. Intermittent peripheral tissue ischemia during coronary ischemia reduces myocardial infarction through a KATP-dependent mechanism: first demonstration of remote ischemic perconditioning. Am J Physiol Heart Circ Physiol. (2007) 292:H1883–90. doi: 10.1152/ajpheart.00617.2006
187. Zheng Q, Zhu JZ, Bao XY, Zhu PC, Tong Q, Huang YY, et al. A preclinical systematic review and meta-analysis of astragaloside IV for myocardial ischemia/reperfusion injury. Front Physiol. (2018) 9:795. doi: 10.3389/fphys.2018.00795
188. Lu M, Tang F, Zhang J, Luan A, Mei M, Xu C, et al. Astragaloside IV attenuates injury caused by myocardial ischemia/reperfusion in rats via regulation of toll-like receptor 4/nuclear factor-κB signaling pathway. Phytother Res. (2015) 29:599–606. doi: 10.1002/ptr.5297
189. Yin B, Hou XW, Lu ML. Astragaloside IV attenuates myocardial ischemia/reperfusion injury in rats via inhibition of calcium-sensing receptor-mediated apoptotic signaling pathways. Acta Pharmacol Sin. (2019) 40:599–607. doi: 10.1038/s41401-018-0082-y
190. Sun M, Huang C, Wang C, Zheng J, Zhang P, Xu Y, et al. Ginsenoside Rg3 improves cardiac mitochondrial population quality: mimetic exercise training. Biochem Biophys Res Commun. (2013) 441:169–74. doi: 10.1016/j.bbrc.2013.10.039
191. Wang Y, Hu Z, Sun B, Xu J, Jiang J, Luo M. Ginsenoside Rg3 attenuates myocardial ischemia/reperfusion injury via Akt/endothelial nitric oxide synthase signaling and the B-cell lymphoma/B-cell lymphoma-associated X protein pathway. Mol Med Rep. (2015) 11:4518–24. doi: 10.3892/mmr.2015.3336
192. Zhang LP, Jiang YC, Yu XF, Xu HL, Li M, Zhao XZ, et al. Ginsenoside Rg3 improves cardiac function after myocardial ischemia/reperfusion via attenuating apoptosis and inflammation. Evid Based Complement Alternat Med. (2016) 2016:6967853. doi: 10.1155/2016/6967853
193. Zhu JR, Tao YF, Lou S, Wu ZM. Protective effects of ginsenoside Rb(3) on oxygen and glucose deprivation-induced ischemic injury in PC12 cells. Acta Pharmacol Sin. (2010) 31:273–80. doi: 10.1038/aps.2010.9
194. Ma L, Liu H, Xie Z, Yang S, Xu W, Hou J, et al. Ginsenoside Rb3 protects cardiomyocytes against ischemia-reperfusion injury via the inhibition of JNK-mediated NF-κB pathway: a mouse cardiomyocyte model. PLoS ONE. (2014) 9:e103628. doi: 10.1371/journal.pone.0103628
195. Khan M, Maryam A, Zhang H, Mehmood T, Ma T. Killing cancer with platycodin D through multiple mechanisms. J Cell Mol Med. (2016) 20:389–402. doi: 10.1111/jcmm.12749
196. Cho SY, Song CH, Lee JE, Choi SH, Ku SK, Park SJ. Effects of platycodin D on reflux esophagitis due to modulation of antioxidant defense systems. Evid Based Complement Alternat Med. (2018) 2018:7918034. doi: 10.1155/2018/7918034
197. Wang Y, Che J, Zhao H, Tang J, Shi G. Platycodin D inhibits oxidative stress and apoptosis in H9c2 cardiomyocytes following hypoxia/reoxygenation injury. Biochem Biophys Res Commun. (2018) 503:3219–24. doi: 10.1016/j.bbrc.2018.08.129
198. Teponno RB, Kusari S, Spiteller M. Recent advances in research on lignans and neolignans. Nat Prod Rep. (2016) 33:1044–92. doi: 10.1039/C6NP00021E
199. Li LN, Xue H, Li X. Three new dibenzocyclooctadiene lignans from kadsura longipedunculata. Planta Med. (1991) 57:169–71. doi: 10.1055/s-2006-960058
200. Song Y, Zhao QJ, Jin YS, Feng CW, Chen HS. Two new triterpenoid acids from Kadsura coccinea. Arch Pharm Res. (2010) 33:1933–6. doi: 10.1007/s12272-010-1207-0
201. Liang CQ, Shi YM, Li XY, Luo RH, Li Y, Zheng YT, et al. Kadcotriones A-C: tricyclic triterpenoids from Kadsura coccinea. J Nat Prod. (2013) 76:2350–4. doi: 10.1021/np400546z
202. Zuo YH, Liu YB, Cheng CS, Yang YP, Xie Y, Luo P, et al. Isovaleroylbinankadsurin A ameliorates cardiac ischemia/reperfusion injury through activating GR dependent RISK signaling. Pharmacol Res. (2020) 158:104897. doi: 10.1016/j.phrs.2020.104897
203. Panossian A, Wikman G. Pharmacology of Schisandra chinensis Bail.: an overview of Russian research and uses in medicine. J Ethnopharmacol. (2008) 118:183–212. doi: 10.1016/j.jep.2008.04.020
204. Checker R, Patwardhan RS, Sharma D, Menon J, Thoh M, Bhilwade HN, et al. Schisandrin B exhibits anti-inflammatory activity through modulation of the redox-sensitive transcription factors Nrf2 and NF-κB. Free Radic Biol Med. (2012) 53:1421–30. doi: 10.1016/j.freeradbiomed.2012.08.006
205. Zhang W, Sun Z, Meng F. Schisandrin B ameliorates myocardial ischemia/reperfusion injury through attenuation of endoplasmic reticulum stress-induced apoptosis. Inflammation. (2017) 40:1903–11. doi: 10.1007/s10753-017-0631-4
206. Sung SH, Kim YC. Hepatoprotective diastereomeric lignans from Saururus chinensis herbs. J Nat Prod. (2000) 63:1019–21. doi: 10.1021/np990499e
207. Kim SJ, Jeong CW, Bae HB, Kwak SH, Son JK, Seo CS, et al. Protective effect of sauchinone against regional myocardial ischemia/reperfusion injury: inhibition of p38 MAPK and JNK death signaling pathways. J Korean Med Sci. (2012) 27:572–5. doi: 10.3346/jkms.2012.27.5.572
208. Kim T, Song B, Cho KS, Lee IS. Therapeutic potential of volatile terpenes and terpenoids from forests for inflammatory diseases. Int J Mol Sci. (2020) 21:1–2. doi: 10.3390/ijms21062187
209. Cho KS, Lim YR, Lee K, Lee J, Lee JH, Lee IS. Terpenes from forests and human health. Toxicol Res. (2017) 33:97–106. doi: 10.5487/TR.2017.33.2.097
210. Gao LW, Zhang J, Yang WH, Wang B, Wang JW. Glaucocalyxin A induces apoptosis in human leukemia HL-60 cells through mitochondria-mediated death pathway. Toxicol In Vitro. (2011) 25:51–63. doi: 10.1016/j.tiv.2010.09.006
211. Xiao X, Cao W, Jiang X, Zhang W, Zhang Y, Liu B, et al. Glaucocalyxin A, a negative Akt regulator, specifically induces apoptosis in human brain glioblastoma U87MG cells. Acta Biochim Biophys Sin. (2013) 45:946–52. doi: 10.1093/abbs/gmt097
212. Liu X, Xu D, Wang Y, Chen T, Wang Q, Zhang J, et al. Glaucocalyxin A ameliorates myocardial ischemia-reperfusion injury in mice by suppression of microvascular thrombosis. Med Sci Monit. (2016) 22:3595–604. doi: 10.12659/MSM.898015
213. Peng Z, Zhang R, Pan L, Pei H, Niu Z, Wang H, et al. Glaucocalyxin A protects H9c2 cells against hypoxia/reoxygenation-induced injury through the activation of Akt/Nrf2/HO-1 pathway. Cell Transplant. (2020) 29:963689720967672. doi: 10.1177/0963689720967672
214. Alven S, Aderibigbe BA. Nanoparticles formulations of artemisinin and derivatives as potential therapeutics for the treatment of cancer, leishmaniasis and malaria. Pharmaceutics. (2020) 12:748. doi: 10.3390/pharmaceutics12080748
215. Shan M, Yu S, Yan H, Guo S, Xiao W, Wang Z, et al. A review on the phytochemistry, pharmacology, pharmacokinetics and toxicology of geniposide, a natural product. Molecules. (2017) 22:1689. doi: 10.3390/molecules22101689
216. Zhou YX, Zhang RQ, Rahman K, Cao ZX, Zhang H, Peng C. Diverse Pharmacological Activities and Potential Medicinal Benefits of Geniposide. Evid Based Complement Alternat Med. (2019) 2019:4925682. doi: 10.1155/2019/4925682
217. Luo X, Wu S, Jiang Y, Wang L, Li G, Qing Y, et al. Inhibition of autophagy by geniposide protects against myocardial ischemia/reperfusion injury. Int Immunopharmacol. (2020) 85:106609. doi: 10.1016/j.intimp.2020.106609
218. Nabavi SM, Habtemariam S, Daglia M, Braidy N, Loizzo MR, Tundis R, et al. Neuroprotective effects of ginkgolide B against ischemic stroke: a review of current literature. Curr Top Med Chem. (2015) 15:2222–32. doi: 10.2174/1568026615666150610142647
219. Gill I, Kaur S, Kaur N, Dhiman M, Mantha AK. Phytochemical ginkgolide B attenuates amyloid-β1-42 induced oxidative damage and altered cellular responses in human neuroblastoma SH-SY5Y cells. J Alzheimers Dis. (2017) 60:S25–40. doi: 10.3233/JAD-161086
220. Shu ZM, Shu XD, Li HQ, Sun Y, Shan H, Sun XY, et al. Ginkgolide B protects against ischemic stroke via modulating microglia polarization in mice. CNS Neurosci Ther. (2016) 22:729–39. doi: 10.1111/cns.12577
221. Zhi Y, Pan J, Shen W, He P, Zheng J, Zhou X, et al. Ginkgolide B inhibits human bladder cancer cell migration and invasion through MicroRNA-223-3p. Cell Physiol Biochem. (2016) 39:1787–94. doi: 10.1159/000447878
222. Liu J, Wu P, Xu Z, Zhang J, Liu J, Yang Z. Ginkgolide B inhibits hydrogen peroxide-induced apoptosis and attenuates cytotoxicity via activating the PI3K/Akt/mTOR signaling pathway in H9c2 cells. Mol Med Rep. (2020) 22:310–6. doi: 10.3892/mmr.2020.11099
223. Zhang R, Xu L, Zhang D, Hu B, Luo Q, Han D, et al. Cardioprotection of ginkgolide B on myocardial ischemia/reperfusion-induced inflammatory injury via regulation of A20-NF-κB pathway. Front Immunol. (2018) 9:2844. doi: 10.3389/fimmu.2018.02844
224. Guo C, Zhang J, Zhang P, Si A, Zhang Z, Zhao L, et al. Ginkgolide B ameliorates myocardial ischemia reperfusion injury in rats via inhibiting endoplasmic reticulum stress. Drug Des Devel Ther. (2019) 13:767–74. doi: 10.2147/DDDT.S179101
225. Sokolov S. [The influence of saponins of Manchurian aralia on the electric activity of the brain]. Biull Eksp Biol Med. (1965) 60:73–7. doi: 10.1007/BF00805046
226. Wang M, Tian Y, Du YY, Sun GB, Xu XD, Jiang H, et al. Protective effects of Araloside C against myocardial ischaemia/reperfusion injury: potential involvement of heat shock protein 90. J Cell Mol Med. (2017) 21:1870–80. doi: 10.1111/jcmm.13107
227. Li XJ, Jiang ZZ, Zhang LY. Triptolide: progress on research in pharmacodynamics and toxicology. J Ethnopharmacol. (2014) 155:67–79. doi: 10.1016/j.jep.2014.06.006
228. Hao M, Li X, Feng J, Pan N. Triptolide protects against ischemic stroke in rats. Inflammation. (2015) 38:1617–23. doi: 10.1007/s10753-015-0137-x
229. Wu C, Wang P, Rao J, Wang Z, Zhang C, Lu L, et al. Triptolide alleviates hepatic ischemia/reperfusion injury by attenuating oxidative stress and inhibiting NF-κB activity in mice. J Surg Res. (2011) 166:e205–13. doi: 10.1016/j.jss.2010.10.005
230. Yu H, Shi L, Zhao S, Sun Y, Gao Y, Sun Y, et al. Triptolide attenuates myocardial ischemia/reperfusion injuries in rats by inducing the activation of Nrf2/HO-1 defense pathway. Cardiovasc Toxicol. (2016) 16:325–35. doi: 10.1007/s12012-015-9342-y
231. Imanshahidi M, Hosseinzadeh H. Pharmacological and therapeutic effects of Berberis vulgaris and its active constituent, berberine. Phytother Res. (2008) 22:999–1012. doi: 10.1002/ptr.2399
232. Zhang J, Ney PA. Role of BNIP3 and NIX in cell death, autophagy, and mitophagy. Cell Death Differ. (2009) 16:939–46. doi: 10.1038/cdd.2009.16
233. Huang Z, Han Z, Ye B, Dai Z, Shan P, Lu Z, et al. Berberine alleviates cardiac ischemia/reperfusion injury by inhibiting excessive autophagy in cardiomyocytes. Eur J Pharmacol. (2015) 762:1–10. doi: 10.1016/j.ejphar.2015.05.028
234. Wang Y, Liu J, Ma A, Chen Y. Cardioprotective effect of berberine against myocardial ischemia/reperfusion injury via attenuating mitochondrial dysfunction and apoptosis. Int J Clin Exp Med. (2015) 8:14513–9.
235. Zhang Q, Bian H, Guo L, Zhu H. Pharmacologic preconditioning with berberine attenuating ischemia-induced apoptosis and promoting autophagy in neuron. Am J Transl Res. (2016) 8:1197–207.
236. Zhu N, Li J, Li Y, Zhang Y, Du Q, Hao P, et al. Berberine protects against simulated ischemia/reperfusion injury-induced H9C2 cardiomyocytes apoptosis in vitro and myocardial ischemia/reperfusion-induced apoptosis in vivo by regulating the mitophagy-mediated HIF-1α/BNIP3 pathway. Front Pharmacol. (2020) 11:367. doi: 10.3389/fphar.2020.00367
237. Zhan G, Zhou J, Liu R, Liu T, Guo G, Wang J, et al. Galanthamine, plicamine, and secoplicamine alkaloids from zephyranthes candida and their anti-acetylcholinesterase and anti-inflammatory activities. J Nat Prod. (2016) 79:760–6. doi: 10.1021/acs.jnatprod.5b00681
238. Mavropoulos SA, Khan NS, Levy ACJ, Faliks BT, Sison CP, Pavlov VA, et al. Nicotinic acetylcholine receptor-mediated protection of the rat heart exposed to ischemia reperfusion. Mol Med. (2017) 23:120–33. doi: 10.2119/molmed.2017.00091
239. Hou X, Fu M, Cheng B, Kang Y, Xie D. Galanthamine improves myocardial ischemia-reperfusion-induced cardiac dysfunction, endoplasmic reticulum stress-related apoptosis, and myocardial fibrosis by suppressing AMPK/Nrf2 pathway in rats. Ann Transl Med. (2019) 7:634. doi: 10.21037/atm.2019.10.108
240. Wu G, Zhou W, Zhao J, Pan X, Sun Y, Xu H, et al. Matrine alleviates lipopolysaccharide-induced intestinal inflammation and oxidative stress via CCR7 signal. Oncotarget. (2017) 8:11621–8. doi: 10.18632/oncotarget.14598
241. Huang WC, Chan CC, Wu SJ, Chen LC, Shen JJ, Kuo ML, et al. Matrine attenuates allergic airway inflammation and eosinophil infiltration by suppressing eotaxin and Th2 cytokine production in asthmatic mice. J Ethnopharmacol. (2014) 151:470–7. doi: 10.1016/j.jep.2013.10.065
242. Sun N, Sun P, Lv H, Sun Y, Guo J, Wang Z, et al. Matrine displayed antiviral activity in porcine alveolar macrophages co-infected by porcine reproductive and respiratory syndrome virus and porcine circovirus type 2. Sci Rep. (2016) 6:24401. doi: 10.1038/srep24401
243. Feng Y, Ying HY, Qu Y, Cai XB, Xu MY, Lu LG. Novel matrine derivative MD-1 attenuates hepatic fibrosis by inhibiting EGFR activation of hepatic stellate cells. Protein Cell. (2016) 7:662–72. doi: 10.1007/s13238-016-0285-2
244. Guo S, Gao C, Xiao W, Zhang J, Qu Y, Li J, et al. Matrine protects cardiomyocytes from ischemia/reperfusion injury by regulating HSP70 expression via activation of the JAK2/STAT3 pathway. Shock. (2018) 50:664–70. doi: 10.1097/SHK.0000000000001108
245. Long J, Song J, Zhong L, Liao Y, Liu L, Li X. Palmatine: A review of its pharmacology, toxicity and pharmacokinetics. Biochimie. (2019) 162:176–84. doi: 10.1016/j.biochi.2019.04.008
246. Wang L, Wang X, Zhang SL, Zhu XM, Liu YQ, Song ZJ, et al. Gastroprotective effect of palmatine against acetic acid-induced gastric ulcers in rats. J Nat Med. (2017) 71:257–64. doi: 10.1007/s11418-016-1057-2
247. Khaksari M, Esmaili S, Abedloo R, Khastar H. Palmatine ameliorates nephrotoxicity and hepatotoxicity induced by gentamicin in rats. Arch Physiol Biochem. (2019) 2019:1–6. doi: 10.1080/13813455.2019.1633354
248. Kim YM, Ha YM, Jin YC, Shi LY, Lee YS, Kim HJ, et al. Palmatine from Coptidis rhizoma reduces ischemia–reperfusion-mediated acute myocardial injury in the rat. Food and Chemical Toxicology. (2009) 47:2097–102. doi: 10.1016/j.fct.2009.05.031
249. Chapa-Oliver AM, Mejía-Teniente L. Capsaicin: From plants to a cancer-suppressing agent. Molecules. (2016) 21:931. doi: 10.3390/molecules21080931
250. Huang J, Liu Z, Xu P, Zhang Z, Yin D, Liu J, et al. Capsaicin prevents mitochondrial damage, protects cardiomyocytes subjected to anoxia/reoxygenation injury mediated by 14-3-3η/Bcl-2. Eur J Pharmacol. (2018) 819:43–50. doi: 10.1016/j.ejphar.2017.11.028
251. Kristensen SB, van Mourik T, Pedersen TB, Sørensen JL, Muff J. Simulation of electrochemical properties of naturally occurring quinones. Sci Rep. (2020) 10:13571. doi: 10.1038/s41598-020-70522-z
252. Zhou ZY, Huang B, Li S, Huang XH, Tang JY, Kwan YW, et al. Sodium tanshinone IIA sulfonate promotes endothelial integrity via regulating VE-cadherin dynamics and RhoA/ROCK-mediated cellular contractility and prevents atorvastatin-induced intracerebral hemorrhage in zebrafish. Toxicol Appl Pharmacol. (2018) 350:32–42. doi: 10.1016/j.taap.2018.04.037
253. Zhao M, Feng Y, Xiao J, Liang J, Yin Y, Chen D. Sodium tanshinone IIA sulfonate prevents hypoxic trophoblast-induced endothelial cell dysfunction via targeting HMGB1 release. J Biochem Mol Toxicol. (2017) 31:21903. doi: 10.1002/jbt.21903
254. Ji B, Zhou F, Han L, Yang J, Fan H, Li S, et al. Sodium tanshinone IIA sulfonate enhances effectiveness Rt-PA treatment in acute ischemic stroke patients associated with ameliorating blood-brain barrier damage. Transl Stroke Res. (2017) 8:334–40. doi: 10.1007/s12975-017-0526-6
255. Xu QQ, Xu YJ, Yang C, Tang Y, Li L, Cai HB, et al. Sodium tanshinone IIA sulfonate attenuates scopolamine-induced cognitive dysfunctions via improving cholinergic system. Biomed Res Int. (2016) 2016:9852536. doi: 10.1155/2016/9852536
256. Han D, Wu X, Liu L, Shu W, Huang Z. Sodium tanshinone IIA sulfonate protects ARPE-19 cells against oxidative stress by inhibiting autophagy and apoptosis. Sci Rep. (2018) 8:15137. doi: 10.1038/s41598-018-33552-2
257. Chen F, Li H, Zhu G, Chen X, Tang Z. Sodium tanshinone IIA sulfonate improves inflammation, aortic endothelial cell apoptosis, disseminated intravascular coagulation and multiple organ damage in a rat heat stroke model. Mol Med Rep. (2017) 16:87–94. doi: 10.3892/mmr.2017.6573
258. Wei B, Li WW, Ji J, Hu QH, Ji H. The cardioprotective effect of sodium tanshinone IIA sulfonate and the optimizing of therapeutic time window in myocardial ischemia/reperfusion injury in rats. Atherosclerosis. (2014) 235:318–27. doi: 10.1016/j.atherosclerosis.2014.05.924
259. Andújar I, Ríos JL, Giner RM, Recio MC. Pharmacological properties of shikonin - a review of literature since (2002). Planta Med. (2013) 79:1685–97. doi: 10.1055/s-0033-1350934
260. Yoshida LS, Kohri S, Tsunawaki S, Kakegawa T, Taniguchi T, Takano-Ohmuro H, et al. Evaluation of radical scavenging properties of shikonin. J Clin Biochem Nutr. (2014) 55:90–6. doi: 10.3164/jcbn.13-107
261. Wang S, Zhu Y, Qiu R. Shikonin protects H9C2 cardiomyocytes against hypoxia/reoxygenation injury through activation of PI3K/Akt signaling pathway. Biomed Pharmacother. (2018) 104:712–7. doi: 10.1016/j.biopha.2018.04.144
262. Dong X, Zhou M, Li Y, Li Y, Ji H, Hu Q. Cardiovascular protective effects of plant polysaccharides: a review. Front Pharmacol. (2021) 12:783641. doi: 10.3389/fphar.2021.783641
263. Newman DJ, Cragg GM, Snader KM. Natural products as sources of new drugs over the period 1981-2002. J Nat Prod. (2003) 66:1022–37. doi: 10.1021/np030096l
264. Omata M, Matsui N, Inomata N, Ohno T. Protective effects of polysaccharide fucoidin on myocardial ischemia-reperfusion injury in rats. J Cardiovasc Pharmacol. (1997) 30:3. doi: 10.1097/00005344-199712000-00003
265. Li C, Gao Y, Xing Y, Zhu H, Shen J, Tian J. Fucoidan, a sulfated polysaccharide from brown algae, against myocardial ischemia–reperfusion injury in rats via regulating the inflammation response. Food Chem Toxicol. (2011) 49:2090–5. doi: 10.1016/j.fct.2011.05.022
266. Khalil A, Tazeddinova D, Aljoumaa K, Kazhmukhanbetkyzy ZA, Orazov A, Toshev AD. Carotenoids: therapeutic strategy in the battle against viral emerging diseases, COVID-19: an overview. Prev Nutr Food Sci. (2021) 26:241–61. doi: 10.3746/pnf.2021.26.3.241
267. Di Mascio P, Kaiser S, Sies H. Lycopene as the most efficient biological carotenoid singlet oxygen quencher. Arch Biochem Biophys. (1989) 274:532–8. doi: 10.1016/0003-9861(89)90467-0
268. Rissanen TH, Voutilainen S, Nyyssönen K, Salonen R, Kaplan GA, Salonen JT. Serum lycopene concentrations and carotid atherosclerosis: the Kuopio Ischaemic Heart Disease Risk Factor Study. Am J Clin Nutr. (2003) 77:133–8. doi: 10.1093/ajcn/77.1.133
269. Sesso HD, Buring JE, Norkus EP, Gaziano JM. Plasma lycopene, other carotenoids, and retinol and the risk of cardiovascular disease in women. Am J Clin Nutr. (2004) 79:47–53. doi: 10.1093/ajcn/79.1.47
270. Tong C, Peng C, Wang L, Zhang L, Yang X, Xu P, et al. Intravenous administration of lycopene, a tomato extract, protects against myocardial ischemia-reperfusion injury. Nutrients. (2016) 8:138. doi: 10.3390/nu8030138
271. Tesoriere L, Ciaccio M, Bongiorno A, Riccio A, Pintaudi AM, Livrea MA. Antioxidant activity of all-trans-retinol in homogeneous solution and in phosphatidylcholine liposomes. Arch Biochem Biophys. (1993) 307:217–23. doi: 10.1006/abbi.1993.1581
272. Shimada J, Taniguchi J, Mori M, Sato Y, Takuwa H, Ito H, et al. Retinol palmitate prevents ischemia-induced cell changes in hippocampal neurons through the Notch1 signaling pathway in mice. Exp Neurol. (2013) 247:182–7. doi: 10.1016/j.expneurol.2013.04.014
273. Tao L, Huang K, Wang J, Xue Y, Zhou Y, He F, et al. Retinol palmitate protects against myocardial ischemia/reperfusion injury via reducing oxidative stress and inhibiting apoptosis. Am J Transl Res. (2019) 11:1510–20.
274. Sarker SD, Nahar L. Progress in the chemistry of naturally occurring coumarins. Prog Chem Org Nat Prod. (2017) 106:241–304. doi: 10.1007/978-3-319-59542-9_3
275. Ko FN, Wu TS, Liou MJ, Huang TF, Teng CM. Vasorelaxation of rat thoracic aorta caused by osthole isolated from Angelica pubescens. Eur J Pharmacol. (1992) 219:29–34. doi: 10.1016/0014-2999(92)90576-P
276. Xu XM, Zhang Y, Qu D, Feng XW, Chen Y, Zhao L. Osthole suppresses migration and invasion of A549 human lung cancer cells through inhibition of matrix metalloproteinase-2 and matrix metallopeptidase-9 in vitro. Mol Med Rep. (2012) 6:1018–22. doi: 10.3892/mmr.2012.1044
277. Lin VC, Chou CH, Lin YC, Lin JN, Yu CC, Tang CH, et al. Osthole suppresses fatty acid synthase expression in HER2-overexpressing breast cancer cells through modulating Akt/mTOR pathway. J Agric Food Chem. (2010) 58:4786–93. doi: 10.1021/jf100352c
278. Matsuda H, Tomohiro N, Ido Y, Kubo M. Anti-allergic effects of cnidii monnieri fructus (dried fruits of Cnidium monnieri) and its major component, osthol. Biol Pharm Bull. (2002) 25:809–12. doi: 10.1248/bpb.25.809
279. Wang XY, Dong WP, Bi SH, Pan ZG, Yu H, Wang XW, et al. Protective effects of osthole against myocardial ischemia/reperfusion injury in rats. Int J Mol Med. (2013) 32:365–72. doi: 10.3892/ijmm.2013.1386
280. Zhu L, Nang C, Luo F, Pan H, Zhang K, Liu J, et al. Esculetin attenuates lipopolysaccharide (LPS)-induced neuroinflammatory processes and depressive-like behavior in mice. Physiol Behav. (2016) 163:184–92. doi: 10.1016/j.physbeh.2016.04.051
281. Wang G, Lu M, Yao Y, Wang J, Li J. Esculetin exerts antitumor effect on human gastric cancer cells through IGF-1/PI3K/Akt signaling pathway. Eur J Pharmacol. (2017) 814:207–15. doi: 10.1016/j.ejphar.2017.08.025
282. He Y, Li C, Ma Q, Chen S. Esculetin inhibits oxidative stress and apoptosis in H9c2 cardiomyocytes following hypoxia/reoxygenation injury. Biochem Biophys Res Commun. (2018) 501:139–44. doi: 10.1016/j.bbrc.2018.04.195
283. Wang Y, Liu M, Chen S, Wu Q. Plantamajoside represses the growth and metastasis of malignant melanoma. Exp Ther Med. (2020) 19:2296–302. doi: 10.3892/etm.2020.8442
284. Liu F, Huang X, He JJ, Song C, Peng L, Chen T, et al. Plantamajoside attenuates inflammatory response in LPS-stimulated human gingival fibroblasts by inhibiting PI3K/AKT signaling pathway. Microb Pathog. (2019) 127:208–11. doi: 10.1016/j.micpath.2018.11.034
285. Han AR, Nam MH, Lee KW. Plantamajoside inhibits UVB and advanced glycation end products-induced MMP-1 expression by suppressing the MAPK and NF-κB pathways in HaCaT cells. Photochem Photobiol. (2016) 92:708–19. doi: 10.1111/php.12615
286. Jung HY, Seo DW, Hong CO, Kim JY, Yang SY, Lee KW. Nephroprotection of plantamajoside in rats treated with cadmium. Environ Toxicol Pharmacol. (2015) 39:125–36. doi: 10.1016/j.etap.2014.11.012
287. Wang Y, Yan D. Plantamajoside exerts antifibrosis effects in the liver by inhibiting hepatic stellate cell activation. Exp Ther Med. (2019) 18:2421–8. doi: 10.3892/etm.2019.7843
288. Zeng G, An H, Fang D, Wang W, Han Y, Lian C. Plantamajoside protects H9c2 cells against hypoxia/reoxygenation-induced injury through regulating the akt/Nrf2/HO-1 and NF-κB signaling pathways. J Recept Signal Transduct Res. (2020) 2020:1–8. doi: 10.1080/10799893.2020.1859534
289. Kuo WW, Wang WJ, Tsai CY, Way CL, Hsu HH, Chen LM. Diallyl trisufide (DATS) suppresses high glucose-induced cardiomyocyte apoptosis by inhibiting JNK/NFκB signaling via attenuating ROS generation. Int J Cardiol. (2013) 168:270–80. doi: 10.1016/j.ijcard.2012.09.080
290. Jeremic JN, Jakovljevic VL, Zivkovic VI, Srejovic IM, Bradic JV, Milosavljevic IM, et al. Garlic derived diallyl trisulfide in experimental metabolic syndrome: metabolic effects and cardioprotective role. Int J Mol Sci. (2020) 21:9100. doi: 10.3390/ijms21239100
291. Bai Y, Tohda C, Zhu S, Hattori M, Komatsu K. Active components from Siberian ginseng (Eleutherococcus senticosus) for protection of amyloid β(25-35)-induced neuritic atrophy in cultured rat cortical neurons. J Nat Med. (2011) 65:417–23. doi: 10.1007/s11418-011-0509-y
292. Wang S, Yang X. Eleutheroside E decreases oxidative stress and NF-κB activation and reprograms the metabolic response against hypoxia-reoxygenation injury in H9c2 cells. Int Immunopharmacol. (2020) 84:106513. doi: 10.1016/j.intimp.2020.106513
293. Zhu Y, Shi YP, Wu D, Ji YJ, Wang X, Chen HL, et al. Salidroside protects against hydrogen peroxide-induced injury in cardiac H9c2 cells via PI3K-Akt dependent pathway. DNA Cell Biol. (2011) 30:809–19. doi: 10.1089/dna.2010.1183
294. Cao LL, Du GH, Wang MW. The effect of salidroside on cell damage induced by glutamate and intracellular free calcium in PC12 cells. J Asian Nat Prod Res. (2006) 8:159–65. doi: 10.1080/1028602042000325645
295. Zhu L, Wei T, Gao J, Chang X, He H, Luo F, et al. The cardioprotective effect of salidroside against myocardial ischemia reperfusion injury in rats by inhibiting apoptosis and inflammation. Apoptosis. (2015) 20:1433–43. doi: 10.1007/s10495-015-1174-5
296. Jose R, Sajitha GR, Augusti KT. A review on the role of nutraceuticals as simple as se(2+) to complex organic molecules such as glycyrrhizin that prevent as well as cure diseases. Indian J Clin Biochem. (2014) 29:119–32. doi: 10.1007/s12291-013-0362-8
297. Rahman S, Sultana S. Glycyrrhizin exhibits potential chemopreventive activity on 12-O-tetradecanoyl phorbol-13-acetate-induced cutaneous oxidative stress and tumor promotion in Swiss albino mice. J Enzyme Inhib Med Chem. (2007) 22:363–9. doi: 10.1080/14756360601074094
298. He SQ, Gao M, Fu YF, Zhang YN. Glycyrrhizic acid inhibits leukemia cell growth and migration via blocking AKT/mTOR/STAT3 signaling. Int J Clin Exp Pathol. (2015) 8:5175–81.
299. Cai X, Wang X, Li J, Chen S. Protective effect of glycyrrhizin on myocardial ischemia/reperfusion injury-induced oxidative stress, inducible nitric oxide synthase and inflammatory reactions through high-mobility group box 1 and mitogen-activated protein kinase expression. Exp Ther Med. (2017) 14:1219–26. doi: 10.3892/etm.2017.4617
300. Chang JS, Chiang LC, Hsu FF, Lin CC. Chemoprevention against hepatocellular carcinoma of Cornus officinalis in vitro. Am J Chin Med. (2004) 32:717–25. doi: 10.1142/S0192415X04002296
301. Jiang WL, Chen XG, Zhu HB, Tian JW. Effect of cornuside on experimental sepsis. Planta Med. (2009) 75:614–9. doi: 10.1055/s-0029-1185383
302. Jiang W-L, Zhang S-M, Tang X-X, Liu H-Z. Protective roles of cornuside in acute myocardial ischemia and reperfusion injury in rats. Phytomedicine. (2011) 18:266–71. doi: 10.1016/j.phymed.2010.07.009
303. Xu G, Zhao X, Fu J, Wang X. Resveratrol increase myocardial Nrf2 expression in type 2 diabetic rats and alleviate myocardial ischemia/reperfusion injury (MIRI). Ann Palliat Med. (2019) 8:565–75. doi: 10.21037/apm.2019.11.25
304. Bhullar KS, Rupasinghe HP. Polyphenols: multipotent therapeutic agents in neurodegenerative diseases. Oxid Med Cell Longev. (2013) 2013:891748. doi: 10.1155/2013/891748
305. Gelderblom M, Leypoldt F, Lewerenz J, Birkenmayer G, Orozco D, Ludewig P, et al. The flavonoid fisetin attenuates postischemic immune cell infiltration, activation and infarct size after transient cerebral middle artery occlusion in mice. J Cereb Blood Flow Metab. (2012) 32:835–43. doi: 10.1038/jcbfm.2011.189
306. Hausenloy DJ, Yellon DM. New directions for protecting the heart against ischaemia-reperfusion injury: targeting the Reperfusion Injury Salvage Kinase (RISK)-pathway. Cardiovasc Res. (2004) 61:448–60. doi: 10.1016/j.cardiores.2003.09.024
307. Zhai P, Sciarretta S, Galeotti J, Volpe M, Sadoshima J. Differential roles of GSK-3β during myocardial ischemia and ischemia/reperfusion. Circ Res. (2011) 109:502–11. doi: 10.1161/CIRCRESAHA.111.249532
308. Damilano F, Perino A, Hirsch E. PI3K kinase and scaffold functions in heart. Ann N Y Acad Sci. (2010) 1188:39–45. doi: 10.1111/j.1749-6632.2009.05081.x
309. Shelton LM, Park BK, Copple IM. Role of Nrf2 in protection against acute kidney injury. Kidney Int. (2013) 84:1090–5. doi: 10.1038/ki.2013.248
310. Ding Y, Chen M, Wang M, Wang M, Zhang T, Park J, et al. Neuroprotection by acetyl-11-keto-β-Boswellic acid, in ischemic brain injury involves the Nrf2/HO-1 defense pathway. Sci Rep. (2014) 4:7002. doi: 10.1038/srep07002
311. Ucar BI, Ucar G, Saha S, Buttari B, Profumo E, Saso L. Pharmacological protection against ischemia-reperfusion injury by regulating the Nrf2-Keap1-ARE signaling pathway. Antioxidants. (2021) 10:60823. doi: 10.3390/antiox10060823
312. Ashrafian H, Czibik G, Bellahcene M, Aksentijević D, Smith AC, Mitchell SJ, et al. Fumarate is cardioprotective via activation of the Nrf2 antioxidant pathway. Cell Metab. (2012) 15:361–71. doi: 10.1016/j.cmet.2012.01.017
313. Zhou J, Xi C, Wang W, Fu X, Jinqiang L, Qiu Y, et al. Triptolide-induced oxidative stress involved with Nrf2 contribute to cardiomyocyte apoptosis through mitochondrial dependent pathways. Toxicol Lett. (2014) 230:454–66. doi: 10.1016/j.toxlet.2014.08.017
314. Zhang WY, Zhang QL, Xu MJ. Effects of propofol on myocardial ischemia reperfusion injury through inhibiting the JAK/STAT pathway. Eur Rev Med Pharmacol Sci. (2019) 23:6339–45. doi: 10.26355/eurrev_201907_18457
315. Stephanou A. Role of STAT-1 and STAT-3 in ischaemia/reperfusion injury. J Cell Mol Med. (2004) 8:519–25. doi: 10.1111/j.1582-4934.2004.tb00476.x
316. Sciarretta S, Volpe M, Sadoshima J. Mammalian target of rapamycin signaling in cardiac physiology and disease. Circ Res. (2014) 114:549–64. doi: 10.1161/CIRCRESAHA.114.302022
317. Yang P, Ling L, Sun W, Yang J, Zhang L, Chang G, et al. Ginsenoside Rg1 inhibits apoptosis by increasing autophagy via the AMPK/mTOR signaling in serum deprivation macrophages. Acta Biochim Biophys Sin. (2018) 50:144–55. doi: 10.1093/abbs/gmx136
Keywords: myocardial ischemia/reperfusion injury, phytochemicals, pharmacology, mechanisms, therapeutic implication
Citation: Chen C, Yu L-T, Cheng B-R, Xu J-L, Cai Y, Jin J-L, Feng R-L, Xie L, Qu X-Y, Li D, Liu J, Li Y, Cui X-Y, Lu J-J, Zhou K, Lin Q and Wan J (2022) Promising Therapeutic Candidate for Myocardial Ischemia/Reperfusion Injury: What Are the Possible Mechanisms and Roles of Phytochemicals? Front. Cardiovasc. Med. 8:792592. doi: 10.3389/fcvm.2021.792592
Received: 10 October 2021; Accepted: 29 December 2021;
Published: 17 February 2022.
Edited by:
Stéphanie Barrere-Lemaire, INSERM U1191 Institut de Génomique Fonctionnelle (IGF), FranceReviewed by:
Claudia Penna, University of Turin, ItalyDennis Cokkinos, Biomedical Research Foundation of the Academy of Athens (BRFAA), Greece
Copyright © 2022 Chen, Yu, Cheng, Xu, Cai, Jin, Feng, Xie, Qu, Li, Liu, Li, Cui, Lu, Zhou, Lin and Wan. This is an open-access article distributed under the terms of the Creative Commons Attribution License (CC BY). The use, distribution or reproduction in other forums is permitted, provided the original author(s) and the copyright owner(s) are credited and that the original publication in this journal is cited, in accordance with accepted academic practice. No use, distribution or reproduction is permitted which does not comply with these terms.
*Correspondence: Qian Lin, bGlucWlhbjYyJiN4MDAwNDA7MTI2LmNvbQ==; Jie Wan, MzIxNDM2MTMmI3gwMDA0MDtxcS5jb20=
†These authors have contributed equally to this work and share first authorship