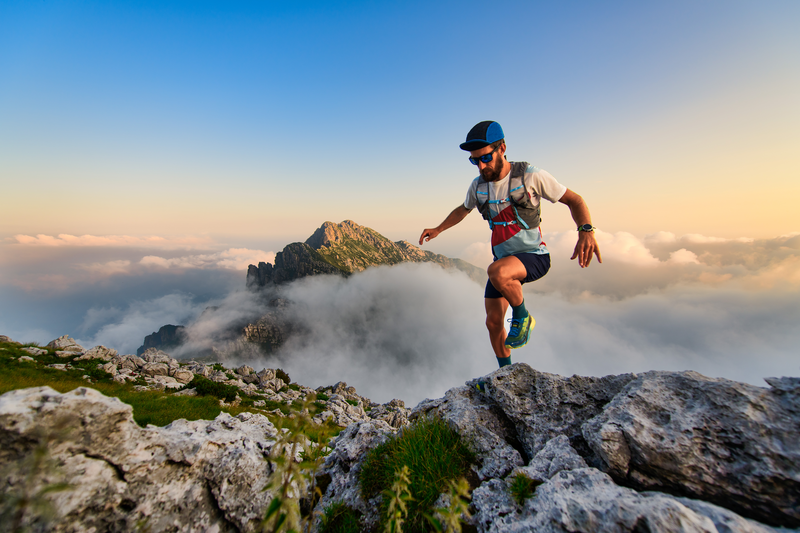
94% of researchers rate our articles as excellent or good
Learn more about the work of our research integrity team to safeguard the quality of each article we publish.
Find out more
ORIGINAL RESEARCH article
Front. Cardiovasc. Med. , 25 October 2021
Sec. General Cardiovascular Medicine
Volume 8 - 2021 | https://doi.org/10.3389/fcvm.2021.750926
This article is part of the Research Topic Cardiovascular Risk Factors: Related vascular injury and new molecular biomarkers View all 23 articles
Objective: Hydrogen sulfide (H2S) is a gaseous signaling molecule and redox factor important for cardiovascular function. Deficiencies in its production or bioavailability are implicated in atherosclerotic disease. However, it is unknown if circulating H2S levels differ between vasculopaths and healthy individuals, and if so, whether H2S measurements can be used to predict surgical outcomes. Here, we examined: (1) Plasma H2S levels in patients undergoing vascular surgery and compared these to healthy controls, and (2) the association between H2S levels and mortality in a cohort of patients undergoing surgical revascularization.
Methods: One hundred and fifteen patients undergoing carotid endarterectomy, open lower extremity revascularization or lower leg amputation were enrolled at a single institution. Peripheral blood was also collected from a matched control cohort of 20 patients without peripheral or coronary artery disease. Plasma H2S production capacity and sulfide concentration were measured using the lead acetate and monobromobimane methods, respectively.
Results: Plasma H2S production capacity and plasma sulfide concentrations were reduced in patients with PAD (p < 0.001, p = 0.013, respectively). Patients that underwent surgical revascularization were divided into high vs. low H2S production capacity groups by median split. Patients in the low H2S production group had increased probability of mortality (p = 0.003). This association was robust to correction for potentially confounding variables using Cox proportional hazard models.
Conclusion: Circulating H2S levels were lower in patients with atherosclerotic disease. Patients undergoing surgical revascularization with lower H2S production capacity, but not sulfide concentrations, had increased probability of mortality within 36 months post-surgery. This work provides insight on the role H2S plays as a diagnostic and potential therapeutic for cardiovascular disease.
Hydrogen sulfide (H2S) is a redox modifying and diffusible gasotransmitter that plays numerous physiologic roles across various organ systems including the cardiovascular system (1). While high levels of exogenous H2S are toxic, increased levels within a supraphysiologic range have been shown to mediate many beneficial effects ranging from stress resistance to longevity (2–4). Exogenous H2S has been shown to extend lifespan and increase stress resistance in Caenorhabditis elegans (C. elegans) and Drosophila melanogaster (D. melanogaster), and to protect mice from lethal levels of hypoxia, although effects on human lifespan and survival remain to be determined (2, 5). H2S has also emerged as a critical mediator of vascular homeostasis (6) in preclinical models through its functions as a vasodilator (7), antioxidant (8), oxygen sensor (9, 10), immunomodulator (11), and anti-inflammatory gas (12). Individuals with diabetes-related vascular inflammation had decreased circulating sulfide levels (13). As atherosclerosis is a chronic inflammatory disease, it is important to note H2S is shown to regulate several atherosclerotic cellular and inflammatory processes (14–17).
While H2S has been implicated in the pathogenesis of multiple cardiovascular disease processes, the measurement of H2S is arduous and often relies on indirect measures and surrogates (18–23). Nevertheless, further characterizing the role of H2S in systemic disease states such as peripheral arterial disease (PAD) may yield important insights on potential diagnostic and therapeutic applications.
Thus, our objective was to assess the clinical relevance of plasma H2S levels in patients suffering from vascular disease. Individuals included in this study were diagnosed with either carotid artery stenosis requiring carotid endarterectomy (CEA), or peripheral artery disease (PAD) necessitating revascularization or amputation secondary to unsalvageable critical limb ischemia. Patients were followed for 36 months post-surgery with clinical outcomes and mortality measured. A baseline control cohort of patients matched for age, sex, and hypertension (7), but with no history of PAD, prior MI, coronary interventions, heart failure, or stroke were included for comparing H2S levels in the diseased vs. healthy state. Our study provides new insight into the relevance of circulating H2S levels in patients suffering from atherosclerosis, and during surgical revascularization.
The study followed the principles of the Declaration of Helsinki and was approved by the Partners Human Research Committee institutional review board (IRB). Informed consent was obtained for prospective collection of demographic and clinical data. All consecutive patients, at a single institution undergoing elective vascular surgery from 2012 to 2015, scheduled for carotid endarterectomy, open lower extremity revascularization, and major lower extremity amputation (secondary to critical limb ischemia) were enrolled. None of the amputations were traumatic. Patients were excluded if they were <18 years, had an emergent indication for the operation or if they were involved in another clinical research study. The cohort baseline risk factors and demographics were collected including age, race, history of PAD, history of stroke or myocardial infarction as well as history of coronary intervention (Supplemental Table 1). To compare levels of H2S between patients with vascular disease vs. healthy patients, an additional group of 20 control subjects were enrolled without documented or diagnosed PAD or CAD. This control cohort was matched for age, sex and hypertension, and was randomly extracted from the Brigham and Women's biobank. No additional clinical information was provided for this control cohort.
On the day of surgery, but prior to surgical intervention, peripheral venous blood was collected into ethylenediaminetetraacetic acid (EDTA) collection tubes at the time of peripheral intravenous line placement. The tube was inverted several times to ensure mixing with the anticoagulant and then transferred to the lab for processing. To obtain plasma all samples were centrifuged at room temperature for 15 min at 2,000 g, plasma was then stored at −80°C for future analysis. All samples were then thawed and processed for H2S production capacity at once to ensure uniformity.
Hydrogen sulfide production capacity was measured using the lead acetate method (24). In brief, plasma was mixed with 150 μl freshly prepared reaction mixture, containing 100 mM L-cysteine and 0.5 mM pyridoxal 5'-phosphate (PLP, aka Vitamin B6) in Phosphate buffered saline (PBS) in a plastic 96-well plate. The plate was then incubated at 37°C with lead acetate embedded filter paper on top. Upon the reaction of H2S with the lead acetate paper, a dark lead sulfide precipitate is produced. The paper was incubated for 6 h, until a detectable, but non-saturated signal was seen.
In vitro H2S production assays to demonstrate sensitivity and validity of the lead acetate assay to detect iron-catalyzed H2S production were similarly performed as previously described (25). Briefly, 150 μL reactions in PBS were set up in 96-well plates. Each reaction contained 10 mM L-cysteine, and according to the respective conditions as indicated in the figures, 0–50 μM FeCl3, 0–50 μM hemin, 0–1,000 μM PLP, and/or 0 to 50 mM homocysteine. The lead acetate filter paper was then placed on top of the reaction plates with a weight on top and incubated at 37°C for 1.5–2 h.
The amount of lead sulfide captured on the paper was quantitated by using the IntDen measurement function in ImageJ software and normalized to the respective control group after subtracting the background. An empty well without plasma, was used as blank value was used for background measurement. These measurements were assessed by 2 independent investigators blinded to group assignment.
Hydrogen sulfide concentration determination using the MBB method was conducted as described previously (26). Briefly: In almost complete darkness due to the light sensitivity of the MBB reagent, 20 μl of 50 mM HEPES (pH = 8.0) buffer and 20 μl of 10 mM monobromo-bimane (Sigma Aldrich) was added to 20 μl of plasma sample. After 10 min in the dark at room temperature, the product sulfide-dibimane was extracted with 200 μl of pure ethyl acetate. The organic supernatant was collected and evaporated to dryness and stored at −20°C until measurement. The solid samples were redissolved prior to HPLC measurements in acetonitrile. Ten microliter was injected and separated on an Agilent Eclipse XDB-C18 (4.6 × 250 mm, 5 μm) using a Merck Hitachi L7000 HPLC instrument with a Thermo UltiMate 3000 fluorescent detector. The elution method employed a 28 min long gradient using water and acetonitrile both containing 0.1% TFA. The detection of the product was carried out using UV-absorbance measurement at 254 nm and fluorescent measurement with extinction at 390 nm and detection at 480 nm. Quantitation was done using a standard calibration curve in aqueous buffered solutions, where H2S concentrations were verified by the DTNB assays. It should be noted that the MBB method measures endogenous sulfide levels that are easily liberated from the bound plasma sulfide pool and hence absolute values largely depend on the applied conditions (temperature, alkylation time, concentration conditions etc). This is the reason why absolute sulfide values obtained here should not be compared with values reported in other studies (23).
The enzymatic activity of CBS in blood samples was measured by an HPLC-MS/MS protocol previously published (27). Briefly, 20 μL of plasma sample was added to 25 μL of solution containing 200 mmol/L Tris-HCl (pH 8.6), 1 mmol/L pyridoxal 5′-phosphate, 1 mmol/L SAM and 40 mmol/L 2,3,3-2H-labeled serine (Cambridge Isotope Laboratories, Inc). Five microliter of starting solution containing 280 mmol/L homocysteine thiolactone in 100 mmol/L Tris-HCl (pH 8.6), 10 mmol/L DTT, 1.225 mol/L NaOH was incubated for 5 min at 37°C to produce homocysteine by thiolactone cleavage, pH was adjusted to 8.6 with 1:1 HCl solution and the solution was added to the plasma mixture. After 4 h of incubation at 37°C, the reaction was quenched by acidification of the mixture with 100 μL of EZ:faast Internal Standard Solution (Phenomenex) containing 3.3 μmol/L of internal standard 3,3,4,4-2H-labeled cystathionine. Sample preparation was carried out using EZ:faast kit (Phenomenex) kit, that included a solid phase extraction step, derivatization with propyl chloroformate, and an extraction into an organic solvent. The prepared samples were separated on an EZ:faast AAA-MS column (250 × 2.0 mm, Phenomenex) using LC and MS settings described in the EZ:faast user manual using a Thermo Scientific UltiMate 3000 HPLC connected to a Thermo Scientific LTQ-XL MS instrument. Concentrations (and enzyme activities) were calculated using the internal standard.
Plasma H2S production capacity and sulfide concentration in vascular surgery patients and healthy controls were compared using Student's t-test. Pearson correlation coefficient was calculated to assess the association between plasma H2S production capacity and plasma sulfide concentration. High low H2S production capacity/free sulfide groups and groups were divided by median split, which was arbitrary. The Kaplan-Meier method was used to estimate survival in high and low H2S production capacity/free sulfide groups and groups were compared using log-rank test. Cox proportional hazard models were fit to estimate mortality hazard ratios between high and low H2S producing groups, corrected for potentially confounding variables (age, gender, BMI). Both unadjusted and adjusted Cox proportional hazard models met assumptions of proportional hazard. To assess the odds of post-surgical complication in high vs. low H2S producing individuals, Fisher's exact test was used. Statistical tests were performed using Graphpad Prism 7 and R version 3.3.2. Kaplan-Meier curves and Cox proportional hazard models were fit in R using the Survival package and Kaplan-Meier curves were visualized using the Survminer package. All reported P values are based on 2-sided tests and P values of <0.05 were considered statistically significant.
The characteristics of the control and the vascular cohort are summarized in Table 1. Mean (SD) age of the control and vascular patients was 68 (2.3) and 69 (9), respectively. These patients only differed in the prevalence of PAD, prior MI/coronary revascularization, and hyperlipidemia (Table 1). The control cohort included a group of 20 subjects, matched for age, sex and hypertension, but with no documented atherosclerotic cardiovascular disease. The vascular cohort included a heterogenous group of 115 patients. Forty nine underwent carotid endarterectomy (asymptomatic n = 34, symptomatic n = 15), 44 infra-inguinal revascularization (claudication n = 21, resting pain n = 15, tissue loss n = 13), and 22 amputation.
Analysis of the baseline blood sample (pre-operative) H2S production capacity assay using the lead acetate-based method, showed that controls had significantly higher H2S production capacity compared to vascular disease patients (Figure 1A; Supplementary Figure 1A; 80.8±12.9 vs. 57.0 ± 8.4 arbitrary units, p < 0.001). Importantly, H2S levels was similarly reduced in patient that underwent carotid endarterectomy (CEA, 58.6 ± 5.79), infra-inguinal revascularization (IGR, 57.1 ± 10.1) and amputation (Amp, 53.3 ± 8.97). Results using the MBB method also showed that healthy controls had significantly greater plasma sulfide levels (Figure 1B; 0.95 ± 0.30 vs. 0.58 ± 0.16 μM, p < 0.05), and was consistent across the underlying vascular surgeries (CEA 0.59 ± 0.17, IGR 0.59 ± 0.17, Amp 0.52 ±0.13; Figure 1B). Although both of these sulfide-based measurements were significantly higher in healthy controls, there was no observable correlation between the measurements (Figure 1C; rs = −0.12, p = 0.34), suggesting that the two methods measure fundamentally different phenomena and/or sulfide pools.
Figure 1. Plasma H2S production capacity and sulfide levels are reduced in patients with vascular disease, with production capacity predicting mortality. (A) Plasma H2S production capacity and (B) plasma sulfide measured by the MBB method from human patients suffering vascular occlusive disease including 115 patients undergoing carotid endarterectomy (CEA), infra-inguinal revascularization (IGR) and amputation (Amp), as well as age-matched individuals (n = 20). Error bars indicate SD; different letters indicate difference p < 0.05 in One Way ANOVA Tukey post-hoc test. (C) Correlation between H2S production capacity and sulfide measured by the MBB method in vascular disease patients. (D) Probability of survival for vascular disease patients during follow up after intervention with low (n = 57) vs. high (n = 57) H2S production capacity and (E) low and high plasma sulfide measurements. High vs. low was determined by median split. P value calculated from log-rank test.
To assess the associations of these H2S measurements with clinical outcomes after surgical procedures, the vascular surgery patients were divided by median split into patients with high and low pre-operative H2S production capacity (Supplementary Figure 1B). Over 36 months of follow up, patients with low H2S production capacity had significantly decreased probability of survival compared to patients with high H2S production capacity (Figure 1D; 54 vs. 82% survival probability, log-rank test p = 0.0029). However, when patients were divided by median split into high and low MBB-measured sulfide measurements, there was no significant association with survival and the trend was reversed such that those with higher sulfide tended to have lower probability of survival (Figure 1E; 71 vs. 52% survival probability, log-rank test = 0.15).
To further assess the robustness of H2S production capacity as a predictor of mortality after a vascular surgery intervention, we generated Cox proportional hazard models to adjust for potential confounding variables. A univariate unadjusted model showed that individuals in the high H2S production capacity group had significantly reduced risk of death during follow up (HR(95% CI) = 0.31(0.11–0.87), coefficient p = 0.025, model Wald p = 0.025). In a Cox proportional hazard model adjusted for age, BMI, gender, smoking history, race, procedure type, diabetes, hyperlipidemia, and renal function, H2S production capacity was significantly associated with survival (HR = 0.95, CI = 0.90–1, coefficient p = 0.07, model Wald p < 0.001; Figure 2).
Figure 2. Hazard ratios of death during follow up period. Hazard ratio estimates and 95% confidence intervals for clinically relevant parameters and plasma H2S production capacity as measured by the lead acetate release method.
Finally, to characterize the biochemical nature of the plasma H2S production capacity measurement, we investigated whether it might be generated by enzymatic or non-enzymatic production. It has been proposed that the H2S generating enzyme cystathionine beta synthase (CBS) is present in human plasma. We measured plasma CBS activities, but found no observable association between plasma CBS activity and H2S production capacity (Figure 3A; r = −0.07, p = 0.71). We next performed the assay under protein denaturing conditions by heating and adding DTT, and found a highly correlated pattern of signals compared to when the assay was performed under non-denaturing conditions (Figure 3B; r = 0.34, p = 0.0002). These results suggest a non-enzymatic process for H2S production in the plasma. Finally, we added chelators and found that EDTA was able to almost completely ablate the H2S release, while EGTA was not (Figure 3C). On the other hand, EDTA had a reverse effect on measured sulfide concentrations by the MBB method (23) corroborating that the two methods measure sulfide released from fundamentally different endogenous pools and/or production mechanisms. This suggests a vital role for free and bound iron as a catalyst in H2S release. In combination with PLP and the substrate L-cysteine, H2S release is ultimately dampened by increasing homocysteine concentrations (Figures 3D–F; Supplementary Figures 1C–E). These results on the likely non-enzymatic nature of the signal are in agreement with the recent thorough characterization of mechanisms of H2S release in blood by Hine et al. (25).
Figure 3. Mechanisms of H2S release from plasma. (A) Correlation between plasma cystathionine-beta synthase activity and plasma H2S release. (B) Correlation between plasma H2S release performed under protein non-denaturing (x-axis) and protein denaturing (y-axis) conditions. (C) H2S release from plasma when incubated with PLP, PLP + EDTA or PLP + EGTA. (D) In vitro H2S release performed using cysteine and increasing concentration of FeCl3, (E) PLP or (F) homocysteine (n = 5 reactions per condition). *p < 0.05, ****p < 0.0001 vs. respective 0uM control.
Pre-clinical studies have suggested that decreased levels of H2S accelerate the development of atherosclerosis (14), and are reduced in the skeletal muscle of CLI patients (1). Here we show that patients with vascular disease have significantly decreased circulating H2S production capacity and sulfide concentrations, compared to subjects with no clinical evidence of coronary or peripheral artery disease. Together, these results suggest that patients with atherosclerotic vascular disease have a decreased capability to generate H2S. Furthermore, patients with higher H2S production capacity measured prior to vascular surgery had reduced post-operative mortality at 36 months follow-up compared to those with lower H2S production. It also suggests possible therapeutic application of H2S donors, to restore or increase H2S levels in humans. Indeed, the administration of exogenous H2S (NaHS, SG1002) protected against ischemia-induced heart failure, and improved overall survival in rodents (28, 29). Such an H2S donor is being tested in clinical trials (NCT01989208). Similarly, exogenous administration of H2S in preclinical models protects from ischemic injury to the liver (2), the brain (30), and the kidney (31), which could have therapeutic benefits in the context or solid organ transplantation, myocardial infarction or stroke. Interestingly, some FDA approved drug, with H2S-releasring properties, such as the ACE inhibitor Zofenopril or sodium thiosulfate, improve vascular function, and limit intimal hyperplasia (unpublished data).
Interestingly, this association was not observed with sulfide levels that were measured by the MBB-method. While this suggest that although both measures are related to sulfide release from bound sulfide reserves, they specifically capture distinct biological phenomena with clinical relevance. Hine and colleagues have recently performed a thorough chemical characterization of the mechanisms of non-enzymatic H2S production in blood, demonstrating that it is catalyzed by iron and vitamin B6 (PLP) with cysteine serving as optimum substrate (25). Although this work gives us many clues, important questions remain about what specific components of the plasma determine and regulate H2S production capacity. One theory revolves around the sulfur containing amino acid homocysteine, which in itself has been long associated with cardiovascular disease risk, but its mechanism of pathology is not well-understood (32). As reported by Nakano et al. (33) and measured via the quantitative analysis of reactive sulfur species using MBB-method similar to our present study, homocysteine captures H2S and/or HS− to form a homocysteine persulfide in cardiac tissue, which could potentially interfere with H2S-related cardiovascular protection (33). Thus, if surgical patients with decreased survival showed an increase in plasma homocysteine levels, then this could explain the dichotomy in H2S release in these patients using the two different methods (lead acetate vs. MBB). Furthermore, in the non-enzymatic production of H2S in plasma catalyzed by iron described previously (25) and confirmed here, PLP still acts as an important co-factor by the formation of a Schiff base and subsequent cysteine-aldimine and thiazolidine five-member ring intermediates prior to iron rapidly catalyzing the release of the sulfide. Importantly, homocysteine itself can also form a Schiff base with PLP, except it will result in a more thermodynamically stable six-member tetrahydrothiazine ring (34). The formation of the stable tetrahydrothiazine ring poses two complications for H2S production in circulation: the first being sequestration of valuable PLP co-factor from both enzymatic and non-enzymatic H2S production where cysteine serves as substrate; and the second being slow H2S production kinetics when iron serves as a catalyst (25), which we demonstrate here. Thus, the suppression of H2S release by increased circulating homocysteine may represent a mechanism explaining the robust association between homocysteine levels and cardiovascular disease.
In addition to addressing the issues brought up in the discussion, the results reported here will aid in the development of specific H2S assays for diagnostic purposes. Further, they will guide therapeutic interventions if these specific determinants are shown to be causal to the disease process. Despite these remaining questions, we demonstrate that the lead acetate assay represents a simple, rapid and very low-cost method which appears to capture substantial information on clinical risk in this population.
Limitations of our study include the quantification of H2S using the lead acetate method measures only relative differences in H2S between individuals and groups, and not absolute differences. Likewise, it primarily serves as a surrogate for the actual amount of H2S produced from available substrates in plasma. It is also important to note that mortality outcomes in this study encompassed two uniquely different patient populations, patients with carotid stenosis and patients with PAD necessitating surgical revascularization or amputation. These differences may influence disparities in mortality outcomes and future work will need to validate these findings in larger cohorts with more homogenous interventions. In addition, we previously demonstrated that dietary restriction (2, 35) or the hypothalamic-pituitary axis (36) were important regulators of endogenous H2S production and downstream signaling (37, 38). These factors, including circulating homocysteine levels, were not specifically assessed here, however, they do illuminate the possibilities that patients with increased survival post-surgery having an H2S production promoting diet and/or endocrine makeup in the days/hours leading to surgery. Nevertheless, we highlight our important results showing that both H2S production capacity and MBB-method-measured sulfide levels in vascular patients were significantly reduced compared to healthy subjects, indicating a potential correlation between H2S and the progression of cardiovascular pathology.
This study shows that patients with vascular disease have significantly decreased circulating H2S production capacity and sulfide concentrations, compared to subjects with no clinical evidence of coronary or peripheral artery disease. In addition, the lead acetate H2S detection represents a simple, rapid and low-cost method to capture clinical risk in patients undergoing vascular surgery. Altogether, results provide further insights into the role of H2S biology in surgical patients and open an avenue for the use of H2S for diagnostics and therapeutics in those with dysfunction of their vascular system.
The original contributions presented in the study are included in the article/Supplementary Material, further inquiries can be directed to the corresponding author/s.
The studies involving human participants were reviewed and approved by Partners Human Research Committee institutional review board. The patients/participants provided their written informed consent to participate in this study.
AL, MM, and KT: participated in the conception, design of the work and the acquisition, and analysis and interpretation of data for the work. JG, CM, PK, WK, GS, MT, SM, TD, JY, and PN: participated in the acquisition and analysis and interpretation of data for the work. CO, CH, and JM: participated in the conception, design of the work, and analysis and interpretation of data for the work. All authors contributed to the article and approved the submitted version.
The Swiss National Science Foundation (PZ00P3-185927) to AL, the National Institutes of Health (R01HL148352) to CH, the Hungarian Thematic Excellence Program, the Hungarian National Research, Development and Innovation Office to PN (TKP2020-NKA-26, KH_126766, and K_129286), and the National Institutes of Health (1P01AG055369-01A1) to JM and SM.
The authors declare that the research was conducted in the absence of any commercial or financial relationships that could be construed as a potential conflict of interest.
All claims expressed in this article are solely those of the authors and do not necessarily represent those of their affiliated organizations, or those of the publisher, the editors and the reviewers. Any product that may be evaluated in this article, or claim that may be made by its manufacturer, is not guaranteed or endorsed by the publisher.
The Supplementary Material for this article can be found online at: https://www.frontiersin.org/articles/10.3389/fcvm.2021.750926/full#supplementary-material
1. Islam KN, Polhemus DJ, Donnarumma E, Brewster LP, Lefer DJ. Hydrogen sulfide levels and nuclear factor-erythroid 2-related factor 2 (NRF2) activity are attenuated in the setting of Critical Limb Ischemia (CLI). J Am Heart Assoc. (2015) 4:e001986. doi: 10.1161/JAHA.115.001986
2. Hine C, Harputlugil E, Zhang Y, Ruckenstuhl C, Lee BC, Brace L, et al. Endogenous hydrogen sulfide production is essential for dietary restriction benefits. Cell. (2015) 160:132–44. doi: 10.1016/j.cell.2014.11.048
3. Kip P, Tao M, Trocha KM, MacArthur MR, Peters HAB, Mitchell SJ, et al. Periprocedural hydrogen sulfide therapy improves vascular remodeling and attenuates vein graft disease. J Am Heart Assoc. (2020) 9:e016391. doi: 10.1161/JAHA.120.016391
4. Longchamp A, Mirabella T, Arduini A, MacArthur MR, Das A, Treviño-Villarreal JH, et al. Amino acid restriction triggers angiogenesis via GCN2/ATF4 regulation of VEGF and H(2)S production. Cell. (2018) 173:117–29.e14. doi: 10.1016/j.cell.2018.03.001
5. Blackstone E, Roth MB. Suspended animation-like state protects mice from lethal hypoxia. Shock. (2007) 27:370–2. doi: 10.1097/SHK.0b013e31802e27a0
6. Kanagy NL, Szabo C, Papapetropoulos A. Vascular biology of hydrogen sulfide. Am J Physiol Cell Physiol. (2017) 312:C537–49. doi: 10.1152/ajpcell.00329.2016
7. Yang G, Wu L, Jiang B, Yang W, Qi J, Cao K, et al. H2S as a physiologic vasorelaxant: hypertension in mice with deletion of cystathionine gamma-lyase. Science. (2008) 322:587–90. doi: 10.1126/science.1162667
8. Ahmad A, Olah G, Szczesny B, Wood ME, Whiteman M, Szabo C. AP39, a mitochondrially targeted hydrogen sulfide donor, exerts protective effects in renal epithelial cells subjected to oxidative stress in vitro and in acute renal injury in vivo. Shock. (2016) 45:88–97. doi: 10.1097/SHK.0000000000000478
9. Olson KR, Whitfield NL, Bearden SE, St Leger J, Nilson E, Gao Y, et al. Hypoxic pulmonary vasodilation: a paradigm shift with a hydrogen sulfide mechanism. Am J Physiol Regul Integr Comp Physiol. (2010) 298:R51–60. doi: 10.1152/ajpregu.00576.2009
10. Olson KR, Whitfield NL. Hydrogen sulfide and oxygen sensing in the cardiovascular system. Antioxid Redox Signal. (2010) 12:1219–34. doi: 10.1089/ars.2009.2921
11. Zanardo RC, Brancaleone V, Distrutti E, Fiorucci S, Cirino G, Wallace JL. Hydrogen sulfide is an endogenous modulator of leukocyte-mediated inflammation. FASEB J. (2006) 20:2118–20. doi: 10.1096/fj.06-6270fje
12. Szabo C. Hydrogen sulphide and its therapeutic potential. Nat Rev Drug Discov. (2007) 6:917–35. doi: 10.1038/nrd2425
13. Jain SK, Bull R, Rains JL, Bass PF, Levine SN, Reddy S, et al. Low levels of hydrogen sulfide in the blood of diabetes patients and streptozotocin-treated rats causes vascular inflammation? Antioxid Redox Signal. (2010) 12:1333–7. doi: 10.1089/ars.2009.2956
14. Mani S, Li H, Untereiner A, Wu L, Yang G, Austin RC, et al. Decreased endogenous production of hydrogen sulfide accelerates atherosclerosis. Circulation. (2013) 127:2523–34. doi: 10.1161/CIRCULATIONAHA.113.002208
15. Zhao ZZ, Wang Z, Li GH, Wang R, Tan JM, Cao X, et al. Hydrogen sulfide inhibits macrophage-derived foam cell formation. Exp Biol Med. (2011) 236:169–76. doi: 10.1258/ebm.2010.010308
16. Wang Y, Zhao X, Jin H, Wei H, Li W, Bu D, et al. Role of hydrogen sulfide in the development of atherosclerotic lesions in apolipoprotein E knockout mice. Arterioscler Thromb Vasc Biol. (2009) 29:173–9. doi: 10.1161/ATVBAHA.108.179333
17. Potor L, Nagy P, Méhes G, Hendrik Z, Jeney V, Petho D, et al. Hydrogen sulfide abrogates hemoglobin-lipid interaction in atherosclerotic lesion. Oxidative Med Cell Longevity. (2018) 2018:3812568. doi: 10.1155/2018/3812568
18. Kondo K, Bhushan S, King AL, Prabhu SD, Hamid T, Koenig S, et al. H(2)S protects against pressure overload-induced heart failure via upregulation of endothelial nitric oxide synthase. Circulation. (2013) 127:1116–27. doi: 10.1161/CIRCULATIONAHA.112.000855
19. Wang K, Ahmad S, Cai M, Rennie J, Fujisawa T, Crispi F, et al. Dysregulation of hydrogen sulfide producing enzyme cystathionine gamma-lyase contributes to maternal hypertension and placental abnormalities in preeclampsia. Circulation. (2013) 127:2514–22. doi: 10.1161/CIRCULATIONAHA.113.001631
20. Fiorucci S, Antonelli E, Mencarelli A, Orlandi S, Renga B, Rizzo G, et al. The third gas: H2S regulates perfusion pressure in both the isolated and perfused normal rat liver and in cirrhosis. Hepatology. (2005) 42:539–48. doi: 10.1002/hep.20817
21. Candela J, Wang R, White C. Microvascular endothelial dysfunction in obesity is driven by macrophage-dependent hydrogen sulfide depletion. Arterioscler Thromb Vasc Biol. (2017) 37:889–99. doi: 10.1161/ATVBAHA.117.309138
22. Nagy P, Palinkas Z, Nagy A, Budai B, Toth I, Vasas A. Chemical aspects of hydrogen sulfide measurements in physiological samples. Biochim Biophys Acta. (2014) 1840:876–91. doi: 10.1016/j.bbagen.2013.05.037
23. Ditrói T, Nagy A, Martinelli D, Rosta A, KoŽich V, Nagy P. Comprehensive analysis of how experimental parameters affect H(2)S measurements by the monobromobimane method. Free Radical Biol Med. (2019) 136:146–58. doi: 10.1016/j.freeradbiomed.2019.04.006
24. Hine C, Mitchell JR. Endpoint or kinetic measurement of hydrogen sulfide production capacity in tissue extracts. Bio Protoc. (2017) 7:e2382. doi: 10.21769/BioProtoc.2382
25. Yang J, Minkler P, Grove D, Wang R, Willard B, Dweik R, et al. Non-enzymatic hydrogen sulfide production from cysteine in blood is catalyzed by iron and vitamin B6. Commun Biol. (2019) 2:194. doi: 10.1038/s42003-019-0431-5
26. KoŽich V, Ditrói T, Sokolová J, KríŽková M, Krijt J, Ješina P, et al. Metabolism of sulfur compounds in homocystinurias. Br J Pharmacol. (2019) 176:594–606. doi: 10.1111/bph.14523
27. Krijt J, Kopecká J, Hnízda A, Moat S, Kluijtmans LA, Mayne P, et al. Determination of cystathionine beta-synthase activity in human plasma by LC-MS/MS: potential use in diagnosis of CBS deficiency. J Inherited Metabolic Dis. (2011) 34:49–55. doi: 10.1007/s10545-010-9178-3
28. Polhemus DJ, Kondo K, Bhushan S, Bir SC, Kevil CG, Murohara T, et al. Hydrogen sulfide attenuates cardiac dysfunction after heart failure via induction of angiogenesis. Circ Heart Fail. (2013) 6:1077–86. doi: 10.1161/CIRCHEARTFAILURE.113.000299
29. Calvert JW, Elston M, Nicholson CK, Gundewar S, Jha S, Elrod JW, et al. Genetic and pharmacologic hydrogen sulfide therapy attenuates ischemia-induced heart failure in mice. Circulation. (2010) 122:11–9. doi: 10.1161/CIRCULATIONAHA.109.920991
30. Wei X, Zhang B, Cheng L, Chi M, Deng L, Pan H, et al. Hydrogen sulfide induces neuroprotection against experimental stroke in rats by down-regulation of AQP4 via activating PKC. Brain Res. (2015) 1622:292–9. doi: 10.1016/j.brainres.2015.07.001
31. Han SJ, Kim JI, Park JW, Park KM. Hydrogen sulfide accelerates the recovery of kidney tubules after renal ischemia/reperfusion injury. Nephrol Dial Transplant. (2015) 30:1497–506. doi: 10.1093/ndt/gfv226
32. Beard RS Jr, Bearden SE. Vascular complications of cystathionine beta-synthase deficiency: future directions for homocysteine-to-hydrogen sulfide research. Am J Physiol Heart Circ Physiol. (2011) 300:H13–26. doi: 10.1152/ajpheart.00598.2010
33. Nakano S, Ishii I, Shinmura K, Tamaki K, Hishiki T, Akahoshi N, et al. Hyperhomocysteinemia abrogates fasting-induced cardioprotection against ischemia/reperfusion by limiting bioavailability of hydrogen sulfide anions. J Mol Med. (2015) 93:879–89. doi: 10.1007/s00109-015-1271-5
34. Glowacki R, Stachniuk J, Borowczyk K, Jakubowski H. Quantification of homocysteine and cysteine by derivatization with pyridoxal 5'-phosphate and hydrophilic interaction liquid chromatography. Anal Bioanal Chem. (2016) 408:1935–41. doi: 10.1007/s00216-016-9308-3
35. Mitchell SJ, Madrigal-Matute J, Scheibye-Knudsen M, Fang E, Aon M, Gonzalez-Reyes JA, et al. Effects of sex, strain, and energy intake on hallmarks of aging in mice. Cell Metab. (2016) 23:1093–112. doi: 10.1016/j.cmet.2016.05.027
36. Hine C, Kim HJ, Zhu Y, Harputlugil E, Longchamp A, Matos MS, et al. Hypothalamic-pituitary axis regulates hydrogen sulfide production. Cell Metab. (2017) 25:1320–33.e5. doi: 10.1016/j.cmet.2017.05.003
37. Bithi N, Link C, Henderson YO, Kim S, Yang J, Li L, et al. Dietary restriction transforms the mammalian protein persulfidome in a tissue-specific and cystathionine gamma-lyase-dependent manner. Nat Commun. (2021) 12:1745. doi: 10.1038/s41467-021-22001-w
Keywords: hydrogen sulfide, peripheral artery disease (PAD), limb ischemia, biomarker, surgery
Citation: Longchamp A, MacArthur MR, Trocha K, Ganahl J, Mann CG, Kip P, King WW, Sharma G, Tao M, Mitchell SJ, Ditrói T, Yang J, Nagy P, Ozaki CK, Hine C and Mitchell JR (2021) Plasma Hydrogen Sulfide Is Positively Associated With Post-operative Survival in Patients Undergoing Surgical Revascularization. Front. Cardiovasc. Med. 8:750926. doi: 10.3389/fcvm.2021.750926
Received: 31 July 2021; Accepted: 13 September 2021;
Published: 25 October 2021.
Edited by:
Yuli Huang, Shunde Hospital, Southern Medical University, ChinaReviewed by:
Nazareno Paolocci, Johns Hopkins University, United StatesCopyright © 2021 Longchamp, MacArthur, Trocha, Ganahl, Mann, Kip, King, Sharma, Tao, Mitchell, Ditrói, Yang, Nagy, Ozaki, Hine and Mitchell. This is an open-access article distributed under the terms of the Creative Commons Attribution License (CC BY). The use, distribution or reproduction in other forums is permitted, provided the original author(s) and the copyright owner(s) are credited and that the original publication in this journal is cited, in accordance with accepted academic practice. No use, distribution or reproduction is permitted which does not comply with these terms.
*Correspondence: Alban Longchamp, YWxiYW4ubG9uZ2NoYW1wQGNodXYuY2g=
†These authors have contributed equally to this work
Disclaimer: All claims expressed in this article are solely those of the authors and do not necessarily represent those of their affiliated organizations, or those of the publisher, the editors and the reviewers. Any product that may be evaluated in this article or claim that may be made by its manufacturer is not guaranteed or endorsed by the publisher.
Research integrity at Frontiers
Learn more about the work of our research integrity team to safeguard the quality of each article we publish.