- 1Institute of Atherosclerosis, College of Basic Medical Sciences, Shandong First Medical University, Shandong Academy of Medical Sciences, Tai'an, China
- 2Department of Pediatrics and Group on the Molecular and Cell Biology of Lipids, Faculty of Medicine and Dentistry, University of Alberta, Edmonton, AB, Canada
The adrenal gland produces steroid hormones to play essential roles in regulating various physiological processes. Our previous studies showed that knockout of hepatic Surf4 (Surf4LKO) markedly reduced fasting plasma total cholesterol levels in adult mice, including low-density lipoprotein and high-density lipoprotein cholesterol. Here, we found that plasma cholesterol levels were also dramatically reduced in 4-week-old young mice and non-fasted adult mice. Circulating lipoprotein cholesterol is an important source of the substrate for the production of adrenal steroid hormones. Therefore, we investigated whether adrenal steroid hormone production was affected in Surf4LKO mice. We observed that lacking hepatic Surf4 essentially eliminated lipid droplets and significantly reduced cholesterol levels in the adrenal gland; however, plasma levels of aldosterone and corticosterone were comparable in Surf4LKO and the control mice under basal and stress conditions. Further analysis revealed that mRNA levels of genes encoding enzymes important for hormone synthesis were not altered, whereas the expression of scavenger receptor class B type I (SR-BI), low-density lipoprotein receptor (LDLR) and 3-hydroxy-3-methyl-glutaryl-CoA reductase was significantly increased in the adrenal gland of Surf4LKO mice, indicating increased de novo cholesterol biosynthesis and enhanced LDLR and SR-BI-mediated lipoprotein cholesterol uptake. We also observed that the nuclear form of SREBP2 was increased in the adrenal gland of Surf4LKO mice. Taken together, these findings indicate that the very low levels of circulating lipoprotein cholesterol in Surf4LKO mice cause a significant reduction in adrenal cholesterol levels but do not significantly affect adrenal steroid hormone production. Reduced adrenal cholesterol levels activate SREBP2 and thus increase the expression of genes involved in cholesterol biosynthesis, which increases de novo cholesterol synthesis to compensate for the loss of circulating lipoprotein-derived cholesterol in the adrenal gland of Surf4LKO mice.
Introduction
The adrenal cortex uses cholesterol as the substrate to produce steroid hormones, thus playing an indispensable role in regulating metabolism, water and salt balance and blood pressure, the immune system, stress response, and sexual development. Under a normal physiological condition, ~80% of cholesterol used in adrenal cortex hormone synthesis is derived from circulating lipoproteins, such as scavenger receptor class B, type I (SR-BI)-mediated selective uptake of cholesteryl ester from high-density lipoprotein (HDL) and low-density lipoprotein receptor (LDLR)-mediated endocytosis of LDL (1). The remaining cholesterol is contributed by de novo biosynthesis from acetate via the mevalonate pathway (2, 3), in which 3-hydroxy-3-methylglutaryl coenzyme A reductase (HMGCR) is the rate-limiting enzyme (4, 5). Cholesterol is stored as cholesteryl ester (CE) in lipid droplets in the adrenal cortex (2, 6, 7), which can be converted to free cholesterol by lipase-mediated lipolysis as needed for steroidogenesis (8–10). Free cholesterol from lipolysis of CE in lipid droplets, de novo biosynthesis, and plasma membrane can be rapidly transported to mitochondria by steroidogenic acute regulatory protein (StAR) (6, 8, 11, 12), where steroid hormones are synthesized from cholesterol by different mitochondrial P450 enzymes and then immediately released to circulation. Adrenal insufficiency characterized by low blood levels of cortisol and aldosterone can lead to a series of systemic clinical symptoms, including hypotension, anorexia, fatigue, syncope, hyponatremia, sexual dysfunction, mental disorders, etc. (3).
Surfeit 4 (Surf4) is a cargo receptor resided on the endoplasmic reticulum (ER) membrane, where it facilitates the transport of secretory proteins from the ER to the Golgi apparatus. Surf4 also mediates the retrograde transport of STING from the Golgi apparatus to the ER (13–19). We and others have found that Surf4 mediates secretion of very low-density lipoprotein (VLDL) (15, 19). Circulating VLDL is catabolized and eventually converted to LDL, which is then cleared from circulation mainly through hepatic LDLR (20). Knockout of LDLR (Ldlr−/−) in mice increases plasma cholesterol levels and risk for the development of atherosclerosis. Mutations in LDLR cause familial hypercholesterolemia (FH) in humans, which is characterized by elevated plasma LDL cholesterol levels and increased risk for cardiovascular disease. Current lipid-lowering drugs, such as statins and PCSK9 inhibitors, reduce plasma cholesterol levels mainly through increasing LDLR levels and thus LDL clearance. Therefore, they cannot effectively reduce plasma LDL cholesterol levels in FH patients.
Knockout of Surf4 in mouse liver (Surf4LKO) and knockdown of Surf4 in Ldlr−/− mice significantly reduce VLDL secretion, leading to a drastic reduction in plasma cholesterol levels (15). However, we did not observe significant hepatic lipid accumulation or notable liver damage in Surf4LKO mice or Surf4 knockdown Ldlr−/− mice, indicating that hepatic Surf4 inhibition is a promising therapeutic target for lowering plasma lipids through suppressing LDL production. However, circulating lipoprotein-derived cholesterol, especially HDL cholesterol, is an important substrate for the production of adrenal cortex steroid hormones (1, 12, 21–24). Therefore, we investigated whether hepatic Surf4 silencing affected the production of adrenal cortex hormones. We found that lipid droplets and cholesterol levels were significantly reduced in the adrenal gland of Surf4LKO mice compared to the control Surf4Flox mice. However, plasma levels of adrenal cortex hormones, including corticosterone, aldosterone, and dehydroepiandrosterone (DHEA), and adrenocorticotropin (ACTH) were comparable in Surf4LKO and Surf4Flox mice. The expression of HMGCR, LDLR and SR-BI was markedly increased in the adrenal gland of Surf4LKO mice. Therefore, knockout of hepatic Surf4 did not affect the production of adrenal cortex hormones despite a significant reduction in plasma and adrenal cholesterol levels.
Materials and Methods
Materials
H&E staining kit and saturated oil red O staining solution were purchased from Beijing Soleibao Technology Co., Ltd. (Beijing, China). Mouse corticosterone, aldosterone, DHEA, and ACTH ELISA kits were from Shanghai Enzyme Link Biotechnology Co., Ltd. (Shanghai, China). Total cholesterol kit was purchased from Qiyi Biotechnology Co., Ltd. (Shanghai, China). Anti-LDLR, HMGCR, SR-BI, and SREBP-2 antibodies were from Abcam. Anti-β-actin and ACAT1 antibodies were from Beijing Boaosen Biotechnology Co., Ltd. (Beijing, China) and Proteintech, respectively. Horseradish enzyme-labeled goat anti-rabbit or mouse IgG was purchased from Beijing Zhongshan Jinqiao Biotechnology Co., Ltd. (Beijing, China). RNAprep Pure Tissue Kit, EasyQuick RT MasterMix, and Top Green qPCR SuperMix were purchased from Tiangen Biochemical Technology Co., Ltd. (Beijing, China), Kangwei Century Biotechnology Co., Ltd. (Beijing, China), and Beijing Quanshijin Biotechnology Co., Ltd. (Beijing, China), respectively.
Animal
Sur4Flox and Surf4LKO mice in C57BL/6 background were generated as described (15) and were maintained in the animal facility at Shandong First Medical University (Taian, China). Three to five mice were housed per cage with free access to H2O in a climate-controlled facility with a 12-h light/dark cycle. After weaning, mice were fed ad libitum a chow diet containing 20% protein, 5% fat, and 48.7% carbohydrates (Keao Xieli, Beijing, China). All animal procedures were approved by Shandong First Medical University's Animal Care and Use Committee.
Histochemistry
The experiments were performed as described (15, 25). Briefly, for H&E staining, tissues were fixed, embedded in paraffin, cut into 8 μm, and mounted on slides. After, the sections were deparaffinized, rehydrated, and then stained with hematoxylin and eosin sequentially. For Oil Red-O staining, fresh tissue samples were embedded in Optimal Cutting Temperature compound, cut into 10 μm, and then mounted on slides. After, the sections were fixed in formalin, stained with Oil Red-O and then hematoxylin. All slices were imaged on a microscope (Nikon, Tokyo, Japan). Relative stained areas were quantified with ImageJ software (National Institute of Health) using color segmentation and threshold analysis.
ELISA
Blood samples were collected from fasted or non-fasted mice. Plasma levels of adrenal hormones and ACTH were measured with their specific ELISA kits according to the manufacturer's instruction. The optical density was measured using a SpectraMax i3x Microplate Reader (Filter: 450 nm).
Quantitative Real-Time PCR
Total RNAs were extracted from mouse tissues using RNAprep Pure Tissue Kit according to the manufacturer's protocols. Complementary DNA (cDNA) was synthesized using EasyQuick RT MasterMix from Kangwei Century Company (China). qRT-PCR was carried out using Top Green qPCR SuperMix. 2−ΔΔct was used to analyze relative gene expression. Gapdh was the control. The primers were designed and synthesized by Shanghai Shenggong Biological Engineering Co., Ltd. and listed in Table 1.
Immunoblotting
Tissue samples were collected from euthanized mice and homogenized in RIPA buffer (50 mM Tris, pH 7.4, 150 mM NaCl, 1% TritonX-100, 1% sodium deoxycholate, 0.1% SDS, sodium orthovanadate, sodium fluoride, EDTA, leupeptin, and PMSF) as described (15). The supernatant was harvested as tissue homogenate after centrifugation. Protein concentrations were determined by the BCA protein assay. Equal amounts of total lysate proteins were applied to SDS-PAGE and then transferred to PVDF membranes (Millipore) by electroblotting. Immunoblotting was performed using specific antibodies as indicated. Antibody binding was detected by HRP-conjugated goat anti-mouse or rabbit IgG antibody, followed with Pierce™ ECL Western Blotting Substrate. The image was acquired and analyzed on a Tanon 5200 automatic chemiluminescence image analysis system. The densitometry was quantified in Image-Pro Plus.
Plasma and Tissue Lipids
Blood samples were collected into EDTA-coated tubes from mice and then centrifuged at 3000 x g for 10 min. Plasma from each mouse was subjected to analysis of triglyceride (TG), total cholesterol (TC), and HDL-C using their specific kits according to the manufacturer's instructions (Applygen, Beijing, China).
Adrenal lipids were extracted using the methyl-tert-butyl ether (MTBE) method as described (15). Briefly, tissues (3–5 mg) were homogenized in 280 μl of cold methanol containing an internal standard of cholesteryl ester (CE) 17:0. In total, 50 μl of homogenates were stored to analyze protein content using a commercial BCA protein assay kit (Solarbio Co., Beijing, China). MTBE (1 ml) was added to the remaining volume of homogenates; the samples were vortexed, rotated for 1 h at room temperature, and then mixed with 325 μl H2O. After, the samples were centrifuged at 10,000 × g for 10 min at 4°C. The upper hydrophobic fraction was collected and dried under nitrogen gas. The samples were dissolved in methanol. Total cholesterol and free cholesterol were measured using an enzymatic kit from Biosino Biotechnology (Beijing, China) and Applygen (Beijing, China), respectively.
Dried lipid extracts were also reconstituted in 200 μl of acetonitrile:2-propanol (1:1, v/v) and then subjected to liquid chromatography tandem mass spectrometry (LC-MS/MS) analysis as described (15). Briefly, LC-MS/MS was performed using a Shimadzu LC-20 AD binary pump system coupled to a SIL-20AC autoinjector and interfaced with an ABI 4000 QTrap mass spectrometer (Sciex, Framingham, MA, USA). Chromatographic separations were carried out on a Waters Symmetry C18 column with a Waters C18 guard column. The mobile phase comprised (A) 10 mM ammonium formate in acetonitrile: water: formic acid (83:17:0.1, v/v/v) and (B) 10 mM ammonium formate in acetonitrile: 2-propanol: formic acid (50:50:0.1, v/v/v). Isocratic elution was performed with 95% B for 16 min. Relative quantification of lipids in samples was carried out based on the intensity of each species divided by the intensity of the internal standards and protein concentrations.
Statistical Analysis
All statistical analyses were carried out by GraphPad Prism version 9.0 (GraphPad Software). The significant differences between groups were determined via a Student's t-test or a Mann Whitney test. All data met normal distribution criteria and variance between groups that was analyzed by F-test showed no significant difference (P > 0.05). Values of all data, unless otherwise indicated, were depicted as mean ± S.D. All experiments, unless indicated, were repeated at least three times. The significance was defined as *p < 0.05, **p < 0.01, ***p < 0.001, and ****p < 0.0001.
Results
Effect of Hepatic Surf4 Silencing on Cholesterol Levels in the Adrenal Gland
We have reported that knockout of hepatic Surf4 impairs VLDL secretion and markedly reduces fasting plasma cholesterol and TG levels in adult mice (15). Here, we found that fasting plasma total cholesterol and TG levels were also dramatically reduced in 4-week-old young Surf4LKO mice (Figures 1A,B). Furthermore, similar to the results of the study on fasting plasma cholesterol levels (15), non-fasting plasma levels of total cholesterol, HDL cholesterol and non-HDL cholesterol were all markedly reduced in Surf4LKO mice (Figures 1C–E). Therefore, the lack of hepatic Surf4 drastically reduced plasma cholesterol levels in young mice and fasted or non-fasted adult mice. Considering that circulating lipoprotein cholesterol is an important substrate for adrenal steroid hormone biosynthesis, we assessed whether the adrenal gland was affected in Surf4LKO mice. As shown in Figures 1F,G, the adrenal glands in Surf4Flox and Surf4LKO mice were similar in size and shape. The ratio of adrenal weight to body weight was also comparable in the two genotypes (Figure 1H). However, their colors were different, white and red in Surf4Flox and Surf4LKO mice, respectively.
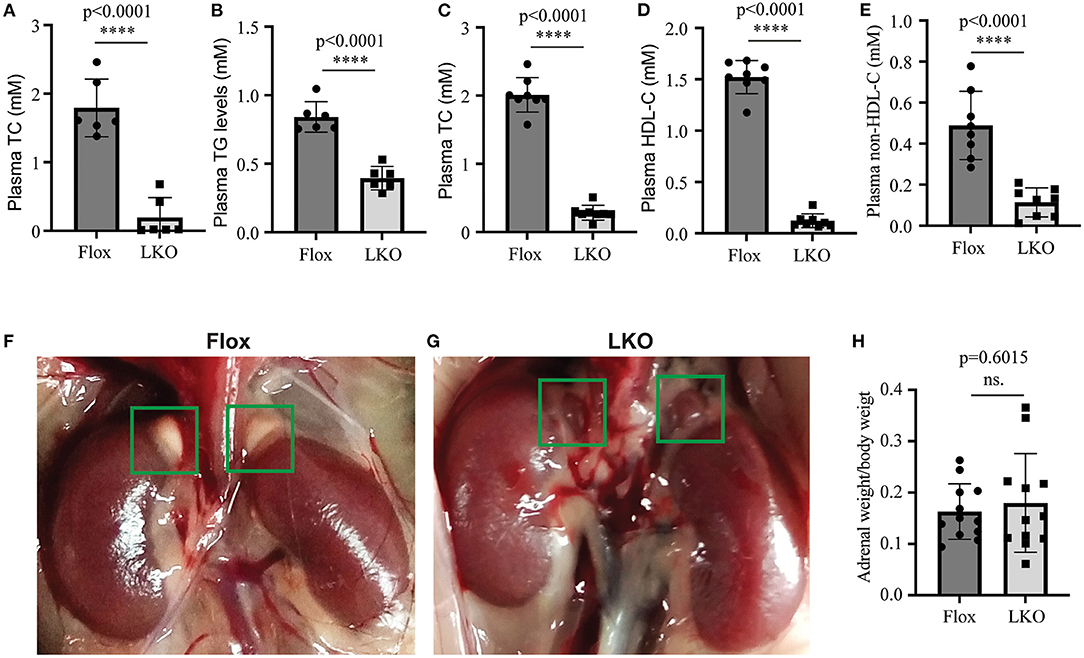
Figure 1. The effect of hepatic knockout of Surf4 on plasma lipids and the adrenal gland. (A–E) Plasma lipid levels. Blood samples were collected from 4-week-old mice (A,B) fasted for 10 h or 14-week-old mice fasted for 10 h and refed for 4 h (C–E). Plasma levels of total cholesterol (TC), triglycerides (TG), and HDL cholesterol (HDL-C) were measured using their specific enzymatic kits (n ≥ 6). Non-HDL-C was calculated by subtracting HDL-C from TC. (F,G) Representative images of the adrenal glands of Surf4Flox (F) and Surf4LKO mice (G) (green frame). Similar results were observed in other mice. (H) Ratio of the adrenal weight to the body weight of the same mouse. Values of all data were mean ± S.D. The significance was defined as ****p < 0.0001 and P > 0.05, no significance (ns.).
Next, we used H&E and Oil Red O staining to analyze the histology of the adrenal gland. As shown in Figure 2A, the adrenal cortex of Surf4Flox mice displayed many vacuoles, which were essentially absent in the adrenal cortex of Surf4LKO mice. Oil Red O staining revealed that the adrenal cortex of Surf4Flox mice contained many lipid droplets stained red (Figure 2B). Conversely, in the adrenal gland of Surf4LKO mice, the staining appeared as small puncta and was markedly reduced (Figures 2B,C), and the levels of total cholesterol and cholesteryl ester (CE) were also significantly reduced (Figures 2D,E). However, adrenal free cholesterol levels were not significantly affected by hepatic knockout of Surf4 (Figure 2F). A detailed analysis of adrenal lipids using LC-MS/MS revealed that the levels of CE18:1, CE20:4, and CE22:6, but not CE 16:1, CE18:0, CE18:2, CE18:3, CE20:3, or CE20:5 were significantly reduced in the adrenal gland of Surf4LKO mice (Table 2). Therefore, lacking hepatic Surf4 significantly reduced cholesteryl ester levels and virtually depleted lipid droplets in the adrenal gland, which could explain the color change observed in the adrenal gland of Surf4LKO mice.
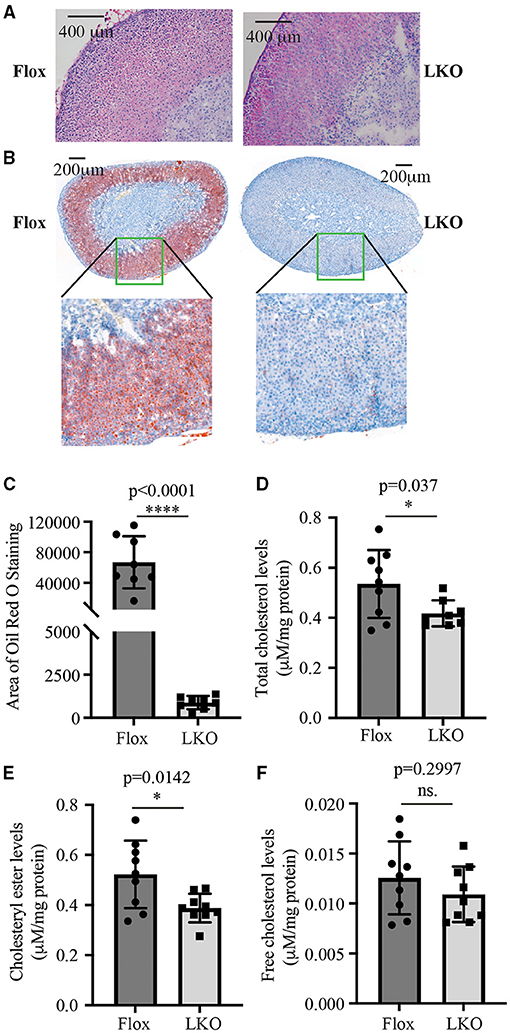
Figure 2. Impact of lacking hepatic Surf4 on the adrenal gland. (A) H&E staining of adrenal sections. (B,C) Oil Red O of adrenal sections. Representative figures were shown. Similar results were obtained in other mice. The images of Oil Red O staining were quantified using ImageJ (C) (8 mice per group). Data were analyzed by a Student's t-test (p < 0.0001) and a Mann Whitney test (p = 0.0002). (D–F) Adrenal total cholesterol (D), cholesteryl ester (E), and free cholesterol (F) levels. Lipids were extracted from mouse adrenal glands using the MTBE method and then subjected to total cholesterol and free cholesterol measurement using their specific enzymatic kit. Cholesteryl ester levels were calculated by subtracting free cholesterol from total cholesterol. Values of all data were mean ± S.D. The significance was defined as *p < 0.05 and ****p < 0.0001, p > 0.05, no significance (ns.).
Effect of Hepatic Surf4 Knockout on the Production of Adrenal Cortex Hormones
The adrenal cortex uses cholesterol as the substrate to produce steroid hormones. Therefore, we used ELISA to measure the plasma levels of aldosterone, corticosterone and DHEA, and found that their levels were comparable in non-fasted Surf4Flox and Surf4LKO mice (Figures 3A–C). We then used qRT-PCR to assess the expression of genes that are crucial for adrenal cortex hormone synthesis. StAR mediates the transport of cholesterol from the outer to the inner membrane of the mitochondrial and is believed to be the rate-limiting step in adrenal corticosteroid synthesis. Cyp11a1 converts cholesterol to pregnenolone. Cyp17a1 is involved in the last step of DHEA synthesis. Cyp11b1 and Cyp11b2 are the key enzymes for aldosterone synthesis, and Cyp21a2 and Cyp11b1 are crucial for corticosteroid synthesis. qRT-PCR data showed that the mRNA levels of these enzymes were not significantly altered in the adrenal gland of Surf4LKO mice (Figure 3D). Taken together, these findings indicate that under basal conditions, the production of adrenal cortex hormone in Surf4LKO mice is not significantly affected.
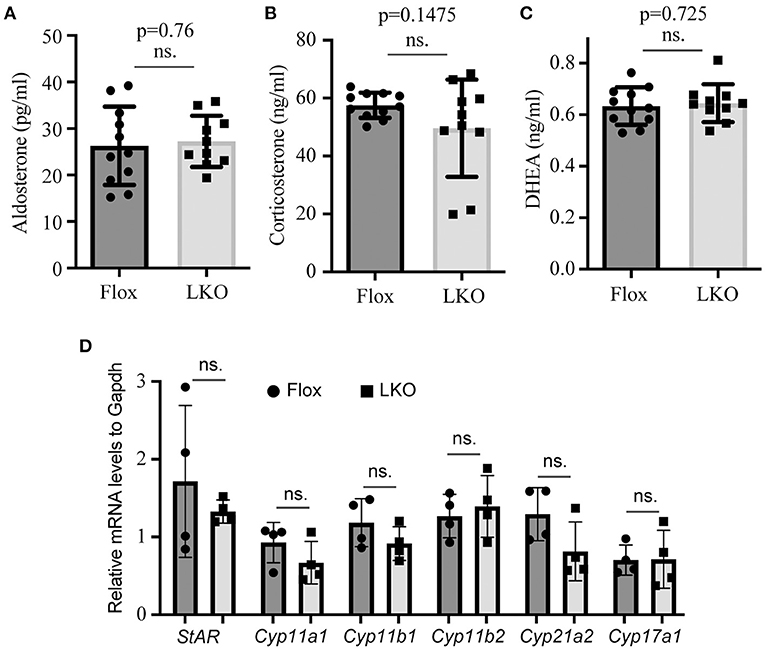
Figure 3. Impact of lacking hepatic Surf4 on plasma levels of adrenal hormones. (A–C) Plasma adrenal hormone levels. Blood samples were collected from non-fasted mice [Surf4Flox (Flox) and Surf4LKO (LKO) mice, 10–14-week-old]. Aldosterone (A), corticosterone (B), and DHEA (C) were measured using their specific ELISA kits. (D) qRT-PCR. Total RNAs were extracted from adrenal glands and then subjected to qRT-PCR. The relative mRNA levels were the ratio of the target's mRNA levels indicated to that of Gapdh at the same condition (n = 4). Values of all data were mean ± S.D. p > 0.05 was defined as no significant difference (ns.).
Stress stimuli, such as fasting and cold exposure, can stimulate the pituitary to release ACTH, which then activates the adrenal cortex to produce corticosterone (12, 26, 27). To evaluate whether the production of adrenal steroid hormones was affected in Surf4LKO mice under stress conditions, we subjected mice to fasting and found that the levels of fasting plasma corticosterone, aldosterone and DEHA were comparable in Surf4Flox and Surf4LKO mice (Figures 4A–C). We then challenged fasted mice with acute cold stimulation at 4°C for up to 4 h. As shown in Figure 4D, the plasma levels of corticosterone were comparable and displayed a similar pattern in Surf4Flox and Surf4LKO mice, increasing after cold stimulation until the 0.5-h time point and then gradually decreasing at the 1.5-h time point. Therefore, the production of corticosterone under stress stimuli is not affected by hepatic Surf4 knockout.
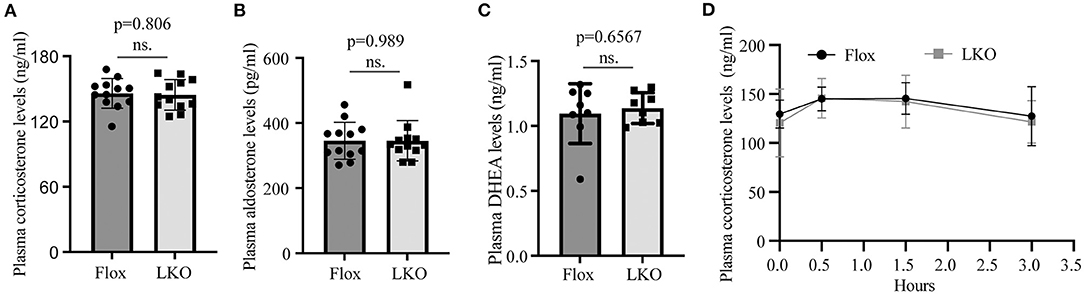
Figure 4. Plasma levels of adrenal steroid hormones. (A–C) Fasting plasma hormone levels. Mice were fasted for 12 h, and blood samples were collected for the measurement of corticosterone (A), aldosterone (B), and DHEA (C) with their specific ELISA kits. (D) Plasma corticosterone levels. Mice fasted for 12 h were subjected to cold stimulation (4°C). Blood samples were collected at the indicated time points. Plasma corticosterone levels were measured using its specific ELISA kit (n = 6). Values of all data were mean ± S.D. p > 0.05 was defined as no significant difference (ns.).
Effect of Hepatic Surf4 Knockout on the Expression of Genes Involved in Cholesterol Metabolism
Adrenal cells take up circulating LDL and HDL cholesterol via LDLR and SR-BI, respectively. They also produce cholesterol via de novo biosynthesis. Therefore, we examined the expression of LDLR, SR-BI, and HMG-CoA reductase, the latter being the rate-limiting enzyme in cholesterol biosynthesis. qRT-PCR showed that the mRNA levels of Scarb, Ldlr, and Hmgcr were all significantly increased in the adrenal gland of Surf4LKO mice (Figure 5A). We also measured the mRNA levels of additional genes involved in cholesterol biosynthesis, including HMG-CoA synthase (Hmgcs1), mevalonate kinase (Mvk), farnesyl diphosphate synthase (Fdps), and squalene synthase (Fdft1), and observed that they were all significantly increased in the adrenal gland of Surf4LKO mice (Figure 5B). Consistently, western blot data revealed an increase in the protein levels of adrenal SR-BI, LDLR and HMGCR in Surf4LKO mice (Figure 5C). SREBP2 upregulates the transcription of genes involved in cholesterol metabolism, such as LDLR, HMGCR, HMG-CoA synthase, squalene synthase, etc. (28). Acyl-CoA:cholesterol acyltransferase 1 (ACAT1) converts free cholesterol to cholesteryl ester. Lacking ACAT1 significantly reduces cellular cholesteryl ester levels (29). Therefore, we measured adrenal SREBP2 and ACAT1 levels. As shown in Figure 5D, the levels of the nuclear form of SREBP2 were increased, while ACAT1 levels were not changed in the adrenal gland of Surf4LKO mice compared to Surf4Flox mice. In addition, ACTH can increase expression of LDLR and SR-BI (30, 31). Therefore, we assessed plasma ACTH levels in Surf4Flox and Surf4LKO mice using ELISA. As shown in Figure 5E, lacking hepatic Surf4 did not significantly affect plasma ACTH levels. Taken together, these findings suggest an increased ability in cholesterol de novo biosynthesis and uptake of circulating lipoproteins in the adrenal gland of Surf4LKO mice.
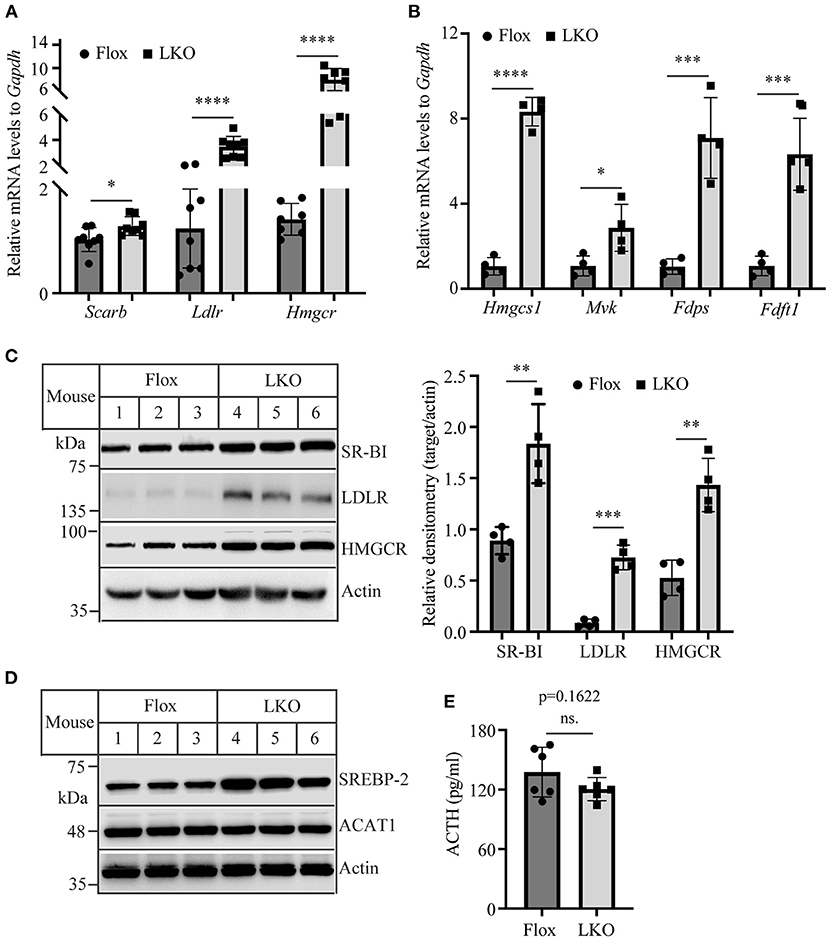
Figure 5. The expression of genes involved in cholesterol metabolism. (A,B) qRT-PCR. The relative mRNA levels were the ratio of the target's mRNA levels indicated to that of Gapdh at the same condition. (C,D) Immunoblotting. The same amount of adrenal homogenate was applied to Western Blot using antibodies as indicated. Representative figures were shown. The densitometry was assessed. The relative densitometry is the densitometry of each target to that of actin in the same sample (C). (E) Plasma levels of ACTH. Mice (10–12-week-old, male) were fasted for 12 h. Plasma was then collected and subjected to the measurement of ACTH using an ELISA kit. Values of all data were mean ± S.D. The significance was defined as *p < 0.05, **p < 0.01, ***p < 0.001, and ****p < 0.0001. p > 0.05 was defined as no significant difference (ns.).
Discussion
Here, we found that (1) knockout of hepatic Surf4 in mice essentially depleted lipid droplets and significantly reduced cholesteryl ester levels in the adrenal glands of Surf4LKO mice; (2) the production of adrenal cortex steroid hormones was comparable in Surf4LKO and Surf4Flox mice under basal and stress conditions; and (3) the expression of genes involved in steroid hormone synthesis was not affected, whereas the expression of SREBP2 and genes involved in cholesterol metabolism was significantly increased in the adrenal gland of Surf4LKO. Taken together, these findings indicate that the ability of the adrenal cortex to produce steroid hormones is not affected by the lack of hepatic Surf4 even though plasma lipoprotein cholesterol levels are drastically reduced in the young and fasted and non-fasted adult mice.
VLDL is exclusively secreted by hepatocytes and can be catabolized into LDL in circulation. Hepatic knockout of Surf4 virtually eliminates VLDL secretion and LDL production in mice. LDLR-mediated LDL endocytosis can provide cholesterol for adrenal steroidogenesis (24, 32). However, several lines of evidence show that SR-BI-mediated selective CE uptake from HDL serves as an important source of cholesterol for adrenal cortex steroidogenesis under stress conditions (1, 12, 21–23). In addition, Bochem et al. reported that HDL-derived cholesterol is an important substrate for adrenal cortex hormone synthesis under a basal condition in humans (33). Knockout of apolipoprotein A-I (apoA-I), the main structural lipoprotein on HDL, reduced plasma HDL-C levels by ~74% and essentially depleted lipid droplets in the adrenal cortex. The production of corticosteroids under a basal or stress condition was also significantly reduced in apoA-I knockout mice (34–36). Like apoA-I knockout mice, Surf4LKO mice display a similar reduction in plasma HDL cholesterol levels under fasting and non-fasting conditions. However, the production of adrenal cortex hormones in Surf4LKO mice was not significantly affected even under stress conditions.
SR-BI mediates the selective uptake of CE from HDL (23, 37). Knockout of SR-BI significantly reduces the production of glucocorticoids under stress conditions even though plasma levels of apoA-I and HDL cholesterol levels remain unchanged and are increased, respectively (1, 21, 37). SR-BI can bind to HDL particles reconstituted with apoA-I, apoA-II, apoE, and apoC-III (38); however, lacking apoA-I significantly reduces the capacity of SR-BI to mediate CE-selective uptake from HDL. Furthermore, the accumulation of CE in steroidogenic cells is essentially disappeared in apoA-I knockout mice (34, 35, 39). These findings indicate that apo A-I is essential for the effective transfer of CE from HDL to adrenal cortex cells. Surf4LKO mice display a significant reduction in plasma levels of HDL cholesterol and apoA-I; however, unlike apoA-I knockout mice that are completely deficient in apoA-I, Surf4LKO mice still retain a small portion of circulating apoA-I and HDL cholesterol (15). The increased expression of SR-BI in the adrenal gland of Surf4LKO mice may enhance the ability of adrenal cortex cells to take up cholesterol from residual circulating HDL, thereby mitigating the adverse effect of decreased plasma cholesterol levels on adrenal cortex steroidogenesis.
Cholesterol biosynthesis provides ~20% of the substrate for steroidogenesis in the adrenal cortex under normal conditions. Total cholesterol levels in the adrenal gland of Surf4LKO mice were significantly reduced, leading to increased levels of the nuclear form of SREBP2. This upregulated the expression of genes involved in cholesterol de novo biosynthesis and LDLR. LDLR mediates endocytosis of LDL and apoE-containing lipoprotein particles, such as VLDL and chylomicron remnants. Plasma apoE levels are comparable in Surf4LKO and Surf4Flox mice (15). Together, our findings suggest that cholesterol biosynthesis and LDLR-mediated endocytosis of apoE-containing lipoproteins may increase in adrenal cortex cells of Surf4LKO mice, which at least partially compensate for the loss of HDL-derived cholesterol.
Deficiency of hepatic Surf4 significantly reduces VLDL secretion, plasma cholesterol levels and the development of atherosclerosis but does not cause notable liver damage or hepatic lipid accumulation in mice (15). Here, we found that knockout of hepatic Surf4 drastically reduces plasma cholesterol in 4-week-old young mice (newly weaned) and non-fasted adult mice, whereas the production of adrenal steroid hormones is not significantly impaired in Surf4LKO mice under normal and stress conditions. These findings indicate that hepatic Surf4 inhibition is a promising therapeutic target for lowering plasma lipid levels in patients whose disease cannot be effectively managed by currently available strategies.
Data Availability Statement
The original contributions presented in the study are included in the article/supplementary material, further inquiries can be directed to the corresponding author/s.
Ethics Statement
The animal study was reviewed and approved by Shandong First Medical University's Animal Care and Use Committee.
Author Contributions
SQ and D-wZ designed the experiments, analyzed data, and wrote the manuscript. XC and ZY performed the experiments, analyzed data, and wrote the first draft. HW, BW, LZ, YZ, BL, and H-mG performed experiments and analyzed data. All authors contributed to the article and approved the submitted version.
Funding
This work was supported by National Natural Science Foundation of China (NSFC 81929002), Academic Promotion Program of Shandong First Medical University (2019QL010 and 2019PT009), and The Natural Sciences and Engineering Research Council of Canada (RGPIN-2016-06479). D-wZ was also supported by grants from Canadian Institutes of Health Research (PS 178091) and the China Institute at the University of Alberta. SQ was supported by 91539114 and ts201511057.
Conflict of Interest
The authors declare that the research was conducted in the absence of any commercial or financial relationships that could be construed as a potential conflict of interest.
Publisher's Note
All claims expressed in this article are solely those of the authors and do not necessarily represent those of their affiliated organizations, or those of the publisher, the editors and the reviewers. Any product that may be evaluated in this article, or claim that may be made by its manufacturer, is not guaranteed or endorsed by the publisher.
References
1. Ito M, Ye X, Wang Q, Guo L, Hao D, Howatt D, et al. SR-BI (Scavenger Receptor BI), not LDL (Low-Density Lipoprotein) receptor, mediates adrenal stress response-brief report. Arterioscler Thromb Vasc Biol. (2020) 40:1830–7. doi: 10.1161/ATVBAHA.120.314506
2. Kraemer FB, Khor VK, Shen WJ, Azhar S. Cholesterol ester droplets and steroidogenesis. Mol Cell Endocrinol. (2013) 371:15–9. doi: 10.1016/j.mce.2012.10.012
3. Lyraki R, Schedl A. Adrenal cortex renewal in health and disease. Nat Rev Endocrinol. (2021) 17:421–34. doi: 10.1038/s41574-021-00491-4
4. Johnson BM, DeBose-Boyd RA. Underlying mechanisms for sterol-induced ubiquitination and ER-associated degradation of HMG CoA reductase. Semin Cell Dev Biol. (2018) 81:121–8. doi: 10.1016/j.semcdb.2017.10.019
5. Luo J, Yang H, Song BL. Mechanisms and regulation of cholesterol homeostasis. Nat Rev Mol Cell Biol. (2020) 21:225–45. doi: 10.1038/s41580-019-0190-7
6. Li Y, Khanal P, Norheim F, Hjorth M, Bjellaas T, Drevon CA, et al. Plin2 deletion increases cholesteryl ester lipid droplet content and disturbs cholesterol balance in adrenal cortex. J Lipid Res. (2021) 62:100048. doi: 10.1016/j.jlr.2021.100048
7. Yu J, Zhang L, Li Y, Zhu X, Xu S, Zhou XM, et al. The adrenal lipid droplet is a new site for steroid hormone metabolism. Proteomics. (2018) 18:e1800136. doi: 10.1002/pmic.201800136
8. Li H, Brochu M, Wang SP, Rochdi L, Cote M, Mitchell G, et al. Hormone-sensitive lipase deficiency in mice causes lipid storage in the adrenal cortex and impaired corticosterone response to corticotropin stimulation. Endocrinology. (2002) 143:3333–40. doi: 10.1210/en.2002-220341
9. Weckman A, Di Ieva A, Rotondo F, Syro LV, Ortiz LD, Kovacs K, et al. Autophagy in the endocrine glands. J Mol Endocrinol. (2014) 52:R151–63. doi: 10.1530/JME-13-0241
10. Sztalryd C, Brasaemle DL. The perilipin family of lipid droplet proteins: gatekeepers of intracellular lipolysis. Biochim Biophys Acta Mol Cell Biol Lipids. (2017) 1862:1221–32. doi: 10.1016/j.bbalip.2017.07.009
11. Deng B, Shen WJ, Dong D, Azhar S, Kraemer FB. Plasma membrane cholesterol trafficking in steroidogenesis. FASEB J. (2019) 33:1389–400. doi: 10.1096/fj.201800697RRR
12. Hoekstra M, Korporaal SJ, van der Sluis RJ, Hirsch-Reinshagen V, Bochem AE, Wellington CL, et al. LCAT deficiency in mice is associated with a diminished adrenal glucocorticoid function. J Lipid Res. (2013) 54:358–64. doi: 10.1194/jlr.M030080
13. Mukai K, Ogawa E, Uematsu R, Kuchitsu Y, Kiku F, Uemura T, et al. Homeostatic regulation of STING by retrograde membrane traffic to the ER. Nat Commun. (2021) 12:61. doi: 10.1038/s41467-020-20234-9
14. Ordonez A, Harding HP, Marciniak SJ, Ron D. Cargo receptor-assisted endoplasmic reticulum export of pathogenic alpha1-antitrypsin polymers. Cell Rep. (2021) 35:109144. doi: 10.1016/j.celrep.2021.109144
15. Wang B, Shen Y, Zhai L, Xia X, Gu HM, Wang M, et al. Atherosclerosis-associated hepatic secretion of VLDL but not PCSK9 is dependent on cargo receptor protein Surf4. J Lipid Res. (2021) 62:100091. doi: 10.1016/j.jlr.2021.100091
16. Lin Z, King R, Tang V, Myers G, Balbin-Cuesta G, Friedman A, et al. The endoplasmic reticulum cargo receptor surf4 facilitates efficient erythropoietin secretion. Mol Cell Biol. (2020) 40:e00180-20. doi: 10.1128/MCB.00180-20
17. Saegusa K, Sato M, Morooka N, Hara T, Sato K. SFT-4/Surf4 control ER export of soluble cargo proteins and participate in ER exit site organization. J Cell Biol. (2018) 217:2073–85. doi: 10.1083/jcb.201708115
18. Shen Y, Wang B, Deng S, Zhai L, Gu HM, Alabi A, et al. Surf4 regulates expression of proprotein convertase subtilisin/kexin type 9 (PCSK9) but is not required for PCSK9 secretion in cultured human hepatocytes. Biochim Biophys Acta Mol Cell Biol Lipids. (2020) 1865:158555. doi: 10.1016/j.bbalip.2019.158555
19. Wang X, Wang H, Xu B, Huang D, Nie C, Pu L, et al. Receptor-mediated er export of lipoproteins controls lipid homeostasis in mice and humans. Cell Metab. (2021) 33:350–366 e7. doi: 10.1016/j.cmet.2020.10.020
20. Goldstein JL, Brown MS. The LDL receptor. Arterioscler Thromb Vasc Biol. (2009) 29:431–8. doi: 10.1161/ATVBAHA.108.179564
21. Hoekstra M, Meurs I, Koenders M, Out R, Hildebrand RB, Kruijt JK, et al. Absence of HDL cholesteryl ester uptake in mice via SR-BI impairs an adequate adrenal glucocorticoid-mediated stress response to fasting. J Lipid Res. (2008) 49:738–45. doi: 10.1194/jlr.M700475-JLR200
22. Temel RE, Trigatti B, DeMattos RB, Azhar S, Krieger M, Williams DL. Scavenger receptor class B, type I (SR-BI) is the major route for the delivery of high density lipoprotein cholesterol to the steroidogenic pathway in cultured mouse adrenocortical cells. Proc Natl Acad Sci USA. (1997) 94:13600–5. doi: 10.1073/pnas.94.25.13600
23. Rigotti A, Trigatti BL, Penman M, Rayburn H, Herz J, Krieger M, et al. targeted mutation in the murine gene encoding the high density lipoprotein (HDL) receptor scavenger receptor class B type I reveals its key role in HDL metabolism. Proc Natl Acad Sci USA. (1997) 94:12610–5. doi: 10.1073/pnas.94.23.12610
24. Faust JR, Goldstein JL, Brown MS. Receptor-mediated uptake of low density lipoprotein and utilization of its cholesterol for steroid synthesis in cultured mouse adrenal cells. J Biol Chem. (1977) 252:4861–71. doi: 10.1016/S0021-9258(17)40133-5
25. Alabi A, Xia XD, Gu HM, Wang F, Deng SJ, Yang N, et al. Membrane type 1 matrix metalloproteinase promotes LDL receptor shedding and accelerates the development of atherosclerosis. Nat Commun. (2021) 12:1889. doi: 10.1038/s41467-021-22167-3
26. van den Beukel JC, Grefhorst A, Quarta C, Steenbergen J, Mastroberardino PG, Lombes M, et al. Direct activating effects of adrenocorticotropic hormone (ACTH) on brown adipose tissue are attenuated by corticosterone. FASEB J. (2014) 28:4857–67. doi: 10.1096/fj.14-254839
27. Bowers SL, Bilbo SD, Dhabhar FS, Nelson RJ. Stressor-specific alterations in corticosterone and immune responses in mice. Brain Behav Immun. (2008) 22:105–13. doi: 10.1016/j.bbi.2007.07.012
28. Goldstein JL, Brown MS. century of cholesterol and coronaries: from plaques to genes to statins. Cell. (2015) 161:161–72. doi: 10.1016/j.cell.2015.01.036
29. Meiner VL, Cases S, Myers HM, Sande ER, Bellosta S, Schambelan M, et al. Disruption of the acyl-CoA:cholesterol acyltransferase gene in mice: evidence suggesting multiple cholesterol esterification enzymes in mammals. Proc Natl Acad Sci USA. (1996) 93:14041–6. doi: 10.1073/pnas.93.24.14041
30. Heikkila P, Arola J, Liu J, Kahri AI, ACTH. regulates LDL receptor and CLA-1 mRNA in the rat adrenal cortex. Endocr Res. (1998) 24:591–3. doi: 10.3109/07435809809032651
31. Shen WJ, Azhar S, Kraemer FB, ACTH. Regulation of Adrenal SR-B1. Front Endocrinol (Lausanne). (2016) 7:42. doi: 10.3389/fendo.2016.00042
32. Illingworth DR, Lees AM, Lees RS. Adrenal cortical function in homozygous familial hypercholesterolemia. Metabolism. (1983) 32:1045–52. doi: 10.1016/0026-0495(83)90075-6
33. Bochem AE, Holleboom AG, Romijn JA, Hoekstra M, Dallinga-Thie GM, Motazacker MM, et al. High density lipoprotein as a source of cholesterol for adrenal steroidogenesis: a study in individuals with low plasma HDL-C. J Lipid Res. (2013) 54:1698–704. doi: 10.1194/jlr.P033449
34. Plump AS, Erickson SK, Weng W, Partin JS, Breslow JL, Williams DL. Apolipoprotein A-I is required for cholesteryl ester accumulation in steroidogenic cells and for normal adrenal steroid production. J Clin Invest. (1996) 97:2660–71. doi: 10.1172/JCI118716
35. Plump AS, Azrolan N, Odaka H, Wu L, Jiang X, Tall A, et al. ApoA-I knockout mice: characterization of HDL metabolism in homozygotes and identification of a post-RNA mechanism of apoA-I up-regulation in heterozygotes. J Lipid Res. (1997) 38:1033–47. doi: 10.1016/S0022-2275(20)37227-8
36. Ouweneel AB, van der Sluis RJ, Nahon JE, Van Eck M, Hoekstra M. Simvastatin treatment aggravates the glucocorticoid insufficiency associated with hypocholesterolemia in mice. Atherosclerosis. (2017) 261:99–104. doi: 10.1016/j.atherosclerosis.2017.02.014
37. Trigatti B, Rayburn H, Vinals M, Braun A, Miettinen H, Penman M, et al. Influence of the high density lipoprotein receptor SR-BI on reproductive and cardiovascular pathophysiology. Proc Natl Acad Sci USA. (1999) 96:9322–7. doi: 10.1073/pnas.96.16.9322
38. Thuahnai ST, Lund-Katz S, Anantharamaiah GM, Williams DL, Phillips MC. quantitative analysis of apolipoprotein binding to SR-BI: multiple binding sites for lipid-free and lipid-associated apolipoproteins. J Lipid Res. (2003) 44:1132–42. doi: 10.1194/jlr.M200429-JLR200
Keywords: proprotein convertase subtilisin/kexin 9, LDL–cholesterol, cholesterol, triglyceride, atherosclerosis, LDL receptor (LDLR)
Citation: Chang X, Zhao Y, Qin S, Wang H, Wang B, Zhai L, Liu B, Gu H-m and Zhang D-w (2021) Loss of Hepatic Surf4 Depletes Lipid Droplets in the Adrenal Cortex but Does Not Impair Adrenal Hormone Production. Front. Cardiovasc. Med. 8:764024. doi: 10.3389/fcvm.2021.764024
Received: 24 August 2021; Accepted: 20 October 2021;
Published: 11 November 2021.
Edited by:
Changcheng Zhou, University of California, Riverside, United StatesReviewed by:
Chieko Mineo, University of Texas Southwestern Medical Center, United StatesMary G. Sorci-Thomas, Medical College of Wisconsin, United States
Copyright © 2021 Chang, Zhao, Qin, Wang, Wang, Zhai, Liu, Gu and Zhang. This is an open-access article distributed under the terms of the Creative Commons Attribution License (CC BY). The use, distribution or reproduction in other forums is permitted, provided the original author(s) and the copyright owner(s) are credited and that the original publication in this journal is cited, in accordance with accepted academic practice. No use, distribution or reproduction is permitted which does not comply with these terms.
*Correspondence: Shucun Qin, c2NxaW5Ac2RmbXUuZWR1LmNu; Da-wei Zhang, ZHpoYW5nQHVhbGJlcnRhLmNh
†These authors have contributed equally to this work