- 1Center for Pulmonary Vascular Diseases, National Center for Cardiovascular Diseases, Fuwai Hospital, Chinese Academy of Medical Sciences and Peking Union Medical College, Beijing, China
- 2Department of Cardiology, Zhongshan Hospital, Fudan University, Shanghai, China
Background: The hemodynamic results of balloon pulmonary angioplasty vary among patients with inoperable chronic thromboembolic pulmonary hypertension (CTEPH). Previous studies revealed that microvasculopathy accounted for residual pulmonary hypertension after pulmonary endarterectomy, which could be reflected by the diffusing capacity for carbon monoxide (DLCO). We aimed to identify whether the DLCO could predict the BPA response.
Materials and Methods: We retrospectively analyzed 75 consecutive patients with inoperable CTEPH who underwent BPA from May 2018 to January 2021 at Fuwai Hospital. According to the hemodynamics at follow-up after the last BPA, patients were classified as “BPA responders” (defined as a mean pulmonary arterial pressure ≤ 30 mmHg and/or a reduction of pulmonary vascular resistance ≥ 30%) or “BPA nonresponders.”
Results: At the baseline, BPA responders had significantly higher DLCO values than nonresponders, although the other variables were comparable. In BPA responders, the DLCO decreased after the first BPA session and then returned to a level similar to the baseline at follow-up. Conversely, the DLCO increased constantly from the baseline to follow-up in nonresponders. Multivariate logistic analysis showed that a baseline DLCO of <70% and a percent change in DLCO between the baseline and the period within 7 days after the first BPA session (ΔDLCO) of > 6% were both independent predictors of an unfavorable response to BPA. Receiver operator characteristic analysis showed that the combination of a baseline DLCO < 70% and ΔDLCO > 6% demonstrated a better area under the curve than either of these two variables used alone.
Conclusions: A baseline DLCO < 70% and ΔDLCO > 6% could independently predict unfavorable responses to BPA. Measuring the DLCO dynamically facilitates the identification of patients who might have unsatisfactory hemodynamic results after BPA.
Introduction
Chronic thromboembolic pulmonary hypertension (CTEPH) is characterized by an organic thromboembolic obstruction of proximal pulmonary arteries and secondary microvasculopathy in nonoccluded areas (1). Although pulmonary endarterectomy (PEA) can effectively alleviate thromboembolic obstruction in proximal pulmonary arteries, it cannot address distal lesions. Moreover, 17–51% of patients still suffer from residual pulmonary hypertension after PEA, which is attributed to advanced microvasculopathy (2). Microvasculopathy compromises the pulmonary membrane diffusion capacity, which could be reflected in a decreased diffusing capacity for carbon monoxide (DLCO) (3, 4).
In patients for which PEA is not appropriate, balloon pulmonary angioplasty (BPA) serves as an alternative treatment and could effectively dilate surgically inaccessible pulmonary arteries (5). Similar to PEA, the hemodynamic results of BPA varied among patients, with some patients showing persistent pulmonary hypertension and exercise intolerance even after several BPA sessions (6). Identifying the predictors of hemodynamic response is essential for guiding the clinical practice of BPA, which has received limited research attention. Akizuki et al. reported that BPA had an immediate effect on the DLCO, and that this effect varied with the lung fields (7). Considering its close relationship with microvasculopathy, we hypothesized that the baseline DLCO and the DLCO percent change between the baseline and the period within 7 days after the first BPA session [ΔDLCO = (DLCO after the first BPA session-DLCO before the first BPA session)/DLCO before the first BPA session] could predict the hemodynamic response to BPA.
Materials and Methods
Study Design and Participants
This current retrospective cohort study was performed in Fuwai Hospital, Chinese Academy of Medical Sciences (Beijing, China). The study protocol was approved by the Ethics Committee of Fuwai Hospital (Approval No: 2020-1275). Each patient provided signed informed consent. We screened all patients with CTEPH who underwent BPA from May 2018 to January 2021 at Fuwai Hospital. The diagnosis of CTEPH was consistent with the 2015 European Society of Cardiology/ERS guidelines (8), and eligibility for BPA was determined after a discussion among multidisciplinary specialists, including pulmonary vascular specialists, PEA surgeons, and interventional cardiologists. Patients without DLCO records at the baseline or after the first BPA session and patients without right heart catheterization (RHC) at follow-up were excluded. The pulmonary function test, cardiopulmonary exercise test, 6-min walk distance (6 MWD), World Health Organization functional class (WHO-FC), and echocardiography results were evaluated within a week prior to and after each BPA session. Patients were reevaluated over 3 months after the last BPA session. Clinical data were collected from electronic medical records by two independent reviewers. Any discordance was resolved by the supervisors (ZZ and ZL).
RHC and BPA Procedure
RHC and BPA were performed as previously described (9). RHC was performed prior to each BPA session to obtain the hemodynamics parameters, including the right atrial pressure, right ventricular pressure, mean pulmonary arterial pressure (mPAP), pulmonary arterial wedge pressure, cardiac output (calculated by Fick's method), and oxygen saturation. Mixed venous oxygen saturation (SvO2) and pulmonary vascular resistance (PVR) were calculated in accordance with standard equations (10). A six Fr guiding catheter [Multipurpose (Cordis Corporation, Bridgewater, New Jersey, USA), Amplatz left catheter (Terumo® Heartrail™ II; Terumo Corporation, Tokyo, Japan) or Judkins right catheter (Terumo® Heartrail™ II; Terumo Corporation, Tokyo, Japan)] was introduced to the pulmonary artery through a seven Fr long sheath (Flexor® Check-Flo® Introducer; Cook Medical, Bloomington, IN, USA). The intervention target was determined based on both pulmonary angiography and lung ventilation/perfusion scintigraphy. Then, a 0.014-inch guide wire (Hi-Torque Pilot 50; Abbot, Santa Clara, CA, USA) was introduced across the target lesion, and, subsequently, the balloon was inflated to dilate the target vessel. A 2 mm × 20 mm balloon was initially used to reduce the risk of complications. In repeated dilations, the inflation pressure and balloon size were dynamically adjusted according to the vessel size and vascular response. For patients with mPAP < 30 mmHg, we will increase balloon size up to the reference vessel diameter. For patients with mPAP between 30 and 40 mmHg, we will increase balloon size up to 80% of the reference vessel diameter. For patients with mPAP over 40 mmHg, we prefer to use a small balloon to avoid complications. After each BPA session, the hemodynamics parameters were measured again by RHC.
Pulmonary Function Tests and Cardiopulmonary Exercise Test
Pulmonary function tests and exercise tests were performed using the COSMED Quark CPET system (COSMED, Rome, Italy) as described previously (11). Forced vital capacity, forced expiratory volume in 1 s, and single-breath DLCO were measured according to the American Thoracic Society/European Respiratory Society criteria (12, 13). To eliminate individual differences, the DLCO reported in the present study was the percentage of predicted DLCO. Peak oxygen consumption (VO2@Peak) was defined as the highest 30-s average oxygen consumption after reaching the anaerobic threshold. Both the V-slope method and ventilator equivalents were applied to detect anaerobic thresholds. Ventilatory efficiency was measured by the minute ventilation to the carbon dioxide output (VE/VCO2) ratio and represented by the VE/VCO2 slope during the incremental exercise.
Definition of BPA Responders and Nonresponders
In line with a previous study (14), patients were classified into either “BPA responders” or “BPA nonresponders” according to the hemodynamic results at follow-up after their last BPA session. “BPA responders” were defined as mPAP ≤ 30 mmHg and/or a reduction of PVR ≥ 30%. Otherwise, the patients were classified as “BPA nonresponders.”
Statistical Analysis
Continuous variables are presented as the mean ± standard deviation or median (interquartile range) according to the data distribution. Categorical variables are given as counts (percentages). An independent-sample t-test, the Mann–Whitney U test, the chi-square test, a paired t-test or the Wilcoxon signed rank test was used to compare clinical parameters between groups where appropriate. Two-way analyses of variance were used to compare the DLCO values at the baseline, after the first BPA session and at follow-up with Bonferroni post hoc tests for pairwise comparisons. Spearman correlation coefficients were first calculated to identify variables potentially associated with the ΔDLCO. To adjust for potential confounding factors, variables with a Spearman correlation coefficient at P < 0.05 and those that were clinically significant were further assessed based on a multivariate linear regression analysis (enter method). A receiver operator characteristic curve analysis was performed to identify the optimal cutoff of the baseline DLCO value and ΔDLCO in predicting unfavorable response to BPA as well as the area under the curve (AUC) of each variable. The DeLong test was used for comparisons of the AUC values. Univariate logistic regression was first performed to identify variables potentially associated with an unfavorable response to BPA. Subsequently, variables with a P-value < 0.05 in the univariate models or of clinical significance were included in the multivariate logistic regression model (enter method). A two-sided P-value < 0.05 was considered statistically significant. Statistical analyses were performed with SPSS 25.0 (IBM SPSS Corp.; Armonk, NY, USA), Prism GraphPad 8 (GraphPad Software, LaJolla, CA, USA), and MedCalc (MedCalc 19.7.2 version, MedCalc Inc., Mariakerke, Belgium).
Results
Baseline Demographics
As shown in Figure 1, a total of 126 patients with CTEPH underwent BPA between May 2018 and January 2021. Among them, 51 patients were excluded for no baseline DLCO records (n = 12), no DLCO records after the first BPA session (n = 17) or no reevaluation RHC at follow-up (n = 22). Of the 75 included patients, the mean age was 57.5 ± 11.9 years old and 40 (52.6%) were female. On average, the patients underwent 2.2 ± 1.3 BPA sessions with 14.8 ± 9.4 subsegmental pulmonary vessels dilated, and the median time interval from the baseline to reevaluation RHC was 30 weeks (interquartile range, 15–60 weeks). At follow-up, 45 achieved an mPAP ≤ 30 mmHg and/or a reduction of PVR ≥ 30%, whereas 30 patients failed.
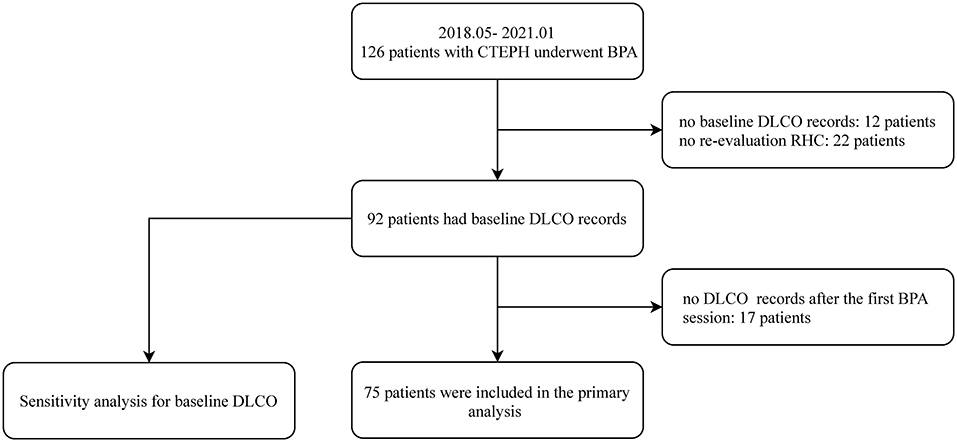
Figure 1. The patient enrollment flow chart. BPA, balloon pulmonary angioplasty; CTEPH, chronic thromboembolic pulmonary hypertension; DLCO, diffusing capacity for carbon monoxide; RHC, right heart catheterization.
BPA Responders vs. Nonresponders
At the baseline, the BPA responders had a significantly higher DLCO (67 ± 12.8 vs. 59.5 ± 13.5%, P = 0.017) than the nonresponders. The rest of the baseline characteristics were comparable between the BPA responders and nonresponders. The details are summarized in Table 1.
During the first BPA session, we found that the number of dilated subsegmental pulmonary vessels was comparable between the BPA responders and nonresponders (5.9 ± 1.7 vs. 5.9 ± 2.2, P = 0.880). We also calculated the proportion of lower lobe vessels (A6–A10) (7) in the dilated subsegmental pulmonary vessels during the first BPA session for each patient. The results showed that the operators mainly dilated lower lobe vessels in both the BPA responder (64.1 ± 20.% of the dilated subsegmental pulmonary vessels) and nonresponder (55.9 ± 25.1% of the dilated subsegmental pulmonary vessels) groups during the first BPA session; moreover, the proportion of lower lobe vessels was comparable between the two groups (P = 0.114). During the first BPA session, BPA nonresponders had a higher incidence of hemoptysis (6.7 vs. 2.2%, P = 0.718) than did responders, although the difference did not reach statistical significance (shown in Table 2).
At follow-up, responders underwent more BPA sessions (2.5 ± 1.4 vs. 1.8 ± 1, P = 0.027) and had more dilated subsegmental pulmonary vessels (16.7 ± 10.4 vs. 11.8 ± 6.9, P = 0.046) than BPA nonresponders. The time interval from the baseline to follow-up tended to be longer in BPA responders (42.5 ± 27.9 vs. 31.4 ± 24.3 weeks, P = 0.124). Reasonably, BPA responders had lower NT-proBNP levels, higher arterial oxygen saturation, and more favorable parameters derived from echocardiography and RHC than nonresponders, although these parameters were comparable at the baseline between the BPA responders and nonresponders. The details are summarized in Table 2.
Effect of BPA on the DLCO
As shown in Figure 2, the time course of DLCO values during the BPA procedure differed between the BPA responders and nonresponders. For the BPA responders, the DLCO decreased within 7 days after the first BPA session (after the first BPA vs. the baseline: 64.3 ± 12.1 vs. 67 ± 12.8%, P = 0.004) and then returned to a level similar to the baseline at follow-up (follow-up vs. baseline: 67.3 ± 13.9 vs. 67 ± 12.8%, P = 0.981). For the BPA nonresponders, the DLCO tended to increase within 7 days after the first BPA session (after first BPA vs. baseline: 60.9 ± 12.1 vs. 59.5 ± 13.5%, P = 0.418), and this trend persisted at follow-up (follow-up vs. baseline: 63.1 ± 13.2 vs. 59.5 ± 13.5%, P = 0.097).
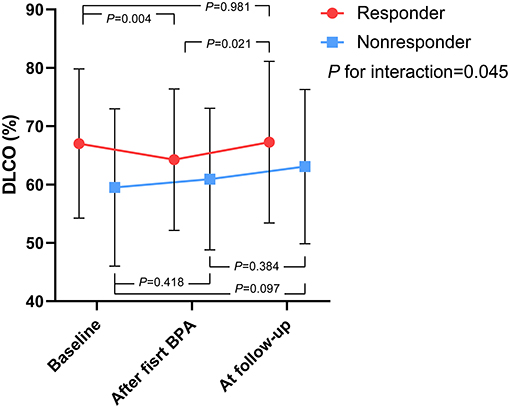
Figure 2. The time course of DLCO values during the BPA procedure, stratified by BPA responders and nonresponders. P for interaction represents the interaction between the outcome of BPA and time course as within-subjects effects. BPA, balloon pulmonary angioplasty; DLCO, diffusing capacity for carbon monoxide.
Parameters Associated With ΔDLCO
The Spearman correlation coefficient showed that the baseline DLCO value (r = −0.340, P = 0.003) was the only variable associated with ΔDLCO among the various demographic, echocardiographic, and hemodynamic parameters. After adjusting for age, sex, body mass index, mPAP, and forced vital capacity percentage change between the baseline and after the first BPA session, the baseline DLCO values were still associated with the ΔDLCO (standardized β coefficient = −0.535, P < 0.001). The details are summarized in Table 3.
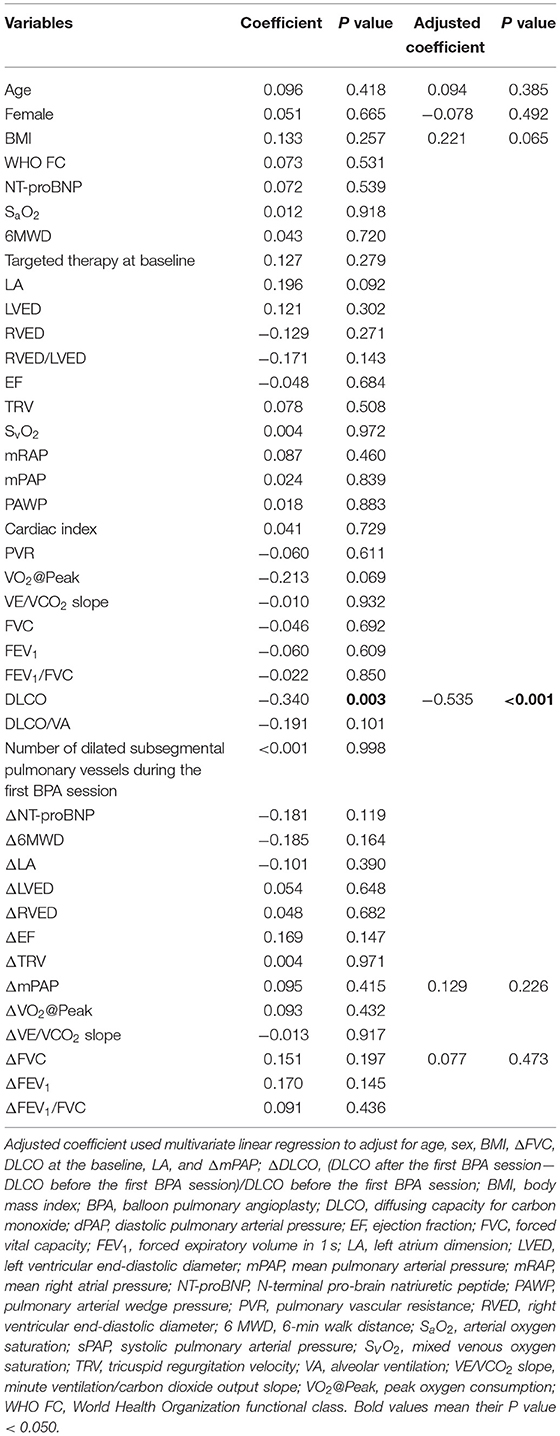
Table 3. Correlation between ΔDLCO and various variables at the baseline or the change of these variables after the first BPA session.
Cutoff Value for DLCO and ΔDLCO in Predicting Unfavorable BPA Response
The receiver operator characteristic curve showed that the best cutoff value in predicting unfavorable BPA response was 70% for DLCO values at the baseline (AUC: 0.628, 95% CI: 0.508–0.737) and 2.4% for ΔDLCO (AUC: 0.683, 95% CI: 0.555–0.812). Previous studies showed that the mean percentage difference between two repeat DLCO measurements ranged from 2.5 to 4.4% in patients without ventilatory dysfunction (15, 16). Meanwhile, our data showed that the mean percentage difference between two repeat measurements of DLCO was 3.5% in patients with pulmonary hypertension [n = 32, the time interval between two repeat measurements should be <7 days, and treatment should not be changed between two repeated measurements (e.g., did not change targeted drug or did not undergo BPA)]. To maximize the generalizability of our conclusion, we reset the cutoff value of ΔDLCO to 6% (AUC: 0.617, 95% CI: 0.497–0.727) (Supplementary Table 1). Accordingly, 51 and 24 patients were classified as DLCO < 70% and DLCO ≥ 70%, respectively; 64 and 11 patients were classified as ΔDLCO ≤ 6% and ΔDLCO > 6%, respectively; and nine patients had DLCO < 70% and ΔDLCO > 6%. Although the AUCs were comparable between the baseline DLCO values and ΔDLCO (P = 0.871), we found that the combination of these two variables (AUC: 0.716, 95% CI: 0.600–0.814) demonstrated a superior AUC than either of the two variables alone (P = 0.041, compared to the baseline DLCO values; P = 0.010, compared to the ΔDLCO) (Figure 3).
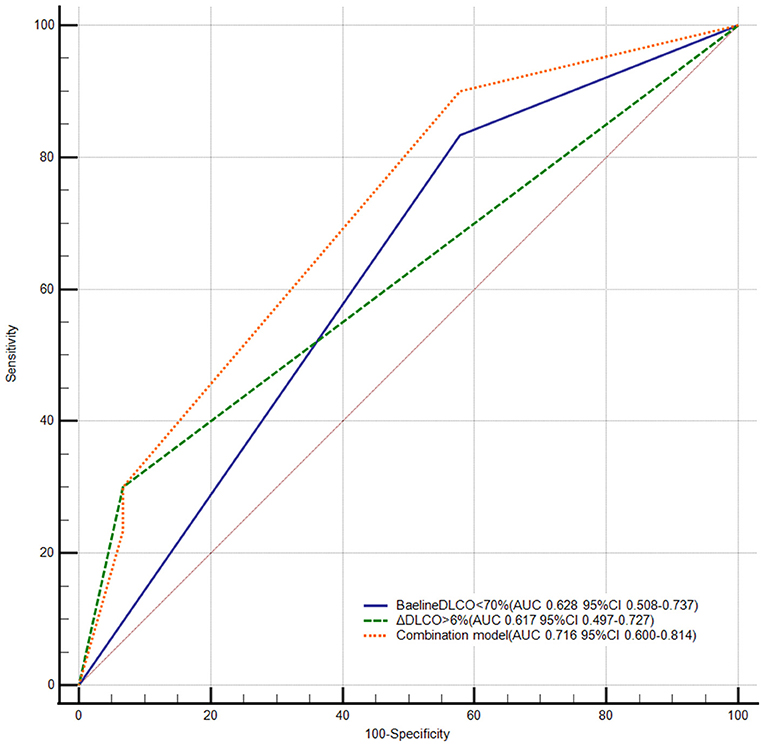
Figure 3. Receiver operator characteristic curve of DLCO in predicting unfavorable response to BPA. BPA, balloon pulmonary angioplasty; DLCO, diffusing capacity for carbon monoxide; ΔDLCO, the percent change of DLCO between the baseline and within 7 days after the first BPA session. DeLong test pairwise comparison: Baseline < DLCO < 70% vs. the combination model P = 0.041; ΔDLCO > 6% vs. the combination model P = 0.010; ΔDLCO > 6% vs. baseline DLCO < 70% P = 0.871.
The clinical characteristics of patients with a baseline DLCO < 70% and DLCO ≥ 70% at the baseline and at follow-up are summarized in Supplementary Tables 2, 3. Compared with the baseline, mPAP and PVR decreased at follow-up in both the baseline DLCO < 70% and DLCO ≥ 70% groups (Figure 4). However, patients with a baseline DLCO ≥ 70% had a significantly higher proportion of BPA responders than those with a DLCO < 70%, although BPA sessions, dilated vessels, and the time interval between the baseline and follow-up were comparable between the two groups (Supplementary Table 3). Similar results were also observed in patients with ΔDLCO ≤ 6% and ΔDLCO > 6% (Figure 4, Supplementary Tables 4, 5).
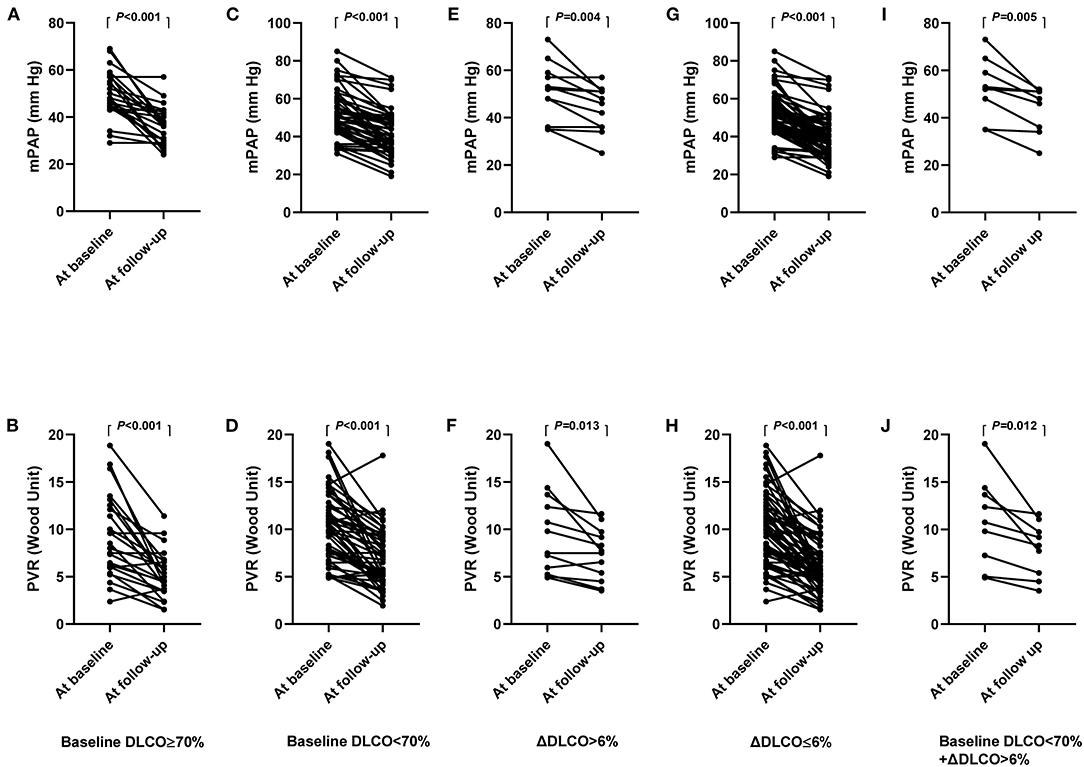
Figure 4. (A–J), hemodynamics at the baseline and follow-up, stratified by baseline DLCO and ΔDLCO. mPAP (A) and PVR (B) in patients with baseline DLCO ≥ 70%. mPAP (C) and PVR (D) in patients with baseline DLCO < 70%. mPAP (E) and PVR (F) in patients with ΔDLCO > 6%. mPAP (G) and PVR (H) in patients with ΔDLCO ≤ 6%. mPAP (I) and PVR (J) in patients with baseline DLCO < 70% and ΔDLCO > 6%. DLCO, diffusing capacity for carbon monoxide; ΔDLCO, the percent change of DLCO between the baseline and within 7 days after the first BPA session. mPAP, mean pulmonary arterial pressure; PVR, pulmonary vascular resistance.
Predictors of Unfavorable Response to BPA
Univariate logistic analysis showed that a baseline DLCO < 70%, ΔDLCO > 6%, BPA session number and dilated pulmonary subsegment number were associated with the BPA response (shown in Table 4). Given their high collinearity with the number of BPA sessions, the number of dilated pulmonary subsegments (r = 0.911, P < 0.001) and the time interval between the baseline and follow-up (r = 0.730, P < 0.001) were excluded from the multivariate logistic regression. After adjusting for the number of BPA sessions, the baseline DLCO < 70% (OR: 4.585, 95% CI: 1.021–20.596, P = 0.047) and ΔDLCO > 6% (OR: 3.666, 95% CI, 1.094–12.290, P = 0.035) were still associated with unfavorable responses to BPA. Further adjustment for the WHO FC, NT-proBNP, and hemodynamic parameters did not attenuate the statistical significance of a baseline DLCO < 70% and ΔDLCO > 6% (shown in Table 5).
Sensitivity Analysis
As shown in Figure 1, 92 patients had baseline DLCO records. We reassessed the predictive value of the baseline DLCO < 70% in these patients. The results showed that the baseline DLCO < 70% was still an independent predictor of unfavorable response to BPA (Supplementary Tables 6–11).
We also compared the baseline characteristics of the included and excluded patients. Except for younger age and better WHO FC in the included patients, exercise tolerance and echocardiographic and hemodynamic parameters were comparable between the included and excluded patients (Supplementary Table 12).
Discussion
In the current study, we demonstrated, for the first time, that the baseline DLCO < 70% and ΔDLCO > 6% were independent predictors of an unfavorable response to BPA. More importantly, the combination of the baseline DLCO < 70% and ΔDLCO > 6% demonstrated a superior predictive ability than either of these two variables alone.
Effect of BPA on the DLCO
We observed the opposite trend in the changes in the DLCO after the first BPA session between BPA responders and nonresponders, although the two groups had similar numbers of dilated subsegmental pulmonary vessels, and both mainly had dilated lower lobe vessels. For BPA responders, the DLCO values decreased after the first BPA session, which was consistent with the work of Akizuki et al. (7), who suggested that, when dilating lower lobe vessels, the elevation of ventilation is disproportional to hemodynamic improvement, which exacerbates the ventilation/perfusion mismatch and results in decreased DLCO (7). In contrast, DLCO values in nonresponders remained unchanged and even tended to increase after the first BPA session (60.9 ± 12.1 vs. 59.5 ± 13.5%, P = 0.418). We provide our own hypothesis for this unique phenomenon. BPA nonresponders had lower baseline DLCO values than responders (Table 1), which indicated that their microvasculopathy was also more advanced. Therefore, nonresponders might have a more dramatic redistribution of pulmonary blood flow from nonoccluded to newly dilated areas after BPA (vascular steal phenomenon) than responders, which has been observed in patients after undergoing PEA and is thought to be related to microvasculopathy (17, 18). Consequently, newly dilated vessels in nonresponders suffered from significantly higher shear stress and wall tension than did responders. Coupled with potential vascular wall damage caused by BPA, red blood cells might leak into alveolar airspaces, which could be overt (hemoptysis) or subclinical. Our hypothesis was further supported by the observation that nonresponders tended to have a higher incidence of hemoptysis than did responders during the first BPA session (6.7 vs. 2.2%, P = 0.718). Previous studies have revealed that alveolar hemorrhage could lead to elevation of DLCO values (19, 20). Taken together, we suggest that the effect of alveolar hemorrhage on DLCO outweighed that of ventilation/perfusion mismatch in BPA nonresponders, which led to the elevation of DLCO values within 7 days after the first BPA session.
We also performed a comprehensive investigation to screen variables potentially associated with the ΔDLCO and spearman coefficient showed that the baseline DLCO value was the only variable associated with the ΔDLCO among the various demographic, echocardiographic, and hemodynamic parameters (Table 3). This association persisted even after adjusting for age, sex, body mass index, and mPAP and forced vital capacity percent change between the baseline and after the first BPA procedure. We thought that the relationship between the baseline DLCO and ΔDLCO might be explained by the fact that they were both external manifestations of microvasculopathy, which further supported our aforementioned hypothesis.
Regarding the long-term effect of BPA on the DLCO (i.e., from “within 7 days after the first BPA session” to “at follow-up),” we found an increasing DLCO trend in both BPA responders and nonresponders. Akizuki et al. (7) also found that the DLCO would decrease in early BPA sessions, which mainly dilated lower lobe vessels and would recover in later BPA sessions, which mainly dilated upper-middle lobe vessels. They suggested that this may be attributed to intervention in the upper-middle lung field, which increases blood flow in these areas and subsequently attenuates ventilation/perfusion mismatch. Similarly, the proportion of upper-middle lobes in subsequent BPA sessions gradually increased in our center (Supplementary Figure S1).
Predictive Value of the Baseline DLCO and ΔDLCO
We found that both the baseline DLCO < 70% and ΔDLCO > 6% could independently predict unfavorable responses to BPA. More importantly, the combination of the baseline DLCO < 70% and ΔDLCO > 6% demonstrated a superior predictive ability than either of these two variables alone, which might also be explained by their associations with microvasculopathy. Previous studies showed that concomitant microvasculopathy had a strong influence on the outcome of PEA (2, 21). Moreover, microvasculopathy is irreversible, and patients with advanced microvascular impairment can still suffer from exercise intolerance and hypoxia even after normalization of pulmonary hemodynamics by PEA or BPA (22, 23). In the present study, the DLCO was comparable between the baseline and follow-up, which was consistent with previous studies (24, 25) and suggested a limited effect of BPA on microvasculopathy.
In addition to the DLCO found in the present study, Tsuji et al. reported that diastolic PAP could also predict residual PH after BPA (6). These authors also thought that the underlying mechanism was attributable to the development of pulmonary microvasculopathy secondary to long-standing pulmonary hypertension. Additionally, Taniguchi et al. reported that poor subpleural perfusion, which also reflects microvasculopathy in patients with inoperable CTEPH, could also predict failure of BPA (14). Compared with diastolic PAP and subpleural perfusion, the DLCO might be of more clinical significance due to its non-invasiveness and wide availability.
Limitation
The current study had several limitations. First, 51 patients were excluded from the primary analysis due to the lack of baseline DLCO records, lack of RHC reevaluation at follow-up, or lack of DLCO records after the first BPA session. However, we performed a sensitivity analysis for the baseline DLCO < 70% in 92 patients, and the results remained unchanged. We also found that the baseline characteristics of the included and excluded patients were comparable. Therefore, the exclusion of these patients should not undermine the conclusions of the current study. Second, the association between the ΔDLCO and microvasculopathy was not pathologically confirmed; thus, further animal or clinical studies are needed. Third, direct evidence for alveolar hemorrhage was not available due to the retrospective nature of the present study. Moreover, potentially subclinical alveolar hemorrhage increases the difficulty of quantifying its impact on the DLCO. Fourth, the number of BPA sessions and dilated vessels were both relatively small for the BPA responders and nonresponders, which means that the nonresponders in our study may convert into responders in future BPA sessions. Furthermore, mPAP and PVR were also decreased significantly in patients with the baseline DLCO < 70% and/or ΔDLCO > 6% after multiple BPA sessions. Thus, the baseline DLCO < 70% and/or ΔDLCO > 6% may not represent a contraindication for BPA. Clinicians could anticipate that patients with the baseline DLCO < 70% and/or ΔDLCO > 6% need more BPA sessions to achieve satisfactory results.
Conclusion
The baseline DLCO < 70% and ΔDLCO > 6% were both independent predictors of unfavorable response to BPA. Measuring the DLCO dynamically could facilitate the identification of patients who might have an unsatisfactory hemodynamic response to BPA.
Data Availability Statement
The original contributions presented in the study are included in the article/Supplementary Material, further inquiries can be directed to the corresponding authors.
Ethics Statement
The studies involving human participants were reviewed and approved by the Ethics Committee of Fuwai Hospital. The patients/participants provided their written informed consent to participate in this study.
Author Contributions
ZL and ZZ contributed to the conception of the study and guarantors of the paper, taking responsibility for the integrity of the work as a whole, from inception to a published article. YZ and XL wrote the manuscript. QZh, QZe, TY, QJ, LY, AD, XM, JL, and CA contributed to data collection. ZL, CX, and QL contributed to the acquisition of funding. All authors contributed to data analysis and interpretation, critically reviewed the manuscript for intellectual content, and had final responsibility for the decision to submit for publication.
Funding
This research article was supported by Beijing Municipal Science and Technology Project [Z181100001718200]; Beijing Municipal Natural Science Foundation [7202168]; CAMS Innovation Fund for Medical Sciences (CIFMS) [2020-I2M-C&T-B-055, 2021-I2M-C&T-B-032]; Double First-Class Discipline Construction Fund of Peking Union Medical College and Chinese Academy of Medical Sciences [2019E-XK04-02]; the Capital's Funds for Health Improvement and Research (CFH) [2020-2-4033, 2020-4-4035]; the Youth Fund of Zhongshan Hospital, Fudan University [Grant No. 2021-016].
Conflict of Interest
The authors declare that the research was conducted in the absence of any commercial or financial relationships that could be construed as a potential conflict of interest.
Publisher's Note
All claims expressed in this article are solely those of the authors and do not necessarily represent those of their affiliated organizations, or those of the publisher, the editors and the reviewers. Any product that may be evaluated in this article, or claim that may be made by its manufacturer, is not guaranteed or endorsed by the publisher.
Supplementary Material
The Supplementary Material for this article can be found online at: https://www.frontiersin.org/articles/10.3389/fcvm.2021.762267/full#supplementary-material
Abbreviations
BPA, Balloon pulmonary angioplasty; CTEPH, chronic thromboembolic pulmonary hypertension; DLCO, diffusing capacity for carbon monoxide; ΔDLCO, Percent change of DLCO between the baseline and within 7 days after the first BPA session; EF, ejection fraction; mPAP, mean pulmonary arterial pressure; NT-proBNP, N-terminal pro-brain natriuretic peptide; PEA, pulmonary endarterectomy; PVR, pulmonary vascular resistance; RHC, right heart catheterization; 6MWD, 6-min walk distance; SVO2, mixed venous oxygen saturation; VE/VCO2 slope, minute ventilation/ carbon dioxide output slope; VO2@Peak, peak oxygen consumption; WHO FC, World Health Organization functional class.
References
1. Kim NH, Delcroix M, Jais X, Madani MM, Matsubara H, Mayer E, et al. Chronic thromboembolic pulmonary hypertension. Eur Respir J. (2019) 53:1801915. doi: 10.1183/13993003.01915-2018
2. Gerges C, Gerges M, Friewald R, Fesler P, Dorfmüller P, Sharma S, et al. Microvascular disease in chronic thromboembolic pulmonary hypertension: hemodynamic phenotyping and histomorphometric assessment. Circulation. (2020) 141:376–86. doi: 10.1161/CIRCULATIONAHA.119.041515
3. Godinas L, Amar D, Montani D, Lau EM, Jais X, Savale L, et al. Lung capillary blood volume and membrane diffusion in precapillary pulmonary hypertension. J Heart Lung Transplant. (2016) 35:647–56. doi: 10.1016/j.healun.2015.12.022
4. Suda R, Tanabe N, Ishida K, Kato F, Urushibara T, Sekine A, et al. Prognostic and pathophysiological marker for patients with chronic thromboembolic pulmonary hypertension: usefulness of diffusing capacity for carbon monoxide at diagnosis. Respirology. (2017) 22:179–86. doi: 10.1111/resp.12883
5. Chen ZW, Wu CK, Kuo PH, Hsu HH, Tsai CH, Pan CT, et al. Efficacy and safety of balloon pulmonary angioplasty in patients with inoperable chronic thromboembolic pulmonary hypertension. J Formos Med Assoc. (2021) 120:947–55. doi: 10.1016/j.jfma.2020.09.016
6. Tsuji A, Ogo T, Ueda J, Fukui S, Morita Y, Fukuda T, et al. Predictors of residual pulmonary hypertension after balloon pulmonary angioplasty in patients with chronic thromboembolic pulmonary hypertension. Int J Cardiol. (2017) 226:118–20. doi: 10.1016/j.ijcard.2016.09.132
7. Akizuki M, Serizawa N, Ueno A, Adachi T, Hagiwara N. Effect of balloon pulmonary angioplasty on respiratory function in patients with chronic thromboembolic pulmonary hypertension. Chest. (2017) 151:643–9. doi: 10.1016/j.chest.2016.10.002
8. Galie N, Humbert M, Vachiery JL, Gibbs S, Lang I, Torbicki A, et al. 2015 ESC/ERS guidelines for the diagnosis and treatment of pulmonary hypertension: the joint task force for the diagnosis and treatment of pulmonary hypertension of the European society of cardiology (ESC) and the European respiratory society (ERS): endorsed by: association for European paediatric and congenital cardiology (AEPC), international society for heart and lung transplantation (ISHLT). Eur Heart J. (2016) 37:67–119. doi: 10.1093/eurheartj/ehv317
9. Jin Q, Zhao ZH, Luo Q, Zhao Q, Yan L, Zhang Y, et al. Balloon pulmonary angioplasty for chronic thromboembolic pulmonary hypertension: state of the art. World J Clin Cases. (2020) 8:2679–702. doi: 10.12998/wjcc.v8.i13.2679
10. Wilkinson JL. Haemodynamic calculations in the catheter laboratory. Heart. (2001) 85:113–20. doi: 10.1136/heart.85.1.113
11. Jin Q, Luo Q, Yang T, Zeng Q, Yu X, Yan L, et al. Improved hemodynamics and cardiopulmonary function in patients with inoperable chronic thromboembolic pulmonary hypertension after balloon pulmonary angioplasty. Respir Res. (2019) 20:250. doi: 10.1186/s12931-019-1211-y
12. Miller MR, Hankinson J, Brusasco V, Burgos F, Casaburi R, Coates A, et al. Standardisation of spirometry. Eur Respir J. (2005) 26:319–38. doi: 10.1183/09031936.05.00034805
13. Macintyre N, Crapo RO, Viegi G, Johnson DC, van der Grinten CP, Brusasco V, et al. Standardisation of the single-breath determination of carbon monoxide uptake in the lung. Eur Respir J. (2005) 26:720–35. doi: 10.1183/09031936.05.00034905
14. Taniguchi Y, Brenot P, Jais X, Garcia C, Weatherald J, Planche O, et al. Poor subpleural perfusion predicts failure after balloon pulmonary angioplasty for nonoperable chronic thromboembolic pulmonary hypertension. Chest. (2018) 154:521–31. doi: 10.1016/j.chest.2018.03.059
15. Dressel H, Filser L, Fischer R, de la Motte D, Steinhaeusser W, Huber RM, et al. Lung diffusing capacity for nitric oxide and carbon monoxide: dependence on breath-hold time. Chest. (2008) 133:1149–54. doi: 10.1378/chest.07-2388
16. Punjabi NM, Shade D, Patel AM, Wise RA. Measurement variability in single-breath diffusing capacity of the lung. Chest. (2003) 123:1082–9. doi: 10.1378/chest.123.4.1082
17. Olman MA, Auger WR, Fedullo PF, Moser KM. Pulmonary vascular steal in chronic thromboembolic pulmonary hypertension. Chest. (1990) 98:1430–4. doi: 10.1378/chest.98.6.1430
18. Moser KM, Metersky ML, Auger WR, Fedullo PF. Resolution of vascular steal after pulmonary thromboendarterectomy. Chest. (1993) 104:1441–4. doi: 10.1378/chest.104.5.1441
19. Martínez-Martínez MU, Oostdam DAH, Abud-Mendoza C. Diffuse alveolar hemorrhage in autoimmune diseases. Curr Rheumatol Rep. (2017) 19:27. doi: 10.1007/s11926-017-0651-y
20. Ewan PW, Jones HA, Rhodes CG, Hughes JM. Detection of intrapulmonary hemorrhage with carbon monoxide uptake. Application in goodpasture's syndrome. N Engl J Med. (1976) 295:1391–6. doi: 10.1056/NEJM197612162952502
21. Galiè N, Kim NH. Pulmonary microvascular disease in chronic thromboembolic pulmonary hypertension. Proc Am Thorac Soc. (2006) 3:571–6. doi: 10.1513/pats.200605-113LR
22. Howden EJ, Ruiz-Carmona S, Claeys M, De Bosscher R, Willems R, Meyns B, et al. Oxygen pathway limitations in patients with chronic thromboembolic pulmonary hypertension. Circulation. (2021) 143:2061–73. doi: 10.1161/CIRCULATIONAHA.120.052899
23. Kikuchi H, Goda A, Takeuchi K, Inami T, Kohno T, Sakata K, et al. Exercise intolerance in chronic thromboembolic pulmonary hypertension after pulmonary angioplasty. Eur Respir J. (2020) 56. doi: 10.1183/13993003.01982-2019
24. Takei M, Kataoka M, Kawakami T, Kuwahira I, Fukuda K. Respiratory function and oxygenation after balloon pulmonary angioplasty. Int J Cardiol. (2016) 212:190–1. doi: 10.1016/j.ijcard.2016.03.061
Keywords: chronic thromboembolic pulmonary hypertension, diffusing capacity for carbon monoxide, balloon pulmonary angioplasty, right heart catheterization, microvasculopathy
Citation: Li X, Zhang Y, Luo Q, Zhao Q, Zeng Q, Yang T, Jin Q, Yan L, Duan A, Liu J, An C, Ma X, Xiong C, Zhao Z and Liu Z (2021) Diffusing Capacity for Carbon Monoxide Predicts Response to Balloon Pulmonary Angioplasty in Patients With Inoperable Chronic Thromboembolic Pulmonary Hypertension. Front. Cardiovasc. Med. 8:762267. doi: 10.3389/fcvm.2021.762267
Received: 21 August 2021; Accepted: 02 November 2021;
Published: 02 December 2021.
Edited by:
Junnan Tang, First Affiliated Hospital of Zhengzhou University, ChinaReviewed by:
Rong Jiang, Tongji University, ChinaZheng-Wei Chen, National Taiwan University, Taiwan
Copyright © 2021 Li, Zhang, Luo, Zhao, Zeng, Yang, Jin, Yan, Duan, Liu, An, Ma, Xiong, Zhao and Liu. This is an open-access article distributed under the terms of the Creative Commons Attribution License (CC BY). The use, distribution or reproduction in other forums is permitted, provided the original author(s) and the copyright owner(s) are credited and that the original publication in this journal is cited, in accordance with accepted academic practice. No use, distribution or reproduction is permitted which does not comply with these terms.
*Correspondence: Zhihui Zhao, MTI1MDE2Nzg5MkBxcS5jb20=; Zhihong Liu, emhpaG9uZ2xpdWZ1d2FpQDE2My5jb20=
†These authors have contributed equally to this work and share first authorship