- 1Department of Pharmacy Practice and Science, College of Pharmacy, University of Arizona, Tucson, AZ, United States
- 2Graduate Interdisciplinary Program in Statistics and Data Science, University of Arizona, Tucson, AZ, United States
- 3Department of Biosystems Engineering, College of Agriculture and Life Sciences, University of Arizona, Tucson, AZ, United States
- 4Department of Epidemiology and Biostatistics, Mel & Enid Zuckerman College of Public Health, University of Arizona, Tucson, AZ, United States
- 5Department of Medicine and the Sarver Heart Center, University of Arizona College of Medicine, Tucson, AZ, United States
Coronary artery reperfusion is essential for the management of symptoms in the patients with myocardial ischemia. However, the benefit of reperfusion often comes at an expense of paradoxical injury, which contributes to the adverse events, and sometimes heart failure. Reperfusion is known to increase the production of reactive oxygen species (ROS). We address whether N-acetylcysteine (NAC) reduces the ROS and alleviates reperfusion injury by improving the clinical outcomes. A literature search for the randomized controlled trials (RCTs) was carried out in the five biomedical databases for testing the effects of NAC in patients undergoing coronary artery reperfusion by percutaneous coronary intervention, thrombolysis, or coronary artery bypass graft. Of 787 publications reviewed, 28 RCTs were identified, with a summary of 2,174 patients. A meta-analysis using the random effects model indicated that NAC administration during or prior to the reperfusion procedures resulted in a trend toward a reduction in the level of serum cardiac troponin (cTn) [95% CI, standardized mean difference (SMD) −0.80 (−1.75; 0.15), p = 0.088, n = 262 for control, 277 for NAC group], and in the incidence of postoperative atrial fibrillation [95% CI, relative risk (RR) 0.57 (0.30; 1.06), p = 0.071, n = 484 for control, 490 for NAC group]. The left ventricular ejection fraction or the measures of length of stay in intensive care unit (ICU) or in hospital displayed a positive trend that was not statistically significant. Among the nine trials that measured ROS, seven showed a correlation between the reduction of lipid peroxidation and improved clinical outcomes. These lines of evidence support the potential benefit of NAC as an adjuvant therapy for cardiac protection against reperfusion injury.
Introduction
A reperfusion injury has long been an unavoidable complication of the coronary artery revascularization procedures for the patients with acute or chronic myocardial ischemia. Although essential for the survival or for the relief of symptoms, reperfusion can contribute to as much as 40% of the final infarct size (1). The most common reperfusion procedure for the patients with myocardial ischemic is percutaneous coronary intervention (PCI). When reperfusion cannot be achieved successfully by PCI alone or in the setting of multivessel coronary disease, open heart surgery of coronary artery bypass graft (CABG) may be performed. Thrombolytic therapy can be prescribed during PCI, or alone when PCI and CABG are not readily available or impossible to perform due to the condition of a patient. One complication for each of these reperfusion treatments is periprocedural myocardial injury (PMI), which is linked to arrhythmias or reinfarction and in some cases heart failure. The release of massive amounts of reactive oxygen species (ROS) during reperfusion is thought to be an important cause of PMI.
Periprocedural myocardial injury is measurable with a number of clinical parameters, such as elevation of circulating cardiac troponins (cTn) or creatine kinase muscle band (CK-MB). Whereas, the amplitude or duration of cTn elevation can be predictive for the adverse events and heart failure (2–4), the extent of PMI is associated with the incidence of post-operative atrial fibrillation (POAF) (5). As a common complication following an open-heart surgery, the incidence of POAF can reach up to 70% in the patients after an elective CABG (6). POAF can cause stroke and increase the length of stay (LOS) in the intensive care unit (ICU) or in hospital. There is evidence supporting the concept that ROS and cytokine storm play a key role in the pathogenesis of POAF (7).
Despite a well-established association, ROS remains a neglected therapeutic target for the patients undergoing coronary reperfusion procedures. Administration of N-acetylcysteine (NAC) before reperfusion is expected to reduce the ROS generation. While a few randomized controlled trials (RCTs) showed a significant inhibition of cTn or CK-MB release or the incidence of POAF, other RCTs did not report positive outcomes. Given these inconsistences, it is prudent to address whether NAC provides a benefit for the coronary reperfusion procedures through a systematic review and meta-analysis approach.
A few meta-analyses have assessed the cardioprotective effect of NAC during cardiac surgery (8–12). However, each of these reports has a limited number of references. More importantly, none of these reports have included consideration of PCI. About 90% of the patients with ST segment elevation myocardial infarct (STEMI) and 50% of the patients with non-STEMI are treated with PCI (13), supporting the importance of PCI when considering the benefit of NAC during reperfusion. Nevertheless, none of these published meta-analyses have determined the impact of NAC on all the common clinical measures, such as elevation of cTn or CK-MB, change in left ventricular ejection fraction (LVEF), and ICU or hospital length of stay (LOS). In addition, whether the clinical outcomes correlate with the reduction of ROS has not been determined. Here, we address the cardioprotective effect of NAC when administered before PCI, CABG, or thrombolysis by summarizing the data from the publications with relevant clinical measures. In addition, the levels of antioxidants and ROS are captured to support the cause-effect relationship.
Methods
The Preferred Reporting Items for Systematic Reviews (PRISMA) guideline was adopted for this systematic literature review using an a-priori inclusion and exclusion criteria (14).
Inclusion and Exclusion Criteria
A-priori inclusion criteria were: (1) the RCTs assessing the effect of NAC in the patients >18 years old who underwent coronary reperfusion by PCI, CABG, or thrombolysis; (2) NAC was administered within 24 h before or during coronary reperfusion; (3) the RCTs should have measured the effect of NAC in comparison to a control group; (4) the control group should have received either placebo or standard care; (5) the published manuscripts and abstracts for the RCTs; (6) the RCTs published in any language; (7) the RCTs should not have selectively included the participants with any degree of renal insufficiency; and (8) the RCTs published from inception to September 18, 2021.
We excluded those RCTs in which the effect of NAC was not compared with a control group, but instead was compared with another pharmacologic agent. In addition, we excluded those RCTs reporting the trials designed for the selective patients with renal insufficiency, since renal insufficiency itself causes increased levels of cTn and CK-MB (15), potentially underestimating the beneficial effect of NAC on cardiac injury.
We considered both the clinical cardiac endpoints and mechanistic measures in this systematic review. The clinical endpoints included biomarkers of myocardial injury (cTn and CK-MB), cardiac contractility (left ventricular ejection fraction, LVEF), infarct size, incidence of POAF, and postoperative ICU or hospital LOS. The mechanistic measures consisted of markers for total antioxidant capacity (TAC) and ROS. To reduce the complexity of the data, we only extracted the serum and urine levels of the non-clinical markers and excluded the measures from the biopsy samples.
Literature Search and Data Extraction
A comprehensive search strategy was developed with the assistance of a health science librarian (Rachel Walden) using a combination of keywords and controlled vocabulary to identify the studies reporting the use of NAC in the patients undergoing coronary artery reperfusion with PCI, CABG, or thrombolysis. The search strategy was developed for PubMed/Medline (NLM) and was subsequently translated to carry out the searches in four other biomedical bibliographic databases: Embase (Elsevier), Web of Science (Clarivate Analytics), Cumulative Index to Nursing and Allied Health Literature (CINAHL), and Cochrane Library (Wiley). In addition to searching the five bibliographic databases, a search of the gray literature (Clinicaltrials.gov) was performed. We searched for the trials from inception through September 18, 2021.
The following keywords were used to create the search strategy: myocardial reperfusion, T-Plasminogen activator, TPA, activase, alteplase, percutaneous transluminal coronary angioplasty, coronary balloon angioplasty, transluminal coronary balloon dilation, percutaneous coronary revascularization, percutaneous coronary intervention, PCI, coronary artery bypass grafting, CABG, aortocoronary bypass, coronary artery bypass surgery, coronary artery bypass, and acetylcysteine (as shown in Supplementary Material for full search strategy).
The primary (SAK) and the secondary reviewer (AMC) independently searched and screened the reports. Rayyan QCRI Systematic Reviews Web Application was used after careful removal of duplicate records (16). No major discrepancies were noted among the two independent reviewers in the shortlisted trials. The primary reviewer extracted the data and assessed the risk of bias for each RCT, while the secondary reviewer validated the data for each publication. Minor discrepancies were noted in the extracted data, which were resolved with discussion reaching a mutual agreement. The PRISMA flowchart summary is shown in Figure 1.
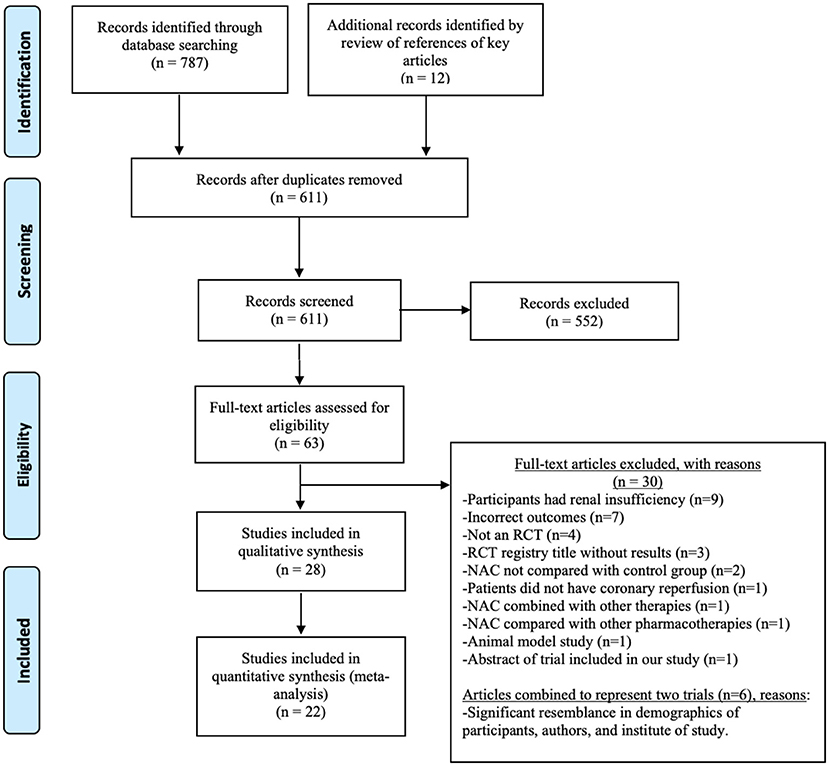
Figure 1. Preferred Reporting Items for Systematic Reviews and Meta-Analyses (PRISMA) flow diagram. The numbers document the literature search results.
Quality Assessment of Included Trials
The revised Cochrane risk of bias tool for randomized trials (RoB2) was applied by the primary and secondary reviewers to assess the risk of bias for each included trial [https://methods.cochrane.org/bias/resources/rob-2-revised-cochrane-risk-bias-tool-randomized-trials (2020, accessed 10 May 2020)]. The following domains were evaluated: random sequence generation, allocation concealment, blinding of patients and personnel, blinding of outcome assessment, and incomplete outcome data. Similar to the data extraction process, minor discrepancies in the risk of bias assessment were resolved through discussion for consensus generation.
Statistical Analysis
The measurements in cTn, CK-MB, LVEF, and LOS were treated as the continuous variables with reported means and SDs, while the incidence of POAF was treated as a dichotomous variable. Instead of applying the fixed effects models, which operate under the assumption that the estimated effects across the studies were pulled from a single population, we employed the random effects models to calculate the pooled effects, as the true effect may derive from a distribution, due to the fact that multiple studies were pulled from the different populations (17). The Sidik–Jonkman estimator was used for estimating the variance of the distribution of the true effect (18). The false positive rate increases when a small number of studies are enrolled and the outcome measures vary largely between the trials (19, 20).
In this NAC meta-analysis, the number of studies for each of six types of outcome measures varied from 5 to 12. Therefore, the Hartung-Knapp-Sidik-Jonkman method was also utilized to generate more robust estimates of the variance of pooled effects (19). When the outcome measures were continuous variables, the standardized mean difference (SMD) was calculated as a measure of effect size, as this is appropriate when different units were used across the studies (21). The SMD standardizes outcome measures in various units so that they are comparable at the same scale. Within a study, the SMD divides the mean difference of values of a measure by the pooled SD, thus SMD becomes a unitless standardized value. Hence, the SMDs can be compared across the studies for the related measures without the consideration of their respective units. The meta-analysis produces a pooled SMD, which denotes a change in the combined SD for a specific measure.
For dichotomous variables, relative risk (RR), a measure of effect size, was used as the likelihood of an event occurring between the two groups (NAC vs. control). The between-study heterogeneity was reported by I2. The individual effect size for each study and its weight, as well as confidence interval (CI) for the individual studies and pooled estimates, were included in the results. All the statistical analyses were performed using R version 3.6 (https://www.r-project.org/) (2013, accessed 10 May 2020). Specifically, the meta-analysis was performed by the packages meta (22), metafor (23), and dmetar (24).
Results
Characteristics of the Trials
Figure 1 shows the PRISMA flowchart and the number of publications evaluated, leading to the selection of 28 trials in 32 publications for this systematic review (25–56). The characteristics of the included trials are summarized in Table 1. Geographically, the reported trials were carried out in 10 countries: Turkey (10), Iran (5), India (3), Germany (2), Uzbekistan (1), Czech Republic (1), Finland (1), Canada (1), Australia (1), Brazil (1), Korea (1), and China (1). The total sample size, by adding the number of patients in the final statistical analyses for each of the 28 included trials, was 2,174. Among the 26 trials with the gender and age distribution indicated as shown in Table 1, the mean age of the patients ranged from 53 to 71.5 years old. The two trials did not disclose the age distribution (30, 56).
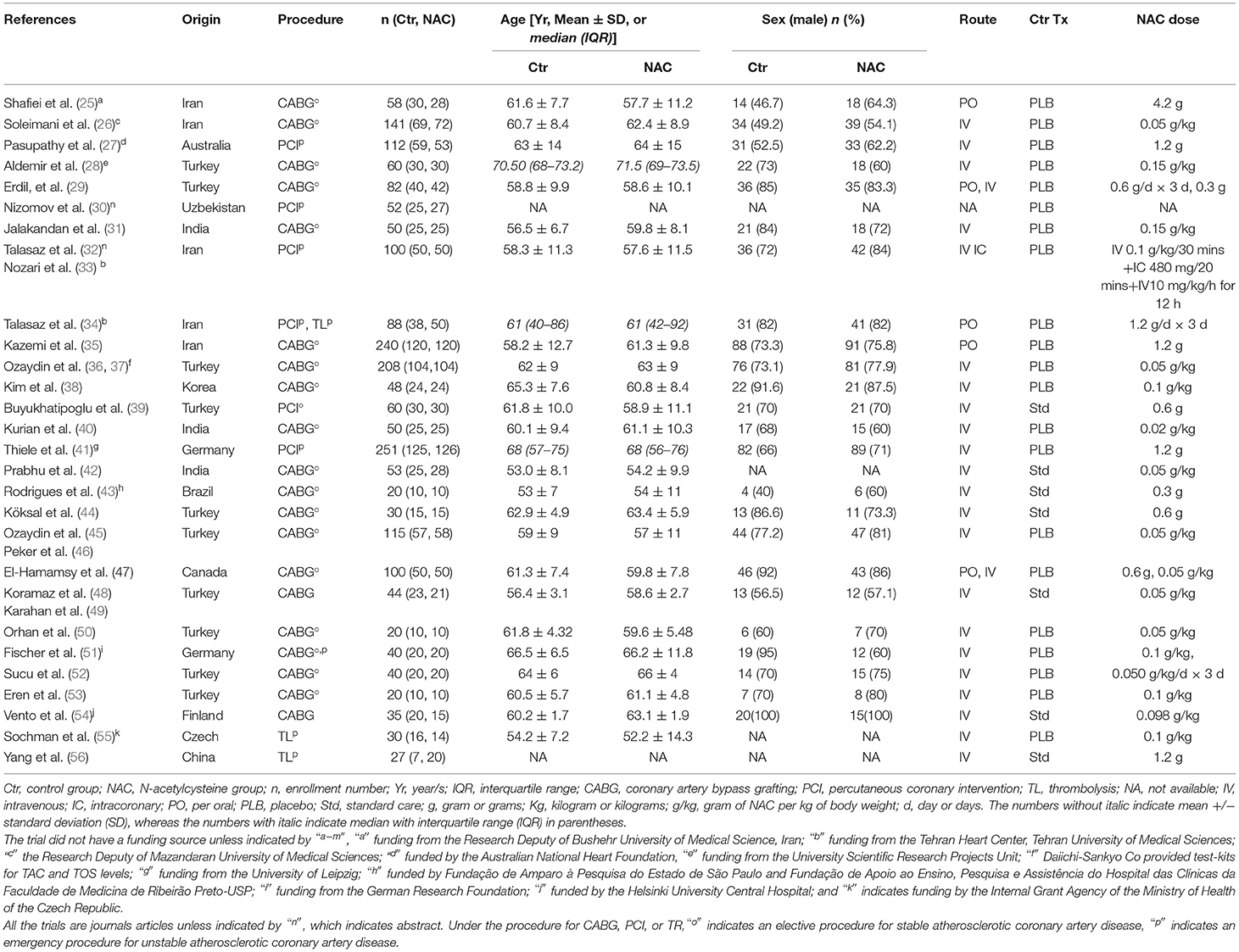
Table 1. The characteristics of 28 randomized controlled trials (RCTs) meeting the inclusion and exclusion criteria.
N-acetylcysteine was administered via intravenous (IV) infusion in the 23 trials and the oral route (PO) in the 3 trials. The two trials administered NAC via both IV and PO. One trial did not report the route of NAC administration or dose (30). The dose of NAC ranged from 20 to 150 mg/kg in the 19 trials, and 0.3–4.2 g in nine trials (Table 1). NAC was administered during coronary reperfusion in the 16 trials, while 8 trials administered NAC within 30–120 min before the start of reperfusion procedures. Four trials administered NAC the same day but before reperfusion procedure without specifying the timing (25, 28, 30, 34).
Among the 32 publications for the 28 trials included, 30 were journal articles and 2 were published abstracts. Twenty trials assessed the effect of NAC during CABG, five during PCI, two during thrombolysis, and one trail during PCI in combination with thrombolysis. Twenty-one trials had placebo controls, whereas seven practiced standard care in the control group. CABG was mostly elective for coronary artery disease, whereas the PCI and thrombolysis cases were urgent for acute coronary syndrome, except one trial where PCI was elective (39). All the included trials were published in English except one in Chinese (56), which was translated to English.
Risk of Bias Analysis
The results from the risk of bias analysis are indicated in Figure 2. Each domain was assigned with a low, unclear, or high risk of bias score. Among the 28 included trials, low risk of bias was noted in the 25 trials, while some concern for risk of bias was noted in 3 trials as indicated in Figure 2. None of the trials showed a high risk of bias. Hence all the trials were included for the synthesis of final results.
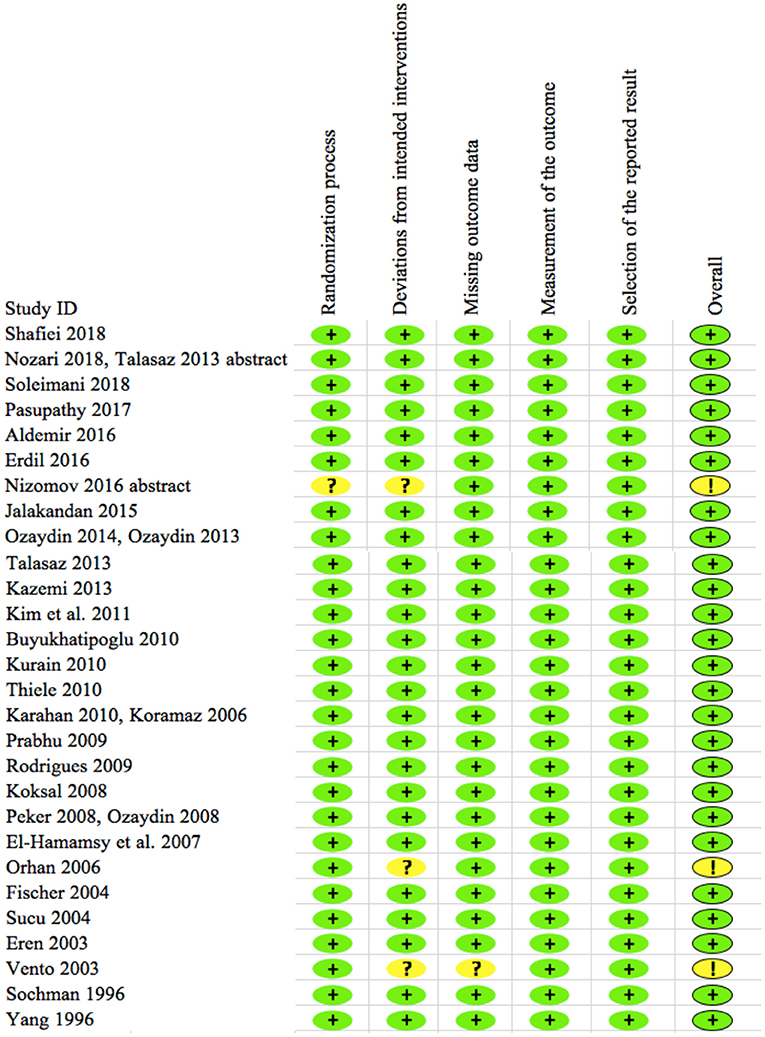
Figure 2. Risk of bias of 28 included trials. The plus sign in green (+) shows “low risk” for bias and the question or exclamation mark in yellow (?/!) shows “some concerns” for bias. None of the trials show high risk for bias per RoB2 analyses.
Effect of NAC Administration on the Clinical Outcomes
Serum cTn Elevation
Eight trials reported the means and SDs for the serum levels of either cardiac troponin I (cTnI) or troponin T (cTnT) following CABG or PCI (25, 33, 34, 40, 42, 46, 47, 49). The units of the measures are indicated in Figure 3 legend. Two of the reports did not include units for troponin (34, 47). The inquires to the authers of one report were not answered. A meta-analysis using SMD allows us to pool the values of cTnI and cTnT in a scaleless format into one analysis (57). This method does not require units for troponin. The means and SDs were extracted from each trial for the meta-analysis, with the form of troponin measured from each trial indicated in the figure legend (Figure 3A). Adding the enrollments from these trials yielded a total number of 271 for NAC and 262 for the control group. With a 95% CI, the pooled SMD was −0.80, with a range from −1.75 to 0.15 (p = 0.088). The value −0.8 implies that cTn decreased by 0.8 times the pooled SD, which was 1.1, as a result of the NAC treatment when compared with placebo or standard care. This indicates a notable decrease in the cTn levels, even though the p-value for such decrease is 0.088, not significant but showing a trend. As expected, a high heterogeneity was observed across the trials (I2 = 92%, p < 0.01).
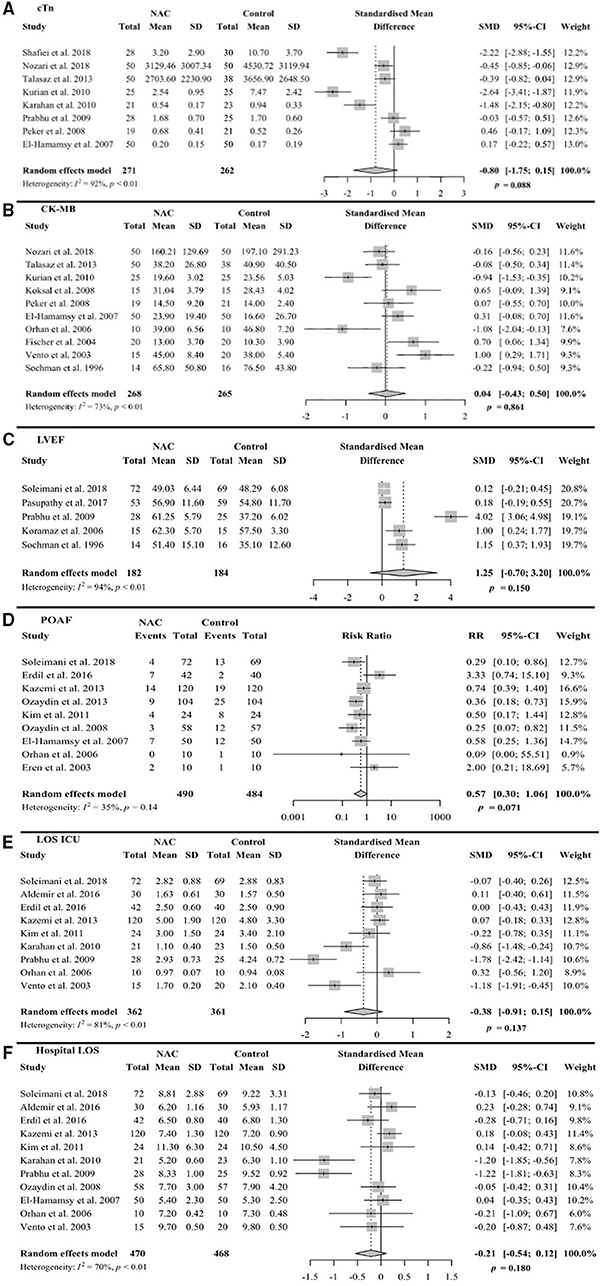
Figure 3. Forest plots of random effects model meta-analysis with 95% confidence interval (CI) comparing NAcetylcysteine (NAC) group vs. control group. The plots are showing standard mean difference (SMD) for continuous variables along with standard deviations (SD) or risk ratio (RR) for binary variables along with events for (A) serum troponin levels at 24 h after procedure. Shafiei et al. (25) measured cTnI in ng/ml, Nozari et al. (33) measured high sensitivity TnT (hs-TnT) in ng/dl, Talasaz et al. (32, 34) measured hs-TnT (unit not available, inquires not answered), Kurian et al. (40) measured cTnI in ng/ml, Karahan et al. (49) measured cTnT in ng/ml, Prabhu et al. (42) measured cTnI in ng/ml, Peker et al. (46) measured cTnT in ng/ml, and El-Hamamsy et al. (47) measured cTnT in ng/l (per response to inquires). (B) Serum CK-MB levels, (C) left ventricular ejection fraction (LVEF), (D) post-operative atrial fibrillation (POAF), (E) length of stay (LOS) in intensive care unit (ICU), and (F) LOS in hospital.
One trial was not entered into the meta-analysis due to reported median and interquartile ranges (IQR) for cTn, instead of means and SDs (43); hence, ineligible for grouping with the rest of the studies to perform the meta-analysis. This study used low dose NAC, 0.3 g, and did not indicate whether the reduction in the median cTn levels was significant due to NAC treatment [NAC group 4.8 (IQR 2.7, 6.0)] vs. control [5.5 (IQR 2.8, 6.4)]. Overall, our meta-analysis of eight trials suggests that there is a trend toward the reduced levels of cTn in the NAC group when compared with the control group with 95% CI.
Serum CK-MB Elevation
The means and SDs for the serum CK-MB concentrations were reported in 10 trials following CABG, PCI, or only pharmacological therapy (33, 34, 40, 44, 46, 47, 50, 51, 54, 55). With a meta-analysis using a 95% CI, we obtained a SMD value of 0.04, ranging from −0.43 to 0.50 (p = 0.861) (Figure 3B). The heterogeneity was moderate across the trials (I2 = 73%, p < 0.01). One trial was not compatible for the meta-analysis, due to reporting median and IQR instead of the means and SDs. This trial indicated no significant difference between the NAC and control groups [338 (IQR 290, 383) vs. 313 (IQR 260, 356) μmol/L/h, p = 0.13] (41). Overall, the NAC treatment had no significant effect on the procedure-associated elevation of CK-MB in the serum.
Infarct Size
Three trials measured the infarct size after a coronary reperfusion procedure at 7 days (27, 41, 55). The infarct size was measured using cardiac magnetic resonance imaging (CMR) (27, 41) or electrocardiography (55). While one trial showed no significant difference [NAC group 17.4% (IQR 9.1, 25.9, n = 126) vs. control group 14.3% (IQR 8.0, 26.2, n = 125), p = 0.47] (41), the two trials reported significantly smaller infarct size in the NAC vs. control groups [11% (IQR 4.1, 16.3, n = 53) vs. 16.5% (IQR 10.7, 24.2, n = 59), p = 0.02; or 16.3 ± 10.5, n = 14 vs. 24.4% ± 9.5, n = 16, p < 0.05) (27, 55). Furthermore, Pasupathy et al. (27) measured infarct size at 3 months and reported a significant reduction with the NAC treatment, with the infarct size in the NAC group being 5% on average (IQR 0.7, 12.4, n = 26) compared with the control group, which was 10.2% (IQR 6.8, 14.8, n = 29 p = 0.02). Overall, the two trials showed significant reduction of infarct size, while one trial showed no significant reduction; hence, the results are inconclusive with regard to whether or not NAC can reduce the infarct size.
Left Ventricular Ejection Fraction
Left ventricular ejection fraction was measured within 7 days after coronary reperfusion in the five trials with a sum of 182 enrollments for NAC and 184 for the control group (26, 27, 42, 48, 55). The reported means and SDs were used for the meta-analysis (Figure 3C). With a 95% CI, the SMD was 1.25 with a range of −0.70 to 3.20 (p = 0.150). The heterogeneity was high across the trials (I2 = 94%, p < 0.01). Although the statistical results do not support that NAC had a significant influence on the LVEF, the distribution of means plus SMD value point to a trend of NAC benefit in improving the LVEF.
Not included in the meta-analysis were 4 trials, with a total enrollment of 442, due to reported median instead of means or the differences in time points of the LVEF measurements (27, 30, 41, 56). Nizomov et al. (30) measured LVEF at 1- and 3-month after PCI and indicated a significantly smaller number of participants with LVEF <50% in the NAC vs. control groups [11% vs. 16% at 1 month, p = 0.046, and 4% vs. 16% at 3 months, p = 0.017], suggesting a benefit of the NAC treatment. Thiele et al. (41) reported that the median values of LVEF measured at 7 days were not significantly different [NAC 52.1% (IQR 43.5, 59.2) vs. control 50.6% (IQR 41.6, 58.6), p = 0.23]. Pasupathy et al. (27) did not find a significant difference (NAC 59.6 ± 11.1% vs. control 56.7 ± 10.5%, p = 0.33) in LVEF measured at 3 months. Yang et al. (56) neither revealed the time point of measurement nor reported significant difference in LVEF between the NAC and control groups (57 vs. 53%, no SDs or p-values provided). Overall, the results are inconclusive based on the reported median values of LVEF.
Post-operative Atrial Fibrillation
The incidence of POAF was reported in the 9 trials after CABG with a total combined patient number of 490 for NAC and 484 for control (26, 29, 35, 36, 38, 45, 47, 50, 53). It is known that POAF is a rare event following PCI, providing an explanation for the lack of POAF in the PCI trials. The number of patients developing POAF after reperfusion was registered either during the postoperative ICU stay or during the first 3 days of hospital stay. Using the binary outcome of the meta-analysis due to the report of events, we obtained the relative risk (RR) value of 0.57 with 95% CI, ranging from 0.30 to 1.06 (p = 0.071). The heterogeneity was low across the trials (I2 = 35%, P = 0.14). The meta-analysis points to a reduction, close to 50%, in the incidence of POAF with NAC treatment (Figure 3D).
LOS in ICU
The nine trials reported the LOS in ICU with means and SDs for an added-up enrollment of 362 for the NAC group or 361 for the control group (26, 28, 29, 35, 38, 42, 49, 50, 54). The meta-analysis yielded SMD −0.38 with 95% CI, ranging −0.91 to 0.15 (p = 0.137, Figure 3E). The heterogeneity was high across the trials (I2 = 81%, p < 0.01). Although the meta-analysis results did not reveal a significant difference per 95% CI, there is a trend toward the reduction of LOS in ICU by the NAC treatment.
LOS in Hospital
Hospital LOS with means and SDs were reported in 11 trials with a total enrollment adding up to 470 (NAC treated) or 468 (control) (26, 28, 29, 35, 38, 42, 45, 47, 49, 50, 54). The meta-analysis produced a SMD of −0.21 with 95% CI, ranging −0.54 to 0.12 (p = 0.180), and high heterogeneity (I2 = 70%, p < 0.01) (Figure 3F). Similar to LOS in ICU, a trend toward the reduction in hospital LOS in the NAC group is shown by the upper boundary of the 95% CI close to 0.
Effect of NAC on the Antioxidant Reservoir and ROS
Eighteen publications contained the measures for antioxidants and ROS, among which the nine trials had clinical outcome measures along with the lipid peroxidation product malondialdehyde (MDA). There is a lack of uniformity in the assays or time point of measurements between the studies, and most of the measures at a specific time point have less than five trials, which is not ideal for a meta-analysis. Nevertheless, for most of the measures, there was consistent reduction between the trials.
Total Antioxidant Capacity (TAC)
Seven trials measured the antioxidant levels after the coronary reperfusion procedures (31, 37, 39, 40, 42, 44, 56) (Table 2). These studies reported the levels of antioxidants at the baseline and different time points after coronary reperfusion, from 10 min to 48 h. The measurements included reduced glutathione (GSH) or the activities of glutathione peroxidase, glutathione reductase, superoxide dismutase, and catalase in the serum. Two trials reported the outcomes as total antioxidant capacity (TAC) without specifying the scaled measures (37, 39). One trial measured the urine levels of TAC in addition to the serum levels (39).
The measurements of GSH between 1 and 12 h showed significant increases in the three trials (Table 2). No significant differences in the activity of glutathione peroxidase were observed, but there was a slight improvement in glutathione reductase in the 2 trials (Table 2). The data on superoxide dismutase and catalase are inconsistent among the 3 trials (Table 2). Ozaydin et al. (37) reported an improvement of TAC at 24–48 h after NAC, but not by Buyukhatipoglu et al. (39). The latter trial used a much lower dose of NAC (0.6 g, which translates to 0.01 g/kg per 60 kg body weight) than the average dose of 0.1 g/kg dose. Overall, there is evidence to support the possibility that the administration of NAC before a coronary reperfusion procedure leads to an increase of glutathione redox system activity as expected.
Reactive Oxygen Species
Fourteen publications reported the levels of ROS markers after the coronary reperfusion procedures (25, 27, 31, 37, 39–42, 44, 48, 49, 52, 53, 56) (Table 3). The time points of measurements include the baseline and 15 min to 72 h after coronary reperfusion. The ROS was measured as MDA, myeloperoxidase (MPO) activity, oxidized glutathione (GSSG), advanced oxidation protein products (AOPP), or oxidized low density lipoprotein (LDL). The two trials reported the outcomes as the total oxidative stress (TOS) or total oxidant capacity (37, 39). All of these trials measured the serum levels of ROS markers except one trial, which also reported the urinary levels in addition to the serum levels (39).
Malondialdehyde was measured in the 9 trials, all of them showed significant reduction with the NAC treatment at different time points regardless of the reperfusion procedure performed, either PCI, CABG, or thrombolytic therapy (Table 3). MPO showed significant reduction in one trial but not the other (Table 3). Decreases of oxidized glutathione were observed in one trial (Table 3). Overall, there is evidence that the administration of NAC before the coronary reperfusion procedure significantly lowers the levels of ROS markers in the patients receiving NAC at various time points as compared with the control group.
Correlation of ROS Reduction With the Clinical Outcomes
Table 4 compares NAC induced improvements in the TAC or ROS reduction with the clinical outcome measures. It is evident that a significant improvement of TAC or ROS reduction due to NAC correlates with the reduced levels of cTn, increased LVEF, and decreased LOS in ICU or hospital. Such correlation supports the cause-effect relationship of TAC or ROS with the improved clinical outcomes. This suggests that NAC might have mediated the improved clinical outcomes through the reduction of ROS.
Sensitivity Analysis
We performed a sensitivity analysis to assess both the between-study heterogeneity and publication bias to ensure that the pooled effects for meta-analysis were indeed robust (58, 59). Between-study heterogeneity may be caused by a trial with either an extreme enrollment size or a larger impact on the pooled effect. To detect an influential trial, the Cook's distance, a well-established influential point detection method, was used (60). A trial may be considered as an influential case if the Cook's distance is >0.45 (17). Supplementary Figure 1 shows the Cook's distance for each measure in the meta-analyses, with the potential influential study highlighted in red. For cTn, CK-MB, or POAF, none of the trials have a Cook's distance over 0.45, indicating that there is no influential trial. For LVEF, LOS ICU, or hospital LOS, one potential influential study was detected, which is by Prabhu et al. (42). To verify if the influential trial affects the summary data or the conclusion, we compared the results from the random-effects model with vs. without the influential trial. Removal of Prabhu et al. (42) trial reduced the heterogeneity for LVEF, LOS ICU, or hospital LOS, but did not improve the p-value or the direction of SMD (Supplementary Table 1), and therefore did not affect our conclusions.
Another potential issue for the meta-analysis is the publication bias due to the trials with a small sample size (17). We checked the small-study effects using the funnel plots, which display the relationship between the SMD of studies against its standard error (61). When there is no publication bias, the distribution of the trials in points (one point represents each trial) is symmetric and fits into the shape of an upside-down funnel. In the case of this NAC meta-analysis, a few trials landed outside the funnel area, but the asymmetry is not across all the different outcome measures (as shown in Supplementary Figure 2). Since visual inspection can be subjective, we performed the Egger's regression test (62) to evaluate the asymmetry quantitatively in the funnel plot for the continuous outcome measures, cTn, CK-MB, LVEF, LOS in ICU, and LOS in hospital, and Peters' regression test (63) for the binary outcome measure POAF. The results are shown in Supplementary Table 2. None of the statistical tests have a significance at the threshold of 0.05, suggesting that the funnel plots are roughly symmetrical. This indicates that the publication bias is not a major concern in the meta-analysis.
Discussion
The administration of NAC prior to the coronary reperfusion procedures was associated with a trend toward the inhibition of cTn elevation, reduced incidence of POAF, and lowered levels of ROS. The decrease of cTn by NAC treatment is considered notable due to the summary SMD being −0.8 in reference to the SD of 1.1 from the meta-analysis of eight trials (Figure 3A). However, the overall p-value of 0.088 suggests that the decrease is close to 0.05 but not truly significant in the statistical analysis using 95% CI. While improvement in LVEF or reduction in ICU and hospital LOS were not statistically significant at 95% CI, the meta-analyses suggested a minor trend toward the improvement for these measures (Figures 3C,E,F). The effect of NAC on infarct size remains inconclusive due to the smaller number of trials. CK-MB represents the only outcome that did not show improvement with the administration of NAC. Given the fact that POAF is associated with older age and an increase in all-cause mortality (64), and whereas the level of cTn elevation predicts the incidence of adverse events and the risk of heart failure (2–4), adding NAC as an adjuvant therapy for reperfusion may provide benefit in these parameters. By decreasing these clinical complications, it could be expected that NAC administration might reduce the adverse events and the development of heart failure as well as possibly improving the long-term mortality.
An acute kidney injury (AKI) is often an additional complication of reperfusion procedures. We did not include this measure in our study due to the lack of such information in majority of the clinical trials on NAC for cardiac protection and the recently published systematic reviews with meta-analysis on the topic. Guo et al. (65) used the random effects model to evaluate the seven clinical trials for the effects of NAC on contrast-induced AKI in the patients with STEMI following PCI. This report showed a significantly reduced rate of AKI and all-cause hospital mortality with NAC compared with the placebo group (65). However, a meta-analysis of eight trials by Mei et al. using the random effects model for perioperative NAC among the patients with cardiac surgery concluded that there was no significant benefit in the prevention of AKI. The American College of Cardiology Foundation (ACCF) and American Heart Association (AHA) Guideline for Coronary Artery Bypass Graft Surgery noted the controversy surrounding the use of NAC for the prevention of CABG-associated AKI (66). However, the benefit of NAC as a potential intervention for POAF was not addressed.
Our data on POAF reduction with NAC are consistent with the published meta-analyses reporting the benefit of NAC for the patients with cardiac surgery. Two meta-analyses used the fixed effects model to determine the impact of NAC on POAF when administered before CABG among the eight trials, and showed a significant reduction of POAF (10, 12). In addition, the reduction of POAF was reported by Liu et al. (9), who summarized 10 publications (without the consideration of redundancy in trials) with meta-analysis using the fixed effects model. Wang et al. (11) registered seven trials for meta-analysis using the random effects model and discovered a trend toward improvement in the incidence of POAF with NAC.
The additional clinical measures are less convincing for the benefit of NAC examining in our data and that of others. Pereira et al. (8), compiled 12 trials for meta-analysis with the random effects model and showed a trend but not statistical significance toward an improvement in the post-operative cardiac insufficiency, ICU LOS, or hospital LOS, and incidence of post-operative acute myocardial infarction or cardiac arrhythmias. Gu et al. (10) did not find that NAC reduced ICU LOS using a fixed effects model for a meta-analysis of four trials. Similarly, Liu et al. (9) did not find significant improvement or a trend toward the improvement of ICU or hospital LOS with five trials. Wang et al. (11) showed neither statistical significance nor a trend toward improvement in the incidence of acute myocardial infarction, the need for ionotropic support, and ICU LOS, or hospital LOS with a random effects model meta-analysis of up to six trials. By consolidating the data from 10 trials, we observed a trend toward but not a significant reduction in LOS in ICU or hospital.
N-acetylcysteine is being used clinically for several decades. The main clinical uses for NAC to date include its mucolytic capacity in bronchi, as an antidote for acetaminophen toxicity, and as a protective agent against contrast-induced nephrotoxicity. NAC as a protective agent against reperfusion injury was first reported in 1992 by Sochman and Peregrin (6, 67, 68), who discovered total recovery of left ventricular function after acute myocardial infarction when NAC was administered along with the coronary artery thrombolysis during the PCI. Multiple RCTs have been published since to address possible beneficial effects of NAC during the coronary artery reperfusion. Twenty-eight of these RCTs reviewed in this study revealed a trend toward the improvement in several clinical measures, with a correlation to reduction of ROS or lipid peroxidation. The correlation approach provides evidence for the mechanistic basis of the observed benefit of NAC.
Strengths and Limitations
We have included three types of coronary artery revascularization procedures for the clinical practice, PCI, thrombolytics, and CABG. This differs from the other published meta-analyses, which focused on one type of reperfusion procedure. Additionally, we have evaluated the most common clinical measures, cTn or CK-MB, LVEF, POAF, and ICU or hospital LOS, and provided a correlation for the levels of antioxidants or ROS to the clinical measures. This differentiates our study from the other published meta-analyses.
The included RCTs were from multiple countries, with most trials having a placebo control. There were minimal losses to follow-up across the trials. The data were generated from multiple healthcare centers with multi-ethnicities due to a diverse distribution of recruitment among the different countries. Additionally, none of the RCTs presented here were funded by a for-profit organization and the risk of bias was low in most of the trials.
The negative factors affecting our analysis power include limited regions of the trials, sample size, gender distribution, and substantial heterogeneity. While there was no restriction on the country or language for trial inclusion, over 50% of the evaluated studies originated from Turkey (10 trials) or Iran (5 trials), and none of the trials were carried out in the United States. Although many factors may explain the uneven distribution for the trial origins, the genetic background in association with a unique region, and the differences in socioeconomic status for the healthcare provision may prohibit extrapolation of the findings to all case scenarios worldwide. Additionally, most of the included trials had an enrollment below 100 individuals. The participants were mostly middle-aged men, prohibiting the generalization to other age groups or female patients.
We have detected a large between-study heterogeneity in most of the outcome measures, with I2 varying from 35 to 94% (Figure 3). Several variables in the trials contributed to the substantial heterogeneity: (a) non-uniform coronary reperfusion procedures, with either PCI, CABG, or thrombolysis in different trials; (b) the dosage and the route of NAC administration differed among the trials, with three trials using the low doses of NAC, 0.3–0.6 g (39, 43, 44); (c) the patient populations carried distinctive diagnoses, from acute coronary syndrome requiring an emergency reperfusion procedure to stable coronary artery diseases treated with an elective reperfusion protocol; (d) a lack of information on timing from the onset of chest pain to the reperfusion procedures. The large regional differences in such timing may affect the clinical outcome of reperfusion and NAC treatment; and (e) the healthcare facility and supportive infrastructure among the different countries or regions may influence the clinical outcome. If it had been possible to increase the sample sizes or reduce the heterogeneity, the statistical analyses would likely have yielded the p-values indicating significant differences supporting the benefit of NAC on multiple clinical outcome measures.
Clinical Implications
Our findings suggest a trend toward the benefit of NAC treatment. The trend in the reduction of cTn suggests a potential reduction of cardiac injury by NAC. It is important to note that NAC, despite its low cost and multiple clinical implications already, is not free of side effects. Nausea and vomiting may be associated with an unpleasant odor during oral intake. For intravenous NAC, an anaphylactoid reaction occurs in 8.2% cases, such as cutaneous (acute flushing, pruritus, and rash) or systemic symptoms (bronchospasm, angioedema, hypotension, and chest pain) (69, 70). Additionally, NAC may have a negative impact on hemostasis in the patients under certain conditions. In a post-hoc analysis of an RCT of NAC in the patients undergoing cardiac surgery with an estimated glomerular filtration rate of ≤60 ml/min, administration of NAC (100 mg/kg IV bolus, followed by 20 mg/kg/h until 4 h after CABG) was associated with a greater blood loss and an increased need for transfusions (71). Therefore, the benefit of NAC remains to be fully established with larger controlled clinical trials measuring multiple clinical end-points. The risk vs. benefit analysis in such a trial would also be needed.
If well done, the RCTs with large numbers of patients were shown to be positive, then the addition of antioxidant therapy to the patients following reperfusion therapy or cardiopulmonary bypass would be a simple and inexpensive therapy. NAC, vitamin C, and other antioxidant agents are generic, inexpensive, generally safe, and would presumably be administered for a relatively short period of time, possibly hours to days. The long-term clinical implications of such therapy are not yet known and would need to be assessed.
Data Availability Statement
The original contributions presented in the study are included in the article/Supplementary Material, further inquiries can be directed to the corresponding author.
Author Contributions
SK: study design, building search strategy for six electronic databases, acquisition of data, qualitative analysis, interpretation of data, writing and drafting the manuscript, and coordinating the project. AC: independently reviewed the literature and evaluated all the selected trials, validated the acquisition of data, provided input, and edit to the manuscript. YL: quantitative analysis and conducted meta-analysis. LA: supervising statistician and manuscript editing. JA: a practicing cardiologist who helped with the clinical interpretation of the data, and manuscript editing. QC: initiated the conception, supervised the study, reviewed the literature, and revised the manuscript. All the authors edited and have approved this version of manuscript to be published.
Funding
Research works under QC's direction are supported by NIH R01 GM125212, R01 GM126165, Holsclaw Endowment, and The University of Arizona College of Pharmacy start-up fund.
Conflict of Interest
The authors declare that the research was conducted in the absence of any commercial or financial relationships that could be construed as a potential conflict of interest.
Publisher's Note
All claims expressed in this article are solely those of the authors and do not necessarily represent those of their affiliated organizations, or those of the publisher, the editors and the reviewers. Any product that may be evaluated in this article, or claim that may be made by its manufacturer, is not guaranteed or endorsed by the publisher.
Acknowledgments
We appreciate the input from librarian Rachel Walden for the literature search. The authors sincerely thank Ms. Hongting Diao for translating one of our included RCTs from Chinese to English, and Mr. Benjamin Yuchen for English editing of the manuscript.
Supplementary Material
The Supplementary Material for this article can be found online at: https://www.frontiersin.org/articles/10.3389/fcvm.2021.752939/full#supplementary-material
References
1. Yellon DM, Hausenloy DJ. Myocardial reperfusion injury. N Engl J Med. (2007) 357:1121–35. doi: 10.1056/NEJMra071667
2. Ottani F, Galvani M, Nicolini FA, Ferrini D, Pozzati A, Di Pasquale G, et al. Elevated cardiac troponin levels predict the risk of adverse outcome in patients with acute coronary syndromes. Am Heart J. (2000) 140:917–27. doi: 10.1067/mhj.2000.111107
3. Amin AP, Mukhopadhyay E, Napan S, Mamtani M, Kelly RF, Kulkarni H. Value of early cardiac troponin I to predict long-term adverse events after coronary artery bypass graft surgery in patients presenting with acute coronary syndromes. Clin Cardiol. (2009) 32:386–92. doi: 10.1002/clc.20579
4. Stelzle D, Shah ASV, Anand A, Strachan FE, Chapman AR, Denvir MA, et al. High-sensitivity cardiac troponin I and risk of heart failure in patients with suspected acute coronary syndrome: a cohort study. Eur Heart J Qual Care Clin Outcomes. (2018) 4:36–42. doi: 10.1093/ehjqcco/qcx022
5. Ben-Yehuda O, Chen S, Redfors B, McAndrew T, Crowley A, Kosmidou I, et al. Impact of large periprocedural myocardial infarction on mortality after percutaneous coronary intervention and coronary artery bypass grafting for left main disease: an analysis from the EXCEL trial. Eur Heart J. (2019) 40:1930–41. doi: 10.1093/eurheartj/ehz113
6. Kaireviciute D, Aidietis A, Lip GY. Atrial fibrillation following cardiac surgery: clinical features and preventative strategies. Eur Heart J. (2009) 30:410–25. doi: 10.1093/eurheartj/ehn609
7. Engelmann MD, Svendsen JH. Inflammation in the genesis and perpetuation of atrial fibrillation. Eur Heart J. (2005) 26:2083–92. doi: 10.1093/eurheartj/ehi350
8. Pereira J, El Dib R, Braz L, Escudero J, Hayes J, Johnston B, et al. N-acetylcysteine use among patients undergoing cardiac surgery: a systematic review and meta-analysis of randomized trials. PLoS ONE. (2019) 14:e0213862. doi: 10.1371/journal.pone.0213862
9. Liu XH, Xu CY, Fan GH. Efficacy of N-acetylcysteine in preventing atrial fibrillation after cardiac surgery: a meta-analysis of published randomized controlled trials. BMC Cardiovasc Disord. (2014) 14:52. doi: 10.1186/1471-2261-14-52
10. Gu WJ, Wu ZJ, Wang PF, Htet Aung LH, Yin RX. N-acetylcysteine supplementation for the prevention of atrial fibrillation after cardiac surgery: a meta-analysis of eight randomized controlled trials. BMC Cardiovasc Disord. (2012) 12:10. doi: 10.1186/1471-2261-12-10
11. Wang G, Bainbridge D, Martin J, Cheng D. N-acetylcysteine in cardiac surgery: do the benefits outweigh the risks? A meta-analytic reappraisal. J Cardiothorac Vasc Anesth. (2011) 25:268–75. doi: 10.1053/j.jvca.2010.04.022
12. Baker WL, Anglade MW, Baker EL, White CM, Kluger J, Coleman CI. Use of N-acetylcysteine to reduce post-cardiothoracic surgery complications: a meta-analysis. Eur J Cardiothorac Surg. (2009) 35:521–7. doi: 10.1016/j.ejcts.2008.11.027
13. Masoudi FA, Ponirakis A, de Lemos JA, Jollis JG, Kremers M, Messenger JC, et al. Trends in U.S. Cardiovascular Care 2016 Report From 4 ACC National Cardiovascular Data Registries. J Am Coll Cardiol. (2017) 69:1427–50. doi: 10.1016/j.jacc.2016.12.005
14. Moher D, Liberati A, Tetzlaff J, Altman DG, Group P. Preferred reporting items for systematic reviews and meta-analyses: the PRISMA statement. Ann Intern Med. (2009) 151:264–9. doi: 10.7326/0003-4819-151-4-200908180-00135
15. Chesnaye NC, Szummer K, Bárány P, Heimbürger O, Magin H, Almquist T, et al. Association between renal function and troponin T over time in stable chronic kidney disease patients. J Am Heart Assoc. (2019) 8:e013091. doi: 10.1093/ndt/gfz106.FP411
16. Ouzzani M, Hammady H, Fedorowicz Z, Elmagarmid A. Rayyan-a web and mobile app for systematic reviews. Syst Rev. (2016) 5:210. doi: 10.1186/s13643-016-0384-4
17. Harrer M, Cuijpers P, Furukawa TA, Ebert DD. Doing Meta-Analysis with R: A Hands-On Guide. Boca Raton, FL; London: Chapmann & Hall/CRC Press (2021). doi: 10.1201/9781003107347
18. Sidik K, Jonkman JN. A comparison of heterogeneity variance estimators in combining results of studies. Stat Med. (2007) 26:1964–81. doi: 10.1002/sim.2688
19. IntHout J, Ioannidis JP, Borm GF. The Hartung-Knapp-Sidik-Jonkman method for random effects meta-analysis is straightforward and considerably outperforms the standard DerSimonian-Laird method. BMC Med Res Methodol. (2014) 14:25. doi: 10.1186/1471-2288-14-25
20. Follmann DA, Proschan MA. Valid inference in random effects meta-analysis. Biometrics. (1999) 55:732–7. doi: 10.1111/j.0006-341X.1999.00732.x
22. Balduzzi S, Rücker G, G S. How to perform a meta-analysis with R: a practical tutorial. Evid Based Mental Health. (2019) 22:153–60. doi: 10.1136/ebmental-2019-300117
23. Viechtbauer W. Conducting meta-analyses in R with the metafor package. J Statist Software. (2010) 36:1–48. doi: 10.18637/jss.v036.i03
24. Harrer M, Cuijpers P, Furukawa T, Ebert DD. dmetar: Companion R Package For The Guide 'Doing Meta-Analysis in R'. R package version 0.9 (2019).
25. Shafiei E, Bahtoei M, Raj P, Ostovar A, Iranpour D, Akbarzadeh S, et al. Effects of N-acetyl cysteine and melatonin on early reperfusion injury in patients undergoing coronary artery bypass grafting: a randomized, open-labeled, placebo-controlled trial. Medicine. (2018) 97:e11383. doi: 10.1097/MD.0000000000011383
26. Soleimani A, Habibi MR, Hasanzadeh Kiabi F, Alipour A, Habibi V, Azizi S, et al. The effect of intravenous N-acetylcysteine on prevention of atrial fibrillation after coronary artery bypass graft surgery: a double-blind, randomised, placebo-controlled trial. Kardiol Pol. (2018) 76:99–106. doi: 10.5603/KP.a2017.0183
27. Pasupathy S, Tavella R, Grover S, Raman B, Procter NEK, Du YT, et al. Early use of N-acetylcysteine with nitrate therapy in patients undergoing primary percutaneous coronary intervention for ST-segment-elevation myocardial infarction reduces myocardial infarct size (the NACIAM Trial [N-acetylcysteine in Acute Myocardial Infarction]). Circulation. (2017) 136:894–903. doi: 10.1161/CIRCULATIONAHA.117.027575
28. Aldemir M, Koca H, Baki E, Carsanba G, Kavrut N, Kavakli A, et al. Effects of N-acetyl cysteine on renal functions evaluated by blood neutrophil gelatinase-associated lipocalin levels in geriatric patients undergoing coronary artery bypass grafting. Anatolian J Cardiol. (2016) 16:504–11. doi: 10.5152/AnatolJCardiol.2015.6287
29. Erdil N, Eroglu T, Akca B, Disli OM, Yetkin O, Colak MC, et al. The effects of N-acetylcysteine on pulmonary functions in patients undergoing on-pump coronary artery surgery: a double blind placebo controlled study. Eur Rev Med Pharmacol Sci. (2016) 20:180–7.
30. Nizomov A, Toshev B, Mukhamedova M, Berdiqulov J, Nizomov A, Toshev B, et al. N-acetylcysteine in a combination therapy reduces myocardial damage in patients with acute coronary syndrome and improves left ventricular function after percutaneous coronary intervention. Atherosclerosis. (2016) 252:E184–5. doi: 10.1016/j.atherosclerosis.2016.07.857
31. Jalakandan B, Bhaskaran K, Hiremath V, Jalakandan B, Bhaskaran K, Hiremath VR. Effect of n-acetylcysteine on oxidative stress in patients undergoing off pump coronary artery bypass grafting. J Evol Medand Dental Sci-jemds. (2015) 4:13754–63. doi: 10.14260/jemds/2015/1963
32. Talasaz A, Salamzadeh J, Khalili H, Eshraghi A, Bahremand M, Talasaz AH, et al. Evaluating the effect of intracoronary N-acetylcysteine on myocardial reperfusion markers following primary percutaneous coronary intervention in patients with STEMI. Eur Heart J. (2013) 34:610. doi: 10.1093/eurheartj/eht309.P3283
33. Nozari Y, Eshraghi A, Talasaz A, Bahremand M, Salamzadeh J, Salarifar M, et al. Protection from reperfusion injury with intracoronary N-acetylcysteine in patients with STEMI undergoing primary percutaneous coronary intervention in a cardiac tertiary center. Am J Cardiovasc Drugs. (2018) 18:213–21. doi: 10.1007/s40256-017-0258-8
34. Talasaz AH, Khalili H, Jenab Y, Salarifar M, Broumand MA, Darabi F. N-Acetylcysteine effects on transforming growth factor-beta and tumor necrosis factor-alpha serum levels as pro-fibrotic and inflammatory biomarkers in patients following ST-segment elevation myocardial infarction. Drugs R D. (2013) 13:199–205. doi: 10.1007/s40268-013-0025-5
35. Kazemi B, Akbarzadeh F, Safaei N, Yaghoubi A, Shadvar K, Ghasemi K. Prophylactic high-dose oral-N-acetylcysteine does not prevent atrial fibrillation after heart surgery: a prospective double blind placebo-controlled randomized clinical trial. Pacing Clin Electrophysiol. (2013) 36:1211–9. doi: 10.1111/pace.12190
36. Ozaydin M, Icli A, Yucel H, Akcay S, Peker O, Erdogan D, et al. Metoprolol vs. carvedilol or carvedilol plus N-acetyl cysteine on post-operative atrial fibrillation: a randomized, double-blind, placebo-controlled study. Eur Heart J. (2013) 34:597–604. doi: 10.1093/eurheartj/ehs423
37. Ozaydin M, Peker O, Erdogan D, Akcay S, Yucel H, Icli A, et al. Oxidative status, inflammation, and postoperative atrial fibrillation with metoprolol vs carvedilol or carvedilol plus N-acetyl cysteine treatment. Clin Cardiol. (2014) 37:300–6. doi: 10.1002/clc.22249
38. Kim JC, Hong SW, Shim JK, Yoo KJ, Chun DH, Kwak YL. Effect of N-acetylcysteine on pulmonary function in patients undergoing off-pump coronary artery bypass surgery. Acta Anaesthesiol Scand. (2011) 55:452–9. doi: 10.1111/j.1399-6576.2011.02407.x
39. Buyukhatipoglu H, Sezen Y, Yildiz A, Bas M, Kirhan I, Ulas T, et al. N-acetylcysteine fails to prevent renal dysfunction and oxidative stress after noniodine contrast media administration during percutaneous coronary interventions. Pol Arch Med Wewn. (2010) 120:383–9. doi: 10.20452/pamw.975
40. Kurian GA, Paddikkala J. N-acetylcysteine and magnesium improve biochemical abnormalities associated with myocardial ischaemic reperfusion in South Indian patients undergoing coronary artery bypass grafting: a comparative analysis. Singapore Med J. (2010) 51:381–8.
41. Thiele H, Hildebrand L, Schirdewahn C, Eitel I, Adams V, Fuernau G, et al. Impact of high-dose N-acetylcysteine versus placebo on contrast-induced nephropathy and myocardial reperfusion injury in unselected patients with ST-segment elevation myocardial infarction undergoing primary percutaneous coronary intervention. The LIPSIA-N-ACC (Prospective, Single-Blind, Placebo-Controlled, Randomized Leipzig Immediate PercutaneouS Coronary Intervention Acute Myocardial Infarction N-ACC) Trial. J Am Coll Cardiol. (2010) 55:2201–9. doi: 10.1016/j.jacc.2009.08.091
42. Prabhu A, Sujatha DI, Kanagarajan N, Vijayalakshmi MA, Ninan B. Effect of N-acetylcysteine in attenuating ischemic reperfusion injury in patients undergoing coronary artery bypass grafting with cardiopulmonary bypass. Ann Vasc Surg. (2009) 23:645–51. doi: 10.1016/j.avsg.2008.12.005
43. Rodrigues AJ, Évora PRB, Bassetto S, Alves L Jr, Scorzoni Filho A, Origuela EA, Vicente WVA. Blood cardioplegia with N-acetylcysteine may reduce coronary endothelial activation and myocardial oxidative stress. Heart Surg Forum. (2009) 12:E44–E8. doi: 10.1532/HSF98.20081134
44. Köksal H, Rahman A, Burma O, Halifeoglu I, Bayar M. The effects of low dose N-acetylcysteine (NAC) as an adjunct to cardioplegia in coronary artery bypass surgery. Anatolian J Cardiol. (2008) 8:437–43.
45. Ozaydin M, Peker O, Erdogan D, Kapan S, Turker Y, Varol E, et al. N-acetylcysteine for the prevention of postoperative atrial fibrillation: a prospective, randomized, placebo-controlled pilot study. Eur Heart J. (2008) 29:625–31. doi: 10.1093/eurheartj/ehn011
46. Peker O, Peker T, Erdogan D, Ozaydin M, Kapan S, Sutcu R, et al. Effects of intravenous N-acetylcysteine on periprocedural myocardial injury after on-pump coronary artery by-pass grafting. J Cardiovasc Surg. (2008) 49:527–31.
47. El-Hamamsy I, Stevens LM, Carrier M, Pellerin M, Bouchard D, Demers P, et al. Effect of intravenous N-acetylcysteine on outcomes after coronary artery bypass surgery: a randomized, double-blind, placebo-controlled clinical trial. J Thorac Cardiovasc Surg. (2007) 133:7–12. doi: 10.1016/j.jtcvs.2006.05.070
48. Koramaz I, Pulathan Z, Usta S, Karahan SC, Alver A, Yaris E, et al. Cardioprotective effect of cold-blood cardioplegia enriched with N-acetylcysteine during coronary artery bypass grafting. Ann Thorac Surg. (2006) 81:613–8. doi: 10.1016/j.athoracsur.2005.08.013
49. Karahan SC, Koramaz I, Altun G, Ucar U, Topbas M, Mentese A, et al. Ischemia-modified albumin reduction after coronary bypass surgery is associated with the cardioprotective efficacy of cold-blood cardioplegia enriched with N-acetylcysteine: a preliminary study. Eur Surg Res. (2010) 44:30–6. doi: 10.1159/000262324
50. Orhan G, Yapici N, Yuksel M, Sargin M, Senay S, Yalçin AS, et al. Effects of N-acetylcysteine on myocardial ischemia-reperfusion injury in bypass surgery. Heart Vessels. (2006) 21:42–7. doi: 10.1007/s00380-005-0873-1
51. Fischer UM, Tossios P, Huebner A, Geissler HJ, Bloch W, Mehlhorn U. Myocardial apoptosis prevention by radical scavenging in patients undergoing cardiac surgery. J Thorac Cardiovasc Surg. (2004) 128:103–8. doi: 10.1016/j.jtcvs.2003.11.034
52. Sucu N, Cinel I, Unlu A, Aytacoglu B, Tamer L, Kocak Z, et al. N-Acetylcysteine for preventing pump-induced oxidoinflammatory response during cardiopulmonary bypass. Surg Today. (2004) 34:237–42. doi: 10.1007/s00595-003-2699-8
53. Eren N, Cakir O, Oruc A, Kaya Z, Erdinc L. Effects of N-acetylcysteine on pulmonary function in patients undergoing coronary artery bypass surgery with cardiopulmonary bypass. Perfusion. (2003) 18:345–50. doi: 10.1191/0267659103pf696oa
54. Vento AE, Nemlander A, Aittomäki J, Salo J, Karhunen J, Rämö OJ. N-acetylcysteine as an additive to crystalloid cardioplegia increased oxidative stress capacity in CABG patients. Scand Cardiovasc J. (2003) 37:349–55. doi: 10.1080/14017430310015406
55. Sochman J, Vrbska J, Musilova B, Rocek M. Infarct Size Limitation: acute N-acetylcysteine defense (ISLAND trial): preliminary analysis and report after the first 30 patients. Clin Cardiol. (1996) 19:94–100. doi: 10.1002/clc.4960190205
56. Yang J, Stafford I, Arstall MA, Horowitz JD. Effects of N-acetylcysteine on oxidation stress in patients with acute myocardial infarction. Chinese J Cardiol. (1996) 24:282–4.
57. Andrade C. Mean Difference, Standardized Mean Difference (SMD), and their use in meta-analysis: as simple as it gets. J Clin Psychiatry. (2020) 81:20f13681. doi: 10.4088/JCP.20f13681
58. Patsopoulos NA, Evangelou E, Ioannidis JP. Sensitivity of between-study heterogeneity in meta-analysis: proposed metrics and empirical evaluation. Int J Epidemiol. (2008) 37:1148–57. doi: 10.1093/ije/dyn065
59. Copas J, Shi JQ. Meta-analysis, funnel plots and sensitivity analysis. Biostatistics. (2000) 1:247–62. doi: 10.1093/biostatistics/1.3.247
60. Cook RD. Detection of influential observation in linear regression. Technometric. (1977) 19:15–8. doi: 10.1080/00401706.1977.10489493
61. Pustejovsky JE, Rogers MA. Testing for funnel plot asymmetry of standardized mean differences. Res Synth Methods. (2019) 10:57–71. doi: 10.1002/jrsm.1332
62. Egger M, Davey Smith G, Schneider M, Minder C. Bias in meta-analysis detected by a simple, graphical test. BMJ. (1997) 315:629–34. doi: 10.1136/bmj.315.7109.629
63. Peters JL, Sutton AJ, Jones DR, Abrams KR, Rushton L. Comparison of two methods to detect publication bias in meta-analysis. JAMA. (2006) 295:676–80. doi: 10.1001/jama.295.6.676
64. Almassi GH, Hawkins RB, Bishawi M, Shroyer AL, Hattler B, Quin JA, et al. New-onset postoperative atrial fibrillation impact on 5-year clinical outcomes and costs. J Thorac Cardiovasc Surg. (2021) 161:1803–10. doi: 10.1016/j.jtcvs.2019.10.150
65. Guo Z, Liu J, Lei L, Xue Y, Liu L, Huang H, et al. Effect of N-acetylcysteine on prevention of contrast-associated acute kidney injury in patients with STEMI undergoing primary percutaneous coronary intervention: a systematic review and meta-analysis of randomised controlled trials. BMJ Open. (2020) 10:e039009. doi: 10.1136/bmjopen-2020-039009
66. Hillis LD, Smith PK, Anderson JL, Bittl JA, Bridges CR, Byrne JG, et al. ACCF/AHA Guideline for Coronary Artery Bypass Graft Surgery. A report of the American College of Cardiology Foundation/American Heart Association Task Force on Practice Guidelines. Developed in collaboration with the American Association for Thoracic Surgery, Society of Cardiovascular Anesthesiologists, and Society of Thoracic Surgeons. J Am Coll Cardiol. (2011). 58:e123–210.
67. Sochman J, Peregrin JH. Total recovery of left ventricular function after acute myocardial infarction: comprehensive therapy with streptokinase, N-acetylcysteine and percutaneous transluminal coronary angioplasty. Int J Cardiol. (1992) 35:116–8. doi: 10.1016/0167-5273(92)90063-9
68. Sochman J. N-acetylcysteine in acute cardiology: 10 years later: what do we know and what would we like to know? J Am Coll Cardiol. (2002) 39:1422–8. doi: 10.1016/S0735-1097(02)01797-7
69. Yarema M, Chopra P, Sivilotti MLA, Johnson D, Nettel-Aguirre A, Bailey B, et al. Anaphylactoid reactions to intravenous N-acetylcysteine during treatment for acetaminophen poisoning. J Med Toxicol. (2018) 14:120–7. doi: 10.1007/s13181-018-0653-9
70. Sandilands EA, Bateman DN. Adverse reactions associated with acetylcysteine. Clin Toxicol. (2009) 47:81–8. doi: 10.1080/15563650802665587
Keywords: N-acetylcysteine, coronary artery bypass, percutaneous coronary intervention, atrial fibrillation, antioxidants, reactive oxygen species, acute coronary syndrome, stable angina
Citation: Khan SA, Campbell AM, Lu Y, An L, Alpert JS and Chen QM (2021) N-Acetylcysteine for Cardiac Protection During Coronary Artery Reperfusion: A Systematic Review and Meta-Analysis of Randomized Controlled Trials. Front. Cardiovasc. Med. 8:752939. doi: 10.3389/fcvm.2021.752939
Received: 03 August 2021; Accepted: 08 October 2021;
Published: 19 November 2021.
Edited by:
Chen Yan, University of Rochester, United StatesReviewed by:
Si Chen, University of Rochester, United StatesTingting Wang, Huazhong University of Science and Technology, China
Jianxin Sun, Thomas Jefferson University, United States
Copyright © 2021 Khan, Campbell, Lu, An, Alpert and Chen. This is an open-access article distributed under the terms of the Creative Commons Attribution License (CC BY). The use, distribution or reproduction in other forums is permitted, provided the original author(s) and the copyright owner(s) are credited and that the original publication in this journal is cited, in accordance with accepted academic practice. No use, distribution or reproduction is permitted which does not comply with these terms.
*Correspondence: Qin M. Chen, cWNoZW4xJiN4MDAwNDA7cGhhcm1hY3kuYXJpem9uYS5lZHU=