- 1Nanjing University of Chinese Medicine, Nanjing, China
- 2Nanjing Hospital of Chinese Medicine Affiliated to Nanjing University of Chinese Medicine, Nanjing, China
Ventricular remodeling is related to the renin-angiotensin-aldosterone system, immune system, and various cytokines involved in inflammation, apoptosis, and cell signal regulation. Accumulated studies have shown that traditional Chinese medicine can significantly inhibit the process of ventricular remodeling, which may be related to the mechanism mentioned above. Here, we conducted a system overview to critically review the cellular and molecular mechanism of traditional Chinese medicine on ventricular remodeling. We mainly searched PubMed for basic research about the anti-ventricular remodeling of traditional Chinese medicine in 5 recent years, and then objectively summarized these researches. We included more than 25 kinds of Chinese herbal medicines including Qi-Li-Qian-Xin, Qi-Shen-Yi-Qi Pill, Xin-Ji-Er-Kang Formula, and Yi-Qi-Wen-Yang Decoction, and found that they can inhibit ventricular remodeling effectively through multi-components and multi-action targets, which are promoting the clinical application of traditional Chinese medicine.
Introduction
Ventricular remodeling, referring to a variety of injuries that change the original substances and cardiac morphology of the heart, is an adaptive response of the body and a pathophysiological process of lesion repair, overall ventricular compensation, and secondary pathophysiology (1). Ventricular remodeling occurs in response to cardiac disease or cardiac damage, and the common causes are myocardial infarction, hypertension, cardiomyopathy, and valvular disease (2, 3). The histological manifestations include the enlargement of the ventricular cavity, a progressive decrease of cardiac function, extracellular collagen deposition, inflammatory cell infiltration, apoptosis, and so on (4). It is also accompanied by neurological and humoral changes, volume overload, and other pathophysiological processes (3, 5). Its mechanism is related to the neuroendocrine system [including sympathetic nervous system (SNS) and renin-angiotensin-aldosterone system (RAAS)], immune system, and various cytokines involved in inflammation, apoptosis, and cell signal regulation (6).
Traditional Chinese medicine, mainly from the East, has shown its idiographic ascendancy in the prevention, therapeutic effect, rehabilitation, and health care of diverse diseases (7, 8). The evidence-based use of traditional Chinese medicine (TCM) keeps a foothold in China and other Asian countries, and with the popularity of TCM in the East, it is increasingly accepted and used by other countries globally (9, 10). Accumulated evidence indicates that TCM has a better effect on ventricular remodeling (VR) compared with western medicine with a single active ingredient, and the relevant mechanism is being carried out. However, we still cannot fully understand the anti-VR mechanism of TCM. Here, we shed light on recent advances in therapeutic VR of TCM and try to list the main anti-VR mechanisms of TCM. We further summarized preclinical findings and described some current problems and challenges to provide a prospect in this field.
Molecular Pathways Involved in Cardiac Remodeling
The pathogenesis of VR is associated with several molecular pathways and their relative importance depends on the underlying cause of VR. Inflammatory signals seem to be more important in VR, which are associated with the intense activation of cytokine cascades (11–13). Furthermore, oxidative stress, apoptosis, and autophagy, transforming growth factor β1 (TGF-β1), neuroendocrine system, and peroxisome proliferators-activated receptor γ (PPARγ) are involved in VR regardless of etiology (13, 14).
Inflammatory Cytokines
In recent years, a large number of studies have confirmed that the immune response triggered by a myocardial injury, myocardial ischemia, and other factors can produce a variety of cytokines (15), and most of them, especially the inflammatory cytokines, including tumor necrosis factor α (TNF-α), interleukin (IL)-6, IL-1β, and IL-10 are involved in the occurrence and development of VR (13, 16). The major inflammatory cytokines that promote VR are TNF-α, IL-1β, and IL-6. Tumor necrosis factor α and IL-1 may promote VR by its effects on cardiomyocytes, macrophages, and the extracellular matrix (ECM). In cardiomyocytes, TNF-α may trigger apoptosis by activating the inherent pathway of cell death (17, 18). In macrophages, TNF-α can stimulate the synthesis of other pro-inflammatory cytokines (17, 19). In white blood cells, IL-1 can activate white blood cells, and stimulate downstream inflammatory responses (17, 20, 21). In fibroblasts, TNF-α and IL-1 can disrupt the balance between matrix metalloproteinases (MMPs) and their inhibitors, leading to the degradation of the ECM (17). Moreover, TNF-α and IL-1 can induce the expression of endothelial adhesion molecules in the microvasculature, leading to the enhancement of adhesive interactions between circulating leukocytes and the endothelial cell lining. Because of this, the inflammatory cells may accumulate in the cardiac microcirculation, finally leading to tissue damage and cardiac dysfunction (17). Interleukin-6 plays different roles via the gp130/STAT3 pathways (17). Interleukin-6 can promote cardiac hypertrophy in cardiomyocytes (22). In fibroblasts, IL-6 promotes proliferation and stimulates ECM synthesis (23). Additionally, IL-6 can regulate the function of macrophages and lymphocytes (24). However, IL-10 can suppress the release of inflammatory mediators by monocyte macrophages, thus inhibiting the secretion of TNF-α, IL-1β, IL-1, IL-6, IL-8, granulocyte-colony stimulating factors, and granulocyte-macrophage-colony stimulating factors caused by lipopolysaccharide and interferon-γ (25–27). Furthermore, IL-10 enhances the anti-inflammatory factor release, such as IL-1 receptor antagonists and soluble TNF-α receptors (25–27).
Heart-Protecting Musk Pill, prepared using Moschus (Shexiang), Panax ginseng C.A.Mey. (Renshen), Bos taurus domesticus Gmelin (Niuhuang), Cinnamomum cassia (L.) J.Presl (Rougui), Liquidambar orientalis Mill. (Suhexiang), Bufo bufo gargarizans Cantor (Chansu), and Borneol (C10H18O, Bingpian), can inhibit inflammatory reactions and VR after acute myocardial infarction by reducing the levels of TNF-α and IL-6, leading to the increase of the maximum value of left ventricular systolic pressure and left ventricular end-systolic pressure and the reduction of the left ventricular end-diastolic pressure, consequently improving the left ventricular function in rats with acute myocardial infarction (28). Xin-Ji-Er-Kang Formula, composed of Panax ginseng C.A.Mey. (Renshen), Polygonatum adnatum S.Yun Liang (Yuzhu), Panax pseudoginseng var. Notoginseng (Burkill) G. Hoo & C.L. Tseng (Sanqi), Allium macrostemon Bunge (Xiebai), Angelica sinensis (Oliv.) Diels (Danggui), Ophiopogon japonicus (Thunb.) Ker Gawl. (Maidong), Schisandra chinensis (Turcz.) Baill. (Wuweizi), Salvia miltiorrhiza Bunge (Danshen), Sophora flavescens Aiton (Kushen), Glycyrrhiza uralensis Fisch. (Gancao), Radix Astragali (Huangqi), Epimedium acuminatum Franch. (Yinyanghuo), Trichosanthes kirilowii Maxim. (Gualou), and Dryobalanopsaromatica C.F. Gaertn. (Longnao), can reduce the expression of TNF-α and IL-1β, and increase the expression of IL-10 to restore the balance between the pro-inflammatory and anti-inflammatory state in mice with Angiotensin II (Ang II)-induced human umbilical vein endothelial cells injury (29). Yi-Qi-Wen-Yang Decoction, composed of Radix Astragali (Huangqi), Sedum erythrostictum Miq. (Jingtian), Aconitum carmichaelii Debeaux (Fuzi), Polyporus umbellatus (Pers.) Fries (Zhuling), Cervus nippon Temminck (Lurong), Curcuma phaeocaulis Valeton (Ezhu), Paeonia lactiflora Pall. (Baishao), and Zingiber officinale Roscoe (Shengjiang), was capable of inhibiting inflammatory responses through upregulating IL-10 and downregulating TNF-α (30).
The nuclear factor kappa B (NF-κB) transcriptional activation pathway is considered to be the main regulator of inflammation (31). Nuclear factor kappa B can bind to the inhibitor of kappa B (IκB) protein in the cytoplasm (32). Under the action of pro-inflammatory cytokines, IκB is phosphorylated and degraded, releasing NF-κB dimer, so that NF-κB can be transferred to the nucleus, which induces the transcription of many genes and leads to the expression of inflammatory proteins, such as TNF-α and IL-6 (33–35).
Qi-Li-Qiang-Xin, which consists of Panax ginseng C.A.Mey. (Renshen), Radix Astragali (Huangqi), Aconitum carmichaelii Debeaux (Fuzi), Salvia miltiorrhiza Bunge (Danshen), Alisma plantago-aquatica subsp. orientale (Sam.) Sam. (Zexie), Carthamus tinctorius L. (Honghua), Polygonatum adnatum S.Yun Liang (Yuzhu), Citrus reticulata Blanco (Chenpi), Ramulus Cinnamomi (Guizhi), and Semen Lepidii/Semen Descurainiae (Tinglizi), can reduce the inflammatory response in a rat model of myocardial infarction through inhibiting the activity of NF-κB, which is mainly by reducing the expression of NF-κB p65 in the nucleus and the phosphorylation of IκB (32). Moreover, Qi-Li-Qiang-Xin improved cardiac function, reduced left ventricular dimension, inhibited interstitial inflammation and fibrosis, increased neovascularization, and attenuated cardiomyocyte apoptosis through the upregulated hypoxia-inducible factor-1α (HIF-1α), vascular endothelial growth factor (VEGF), and enhanced phosphorylation of Akt (36). Qi-Shen-Yi-Qi Pill, which consists of Radix Astragali (Huangqi), Salvia miltiorrhiza Bunge (Danshen), Panax pseudoginseng var. Notoginseng (Burkill) G. Hoo & C.L. Tseng (Sanqi), and Dalbergia odorifera T. Chen (Jiangxiang), improved cardiac remodeling accompanied with a restoration of the Ang II-NADPH oxidase-reactive oxygen species (ROS)-MMPs pathways and reduction of the TNF-α/NFκB and IL-6/STAT3 pathways (37). Qing-Da Granule, composed of Gastrodia elata Blume (Tianma), Scutellaria baicalensis Georgi (Huangqin), Uncaria rhynchophylla (Miq.) Miq. ex Havil. (Gouteng), and Nelumro nucifera Gaertn. (Lianzixin), can reduce the infiltration of macrophages and the activation of proinflammatory cytokines (TNF-α, IL-6) by inhibiting the NF-κB pathway in spontaneously hypertensive rats (38).
Oxidative Stress
Oxidative stress is due to the loss of balance of redox between the level of ROS and endogenous antioxidant capacity, which causes the relative deficiency of antioxidant capacity and the relative increase of intracellular ROS (39–41). The increase of some toxic ROS leads to the loss of cell function, gene mutation, and even death (14, 42). Studies have shown that ROS is related to myocardial infarction and myocardial hypertrophy (43, 44). The stimulation of VR by oxidative stress involves the activation of several downstream signal pathways. First, ROS can activate a variety of hypertrophic signal kinases and transcription factors, including mitogen-activated protein kinase (MAPK), c-Jun N-terminal kinase (JNK), p38, NF-κB, and Akt kinases, to promote cardiac hypertrophy and cardiomyocyte apoptosis (43, 45, 46). Second, ROS can activate poly [ADP-ribose] polymerase 1 (PARP-1) by causing DNA strand breaks. Poly [ADP-ribose] polymerase 1 can regulate the expression of many inflammatory mediators and promote the progress of cardiac remodeling (43). Third, ROS can cause calcium ion (Ca2+) overload, increasing the mitochondrial permeability, and leading to the loss of mitochondrial inner membrane transmembrane potential, and then results in cell death (43, 46). Fourth, ROS can stimulate the proliferation of cardiac fibroblasts, activate MMPs, and lead to ECM remodeling (46, 47).
Xanthine oxidase is the main source of ROS in the cardiovascular system, and the inhibition of its enzyme activity can greatly reduce the production of ROS (48, 49). Qi-Li-Qiang-Xin can significantly reduce the activity of serum xanthine oxidase in rats after myocardial infarction and enhance the ability of myocardial tissue to scavenge oxygen (O2−) and hydroxyl radicals (50). Under the condition of chronic intermittent hypoxia in mice, Sheng-Mai-San can increase the activities of antioxidant enzymes (superoxide dismutase and catalase) and reduce the contents of malondialdehyde and 4-HNE (51). In addition, Sheng-Mai-San can also reduce the level of serum myeloperoxidase (52).
As the main vascular endothelial relaxing factor, nitric oxide (NO) has the effect of relaxing vascular smooth muscles, scavenging free radicals, and inhibiting lipid peroxidation. However, TNF-α can decrease NO content, because TNF-α can not only activate the nicotinamide adenine dinucleotide phosphate (NADPH) oxidase and make the superoxide produced by it react with NO, but also can inhibit the initiator of endothelial NO synthases (eNOS) and disturb the stability of the eNOS gene, leading to the decrease of the activity level of eNOS and NO (53, 54). However, Xin-Ji-Er-Kang Formula could significantly decrease the level of TNF-α, inhibit the activation of NADPH oxidase, reduce the uncoupling of eNOS and the production of ROS, and increase the activity of eNOS and NO content, thus improving the function of vascular endothelium (29, 55). The Xin-Ji-Er-Kang Formula has blunted the decrease of superoxide dismutase, NO, and the increase in malondialdehyde and Ang II serum contents, myocardial cross-section area, collagen volume fraction, and perivascular circumferential collagen area compared with the hypertensive model group. It also reduced the serum content of hydroxyproline while increasing the tetrahydrobiopterin levels in cardiac tissue by suppressing the JNK/MAPK pathway (56). Yi-Qi-Fu-Mai Powder Injection, redeveloped from Sheng-Mai-San, can decrease ROS generation, malondialdehyde content, and increase NO contents to alleviate the injury of vascular endothelial cells induced by hypoxia/reoxygenation (57). The mitochondria are the main target and source of ROS. Excessive ROS will not only lead to the non-specific damage of cells but also lead to mitochondrial dysfunction (58, 59). Yi-Qi-Fu-Mai Powder Injection can reduce the production of ROS and improve mitochondrial function by down-regulating the expression of NADPH oxidase subunits, such as NOX2, p67 phox, and NOX4 (60). However, due to the lack of experimental data, it is not clear whether TCM can remove all types of ROS.
Apoptosis Factors and Autophagy
Cardiomyocyte apoptosis is closely related to the occurrence and development of autophagy and VR (61). Caspase, Bcl-2, and Fas proteins are the major regulators of apoptosis. Many factors, such as AngII, inflammatory factors, and ROS, can promote the expression of apoptosis signals, and then promote apoptosis. Qi-Shen-Yi-Qi Pills could inhibit the expression of Fas ligand and p53 by activating the expression of murine double minute 2 and play an anti-apoptosis role in cardiomyocytes (62). Qi-Shen-Yi-Qi Pills could reduce inflammatory reaction by down-regulating the expression of inflammatory cytokines (TNF-α and IL-6) (62). Cyclooxygenase 2, which is highly linked with inflammatory cytokines, could be inhibited by Qi-Shen-Yi-Qi Pills to limit the conversion of arachidonic acid to prostaglandin E2 (PGE2), and we know that PGE2 and its receptors could induce apoptosis by increasing the transcriptional activity of the p53 gene and the expression of Fas ligand (63–65). Qi-Shen-Yi-Qi Pills could remarkably reduce the expression of PGE2 and its receptors (62). The proportion of Bax/Bcl-2 protein is the key factor to determine the inhibitory effect on apoptosis (66). The activation of the PI3K/Akt signal has been proved to prevent cardiomyocyte apoptosis and protect the myocardium (36). Through upregulating the expression of neuregulin-1, Qi-Li-Qiang-Xin can activate the PI3K/Akt signal pathway, promote Akt phosphorylation, stimulate the expression of vascular growth factor, and activate the anti-apoptosis protein, leading to the inhibition of the proportion of Bax/Bcl-2 and the expression of Caspase-3 (36). Yi-Qi-Wen-Yang Decoction suppressed the upregulated Bax, cleaved caspase-3 and PARP, and increased the downregulated Bcl-2 through activating the IL-10/Stat3 signaling and inactivating the NF-Kb P65 signaling to inhibit cardiomyocyte apoptosis (30). Qi-Li-Qiang-Xin can also inhibit cardiomyocyte apoptosis in non-infarcted zone rats by reducing the production of ROS (67). In addition, Qi-Li-Qiang-Xin can also reduce the expression of p53 (36, 67). By upregulating the expression of osteoprotegerin and tumor necrosis factor-related apoptosis-inducing ligand, Tian-Ma-Gou-Teng Decoction, made up of Uncaria rhynchophylla (Miq.) Miq. ex Havil. (Gouteng), Gastrodia elata Blume (Tianma), Scutellaria baicalensis Georgi (Huangqin), Eucommia ulmoides Oliv. (Duzhong), Achyranthes bidentata Blume (Niuxi), Cichlanthus chinensis (DC.) Tiegh. (Sangjisheng), Haliotis diversicolor Reeve (Shijueming), Gardenia jasminoides J.Ellis (Zhizi), Leonurus japonicus Houtt. (Yimucao), Polygonum multiflorum Thunb. (Heshouwu), and Poria cocos (Schw.) Wolf (Fuling), can activate p-Akt and inhibit caspase cascade to play the role of inhibiting cardiomyocyte apoptosis (68). Lu-Hong Formula, consisting of Cervus nippon Temminck (Lurong), Carthamus tinctorius L. (Honghua), Radix Astragali (Huangqi), Codonopsis affinis Hook.f. & Thomson (Dangshen), Cinnamomum cassia (L.) J.Presl (Rougui), and Semen Lepidii/Semen Descurainiae (Tinglizi) can reduce cardiomyocyte apoptosis by down-regulating the expression of caspase-3 (69). Huo-Xue-Qian-Yang Decoction, consisting of Salvia miltiorrhiza Bunge (Danshen), Hirudo nipponica Whitman (Shuizhi), Uncaria rhynchophylla (Miq.) Miq. ex Havil. (Gouteng), Haliotis diversicolor Reeve (Shijueming), Crataegus pinnatifida Bunge (Shanzha), and Zea mays L. (Yumixu), can reduce the endoplasmic reticulum stress and downregulate the activating transcription factor 6-C/EBP homologous protein signaling pathway to inhibit cardiac apoptosis to ameliorate VR (70).
Cell survival requires the participation of apoptosis and autophagy. The interaction between these two pathways is complex and critical. Autophagy can promote survival by inhibiting apoptosis under several different conditions, and sometimes lead to cell death by cooperating with apoptosis or as a supplement to apoptosis defects. Tong-Xin-Luo, Tong-Guan Capsules, and Qi-Dan-Li-Xin Pills can all play the role of anti-VR by anti-cardiomyocyte apoptosis and promoting cardiomyocyte autophagy (71–73). Rapamycin mechanical target is a key regulatory protein involved in protein production, cell growth/proliferation, autophagy, lysosomal function, and the metabolic pathway, and is considered to be the central regulator of cell growth. Tong-Xin-Luo phosphorylates TSC2 and RAPTOR by activating adenosine monophosphate (AMP)-activated protein kinase (AMPK), which leads to the decrease of rapamycin mechanical target (mTOR) phosphorylation and increasing cardiomyocyte autophagy (71). Tong-Guan Capsule promotes cardiomyocyte autophagy in the myocardial infarction mouse model by activating Sirt1 and down-regulating the mTOR/P70/S6K/4EBP1 pathway (72). It can also deacetylate p53 to inhibit apoptosis. Qi-Dan-Li-Xin Pill also promotes the autophagy of cardiomyocytes by down-regulating the mTOR/P70/S6K/4EBP1 pathway (73). Yang-Xin-Kang Tablets, prepared using Panax ginseng C.A.Mey. (Renshen), Radix Astragali (Huangqi), Ophiopogon japonicus (Thunb.) Ker Gawl. (Maidong), Schisandra chinensis (Turcz.) Baill. (Wuweizi), and Ilex pubescens Hook. & Arn. (Maodongqing), protects against myocardial injury after myocardial infarction by inhibiting the AMPK/mTOR signal pathway and excessive autophagy (74).
TGF-β1
Transforming growth factor β may be the most characteristic fibrotic growth factor (75). Transforming growth factor β has three subtypes (TGF-β1, 2, and 3), encoded by three different genes. It is currently the subject of most studies, and its overexpression leads to myocardial fibrosis (13). In addition to TGF-β, the signaling pathways associated with TGF-β are also involved in myocardial fibrosis. The TGF-β/Smads signaling pathway is the classical pathway of myocardial fibrosis. The abnormal activation of the TGF-β/Smads signal pathway can promote the proliferation of fibroblasts and the production of collagen and ECM, leading to the process of the aggravation of the myocardial fibrosis (76, 77). Some research has shown that TGF-β and its signaling pathways can be activated by Ang II, ROS, and inflammatory factors (13, 17, 78, 79).
Lu-Hong Formula can inhibit the expression of TGF-β1, and then inhibit the expression of collagen type I and III, and fibronectin genes and proteins, leading to the inhibition of the proliferation and deposition of collagen in the left ventricle in pressure-overloaded rats (69). Guanxin V could inhibit the TGF-β1 pathway to exert an anti-VR effect (80). Bu-Yang-Huan-Wu Decoction, prepared using Radix Astragali (Huangqi), Angelica sinensis (Oliv.) Diels (Danggui), Paeonia lactiflora Pall. (Chishao), Ligusticum chuanxiong (Chuanxiong), Prunus persica (L.) Batsch (Taoren), Carthamus tinctorius L. (Honghua), and Lumbricus (Dilong), can inhibit the activation of Smad3 and Smad4, which then inhibit the transcription of pro-fibrotic molecules, including the transcription of α-smooth muscle actin, collagen, and tissue inhibitors of MMPs, and finally, reduce the activation of myofibroblasts and matrix deposition, leading to the alleviation of pressure overload-induced cardiac remodeling (81). Dan-Qi soft capsules, Tong-Guan capsules, and Qi-Li-Qiang-Xin can inhibit the differentiation and formation of myofibroblasts by inhibiting the TGF-β1/Smad3 pathway, having an effect of inhibiting VR (32, 82, 83). Moreover, Qi-Li-Qiang-Xin may also promote the TGF-β3/Smad7 signal pathway to play an anti-VR effect (84). Ling-Gui-Zhu-Gan Decoction, composed of Poria cocos (Schw.) Wolf (Fuling), Ramulus Cinnamomi (Guizhi), Atractylis macrocephala (Koidz.) Hand.-Mazz. (Baizhu), and Glycyrrhiza uralensis Fisch. (Gancao), can significantly improve the pathological changes of the myocardial tissues, increase the left ventricular systolic pressure, left ventricular pressure maximum contraction rate, and left ventricular pressure maximum relaxation rate (85). Besides this, it can also decrease the left ventricular end-diastolic pressure and reduce the whole heart weight index and left ventricular weight index in rats with acute myocardial infarction (85). All of these are achieved by regulating the TGF-β/Smads pathway (85). Xin-Fu-Li Granule is a compound TCM that consists of extracts from Radix Astragali (Huangqi), Panax ginseng C.A.Mey. (Renshen), Salvia miltiorrhiza Bunge (Danshen), Ligusticum chuanxiong (Chuanxiong), Alisma plantago-aquatica subsp. orientale (Sam.) Sam. (Zexie), Angelica sinensis (Oliv.) Diels (Danggui), Semen Lepidii/Semen Descurainiae (Tinglizi), Chaenomeles speciosa (Sweet) Nakai (Mugua), Areca catechu L. (Binglang), and Ophiopogon japonicus (Thunb.) Ker Gawl. (Maidong), and can improve ventricular reconstruction and inhibit myocardial fibrosis in rats with acute myocardial infarction by regulating the TGF-β/Smads pathway (86).
What we should pay attention to is that TGF-β can also activate several other non-classical pathways, such as ras/ methyl ethyl ketone (MEK)/ extracellular signal-regulated kinases (ERK), p38, and JNK (78). The Si-Miao-Yong-An Decoction can significantly improve cardiac function and inhibit myocardial fibrosis in pressure-overloaded rats by inhibiting TGF-β1/Smad and TGF-β1/Tak1/p38 signal pathway (87). The connective tissue growth factor (CTGF) is the downstream effect factor of TGF-β1 and only mediates the negative effect of TGF-β1 (88, 89). Transforming growth factor β1 can induce the expression of CTGF, and CTGF can also enhance the TGF-β1 signal pathway (90, 91). Finally, a vicious circle is formed, resulting in the accumulation of ECM, and myocardial fibrosis occurs. Qi-Shen-Yi-Qi pills can inhibit the expression of CTGF protein and mRNA by regulating the TGF-β1/CTGF pathway and reducing the myocardial collagen deposition, leading to the improvement of VR on experimental autoimmune myocarditis rats (92).
Matrix metalloproteinases, acting as an activator in TGF-β (13), also play an important role in myocardial fibrosis. The abnormal increase and excessive deposition of a myocardial ECM plays a very important role in the occurrence and development of myocardial hypertrophy and myocardial fibrosis. Matrix metalloproteinases are a family of zinc-dependent proteases, which mainly participate in the metabolism of ECM and can reduce and release almost all ECM components except polysaccharides (93). After tissue damage, the regulation of MMPs activity is out of control, the ratio of MMPs to tissue inhibitor of metalloproteinase is out of balance, and myocardial ECM accumulates excessively, which leads to myocardial fibrosis and VR (94–96). Si-Miao-Yong-An Decoction can increase the expression of MMP9 and decrease the expression of TIMP2 to promote the degradation of collagen and reduce the synthesis of collagen, leading to the inhibition of myocardial fibrosis (87). Dan-Shen Injection can prevent left VR by inhibiting the activation of MMP2 and MMP9 to improve ejection fraction and left ventricular stroke volume in rats with myocardial infarction (97).
Nuroendocrine System
SNS
Sympathetic activity is one of the main exogenous factors in the regulation of VR. After any type of myocardial injury, adrenaline can be activated instantly, which is the main means of increasing the heart rate and contractility and playing the role of stabilizing cardiac function (98). However, long-term activation will lead to VR. The increase of catecholamine secretion can cause cardiomyocyte necrosis and apoptosis due to hypoxia, increased sarcolemmal permeability, calcium overload, the elevation of cyclic AMP (cAMP), and formation of oxidative catecholamine metabolites (98–101). Norepinephrine can stimulate fetal gene reprogramming, promote fibroblasts and protein synthesis, and aggravate VR (100, 102). Sheng-Mai-San, consisted of Panax ginseng C.A.Mey. (Renshen), Ophiopogon japonicus (Thunb.) Ker Gawl. (Maidong), and Schisandra chinensis (Turcz.) Baill. (Wuweizi), can reduce the contents of norepinephrine and 5-hydroxytryptamine in rats after myocardial infarction by regulating the activity of the SNS, and then the myocardial contraction and heart rate were slowed down (103). Additionally, Sheng-Mai-San may inhibit the activation of the hypothalamic-pituitary-adrenal axis by reducing the levels of IL-6 and TNF-α in patients with heart failure, and then control the activity of SNS and the secretion of neuropeptides. Qing-Da Granule, composed of Gastrodia elata Blume (Tianma), Scutellaria baicalensis Georgi (Huangqin), Uncaria rhynchophylla (Miq.) Miq. ex Havil. (Gouteng), and Nelumro nucifera Gaertn. (Lianzixin), can dilate blood vessels, reduce vascular tension, and inhibit vascular remodeling by suppressing the activation of the L-type Ca2+channel and inhibiting the influx of Ca2+ (104). Related research has shown that Ca2+ plays an important role in the SNS (105). The generation of sympathetic activity depends on the action potential produced by the influx of Ca2+, so the inhibition of Ca2+ influx can inhibit the sympathetic nerve activity (101, 106).
RAAS
The RAAS plays a very important role in the occurrence and development of VR (107, 108). The chronic activation of RAAS leads to a long-term increase in the levels of Ang II and aldosterone, both of which are involved in pathological processes including cardiomyocyte hypertrophy, interstitial fibrosis, and cardiomyocyte apoptosis (109, 110). Ang II, the main effector of RAAS, can not only activate the SNS and increase the heart rate and contractility but also cause systemic vasoconstriction which leads to an increase in the total peripheral resistance and aggravate cardiac load (110, 111). Ang II can stimulate different cytokines, such as endothelin-1 (ET-1), aldosterone, brain natriuretic peptide (BNP), TNF-α, and IL, and these cytokines are involved in the process of VR via different ways (112–116). Ang II can induce ET-1 by ROS and ERK (78, 117), and ET-1 can not only contract blood vessels but also induce fibroblasts to produce ECM (118). Ang II can induce aldosterone production in the adrenal cortex, and aldosterone can induce cardiomyocyte apoptosis and cardiomyocyte hypertrophy by activating oxidative stress and regulating ion channels (119, 120). Related research has shown that Ang II can increase the secretion of TNF-α and IL-6 (121), and the mechanism of their participation in VR was described in the following paragraphs. Ang II can stimulate the release of natriuretic peptides (122, 123). The long-term overstimulation of RAAS in chronic heart failure promotes increased sodium and water retention, which leads to increased pressure in the left ventricle and atrium, and finally stimulates the synthesis and secretion of BNP (123). Moreover, Ang II can upregulate the TGF-β1 expression by binding to Ang II type 1 receptors (AT1R) (78, 124), and then the TGF-β/Smads signal pathway is activated which is related to myocardial fibrosis (78).
Guanxin V consists of Codonopsis affinis Hook.f. & Thomson (Dangshen), Ophiopogon japonicus (Thunb.) Ker Gawl. (Maidong), Schisandra chinensis (Turcz.) Baill. (Wuweizi), Rehmannia chingii H.L. Li (Dihuang), Salvia miltiorrhiza Bunge (Danshen), and Paeonia lactiflora Pall. (Chishao) (80, 125) and has a significant effect on VR (126). Mechanistically, in an animal model, Guanxin V can inactivate RAAS in VR after acute myocardial infarction through the non-angiotensin converting enzyme pathway, which significantly reduces the level of Ang II, reduces the infarct size after acute myocardial infarction, protect cardiac function, reduce myocardial fibrosis, and thus, reduce VR (127). Poge Heart-Saving Decoction, prepared with Aconitum carmichaelii Debeaux (Fuzi), Zingiber officinale Roscoe (Ganjiang), Glycyrrhiza uralensis Fisch. (Gancao), Cornus officinalis Siebold & Zucc. (Shanzhuyu), Os Draconis (Longgu), Concha Ostreae (Muli), Magnetitum (Cishi), Panax ginseng C.A.Mey. (Renshen), and Moschus (Shexiang), can reduce the left ventricular end-diastolic dimension and left ventricular end-systolic dimension by inhibiting the level of RAAS, especially aldosterone and Ang II levels, and finally, decrease the post-load of the heart and increase the ejection fraction, reverse VR, and improve cardiac function (128). Jiajian Yu-Nv-Jian, containing Gypsum (CaSO4·2H2O, Shigao), Anemarrhena asphodeloides Bunge (Zhimu), Scrophularia microdonta Franch. (Xuanshen), Rehmannia chingii H.L. Li (Dihuang), and Ophiopogon japonicus (Thunb.) Ker Gawl. (Maidong), can significantly reduce the experimental cardiac remodeling by improving hemodynamics and inhibiting the activation of RAAS. It can also reduce the production of ET-1 and the contents of Ang II, aldosterone, and hydroxyproline, and down-regulate the expression of AT1R, TNF-α, and TGF-β1 (113). Morphologically, Jiajian Yu-Nv-Jian decreased the cross-sectional area of cardiomyocytes, collagen volume fraction, collagen types I and III, and the perivascular collagen area (113). RAAS mediates the development of VR mainly by inducing the generation of Ang II, and TCM has confirmed that it can inhibit the level of Ang II not only by classical RAAS but also by the non-angiotensin converting enzyme pathway. However, we do not figure out the mechanism of Ang II produced by the non-angiotensin converting enzyme pathway.
PPARγ
PPARγ is a nuclear receptor that can change the transcription of many target genes (129, 130). It is mainly related to energy metabolism. Peroxisome proliferator-activated receptor γ can stimulate the transcription of genes related to lipid metabolism and promotes adipocyte differentiation. Moreover, PPARγ can also suppress the production of proinflammatory cytokines and then inhibit proliferation and migration (130). The PPARγ coactivator 1α (PGC-1α) is the main regulator of lipid catabolism, oxidative metabolism, mitochondrial metabolism, and biogenesis-related genes, reflecting the dysfunction of the mitochondria, which plays an important role in the control of myocardial metabolism (131–133). High levels of PGC-1α may be related to higher levels of oxidative metabolism, higher oxygen consumption, and lower general oxidative stress, while lower levels of PGC-1α may be related to increased dependence on glycolysis, lower oxygen consumption, and higher ROS levels (131, 132). By upregulating the expression of PPARα and PPARγ, Shen-Qi-Fu-Zheng Injection extracted from Codonopsis affinis Hook.f. & Thomson (Dangshen) and Radix Astragali (Huangqi), can interfere with the metabolic process of the injury, inhibit ischemic cardiac structural and functional disorders such as myocardial hypertrophy and VR, effectively improve the damaged cardiac function, and achieve the protective effect of ischemia/reperfusion injury (134). Qi-Li-Qiang-Xin can improve cardiac energy metabolism by up-regulating PGC-1α and PPARγ, which alleviate myocardial hypertrophy and cardiac remodeling, and significantly improve cardiac function, including ejection fraction and fraction shortening (135–138). However, due to the lack of sufficient experimental data, we do not yet understand the mechanism of TCM suppressing PPARγ and PGC-1α.
Discussion
Ventricular remodeling is a process of a series of morphological and structural changes in cardiomyocytes, collagen grids, and vascular beds, which is the basic mechanism of heart failure, so it is of great significance to study the pathogenesis of VR for the prevention and treatment of heart failure.
The inflammatory response is one of the key factors in VR. To promote the repair of injured areas, the damaged myocardium will release its intracellular contents and cause inflammation by activating the innate immune mechanism. Neutrophils were first recruited, followed by pro-inflammatory monocytes/macrophages and lymphocytes (11, 12). These cells remove dead cells and matrix fragments from the damaged areas through different pathways and then activate the repair pathways needed for scar formation. However, the aggravation, prolongation, or expansion of the inflammatory response can lead to more severe remodeling and dysfunction. Excessive early inflammation may increase matrix degradation, leading to heart rupture (11). Prolonged inflammation may damage collagen deposition, resulting in the formation of scars with reduced tensile strength, and finally, the increase of the chamber dilatation (11). The enhanced expression of pro-inflammatory mediators can stimulate the production of ROS, activating the pro-apoptosis pathway, and inducing further loss of cardiomyocytes (43). Finally, defective control of inflammatory response may lead to inflammatory infiltration extending into the non-infarcted myocardium, enhancing fibrosis and worsening the diastolic function (11, 17). In addition, due to the overload of antioxidant defense in the damaged heart, ROS production, and induced inflammatory signals, such as IL-1 β, directly inhibit myocardial function (46).
To maintain the basic function of the damaged heart, SNS and RAAS can be activated. However, the excessive activation of SNS will lead to the excessive accumulation of catecholamines, which is linked to cardiomyocyte apoptosis and myocardial fibrosis. The activation of RAAS will cause too much Ang II generation and it can participate in a variety of reactions. Ang II can promote the release of inflammatory cytokines and ROS through AT1R (139). In addition, Ang II is involved in myocardial fibrosis. Ang II can up-regulate the expression of TGF-β, which plays an important role in VR (139). Transforming growth factor -β can not only increase the production of ECM but also upregulate the expression of mesenchymal makers by activating the TGF-β/Smad3 signal pathway, leading to the formation of myofibroblasts (78, 139). What is more, Ang II can also promote the production of aldosterone, which can upregulate the expression of α-smooth muscle actin and promote myocardial fibrosis (139).
In addition to the mechanism described above, the energy metabolism of cardiomyocytes also affects the process of VR. Abnormal energy metabolism will lead to ROS production and lead to a series of chain reactions, such as inflammation, activation of the neuroendocrine system, and cardiomyocyte apoptosis, thus, improving the myocardial energy metabolism is helpful to improve VR.
Because of its multi-components and multi-targets, TCM has good effects on the prevention and treatment of VR. From the literature we enumerated, Qi-Li-Qiang-Xin is the most studied drug. Based on a variety of studies on Qi-Li-Qiang-Xin, we can find that it can exert the effect of anti-myocardial apoptosis and myocardial fibrosis by inhibiting the production of inflammatory factors, reducing the production of ROS, up-regulating PGC-1α and PPARγ, inhibiting the TGF-β1/Smad3 pathway, and activating the TGF-β3/Smad7 signal pathway. Other TCM, like Qi-Shen-Yi-Qi, can also reduce VR by reducing inflammation and inhibiting the TGF-β1/CTGF pathway. Traditional Chinese medicine improves VR mainly by acting on cardiomyocytes, fibroblasts, and inflammatory cells, but due to the limitations of related experiments, it is not clear what kind of inflammatory cells TCM can reduce. From Table 1, we can find that many TCM has only done related research on one of the mechanism mentioned above, which is not beneficial to our further understanding of the anti-VR mechanism of TCM. Perhaps we can speculate the multiple mechanisms of anti-VR from the same components of different TCM, but we do not know whether the previous interactions of different TCM have changed the structure of the active components of TCM, which requires us to further improve the basic research of TCM against VR.
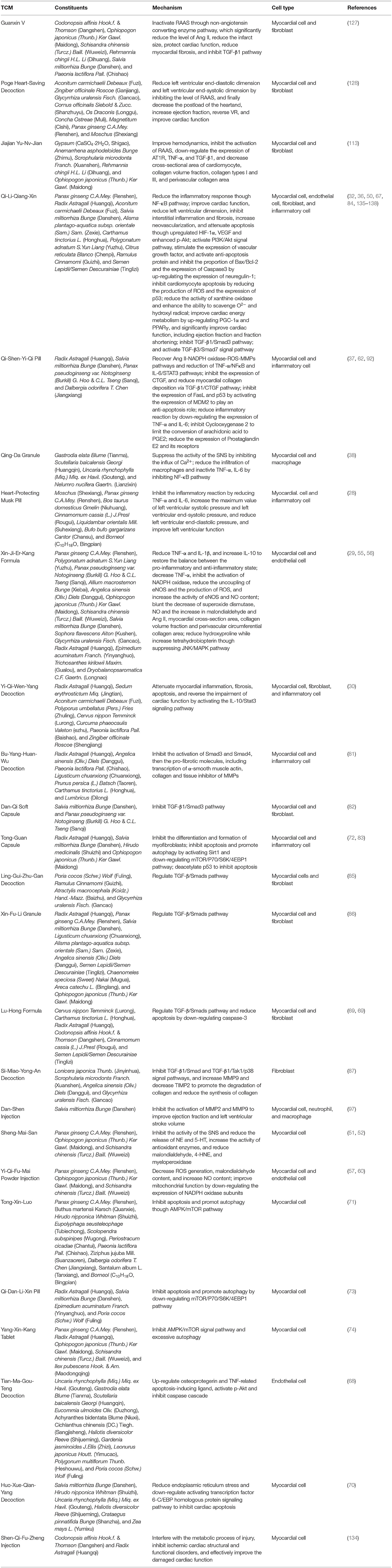
Table 1. Details of constituents and mechanism of traditional Chinese medicine (TCM) for ventricular remodeling (VR).
Conclusion
The occurrence of VR is the result of the joint action of a variety of mechanisms (Table 2). In recent years, with the popularity of TCM, the advantages of TCM in the treatment of VR are more and more obvious (140). We do not comment on any monomer here, because the TCM formula consists of dozens of ingredients with numerous chemical molecules, making it difficult to elucidate the therapeutic mechanism of TCM (141–144). Through our research, we verify that TCM inhibits the process of VR through the single or multiple pathways and combined use of multiple drugs again. Some TCM, such as Qi-Li-Qiang-Xin, Qi-Shen-Yi-Qi Pill, Xin-Ji-Er-Kang Formula, and Yi-Qi-Wen-Yang Decoction, could play a role in many pathological conditions, including apoptosis, oxidative stress, and inflammation. From the above discussion, because of its multi-components and multi-action targets, TCM can inhibit VR effectively. However, the conclusion we got is based on a summary of numerous basic experiments, and this just provides evidence for subsequent clinical applications. We still need extensive clinical trials to assess the effectiveness and safety of TCM. Besides this, due to the holistic view and syndrome differentiation of TCM, we need to use different TCM formulas for patients with different syndromes, and we cannot establish treatment standards, which greatly increases the difficulty of clinical trials. In addition, the diversity of components also shows that it is difficult to identify the precise targets, which requires us to continue to improve the relevant technology.
Author Contributions
Y-CZ, BL, and NG designed the study, acquired and researched the data for the article, and discussed its content. Y-CZ wrote the manuscript. NG and BL revised the manuscript. All authors read and approved the final manuscript.
Funding
This work was partly supported by the National Natural Science Foundation of China (81774229), Research and Practice Innovation Plan for Postgraduates of Jiangsu, China (KYCX21_1641), Jiangsu Universities Nursing Advantage Discipline Project (2019YSHL095), and Jiangsu Leading Talent Project of Traditional Chinese Medicine (Jiangsu TCM 2018 No. 4).
Conflict of Interest
The authors declare that the research was conducted in the absence of any commercial or financial relationships that could be construed as a potential conflict of interest.
Publisher's Note
All claims expressed in this article are solely those of the authors and do not necessarily represent those of their affiliated organizations, or those of the publisher, the editors and the reviewers. Any product that may be evaluated in this article, or claim that may be made by its manufacturer, is not guaranteed or endorsed by the publisher.
Acknowledgments
We thank all those who are committed to TCM and VR.
References
1. Biere L, Donal E, Jacquier A, Croisille P, Genee O, Christiaens L, et al. A new look at left ventricular remodeling definition by cardiac imaging. Int J Cardiol. (2016) 209:17–9. doi: 10.1016/j.ijcard.2016.02.009
2. Cheng X, Wang L, Wen X, Gao L, Li G, Chang G, et al. TNAP is a novel regulator of cardiac fibrosis after myocardial infarction by mediating TGF-beta/Smads and ERK1/2 signaling pathways. EBioMedicine. (2021) 67:103370. doi: 10.1016/j.ebiom.2021.103370
3. Constant Dit Beaufils A-L, Huttin O, Jobbe-Duval A, Senage T, Filippetti L, Piriou N, et al. Replacement myocardial fibrosis in patients with mitral valve prolapse: relation to mitral regurgitation, ventricular remodeling, and arrhythmia. Circulation. (2021) 143:1763–74. doi: 10.1161/CIRCULATIONAHA.120.050214
4. Azevedo PS, Polegato BF, Minicucci MF, Paiva SA, Zornoff LA. Cardiac remodeling: concepts, clinical impact, pathophysiological mechanisms and pharmacologic treatment. Arq Bras Cardiol. (2016) 106:62–9. doi: 10.5935/abc.20160005
5. Perera-Gonzalez M, Kiss A, Kaiser P, Holzweber M, Nagel F, Watzinger S, et al. The role of tenascin C in cardiac reverse remodeling following banding-debanding of the ascending aorta. Int J Mol Sci. (2021) 22:2023. doi: 10.3390/ijms22042023
6. Fan D-C, Qi J-Y, Zhang M-Z. Insights of Chinese medicine on ventricular remodeling: multiple-targets, individualized-treatment. Chin J Integr Med. (2017) 23:643–7. doi: 10.1007/s11655-017-2415-y
7. Chao J, Dai Y, Verpoorte R, Lam W, Cheng Y-C, Pao L-H, et al. Major achievements of evidence-based traditional Chinese medicine in treating major diseases. Biochem Pharmacol. (2017) 139:94–104. doi: 10.1016/j.bcp.2017.06.123
8. Hao P-P, Jiang F, Cheng J, Ma L-Y, Zhang Y, Zhao Y-X. Traditional Chinese medicine for cardiovascular disease: evidence and potential mechanisms. J Am Coll Cardiol. (2017) 69:2952–66. doi: 10.1016/j.jacc.2017.04.041
9. Liang B, Zou F-H, Fu L, Liao H-L. Chinese herbal medicine Dingji Fumai decoction for ventricular premature contraction: a real-world trial. Biomed Res Int. (2020) 2020:5358467. doi: 10.1155/2020/5358467
10. Liang B, Zhou Y, Fu L, Liao H-L. Antiarrhythmic mechanisms of Chinese herbal medicine Dingji Fumai Decoction. Evid Based Complement Alternat Med. (2020) 2020:9185707. doi: 10.1155/2020/9185707
11. Frangogiannis NG. Regulation of the inflammatory response in cardiac repair. Circ Res. (2012) 110:159–73. doi: 10.1161/CIRCRESAHA.111.243162
12. Kologrivova I, Shtatolkina M, Suslova T, Ryabov V. Cells of the immune system in cardiac remodeling: main players in resolution of inflammation and repair after myocardial infarction. Front Immunol. (2021) 12:664457. doi: 10.3389/fimmu.2021.664457
13. Kong P, Christia P, Frangogiannis NG. The pathogenesis of cardiac fibrosis. Cell Mol Life Sci. (2014) 71:549–74. doi: 10.1007/s00018-013-1349-6
14. Liang B, Zhu Y-C, Lu J, Gu N. Effects of traditional chinese medication-based bioactive compounds on cellular and molecular mechanisms of oxidative stress. Oxid Med Cell Longev. (2021) 2021:3617498. doi: 10.1155/2021/3617498
15. He X, Liang B, Gu N. Th17/Treg imbalance and atherosclerosis. Dis Markers. (2020) 2020:8821029. doi: 10.1155/2020/8821029
16. Gouyou B, Grun K, Kerschenmeyer A, Villa A, Matasci M, Schrepper A, et al. Therapeutic evaluation of antibody-based targeted delivery of interleukin 9 in experimental pulmonary hypertension. Int J Mol Sci. (2021) 22:3460. doi: 10.3390/ijms22073460
17. Hanna A, Frangogiannis NG. Inflammatory cytokines and chemokines as therapeutic targets in heart failure. Cardiovasc Drugs Ther. (2020) 34:849–63. doi: 10.1007/s10557-020-07071-0
18. Haudek SB, Taffet GE, Schneider MD, Mann DL, TNF. provokes cardiomyocyte apoptosis and cardiac remodeling through activation of multiple cell death pathways. J Clin Invest. (2007) 117:2692–701. doi: 10.1172/JCI29134
19. Hamid T, Gu Y, Ortines RV, Bhattacharya C, Wang G, Xuan YT, et al. Divergent tumor necrosis factor receptor-related remodeling responses in heart failure: role of nuclear factor-kappaB and inflammatory activation. Circulation. (2009) 119:1386–97. doi: 10.1161/CIRCULATIONAHA.108.802918
20. Sager HB, Heidt T, Hulsmans M, Dutta P, Courties G, Sebas M, et al. Targeting Interleukin-1β Reduces Leukocyte Production After Acute Myocardial Infarction. Circulation. (2015) 132:1880–90. doi: 10.1161/CIRCULATIONAHA.115.016160
21. Saxena A, Chen W, Su Y, Rai V, Uche OU Li N, et al. IL-1 induces proinflammatory leukocyte infiltration and regulates fibroblast phenotype in the infarcted myocardium. J Immunol. (2013) 191:4838–48. doi: 10.4049/jimmunol.1300725
22. Meléndez GC, McLarty JL, Levick SP, Du Y, Janicki JS, Brower GL. Interleukin 6 mediates myocardial fibrosis, concentric hypertrophy, and diastolic dysfunction in rats. Hypertension. (2010) 56:225–31. doi: 10.1161/HYPERTENSIONAHA.109.148635
23. Chou CH, Hung CS, Liao CW, Wei LH, Chen CW, Shun CT, et al. IL-6 trans-signalling contributes to aldosterone-induced cardiac fibrosis. Cardiovasc Res. (2018) 114:690–702. doi: 10.1093/cvr/cvy013
24. Sackett SD, Otto T, Mohs A, Sander LE, Strauch S, Streetz KL, et al. Myeloid cells require gp130 signaling for protective anti-inflammatory functions during sepsis. FASEB J. (2019) 33:6035–44. doi: 10.1096/fj.201802118R
25. Huber S, Gagliani N, Esplugues E, O'Connor WJ, Huber FJ, Chaudhry A, et al. Th17 cells express interleukin-10 receptor and are controlled by Foxp3− and Foxp3+ regulatory CD4+ T cells in an interleukin-10-dependent manner. Immunity. (2011) 34:554–65. doi: 10.1016/j.immuni.2011.01.020
26. Kany S, Vollrath JT, Relja B. Cytokines in inflammatory disease. Int J Mol Sci. (2019) 20:6008. doi: 10.3390/ijms20236008
27. Xu Y, Hong S, Zhao X, Wang S, Xu Z, Ding S, et al. Acupuncture alleviates rheumatoid arthritis by immune-network modulation. Am J Chin Med. (2018) 46:997–1019. doi: 10.1142/S0192415X18500520
28. Cen W, Chen Z, Gu N, Hoppe R. Prevention of AMI induced ventricular remodeling: inhibitory effects of heart-protecting musk pill on IL-6 and TNF-Alpha. Evid Based Complement Alternat Med. (2017) 2017:3217395. doi: 10.1155/2017/3217395
29. Ding L, Cheng P, Wang L, Hu J, Zhang Y-X, Cai G-W, et al. The protective effects of polysaccharide extract from Xin-Ji-Er-Kang formula on Ang II-induced HUVECs injury, L-NAME-induced hypertension and cardiovascular remodeling in mice. BMC Complement Altern Med. (2019) 19:127. doi: 10.1186/s12906-019-2539-z
30. Li H, Gong Z-J, He Y, Huang J-J, Jiang Y-N, Liu Y-Y, et al. YQWY decoction improves myocardial remodeling via activating the IL-10/Stat3 signaling pathway. Evid Based Complement Alternat Med. (2020) 2020:7532892. doi: 10.1155/2020/7532892
31. Wang L, Zhang N, Han D, Su P, Chen B, Zhao W, et al. MTDH promotes intestinal inflammation by positively regulating TLR signaling. J Crohns Colitis. (2021) 2021:jjab086. doi: 10.1093/ecco-jcc/jjab086
32. Han A, Lu Y, Zheng Q, Zhang J, Zhao Y, Zhao M, et al. Qiliqiangxin attenuates cardiac remodeling via inhibition of TGF-beta1/Smad3 and NF-kappaB signaling pathways in a rat model of myocardial infarction. Cell Physiol Biochem. (2018) 45:1797–806. doi: 10.1159/000487871
33. Nam N-H. Naturally occurring NF-kappaB inhibitors. Mini Rev Med Chem. (2006) 6:945–51. doi: 10.2174/138955706777934937
34. Xie Z, Singh M, Singh K. Differential regulation of matrix metalloproteinase-2 and−9 expression and activity in adult rat cardiac fibroblasts in response to interleukin-1beta. J Biol Chem. (2004) 279:39513–9. doi: 10.1074/jbc.M405844200
35. Timmers L, van Keulen JK, Hoefer IE, Meijs MF, van Middelaar B, den Ouden K, et al. Targeted deletion of nuclear factor kappaB p50 enhances cardiac remodeling and dysfunction following myocardial infarction. Circ Res. (2009) 104:699–706. doi: 10.1161/CIRCRESAHA.108.189746
36. Wang J, Zhou J, Ding X, Zhu L, Jiang K, Fu M, et al. Qiliqiangxin improves cardiac function and attenuates cardiac remodeling in rats with experimental myocardial infarction. Int J Clin Exp Pathol. (2015) 8:6596–606.
37. Li C, Wang Y, Qiu Q, Shi T, Wu Y, Han J, et al. Qishenyiqi protects ligation-induced left ventricular remodeling by attenuating inflammation and fibrosis via STAT3 and NF-kappaB signaling pathway. PLoS ONE. (2014) 9:e104255. doi: 10.1371/journal.pone.0104255
38. Wu X, Shen A, Bao L, Wu M, Lin X, Wang H, et al. Qingda granules attenuate hypertensive cardiac remodeling and inflammation in spontaneously hypertensive rats. Biomed Pharmacother. (2020) 129:110367. doi: 10.1016/j.biopha.2020.110367
39. Filomeni G, De Zio D, Cecconi F. Oxidative stress and autophagy: the clash between damage and metabolic needs. Cell Death Differ. (2015) 22:377–88. doi: 10.1038/cdd.2014.150
40. Sies H. Oxidative stress: a concept in redox biology and medicine. Redox Biol. (2015) 4:180–3. doi: 10.1016/j.redox.2015.01.002
41. Sinha N, Dabla PK. Oxidative stress and antioxidants in hypertension-a current review. Curr Hypertens Rev. (2015) 11:132–42. doi: 10.2174/1573402111666150529130922
42. Senoner T, Dichtl W. Oxidative stress in cardiovascular diseases: still a therapeutic target? Nutrients (2019) 11:2090. doi: 10.3390/nu11092090
43. Tsutsui H, Kinugawa S, Matsushima S. Oxidative stress and heart failure. Am J Physiol Heart Circ Physiol. (2011) 301:H2181–90. doi: 10.1152/ajpheart.00554.2011
44. Pena E, Brito J, El Alam S, Siques P. Oxidative stress, kinase activity and inflammatory implications in right ventricular hypertrophy and heart failure under hypobaric hypoxia. Int J Mol Sci. (2020) 21:6421. doi: 10.3390/ijms21176421
45. Hirotani S, Otsu K, Nishida K, Higuchi Y, Morita T, Nakayama H, et al. Involvement of nuclear factor-kappaB and apoptosis signal-regulating kinase 1 in G-protein-coupled receptor agonist-induced cardiomyocyte hypertrophy. Circulation. (2002) 105:509–15. doi: 10.1161/hc0402.102863
46. Hori M, Nishida K. Oxidative stress and left ventricular remodelling after myocardial infarction. Cardiovasc Res. (2009) 81:457–64. doi: 10.1093/cvr/cvn335
47. Jia G, Hill MA, Sowers JR. Diabetic cardiomyopathy: an update of mechanisms contributing to this clinical entity. Circ Res. (2018) 122:624–38. doi: 10.1161/CIRCRESAHA.117.311586
48. Mellin V, Isabelle M, Oudot A, Vergely-Vandriesse C, Monteil C, Di Meglio B, et al. Transient reduction in myocardial free oxygen radical levels is involved in the improved cardiac function and structure after long-term allopurinol treatment initiated in established chronic heart failure. Eur Heart J. (2005) 26:1544–50. doi: 10.1093/eurheartj/ehi305
49. Doehner W, Landmesser U. Xanthine oxidase and uric acid in cardiovascular disease: clinical impact and therapeutic options. Semin Nephrol. (2011) 31:433–40. doi: 10.1016/j.semnephrol.2011.08.007
50. Xiao J, Deng S-B, She Q, Li J, Kao G-Y, Wang J-S, et al. Traditional Chinese medicine Qili qiangxin inhibits cardiomyocyte apoptosis in rats following myocardial infarction. Exp Ther Med. (2015) 10:1817–23. doi: 10.3892/etm.2015.2759
51. Chai C-Z, Mo W-L, Zhuang X-F, Kou J-P, Yan Y-Q, Yu B-Y. Protective effects of Sheng-Mai-San on right ventricular dysfunction during chronic intermittent hypoxia in mice. Evid Based Complement Alternat Med. (2016) 2016:4682786. doi: 10.1155/2016/4682786
52. Li F, Fan X-X, Chu C, Zhang Y, Kou J-P, Yu B-Y, et al. Strategy for optimizing the combination of active components based on chinese medicinal formula Sheng-Mai-San for myocardial ischemia. Cell Physiol Biochem. (2018) 45:1455–71. doi: 10.1159/000487572
53. Neumann P, Gertzberg N, Johnson A. TNF-alpha induces a decrease in eNOS promoter activity. Am J Physiol Lung Cell Mol Physiol. (2004) 286:L452–9. doi: 10.1152/ajplung.00378.2002
54. Arriero MM, Rodríguez-Feo JA, Celdrán Á, Miguel LSDE, González-Fernández F, Fortes J, et al. Expression of endothelial nitric oxide synthase in human peritoneal tissue: regulation by Escherichia coli lipopolysaccharide. J Am Soc Nephrol. (2000) 11:1848–56. doi: 10.1681/ASN.V11101848
55. Wang X-Y, Huang G-Y, Lian F-Z, Pan M, Ruan C-S, Ling X-X, et al. Protective effect of Xin-Ji-Er-Kang on cardiovascular remodeling in high-salt induced hypertensive mice: role ofoxidative stress and endothelial dysfunction. Biomed Pharmacother. (2019) 115:108937. doi: 10.1016/j.biopha.2019.108937
56. Guo K, Lan C-z, Yu T-t, Huang L-l, Wang X-h, Pan C, et al. Effects of Xin-Ji-Er-Kang formula on 2K1C-induced hypertension and cardiovascular remodeling in rats. J Ethnopharmacol. (2014) 155:1227–35. doi: 10.1016/j.jep.2014.07.006
57. Li F, Tan Y-S, Chen H-L, Yan Y, Zhai K-F, Li D-P, et al. Identification of schisandrin as a vascular endothelium protective component in YiQiFuMai Powder Injection using HUVECs binding and HPLC-DAD-Q-TOF-MS/MS analysis. J Pharmacol Sci. (2015) 129:1–8. doi: 10.1016/j.jphs.2015.02.003
58. Saberi M, Zhang X, Mobasheri A. Targeting mitochondrial dysfunction with small molecules in intervertebral disc aging and degeneration. GeroScience. (2021) 43:517–37. doi: 10.1007/s11357-021-00341-1
59. Chen W, Guo C, Feng H, Chen Y. Mitochondria: novel mechanisms and therapeutic targets for secondary brain injury after intracerebral hemorrhage. Front Aging Neurosci. (2021) 12:615451. doi: 10.3389/fnagi.2020.615451
60. Zhang Y, Zhang L, Zhang Y, Fan X, Yang W, Yu B, et al. YiQiFuMai powder injection attenuates coronary artery ligation-induced heart failure through improving mitochondrial function via regulating ROS generation and CaMKII signaling pathways. Front Pharmacol. (2019) 10:381. doi: 10.3389/fphar.2019.00381
61. Xin T, Lu C. Irisin activates Opa1-induced mitophagy to protect cardiomyocytes against apoptosis following myocardial infarction. Aging. (2020) 12:4474–88. doi: 10.18632/aging.102899
62. Wang J, Li C, Cao Y, Wang Q, Lu L, Chang H, et al. Mechanism of QSYQ on anti-apoptosis mediated by different subtypes of cyclooxygenase in AMI induced heart failure rats. BMC Complement Altern Med. (2015) 15:352. doi: 10.1186/s12906-015-0869-z
63. Qiu H, Liu J-Y, Wei D, Li N, Yamoah EN, Hammock BD, et al. Cardiac-generated prostanoids mediate cardiac myocyte apoptosis after myocardial ischaemia. Cardiovasc Res. (2012) 95:336–45. doi: 10.1093/cvr/cvs191
64. Liu P, Xu B, Cavalieri TA, Hock CE. Pifithrin-alpha attenuates p53-mediated apoptosis and improves cardiac function in response to myocardial ischemia/reperfusion in aged rats. Shock. (2006) 26:608–14. doi: 10.1097/01.shk.0000232273.11225.af
65. van Empel VPM, Bertrand ATA, Hofstra L, Crijns HJ, Doevendans PA, De Windt LJ. Myocyte apoptosis in heart failure. Cardiovasc Res. (2005) 67:21–9. doi: 10.1016/j.cardiores.2005.04.012
66. Xu Y, Zhang Y, Xu Y, Zang G, Li B, Xia H, et al. Activation of CD137 signaling promotes macrophage apoptosis dependent on p38 MAPK pathway-mediated mitochondrial fission. Int J Biochem Cell Biol. (2021) 2021:106003. doi: 10.1016/j.biocel.2021.106003
67. Liang T, Zhang Y, Yin S, Gan T, An T, Zhang R, et al. Cardio-protecteffect of qiliqiangxin capsule on left ventricular remodeling, dysfunction and apoptosis in heart failure rats after chronic myocardial infarction. Am J Transl Res. (2016) 8:2047–58.
68. Deng L-H, Li L, Zhai Y, Michael S, Yang C-Y, Guo R, et al. Tianma gouteng decoction exerts cardiovascular protection by upregulating OPG and TRAIL in spontaneously hypertensive rats. Evid Based Complement Alternat Med. (2020) 2020:3439191. doi: 10.1155/2020/3439191
69. Liu Q, Qu H-Y, Zhou H, Rong J-F, Yang T-S, Xu J-J, et al. Luhong formula has a cardioprotective effect on left ventricular remodeling in pressure-overloaded rats. Evid Based Complement Alternat Med. (2020) 2020:4095967. doi: 10.1155/2020/4095967
70. Zhou X, Lu B, Fu D, Gui M, Yao L, Li J. Huoxue Qianyang decoction ameliorates cardiac remodeling in obese spontaneously hypertensive rats in association with ATF6-CHOP endoplasmic reticulum stress signaling pathway regulation. Biomed Pharmacother. (2020) 121:109518. doi: 10.1016/j.biopha.2019.109518
71. Li Q, Li N, Cui H-H, Tian X-Q, Jin C, Chen G-H, et al. Tongxinluo exerts protective effects via anti-apoptotic and pro-autophagic mechanisms by activating AMPK pathway in infarcted rat hearts. Exp Physiol. (2017) 102:422–35. doi: 10.1113/EP086192
72. Mao S, Chen P, Li T, Guo L, Zhang M. Tongguan capsule mitigates post-myocardial infarction remodeling by promoting autophagy and inhibiting apoptosis: role of Sirt1. Front Physiol. (2018) 9:589. doi: 10.3389/fphys.2018.00589
73. Shi B, Huang Y, Ni J, Chen J, Wei J, Gao H, et al. Qi Dan Li Xin pill improves chronic heart failure by regulating mTOR/p70S6k-mediated autophagy and inhibiting apoptosis. Sci Rep. (2020) 10:6105. doi: 10.1038/s41598-020-63090-9
74. Ren P-H, Zhang Z-M, Wang P, Zhu H-P, Li Z-Q. Yangxinkang tablet protects against cardiac dysfunction and remodelling after myocardial infarction in rats through inhibition of AMPK/mTOR-mediated autophagy. Pharm Biol. (2020) 58:321–7. doi: 10.1080/13880209.2020.1748662
75. Hu HH, Chen DQ, Wang YN, Feng YL, Cao G, Vaziri ND, et al. New insights into TGF-β/Smad signaling in tissue fibrosis. Chem Biol Interact. (2018) 292:76–83. doi: 10.1016/j.cbi.2018.07.008
76. Santibañez JF, Quintanilla M, Bernabeu C. TGF-β/TGF-β receptor system and its role in physiological and pathological conditions. Clin Sci. (2011) 121:233–51. doi: 10.1042/CS20110086
77. Lei B, Hitomi H, Mori T, Nagai Y, Deguchi K, Mori H, et al. Effect of efonidipine on TGF-β1-induced cardiac fibrosis through Smad2-dependent pathway in rat cardiac fibroblasts. J Pharmacol Sci. (2011) 117:98–105. doi: 10.1254/jphs.11065FP
78. Leask A. Potential therapeutic targets for cardiac fibrosis: TGFbeta, angiotensin, endothelin, CCN2, and PDGF, partners in fibroblast activation. Circ Res. (2010) 106:1675–80. doi: 10.1161/CIRCRESAHA.110.217737
79. AlQudah M, Hale TM, Czubryt MP. Targeting the renin-angiotensin-aldosterone system in fibrosis. Matrix Biol. (2020) 91-92:92–108. doi: 10.1016/j.matbio.2020.04.005
80. Liang B, Liang Y, Li R, Zhang H, Gu N. Integrating systematic pharmacology-based strategy and experimental validation to explore the synergistic pharmacological mechanisms of Guanxin V in treating ventricular remodeling. Bioorg Chem. (2021) 115:105187. doi: 10.1016/j.bioorg.2021.105187
81. Chen H, Song H, Liu X, Tian J, Tang W, Cao T, et al. Buyanghuanwu Decoction alleviated pressure overload induced cardiac remodeling by suppressing Tgf-β/Smads and MAPKs signaling activated fibrosis. Biomed Pharmacother. (2017) 95:461–8. doi: 10.1016/j.biopha.2017.08.102
82. Ma S, Ma J, Mai X, Zhao X, Guo L, Zhang M. Danqi soft capsule prevents infarct border zone remodelling and reduces susceptibility to ventricular arrhythmias in post-myocardial infarction rats. J Cell Mol Med. (2019) 23:5454–65. doi: 10.1111/jcmm.14428
83. Ma S, Ma J, Zhou Y, Guo L, Bai J, Zhang M. Tongguan capsule derived-herb ameliorates remodeling at infarcted border zone and reduces ventricular arrhythmias in rats after myocardial infarction. Biomed Pharmacother. (2019) 120:109514. doi: 10.1016/j.biopha.2019.109514
84. Sun X, Chen G, Xie Y, Jiang D, Han J, Chen F, et al. Qiliqiangxin improves cardiac function and attenuates cardiac remodelling in doxorubicin-induced heart failure rats. Pharm Biol. (2020) 58:417–26. doi: 10.1080/13880209.2020.1761403
85. Wang L, Shi H, Huang J-L, Xu S, Liu P-P. Linggui Zhugan decoction inhibits ventricular remodeling after acute myocardial infarction in mice by suppressing TGF-β/Smad signaling pathway. Chin J Integr Med. (2020) 26:345–52. doi: 10.1007/s11655-018-3024-0
86. Ma J, Li Z-Y, Liang X-P, Guo C-X, Lu P-P, Ma L-H. Xinfuli Granule improves post-myocardial infarction ventricular remodeling and myocardial fibrosis in rats by regulating TGF-β/Smads signaling pathway. J Geriatr Cardiol. (2017) 14:301–7. doi: 10.11909/j.issn.1671-5411.2017.05.005
87. Su C, Wang Q, Luo H, Jiao W, Tang J, Li L, et al. Si-Miao-Yong-An decoction attenuates cardiac fibrosis via suppressing TGF-β1 pathway and interfering with MMP-TIMPs expression. Biomed Pharmacother. (2020) 127:110132. doi: 10.1016/j.biopha.2020.110132
88. Kunzmann S, Schmidt-Weber C, Zingg J-M, Azzi A, Kramer BW, Blaser K, et al. Connective tissue growth factor expression is regulated by histamine in lung fibroblasts: potential role of histamine in airway remodeling. J Allergy Clin Immunol. (2007) 119:1398–407. doi: 10.1016/j.jaci.2007.02.018
89. Kunzmann S, Speer CP, Jobe AH, Kramer BW. Antenatal inflammation induced TGF-beta1 but suppressed CTGF in preterm lungs. Am J Physiol Lung Cell Mol Physiol. (2007) 292:L223–31 doi: 10.1152/ajplung.00159.2006
90. Chen MM, Lam A, Abraham JA, Schreiner GF, Joly AH, CTGF. expression is induced by TGF- beta in cardiac fibroblasts and cardiac myocytes: a potential role in heart fibrosis. J Mol Cell Cardiol. (2000) 32:1805–19. doi: 10.1006/jmcc.2000.1215
91. Vainio LE, Szabo Z, Lin R, Ulvila J, Yrjola R, Alakoski T, et al. Connective tissue growth factor inhibition enhances cardiac repair and limits fibrosis after myocardial infarction. JACC Basic Transl Sci. (2019) 4:83–94. doi: 10.1016/j.jacbts.2018.10.007
92. Lv S, Wu M, Li M, Wang Q, Xu L, Wang X, et al. Effect and mechanism of QiShenYiQi Pill on experimental autoimmune myocarditis rats. Med Sci Monit. (2016) 22:752–6. doi: 10.12659/MSM.895655
93. Tousoulis D, Kampoli AM, Papageorgiou N, Antoniades C, Siasos G, Latsios G, et al. Matrix metallopropteinases in heart failure. Curr Top Med Chem. (2012) 12:1181–91. doi: 10.2174/1568026611208011181
94. Cokkinos DV, Pantos C. Myocardial remodeling, an overview. Heart Fail Rev. (2011) 16:1–4. doi: 10.1007/s10741-010-9192-4
95. Fix C, Carver-Molina A, Chakrabarti M, Azhar M, Carver W. Effects of the isothiocyanate sulforaphane on TGF-β1-induced rat cardiac fibroblast activation and ECM interactions. J Cell Physiol. (2019) 234:13931–41. doi: 10.1002/jcp.28075
96. Vanhoutte D, Heymans S. TIMPs and cardiac remodeling: “Embracing the MMP-independent-side of the family.” J Mol Cell Cardiol. (2010) 48:445–53. doi: 10.1016/j.yjmcc.2009.09.013
97. Wang L, Yu J, Fordjour PA, Xing X, Gao H, Li Y, et al. Danshen injection prevents heart failure by attenuating post-infarct remodeling. J Ethnopharmacol. (2017) 205:22–32. doi: 10.1016/j.jep.2017.04.027
98. Bristow MR, Quaife RA. The adrenergic system in pulmonary arterial hypertension: bench to bedside (2013 Grover Conference series). Pulm Circ. (2015) 5:415–23. doi: 10.1086/682223
99. Lu M, Wang H, Wang J, Zhang J, Yang J, Liang L, et al. Astragaloside IV protects against cardiac hypertrophy via inhibiting the Ca2+/CaN signaling pathway. Planta Med. (2014) 80:63–9. doi: 10.1055/s-0033-1360129
100. Böhm M, Maack C. Treatment of heart failure with beta-blockers. Mechanisms and results Basic Res Cardiol. (2000) 95 Suppl 1:I15–24. doi: 10.1007/s003950070004
101. Triposkiadis F, Karayannis G, Giamouzis G, Skoularigis J, Louridas G, Butler J. The sympathetic nervous system in heart failure physiology, pathophysiology, and clinical implications. J Am Coll Cardiol. (2009) 54:1747–62. doi: 10.1016/j.jacc.2009.05.015
102. Kaludercic N, Takimoto E, Nagayama T, Feng N, Lai EW, Bedja D, et al. Monoamine oxidase A-mediated enhanced catabolism of norepinephrine contributes to adverse remodeling and pump failure in hearts with pressure overload. Circ Res. (2010) 106:193–202. doi: 10.1161/CIRCRESAHA.109.198366
103. Xu N, Qiu C, Wang W, Wang Y, Chai C, Yan Y, et al. HPLC/MS/MS for quantification of two types of neurotransmitters in rat brain and application: myocardial ischemia and protection of Sheng-Mai-San. J Pharm Biomed Anal. (2011) 55:101–8. doi: 10.1016/j.jpba.2010.12.015
104. Huang Y, Wu X, Wu M, Chu J, Yu N, Shen A, et al. Antihypertensive and Vasodilatory Effects of Qingda Granules by Suppression of Calcium Influx and the AKT Pathway. J Cardiovasc Pharmacol. (2019) 74:549–57. doi: 10.1097/FJC.0000000000000686
105. Huang R, Wang Y, Li J, Jiang X, Li Y, Liu B, et al. Ca(2+)-independent but voltage-dependent quantal catecholamine secretion (CiVDS) in the mammalian sympathetic nervous system. Proc Natl Acad Sci USA. (2019) 116:20201–9. doi: 10.1073/pnas.1902444116
106. Südhof TC. The molecular machinery of neurotransmitter release (Nobel lecture). Angew Chem Int Ed Engl. (2014) 53:12696–717. doi: 10.1002/anie.201406359
107. Yu B, Chen H, Guo X-Q, Hua H, Guan Y, Cui F, et al. CIHH protects the heart against left ventricular remodelling and myocardial fibrosis by balancing the renin-angiotensin system in SHR. Life Sci. (2021) 278:119540. doi: 10.1016/j.lfs.2021.119540
108. Docherty KF, Campbell RT, Brooksbank KJM, Dreisbach JG, Forsyth P, Godeseth RL, et al. The effect of neprilysin inhibition on left ventricular remodeling in patients with asymptomatic left ventricular systolic dysfunction late after myocardial infarction. Circulation. (2021). doi: 10.1161/CIRCULATIONAHA.121.054892
109. Hartupee J, Mann DL. Neurohormonal activation in heart failure with reduced ejection fraction. Nat Rev Cardiol. (2017) 14:30–8. doi: 10.1038/nrcardio.2016.163
110. Navar LG. Physiology: hemodynamics, endothelial function, renin-angiotensin-aldosterone system, sympathetic nervous system. J Am Soc Hypertens. (2014) 8:519–24. doi: 10.1016/j.jash.2014.05.014
111. Murphy SP, Ibrahim NE, Januzzi JL. Jr. Heart Failure With Reduced Ejection Fraction: A Review Jama. (2020) 324:488–504. doi: 10.1001/jama.2020.10262
112. Zhao X-S, Pan W, Bekeredjian R, Shohet RV. Endogenous endothelin-1 is required for cardiomyocyte survival in vivo. Circulation. (2006) 114:830–7. doi: 10.1161/CIRCULATIONAHA.105.577288
113. Du J, Gu WL, Chen CX, Wang Y, Lv J. Protective and therapeutic effects of chinese medicine formula jiajian yunvjian on experimental cardiac remodeling after myocardial infarction induced by coronary artery ligation. Evid Based Complement Alternat Med. (2015) 2015:949656. doi: 10.1155/2015/949656
114. Chen Q, Zhang D, Bi Y, Zhang W, Zhang Y, Meng Q, et al. The protective effects of liguzinediol on congestive heart failure induced by myocardial infarction and its relative mechanism. Chin Med. (2020) 15:63. doi: 10.1186/s13020-020-00345-7
115. Liu Y, Lv H, Tan R, An X, Niu X-H, Liu Y-J, et al. Platelets promote Ang II (Angiotensin II)-induced atrial fibrillation by releasing TGF-β1 (transforming growth factor-β1) and interacting with fibroblasts. Hypertension. (2020) 76:1856–67. doi: 10.1161/HYPERTENSIONAHA.120.15016
116. Chiang M-T, Chen IM, Hsu F-F, Chen Y-H, Tsai M-S, Hsu Y-W, et al. Gal-1 (Galectin-1) upregulation contributes to abdominal aortic aneurysm progression by enhancing vascular inflammation. Arterioscler Thromb Vasc Biol. (2021) 41:331–45. doi: 10.1161/ATVBAHA.120.315398
117. Shephard P, Hinz B, Smola-Hess S, Meister JJ, Krieg T, Smola H. Dissecting the roles of endothelin, TGF-beta and GM-CSF on myofibroblast differentiation by keratinocytes. Thromb Haemost. (2004) 92:262–74. doi: 10.1160/TH03-11-0669
118. Leask A. Targeting the TGFbeta, endothelin-1 and CCN2 axis to combat fibrosis in scleroderma. Cell Signal. (2008) 20:1409–14. doi: 10.1016/j.cellsig.2008.01.006
119. Callera GE, Montezano AC, Yogi A, Tostes RC, He Y, Schiffrin EL, et al. c-Src-dependent nongenomic signaling responses to aldosterone are increased in vascular myocytes from spontaneously hypertensive rats. Hypertension. (2005) 46:1032–8. doi: 10.1161/01.HYP.0000176588.51027.35
120. Petramala L, Concistrè A, Olmati F, Saracino V, Chimenti C, Frustaci A, et al. Cardiomyopathies and adrenal diseases. Int J Mol Sci. (2020) 21:5047. doi: 10.3390/ijms21145047
121. Liang ES, Bai WW, Wang H, Zhang JN, Zhang F, Ma Y, et al. PARP-1 (Poly[ADP-Ribose] Polymerase 1) inhibition protects from Ang II (Angiotensin II)-induced abdominal aortic aneurysm in mice. Hypertension. (2018) 72:1189–99. doi: 10.1161/HYPERTENSIONAHA.118.11184
122. Bae EH, Ma SK, Lee J, Kim SW. Altered regulation of renal nitric oxide and atrial natriuretic peptide systems in angiotensin II-induced hypertension. Regul Pept. (2011) 170:31–7. doi: 10.1016/j.regpep.2011.05.005
123. Díez J. Chronic heart failure as a state of reduced effectiveness of the natriuretic peptide system: implications for therapy. Eur J Heart Fail. (2017) 19:167–76. doi: 10.1002/ejhf.656
124. Watanabe T, Barker TA, Berk BC. Angiotensin II and the endothelium: diverse signals and effects. Hypertension. (2005) 45:163–9. doi: 10.1161/01.HYP.0000153321.13792.b9
125. Liang B, Zhang X-X, Gu N. Virtual screening and network pharmacology-based synergistic mechanism identification of multiple components contained in Guanxin V against coronary artery disease. BMC Complement Med Ther. (2020) 20:345. doi: 10.1186/s12906-020-03133-w
126. Liang B, Qu Y, Zhao Q-F, Gu N. Guanxin V for coronary artery disease: A retrospective study. Biomed Pharmacother. (2020) 128:110280. doi: 10.1016/j.biopha.2020.110280
127. Zhang X-X, Shao C-L, Cheng S-Y, Zhu Y, Liang B, Gu N. Effect of Guanxin V in animal model of acute myocardial infarction. BMC Complement Med Ther. (2021) 21:72. doi: 10.1186/s12906-021-03211-7
128. Liu L, Mo Y, Wu B, Yu Z, Sun B. Effect of traditional chinese medicine poge heart-saving decoction on cardiac function in heart failure rat model. Evid Based Complement Alternat Med. (2020) 2020:8762509. doi: 10.1155/2020/8762509
129. Shimano M, Tsuji Y, Inden Y, Kitamura K, Uchikawa T, Harata S, et al. Pioglitazone, a peroxisome proliferator-activated receptor-gamma activator, attenuates atrial fibrosis and atrial fibrillation promotion in rabbits with congestive heart failure. Heart Rhythm. (2008) 5:451–9. doi: 10.1016/j.hrthm.2007.12.010
130. Frantz S, Hu K, Widder J, Bayer B, Witzel CC, Schmidt I, et al. Peroxisome proliferator activated-receptor agonism and left ventricular remodeling in mice with chronic myocardial infarction. Br J Pharmacol. (2004) 141:9–14. doi: 10.1038/sj.bjp.0705585
131. Fabregat-Andrés Ó, Ridocci-Soriano F, Estornell-Erill J, Corbí-Pascual M, Valle-Muñoz A, Berenguer-Jofresa A, et al. Blood PGC-1α concentration predicts myocardial salvage and ventricular remodeling after ST-segment elevation acute myocardial infarction. Rev Esp Cardiol. (2015) 68:408–16. doi: 10.1016/j.rec.2014.05.020
132. Fabregat-Andres O, Paredes F, Monsalve M, Milara J, Ridocci-Soriano F, Gonzalez-Hervas S, et al. mRNA PGC-1α levels in blood samples reliably correlates with its myocardial expression: study in patients undergoing cardiac surgery. Anatolian journal of cardiology. (2016) 16:622–9. doi: 10.5152/AnatolJCardiol.2015.6466
133. Sun C-K, Chang L-T, Sheu J-J, Chiang C-H, Lee F-Y, Wu C-J, et al. Bone marrow-derived mononuclear cell therapy alleviates left ventricular remodeling and improves heart function in rat-dilated cardiomyopathy. Crit Care Med. (2009) 37:1197–205. doi: 10.1097/CCM.0b013e31819c0667
134. Liao J, Hao C, Huang W, Shao X, Song Y, Liu L, et al. Network pharmacology study reveals energy metabolism and apoptosis pathways-mediated cardioprotective effects of Shenqi Fuzheng. J Ethnopharmacol. (2018) 227:155–65. doi: 10.1016/j.jep.2018.08.029
135. Gao RR, Wu XD, Jiang HM, Zhu YJ, Zhou YL, Zhang HF, et al. Traditional Chinese medicine Qiliqiangxin attenuates phenylephrine-induced cardiac hypertrophy via upregulating PPARγ and PGC-1α. Ann Transl Med. (2018) 6:153. doi: 10.21037/atm.2018.04.14
136. Wang H, Zhang X, Yu P, Zhou Q, Zhang J, Zhang H, et al. Traditional chinese medication qiliqiangxin protects against cardiac remodeling and dysfunction in spontaneously hypertensive rats. Int J Med Sci. (2017) 14:506–14. doi: 10.7150/ijms.18142
137. Tao L, Shen S, Fu S, Fang H, Wang X, Das S, et al. Traditional chinese medication qiliqiangxin attenuates cardiac remodeling after acute myocardial infarction in mice. Sci Rep. (2015) 5:8374. doi: 10.1038/srep08374
138. Shen S, Jiang H, Bei Y, Zhang J, Zhang H, Zhu H, et al. Qiliqiangxin attenuates adverse cardiac remodeling after myocardial infarction in ovariectomized mice via activation of PPARγ. Cell Physiol Biochem. (2017) 42:876–88. doi: 10.1159/000478641
139. Balakumar P, Sambathkumar R, Mahadevan N, Muhsinah AB, Alsayari A, Venkateswaramurthy N, et al. A potential role of the renin-angiotensin-aldosterone system in epithelial-to-mesenchymal transition-induced renal abnormalities: Mechanisms and therapeutic implications. Pharmacol Res. (2019) 146:104314. doi: 10.1016/j.phrs.2019.104314
140. Chen R, Zhu C, Xu L, Gu Y, Ren S, Bai H, et al. An injectable peptide hydrogel with excellent self-healing ability to continuously release salvianolic acid B for myocardial infarction. Biomaterials. (2021) 274:120855. doi: 10.1016/j.biomaterials.2021.120855
141. Gu WL, Chen CX, Huang XY, Gao JP. The effect of angoroside C on pressure overload-induced ventricular remodeling in rats. Phytomedicine. (2015) 22:705–12. doi: 10.1016/j.phymed.2015.05.002
142. Gao Y, Gao JP, Chen CX, Wang HL, Guo J, Wu R. Beneficial effects of houttuynin on ventricular remodeling induced by coronary artery ligation in rats. Eur J Pharmacol. (2014) 740:200–8. doi: 10.1016/j.ejphar.2014.07.015
143. Gao Y, Gao J, Chen C, Wang H, Guo J, Wu R. Cardioprotective effect of polydatin on ventricular remodeling after myocardial infarction in coronary artery ligation rats. Planta Med. (2015) 81:568–77. doi: 10.1055/s-0035-1545907
Keywords: ventricular remodeling, traditional Chinese medicine, mechanism, cardiovascular disease, review
Citation: Zhu Y-C, Liang B and Gu N (2021) Cellular and Molecular Mechanism of Traditional Chinese Medicine on Ventricular Remodeling. Front. Cardiovasc. Med. 8:753095. doi: 10.3389/fcvm.2021.753095
Received: 04 August 2021; Accepted: 25 October 2021;
Published: 01 December 2021.
Edited by:
Xiaofeng Yang, Temple University, United StatesReviewed by:
Kawa Dizaye, Hawler Medical University, IraqRuijing Zhang, Second Hospital of Shanxi Medical University, China
Copyright © 2021 Zhu, Liang and Gu. This is an open-access article distributed under the terms of the Creative Commons Attribution License (CC BY). The use, distribution or reproduction in other forums is permitted, provided the original author(s) and the copyright owner(s) are credited and that the original publication in this journal is cited, in accordance with accepted academic practice. No use, distribution or reproduction is permitted which does not comply with these terms.
*Correspondence: Ning Gu, guning@njucm.edu.cn
†These authors have contributed equally to this work and share first authorship