- 1Department of Nuclear Medicine, National Taiwan University Hospital, National Taiwan University College of Medicine, Taipei, Taiwan
- 2Institute of Epidemiology and Preventive Medicine, College of Public Health, National Taiwan University, Taipei, Taiwan
- 3Department of Internal Medicine, National Taiwan University Hospital, National Taiwan University College of Medicine, Taipei, Taiwan
- 4Department of Nuclear Medicine, Far Eastern Memorial Hospital, New Taipei City, Taiwan
- 5Division of Cardiology, Cardiovascular Medical Center, Far Eastern Memorial Hospital, New Taipei City, Taiwan
- 6National Yang Ming Chao Tung University School of Medicine, Taipei, Taiwan
Background: The aim of this study was to determine whether, and if so how, attenuation correction (AC) improves the diagnostic performance of myocardial perfusion imaging (MPI) in different coronary artery-supplied territories, using coronary angiography as the reference standard.
Methods: PubMed and EMBASE were searched until December 2020 for studies evaluating AC MPI for the diagnosis of coronary artery disease (CAD) with vessel-based data. Methodological quality was assessed using the Quality Assessment of Diagnostic Accuracy Studies tool. For each study, the sensitivity, specificity, diagnostic odds ratios and areas under summary receiver operating characteristic curves (AUC) with 95% confidence intervals were calculated to determine the diagnostic accuracy of AC compared to non-AC MPI. A bivariate mixed-effects model was used to pool the data. Subgroup analyses considering the type of radiotracer and type of AC were performed.
Results: A total of 264 articles were screened, of which 22 studies (2,608 patients) were enrolled. Significant improvements in specificity [0.78 vs. 0.58 in overall CAD, 0.87 vs. 0.61 in right coronary artery (RCA)] and diagnostic odds ratios (16 vs. 8 in overall CAD, 18 vs. 7 in RCA) after AC were shown in overall CAD at a patient level and RCA stenosis. Improvements in AUC were also noted. MPI had a similar diagnostic performance for detecting left anterior descending and left circumflex coronary artery stenosis with or without AC. There were trends of decreased sensitivity after AC, but none were significant. Diagnostic odds ratio showed significant improvement after AC only in the technetium-99m subgroup.
Conclusion: The results of this study suggest that AC should be applied to MPI to improve the diagnosis of CAD regardless of which type of radiotracer, and that AC MPI can improve the specificity of detecting RCA stenosis.
Introduction
Coronary artery disease (CAD) is a major cause of mortality and morbidity worldwide. Mortality and morbidity due to CAD are associated with the number of territories at risk, and are highest for left main and proximal left anterior descending (LAD) coronary artery lesions, lowest for right coronary artery (RCA) disease, and intermediate for non-proximal LAD and left circumflex artery (LCX) disease (1–4). Choosing the correct target vessel for intervention is crucial when performing coronary angiography (CAG) and interventional procedures, especially in multi-vessel disease (5–7).
Myocardial perfusion imaging (MPI) with single photon emission computed tomography (SPECT) is a well-established diagnostic tool for CAD, and it has been shown to have value in treatment decision-making before CAG (8, 9). However, attenuation artifacts may cause misinterpretation and decrease the diagnostic accuracy (10). Attenuation artifacts in MPI are caused by the different densities of various thoracic tissues, resulting in different attenuation patterns in different coronary territories (10). Although attenuation correction (AC) was developed and suggested to overcome this artifact, was not routinely done in clinical practice (11). Also, the performance of AC is different in each part of the myocardium, and sex differences also exist (12). The magnitude of the attenuation effect are less intense at higher energy radiotracers (10). Whether higher energy radiotracer like technetium-99m [99mTc] needed AC is a question.
Previous studies have evaluated the effect of AC in the patient-based diagnostic performance for CAD and assessed its effect in individual coronary arteries with stenosis. For example, some studies have reported that AC can increase the specificity of detecting RCA stenosis (13–15), however the results have been inconsistent with regards to LAD and LCX disease. One study reported no difference in specificity (13), another showed decreased specificity (15), and another showed increased specificity (14). Furthermore, another study showed increased diagnostic sensitivity in three vessel territories, increased specificity in the LAD and LCX, but no change in the RCA (16). Significant discordances were noted between these studies. Moreover, most MPI studies have been heterogeneous with rather small study populations.
Therefore, the aim of this study was to evaluate the diagnostic performance of MPI at a vessel-based level using a meta-analysis method, and compare AC and non–attenuation corrected (NAC) MPI, using CAG as the reference standard. In addition, subgroup analyses (considering the type of radiotracer, 99mTc vs. thallium-201 [201Tl], radionuclide source AC [RAC] vs. computed tomography AC [CTAC] and definition of CAD, 50% vs. 70% stenosis) were performed.
Materials and Methods
Search Strategy
PubMed and EMBASE were searched for English-language and human studies from inception to 1st December 2020 for studies evaluating AC MPI for the diagnosis of CAD with vessel-based data provided. The search keywords included myocardial perfusion, SPECT, and AC. Conference articles were excluded as most did not provide precise data [i.e., true positives (TP), false positives (FP), false negatives (FN), and true negatives (TN)]. Medical Subject Headings terms were used to maximize the sensitivity of the search.
Data Extraction
The search and selection strategies were carried out in accordance with the Preferred Reporting Items for Systematic Reviews and Meta-Analyses (PRISMA) guidelines (17). The detailed review protocol was registered on PROSPERO (registration number: CRD42019124218) (18). Available from: https://www.crd.york.ac.uk/prospero/display_record.php?ID=CRD42019124218. Article titles and abstracts were reviewed for eligibility. The inclusion criteria were studies in which: (1) AC MPI was assessed as a diagnostic tool to evaluate patients for the presence of at least one coronary artery stenosis; (2) coronary artery stenosis was defined as ≥50% diameter stenosis on CAG; (3) CAG was used as the reference test; and (4) the absolute numbers of TP, FP, TN, and FN were available or these data were derivable from the results presented. The exclusion criteria were: (1) studies with duplicate subject enrollment; (2) technology, image quality, review, or continuing education studies; (3) studies with no diagnostic performance or vessel-based data provided; (4) studies that used a reference test other than CAG; (5) and studies in which TP, FP, TN, and FN data could not be extracted. We checked all references in the included articles manually, and articles which met the inclusion criteria were also enrolled.
Data Extraction
Two researchers (JYH and CKH) independently performed data extraction. The extracted information included author, journal, year of publication, and country; details of the study design; patient demographic features (such as numbers of patients, mean age, percentage of males, and indication for MPI); imaging technique (such as type of AC, type of perfusion radiotracer, type of stress); imaging protocol (scatter correction, gated); brand of imaging device and interpretation method; definition of CAD; and numbers of TP, FP, TN, and FN at a patient level and at each coronary artery level. If a study reported more than one pair of sensitivities and specificities at different cutoff points, different imaging techniques, different CAD definitions, or different experienced interpreters, the pair reported in the abstract (19) and the pair with the highest sensitivity (16, 20) were extracted. Disagreements between the two researchers were resolved by consensus.
Quality Assessment
Methodological quality was assessed using the Quality Assessment of Diagnostic Accuracy Studies (QUADAS; scale, 0–14) tool (21). We chose QUADAS due to its clearer definitions and answers to assessment questions. In brief, the QUADAS tool is comprised of 14 items: covered patient spectrum, reference standard, disease progression bias, verification bias, review bias, clinical review bias, incorporation bias, test execution, study withdrawals, and indeterminate results. A score of 7–14 is considered to be high quality, and a score below 7 low quality.
Statistical Analysis
All data from each eligible study were extracted. Continuous variables were expressed as mean values, and categorical variables were expressed as percentages. On the basis of extracted 2 by 2 contingency tables, pooled estimates for diagnostic performance, including sensitivity, specificity, diagnostic odds ratio (DOR), summary receiver-operating characteristic (ROC) curve, and area under the curve (AUC) with 95% confidence intervals (CIs) were calculated. Between-study statistical heterogeneity was assessed using I2 and the Cochrane Q test on the basis of mixed-effects analysis (22). Publication bias was examined using the effective sample size funnel plot and associated regression test of asymmetry described by Deeks et al., with a P-value of <0.10 for the slope coefficient indicating significant asymmetry (23). Diagnostic performance estimates across studies and comparisons between different index tests were analyzed using a bivariate mixed-effects regression model at the patient level and at each coronary artery level (24, 25).
The assumption of the bivariate model is that the sensitivities and specificities (after logit transformation) from each individual study within the meta-analysis are approximately normally distributed around a mean value, with a certain amount of variability around this mean, resulting in bivariate normal distribution. We analyzed the bivariate model using linear mixed-model techniques. The parameters of the bivariate model were estimated in a single model to incorporate correlations between sensitivities and specificities. The summary ROC curves were also created using this model (24).
Additional subgroup analyses were performed, classified by the type of AC (CTAC or RAC), type of radiotracer (201Tl or 99mTc) and definition of CAD (50% or 70% stenosis). All statistical analyses were performed using STATA (version 14; StataCorp LP, College Station, TX) and SAS (version 9.4; SAS Institute Inc., Cary, NC).
Results
Study Characteristics
Two hundred and twenty-seven and 198 articles limited to humans and the English language and excluding conference articles were retrieved through the searches of PubMed and EMBASE, respectively (Figure 1). After excluding duplicate articles, 264 articles remained. On the basis of title and abstract, an additional 196 articles were excluded. After full text review, 49 additional articles were excluded, and the reasons are shown in Figure 1. Three studies were included after manually checking the reference lists (19, 26, 27). The flowchart of study selection is shown in Figure 1.
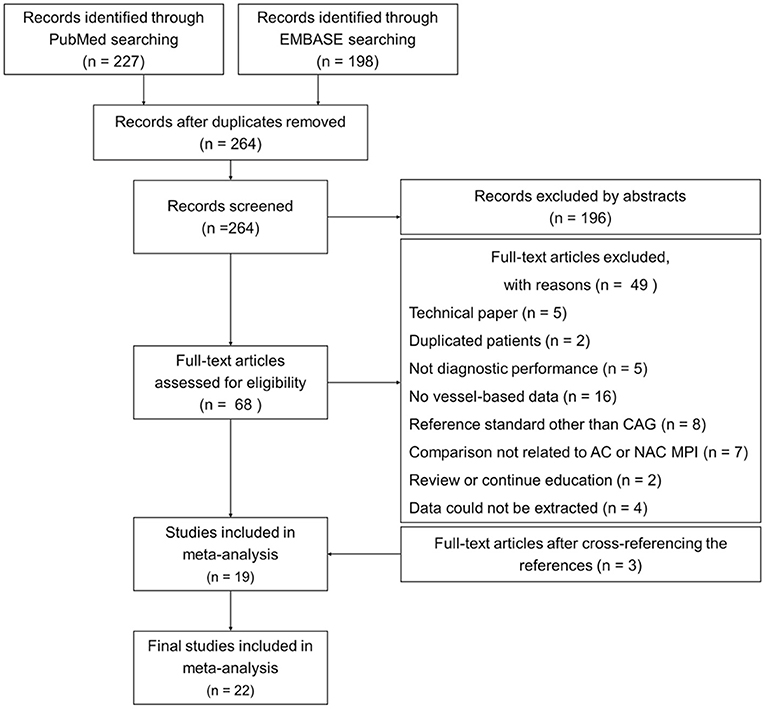
Figure 1. Flowchart of study selection. A total of 22 studies (2,608 patients) were included in the final analysis. AC, attenuation correction; CAG, coronary angiography; MPI, myocardial perfusion imaging; NAC, non-AC.
The final analysis included 22 studies (2,608 patients), of which 18 studies (2,428 patients) reported data on CAD at a patient-level, 21 studies (2,504 patients) reported data on LAD ischemia, 20 studies (2,448 patients) reported data on LCX ischemia, and 22 studies (2,606 patients) reported data on RCA ischemia. Five studies used 201Tl (13, 14, 28–30), 15 studies used 99mTc, and two studies used dual radiotracers (19, 27). Twelve studies used CTAC, seven studies used RAC (19, 27, 29, 31–34), two studies used AC with segmentation of scatter and photo-peak window data (SSPAC) (35, 36), and one study used computer-generated Chang AC (30). The detailed characteristics of each study are shown in Table 1 (13–16, 19, 20, 26–41).
The methodological quality of the 22 studies was assessed using the QUADAS tool. All of the studies were scored above 7, indicating good quality (21). Most studies were found to have problems with unclear masking during interpretation of the reference test, masked reading of the index test, lack of reporting for un-interpretable results, and no explanation for withdrawals in QUADAS assessment, which may have resulted in bias.
The differences between the studies could potentially have affected the pooled diagnostic performance of MPI. The I2 index showed substantial heterogeneity with regards to sensitivity and specificity for all index tests. The highest was 95.92% for measuring the specificity of detecting LCX stenosis with CTAC, and the lowest was 39.22% for measuring the specificity of detecting LAD stenosis with RAC. To assess publication bias, we chose the performance in detecting RCA stenosis with all AC data. This was because attenuation and AC are known to affect the RCA territory the most, and also because all of the included studies reported data on detecting RCA stenosis. Funnel plots and regression tests showed a statistically non-significant P-value (P = 0.14) for the slope coefficient, indicating symmetry in the data and a low likelihood of publication bias (23).
Diagnostic Performance
The pooled estimates representing diagnostic performance are shown in Table 2. There were no significant differences in pooled sensitivities across the studies, ranging from 0.64 to 0.82 for diagnosing CAD at a patient level or detecting LAD, LCX, or RCA stenosis. The pooled specificities across the studies were 0.78 (95% CI, 0.71–0.83), 0.82 (0.75–0.86), 0.74 (0.62–0.83), and 0.58 (0.48–0.67) for all AC, CTAC, RAC, and all NAC, respectively, when diagnosing CAD at a patient level; 0.81 (0.75–0.86), 0.82 (0.72–0.89), 0.80 (0.76–0.84), and 0.79 (0.70–0.85) for all AC, CTAC, RAC, and all NAC when detecting LAD stenosis; 0.91 (0.83–0.95), 0.89 (0.76–0.96), 0.92 (0.83–0.96), and 0.86 (0.76–0.92) for all AC, CTAC, RAC, and all NAC when detecting LCX stenosis; and 0.87 (0.82–0.91), 0.88 (0.79–0.93), 0.87 (0.82–0.90), and 0.61 (0.51–0.71) for all AC, CTAC, RAC, and all NAC when detecting RCA stenosis. The pooled DORs, which are regarded as being minimally affected by verification bias, were 16 (11–24), 15 (10–22), 16 (8–33), and 8 (6–12) for all AC, CTAC, RAC, and all NAC, respectively, when diagnosing CAD at a patient level; 13 (9–20)s, 15 (9–26), 11 (6–21), and 9 (6–13) for all AC, CTAC, RAC, and all NAC when detecting LAD stenosis; 18 (10–32), 15 (7–33), 20 (8–52), and 11 (6–20) for all AC, CTAC, RAC, and all NAC when detecting LCX stenosis; and 18 (11–29), 18 (9–36), 19 (10–37), and 7 (5–10) for all AC, CTAC, RAC, and all NAC when detecting RCA stenosis.
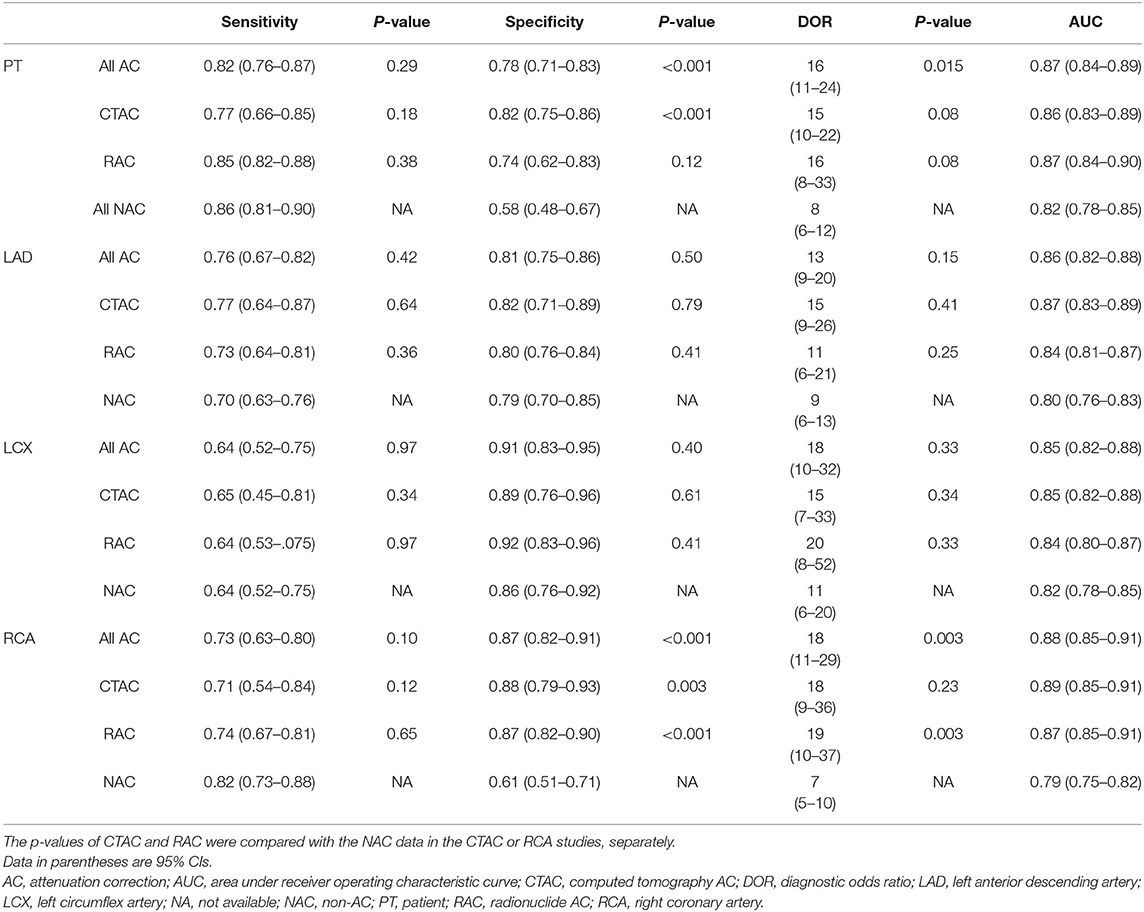
Table 2. Diagnostic performance of MPI, pooled sensitivity, specificity, diagnostic OR and area under the receiver operating characteristic curve of all AC, CTAC, RAC, and NAC in diagnosing CAD at a patient level and detecting LAD, LCX, and RCA stenosis.
The summary ROC curves for diagnosing CAD at a patient level and detecting LAD, LCX and RCA stenosis are shown in Figures 2–5. The AUCs for all AC, CTAC, RAC, and all NAC were 0.87 (0.84–0.89), 0.86 (0.83–0.89), 0.87 (0.84–0.90), and 0.82 (0.78–0.85), respectively, when diagnosing CAD at a patient level; 0.86 (0.82–0.88), 0.87 (0.83–0.89), 0.84 (0.81–0.87), and 0.80 (0.76–0.83) when detecting LAD stenosis; 0.85 (0.82–0.88), 0.85 (0.82–0.88), 0.84 (0.80–0.87), and 0.82 (0.78–0.85) when detecting LCX stenosis; and 0.88 (0.85–0.91), 0.89 (0.85–0.91), 0.87 (0.85–0.91), and 0.79 (0.75–0.82) when detecting RCA stenosis.
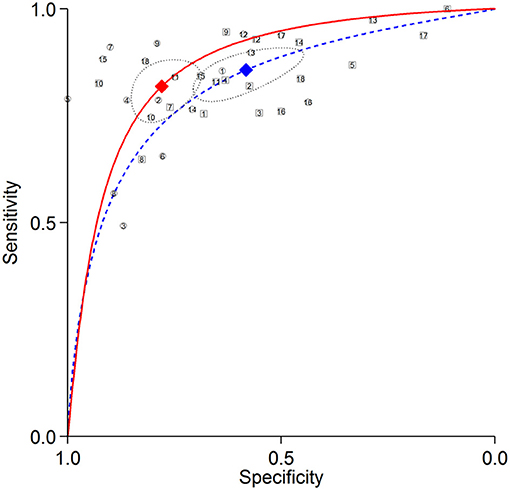
Figure 2. Summary ROC curves of diagnosing CAD at a patient level. Comparisons of summary ROC curves between all AC (solid line) vs. all NAC (dashed line). Each circle (AC) and square (NAC) represent an individual included study. Diamonds represent summary operating points of pooled sensitivity and specificity. AC, attenuation correction; CAD, coronary artery disease; NAC, non-AC; ROC, receiver operating characteristic.
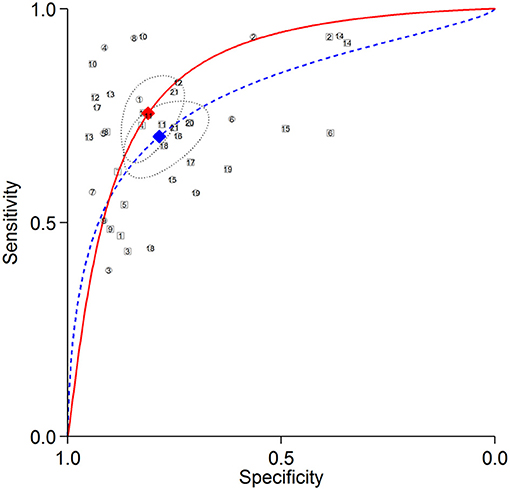
Figure 3. Summary ROC curves of detecting LAD stenosis. Comparisons of summary ROC curves between all AC (solid line) vs. all NAC (dashed line). Each circle (AC) and square (NAC) represent an individual included study. Diamonds represent summary operating points of pooled sensitivity and specificity. AC, attenuation correction; CAD, coronary artery disease; LAD, left anterior descending artery; NAC, non-AC; ROC, receiver operating characteristic.
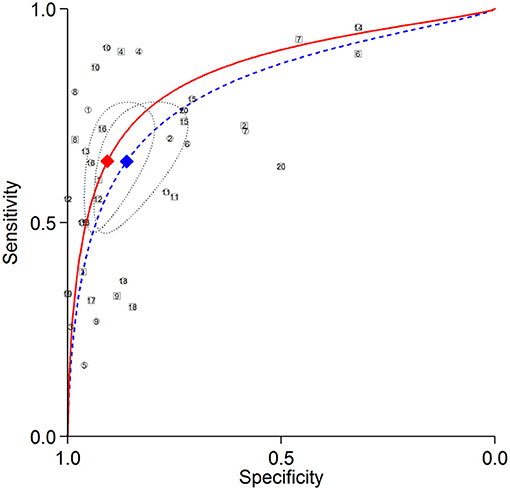
Figure 4. Summary ROC curves of detecting LCX stenosis. Comparisons of summary ROC curves between all AC (solid line) vs. all NAC (dashed line). Each circle (AC) and square (NAC) represent an individual included study. Diamonds represent summary operating points of pooled sensitivity and specificity. AC, attenuation correction; CAD, coronary artery disease; LCX, left circumflex artery; NAC, non-AC; ROC, receiver operating characteristic.
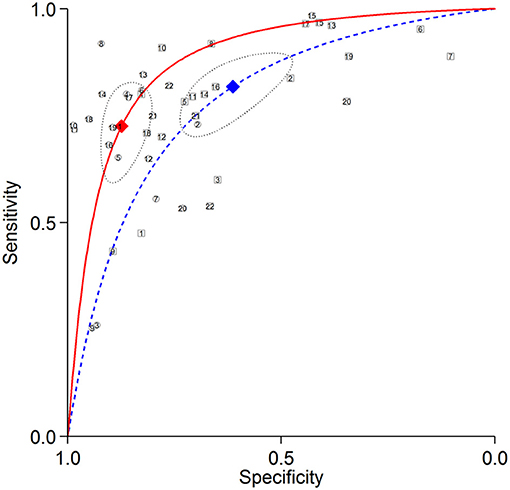
Figure 5. Summary ROC curves of detecting RCA stenosis. Comparisons of summary ROC curves between all AC (solid line) vs. all NAC (dashed line). Each circle (AC) and square (NAC) represent an individual included study. Diamonds represent summary operating points of pooled sensitivity and specificity. AC, attenuation correction; CAD, coronary artery disease; NAC, non-AC; RCA, right coronary artery; ROC, receiver operating characteristic.
There was no significant difference in sensitivity between AC (including all AC, CTAC, and RAC) and NAC. There were significant differences in specificity between AC and NAC in diagnosing CAD at a patient level and detecting RCA stenosis, however no significant differences were noted in detecting LAD and LCX stenosis. When the diagnostic value was represented by DOR, significant differences between AC and NAC were also noted in diagnosing CAD at a patient level and detecting RCA stenosis.
We also analyzed the pooled diagnostic performance of studies with an interval between the index MPI and CAG within 3 months. 16 studies were included in the analysis and showed the same result as the whole meta-analysis with significant improvement of specificity and DOR after AC in diagnosing CAD at a patient level and detecting RCA stenosis. There were no significant differences in detecting LAD and LCX stenosis, either in sensitivity, specificity or DOR. The pooled estimates representing diagnostic performance of studies with interval between MPI and CAG within 3 months are shown in Supplementary Table 1.
CTAC vs. RAC
The results of subgroup analysis of the type of AC were similar to those of the included studies as a whole (details are shown in Supplementary Table 2). There were no significant differences in pooled sensitivities between AC and NAC in the CTAC and RAC subgroups, or in diagnosing CAD at a patient level and detecting LAD, LCX, or RCA stenosis. Increased specificity after AC was noted in diagnosing CAD at a patient level and detecting RCA stenosis. However, a significant difference was noted only in the CTAC subgroup, but not in the RAC subgroup when diagnosing CAD at a patient level. Significant differences were noted in both CTAC and RAC subgroups when detecting RCA stenosis. Increased DOR after AC in both subgroups was noted in diagnosing CAD at a patient level and in detecting LAD, LCX, and RCA stenosis. However, a significant difference was noted only in the RAC subgroup when detecting RCA stenosis. Summary ROC curves are showed in Supplementary Figure 1. Better AUCs were noted in both the CTAC and RAC subgroups. The differences in AUCs were most obvious in detecting RCA stenosis in both the CTAC and RAC subgroups.
201Tl vs. 99mTc
Subgroup analysis of the type of radiotracer was only done in detecting LAD, LCX and RCA stenosis, because only two studies used 201Tl as the tracer and also provided data of diagnosing CAD at a patient level (14, 30). The two studies which used dual tracers were classified into the 99mTc subgroup. Ficaro et al. used a 99mTc/201Tl stress/rest protocol, and it is well-known that the major effect of a stress MPI protocol is seen when diagnosing CAD (19). Of the 112 patients in Links' study, only 16 used 201Tl as the emission tracer (27). Significant improvements in specificity after AC were noted in both 201Tl and 99mTc subgroups only when detecting RCA stenosis. DOR showed significant improvement after AC only in the 99mTc subgroup (p = 0.012) when detecting RCA stenosis. There was an improvement in DOR in the 201Tl subgroup when detecting RCA stenosis, but it was not statistically significant (p = 0.24). Detailed data are shown in Supplementary Table 3, and summary ROC curves are shown in Supplementary Figure 2.
Definition of CAD, 50% vs. 70% Stenosis
The results of subgroup analysis of the definition of CAD of either 50%, 70% stenosis were similar to those of the included studies as a whole (details are shown in Supplementary Table 4).
Increased specificity after AC in both subgroups was noted in diagnosing CAD at a patient level and in detecting LAD, LCX, and RCA stenosis. However, a significant difference was noted only in the 70% stenosis subgroup when diagnosing CAD at a patient level. Significant differences were noted in both 50% and 70% stenosis subgroups when detecting RCA stenosis. Increased DOR after AC in both subgroups was noted in diagnosing CAD at a patient level and in detecting LAD, and RCA stenosis. However, significant differences were noted only in 70% stenosis subgroup when diagnosing CAD at a patient level and RCA stenosis. Borderline significance (p = 0.05) was noted in the 50% stenosis subgroup when detecting RCA stenosis.
Discussion
This study showed that AC significantly improved the specificity of detecting RCA disease compared to NAC. Significant improvements were also seen in the subgroup analyses of CTAC vs. NAC and RAC vs. NAC, however no significant differences in sensitivity were noted between all AC, CTAC, RAC, and NAC. Regarding the DOR in the detection of RCA stenosis, significant differences between all AC vs. NAC and RAC vs. NAC were noted. In addition, there was no significant change in the diagnostic performance after AC for LAD and LCX stenosis.
The latest meta-analysis evaluating the diagnostic performance of MPI was conducted by Xu et al. (42). They performed a meta-analysis evaluating the diagnostic performance of cardiac magnetic resonance (CMR), MPI and positron emission tomography (PET) and reported that MPI had a pooled sensitivity of 0.83 and a pooled specificity of 0.77. Xu also performed a vessel-based analysis, in which MPI showed a pooled sensitivity of 0.71 and specificity of 0.84 at a vessel level. Only 6.7% of the included articles used MPI with AC. However, no definite comparisons between the diagnostic performance of MPI with or without AC were performed, and the diagnostic accuracy in each diseased coronary artery was not mentioned. We hypothesized that the relatively lower specificity of MPI comparing with CMR and PET can be improved after AC.
Nudi et al. evaluated the diagnostic performance of MPI specifically using a cadmium-zinc-telluride (CZT) camera, and reported that CZT-MPI had satisfactory sensitivity of 0.84, but only a suboptimal specificity of 0.69 for diagnosing angiographically significant CAD (43). The suboptimal specificity may be related to attenuation artifacts. Only two of their included studies used a CZT camera equipped with CT, and the pooled estimates were similar to the NAC results of the current meta-analysis. We hypothesized that additional CTAC would also improve the specificity of CZT-MPI. With the development of newer cameras, the CT in SPECT/CT can also perform anatomic imaging of coronary arteries. Combining anatomic and functional hybrid imaging can overcome the limitations inherent to anatomic and functional testing alone, and allow for a more accurate diagnosis and guidance for interventions in patients with CAD (11, 44).
Takx et al. conducted a meta-analysis to evaluate the diagnostic accuracy of MPI, echocardiography, CMR, PET, and CT compared with invasive CAG with fractional flow reserve (FFR) for the diagnosis of hemodynamically significant CAD. Stress MPI showed a pooled sensitivity of 0.74 and pooled specificity of 0.79 at a patient level, and a pooled sensitivity of 0.61 and pooled specificity of 0.84 at a vessel level (45). In their meta-analysis, four studies used AC, five studies did not use AC, and one study did not specify whether AC was used. Comparisons between the diagnostic performance of MPI with or without AC and the diagnostic accuracy in each diseased coronary artery were still not performed. Danad et al. conducted a meta-analysis evaluating the diagnostic performance of MPI, stress echocardiography, invasive CAG, coronary computed tomography angiography (CCTA), FFR derived from CCTA, and CMR when directly compared with FFR done during CAG as the reference standard. MPI showed a pooled sensitivity of 0.70 and pooled specificity of 0.78. Danad also performed a vessel-based analysis, in which MPI showed a pooled sensitivity of 0.57 and pooled specificity of 0.75 at a vessel level (46). However, the diagnostic performance of detecting each stenosed coronary artery was still not mentioned. There was low sensitivity in vessel-based analysis compared with the current study. This may be because only six studies (282 patients) were included and with a prevalence of multi-vessel CAD ranging from 28% to 100%. Multi-vessel CAD might be missed by MPI due to balanced ischemia; however, detailed imaging protocols (such as AC or NAC) were not mentioned in data extraction. Danad et al. also performed the prospective PACIFIC trial, evaluating the diagnostic accuracy of CCTA, MPI, and PET compared with FFR. MPI showed a relatively low sensitivity of 0.57 and a very high specificity of 0.94. They claimed that the high specificity may be due to the use of CTAC (47). After applying the real gold-standard (FFR), the sensitivity of MPI seemed to be lower than using CAG as reference alone.
Only one study used CZT detector was included in our meta-analysis. CZT cameras had high spatial resolution, photon sensitivity, better image quality and can provide more accurate depiction of myocardial perfusion. Although, there were different attenuation patterns between CZT and traditional SPECT scanners (48) and the diagnostic performance of the CZT camera was already great without AC (49), the application of AC can also improve diagnostic performance, regarding specificity (39).
No previous meta-analysis has evaluated the diagnostic performance of MPI in the three coronary arteries individually. Differences in the diagnostic performance between patient level and vessel level have been shown in several studies (46, 50). Contemporary non-invasive imaging should not only focus on diagnosing CAD as a whole, but also evaluate the vascular distribution of disease (51). The diagnostic performance of MPI of the three coronary arteries can provide information on the burden of ischemia in CAD.
Both CTAC and RAC significantly improved the specificity of detecting RCA stenosis, however only CTAC significantly improved the specificity of diagnosing CAD at a patient level. Recently, with the development of new collimators, reconstruction methods and imaging protocols, the CTAC radiation dose has been significantly reduced while preserving image quality and quantitative measurements (52, 53). The dose reduction is greatest for larger patients (54), in whom AC is most commonly required (14).
In our subgroup analysis of different tracers, although a significant improvement in specificity after AC was noted in both subgroups when detecting RCA stenosis, there was only a significant improvement in DOR after AC in the 99mTc subgroup. The effects of attenuation are more intense at lower energies (10), such as with 201Tl. Therefore, it is commonly assumed that attenuation artifacts may not be as much of an issue when using 99mTc as a tracer in MPI. However, in our study, AC significantly improved specificity in both subgroups and improved DOR only in the 99mTc subgroup when detecting RCA stenosis. PET was generally considered to be a better modality for detection of CAD, AC was applied inherently (55). Consequently, we recommend routine AC when performing MPI, regardless of which type of tracer or whether ECG-gated is used.
Our previous meta-analysis focused on different imaging techniques (AC vs. NAC) in MPI, but only in diagnosing the existence of CAD at a patient level (56). The accuracy of identifying territories in jeopardy is clinically important, however it has not been reported before. This is the first study to report the sensitivity, specificity, DOR and AUC of MPI with and without AC in different coronary arteries. We included 22 studies with 2,608 patients which evaluated both AC and NAC results in the same participants. Statistical comparisons between two different index tests require direct comparisons between the same individual data. No publication bias was identified among the selected studies, and therefore the results can be considered robust.
This study has several limitations. First, heterogeneity was noted among the included studies. In order to generalize our results, we have to broad the included studies to all studies that evaluate the effect of AC in individual coronary artery stenosis, there might be some substantial heterogeneities between the included studies. However, all of the included studies followed the guidelines of the American Society of Nuclear Cardiology and the American Heart Association, and the diagnostic performance of MPI remained acceptable and stable regardless of the protocols. Second, the included studies mainly used visual analysis and lacked a standard semiquantitative method. Third, verification bias existed because the results of MPI, which may have affected referral to invasive CAG. Only one study performed CAG irrespective of the MPI results (27). Fourth, non-perfusion parameters or ECG-gated information was not routinely used for interpretation, which may have underestimated the diagnostic accuracy of the study. Therefore, the true diagnostic performance of MPI could be better than the reported estimates after combining these parameters. Also, the information from ECG-gated and AC cannot be replaced by each other (40, 57). Fifth, none of the included studies used FFR analysis in addition to quantitative CAG as the reference standard. However, quantitative CAG alone does not predict the functional significance of coronary lesions or micro-vascular disease (58). Sixth, subgroup analysis to define which category of patients, such as gender or body habitus, may benefit most from AC could not be established, due to lack of individual data from the included articles. Seventh, only one CZT study was included and there is a concern to generalize our findings in this newer scanner (39). However, Caobelli's results were concordant to our main findings. Finally, differences between the AUCs of AC and NAC could not be calculated because of a lack of information regarding the relationships between AC and NAC in patients with and without CAD. Therefore, we calculated DORs as acceptable substitutes.
Conclusion
The results of this study suggest that AC should be applied to MPI to improve the diagnosis of CAD, and that AC MPI can improve the specificity of detecting RCA stenosis.
Data Availability Statement
The original contributions presented in the study are included in the article/Supplementary Material, further inquiries can be directed to the corresponding author/s.
Author Contributions
J-YH, K-LC, and Y-WW conceived and designed this study. J-YH, C-KH, and R-FY were responsible for analysis and interpretation of the data. J-YH and C-KH were responsible for writing the manuscript. K-LC and Y-WW took part in manuscript review and revision. All authors contributed to the article and approved the submitted version.
Funding
This study was partly supported by grants MOST 107-2314-B-418-006-MY3, MOST 108-2314-B-418-002-MY3 (from Y-WW), MOST 106-2314-B-002−049-MY3, MOST 109-2314-B-002-253, and MOST 110-2314-B-002-141 (from J-YH) from the Ministry of Science and Technology of Taiwan. The funders had no role in study design, data collection and analysis, decision to publish, or preparation of the manuscript.
Conflict of Interest
The authors declare that the research was conducted in the absence of any commercial or financial relationships that could be construed as a potential conflict of interest.
Publisher's Note
All claims expressed in this article are solely those of the authors and do not necessarily represent those of their affiliated organizations, or those of the publisher, the editors and the reviewers. Any product that may be evaluated in this article, or claim that may be made by its manufacturer, is not guaranteed or endorsed by the publisher.
Supplementary Material
The Supplementary Material for this article can be found online at: https://www.frontiersin.org/articles/10.3389/fcvm.2021.756060/full#supplementary-material
References
1. Cutlip DLT. Revascularization in patients with stable coronary artery disease: coronary artery bypass graft surgery versus percutaneous coronary intervention. Waltham, MA: GS, editor: UpToDate (2020) (accessed November 04, 2020).
2. Califf RM, Tomabechi Y, Lee KL, Phillips H, Pryor DB, Harrell FE, et al. Outcome in one-vessel coronary artery disease. Circulation. (1983) 67:283–90. doi: 10.1161/01.CIR.67.2.283
3. Warnes CA, Roberts WC. Sudden coronary death: relation of amount and distribution of coronary narrowing at necropsy to previous symptoms of myocardial ischemia, left ventricular scarring and heart weight. Am J Cardiol. (1984) 54:65–73. doi: 10.1016/0002-9149(84)90305-9
4. Brener SJ, Witzenbichler B, Maehara A, Dizon J, Fahy M, El-Omar M, et al. Infarct size and mortality in patients with proximal versus mid left anterior descending artery occlusion: the Intracoronary Abciximab and Aspiration Thrombectomy in Patients With Large Anterior Myocardial Infarction (INFUSE-AMI) trial. Am Heart J. (2013) 166:64–70. doi: 10.1016/j.ahj.2013.03.029
5. Levine GN, Bates ER, Blankenship JC, Bailey SR, Bittl JA, Cercek B, et al. 2015 ACC/AHA/SCAI focused update on primary percutaneous coronary intervention for patients with ST-elevation myocardial infarction: an Update of the 2011 ACCF/AHA/SCAI Guideline for Percutaneous Coronary Intervention and the 2013 ACCF/AHA Guideline for the Management of ST-Elevation Myocardial Infarction: a report of the American College of Cardiology/American Heart Association Task Force on Clinical Practice Guidelines and the Society for Cardiovascular Angiography and Interventions. Circulation. (2016) 133:1135–47. doi: 10.1002/ccd.26325
6. Neumann FJ, Sousa-Uva M, Ahlsson A, Alfonso F, Banning AP, Benedetto U, et al. 2018 ESC/EACTS Guidelines on myocardial revascularization. Eur Heart J. (2019) 40:87–165. doi: 10.1093/eurheartj/ehy855
7. Yamagishi M, Tamaki N, Akasaka T, Ikeda T, Ueshima K, Uemura S, et al. JCS 2018 Guideline on diagnosis of chronic coronary heart diseases. Circ J. (2021) 85:402–572. doi: 10.1253/circj.CJ-19-1131
8. Cho SG, Kim J, Song HC. Debates over NICE Guideline update: what are the roles of nuclear cardiology in the initial evaluation of stable chest pain? Nucl Med Mol Imaging. (2019) 53:301–12. doi: 10.1007/s13139-019-00607-9
9. Knuuti J, Wijns W, Saraste A, Capodanno D, Barbato E, Funck-Brentano C, et al. 2019 ESC Guidelines for the diagnosis and management of chronic coronary syndromes. Eur Heart J. (2020) 41:407–77. doi: 10.1093/eurheartj/ehz425
10. Patton JA, Turkington TG. SPECT/CT physical principles and attenuation correction. J Nucl Med Technol. (2008) 36:1–10. doi: 10.2967/jnmt.107.046839
11. Thompson RC. CT attenuation correction for thallium SPECT MPI and other benefits of multimodality imaging. J Nucl Cardiol. (2019) 26:1596–8. doi: 10.1007/s12350-018-1255-2
12. Takahashi T, Tanaka H, Kozono N, Tanakamaru Y, Idei N, Ohashi N, et al. Characteristics of images of angiographically proven normal coronary arteries acquired by adenosine-stress thallium-201 myocardial perfusion SPECT/CT-IQ SPECT with CT attenuation correction changed stepwise. Ann Nucl Med. (2015) 29:256–67. doi: 10.1007/s12149-014-0935-5
13. Utsunomiya D, Tomiguchi S, Shiraishi S, Yamada K, Honda T, Kawanaka K, et al. Initial experience with X-ray CT based attenuation correction in myocardial perfusion SPECT imaging using a combined SPECT/CT system. Ann Nucl Med. (2005) 19:485–9. doi: 10.1007/BF02985576
14. Huang JY, Yen RF, Lee WC, Huang CK, Hsu PY, Cheng MF, et al. Improved diagnostic accuracy of thallium-201 myocardial perfusion single-photon emission computed tomography with CT attenuation correction. J Nucl Cardiol. (2019) 26:1584–95. doi: 10.1007/s12350-018-1230-y
15. Huang R, Li F, Zhao Z, Liu B, Ou X, Tian R, et al. Hybrid SPECT/CT for attenuation correction of stress myocardial perfusion imaging. Clin Nucl Med. (2011) 36:344–9. doi: 10.1097/RLU.0b013e318212c525
16. Masood Y, Liu YH, Depuey G, Taillefer R, Araujo LI, Allen S, et al. Clinical validation of SPECT attenuation correction using x-ray computed tomography-derived attenuation maps: multicenter clinical trial with angiographic correlation. J Nucl Cardiol. (2005) 12:676–86. doi: 10.1016/j.nuclcard.2005.08.006
17. Moher D, Liberati A, Tetzlaff J, Altman DG. Preferred reporting items for systematic reviews and meta-analyses: the PRISMA statement. J Clin Epidemiol. (2009) 62:1006–12. doi: 10.1016/j.jclinepi.2009.06.005
18. Page MJ, Shamseer L, Tricco AC. Registration of systematic reviews in PROSPERO: 30,000 records and counting. Syst Rev. (2018) 7:32. doi: 10.1186/s13643-018-0699-4
19. Ficaro EP, Fessler JA, Shreve PD, Kritzman JN, Rose PA, Corbett JR. Simultaneous transmission/emission myocardial perfusion tomography. Diagnostic accuracy of attenuation-corrected 99mTc-sestamibi single-photon emission computed tomography. Circulation. (1996) 93:463–73. doi: 10.1161/01.CIR.93.3.463
20. Sharma P, Patel CD, Karunanithi S, Maharjan S, Malhotra A. Comparative accuracy of CT attenuation-corrected and non-attenuation-corrected SPECT myocardial perfusion imaging. Clin Nucl Med. (2012) 37:332–8. doi: 10.1097/RLU.0b013e31823ea16b
21. Whiting P, Rutjes AW, Reitsma JB, Bossuyt PM, Kleijnen J. The development of QUADAS: a tool for the quality assessment of studies of diagnostic accuracy included in systematic reviews. BMC Med Res Methodol. (2003) 3:25. doi: 10.1186/1471-2288-3-25
22. Thompson SG. Why sources of heterogeneity in meta-analysis should be investigated. BMJ. (1994) 309:1351–5. doi: 10.1136/bmj.309.6965.1351
23. Deeks JJ, Macaskill P, Irwig L. The performance of tests of publication bias and other sample size effects in systematic reviews of diagnostic test accuracy was assessed. J Clin Epidemiol. (2005) 58:882–93. doi: 10.1016/j.jclinepi.2005.01.016
24. Reitsma JB, Glas AS, Rutjes AWS, Scholten RJPM, Bossuyt PM, Zwinderman AH. Bivariate analysis of sensitivity and specificity produces informative summary measures in diagnostic reviews. J Clin Epidemiol. (2005) 58:982–90. doi: 10.1016/j.jclinepi.2005.02.022
25. Hamza TH, van Houwelingen HC, Stijnen T. The binomial distribution of meta-analysis was preferred to model within-study variability. J Clin Epidemiol. (2008) 61:41–51. doi: 10.1016/j.jclinepi.2007.03.016
26. Raza H, Jadoon LK, Mushtaq S, Jabeen A, Maqbool M, Ain MU, et al. Comparison of non-attenuation corrected and attenuation corrected myocardial perfusion SPECT. Egypt J Radiol Nucl Med. (2016) 47:783–92. doi: 10.1016/j.ejrnm.2016.05.006
27. Links JM, Becker LC, Rigo P, Taillefer R, Hanelin L, Anstett F, et al. Combined corrections for attenuation, depth-dependent blur, and motion in cardiac SPECT: a multicenter trial. J Nucl Cardiol. (2000) 7:414–25. doi: 10.1067/mnc.2000.108350
28. Konishi T, Nakajima K, Okuda K, Yoneyama H, Matsuo S, Shibutani T, et al. IQ-SPECT for thallium-201 myocardial perfusion imaging: effect of normal databases on quantification. Ann Nucl Med. (2017) 31:454–61. doi: 10.1007/s12149-017-1170-7
29. Vidal R, Buvat I, Darcourt J, Migneco O, Desvignes P, Baudouy M, et al. Impact of attenuation correction by simultaneous emission/transmission tomography on visual assessment of 201Tl myocardial perfusion images. J Nucl Med. (1999) 40:1301–9.
30. Birkenfeld B, Buscombe JR, Coghlan G, Lipkin DP, Hilson AJ. Computer-generated attenuation correction does not improve the accuracy of myocardial perfusion scintigraphy. Nucl Med Commun. (1997) 18:358–62. doi: 10.1097/00006231-199704000-00176
31. Xu Y, Fish M, Gerlach J, Lemley M, Berman DS, Germano G, et al. Combined quantitative analysis of attenuation corrected and non-corrected myocardial perfusion SPECT: method development and clinical validation. J Nucl Cardiol. (2010) 17:591–9. doi: 10.1007/s12350-010-9220-8
32. Grossman GB, Garcia EV, Bateman TM, Heller GV, Johnson LL, Folks RD, et al. Quantitative Tc-99m sestamibi attenuation-corrected SPECT: development and multicenter trial validation of myocardial perfusion stress gender-independent normal database in an obese population. J Nucl Cardiol. (2004) 11:263–72. doi: 10.1016/j.nuclcard.2004.02.007
33. Banzo I, Pena FJ, Allende RH, Quirce R, Carril JM. Prospective clinical comparison of non-corrected and attenuation- and scatter-corrected myocardial perfusion SPECT in patients with suspicion of coronary artery disease. Nucl Med Commun. (2003) 24:995–1002. doi: 10.1097/00006231-200309000-00008
34. Hendel RC, Berman DS, Cullom SJ, Follansbee W, Heller GV, Kiat H, et al. Multicenter clinical trial to evaluate the efficacy of correction for photon attenuation and scatter in SPECT myocardial perfusion imaging. Circulation. (1999) 99:2742–9. doi: 10.1161/01.CIR.99.21.2742
35. Yamauchi Y, Kanzaki Y, Otsuka K, Hayashi M, Okada M, Nogi S, et al. Novel attenuation correction of SPECT images using scatter photopeak window data for the detection of coronary artery disease. J Nucl Cardiol. (2014) 21:109–17. doi: 10.1007/s12350-013-9814-z
36. Yamauchi Y, Kanzaki Y, Hayashi M, Arai M, Morita H, Komori T, et al. Improved diagnosis of the number of stenosed coronary artery vessels by segmentation with scatter and photo-peak window data for attenuation correction in myocardial perfusion SPECT. J Nucl Cardiol. (2019) 26:574–81. doi: 10.1007/s12350-017-1058-x
37. Xin WC, Shao XL, Wang YT, Wang JF, Wang XS, Yang L, et al. Is there an incremental value to use myocardial perfusion imaging with or without CT attenuation for the diagnosis of coronary artery disease? A study in Chinese patients. Hell J Nucl Med. (2018) 21:48–54. doi: 10.1967/s002449910706
38. Plachcinska A, Wlodarczyk M, Kovacevic-Kusmierek K, Bienkiewicz M, Drozdz J, Chizynski K, et al. Diagnostic performance of myocardial perfusion single-photon emission computed tomography with attenuation correction. Kardiol Pol. (2016) 74:32–9. doi: 10.5603/KP.a2015.0100
39. Caobelli F, Akin M, Thackeray JT, Brunkhorst T, Widder J, Berding G, et al. Diagnostic accuracy of cadmium-zinc-telluride-based myocardial perfusion SPECT: impact of attenuation correction using a co-registered external computed tomography. Eur Heart J Cardiovasc Imaging. (2016) 17:1036–43. doi: 10.1093/ehjci/jev312
40. Genovesi D, Giorgetti A, Gimelli A, Kusch A, D'Aragona Tagliavia I, Casagranda M, et al. Impact of attenuation correction and gated acquisition in SPECT myocardial perfusion imaging: results of the multicentre SPAG (SPECT Attenuation Correction vs Gated) study. Eur J Nucl Med Mol Imaging. (2011) 38:1890–8. doi: 10.1007/s00259-011-1855-4
41. Bajen MT, Ricart Y, Rodriguez-Gasen A, Mora J, Quintana ME, Benitez A, et al. Clinical utility of attenuation correction with X-rays in myocardial perfusion SPECT studies. Rev Esp Med Nucl. (2007) 26:359–66. doi: 10.1016/S1578-200X(07)70067-0
42. Xu J, Cai F, Geng C, Wang Z, Tang X. Diagnostic performance of CMR, SPECT, and PET imaging for the identification of coronary artery disease: a meta-analysis. Front Cardiovasc Med. (2021) 8:621389. doi: 10.3389/fcvm.2021.621389
43. Nudi F, Iskandrian AE, Schillaci O, Peruzzi M, Frati G, Biondi-Zoccai G. Diagnostic accuracy of myocardial perfusion imaging with CZT technology: systemic review and meta-analysis of comparison with invasive coronary angiography. JACC Cardiovasc Imaging. (2017) 10:787–94. doi: 10.1016/j.jcmg.2016.10.023
44. Nudi F, Biondi-Zoccai G, Romagnoli A, Schillaci O, Nudi A, Versaci F. Hybrid anatomo-functional imaging of coronary artery disease: beneficial irrespective of its core components. J Nucl Cardiol. (2019) 26:752–62. doi: 10.1007/s12350-018-01562-2
45. Takx RA, Blomberg BA, El Aidi H, Habets J, de Jong PA, Nagel E, et al. Diagnostic accuracy of stress myocardial perfusion imaging compared to invasive coronary angiography with fractional flow reserve meta-analysis. Circ Cardiovasc Imaging. (2015) 8:e002666. doi: 10.1161/CIRCIMAGING.114.002666
46. Danad I, Szymonifka J, Twisk JWR, Norgaard BL, Zarins CK, Knaapen P, et al. Diagnostic performance of cardiac imaging methods to diagnose ischaemia-causing coronary artery disease when directly compared with fractional flow reserve as a reference standard: a meta-analysis. Eur Heart J. (2017) 38:991–8. doi: 10.1093/eurheartj/ehw095
47. Danad I, Raijmakers PG, Driessen RS, Leipsic J, Raju R, Naoum C, et al. Comparison of coronary CT angiography, SPECT, PET, and hybrid imaging for diagnosis of ischemic heart disease determined by fractional flow reserve. JAMA Cardiol. (2017) 2:1100–7. doi: 10.1001/jamacardio.2017.2471
48. Oddstig J, Martinsson E, Jögi J, Engblom H, Hindorf C. Differences in attenuation pattern in myocardial SPECT between CZT and conventional gamma cameras. J Nucl Cardiol. (2019) 26:1984–91. doi: 10.1007/s12350-018-1296-6
49. Gimelli A, Liga R, Duce V, Kusch A, Clemente A, Marzullo P. Accuracy of myocardial perfusion imaging in detecting multivessel coronary artery disease: a cardiac CZT study. J Nucl Cardiol. (2017) 24:687–95. doi: 10.1007/s12350-015-0360-8
50. Driessen RS, Danad I, Stuijfzand WJ, Raijmakers PG, Schumacher SP, van Diemen PA, et al. Comparison of coronary computed tomography angiography, fractional flow reserve, and perfusion imaging for ischemia diagnosis. J Am Coll Cardiol. (2019) 73:161–73. doi: 10.1016/j.jacc.2018.10.056
51. Casolo G, Del Meglio J, Tessa C. Epidemiology and pathophysiologic insights of coronary atherosclerosis relevant for contemporary non-invasive imaging. Cardiovasc Diagn Therapy. (2020) 10:1906–17. doi: 10.21037/cdt-20-157
52. Juan Ramon A, Yang Y, Pretorius PH, Slomka PJ, Johnson KL, King MA, et al. Investigation of dose reduction in cardiac perfusion SPECT via optimization and choice of the image reconstruction strategy. J Nucl Cardiol. (2018) 25:2117–28. doi: 10.1007/s12350-017-0920-1
53. Grossmann M, Giannopoulos AA, Bechtiger FA, Messerli M, Schwyzer M, Benz DC, et al. Ultra-low-dose computed tomography for attenuation correction of cadmium-zinc-telluride single photon emission computed tomography myocardial perfusion imaging. J Nucl Cardiol. (2020) 27:228–37. doi: 10.1007/s12350-018-1303-y
54. O'Shaughnessy E, Dixon KL. Reducing CT dose in myocardial perfusion SPECT/CT. Nucl Med Commun. (2015) 36:1150–4. doi: 10.1097/MNM.0000000000000366
55. Koepfli P, Hany TF, Wyss CA, Namdar M, Burger C, Konstantinidis AV, et al. CT attenuation correction for myocardial perfusion quantification using a PET/CT hybrid scanner. J Nucl Med. (2004) 45:537–42.
56. Huang JY, Huang CK, Yen RF, Wu HY, Tu YK, Cheng MF, et al. Diagnostic performance of attenuation-corrected myocardial perfusion imaging for coronary artery disease: a systematic review and meta-analysis. J Nucl Med. (2016) 57:1893–8. doi: 10.2967/jnumed.115.171462
57. Xin W, Yang X, Wang J, Shao X, Zhang F, Shi Y, et al. Gated single-photon emission computed tomography myocardial perfusion imaging is superior to computed tomography attenuation correction in discriminating myocardial infarction from attenuation artifacts in men and right coronary artery disease. Nucl Med Commun. (2019) 40:491–8. doi: 10.1097/MNM.0000000000001009
Keywords: attenuation correction, myocardial perfusion imaging, single photon emission computed tomography, coronary artery, meta-analysis
Citation: Huang J-Y, Huang C-K, Yen R-F, Chien K-L and Wu Y-W (2021) Diagnostic Effect of Attenuation Correction in Myocardial Perfusion Imaging in Different Coronary Arteries: A Systematic Review and Meta-Analysis. Front. Cardiovasc. Med. 8:756060. doi: 10.3389/fcvm.2021.756060
Received: 10 August 2021; Accepted: 15 September 2021;
Published: 12 October 2021.
Edited by:
Salah D. Qanadli, University of Lausanne, SwitzerlandReviewed by:
Wolfgang Rottbauer, Ulm University Medical Center, GermanyRiccardo Liga, Pisana University Hospital, Italy
Copyright © 2021 Huang, Huang, Yen, Chien and Wu. This is an open-access article distributed under the terms of the Creative Commons Attribution License (CC BY). The use, distribution or reproduction in other forums is permitted, provided the original author(s) and the copyright owner(s) are credited and that the original publication in this journal is cited, in accordance with accepted academic practice. No use, distribution or reproduction is permitted which does not comply with these terms.
*Correspondence: Kuo-Liong Chien, klchien@ntu.edu.tw; Yen-Wen Wu, wuyw0502@gmail.com
†These authors have contributed equally to this work