- 1Department of Biomedical Sciences for Health, University of Milano, Milan, Italy
- 2Cardiology University Department, IRCCS Policlinico San Donato, Milan, Italy
- 3Department of Cardiovascular Diseases, University of Siena, Siena, Italy
- 4Department of Biological Sciences, University of Milano, Milan, Italy
- 5Cardiology Division, San Paolo Hospital, Milan, Italy
The left atrium (LA) is emerging as a key element in the pathophysiology of several cardiac diseases due to having an active role in contrasting heart failure (HF) progression. Its morphological and functional remodeling occurs progressively according to pressure or volume overload generated by the underlying disease, and its ability of adaptation contributes to avoid pulmonary circulation congestion and to postpone HF symptoms. Moreover, early signs of LA dysfunction can anticipate and predict the clinical course of HF diseases before the symptom onset which, particularly, also applies to patients with increased risk of HF with still normal cardiac structure (stage A HF). The study of LA mechanics (chamber morphology and function) is moving from a research interest to a clinical application thanks to a great clinical, prognostic, and pathophysiological significance. This process is promoted by the technological progress of cardiac imaging which increases the availability of easy-to-use tools for clinicians and HF specialists. Two-dimensional (2D) speckle tracking echocardiography and feature tracking cardiac magnetic resonance are becoming essential for daily practice. In this context, a deep understanding of LA mechanics, its prognostic significance, and the available approaches are essential to improve clinical practice. The present review will focus on LA mechanics, discussing atrial physiology and pathophysiology of main cardiac diseases across the HF stages with specific attention to the prognostic significance. Imaging techniques for LA mechanics assessment will be discussed with an overlook on the dynamic (under stress) evaluation of the chamber.
Introduction
The notion of the left atrium (LA) in heart failure (HF) pathophysiology has progressively moved from a by-stander chamber to a central and active element for cardiovascular balance (1, 2). The anatomical, mechanical, hemodynamical, electrical, and rheological roles of LA have been recognized and understood, especially in overt HF clinical syndrome (3, 4). The increasing availability of non-invasive approaches for LA mechanics (structural and functional properties) assessment has progressively moved the study of atrial chamber from a research interest to a clinical tool and necessity (5).
Heart failure (HF) syndrome starts with the presence of predisposing factors (stage A) and progresses with overt structural heart diseases (stage B) to a wide spectrum of clinical phenotypes (stages C and D) (6). The clinical manifestation may occur at different times of heart structural and functional changes. LA plays a major role in the physiological coupling of left ventricle (LV) filling pressures and pulmonary circulation hemodynamic (7, 8). The adaptive remodeling, aimed at contrasting volume or pressure overload and maintaining an adequate LV filling, is time and size limited. The exhaustion of compensatory mechanisms translates into overt HF or into a worsening of clinical conditions.
Cardiovascular imaging is further evolving from a morphology-based tool to a unique in vivo opportunity to address heart function and structure (9, 10). The understanding of LA mechanics changed with the introduction of myocardial deformation analysis and its multimodality use during stress conditions (5). The use of pharmacological or physical stressors, in order to challenge the presence of a specific ischemic, contractile, flow or diastolic “reserve” has become a standard approach with diagnostic and prognostic importance (11). The study of LA mechanics is developing in the same direction and moving toward a dynamic assessment (at rest and under stress in specific exercise) for early diagnosis and prognostic stratification of patients with HF.
The aim of the present review is to discuss the non-invasive evaluation of LA mechanics and its pathophysiological and prognostic significance across the spectrum of HF stages (including stages A and B, with the most clinically relevant structural heart diseases) with a specific focus on the available methods and the dynamic assessment. The contents will be presented according with the natural history of disease progression, starting with the conditions at risk (stage A) and proceeding with the cardiac structural diseases (stage B) and overt HF (stage C).
LA Physiology
The LA is the inflow chamber of left-side heart and is responsible for blood accommodation from the pulmonary circulation and for diastolic LV filling (12). The peculiar attachment of pulmonary veins facilitates the blood flow during ventricular systole and isovolumetric relaxation (the so-called reservoir function) which are responsible for about 40–50% of stroke volume (SV) (13). The conduit function corresponds to the early LV diastole, or when blood flows directly from pulmonary veins to LV throughout the LA, and it accounts for the 20–30% of SV. Late diastole is characterized by active LA contraction that provides final diastolic LV filling (about 20–30% of SV). The optimal chamber function requires electromechanical synchronization, and it is strictly influenced by LV mechanical properties, transmitral gradients, and LA chamber compliance.
During exercise, the heart pumps more forcefully to generate adequate cardiac output (CO) as required by peripheral demands. At low level exercise, CO rises thanks to the SV and heart rate increase, while at heavier workload, SV maintains a plateau with chronotropic response becoming predominant (14). LA guarantees an adequate blood flow to LV during the progressive shortening of diastolic period. In normal subjects, LA volume lowers, and ejection fraction increases at an earlier stage, assuring about the 40% of flow increase with the enhancement of conduit function (15). Then, conduit and contractile phases are overlapped due to a further shortening of diastolic period, therefore, the LV filling is maintained by an additional increase of LV suction capacity during the reservoir phase.
The interplay between the active ventricular relaxation and a coherent LA response is crucial to provide adequate LV filling. Therefore, CO increase and when one or both of them fails to adapt during exertion, the unbalance causes an increase in LV filling and LA pressures, affecting the upstream pulmonary circulation (16, 17). In early pathological stages, e.g., in HF, the abnormal hemodynamic behavior can arise only during exertion, producing the typical effort-related dyspnea. In the advanced phase of the disease, the LA remodeling ends up with different degrees of enlargement, loss of function, and increase of stiffness generally associated with chronic and severe symptoms (18–20).
From the hemodynamic point of view, the pressure-volume (PV) loops provide a unique description of the complex physiological function, unfortunately limited by a low feasibility in clinical practice. The “eight-shaped” loop develops across the three steps of cardiac cycle, defining the active work of the chamber (left component of the loop). In HF syndrome, the PV loop shifts upward and rightward according to the chamber compliance to the pressure and volume overload (Figure 1). The use of myocardial deformation to study LA chamber represents a non-invasive technique, alternative to cardiac catheterization, and may be able to provide specific insights on chamber physiology both in control conditions and during stress conditions (physical or pharmacological).
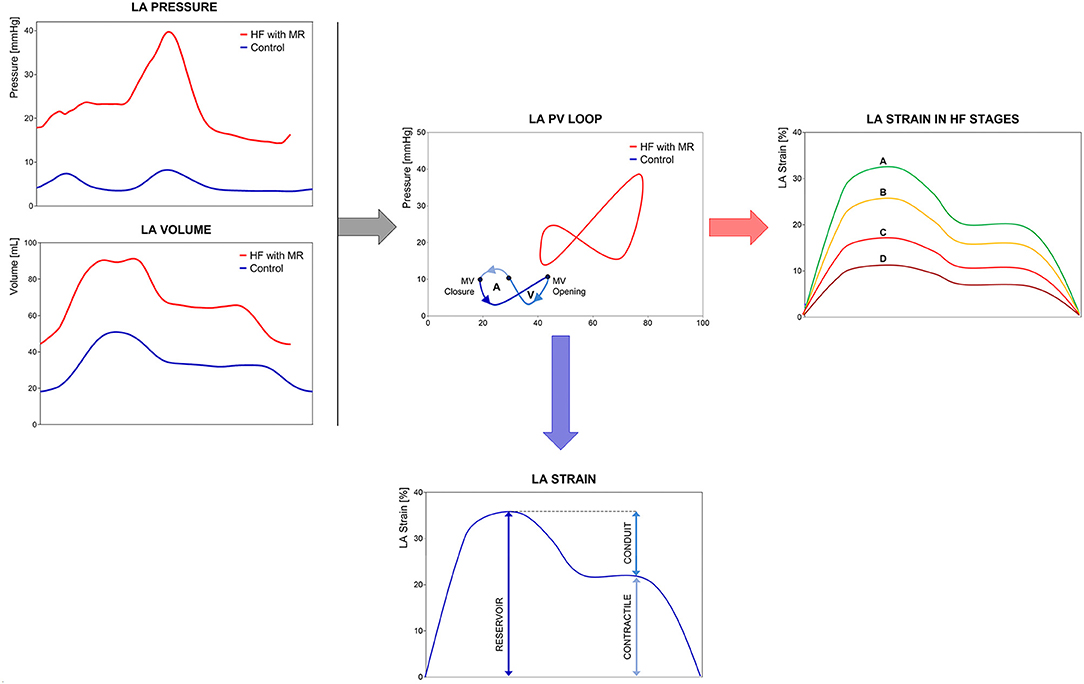
Figure 1. Left Atrium (LA) mechanics and physiology. Comparison of LA mechanics and physiology in a control subject vs. a heart failure with reduced ejection fraction (HFrEF) patient: (left) real LA pressure and volume traces in a control subject and HFrEF patient with severe mitral regurgitation; (center) LA Pressure-Volume loops in the same subjects; (right) representation of progressive LA longitudinal strain decline in the HF stages; (bottom) average LA longitudinal strain with three phasic components. LA pressure-volume (PV) loops show the right- and upward shifting of the loop of HF patient respect to the control subject, with loss of active atrial contraction and MR-related pressure increase. LA longitudinal strain allows the study of chamber phasic function providing physiopathological insights consistent with LA PV loop analysis. Abbreviations as in the text.
Cardiovascular Imaging to Evaluate LA Mechanics
Transthoracic echocardiography (TTE) and cardiovascular magnetic resonance (CMR) are commonly used in clinical practice for the assessment of all cardiac chambers, including LA. Both techniques can be used at rest or under stress conditions. Two- and three-dimensional (2D and 3D) TTEs have the advantage to be widely available, feasible, and time-effective, while CMR is the reference approach for cardiac volumes quantification and tissue characterization (Figure 2).
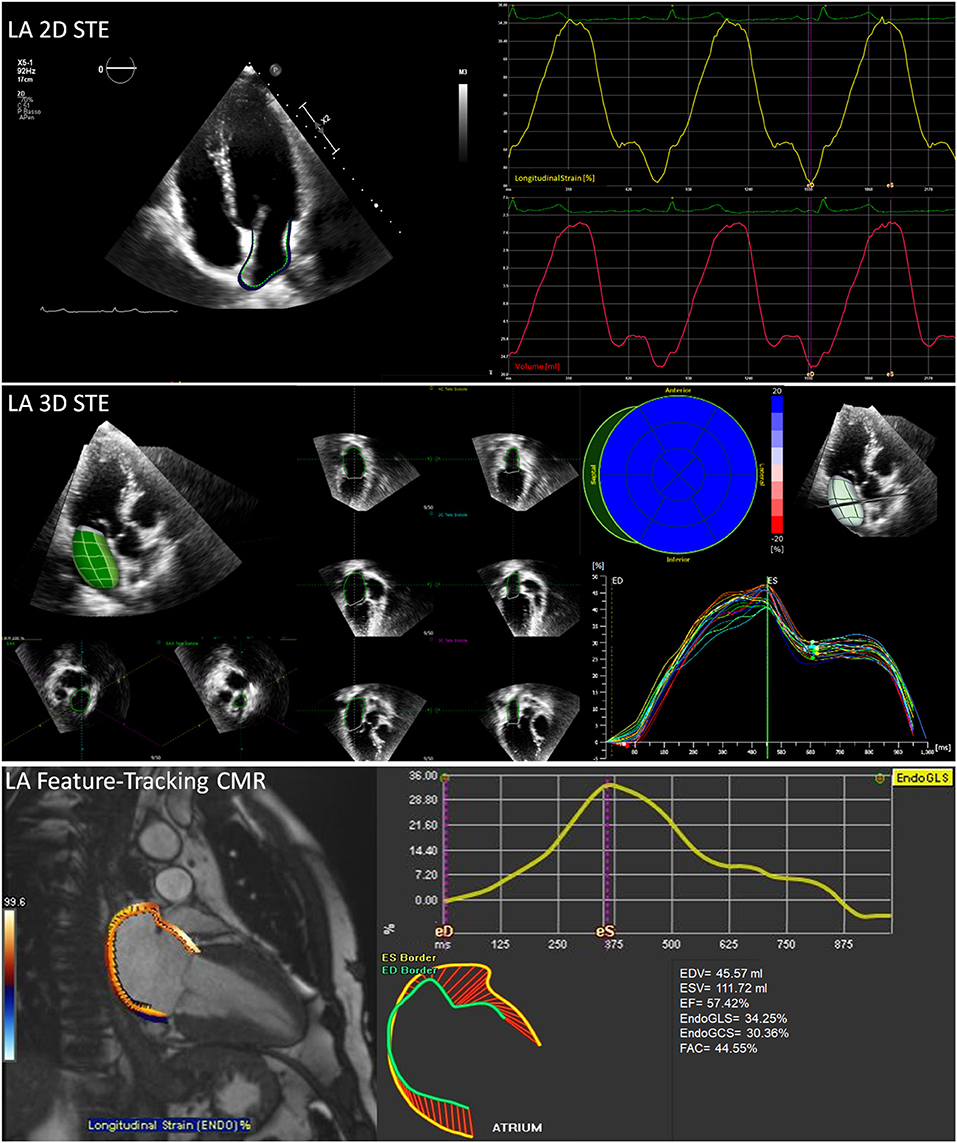
Figure 2. Non-invasive assessment of LA mechanics. Some elaboration and output examples of the mostly used imaging techniques are reported: (top) 2D speckle tracking echocardiography with atrial border contouring (top left), average longitudinal endocardial strain and volume changes (top right); (middle) 3D speckle tracking echocardiography with 3D LA volume reconstruction (middle left) and multi-segment longitudinal endocardial strain; (bottom) feature-tracking cardiovascular magnetic resonance (CMR) with atrial border contouring (left) and average longitudinal endocardial strain (bottom right). Abbreviations as in the text.
Parameters Describing LA Function
Myocardial deformation analysis has been applied in several clinical conditions. Table 1 provides an overview of the most relevant parameters describing LA mechanics with reference values and lower limits of normality (LLN) related to the imaging technique and with specific comments. Tables 2, 3 show the cut-off values of parameters with prognostic significance in patients at risk of HF (stage A) or with cardiac structural abnormalities (stage B) and in subjects with overt HF (stage C), respectively. Current recommendations (56) support the use of longitudinal strain due to the large amount of available literature and to the limited reliability of radial and circumferential deformation related to the low thickness of LA wall. Moreover, a global rather than regional analysis is recommended to overcome geometrical and anatomical limitations. LA function changes with the age being a dynamic instead of a static condition. Normal references are therefore presented according with age distribution when available. Figure 1 represents LA pressure, volume, and strain waveform in healthy subject and HF patient.
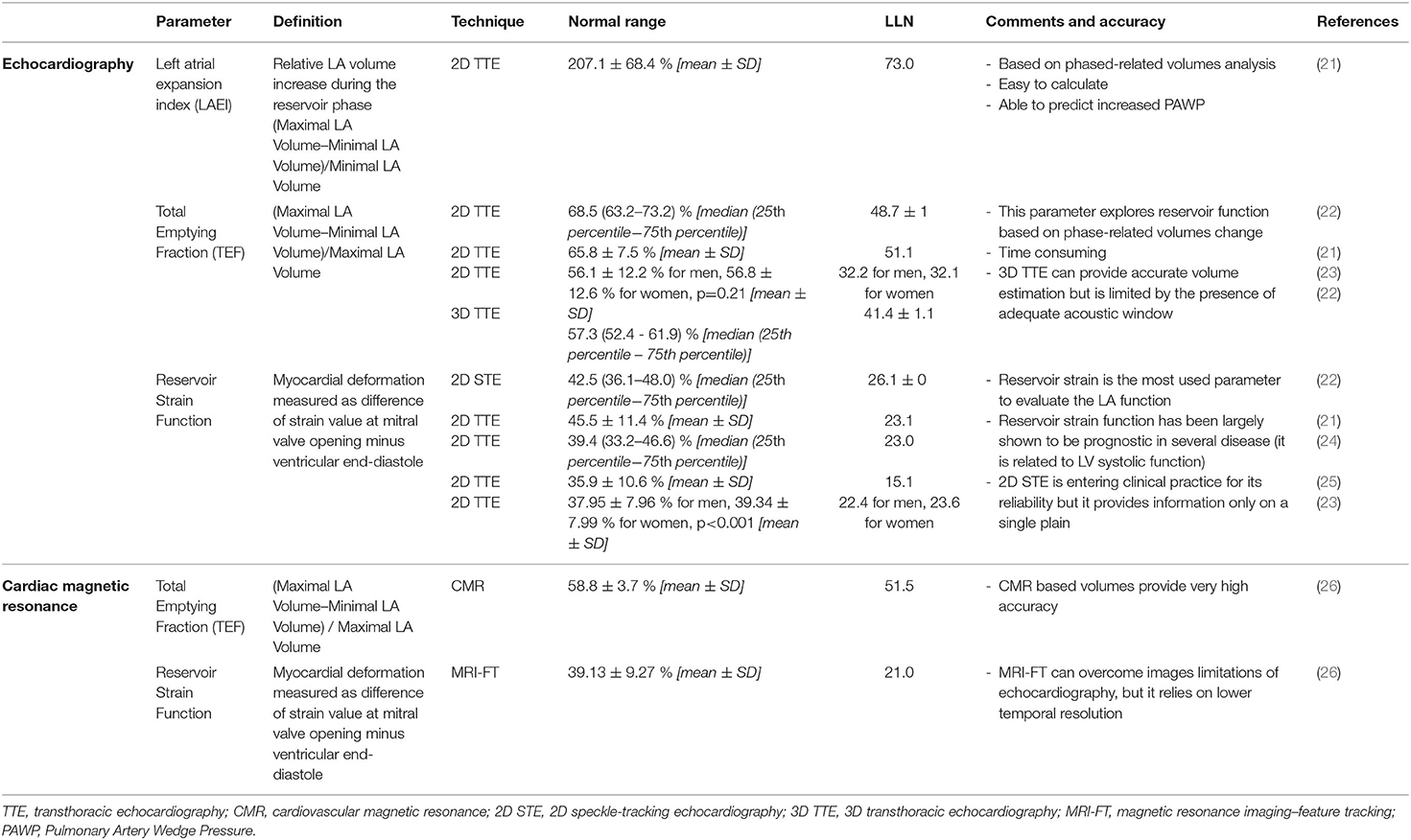
Table 1. Most relevant parameters describing left atrium (LA) mechanics with reference values and lower limits of normality (LLN).
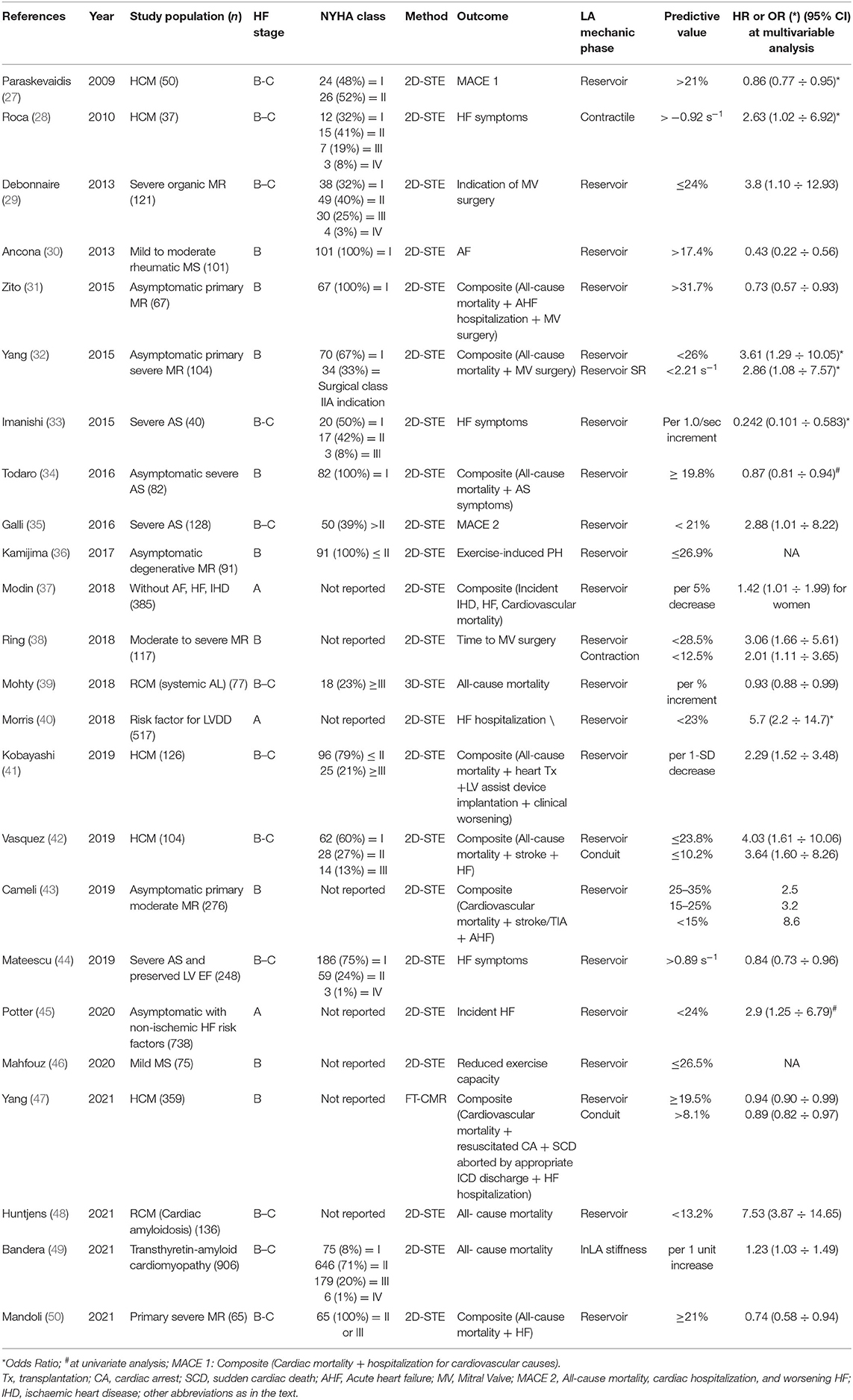
Table 2. Summary of studies showing the prognostic value of LA mechanics in patient at risk of heart failure (HF; stage A) or with cardiac structural abnormalities (stage B) with respect to hard and soft outcomes.
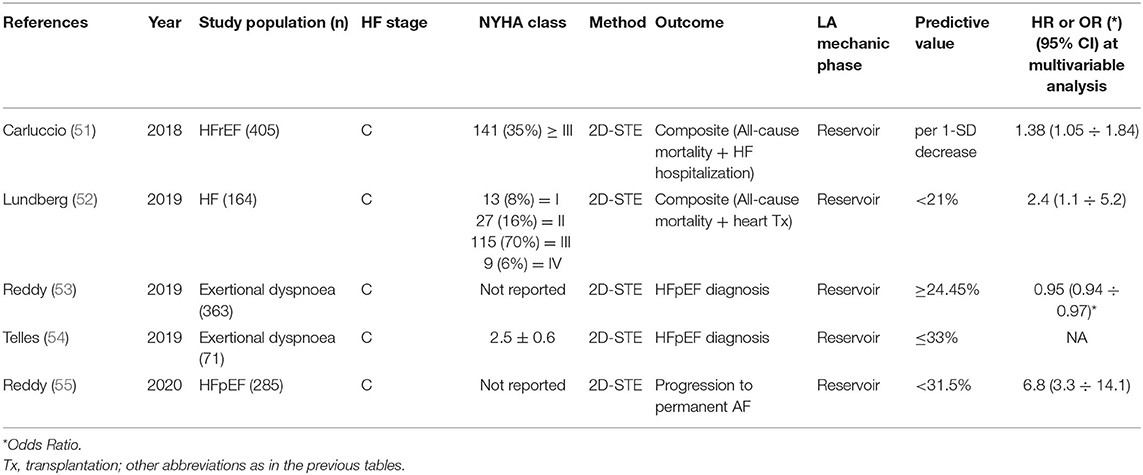
Table 3. Summary of studies showing the prognostic value of LA mechanics in HF (stage C) with respect to hard and soft outcomes.
Echocardiography
The quantification of LA size has largely evolved from parasternal long axis diameter (57) to apical 4-chamber area, estimated LA volumes (58), emptying fractions (related to reservoir, conduit and contraction phases) (21, 59, 60), and 3D real-time volume (61). Notably, the usage of 2D conventional 4- and 2-chamber views (i.e., left ventricular focused) for the computation of LA size is a common source of volume underestimation, since LV and LA axes do not lie in the same plane. Hence, dedicated apical LA views should be exploited (58). The algorithm used for volume calculation represents another source of potential bias, having been shown that the area-length method provides larger volumes than Simpson disk summation (62). Nevertheless, the LA expansion index, derived by volumes estimated using Simpson disk summation, has been recently shown to predict the presence of increased pulmonary wedge pressure. In a large cohort of patients, the index has been validated with invasive right heart catheterization, showing an accuracy higher than standard echocardiographic indices (63).
The static volume has been enriched by functional assessment based on phasic changes of volume (64). However, despite the prognostic significance (65) and greater reliability of 3D vs. 2D assessment, this approach is not commonly used in clinical practice. The study of myocardial deformation, first with tissue Doppler imaging (5, 66), then with 2D speckle-tracking echocardiography (2DSTE) (23–25), has become the most widely used approach, also showing high intra and inter-individual reproducibility (67). Extensive recommendations for myocardial deformation imaging have been endorsed by the European Association of Cardiovascular Imaging (EACVI)/American Society of Echocardiography (ASE)/Industry Task Force (56) covering specific indications to standardize LA assessment. Briefly, using a non-foreshortened apical 4-chamber view with temporal resolution of at least >50 Hz, LA endocardial contour should be manually drawn (<3 mm of thickness). End-diastole, corresponding to R wave at ECG trace, is commonly used as reference since it has shown to provide a slightly higher feasibility and a lower wasting time compared to methods using atrial contraction as reference (68).
A recent meta-analysis (69) systematically reviewed 10 studies that computed pre-interventional 2DSTE LA strain capacity to predict AF recurrence in patients that underwent catheter ablation. A subgroup analysis was performed comparing studies that exploited GE EchoPac® and those that used TomTec® (vendor-independent software) showing that the mean strain values differed significantly between the two subgroups, both in patients with and without AF recurrence and those without. Moreover, the cut-off value predicting AF recurrences and assessed through GE Echopac (18.1%) was substantially different from the one calculated for all the studies (21.9%). A similar difference has been reported in the EACVI Normal Reference Ranges for Echocardiography (NORRE) study where the 3D LA volume and the LA strain rate significantly differed according to the different kind of vendor used. Therefore, intervendor variability should be considered in clinical setting and in the design of single and multicenter trials. Of note, all the reviewed studies were performed prior to the release of the EACVI/ASE/Industry Task Force consensus document.
Normality ranges for 2DSTE-derived LA strain have been established on a cohort of 371 healthy subjects (22) enrolled in the EACVI NORRE study and evaluated using a vendor-independent software (2D Cardiac Performance Analysis, TomTec Imaging System®, Munich, Germany). Multivariable analysis showed that only age is independently associated with all the LA strain components, while no differences in gender were reported. In addition, LA reservoir and conduit strain progressively decrease with age, while contractile function slightly increases (Table 1 and Supplementary Table 1).
Remarkably, 2DSTE analysis is intrinsically limited by the ability of exploring only a static bi-dimensional plan where a very mobile LA endocardium moves throughout at every beat. 3D echocardiography (3DE) is therefore emerging as an alternative approach to capture the overall LA volume and the complex motion along the three dimensions. Starting from a single LA dataset, 3DE allows the assessment of LA volumes at multiple time points during the cardiac cycle. Data from several studies (70–72) demonstrated that 3DE-derived LA volumes are more accurate and reproducible than those calculated by 2D echocardiography if compared to CMR. Only a few published studies have reported reference values of 3DE-derived LA volume. Reference values have been proposed based on a LA-dedicated software used on a cohort of 276 healthy volunteers (61) and were reported to be significantly larger (normality range 18–43 ml/m2) than those obtained through 2D Simpson's method, in agreement with previous reports (73). Despite the need of excellent image quality and the dependence on lower temporal resolution (frame rate > 20 volumes per second), 3DE outperforms 2D echo in the assessment of LA volume and, when available, is recommended in routine clinical practice (70, 72).
Left atrium (LA) mechanics can be studied with 3D speckle-tracking (3DSTE) algorithms implemented in commercial software. These tools compute LA strain along three spatial dimensions (longitudinal, circumferential, and radial), thus leading to deformation values that are truly able to assess the complex 3D motion of the chamber. Different studies (74–76) reported 3DSTE as a faster and more reproducible method than 2DSTE for the measurement of LA strain. The ability of 3DSTE to identify LA functional impairments has been shown in patients affected by type 1 diabetes mellitus (77), hypertrophic cardiomyopathy (78), hypertension (79), amyloidosis (39), and inappropriate sinus tachycardia (80). Few studies addressed the normal values of 3DSTE-derived LA longitudinal strain on healthy people reporting lower values when compared with 2DSTE (75, 76).
A specific strength of echocardiography is represented by its application during physical exercise. If the patient is capable of exercise, stress echocardiography can be performed with a treadmill or a cycle ergometer (upright or supine). With treadmill, the Bruce protocol is followed and images are acquired at rest, immediately after peak exercise, and at recovery (81). Using tiltable ergometer, images are continuously acquired at baseline, at each 25 W step, at peak stress, and during recovery (82). To be successful, bicycle stress tests need the cooperation of the patient and the perfect coordination of the clinicians. In most of the cases, test interpretation is then performed through the comparison of resting and peak images (83).
In order to specifically assess the LA function during stress test, images should be acquired with dedicated 4- and 2-chamber views at rest and under exercise, optimizing the sector width and depth. At baseline, the frame rate should be at least 60–70 per second, while 80–90 per second during exercise, compensating for the heart rate increase. Septal e', lateral e', and E wave of mitral inflow should be measured to allow the atrial stiffness estimation according to the formula E/e'/LA reservoir function. Evaluating the atrial function at rest and during exercise with this method is considered reliable and efficient to detect changes in atrial stiffness (84). Specific attention should be paid to E and A waves fusion occurring at HR >100–110 beats per minute. The current European consensus for the diagnostic workup of heart failure with preserved ejection fraction (HFpEF) indicates the use of exercise echocardiography when the diagnostic score is not conclusive. The recommendation consists in measuring E/e' at earlier stages of exercise (when E and A are still separated) or during recovery when E and A are no longer fused (85).
Cardiovascular Magnetic Resonance
Cardiovascular magnetic resonance (CMR) is characterized by a high reproducibility and good non-isotropic spatial resolution (in clinical practice slices have 8 mm of thickness with in-plane resolution of 1.5–2.5 mm). Steady State Free Precession (SSFP) provides a greater endocardial signal compared to echocardiography due to the excellent blood-endocardium contrast. Nevertheless, the echocardiographic in-plane resolution can be greater according with the used ultrasound frequency (86). Acquiring unseparated slices encompassing the entire LA during the whole cardiac cycle allows the measurement of LA SV, ejection fraction, and volumes during the whole cardiac cycle, providing phase-related emptying fractions (as described for echocardiography). This approach has the unique strength of measuring real volumes with the highest accuracy, also in very remodeled chambers. However, since Simpson's method is time consuming, the biplane area-length method, which is based on the manual tracing of the LA walls in cine-sequences of 4- and 2-chamber views, it is more frequently used despite a possible underestimation related to non-LA dedicated slices (87).
A unique feature of CMR is the identification of scar with gadolinium and its use is a routine practice in LV evaluation, while the thinness of LA wall does not allow a common and wide application. Nevertheless, the Delayed-Enhancement MRI Determinant of Successful Radiofrequency Catheter Ablation of Atrial Fibrillation (DECAAF) study, a multicenter study conducted at 15 different clinical centers (88), showed the feasibility of LA assessment, reporting that the presence of atrial scar was associated with arrhythmia recurrence in patients who underwent catheter ablation (89). Moreover, since LA fibrosis is already present in the early stages of AF, late gadolinium enhancement (LGE) imaging has been proposed to discriminate patients at risk for AF (90).
Magnetic Resonance Imaging Feature Tracking (MRI-FT) is a technique which is similar to the echocardiographic speckle tracking. Regional longitudinal strain (LS) and radial motion fraction indices are measured along the atrial wall providing a quantification of standard phasic strain (91, 92). The normality range for MRI-FT-derived LA strain values have been reported on a cohort of 112 healthy volunteers (26). Data were analyzed through a commercial software (Circle Cardiovascular Imaging®, Calgary, Canada), and optimal intra-observer and interobserver reproducibility for all strain values was described. LA contractile strain increased significantly with age (p < 0.001 for all) and the LA conduit function gradually decreased (p = 0.02), while LA reservoir function does not vary significantly with age (p = 0.19). Additionally, no differences between gender were reported. Further investigations on larger cohorts of patients with different vendor softwares are still required to obtain the normal MRI-FT derived LA strain values.
Recommendations of Guidelines
Current European and American guidelines about patients at risk of HF, subjects with cardiac structural abnormalities, or with overt HF are still based on the evaluation of standard LA parameters, mainly focused on the size of the chamber (93–103). Nevertheless, several consensuses pointed out the clinical and prognostic relevance of LA mechanics assessment (2, 85, 104–108). They also specified the need of additional standardization of parameters analysis and interpretation, and of wider prospective studies to define a specific role in clinical diagnostic work up.
The assessment of LA myocardial deformation has been recognized as a promising tool to evaluate LV diastolic dysfunction, especially in those patients with inconclusive classification based on current algorithm (107). Moreover, the consensus statement on HFpEF diagnostic workup indicates the LA mechanics as new promising markers requiring additional investigation (85). The additional value of LA function has been acknowledged in hypertrophic cardiomyopathy and HF regardless the LV systolic function, especially to investigate the burden of LA pressure overload (104–106). Moreover, the central role of LA has been fully defined in a consensus on atrial cardiomyopathies (i.e., any complex of structural, architectural, contractile, or electrophysiological changes affecting the atria with the potential to produce clinically-relevant manifestations) stressing the usefulness of myocardial deformation to assess atrial physiology and arrhythmic burden (2). Recently, a large multicenter study and an expert consensus of the EACVI on multimodality imaging in HFpEF highlighted the clinical significance of LA reservoir strain in detecting elevated LV filling pressures (108, 109). Of note, the expert consensus of the EACVI highlighted that the main usefulness of LA reservoir strain in the diagnosis of HFpEF or in the evaluation of LV filling pressures is in the setting of indeterminate echocardiographic findings (108). Moreover, the expert consensus of the EACVI remarked that the usefulness of LA reservoir strain is limited in the diagnosis of HFpEF or in the evaluation of LV filling pressures in patients with AF or with history of recent AF (108).
LA Mechanics in HF Stages
Stage A
Stage A is defined by any condition increasing the risk for HF but without structural heart disease or symptoms (110). All cardiovascular risk factors, including chronic kidney disease (CKD), may be considered as stage A HF, requiring specific therapeutic interventions to prevent the transition to stage B and C.
Systemic hypertension and diabetes have been shown to be associated with early reduction of all LA myocardial deformation components in subjects with normal LA dimensions (111). In hypertensive patients, the impairment of reservoir, conduit, and contractile function has been related to LV global longitudinal strain (GLS) and contractile reserve explored by dobutamine stress test and confirming the interplay between LA and LV function (112). However, the relationship between LA and LV function is stronger when atrial chamber is not dilated and seems to be less relevant when LA dilatation occurs (113, 114). A direct atrial damage, such as in diabetic myopathy, may be responsible of further chamber enlargement and function impairment independently from the degree of diastolic dysfunction (115).
Several studies showed the prognostic significance of LA mechanics in stage A HF (37, 40, 45, 116). Reservoir function has been shown to predict a composite cardiovascular end-point in a mixed population with cardiovascular risk factors and a low percentage of previous myocardial infarct and HF (116). More recently, Morris et al. investigated the additive value of LA strain analysis compared to chamber enlargement in a large population of subjects with CV risk factors. LA strain abnormalities resulted to be more prevalent than dilatation, better correlated with LV diastolic dysfunction, and independently associated with the risk of HF hospitalization during 2-years follow-up (40). The prognostic value of reservoir function was therefore confirmed in the general population and in the elderly subjects. A sub-study of Copenhagen City Heart Study considering 385 subjects without a history of cardiovascular disease showed that LA reservoir function predicted cardiovascular morbidity and mortality at the univariable analysis. However, the prognostic value was modified by sex, resulting in an independent predictor only in the female population (37). The use of reservoir to classify diastolic dysfunction in a large cohort of elderly people allowed a significant reduction of indeterminate cases, resulting in independently associated with the incidence of HF (45). In CKD where the activation of renin-angiotensin-aldosterone pathway may lead to early cardiac fibrotic remodeling, LA reservoir and enlargement emerged as early markers of cardiac involvement (117). Moreover, the reservoir function emerged as the only independent predictor of cardiovascular death and major adverse cardiovascular events in stage 3–4 CKD with higher predictive ability compared to other clinical risk scores, LV, and LA parameters (118).
Stage A HF is characterized by early cardiac remodeling, involving LV and LA. LA mechanics reflect the degree of functional and morphological chamber adaptation, providing prognostic information of additional value with respect to standard parameters.
Stages B and C
Stage B and C HF have in common the element of structural heart disease while they differ for the presence of prior or current symptoms. Valvular disease is typically considered as an example of stage B due to the intrinsic high risk of HF associated with an un-prompt management. We therefore reviewed the most common diseases affecting mitral and aortic valve, along with the cardiomyopathies predisposing to stage C HF.
Moreover, we reviewed stage B and C together, as dyspnea is commonly reported in clinical practice, especially in the elderly, and is a symptom without high specificity. We also specified in Tables 2, 3 which HF stage has been considered in the reported studies.
Diseases Affecting Mitral Valve
Mitral valve (MV) disease corresponds to the stage B of the American Heart Association HF classification (119), according to the presence of structural heart disease potentially responsible for symptoms onset. MV disease directly expose pulmonary circulation to volumetric and/or pressure overload due to the absence of protective valves between LA and pulmonary veins. Indeed, the LA exerts a watershed effect between the MV (or the LV) and pulmonary circulation. Chronic pressure and volume overload may lead to important LA remodeling characterized by wall fibrosis, dilatation, loss of compliance, and dysfunction directly affecting pulmonary hemodynamics (120).
Mitral Regurgitation
The fibrotic process affecting LA secondary to MV diseases has been extensively reported in animal models. Increased levels of atrial collagen I in miniature pigs with chronic MR, mediated by the suppression of the histone deacetylase SIRT1 (silent information regulator 1), have been reported (121). Using a similar animal model, the upregulation of fibrosis-related gene transcription has also been demonstrated in LA walls, along with the increasing of angiotensin II tissue concentrations (122). In humans, analogous findings were described by Butts et al. who observed an important chymase activation in the LA walls of patients with MR, responsible for higher degrees of fibrosis, chamber enlargement, and decreased total emptying fraction (123).
Although a direct measure of LA fibrosis is very challenging in clinical practice, the early effects of such process may arise as a loss of compliance and a stiffness increase detectable through myocardial deformation analysis even before the chamber enlargement occurs. Cameli et al. demonstrated the usefulness of reservoir function, assessed through two-dimension speckle tracking echocardiography (2D-STE), to study the extent of LA fibrosis and the loss of function in 46 patients with severe MR. They showed a close negative correlation between the degree of fibrosis and the reservoir function providing histologic assessment in atrial samples obtained during cardiac surgery (124). The histologic analysis has also been used to demonstrate the correlation between the loss of reservoir function and the severity of fibrofatty myocardial replacement in 13 patients with organic MR studied with feature tracking CMR. Interestingly, the volumetric remodeling did not correlate with the degree of histological derangement that resulted with better reflected by reservoir function (125).
The remodeling process is progressive and characterized by two main phases: an early adaptation where the chamber is able to enlarge maintaining a normal SV, and a second phase where maladaptive remodeling prevails. Animal studies showed the association between MR progression and the bi-phasic atrial SV adaptation. At earlier stage, the LA enlargement favors the atrial shortening, which is essential to maintain adequate SV. Later, the SV starts to decline as the regurgitation progresses due to the shift of volume-force relationship toward a more unfavorable position (126). In humans, a significant negative correlation between ERO, reservoir, and contractile function has been reported in 102 patients with MR, including 14 patients with primary and 88 patients with secondary MR. Most of the examined cohort (84%) had a non-severe regurgitation with ERO lower to 0.2 cm2, demonstrating that even a mild degree of MR may lead to significant LA remodeling (127). A similar result has been recently confirmed in 80 patients with mild (n = 15), moderate (n = 20), and severe (n = 45) degenerative MR studied with 3D transthoracic echocardiography and vector velocity imaging. LA contractility (responsible of the active SV component) increased in response to greater LA Volume before atrial contraction (LAVpreA) up to a point beyond which the active component decreased (128). This mechanism has been further confirmed in analyzing global and regional LA mechanics in 27 patients with chronic primary MR. Compared to controls, the LA ejection force (21.5 vs. 12.3 kilodynes), the reservoir strain (32.91 ± 14.26 vs 23.14 ± 7.96%,), reservoir strain rate (2.65 ± 0.87 vs 1.62 ± 0.53 s−1), conduit strain rate (−2.02 ± 0.58 vs. −1.29 ± 0.59 s−1), contractile strain rate (−2.55 ± 1.31 vs. −1.98 ± 0.65 s−1), and the LA contractile tissue velocity (A′) (−5.39 ± 1.95 vs. −6.91 ± 1.80 cm/s) resulted to be all impaired, despite a similar global LA ejection fraction (31.34 vs. 29.23%), confirming the importance of active LA contraction in providing adequate LV filling (129).
In more advanced stages of MR, all the components of LA mechanic may be impaired (Figure 3). In 43 patients with chronic primary MR, due to myxomatous valve disease, LA reservoir and contractile function, and the LA EF were impaired, whereas the conduit function was preserved. Interestingly, regional differences in LA contractility emerged in the anterior wall, probably due to the eccentricity of the systolic, anteriorly directed regurgitation jet, hitting the anterior wall and altering local wall mechanics (130). LA subclinical dysfunction has been reported in 50 patients with MV prolapse determining mild (n = 14), moderate (n = 19), and severe (n = 17) MR. Reservoir function resulted to be progressively impaired through the MR degrees, showing negative correlations with EROA, vena contracta, LA area, and LA volume and positive correlations with LV LS and untwisting rate (131).
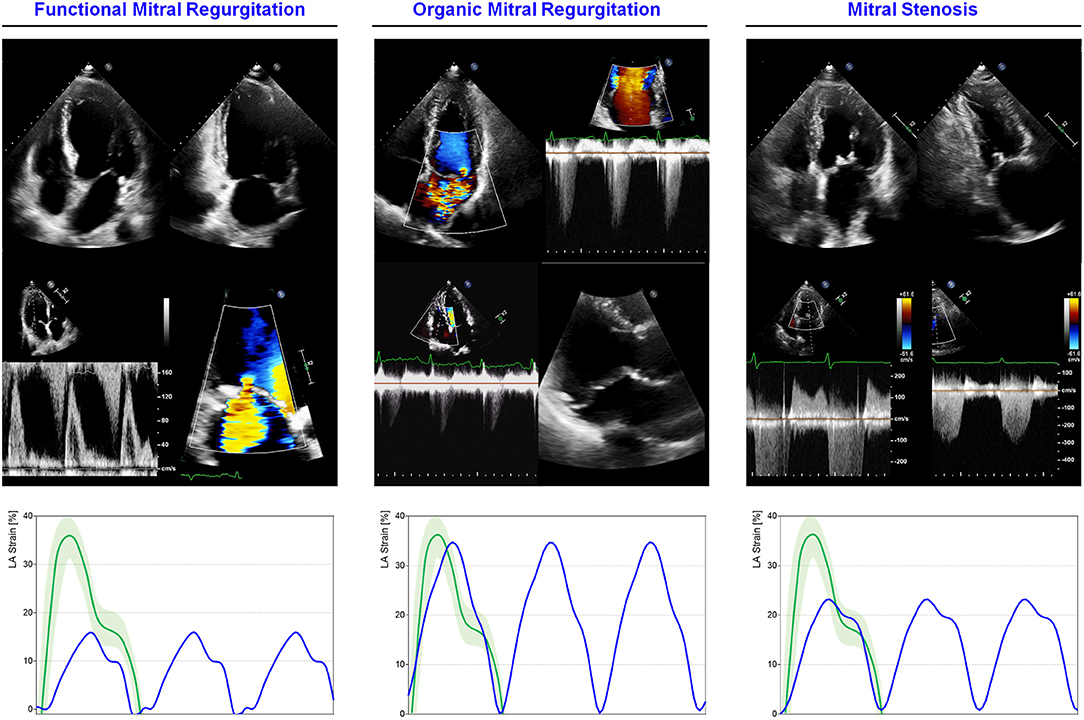
Figure 3. LA mechanics in mitral valve diseases: Examples of echocardiographic images and LA strain are shown for (left) functional MR in HF subjects with severely impaired LA function; (middle) severe MR secondary to bi-leaflet valve prolapse with preserved LA function; (right) moderate mitral stenosis with moderately impaired LA function. Strain traces are cartoons realized with real values of the reported cases. Reference values for LA strain are shown in green. Note that in organic MR, LA is still able to maintain adequate reservoir function differently from functional MR where LA function is exhausted for to the coexistence of HF. Abbreviations as in the text.
The LA mechanics analysis can be of additional value for symptoms prediction in subjects with MR. Using 3D speckle tracking (3DST), Saraiva et al. assessed the correlation between LA function and pulmonary pressures in 71 patients with organic chronic MR. They reported an association between pulmonary systolic pressures, LA reservoir, and contractile function and reservoir strain rate (132). Moreover, reservoir function was correlated with a worse functional capacity and HF symptoms (NYHA III) in patients with chronic severe primary MR. Interestingly, this parameter was also linked to age and diabetes mellitus, suggesting a more accelerated LA remodeling in diabetic patients (133).
The assessment of LA mechanics has a role in prognostic stratification, identifying subjects with more advanced disease stage. In 67 asymptomatic patients with chronic primary MR, a reduced LA reservoir (<31.7%) and LV untwisting rate (< −87.9°/s) were able to identify subjects who experienced hospitalization for HF, MV surgery, or death during follow-up (24.8 ± 17 months), confirming that impaired LA mechanics and not the regurgitation severity are linked to the outcome (31). Similarly, Yang et al. examined the prognostic significance of LA mechanics in 104 patients with asymptomatic chronic severe primary MR. At follow-up (13.2 ± 9.5 months), low reservoir function (odds ratio, 3.606; 95% CI, 1.294–10.052; p = 0.014) and low reservoir strain rate (odds ratio, 2.857; 95% CI, 1.078–7.572; p = 0.035) predicted the incidence of cardiovascular mortality or MV surgery due to new-onset HF (32). Another large study on 395 asymptomatic patients with primary degenerative MR of moderate severity is in agreement. Impaired reservoir strain, LA emptying fraction, larger LA indexed volume, and lower LV strain emerged as independent predictors of cardiovascular events (AF, stroke/ transient ischemic attack, acute HF, and cardiovascular death). A global reservoir function lower than 35% emerged as the best predictor of adverse outcome during a follow-up of 3.5 ± 1.6 years (AUC of global reservoir function:0.87) (43). Similar findings have been reported in 117 subjects with moderate to severe MR due to prolapse. LA emptying fraction (HR, 2.59), reservoir strain (HR, 3.06), and contractile strain (HR, 2.01) were independently associated with cardiac surgery or all-cause mortality (38).
The serial assessment of LA mechanics may provide early insights on the chamber remodeling progression. Fifty-five patients with severe chronic MR caused by mitral prolapse or flail underwent multiple echocardiographic evaluation during a follow up of ≤ 3 months. The variation of strain rate of reservoir function from baseline to follow-up emerged as the only predictor of accelerated LA remodeling (ΔLAVi ≥ median value). Additionally, a poor baseline reservoir strain rate was significantly associated with hastened deterioration of the same parameter during the follow-up period (134).
The surgical timing for MR is another clinical context where the assessment of LA mechanics may improve patient management. In a large cohort of patients with MV prolapse and different MR degrees, total LA emptying fraction [odds ratio (OR):0.78; p < 0.001], reservoir function (OR:0.91; p = 0.028), and contractile function (OR:0.86; p = 0.021) emerged as independent predictors for surgery indication. Total LA emptying fraction <50% demonstrated a 91% sensitivity and 92% specificity for predicting surgical indication (135). LA reservoir strain can be used to predict outcome after surgical correction in patients with chronic severe organic MR, with values lower than 24% identifying the worse survival during a median follow up of 6.4 years (29). Similar results have been confirmed in a cohort of 71 patients with primary severe MR undergoing surgical treatment, where LA reservoir function resulted as independent predictor of clinical and functional outcome and of LA fibrosis. Considering a composite event of HF and/or cardiovascular death, 5-year event-free survival was 90 ± 5% for LA reservoir strain ≥21% and 30 ± 9% for reservoir strain <21% (p < 0.0001). Moreover, it was associated with an improvement of NYHA class and Borg scale after surgery (50). Preoperative reservoir strain, LAVi, and age may also predict valve repair or replacement or atrial inverse remodeling, defined as a percentage of decrease in LA volume index (136).
Differently from organic or primary MR, that is a clinical condition leading to HF if untreated, functional, or secondary MR frequently comes as a direct consequence of LV dilatation and dysfunction, further impacting on prognosis and clinical status (137–140). Rest assessment of LA mechanics in HF with severe MR has a role in prognostic stratification (Figure 3). Palmiero et al. investigated LA function in 97 patients with HFrEF and severe functional MR identifying the LA emptying fraction as an independent predictor of cardiovascular death (141).
The evaluation of LA mechanics during exercise may provide additive information, with specific insights on valvular and functional reserve (142, 143). In asymptomatic patients with degenerative MR, the presence of exercise-induced pulmonary hypertension (PH) was associated with lower reservoir function with the 26.9% threshold being independent predictor of a worse symptom-free survival (36). The difference in atrial function between primary and secondary MR has been studied by Sugimoto et al. with exercise stress echocardiography and CPET in 196 patients with primary and secondary MR, including 66 HFrEF, 19 HFpEF, and 30 HF with mid-range ejection fraction (HFmrEF). Exercise reservoir strain and contractile function were impaired in any MR type but with secondary MR exhibiting a worse atrial reservoir function. This was associated with a worsen exercise performance, limited CO increase, impaired right ventricular–to–pulmonary circulation coupling, and the highest event rate. Furthermore, LA strain during exercise was predictive of all-cause mortality and hospitalization for HF (144).
Organic MR is characterized by progressive LA remodeling consisting of atrial wall fibrotic replacement leading to a loss of reservoir and contractile function, with prognostic significance for surgery prediction. Functional MR may present with an impairment of greater severity. The exercise-related LA functional reserve is associated with the exercise capacity and clinical outcome.
Mitral Stenosis
Only fewer studies have been conducted to assess the impact of mitral stenosis (MS) on LA mechanics. Myocardial structural remodeling in MS is a known morphologic substrate of LA dysfunction that may lead to AF and adverse outcome (145). Thus, assessment of LA function in combination with LA volumetry may help guiding clinical decisions in patients with MS. Caso et al. assessed the prognostic role of LA function in 53 asymptomatic patients with rheumatic MS, finding that the best predictor of adverse events (defined as symptoms, hospitalization for cardiac cause, AF, thrombo-embolic events, valvular surgery, or percutaneous commissurotomy) at 3-year follow-up was the average LA peak systolic strain rate (cut-off value of 1.69 s−1), having a sensitivity of 88%, and a specificity of 80.6% (AUC:0.852) (146). Atrial mechanics analysis may be useful to predict the risk of AF in MS (Figure 3). In 81 patients with MS, an impairment of reservoir strain was observed in patient who developed arrhythmia at 5-year follow-up (13.4 ± 4.6 vs. 19 ± 5.2, p < 0.001) (147). Similarly, in a large cohort of asymptomatic patients with rheumatic MS that was followed up during 4 years, reservoir function was the best predictor of AF at multivariable analysis (AUC of.761 for a cut-off value of 17.4%) (30).
In order to assess possible correlation between LA function and exercise capacity in subjects with MS, Jung et al. evaluated the LA compliance (defined as 1,270 × mitral valve area by planimetry/E-wave downslope) during exercise in 33 asymptomatic patients with significant MS. Decreased LA compliance at an early stage of exercise (50 W during bicycle exercise) was an independent predictor of exercise intolerance. Moreover, a positive relationship was also noted between the chamber compliance and the pressure response of pulmonary circulation, with a more impaired LA compliance in patients who developed dyspnea at an early stage of exercise (148). Mahfouz et al. performed stress echocardiography to assess exercise intolerance in 75 patients with MV area of 1.81 ± 0.13 cm2 and compared them with 40 healthy control subjects. Interestingly, 44% of asymptomatic patients with mild MS had exercise intolerance, and reservoir strain was significantly associated with exercise capacity in patients with mild MS (cut-off value: reservoir strain ≤ 26.5%) (46). Similarly, Chien et al. investigated the relationship between LA deformation as measured by 2DSTE derived LA strain and HF symptoms in 69 subjects with rheumatic MS, and found that NYHA class independently correlated with LA reservoir strain and reservoir strain rate (149).
LA reservoir function and compliance are related to exercise tolerance in MS. Reservoir function may predict the occurrence of AF.
Diseases Affecting Aortic Valve
Aortic Stenosis
In aortic stenosis (AS), the outflow obstruction caused by a valve narrowing determines LV concentric hypertrophy and a strong predisposition to HF. The increased afterload is responsible for LV pressure overload, hypertrophy, myocardial fibrosis, impaired relaxation, and finally LA abnormal mechanics. At early stages, LA function is preserved, thus helping in the maintenance of optimal CO, but at later stages, atrial dilatation and dysfunction occur with different mechanisms (150). It has been shown that only the reservoir function impairs progressively with AS severity (151). On the contrary, contractile function seems initially enhanced in subjects with severe valvular disease without pulmonary hypertension. The enhanced contractile function acts as a compensatory mechanism driven by Frank-Starling law (LA myocytes length is augmented since LA volume is increased). Once this mechanism is exhausted, the LA contractile function starts to decline, and the chamber starts to dilate.
The occurrence of HF symptoms, such as dyspnea in AS, may represent the decline of clinical conditions leading to unfavorable outcome. However, in clinical practice it may be challenging to correlate the symptom with the disease progression, since the dyspnea is a common condition in elderly people, but is not necessarily of pathologic significance. LA mechanics can provide useful insights in discriminating the origin of symptoms (Figure 4). A retrospective study on 40 patients with severe AS identified contractile function, assessed through 2DSTE, as the only independent predictor of HF symptoms (dyspnea, angina, dizziness, and syncope upon exertion) at multivariate logistic regression (OR = 0.242, p = 0.002) including AS severity, BNP, and LV diastolic function (cut-off: LA contractile strain rate <1.01 s−1) (33). Recently, in a large cohort of 248 patients (202 symptomatic and 46 asymptomatic) with severe AS and preserved LV EF, the reservoir function emerged as the only parameter independently correlated with the presence of HF symptom, while LA dimensions and the echocardiographic parameters of both LV systolic and diastolic functions did not (44). Moreover, LA longitudinal strain parameters were inversely correlated with the worsening of NYHA class. These findings are consistent with the greater ability of LA mechanics in predicting prognosis when compared with LV mechanics analysis (34, 152, 153). Galli et al. (35) demonstrated that LA reservoir function (<21%) is predictive of major adverse cardiac events and HF in 128 symptomatic and asymptomatic patients with severe AS, while LV function parameters (ejection fraction and global longitudinal strain) are not. This result has been further confirmed in a recent study on 182 symptomatic and asymptomatic patients with moderate and severe AS (154). Moderate AS showed greater values of LA reservoir (23.1 vs. 13.8%, p < 0.001), conduit (11.5 vs. 6.5%, p < 0.001), and contractile function (11.5 vs. 7.1%, p < 0.001) when compared to severe valvular disease. On the other hand, no differences emerged when comparing LV EF, systolic, and diastolic diameters in the two populations (p > 0.1). The stronger prognostic and clinical significance of LA vs LV mechanics is attributable to the specific disease pathophysiology. The LV remodeling impacts on LA that dilates, enhancing reservoir and contractile function. This compensatory mechanism does not last for long time producing further pressure overload in pulmonary circulation and symptoms appearance. The inverse correlation between LA reservoir strain and PH in patients with severe AS and preserved LVEF, reflects this mechanism (155).
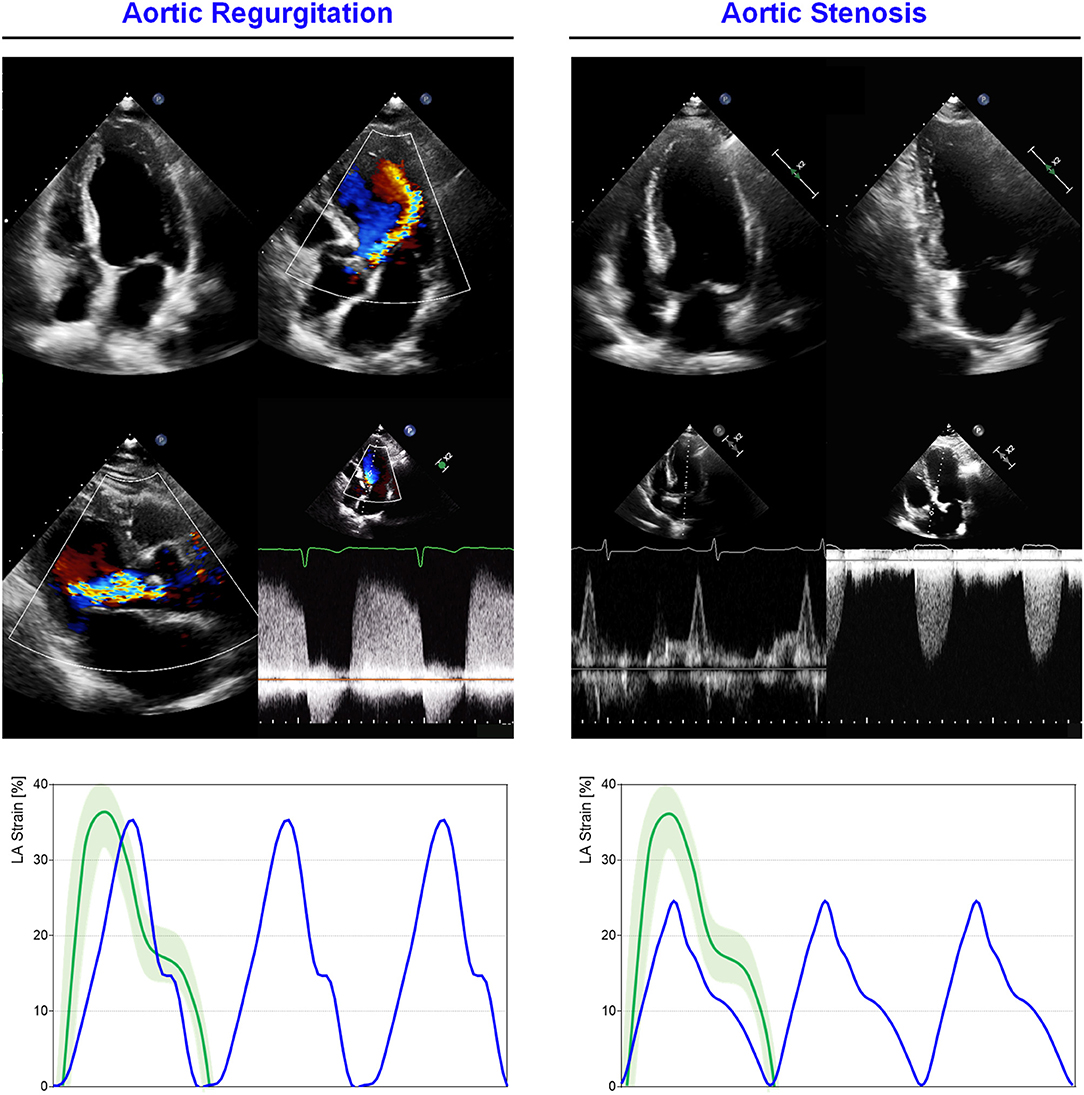
Figure 4. LA mechanics in aortic valve diseases: Examples of echocardiographic images and LA strain are shown for (left) severe aortic regurgitation (AR); (right) severe aortic stenosis (AS). Strain traces are cartoons realized with real values of the reported cases. Reference values for LA strain are shown in green. Note that reservoir function in AS is impaired, consistently with increased LA pressures secondary to concentric LV hypertrophic remodeling, while in AR the degree of reservoir impairment is lower. Abbreviations as in the text.
The importance of LA mechanics in predicting outcome in patients affected by severe AS has been corroborated by several studies reporting LA “reverse remodeling” in patients who underwent transcatheter aortic valve replacement (TAVR). LA enlargement has been reported as a marker of HF readmission after TAVR. In a large retrospective cohort of 546 patients, LA dilatation, identified by parasternal diameter (48.4 ± 7.9 vs. 43.1 ± 7.2 mm, p < 0.0001), was independently associated with readmission for congestive HF at 1-year follow-up (156). TAVR is generally associated with an improvement of LA mechanics, in particular, reservoir function, as assessed with 2DSTE 6 months after treatment (157). A similar improvement of LA reservoir and conduit function has been described after a longer follow up of 12 months, regardless of the AS severity, in 54 mixed severe AS (24 normal LVEF and normal flow, 16 with paradoxical low flow low gradient, and 14 with a reduced LVEF) (158).
According with the pathophysiology of AS disease, the exercise is the natural condition able to trigger LV afterload increase, LV, and LA pressure overload and therefore dynamic pulmonary congestion. While in healthy subjects, the LA reservoir and conduit functions are enhanced under stress conditions (16), the hypertrophic LV is expected to prevent relaxation and diastolic suction due to the lower compliance that chronically overloads the atrial chamber (15). Along with disease progression, LA exhausts its functional reserve leading to pulmonary congestion and symptoms appearance. Based on this pathophysiology, the rationale for dynamic assessment of LA mechanics is strong but still underused. Exercise testing with simultaneous invasive hemodynamic monitoring and Doppler echocardiography have been used to evaluate 39 patients with asymptomatic severe AS. LA size (LAVi ≥ 35 ml/m2) reflected the hemodynamic burden (augmented PCWP and PAP, decreased CI) and was associated at univariate Cox analysis with poor outcome (composite end-point of hospital admissions for AF, HF, and acute coronary syndrome, aortic valve replacement, and death), being a potential marker of increased hemodynamic burden during exercise. Moreover, this study suggested that when LA dilatation is overt and E/e′ is also increased, the pulmonary circulation overload is augmented, especially under stress conditions (159).
The study of LA mechanics has been shown to be more informative compared to the LV study, especially for the prediction of HF and functional capacity. LA reservoir function is a marker of inverse remodeling occurring after AVR. The assessment during exercise is a promising frontier for the identification of asymptomatic patients at higher risk.
Aortic Regurgitation
The backward flow caused by aortic regurgitation (AR) may affect LV mechanics imposing chronic volume overload, increased LV stiffness, chamber dilatation, and dysfunction. The process can persist for a long period before symptoms onset due to the ability of the LV to compensate hemodynamic overload (67). LA involvement may result from several mechanisms, including impaired LV diastolic function, fibrosis, reduced compliance, and secondary MR (160), reflecting the AR stage.
A direct correlation between LA reservoir function and PH has been reported in patients with moderate or severe AR, with a 6% increase of PH risk for each unit of LA strain decrease (151). Recently, a large study on 554 patients with bicuspid aortic valve and moderate or severe AR showed that the LA enlargement (LAVI ≥ 35 ml/m2) was independently associated with adverse outcome (aortic valve surgery or mortality), when compared with patients with normal LAVi (43 and 60% vs. 23 and 36%, at 1 and 5 years of follow-up, respectively, p < 0.001) (161). In addition to LA enlargement, its contractile function is impaired in severe AR, as reported in 65 patients scheduled for AVR and assessed with 2DSTE. The evaluation 1 year after surgery showed a reduction in LAVi (38 vs. 32 ml/m2, p < 0.001), and an improvement in both LA reservoir and contractile function (26 vs. 29% and 11 vs. 15%, respectively, p < 0.01) (162). These findings suggest that the volume overload imposed by AR affects LV morphology and function, along with LA mechanics, through diastolic impairment.
Although AR and AS are two distinct models of LV overload (AS determines a pressure overload while AR volume overload). They share some common effects on the LA mechanics (Figure 4). Cioffi et al. (163) compared 141 AS patients with 42 AR looking at LV geometry, LA size and function. In addition to LA size and ejection force significantly greater in AS group (Maximal LA Volume: 26 ± 7 vs 218 ± ml/m2, p = 0.0009), LA enlargement has a positive correlation with LV mass depending on LV pattern. In particular, the concentric LV pattern is related to a greater LA volume and contractile function, irrespectively of valve disease, whereas the eccentric LV geometry does not determine a relation between LA size and LV mass.
Exercise testing can unmask patients reporting to be asymptomatic. Assessment of contractile reserve is a key element to reveal subclinical LV dysfunction. The absence of contractile reserve is more predictive of the development of systolic dysfunction both at follow-up (medical therapy) and postoperatively than parameters obtained at rest in subjects with severe AR (164). Patients with severe AR may show an exercise-induced fall in LVEF due to the hemodynamic consequences of volume overload and increased afterload (165). However, the reliability of this finding in predicting outcome is controversial and it is not specifically addressed in the most recent ACC/AHA and European society of cardiology (ESC) guidelines (93, 166). If the use of exercise is logical to trigger effort-related symptoms, then, in AR, the diastole shortening induced by chronotropic response can potentially reduce the regurgitation severity, hindering AR quantification (11). AR has been evaluated through a standard cardiac 1.5-T CMR scanner under steady-state submaximal exercise and at rest. The AR % decreased during exercise from 35 (9–26, 39, 56–92) % at rest to 16 (7–25, 56–72)% during exercise, p = 0.003. In addition, AR at rest correlates with an increase of cardiac index during submaximal exercise (R2 = 0.64; p = 0.001) (167). These findings support the use of submaximal exercise to evaluate LA adaptation during effort, favoring the use of exercise echocardiography to detect an abnormal response at early stages.
LA enlargement, loss of reservoir, and contractile function have been related to AR severity and adverse outcome in preliminary results. AVR has been shown to have a positive impact on reservoir and contractile function.
Diseases Affecting Cardiac Muscle
Hypertrophic Cardiomyopathy
Hypertrophic cardiomyopathy (HCM) is the most common genetic heart disorder with a prevalence of 1/200 people (168). Regardless the specific etiology, the advanced disease is characterized by LV hypertrophy, diastolic dysfunction, and increased LV filling pressures. LA adapts enlarging, increasing its contractile function until functional reserve exhausts resulting in overt dysfunction. 2DSTE has been used to describe global and phase-specific function in HCM patients (Figure 5). Total atrial deformation (defined as the sum of maximum positive and maximum negative strain during a cardiac cycle) has been shown to be significantly lower in HCM patients when compared to control subjects (169). All the three components of LA mechanics seems to be impaired in HCM patients compared to controls, in particular, strain rate at reservoir, conduit, and contractile phase has been showed to be 13, 17, and 10%, respectively lower (2.0 ± 0.6 vs. 2.3 ± 0.5 s−1, 1.9 ± 0.8 vs. 2.3 ± 0.7 s−1, 2.6 ± 0.8 vs. 3.0 ± 0.8 s−1; p < 0.05) (170). The degree of LV hypertrophy and fibrosis, assessed through CMR, is directly proportional to the degree of LA impairment. Compared with healthy controls, LA conduit function in HCM is impaired, even without extensive LGE, thus with mild or absent LV fibrosis. Conversely, LA contractile function is reduced only in HCM patients with a higher degree of fibrosis, leading to a more advanced diastolic dysfunction, consequent to LA enlargement and functional impairment (171). A more advanced atrial myopathy and disfunction play a specific role in determining symptoms. Contractile function, explored with 2DSTE, emerged as the only independent predictor of HF symptoms with a cut-off of −0.92 s−1 for contractile strain rate (sensitivity: 75%, specificity: 83%, area under the curve:0.83) in a series of 37 HCM patients, with enlarged atria compared to controls (28). In less advanced disease, LA dysfunction can be already present even if the chamber size is still in the normal range. In non-obstructive HCM patients with normal LA size and contractile function, reservoir and conduit components resulted impaired when compared with healthy controls. This finding is consistent with a lower degree of LV fibrosis, earlier disease stage and more preserved atrial physiology (172). On the other hand, LA function further lowers if LV outflow tract obstruction is present, as demonstrated comparing 50 obstructive, 50 non-obstructive and 50 healthy patients studied with feature-tracking CMR. The presence of obstruction has a great impact on LA EF (42.3 ± 8 vs. 47.2 ± 9%; p = 0.004), reservoir strain (14.5 ± 4 vs. 17.7 ± 5%; p = 0.002), strain rate (0.59 ± 0.2 vs.73 ± 0.2 s−1; p = 0.001), contractile strain (6.1 ± 2 vs 7.5 ± 3%; p = 0.01), and strain rate (−0.44 ± 0.1 vs −0.58 ± 0.25 s−1; p = 0.004) when compared to the non-obstructive HCM group (173) (Figure 5).
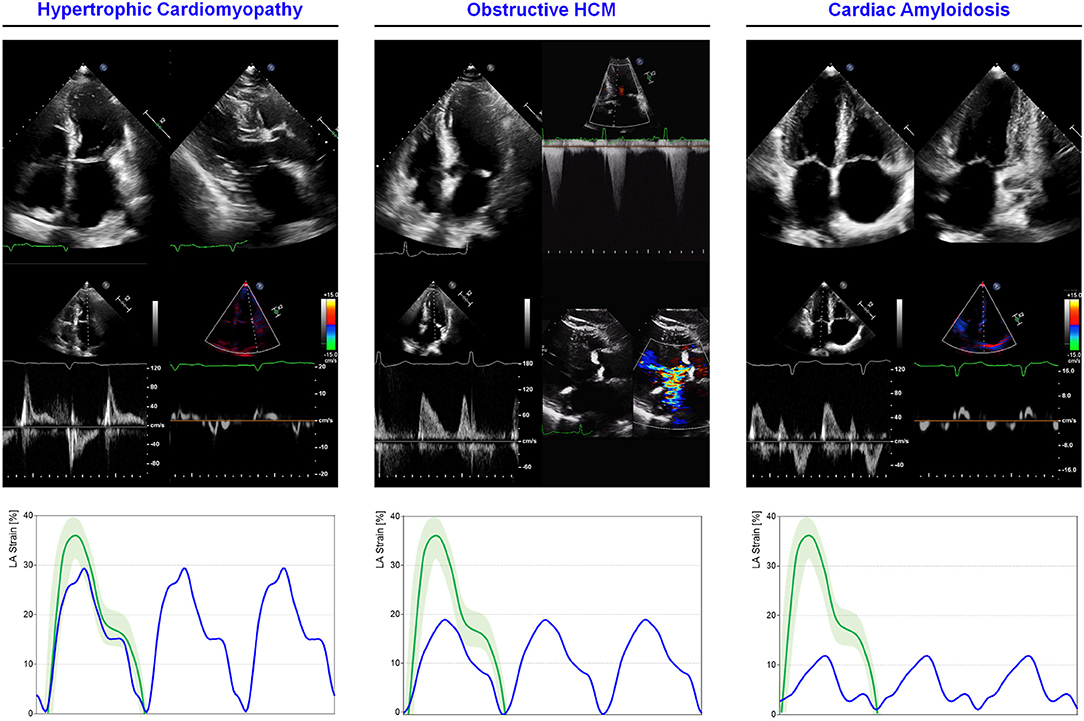
Figure 5. LA mechanics in cardiomyopathies: Examples of echocardiographic images and LA strain are shown for (left) non-obstructive HCM, (middle) obstructive HCM and (right) AL cardiac amyloidosis. Strain traces are cartoons realized with real values of the reported cases. Reference values for LA strain are shown in green. Note that non-obstructive HCM presents severe diastolic dysfunction while obstructive HCM has a greater impairment of reservoir function; AL cardiac amyloidosis presents very depressed reservoir function consistent with severe diastolic dysfunction and LA amyloid infiltration. Abbreviations as in the text.
Different studies evaluated the prognostic role of LA function assessment in HCM patients. An early report identified total LA strain (the sum of reservoir and contractile function) as the strongest predictor of short term (12-month) outcome of death or hospitalization for cardiovascular causes, with the optimal cut-off of 21% (odds ratio 0.858, 95% CI 0.771–0.954, p = 0.005). The same parameter was also informative for the occurrence of AF requiring hospitalization (odds ratio 0.853, 95% CI 0.748–0.972, p = 0.017) (27). The long-term prognostic significance of LA function was further demonstrated in a large series of HCM subjects and controls, followed-up for 55 months. Total LA strain (−17.4%; p < 0.001), LV outflow tract obstruction (p < 0.001), and E/e' (10.3; p = 0.02) emerged as independent predictors of the composite endpoint of all-cause death, heart transplantation, LV assist device implantation, and clinical worsening (41). Recently, the prognostic value of LA function assessment has been addressed with the use of CMR. During a long follow up-up of 40.9 months, 59 patients with HCM (19.7%) out of 359 experienced the composite endpoint of cardiovascular death, resuscitated cardiac arrest, sudden cardiac death aborted by appropriate ICD discharge, and HF hospital admission. Reservoir and conduit components (HR, 0.94 and 0.89; p = 0.019 and 0.006, respectively) emerged as independent predictors of outcome, also after correcting for the extent of LV fibrosis, confirming the prognostic significance of LA mechanics and expanding the clinical applications of CMR-based myocardial deformation analysis (47).
Throughout the clinical history of HCM, the occurrence of AF marks a critical point potentially impacting on HF symptoms and prognosis. The study of LA mechanics can be informative on the occurrence of new-onset arrhythmia. In large series of HCM patients studied with 2DSTE, LA volume and reservoir strain were able to predict the occurrence of new-onset AF during a follow-up of 4.8 ± 3.7 years. The presence of LA reservoir strain >23.4% predicted a superior 5-year AF-free survival (98 vs. 74%, p = 0.002) (174). This finding has been recently confirmed by Vasquez et al. who reported a similar threshold for reservoir function (> 23.8%) and a threshold of 10.2% for conduit function to predict event-free survival during a follow-up of 5.83 ± 0.3 years (events defined as heart failure, stroke, and death) (42).
In HCM patients, LA mechanics may partially restore after surgical and non-surgical therapy, as observed in a subset of 20 patients with obstructive HCM who underwent septal myomectomy, after which LAEF (41.6 ± 13 vs 48.4 ± 10%; p =0.006), reservoir (14.1 ± 6 vs. 17.3 ± 7%; p = 0.01) and contractile function (6.8 ± 4 vs 9.8 ± 5%; 0.0001) increased at CMR (173). The reversibility of reservoir impairment after treatment provides an additional explanation of the clinical response to the septal reduction. These findings, obtained with non-invasive approach, are consistent with previous data reporting an improvement of LV relaxation with consequent increase of LV passive filling volume, decrease in LA volumes, ejection force (defined as: 0.5 x 1.06 x mitral annulus area x (peak A2), in kdyne), kinetic energy (0.5 x 1.06 x LA SV x (peak A2) in kerg), and a parallel reduction of NYHA class, with longer exercise duration (p < 0.05) (175).
Left Atrium (LA) mechanics progressively disrupt in HCM, reflecting the disease severity and fibrosis extension. Reservoir function is the first to decline, especially when HCM determines LVOT obstruction. All the components of atrial function are able to predict the outcome, representing valid prognostic markers.
Restrictive Cardiomyopathy
Despite restrictive cardiomyopathies (RCMs) are known to be the least common among the heart muscle diseases, they include a wide group of conditions characterized by different pathogenesis, clinical presentation, diagnostic workflow, treatment, and prognosis (176). Some possible etiologies include infiltrative disorders, such as amyloidosis or sarcoidosis, storage disorders like Fabry disease, and idiopathic RCM. The ventricular myocardium generally presents with increased stiffness, responsible for the characteristic diastolic dysfunction, elevation of filling pressures, and atria dilation. Systolic function is usually preserved until the late stages of the disease. During exercise, the poor compliance of the ventricles hinders the rapid venous return, resulting in an important rise of the filling pressures and in a limited increase of SV (177). The progressive atrial enlargement may contribute to the onset of HF symptoms, atrial arrhythmias, or secondary atrioventricular valvular regurgitation.
In cardiac amyloidosis (CA), the LA dysfunction has been repeatedly studied. Loss of all components of atrial function characterizes cardiac amyloidosis regardless of the etiology (light chain, mutant or wild-type transthyretin). However, among the amyloidosis subtypes, transthyretin amyloidosis (ATTR) wild type generally present the worst reservoir and contractile function. Of note, even after adjusting for LA size, LV EF, and LV filling pressures, all LA function components are generally impaired in CA patients, when assessed with 2DSTE (Figure 5) (39, 178, 179). A significative alteration in the reservoir and contractile function has also been recorded with real-time 3D echocardiography, and there is evidence that, according to the progression of the disease, the LA mechanics gradually undergoes greater impairment (39, 179). Recently, myocardial deformation of both LA and LV have been shown to be linked to prognosis in CA. In particular, a reservoir function <13.2% demonstrated a 7.5-fold increased risk of all-cause mortality over a median follow-up of 5 years (95% CI 3.8–14.7, p < 0.001) in a cohort of 136 patients (48). Remarkably, in a large population of >900 subjects with ATTR, the LA stiffness, estimated as the ratio between E/e' and reservoir function, has been shown to be an independent marker of prognosis, after adjustment for the main echocardiographic and clinical parameters. In the same population, the presence of LA electro-mechanical dissociation (absence of valid mechanical contraction despite sinus rhythm at ECG) emerged as a distinct phenotype with impaired outcome, similar to subjects with AF (49). LA mechanics are therefore significantly impaired in infiltrative disease, with a growing evidence of a direct LA involvement contributing to the severe mechanics impairment.
Unlike cardiac amyloidosis, primary LA involvement is less clear in sarcoidosis. Small studies using 2DSTE reported impairment of LA reservoir function in sarcoidotic subjects when compared to controls, with a negative correlation with the disease stage. However, it is unclear if the loss of function is a consequence of a primary atrial involvement or simply a consequence of LV dysfunction (180, 181).
Left atrium (LA) reservoir function, evaluated by 2DSTE, has been reported to be significantly impaired in patients with Fabry disease even in presence of normal echocardiographic assessment (182, 183). It is still unclear if these findings can be primarily explained by the increase of LV filling pressures or by the direct depositions of sphingolipids in the LA. However, LA stiffness seems to be an early marker of atrial remodeling, already altered before the occurrence of LV hypertrophy (184). Conversely, a relevant impairment in atrial conduit function has been reported only in presence of LVH, and this may be justified by a more advanced LV diastolic dysfunction (184). Using CMR with T1 mapping to classify patients with Fabry disease, Bernardini et al. reported a progressive impairment of reservoir function, assessed with FT-CMR, according with the reduction of T1 mapping (index of subclinical disease) or the presence of LV hypertrophy (overt heart involvement) (185). Nevertheless, in another study, significant differences in the three LA function components were found only in Fabry disease with significant LV hypertrophy (186).
Despite the heterogeneity of restrictive diseases, the impairment of reservoir function has been reported as a common pathophysiological element. In aTTR-CA, the estimation of LA stiffness emerged as a strong and independent prognostic marker.
Dilated Cardiomyopathy
Dilated cardiomyopathy (DCM) is a complex pathological condition coursing with HF and representing the most common indication for heart transplantation worldwide (187), and it is characterized by the presence of LV dilatation and systolic dysfunction, worsened by abnormal LV filling pressures and functional mitral regurgitation in the most advanced stages (188, 189). At earlier stages, LA contractile function is augmented to maintain adequate LV filling, but later it decreases as a consequence of the increased afterload.
In a series of 160 DCM and 154 ischemic patients, studied with 2DSTE and CPET, LA reservoir and contractile functions were significantly reduced in the DCM group, with LA lateral wall reservoir and LA volume predictive of peak VO2 (both p < 0.001) (190). Similar findings were reported by Cao et al. in 32 ischemic, 26 DCM, and 32 control patients where reservoir and contractile functions were more impaired in DMC subjects (191).
The prognostic role of contractile function loss and LA dilatation has been demonstrated in a large cohort of patients with DCM (192) and recently confirmed with the use of CMR (193). LA maximal volume (LAmax) resulted to be effective in predicting the occurrence of a composite endpoint including death or heart transplantation in a population of 337 patients with DCM who were followed-up for a mean period of 41 ± 29 months. Notably, patients with an increased LA volume (LAVi> 68.5 ml/m2) had a risk ratio of 3.8 compared with those with a preserved LA volume (194). In line with these results, indexed LA area assessed with standard echocardiography (with an optimal cut-off > 13 cm2/m2) emerged as the strongest index associated with the same composite outcome both in a univariate and a multivariate model in 275 DCM patients, whom events were recorded over a mean follow-up of 67 months (HR 6.58, 95% CI 2.43–17.86, p < 0.001 and HR 3.2, 95% CI 1.06-9.23, p = 0.038, respectively) (195).
The reliability of these results is confirmed by similar findings obtained assessing the LA geometry with CMR. In particular, LAVi resulted to be an independent predictor of a composite endpoint including all-cause mortality or cardiac transplantation evaluated in 483 consecutive patients affected by non-ischemic DCM who were prospectively followed-up over a median period of 5.3 years (HR per 10 ml/m2 1.08, 95% CI 1.01–1.15, p = 0.022). Furthermore, patients with an increased LAVi (> 72 ml/m2) showed a three-fold elevated risk of death or transplantation (HR 3.00, 95% CI 1.92–4.70, p < 0.001). LAVi was also independently associated with the secondary composite endpoints of cardiovascular mortality or cardiac transplantation (HR per 10 ml/m2 1.11; 95% CI 1.04–1.19, p = 0.003), and HF death, HF hospitalization, or cardiac transplantation (HR per 10 ml/m2 1.11; 95% CI 1.04–1.18; p = 0.001) (196).
Of note, some LA morphological and functional parameters demonstrated to have a prognostic role when evaluated under stress. In particular, in 84 DCM patients studied with dobutamine stress echocardiography and followed-up for a mean period of 17.0 ± 11.8 months, LAVi (HR 1.060, 95% CI, 1.035–1.087; p < 0.001) besides the variation of systolic LA strain (HR, 0.971, 95% CI, 0.946–0.996, p = 0.02) and the variation of passive LA strain (HR 0.942, 95% CI, 0.914–0.971, p < 0.001) emerged as independent predictors of cardiovascular events in two different multivariate Cox models. Interestingly, including LA strain parameters at rest and under dobutamine into multivariate Cox analysis provides an incremental benefit in predicting adverse cardiovascular events (197).
Left atrium (LA) enlargement and loss of reservoir function occurring in DCM reflects the disease severity and have a prognostic significance for composite endpoints. The use of dobutamine to test the LA functional reserve may have an incremental value in risk stratification.
Heart Failure With Reduced, Mildly Reduced, and Preserved EF
Left atrium (LA) represents the physiological escape for the augmented LV filling pressures occurring in every type of HF syndrome (Figure 6). According with the HF duration and onset velocity, LA reacts to pressure overload adapting its dimensions (198), function, and compliance, and plays a dominant role in the disease progression (199). The remodeling ability of LA directly impacts on pulmonary circulation, eventually leading to pulmonary capillary involvement, pulmonary artery hypertension (200), and RV failure (201). Nevertheless, differences exist in the remodeling process occurring in HF with reduced vs. preserved LV EF. A greater chamber enlargement and a greater increase in LA stiffness and pressures have been described in HF with reduced ejection fraction (HFrEF) and HF with preserved ejection fraction (HFpEF), respectively through the use of right heart catheterization (RHC) and echocardiography in a large cohort of mixed HF patients (202). In HFrEF, LA dilatation is directly related to LV disease progression, primarily through the hemodynamic effects, while in HFpEF different disease pathways (i.e., inflammation and direct atrial myopathy) may interact resulting in a more complex LA remodeling (203). Nevertheless, the LA dysfunction similarly impacts on pulmonary circulation in both phenotypes, resulting strictly correlated with pulmonary vascular disease and RV dysfunction (202, 204, 205). The loss of atrial compliance, reflected by an increased stiffness (non-invasively estimated as the ratio between E/e' and reservoir function), has been showed to be predictive of HF hospitalization and cardiac death in HFrEF and HFmrEF patients (206). LA stiffness estimation has the advantage of exploring the mechanical behavior of the chamber, combining the expansibility properties with the degree of LV pressure overload.
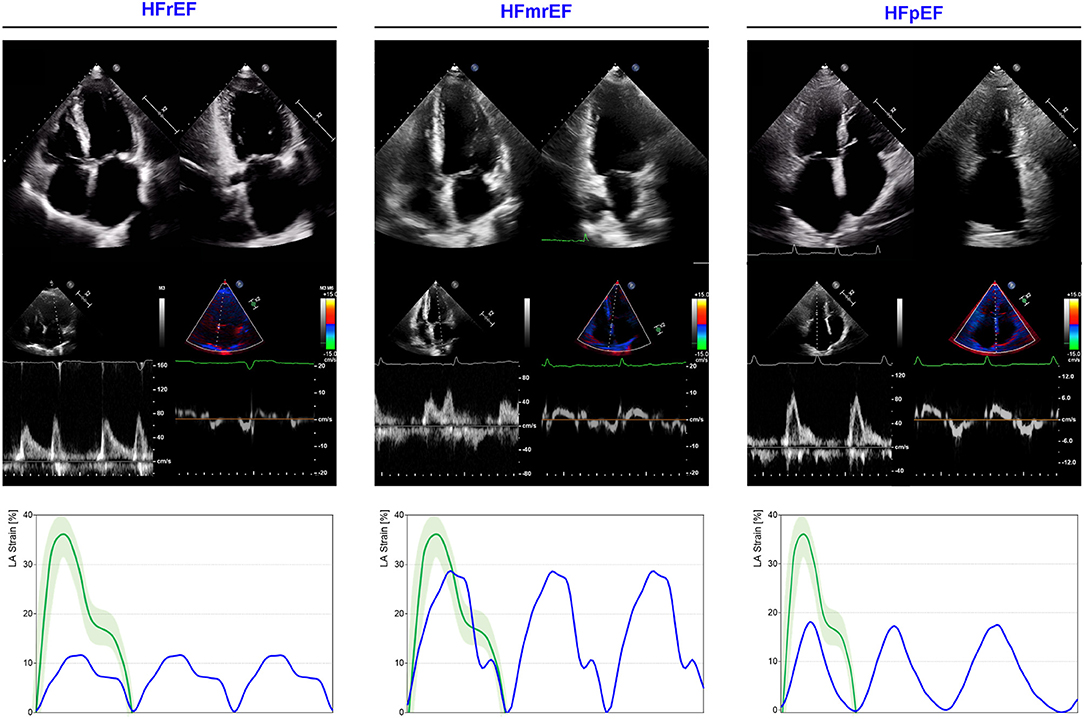
Figure 6. LA mechanics in HF subtypes. Examples of echocardiographic images and LA strain are shown for HFrEF (left), HF with mid-range ejection refraction (HFmrEF; middle) and HF with preserved ejection refraction (HFpEF; right). Strain traces are cartoons realized with real values of the reported cases. Reference values for LA strain are shown in green. Note that HFrEF has a very depressed LA function when compared with HFmrEF (expression of greater disease severity), while HFpEF presents a significantly impaired reservoir function (without contraction component for the presence of atrial fibrillation), similar to that observed in HFrEF. Abbreviations as in the text.
The non-invasive estimation of LA pressure is a cornerstone of echocardiographic evaluation, providing information on the hemodynamic conditions of LA-pulmonary circulation unit (107). An integrative approach, considering diastolic parameters, LA dimensions, and mechanics may improve the hemodynamic assessment and provide additional prognostic information. The assessment of LA reservoir function with 2DSTE improves the detection of LV diastolic dysfunction in subjects with preserved LV EF and LA size, improving the current diastolic function algorithms (45) and resulting associated with a higher risk of HF hospitalization, even after adjusting for age and sex (40).
In the context of HFrEF, LA reservoir function is an independent predictor of adverse outcomes (a combined end-points of all-cause mortality and HF hospitalization) in stable patients, with an incremental predictive value compared to standard parameters of LV function (51, 207) and LA dilatation (208). The loss of reservoir function has a prognostic significance also in the context of acute HF in subjects with preserved sinus rhythm. Moreover, reservoir function correlates with functional impairment and presents a better ability in predicting poor quality of life when compared to LA volume and LV dysfunction (67). The tight relationship between LV systolic and LA reservoir function (being the LV base downward displacement one of the main determinants) has been raised as a matter of concern about the independent prognostic significance of atrial deformation (209). However, its incremental predictive value, compared to standard parameters of LV function (51, 207) and LA dilatation (208), has been demonstrated. As for systolic and diastolic components of LV mechanics, LA function reflects several factors and interactions. Indeed, the parameters describing the single phases should not be considered per se but rather as a part of a more complex system.
The interest on LA mechanics has grown especially for the investigation of physiology, for early diagnosis and prognostic stratification of HFpEF, based on the key role of the atrium. Longitudinal data from a large study cohort showed that the loss of atrial reservoir function is associated with increased risk of HF hospitalization, even after adjusting for clinical risk factors, NTproBNP and echocardiographic parameters, in patients with coronary artery disease and preserved LV EF (65). Similarly, in patients with definite diagnosis of HFpEF enrolled in the TOPCAT trial, LA reservoir function emerged as a valid predictor of HF hospitalization (210). The effort tolerance, the most common symptom in HFpEF, is strictly modulated by reservoir function, being associated with abnormal pulmonary vascular resistance and impaired functional capacity (peakVO2) (211). The LA emptying function and LV filling properties are both correlated with NTproBNP levels in HFpEF subjects, as shown in a RELAX trial sub-study (212).
In a large cohort of 363 symptomatic patients, LA reservoir (cut off: <24.5%) function and compliance (estimated as the ratio between reservoir and E/e', cut off: 3) outperformed E/e', LA enlargement, tricuspid regurgitation velocity, LV hypertrophy and LV global longitudinal strain (GLS) in diagnosing HFpEF, using exercise RHC as a diagnostic standard (53). The impairment of reservoir function has been linked with the progression of AF burden in HFpEF. Combining RHC and echocardiographic evaluation in a cohort of 285 HFpEF patients, the presence of a reservoir function <31.5% and a compliance <5.7%/mmHg has been associated, respectively, with a hazard ratio (HR) of 6.8 and 6.0 for the progression toward worse AF stage (55). This finding supports a model of electro-mechanical coupling, expression of a remodeling process where reservoir and contractile function are influenced also by electrical properties.
Along with the remodeling process affecting LA in HFpEF, the occurrence of mitral regurgitation (MR) represents a further step associated with a greater hemodynamic severity and a poorer functional capacity. Interestingly, the presence of LA disfunction (defined as LA reservoir <24.5%) remains an independent predictor of HF or cardiovascular death, even after adjusting for age, gender, BMI, LV EF, and the presence of MR itself, confirming the prognostic importance of the chamber.
The assessment of rest LA reservoir function has been validated in the diagnostic workup of HFpEF. Ye et al. tested the predictive role of rest reservoir function in identifying abnormal exercise-induced LV filling pressure (as defined by 2016 AHA guidelines for diastolic evaluation) in a cohort of 669 subjects. The addition of LA reservoir function to the currently recommended diagnostic work-up improved the diagnostic accuracy (AUC from 0.71 to 0.80, p = 0.01) with a reported 28% higher odds of developing elevated exercise LV filling pressure per 1% of reservoir function decrease (213).
The interaction between LA function and exercise capacity represents another area of interest in all HF phenotypes. During physical effort, LA plays a major role in ensuring adequate and rapid LV filling. The abnormal rise of LV end-diastolic pressure during exercise, typical of both HFrEF and HFpEF, prevents the physiological emptying of LA, leading to a rise in atrial pressures during diastole. The interplay between atrial function and effort tolerance has been variably shown in all HF phenotypes, using different approaches, including standard echocardiography, myocardial deformation and radionuclide assessment (19, 214, 215). A large study on 486 subjects, symptomatic for chest pain or dyspnea, with preserved LV ejection fraction, explored the determinants of exercise capacity with echocardiography. LA reservoir function, E/e', age, male gender and BMI emerged as independent predictors of effort tolerance (19). A similar result has been recently reported in HFpEF patients of a German registry, where a LA reservoir <22% was able to predict impaired functional capacity after adjustment for common variables and log-NTproBNP (216). The reservoir function is not exclusively linked to functional capacity. Von Roeder et al. investigated the role of the different components of LA mechanics reporting a strong association between impaired conduit function and reduced early LV filling in HFpEF, by using a multimodality approach. The loss of conduit function limits the early LV filling and therefore the SV, one of the CO component, resulting in restricted peak VO2 during exercise (171).
The study of LA mechanics at rest may predict the exercise response in HF patients, as demonstrated in a cohort of 164 HF patients (56% with preserved EF) who underwent to rest and exercise RHC. LA reservoir function (with a threshold of 21 and 17% in HFrEF and HFpEF, respectively) predicted rest or exercise elevated pulmonary capillary wedge pressure (PCWP) with higher accuracy than recommended algorithm (AUC: 0.80 vs.69, p < 0.001) (52). The strong correlation between LA function and the degree of pulmonary congestion has been recently confirmed by Telles et al. (54) using simultaneous RHC and strain analysis in 49 HFpEF and 22 subjects with non-cardiac dyspnea. Reservoir and contractile LA function correlated with exercise PCWP, remaining independent predictors after adjustment for other variables, and showing a good diagnostic accuracy with a reservoir cut-off of 33%. LA reservoir function directly reflects the pulmonary hemodynamic status and the response to the unloading effect of diuretic therapy. Deferm et al. (7) showed a strong and rapid improvement of reservoir function during and after acute pulmonary decongestion in 31 acute HFrEF patients with invasive pressure monitoring and serial echocardiographic assessment. Remarkably, the contractile function slowly recovered during the observation period, suggesting the persistence of a great stunning condition predominantly affecting atrial contraction.
The use of stress test to explore the chamber reserve represents the emerging frontier of LA mechanics assessment. This approach can acquire a clinical relevance in specific context, such as the HFpEF diagnostic workup, where the diagnostic gold standard (invasive hemodynamic at rest and during exercise) requires an uncommon level of expertise, still not widely available (93, 166). The use of cardiovascular imaging in dynamic conditions—to test LA reserve—may provide a more effective recognition of pathological response than a rest-limited assessment. Obokata et al. (217) explored the use of passive leg lift in testing the LA reserve to discriminate HFpEF from hypertensive patients. They confirmed that LA dilatation in HFpEF occurs to maintain an adequate SV at rest. Nevertheless, during passive volume overload, HFpEF presents with a reduced reservoir and contractile function, provoking a blunted SV increase during exercise. Remarkably, the use of such a simple stressor, better discriminated HFpEF from hypertensive patients, showing additional diagnostic value compared to conventional parameters. The significance of LA mechanics during exercise has been reported in a large population of mixed HF patients who underwent exercise-echocardiography and cardiopulmonary exercise test (CPET). The study of atrial myocardial deformation during the early phase of exercise showed that peak SV, CO, and cardiac power output were all associated with a greater reservoir function reserve, triggered by exercise. As suggested by the data collected during rest evaluation, a deficient reservoir reserve during exercise affects the LV filling and the backward flow to pulmonary circulation leading to blunted CO and pulmonary circulation retrograde overload (218). Interestingly, the loss of LA reservoir reserve has been observed irrespectively from LV EF and other hemodynamic factors, being a marker for the occurrence of HF hospitalization and death. Finally, the presence of functional MR in HFrEF subjects was associated with a further reduction in exercise-related LA reservoir function, confirming the additional detrimental effect of volume overload leading to earlier dilatation and exhaustion of atrial function (144), as represented in Figure 7.
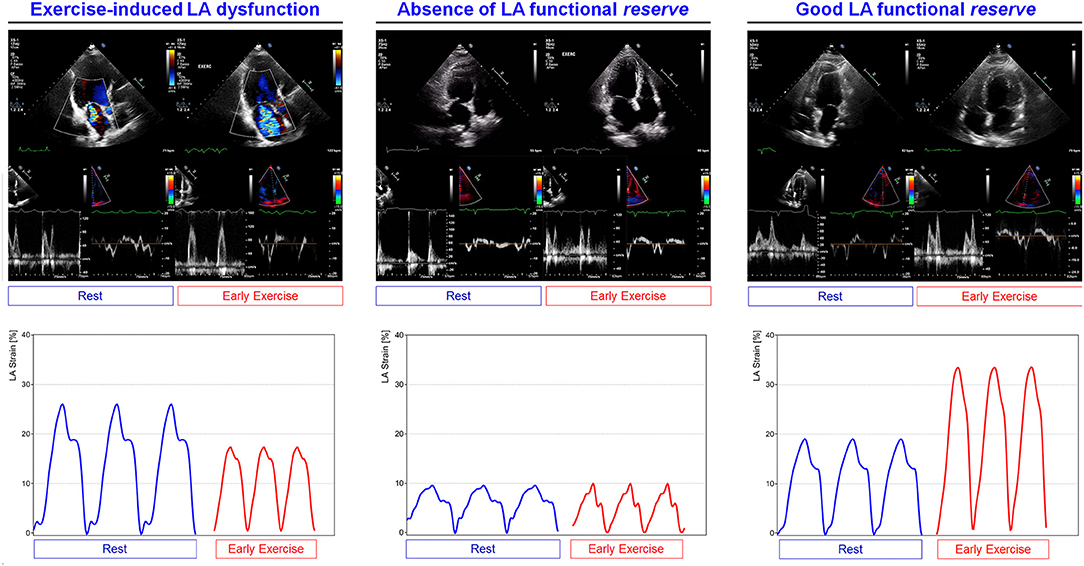
Figure 7. LA functional reserve assessed during exercise in HFrEF. Examples of rest-stress traces of patients with different severity of HFrEF. (left) Exercise-induced LA dysfunction; (middle) absence of LA functional reserve in severely impaired LA mechanics; (right) good LA functional reserve. Note that during early stage of exercise, LA mechanics can improve or reduce according with the presence of functional reserve, expression of multiple factors influencing LA function. In the first case on the left, the presence of exercise-induced mitral regurgitation produces additional volume overload responsible of further reservoir function worsening. Abbreviations as in the text.
The absence of LA reservoir function reserve, in both HFrEF and HFpEF, has been studied with respect to functional phenotypes and right ventricle-pulmonary circulation unit, using a combined exercise-echocardiography and CPET approach. Compared to control subjects, a limited or absent reservoir function reserve was observed in HFpEF and HFrEF, respectively, during early exercise phase. Remarkably, the exercise-induced LA reservoir function correlated with TAPSE/PAPS ratio, a marker of right ventricle-pulmonary circulation coupling, and with VE/VCO2, an index of ventilatory efficiency, in both types of HF syndrome (219).
Left atrium (LA) adaptation to abnormal pressure overload imposed by HF is a crucial determinant of hemodynamic and functional conditions. Reservoir function is a key parameter to address the global function of the chamber, the presence of functional reserve and to use in diagnostic workup and prognostic stratification.
Conclusions
Left atrium (LA) remodeling plays a central role in cardiac diseases due to the ability of the chamber in adapting to abnormal hemodynamic conditions, generated by the underlying disease, and to protect pulmonary circulation. The assessment of LA mechanics (especially reservoir function), with 2DSTE or FT-CMR, is very informative on the stage of disease progression and on the risk stratification. The evaluation under stress conditions, mainly during physical exercise, is a great potential for the additional insights on the LA functional reserve.
Author Contributions
FB, AM, MF, GG, and NV organized database and wrote the manuscript. All authors contributed to manuscript revision, read, and approved the submitted version.
Funding
The article has been funded by Gruppo Ospedaliero San Donato Foundation.
Conflict of Interest
The authors declare that the research was conducted in the absence of any commercial or financial relationships that could be construed as a potential conflict of interest.
Publisher's Note
All claims expressed in this article are solely those of the authors and do not necessarily represent those of their affiliated organizations, or those of the publisher, the editors and the reviewers. Any product that may be evaluated in this article, or claim that may be made by its manufacturer, is not guaranteed or endorsed by the publisher.
Supplementary Material
The Supplementary Material for this article can be found online at: https://www.frontiersin.org/articles/10.3389/fcvm.2021.750139/full#supplementary-material
References
1. Triposkiadis F, Pieske B, Butler J, Parissis J, Giamouzis G, Skoularigis J, et al. Global left atrial failure in heart failure. Eur J Heart Fail. (2016) 18:1307–20. doi: 10.1002/ejhf.645
2. Goette A, Kalman JM, Aguinaga L, Akar J, Cabrera JA, Chen SA, et al. EHRA/HRS/APHRS/SOLAECE expert consensus on atrial cardiomyopathies: Definition, characterization, and clinical implication. Hear Rhythm. (2017) 14:e3–e40. doi: 10.1016/j.hrthm.2016.05.028
3. Bisbal F, Baranchuk A, Braunwald E, Bayés de. Luna A, Bayés-Genís A. Atrial failure as a clinical entity: JACC review topic of the week. J Am Coll Cardiol. (2020) 75:222–32. doi: 10.1016/j.jacc.2019.11.013
4. Thomas L, Marwick TH, Popescu BA, Donal E, Badano LP. Left atrial structure and function, and left ventricular diastolic dysfunction: JACC state-of-the-art review. J Am Coll Cardiol. (2019) 73:1961–77. doi: 10.1016/j.jacc.2019.01.059
5. Vieira MJ, Teixeira R, Gonçalves L, Gersh BJ. Left atrial mechanics: Echocardiographic assessment and clinical implications. J Am Soc Echocardiogr. (2014) 27:463–78. doi: 10.1016/j.echo.2014.01.021
6. Yancy CW, Jessup M, Bozkurt B, Butler J, Casey DE, Colvin MM, et al. 2017 ACC/AHA/HFSA focused update of the 2013 ACCF/AHA guideline for the management of heart failure: A report of the American College of Cardiology/American Heart Association Task Force on Clinical Practice Guidelines and the Heart Failure Society of Amer. J Am Coll Cardiol. (2017) 70:776–803. doi: 10.1016/j.jacc.2017.04.025
7. Deferm S, Martens P, Verbrugge FH, Bertrand PB, Dauw J, Verhaert D, et al. Mechanics in decompensated heart failure: insights from strain echocardiography with invasive hemodynamics. JACC Cardiovasc Imaging. (2020) 13:1107–15. doi: 10.1016/j.jcmg.2019.12.008
8. Bandera F, Generati G, Pellegrino M, Donghi V, Alfonzetti E, Gaeta M, et al. Role of right ventricle and dynamic pulmonary hypertension on determining ΔVO2/Δwork rate flattening. Circ Hear Fail. (2014) 7:782–90. doi: 10.1161/CIRCHEARTFAILURE.113.001061
9. Bandera F, Baghdasaryan L, Mandoli GE, Cameli M. Multimodality imaging predictors of sudden cardiac death. Heart Fail Rev. (2020) 25:427–46. doi: 10.1007/s10741-019-09893-z
10. Guazzi M, Naeije R, Arena R, Corrà U, Ghio S, Forfia P, et al. Echocardiography of right ventriculoarterial coupling combined with cardiopulmonary exercise testing to predict outcome in heart failure. Chest. (2015) 148:226–34. doi: 10.1378/chest.14-2065
11. Lancellotti P, Pellikka PA, Budts W, Chaudhry FA, Donal E, Dulgheru R, et al. The clinical use of stress echocardiography in non-ischaemic heart disease: recommendations from the european association of cardiovascular imaging and the american society of echocardiography. J Am Soc Echocardiogr. (2017) 30:101–38. doi: 10.1016/j.echo.2016.10.016
12. Williams JF, Sonnenblick EH, Braunwald E. Determinants of atrial contractile force in the intact heart. Am J Physiol. (1965) 209:1061–8. doi: 10.1152/ajplegacy.1965.209.6.1061
13. Kilner PJ, Yang GZ, Wilkest AJ, Mohladdlin RH, Firmin DN, Yacoub MH. Asymmetric redirection of flow through the heart. Nature. (2000) 404:759–61. doi: 10.1038/35008075
14. Guazzi M, Bandera F, Ozemek C, Systrom D, Arena R. Cardiopulmonary exercise testing: what is its value? J Am Coll Cardiol. (2017) 70:1618–36. doi: 10.1016/j.jacc.2017.08.012
15. Bhatt A, Flink L, Lu DY, Fang Q, Bibby D, Schiller NB. Exercise physiology of the left atrium: Quantity and timing of contribution to cardiac output. Am J Physiol - Hear Circ Physiol. (2021) 320:H575–83. doi: 10.1152/AJPHEART.00402.2020
16. Wright SP, Dawkins TG, Eves ND, Shave R, Tedford RJ, Mak S. Hemodynamic function of the right ventricular-pulmonary vascular-left atrial unit: normal responses to exercise in healthy adults. Am J Physiol - Hear Circ Physiol. (2021) 320:H923–41. doi: 10.1152/AJPHEART.00720.2020
17. Guazzi M, Generati G, Borlaug B, Alfonzetti E, Sugimoto T, Castelvecchio S, et al. Redistribution of cardiac output during exercise by functional mitral regurgitation in heart failure: Compensatory O2 peripheral uptake to delivery failure. Am J Physiol - Hear Circ Physiol. (2020) 319:H100–8. doi: 10.1152/ajpheart.00125.2020
18. Roşca M, Lancellotti P, Popescu BA, Piérard LA. Left atrial function: Pathophysiology, echocardiographic assessment, and clinical applications. Heart. (2011) 97:1982–9. doi: 10.1136/heartjnl-2011-300069
19. Kusunose K, Motoki H, Popovic ZB, Thomas JD, Klein AL, Marwick TH. Independent association of left atrial function with exercise capacity in patients with preserved ejection fraction. Heart. (2012) 98:1311–7. doi: 10.1136/heartjnl-2012-302007
20. Leite L, Mendes SL, Baptista R, Teixeira R, Oliveira-Santos M, Ribeiro N, et al. Left atrial mechanics strongly predict functional capacity assessed by cardiopulmonary exercise testing in subjects without structural heart disease. Int J Cardiovasc Imaging. (2017) 33:635–42. doi: 10.1007/s10554-016-1045-3
21. Morris DA, Takeuchi M, Krisper M, Köhncke C, Bekfani T, Carstensen T, et al. Normal values and clinical relevance of left atrial myocardial function analysed by speckle-tracking echocardiography:multicentre study. Eur Heart J Cardiovasc Imaging. (2015) 16:364–72. doi: 10.1093/ehjci/jeu219
22. Sugimoto T, Robinet S, Dulgheru R, Bernard A, Ilardi F, Contu L, et al. Echocardiographic reference ranges for normal left atrial function parameters: Results from the EACVI NORRE study. Eur Heart J Cardiovasc Imaging. (2018) 19:630–8. doi: 10.1093/ehjci/jey018
23. Liao JN, Chao TF, Kuo JY, Sung KT, Tsai JP, Lo CI, et al. Age, sex, and blood pressure-related influences on reference values of left atrial deformation and mechanics from a large-scale asian population. Circ Cardiovasc Imaging. (2017) 10:1–16. doi: 10.1161/CIRCIMAGING.116.006077
24. Nielsen AB, Skaarup KG, Hauser R, Johansen ND, Lassen MCH, Jensen GB, Schnohr P, Møgelvang R, Biering-Sørensen T. Normal values and reference ranges for left atrial strain by speckle-tracking echocardiography: the Copenhagen City Heart Study. Eur Hear J Cardiovasc Imaging. (2021) 1–10. doi: 10.1093/ehjci/jeab201
25. Sun BJ, Park JH, Lee M, Choi JO, Lee JH, Shin MS, et al. Normal reference values for left atrial strain and its determinants from a large Korean multicenter registry. J Cardiovasc Imaging. (2020) 28:186–98. doi: 10.4250/jcvi.2020.0043
26. Truong VT, Palmer C, Wolking S, Sheets B, Young M, Ngo TNM, et al. Normal left atrial strain and strain rate using cardiac magnetic resonance feature tracking in healthy volunteers. Eur Heart J Cardiovasc Imaging. (2020) 21:446–53. doi: 10.1093/ehjci/jez157
27. Paraskevaidis IA, Farmakis D, Papadopoulos C, Ikonomidis I, Parissis J, Rigopoulos A, et al. Two-dimensional strain analysis in patients with hypertrophic cardiomyopathy and normal systolic function: A 12-month follow-up study. Am Heart J. (2009) 158:444–50. doi: 10.1016/j.ahj.2009.06.013
28. Roca M, Popescu BA, Beladan CC, Clin A, Muraru D, Popa EC, et al. Left atrial dysfunction as a correlate of heart failure symptoms in hypertrophic cardiomyopathy. J Am Soc Echocardiogr. (2010) 23:1090–8. doi: 10.1016/j.echo.2010.07.016
29. Debonnaire P, Leong DP, Witkowski TG, Al Amri I, Joyce E, Katsanos S, et al. Left atrial function by two-dimensional speckle-tracking echocardiography in patients with severe organic mitral regurgitation: Association with guidelines-based surgical indication and postoperative (long-term) survival. J Am Soc Echocardiogr. (2013) 26:1053–62. doi: 10.1016/j.echo.2013.05.019
30. Ancona R, Comenale Pinto S, Caso P, Di Salvo G, Severino S, D'Andrea A, et al. Two-dimensional atrial systolic strain imaging predicts atrial fibrillation at 4-year follow-up in asymptomatic rheumatic mitral stenosis. J Am Soc Echocardiogr. (2013) 26:270–7. doi: 10.1016/j.echo.2012.11.016
31. Zito C, Manganaro R, Khandheria B, Oreto G, Cusmà-Piccione M, Todaro MC, et al. Usefulness of left atrial reservoir size and left ventricular untwisting rate for predicting outcome in primary mitral regurgitation. Am J Cardiol. (2015) 116:1237–44. doi: 10.1016/j.amjcard.2015.07.038
32. Yang LT, Liu YW, Shih JY, Li YH, Tsai LM, Luo CY, et al. Predictive value of left atrial deformation on prognosis in severe primary mitral regurgitation. J Am Soc Echocardiogr. (2015) 28:1309–17.e4. doi: 10.1016/j.echo.2015.07.004
33. Imanishi J, Tanaka H, Sawa T, Motoji Y, Miyoshi T, Mochizuki Y, et al. Association of left atrial booster-pump function with heart failure symptoms in patients with severe aortic stenosis and preserved left ventricular ejection fraction. Echocardiography. (2015) 32:758–67. doi: 10.1111/echo.12733
34. Todaro MC, Carerj S, Khandheria B, Cusmà-Piccione M, La Carrubba S, Antonini-Canterin F, et al. Usefulness of atrial function for risk stratification in asymptomatic severe aortic stenosis. J Cardiol. (2016) 67:71–9. doi: 10.1016/j.jjcc.2015.04.010
35. Galli E, Fournet M, Chabanne C, Lelong B, Leguerrier A, Flecher E, et al. Prognostic value of left atrial reservoir function in patients with severe aortic stenosis: A 2D speckle-tracking echocardiographic study. Eur Heart J Cardiovasc Imaging. (2016) 17:533–41. doi: 10.1093/ehjci/jev230
36. Kamijima R, Suzuki K, Izumo M, Kuwata S, Mizukoshi K, Takai M, et al. Predictors of exercise-induced pulmonary hypertension in patients with asymptomatic degenerative mitral regurgitation: mechanistic insights from 2D speckle-tracking echocardiography. Sci Rep. (2017) 7:1–11. doi: 10.1038/srep40008
37. Modin D, Biering-Sørensen SR, Møgelvang R, Alhakak AS, Jensen JS, Biering-Sørensen T. Prognostic value of left atrial strain in predicting cardiovascular morbidity and mortality in the general population. Eur Heart J Cardiovasc Imaging. (2019) 20:804–15. doi: 10.1093/ehjci/jey181
38. Ring L, Abu-Omar Y, Kaye N, Rana BS, Watson W, Dutka DP, et al. Left atrial function is associated with earlier need for cardiac surgery in moderate to severe mitral regurgitation: usefulness in targeting for early surgery. J Am Soc Echocardiogr. (2018) 31:983–91. doi: 10.1016/j.echo.2018.03.011
39. Mohty D, Petitalot V, Magne J, Fadel BM, Boulogne C, Rouabhia D, et al. Left atrial function in patients with light chain amyloidosis: A transthoracic 3D speckle tracking imaging study. J Cardiol. (2018) 71:419–27. doi: 10.1016/j.jjcc.2017.10.007
40. Morris DA, Belyavskiy E, Aravind-Kumar R, Kropf M, Frydas A, Braunauer K, et al. Potential usefulness and clinical relevance of adding left atrial strain to left atrial volume index in the detection of left ventricular diastolic dysfunction. JACC Cardiovasc Imaging. (2018) 11:1405–15. doi: 10.1016/j.jcmg.2017.07.029
41. Kobayashi Y, Moneghetti KJ, Bouajila S, Stolfo D, Finocchiaro G, Kuznetsova T, et al. Time based vs. strain basedmyocardial performance indices in hypertrophic cardiomyopathy, themerging role of left atrial strain. Eur Heart J Cardiovasc Imaging. (2019) 20:334–42. doi: 10.1093/ehjci/jey097
42. Vasquez N, Ostrander BT, Lu DY, Ventoulis I, Haileselassie B, Goyal S, et al. Low left atrial strain is associated with adverse outcomes in hypertrophic cardiomyopathy patients. J Am Soc Echocardiogr. (2019) 32:593–603.e1. doi: 10.1016/j.echo.2019.01.007
43. Cameli M, Pastore MC, Righini FM, Mandoli GE, D'Ascenzi F, Lisi M, et al. Prognostic value of left atrial strain in patients with moderate asymptomatic mitral regurgitation. Int J Cardiovasc Imaging. (2019) 35:1597–604. doi: 10.1007/s10554-019-01598-6
44. Mateescu AD, Călin A, Beladan CC, Roşca M, Enache R, Băicuş C, et al. Left atrial dysfunction as an independent correlate of heart failure symptoms in patients with severe aortic stenosis and preserved left ventricular ejection fraction. J Am Soc Echocardiogr. (2019) 32:257–66. doi: 10.1016/j.echo.2018.11.007
45. Potter EL, Ramkumar S, Kawakami H, Yang H, Wright L, Negishi T, et al. Association of asymptomatic diastolic dysfunction assessed by left atrial strain with incident heart failure. JACC Cardiovasc Imaging. (2020) 13:2316–26. doi: 10.1016/j.jcmg.2020.04.028
46. Mahfouz RA, Gouda M, Abdelhamed M. Relation between left atrial strain and exercise tolerance in patients with mild mitral stenosis: An insight from 2D speckle-tracking echocardiography. Echocardiography. (2020) 37:1406–12. doi: 10.1111/echo.14818
47. Yang F, Wang L, Wang J, Pu L, Xu Y, Li W, et al. Prognostic value of fast semi-automated left atrial long-axis strain analysis in hypertrophic cardiomyopathy. J Cardiovasc Magn Reson. (2021) 23:1–12. doi: 10.1186/s12968-021-00735-2
48. Huntjens PR, Zhang KW, Soyama Y, Karmpalioti M, Lenihan DJ, Gorcsan J. Prognostic utility of echocardiographic atrial and ventricular strain imaging in patients with cardiac amyloidosis. JACC Cardiovasc Imaging. (2021) 1–12. doi: 10.1016/j.jcmg.2021.01.016
49. Bandera F, Martone R, Chacko L, Ganesananthan S, Gilbertson JA, Ponticos M, et al. Clinical importance of left-atrial infiltration in cardiac transthyretin amyloidosis. JACC Cardiovasc Imaging. S1936-878X(21)00512-X. doi: 10.1016/j.jcmg.2021.06.022
50. Mandoli GE, Pastore MC, Benfari G, Bisleri G, Maccherini M, Lisi G, et al. Left atrial strain as a pre-operative prognostic marker for patients with severe mitral regurgitation. Int J Cardiol. (2021) 324:139–45. doi: 10.1016/j.ijcard.2020.09.009
51. Carluccio E, Biagioli P, Mengoni A, Francesca Cerasa M, Lauciello R, Zuchi C, et al. Left atrial reservoir function and outcome in heart failure with reduced ejection fraction. Circ Cardiovasc Imaging. (2018) 11:e007696. doi: 10.1161/CIRCIMAGING.118.007696
52. Lundberg A, Johnson J, Hage C, Bäck M, Merkely B, Venkateshvaran A, et al. Left atrial strain improves estimation of filling pressures in heart failure: a simultaneous echocardiographic and invasive haemodynamic study. Clin Res Cardiol. (2019) 108:703–15. doi: 10.1007/s00392-018-1399-8
53. Reddy YNV, Obokata M, Egbe A, Yang JH, Pislaru S, Lin G, et al. Left atrial strain and compliance in the diagnostic evaluation of heart failure with preserved ejection fraction. Eur J Heart Fail. (2019) 21:891–900. doi: 10.1002/ejhf.1464
54. Telles F, Nanayakkara S, Evans S, Patel HC, Mariani JA, Vizi D, et al. Impaired left atrial strain predicts abnormal exercise haemodynamics in heart failure with preserved ejection fraction. Eur J Heart Fail. (2019) 21:495–505. doi: 10.1002/ejhf.1399
55. Reddy YNV, Obokata M, Verbrugge FH, Lin G, Borlaug BA. Atrial dysfunction in patients with heart failure with preserved ejection fraction and atrial fibrillation. J Am Coll Cardiol. (2020) 76:1051–64. doi: 10.1016/j.jacc.2020.07.009
56. Badano LP, Kolias TJ, Muraru D, Abraham TP, Aurigemma G, Edvardsen T, et al. Standardization of left atrial, right ventricular, and right atrial deformation imaging using two-dimensional speckle tracking echocardiography: A consensus document of the EACVI/ASE/Industry Task Force to standardize deformation imaging. Eur Heart J Cardiovasc Imaging. (2018) 19:591–600. doi: 10.1093/ehjci/jey042
57. Lang RM, Bierig M, Devereux RB, Flachskampf FA, Foster E, Pellikka PA, et al. Recommendations for chamber quantification: A report from the American Society of Echocardiography's guidelines and standards committee and the Chamber Quantification Writing Group, developed in conjunction with the European Association of Echocardiograph. J Am Soc Echocardiogr. (2005) 18:1440–63. doi: 10.1016/j.echo.2005.10.005
58. Lang RM, Badano LP, Victor MA, Afilalo J, Armstrong A, Ernande L, et al. Recommendations for cardiac chamber quantification by echocardiography in adults: An update from the American Society of Echocardiography and the European Association of Cardiovascular Imaging. J Am Soc Echocardiog.r. (2015) 28:1–39.e14. doi: 10.1016/j.echo.2014.10.003
59. Singh A, Singulane CS, Miyoshi T, Prado AD, Addetia K, Bellino M, et al. Normal values of left atrial size and function and the impact of age: results of the world alliance societies of echocardiography study. J Am Soc Echocardiogr. (2021). doi: 10.1016/j.echo.2021.08.008. [Epub ahead of print].
60. Nikitin NP, Witte KKA, Thackray SDR, Goodge LJ, Clark AL, Cleland JGF. Effect of age and sex on left atrial morphology and function. Eur J Echocardiogr. (2003) 4:36–42. doi: 10.1053/euje.2002.0611
61. Badano LP, Miglioranza MH, MihAilA S, Peluso D, Xhaxho J, Marra MP, et al. Left atrial volumes and function by three-dimensional echocardiography: Reference values, accuracy, reproducibility, and comparison with two-dimensional echocardiographic measurements. Circ Cardiovasc Imaging. (2016) 9:1–12. doi: 10.1161/CIRCIMAGING.115.004229
62. Surkova E, Badano LP, Genovese D, Cavalli G, Lanera C, Bidviene J, et al. Clinical and prognostic implications of methods and partition values used to assess left atrial volume by two-dimensional echocardiography. J Am Soc Echocardiogr. (2017) 30:1119–29. doi: 10.1016/j.echo.2017.07.015
63. Genovese D, Muraru D, Marra MP, Carrer A, Previtero M, Palermo C, Tarantini G, et al. Left atrial expansion index for noninvasive estimation of pulmonary capillary wedge pressure: a cardiac catheterization validation study. J Am Soc Echocardiogr. (2021) 1–11. doi: 10.1016/j.echo.2021.07.009
64. Blume GG, McLeod CJ, Barnes ME, Seward JB, Pellikka PA, Bastiansen PM, et al. Left atrial function: Physiology, assessment, and clinical implications. Eur J Echocardiogr. (2011) 12:421–30. doi: 10.1093/ejechocard/jeq175
65. Welles CC, Ku IA, Kwan DM, Whooley MA, Schiller NB, Turakhia MP. Left atrial function predicts heart failure hospitalization in subjects with preserved ejection fraction and coronary heart disease: Longitudinal data from the heart and soul study. J Am Coll Cardiol. (2012) 59:673–80. doi: 10.1016/j.jacc.2011.11.012
66. Sirbu C, Herbots L, D'hooge J, Claus P, Marciniak A, Langeland T, et al. Feasibility of strain and strain rate imaging for the assessment of regional left atrial deformation: A study in normal subjects. Eur J Echocardiogr. (2006) 7:199–208. doi: 10.1016/j.euje.2005.06.001
67. Cameli M, Sciaccaluga C, Mandoli GE, D'Ascenzi F, Tsioulpas C, Mondillo S. The role of the left atrial function in the surgical management of aortic and mitral valve disease. Echocardiography. (2019) 36:1559–65. doi: 10.1111/echo.14426
68. Cameli M, Miglioranza MH, Magne J, Mandoli GE, Benfari G, Ancona R, et al. Multicentric atrial strain COmparison between two different modalities: MASCOT HIT study. Diagnostics. (2020) 10:946. doi: 10.3390/diagnostics10110946
69. Mouselimis D, Tsarouchas AS, Pagourelias ED, Bakogiannis C, Theofilogiannakos EK, Loutradis C, et al. Left atrial strain, intervendor variability, and atrial fibrillation recurrence after catheter ablation: A systematic review and meta-analysis. Hell J Cardiol. (2020) 61:154–64. doi: 10.1016/j.hjc.2020.04.008
70. Jenkins C, Bricknell K, Marwick TH. Use of real-time three-dimensional echocardiography to measure left atrial volume: Comparison with other echocardiographic techniques. J Am Soc Echocardiogr. (2005) 18:991–7. doi: 10.1016/j.echo.2005.03.027
71. Artang R, Migrino RQ, Harmann L, Bowers M, Woods TD. Left atrial volume measurement with automated border detection by 3-dimensional echocardiography: Comparison with magnetic resonance imaging. Cardiovasc Ultrasound. (2009) 7:1–8. doi: 10.1186/1476-7120-7-16
72. Mor-Avi V, Yodwut C, Jenkins C, Khl H, Nesser HJ, Marwick TH, et al. Real-time 3D echocardiographic quantification of left atrial volume: Multicenter study for validation with CMR. JACC Cardiovasc Imaging. (2012) 5:769–77. doi: 10.1016/j.jcmg.2012.05.011
73. Aune E, Baekkevar M, Roislien J, Rodevand O, Otterstad JE. Normal reference ranges for left and right atrial volume indexes and ejection fractions obtained with real-time three-dimensional echocardiography. Eur J Echocardiogr. (2009) 10:738–44. doi: 10.1093/ejechocard/jep054
74. Mochizuki A, Yuda S, Oi Y, Kawamukai M, Nishida J, Kouzu H, et al. Assessment of left atrial deformation and synchrony by three-dimensional speckle-tracking echocardiography: Comparative studies in healthy subjects and patients with atrial fibrillation. J Am Soc Echocardiogr. (2013) 26:165–74. doi: 10.1016/j.echo.2012.10.003
75. Nemes A, Kormányos Á, Domsik P, Kalapos A, Lengyel C, Forster T. Normal reference values of three-dimensional speckle-tracking echocardiography-derived left atrial strain parameters (results from the MAGYAR-Healthy Study). Int J Cardiovasc Imaging. (2019) 35:991–8. doi: 10.1007/s10554-019-01559-z
76. Nabeshima Y, Kitano T, Takeuchi M. Reliability of left atrial strain reference values: A 3D echocardiographic study. PLoS ONE. (2021) 16:1–18. doi: 10.1371/journal.pone.0250089
77. Nemes A, Piros GÁ, Lengyel C, Domsik P, Kalapos A, Várkonyi TT, et al. Complex evaluation of left atrial dysfunction in patients with type 1 diabetes mellitus by three-dimensional speckle tracking echocardiography: Results from the MAGYAR-path study. Anatol J Cardiol. (2016) 16:587–93. doi: 10.5152/AnatolJCardiol.2015.6225
78. Domsik P, Kalapos A, Chadaide S, Sepp R, Hausinger P, Forster T, et al. Three-dimensional speckle tracking echocardiography allows detailed evaluation of left atrial function in hypertrophic cardiomyopathy-insights from the MAGYAR-Path Study. Echocardiography. (2014) 31:1245–52. doi: 10.1111/echo.12568
79. Furukawa A, Ishii K, Hyodo E, Shibamoto M, Komasa A, Nagai T, et al. Three-dimensional speckle tracking imaging for assessing left atrial function in hypertensive patients with paroxysmal atrial fibrillation. Int Heart J. (2016) 57:705–11. doi: 10.1536/ihj.16-121
80. Dogdus M, Yenercag M, Akhan O, Gok G. Assessment of left atrial mechanics and left ventricular functions using 3D speckle-tracking echocardiography in patients with inappropriate sinus tachycardia. Int J Cardiovasc Imaging. (2020) 36:1489–95. doi: 10.1007/s10554-020-01858-w
81. Pellikka PA, Arruda-Olson A, Chaudhry FA, Chen MH, Marshall JE, Porter TR, et al. Guidelines for Performance, Interpretation, and Application of Stress Echocardiography in Ischemic Heart Disease: From the American Society of Echocardiography. J Am Soc Echocardiogr. (2020) 33:1–41.e8. doi: 10.1016/j.echo.2019.07.001
82. Pellikka PA, Nagueh SF, Elhendy AA, Kuehl CA, Sawada SG. American society of echocardiography recommendations for performance, interpretation, and application of stress echocardiography. J Am Soc Echocardiogr. (2007) 20:1021–41. doi: 10.1016/j.echo.2007.07.003
83. Sicari R, Nihoyannopoulos P, Evangelista A, Kasprzak J, Lancellotti P, Poldermans D, et al. Stress echocardiography expert consensus statement. Eur J Echocardiogr. (2008) 9:415–37. doi: 10.1093/ejechocard/jen175
84. Zertuche DR, Arbucci R, Sevilla D, Rousse G, Lowenstein D, Israel MR, et al. The reservoir function functional evaluation of the left atrium by two-dimensional strain during rest and exercise stress. Rev Argent Cardiol. (2017) 85:498–504. doi: 10.7775/rac.v85.i6.11833
85. Pieske B, Tschöpe C, De Boer RA, Fraser AG, Anker SD, Donal E, et al. How to diagnose heart failure with preserved ejection fraction: The HFA-PEFF diagnostic algorithm: A consensus recommendation from the Heart Failure Association (HFA) of the European Society of Cardiology (ESC). Eur Heart J. (2019) 40:3297–317. doi: 10.1093/eurheartj/ehz641
86. Guglielmo M, Baggiano A, Muscogiuri G, Fusini L, Andreini D, Mushtaq S, et al. Multimodality imaging of left atrium in patients with atrial fibrillation. J Cardiovasc Comput Tomogr. (2019) 13:340–6. doi: 10.1016/j.jcct.2019.03.005
87. Panovsky R, Pleva M, Feitova V, Kruzliak P, Meluzin J, Kincl V, et al. Left atrium assessment: The evolving role of MRI. J Cardiovasc Med. (2015) 16:671–80. doi: 10.2459/JCM.0000000000000155
88. Siebermair J, Kholmovski EG, Marrouche N. Assessment of left atrial fibrosis by late gadolinium enhancement magnetic resonance imaging: methodology and clinical implications. JACC Clin Electrophysiol. (2017) 3:791–802. doi: 10.1016/j.jacep.2017.07.004
89. Marrouche NF, Wilber D, Hindricks G, Jais P, Akoum N, Marchlinski F, et al. Association of atrial tissue fibrosis identified by delayed enhancement MRI and atrial fibrillation catheter ablation: The DECAAF study. JAMA - J Am Med Assoc. (2014) 311:498–506. doi: 10.1001/jama.2014.3
90. Mahnkopf C, Badger TJ, Burgon NS, Daccarett M, Haslam TS, Badger CT, et al. Evaluation of the left atrial substrate in patients with lone atrial fibrillation using delayed-enhanced MRI: Implications for disease progression and response to catheter ablation. Hear Rhythm. (2010) 7:1475–81. doi: 10.1016/j.hrthm.2010.06.030
91. Evin M, Cluzel P, Lamy J, Rosenbaum D, Kusmia S, Defrance C, et al. Assessment of left atrial function by MRI myocardial feature tracking. J Magn Reson Imaging. (2015) 42:379–89. doi: 10.1002/jmri.24851
92. Evin M, Redheuil A, Soulat G, Perdrix L, Ashrafpoor G, Giron A, et al. Left atrial aging: A cardiac magnetic resonance feature-tracking study. Am J Physiol - Hear Circ Physiol. (2016) 310:H542–9. doi: 10.1152/ajpheart.00504.2015
93. Nishimura RA, Otto CM, Bonow RO, Carabello BA, Erwin JP, Guyton RA, et al. 2014 AHA/ACC guideline for the management of patients with valvular heart disease: Executive summary :A report of the American college of cardiology/American heart association task force on practice guidelines. Diagnostics (Basel). (2014) 10:946. doi: 10.1161/CIR.0000000000000029
94. Yancy CW, Jessup M, Bozkurt B, Butler J, Casey DE, Drazner MH, et al. ACCF / AHA Practice Guideline 2013 ACCF / AHA Guideline for the Management of Heart Failure A Report of the American College of Cardiology Foundation / American Heart Association Task Force on Practice Guidelines. J Am Coll Cardiol. (2013) 240–327. doi: 10.1161/CIR.0b013e31829e8776
95. Bozkurt B, Aguilar D, Deswal A, Dunbar SB, Francis GS, Horwich T, et al. Contributory risk and management of comorbidities of hypertension, obesity, diabetes mellitus, hyperlipidemia, and metabolic syndrome in chronic heart failure: a scientific statement from the American Heart Association. Circulation. (2016) 134:e535–e578. doi: 10.1161/CIR.0000000000000450
96. Whelton PK, Carey RM, Aronow WS, Casey DE, Collins KJ, Himmelfarb CD, et al. 2017 ACC/AHA/AAPA/ABC/ACPM/AGS/APhA/ ASH/ASPC/NMA/PCNA guideline for the prevention, detection, evaluation, and management of high blood pressure in adults a report of the American College of Cardiology/American Heart Association Task Force on Clinical pr. Hypertension. (2018) 71:1269–324. doi: 10.1161/HYP.0000000000000065
97. Vahanian A, Beyersdorf F, Praz F, Milojevic M, Baldus S, Bauersachs J, et al. 2021 ESC/EACTS Guidelines for the management of valvular heart disease. Eur Heart J. (2021) 1–73. doi: 10.1093/eurheartj/ehab395
98. Ommen SR, Mital S, Burke MA, Day SM, Deswal A, Elliott P, et al. 2020 AHA/ACC guideline for the diagnosis and treatment of patients with hypertrophic cardiomyopathy. Circulation. (2020) 142:e558–e631. doi: 10.1161/cir.0000000000000937
99. McDonagh TA, Metra M, Adamo M, Gardner RS, Baumbach A, Böhm M, et al. 2021 ESC Guidelines for the diagnosis and treatment of acute and chronic heart failure. Eur Heart J. (2021) 42:3599–726. doi: 10.1093/eurheartj/ehab368
100. Mancia G, De Backer G, Dominiczak A, Cifkova R, Fagard R, Germano G, et al. 2007 Guidelines for the management of arterial hypertension: the task force for the management of arterial hypertension of the European Society of Hypertension (ESH) and of the European Society of Cardiology (ESC). J Hypertens. (2007) 25:1105–87. doi: 10.1097/HJH.0b013e3281fc975a
101. Hindricks G, Potpara T, Dagres N, Bax JJ, Boriani G, Dan GA, et al. 2020 ESC Guidelines for the diagnosis and management of atrial fibrillation developed in collaboration with the European Association for Cardio-Thoracic Surgery (EACTS). Eur Heart J. (2021) 42:373–498. doi: 10.1093/eurheartj/ehaa612
102. Elliott PM, Uk C, Anastasakis A, Germany MAB, Germany MB, Cecchi F, et al. 2014 ESC Guidelines on diagnosis and management of hypertrophic cardiomyopathy The Task Force for the Diagnosis and Management of Hypertrophic Cardiomyopathy of the European Society of Cardiology (ESC). Eur Heart J. (2014) 2733–79. doi: 10.1093/eurheartj/ehu284
103. Dorbala S, Ando Y, Bokhari S, Dispenzieri A, Falk RH, Ferrari VA, et al. ASNC / AHA / ASE / EANM / HFSA / ISA / SCMR / SNMMI Expert Consensus Recommendations for Multimodality Imaging in Cardiac Amyloidosis. Circ Cardiovasc Imaging. (2021) 685–725. doi: 10.1161/HCI.0000000000000029
104. Cardim N, Galderisi M, Edvardsen T, Plein S, Popescu BA, D'Andrea A, et al. Role of multimodality cardiac imaging in the management of patients with hypertrophic cardiomyopathy: An expert consensus of the European Association of Cardiovascular Imaging Endorsed by the Saudi Heart Association. Eur Heart J Cardiovasc Imaging. (2015) 16:280–280hh. doi: 10.1093/ehjci/jeu291
105. Celutkiene J, Plymen CM, Flachskampf FA, de Boer RA, Grapsa J, Manka R, et al. Innovative imaging methods in heart failure: a shifting paradigm in cardiac assessment. Position statement on behalf of the Heart Failure Association of the European Society of Cardiology. Eur J Heart Fail. (2018) 20:1615–33. doi: 10.1002/ejhf.1330
106. Galderisi M, Cosyns B, Edvardsen T, Cardim N, Delgado V, Di Salvo G, et al. Standardization of adult transthoracic echocardiography reporting in agreement with recent chamber quantification, diastolic function, and heart valve disease recommendations: An expert consensus document of the European Association of Cardiovascular Imag. Eur Heart J Cardiovasc Imaging. (2017) 18:1301–10. doi: 10.1093/ehjci/jex244
107. Nagueh SF, Smiseth OA, Appleton CP, Byrd BF, Dokainish H, Edvardsen T, et al. Recommendations for the evaluation of left ventricular diastolic function by echocardiography: an update from the American Society of Echocardiography and the European Association of Cardiovascular Imaging. J Am Soc Echocardiogr. (2016) 29:277–314. doi: 10.1016/j.echo.2016.01.011
108. Smiseth OA, Morris DA, Cardim N, Cikes M, Delgado V, Donal E, et al. Multimodality imaging in patients with heart failure and preserved ejection fraction: an expert consensus document of the European Association of Cardiovascular Imaging. Eur Hear J Cardiovasc Imaging. (2021) 1–28. doi: 10.1093/ehjci/jeab154
109. Inoue K, Khan FH, Remme EW, Ohte N, García-Izquierdo E, Chetrit M, et al. Determinants of left atrial reservoir and pump strain and use of atrial strain for evaluation of left ventricular filling pressure. Eur Hear J Cardiovasc Imaging. (2021) 1–10. doi: 10.1093/ehjci/jeaa415
110. Hunt SA, Abraham WT, Chin MH, Feldman AM, Francis GS, Ganiats TG, et al. ACCF / AHA Practice Guideline : Full Text Practice Guideline : Full Text 2009 Focused Update Incorporated Into the ACC / AHA 2005 Guidelines for the Diagnosis and Management of Heart Failure in Adults A Report of the American College of Cardiology Foundatation/American Heart Association Task Force on Practice Guidelines: developed in collaboration with the International Society for Heart and Lung Transplantation. Circulation. (2014) 119:e391–479. doi: 10.1161/CIRCULATIONAHA.109.192065
111. Mondillo S, Cameli M, Caputo ML, Lisi M, Palmerini E, Padeletti M, et al. Early detection of left atrial strain abnormalities by speckle-tracking in hypertensive and diabetic patients with normal left atrial size. J Am Soc Echocardiogr. (2011) 24:898–908. doi: 10.1016/j.echo.2011.04.014
112. Fung MJ, Thomas L, Leung DY. Left atrial function: Correlation with left ventricular function and contractile reserve in patients with hypertension. Echocardiography. (2018) 35:1596–605. doi: 10.1111/echo.14051
113. Gan GCH, Bhat A, Chen HHL, Fernandez F, Byth K, Eshoo S, et al. Determinants of LA reservoir strain: Independent effects of LA volume and LV global longitudinal strain. Echocardiography. (2020) 37:2018–28. doi: 10.1111/echo.14922
114. Ramkumar S, Yang H, Wang Y, Nolan M, Negishi T, Negishi K, et al. Association of the active and passive components of left atrial deformation with left ventricular function. J Am Soc Echocardiogr. (2017) 30:659–66. doi: 10.1016/j.echo.2017.03.014
115. Kadappu KK, Boyd A, Eshoo S, Haluska B, Yeo AET, Marwick TH, et al. Changes in left atrial volume in diabetes mellitus: More than diastolic dysfunction? Eur Heart J Cardiovasc Imaging. (2012) 13:1016–23. doi: 10.1093/ehjci/jes084
116. Cameli M, Lisi M, Focardi M, Reccia R, Natali BM, Sparla S, et al. Left atrial deformation analysis by speckle tracking echocardiography for prediction of cardiovascular outcomes. Am J Cardiol. (2012) 110:264–9. doi: 10.1016/j.amjcard.2012.03.022
117. Kadappu KK, Abhayaratna K, Boyd A, French JK, Xuan W, Abhayaratna W, et al. Independent echocardiographic markers of cardiovascular involvement in chronic kidney disease: the value of left atrial function and volume. J Am Soc Echocardiogr. (2016) 29:359–67. doi: 10.1016/j.echo.2015.11.019
118. Gan GCH, Kadappu KK, Bhat A, Fernandez F, Gu KH, Cai L, et al. Left atrial strain is the best predictor of adverse cardiovascular outcomes in patients with chronic kidney disease. J Am Soc Echocardiogr. (2021) 34:166–75. doi: 10.1016/j.echo.2020.09.015
119. Bozkurt B, Hershberger RE, Butler J, Grady KL, Heidenreich PA, Isler ML, et al. 2021 ACC/AHA key data elements and definitions for heart failure: a report of the American College of Cardiology/American Heart Association task force on clinical data standards (writing committee to develop clinical data standards for heart failure). Circ Cardiovasc Qual Outcomes. (2021) 14:e000102. doi: 10.1161/HCQ.0000000000000102
120. Geuzebroek GSC, Van Amersfoorth SCM, Hoogendijk MG, Kelder JC, Van Hemel NM, De Bakker JMT, et al. Increased amount of atrial fibrosis in patients with atrial fibrillation secondary to mitral valve disease. J Thorac Cardiovasc Surg. (2012) 144:327–33. doi: 10.1016/j.jtcvs.2011.09.022
121. Zhang D, Li B, Li B, Tang Y. Regulation of left atrial fibrosis induced by mitral regurgitation by SIRT1. Sci Rep. (2020) 10:1–9. doi: 10.1038/s41598-020-64308-6
122. Chen MC, Chang JP, Chang TH, Hsu S Da, Huang H Da, Ho WC, et al. Unraveling regulatory mechanisms of atrial remodeling of mitral regurgitation pigs by gene expression profiling analysis: Role of type i angiotensin II receptor antagonist. Transl Res. (2015) 165:599–620. doi: 10.1016/j.trsl.2014.11.005
123. Butts B, Ahmed MI, Bajaj NS, Cox Powell P, Pat B, Litovsky S, et al. Reduced left atrial emptying fraction and chymase activation in pathophysiology of primary mitral regurgitation. JACC Basic to Transl Sci. (2020) 5:109–22. doi: 10.1016/j.jacbts.2019.11.006
124. Cameli M, Lisi M, Righini FM, Massoni A, Natali BM, Focardi M, et al. Usefulness of atrial deformation analysis to predict left atrial fibrosis and endocardial thickness in patients undergoing mitral valve operations for severe mitral regurgitation secondary to mitral valve prolapse. Am J Cardiol. (2013) 111:595–601. doi: 10.1016/j.amjcard.2012.10.049
125. Huber AT, Lamy J, Rahhal A, Evin M, Atassi F, Defrance C, et al. Cardiac MR strain: A noninvasive biomarker of fibrofatty remodeling of the left atrial myocardium. Radiology. (2018) 286:83–92. doi: 10.1148/radiol.2017162787
126. Kihara Y, Sasayama S, Miyazaki S, Onodera T, Susawa T, Nakamura Y, et al. Role of the left atrium in adaptation of the heart to chronic mitral regurgitation in conscious dogs. Circ Res. (1988) 62:543–53. doi: 10.1161/01.RES.62.3.543
127. Inciardi RM, Rossi A, Bergamini C, Benfari G, Maffeis C, Greco C, et al. Mitral regurgitation, left atrial structural and functional remodelling and the effect on pulmonary haemodynamics. Eur J Heart Fail. (2020) 22:499–506. doi: 10.1002/ejhf.1677
128. Ren B. de Groot-de Laat LE, Geleijnse ML. Left atrial function in patients with mitral valve regurgitation. Am J Physiol Hear Circ Physiol. (2014) 307:H1430–7. doi: 10.1152/ajpheart.00389.2014
129. Borg AN, Pearce KA, Williams SG, Ray SG. Left atrial function and deformation in chronic primary mitral regurgitation. Eur J Echocardiogr. (2009) 10:833–40. doi: 10.1093/ejechocard/jep085
130. Moustafa SE, Alharthi M, Kansal M, Deng Y, Chandrasekaran K, Mookadam F. Global left atrial dysfunction and regional heterogeneity in primary chronic mitral insufficiency. Eur J Echocardiogr. (2011) 12:384–93. doi: 10.1093/ejechocard/jer033
131. Zito C, Carerj S, Todaro MC, Cusmà-Piccione M, Caprino A, Di Bella G, et al. Myocardial deformation and rotational profiles in mitral valve prolapse. Am J Cardiol. (2013) 112:984–90. doi: 10.1016/j.amjcard.2013.05.031
132. Saraiva RM, Yamano T, Matsumura Y, Takasaki K, Toyono M, Agler DA, et al. Left atrial function assessed by real-time 3-dimensional echocardiography is related to right ventricular systolic pressure in chronic mitral regurgitation. Am Heart J. (2009) 158:309–16. doi: 10.1016/j.ahj.2009.05.030
133. Yang LT, Shih JY, Liu YW Li YH, Tsai LM, Luo CY, Tsai WC. Effects of left atrial strain on functional capacity in chronic severe mitral regurgitation. Int J Cardiol. (2013) 168:e151–3. doi: 10.1016/j.ijcard.2013.08.070
134. Yang LT, Tsai WC, Luo CY Li YH, Tsai LM. Role of left atrial reservoir strain rate in left atrial remodeling in severe mitral regurgitation. J Med Ultrasound. (2017) 25:16–23. doi: 10.1016/j.jmu.2016.09.003
135. Ring L, Rana BS, Wells FC, Kydd AC, Dutka DP. Atrial function as a guide to timing of intervention in mitral valve prolapse with mitral regurgitation. JACC Cardiovasc Imaging. (2014) 7:225–32. doi: 10.1016/j.jcmg.2013.12.009
136. Candan O, Ozdemir N, Aung SM, Hatipoglu S, Karabay CY, Guler A, et al. Atrial longitudinal strain parameters predict left atrial reverse remodeling after mitral valve surgery: A speckle tracking echocardiography study. Int J Cardiovasc Imaging. (2014) 30:1049–56. doi: 10.1007/s10554-014-0433-9
137. Bursi F, Barbieri A, Grigioni F, Reggianini L, Zanasi V, Leuzzi C, et al. Prognostic implications of functional mitral regurgitation according to the severity of the underlying chronic heart failure: A long-term outcome study. Eur J Heart Fail. (2010) 12:382–8. doi: 10.1093/eurjhf/hfq014
138. Kajimoto K, Sato N, Takano T. Functional mitral regurgitation at discharge and outcomes in patients hospitalized for acute decompensated heart failure with a preserved or reduced ejection fraction. Eur J Heart Fail. (2016) 18:1051–9. doi: 10.1002/ejhf.562
139. Goliasch G, Bartko PE, Pavo N, Neuhold S, Wurm R, Mascherbauer J, et al. Refining the prognostic impact of functional mitral regurgitation in chronic heart failure. Eur Heart J. (2018) 39:39–46. doi: 10.1093/eurheartj/ehx402
140. Suzuki T, Izumo M, Suzuki K, Koto D, Tsukahara M, Teramoto K, et al. Prognostic value of exercise stress echocardiography in patients with secondary mitral regurgitation: a long-term follow-up study. J Echocardiogr. (2019) 17:147–56. doi: 10.1007/s12574-018-0404-6
141. Palmiero G, Melillo E, Ferro A, Carlomagno G, Sordelli C, Ascione R, et al. Significant functional mitral regurgitation affects left atrial function in heart failure patients: Haemodynamic correlations and prognostic implications. Eur Heart J Cardiovasc Imaging. (2019) 20:1012–9. doi: 10.1093/ehjci/jez036
142. Bandera F, Barletta M, Fontana M, Boveri S, Ghizzardi G, Alfonzetti E, et al. Exercise-induced mitral regurgitation and right ventricle to pulmonary circulation uncoupling across the heart failure phenotypes. Am J Physiol Hear Circ Physiol J Physiol Hear Circ Physiol. (2021) 320:H642–H653. doi: 10.1152/ajpheart.00507.2020
143. Bandera F, Generati G, Pellegrino M, Garatti A, Labate V, Alfonzetti E, et al. Mitral regurgitation in heart failure: Insights from CPET combined with exercise echocardiography. Eur Heart J Cardiovasc Imaging. (2017) 18:296–303. doi: 10.1093/ehjci/jew096
144. Sugimoto T, Bandera F, Generati G, Alfonzetti E, Barletta M, Losito M, et al. Left atrial dynamics during exercise in mitral regurgitation of primary and secondary origin: pathophysiological insights by exercise echocardiography combined with gas exchange analysis. JACC Cardiovasc Imaging. (2020) 13:25–40. doi: 10.1016/j.jcmg.2018.12.031
145. Bonow RO, Carabello BA, Chatterjee K, de Leon AC, Faxon DP, Freed MD, et al. 2008 Focused update incorporated into the ACC/AHA 2006 guidelines for the management of patients with valvular heart disease: a report of the American College of Cardiology/American Heart Association Task Force on Practice Guidelines (Writing Committee to Revise the 1998 Guidelines for the Management of Patients With Valvular Heart Disease): endorsed by the society of cardiovascular anesthesiologists, society for cardiovascular angiography and interventions, and society of thoracic surgeons. Circulation. (2008) 118:e523–661. doi: 10.1161/CIRCULATIONAHA.108.190748
146. Caso P, Ancona R, Di Salvo G, Comenale Pinto S, Macrino M, Di Palma V, et al. Atrial reservoir function by strain rate imaging in asymptomatic mitral stenosis: Prognostic value at 3 year follow-up. Eur J Echocardiogr. (2009) 10:753–9. doi: 10.1093/ejechocard/jep058
147. Candan O, Gecmen C, Kalayci A, Dogan C, Bayam E, Ozkan M. Left atrial electromechanical conduction time predicts atrial fibrillation in patients with mitral stenosis: a 5-year follow-up speckle-tracking echocardiography study. Int J Cardiovasc Imaging. (2017) 33:1491–501. doi: 10.1007/s10554-017-1140-0
148. Jung MH, Jung HO, Lee JW, Youn HJ. Decreases in left atrial compliance during early-stage exercise are related to exercise intolerance in asymptomatic significant mitral stenosis. Echocardiography. (2017) 34:1633–9. doi: 10.1111/echo.13666
149. Chien CY, Chen CW, Lin TK, Lin Y, Lin JW Li Y Da, Chen CH, et al. Atrial deformation correlated with functional capacity in mitral stenosis patients. Echocardiography. (2018) 35:190–5. doi: 10.1111/echo.13770
150. O'Connor K, Magne J, Rosca M, Pirard LA, Lancellotti P. Impact of aortic valve stenosis on left atrial phasic function. Am J Cardiol. (2010) 106:1157–62. doi: 10.1016/j.amjcard.2010.06.029
151. Salas-Pacheco JL, Ávila-Vanzzini N, Eugenia REM, Arias-Godínez JA. Left atrium function by 2D speckle tracking in aortic valve disease. Echocardiography. (2016) 33:1828–34. doi: 10.1111/echo.13368
152. Rusinaru D, Bohbot Y, Kowalski C, Ringle A, Maréchaux S, Tribouilloy C. Left atrial volume and mortality in patients with aortic stenosis. J Am Heart Assoc. (2017) 6: e006615. doi: 10.1161/JAHA.117.006615
153. Ferreira J, Gonçalves V, Marques-Alves P, Martins R, Monteiro S, Teixeira R, et al. Left atrial functional assessment and mortality in patients with severe aortic stenosis with sinus rhythm. Cardiovasc Ultrasound. (2021) 19:1–12. doi: 10.1186/s12947-020-00231-0
154. Marques-Alves P, Marinho AV, Teixeira R, Baptista R, Castro G, Martins R, et al. Going beyond classic echo in aortic stenosis: Left atrial mechanics, a new marker of severity. BMC Cardiovasc Disord. (2019) 19:1–8. doi: 10.1186/s12872-019-1204-2
155. Calin A, Mateescu AD, Rosca M, Beladan CC, Enache R, Botezatu S, et al. Left atrial dysfunction as a determinant of pulmonary hypertension in patients with severe aortic stenosis and preserved left ventricular ejection fraction. Int J Cardiovasc Imaging. (2017) 33:1939–47. doi: 10.1007/s10554-017-1211-2
156. Durand E, Doutriaux M, Bettinger N, Tron C, Fauvel C, Bauer F, et al. Incidence, prognostic impact, and predictive factors of readmission for heart failure after transcatheter aortic valve replacement. JACC Cardiovasc Interv. (2017) 10:2426–36. doi: 10.1016/j.jcin.2017.09.010
157. D'Andrea A, Padalino R, Cocchia R, Di Palma E, Riegler L, Scarafile R, et al. Effects of transcatheter aortic valve implantation on left ventricular and left atrial morphology and function. Echocardiography. (2015) 32:928–36. doi: 10.1111/echo.12808
158. Spethmann S, Baldenhofer G, Dreger H, Stüer K, Sanad W, Saghabalyan D, et al. Recovery of left ventricular and left atrial mechanics in various entities of aortic stenosis 12 months after TAVI. Eur Heart J Cardiovasc Imaging. (2014) 15:389–98. doi: 10.1093/ehjci/jet166
159. Christensen NL, Dahl JS, Carter-Storch R, Bakkestrøm R, Jensen K, Steffensen FH, et al. Association between left atrial dilatation and invasive hemodynamics at rest and during exercise in asymptomatic aortic stenosis. Circ Cardiovasc Imaging. (2016) 9:1–9. doi: 10.1161/CIRCIMAGING.116.005156
160. Tsang Teresa SM, Barnes Marion E, Gersh Bernard J, Bailey Kent R, Seward JB. Left atrial volume as amorphophysiologic expression of leftventricular diastolic dysfunction and relation to cardiovascular risk burden. Am J Cardiol. (2002) 90:1284–9. doi: 10.1016/s0002-9149(02)02864-3
161. Butcher SC, Fortuni F, Kong W, Vollema EM, Prevedello F, Perry R, et al. Prognostic implications of left atrial dilation in aortic regurgitation due to bicuspid aortic valve. Heart. (2021) 1–8. doi: 10.1136/heartjnl-2020-318907
162. Jenner J, Ilami A, Petrini J, Eriksson P, Franco-Cereceda A, Eriksson MJ, et al. Pre- and postoperative left atrial and ventricular volumetric and deformation analyses in severe aortic regurgitation. Cardiovasc Ultrasound. (2021) 19:1–10. doi: 10.1186/s12947-021-00243-4
163. Cioffi G, Stefenelli C. Comparison of left ventricular geometry and left atrial size and function in patients with aortic stenosis vs. those with pure aortic regurgitation. Am J Cardiol. (2002) 90:601–6. doi: 10.1016/S0002-9149(02)02563-8
164. Wahi S, Haluska B, Pasquet A, Case C, Rimmerman CM, Marwick TH. Exercise echocardiography predicts development of left ventricular dysfunction in medically and surgically treated patients with asymptomatic severe aortic regurgitation. Heart. (2000) 84:606–14. doi: 10.1136/heart.84.6.606
165. Gentry JL, Phelan D, Desai MY, Griffin BP. The role of stress echocardiography in valvular heart disease: a current appraisal. Cardiol. (2017) 137:137–50. doi: 10.1159/000460274
166. Vahanian A, Alfieri O, Andreotti F, Antunes MJ, Barón-Esquivias G, Baumgartner H, et al. Guidelines on the management of valvular heart disease (version 2012). Eur Heart J. (2012) 33:2451–96. doi: 10.1093/eurheartj/ehs109
167. Stern H, Calavrezos L, Meierhofer C, Steinlechner E, Müller J, Hager A, et al. Physical exercise reduces aortic regurgitation: Exercise magnetic resonance imaging. JACC Cardiovasc Imaging. (2014) 7:314–5. doi: 10.1016/j.jcmg.2013.10.013
168. Semsarian C, Ingles J, Maron MS, Maron BJ. New perspectives on the prevalence of hypertrophic cardiomyopathy. J Am Coll Cardiol. (2015) 65:1249–54. doi: 10.1016/j.jacc.2015.01.019
169. Paraskevaidis IA, Panou F, Papadopoulos C, Farmakis D, Parissis J, Ikonomidis I, et al. Evaluation of left atrial longitudinal function in patients with hypertrophic cardiomyopathy: A tissue Doppler imaging and two-dimensional strain study. Heart. (2009) 95:483–9. doi: 10.1136/hrt.2008.146548
170. Eshoo S, Semsarian C, Ross DL, Marwick TH, Thomas L. Comparison of left atrial phasic function in hypertrophic cardiomyopathy vs. systemic hypertension using strain rate imaging. Am J Cardiol. (2011) 107:290–6. doi: 10.1016/j.amjcard.2010.08.071
171. Von Roeder M, Rommel KP, Kowallick JT, Blazek S, Besler C, Fengler K, et al. Influence of left atrial function on exercise capacity and left ventricular function in patients with heart failure and preserved ejection fraction. Circ Cardiovasc Imaging. (2017) 10:1–9. doi: 10.1161/CIRCIMAGING.116.005467
172. Yang Y, Yin G, Jiang Y, Song L, Zhao S, Lu M. Quantification of left atrial function in patients with non-obstructive hypertrophic cardiomyopathy by cardiovascular magnetic resonance feature tracking imaging: A feasibility and reproducibility study. J Cardiovasc Magn Reson. (2020) 22:1–11. doi: 10.1186/s12968-019-0589-5
173. Williams LK, Chan RH, Carasso S, Durand M, Misurka J, Crean AM, et al. Effect of left ventricular outflow tract obstruction on left atrial mechanics in hypertrophic cardiomyopathy. Biomed Res Int. (2015) 2015. doi: 10.1155/2015/481245
174. Debonnaire P, Joyce E, Hiemstra Y, Mertens BJ, Atsma DE, Schalij MJ, et al. Left atrial size and function in hypertrophic cardiomyopathy patients and risk of new-onset atrial fibrillation. Circ Arrhythmia Electrophysiol. (2017) 10:1–15. doi: 10.1161/CIRCEP.116.004052
175. Nagueh SF, Lakkis NM, Middleton KJ, Killip D, Zoghbi WA, Quiñones MA, et al. Changes in left ventricular filling and left atrial function six months after nonsurgical septal reduction therapy for hypertrophic obstructive cardiomyopathy. J Am Coll Cardiol. (1999) 34:1123–8. doi: 10.1016/S0735-1097(99)00341-1
176. Muchtar E, Blauwet LA, Gertz MA. Restrictive cardiomyopathy: Genetics, pathogenesis, clinical manifestations, diagnosis, and therapy. Circ Res. (2017) 121:819–37. doi: 10.1161/CIRCRESAHA.117.310982
177. Pereira NL, Grogan M, Dec GW. Spectrum of restrictive and infiltrative cardiomyopathies: part 1 of a 2-part series. J Am Coll Cardiol. (2018) 71:1130–48. doi: 10.1016/j.jacc.2018.01.016
178. Nochioka K, Quarta CC, Claggett B, Roca GQ, Rapezzi C, Falk RH, et al. Left atrial structure and function in cardiac amyloidosis. Eur Heart J Cardiovasc Imaging. (2017) 18:1128–37. doi: 10.1093/ehjci/jex097
179. Mohty D, Boulogne C, Magne J, Varroud-Vial N, Martin S, Ettaif H, et al. Prognostic value of left atrial function in systemic light-chain amyloidosis: A cardiac magnetic resonance study. Eur Heart J Cardiovasc Imaging. (2016) 17:961–9. doi: 10.1093/ehjci/jew100
180. Tigen K, Sunbul M, Karaahmet T, Tasar O, Dundar C, Yalcinsoy M, et al. Early detection of Bi-ventricular and atrial mechanical dysfunction using two-dimensional speckle tracking echocardiography in patients with sarcoidosis. Lung. (2015) 193:669–75. doi: 10.1007/s00408-015-9748-0
181. Degirmenci H, Demirelli S, Arisoy A, Ermiş E, Araz Ö, Bakirci EM, et al. Myocardial deformation and total atrial conduction time in the prediction of cardiac involvement in patients with pulmonary sarcoidosis. Clin Respir J. (2017) 11:68–77. doi: 10.1111/crj.12307
182. Morris DA, Blaschke D, Canaan-Kühl S, Krebs A, Knobloch G, Walter TC, et al. Global cardiac alterations detected by speckle-tracking echocardiography in Fabry disease: left ventricular, right ventricular, and left atrial dysfunction are common and linked to worse symptomatic status. Int J Cardiovasc Imaging. (2015) 31:301–13. doi: 10.1007/s10554-014-0551-4
183. Pichette M, Serri K, Pagé M, Di LZ, Bichet DG, Poulin F. Impaired left atrial function in fabry disease: a longitudinal speckle-tracking echocardiography study. J Am Soc Echocardiogr. (2017) 30:170–179.e2. doi: 10.1016/j.echo.2016.10.014
184. Boyd AC, Lo Q, Devine K, Tchan MC, Sillence DO, Sadick N, et al. Left atrial enlargement and reduced atrial compliance occurs early in fabry cardiomyopathy. J Am Soc Echocardiogr. (2013) 26:1415–23. doi: 10.1016/j.echo.2013.08.024
185. Bernardini A, Camporeale A, Pieroni M, Pieruzzi F, Figliozzi S, Lusardi P, et al. Atrial dysfunction assessed by cardiac magnetic resonance as an early marker of fabry cardiomyopathy. JACC Cardiovasc Imaging. (2020) 13:2262–4. doi: 10.1016/j.jcmg.2020.05.011
186. Putko BN, Yogasundaram H, Chow K, Pagano J, Khan A, Paterson DI, et al. Normal left-atrial structure and function despite concentric left-ventricular remodelling in a cohort of patients with Anderson-Fabry disease. Eur Heart J Cardiovasc Imaging. (2015) 16:1129–36. doi: 10.1093/ehjci/jev057
187. Maron BJ, Towbin JA, Thiene G, Antzelevitch C, Corrado D, Arnett D, et al. Contemporary definitions and classification of the cardiomyopathies: An American Heart Association Scientific Statement from the Council on Clinical Cardiology, Heart Failure and Transplantation Committee; Quality of Care and Outcomes Research and Functio. Circulation. (2006) 113:1807–16. doi: 10.1161/CIRCULATIONAHA.106.174287
188. Weintraub RG, Semsarian C, Macdonald P. Dilated cardiomyopathy. Lancet. (2017) 390:400–14. doi: 10.1016/S0140-6736(16)31713-5
189. Rossi A, Dini FL, Faggiano P, Agricola E, Cicoira M, Frattini S, et al. Independent prognostic value of functional mitral regurgitation in patients with heart failure. A quantitative analysis of 1256 patients with ischaemic and non-ischaemic dilated cardiomyopathy. Heart. (2011) 97:1675–1680. doi: 10.1136/hrt.2011.225789
190. D'Andrea A, Caso P, Romano S, Scarafile R, Cuomo S, Salerno G, et al. Association between left atrial myocardial function and exercise capacity in patients with either idiopathic or ischemic dilated cardiomyopathy: A two-dimensional speckle strain study. Int J Cardiol. (2009) 132:354–63. doi: 10.1016/j.ijcard.2007.11.102
191. Cao S, Zhou Q, Chen JL, Hu B, Guo RQ. The differences in left atrial function between ischemic and idiopathic dilated cardiomyopathy patients: A two-dimensional speckle tracking imaging study. J Clin Ultrasound. (2016) 44:437–45. doi: 10.1002/jcu.22352
192. Negishi K, Negishi T, Zardkoohi O, Ching EA, Basu N, Lwilkoff B, et al. Left atrial booster pump function is an independent predictor of subsequent life-Threatening ventricular arrhythmias in non-ischaemic cardiomyopathy. Eur Heart J Cardiovasc Imaging. (2016) 17:1153–60. doi: 10.1093/ehjci/jev333
193. Yazaki M, Nabeta T, Inomata T, Maemura K, Oki T, Fujita T, et al. Clinical significance of left atrial geometry in dilated cardiomyopathy patients: A cardiovascular magnetic resonance study. Clin Cardiol. (2021) 44:222–9. doi: 10.1002/clc.23529
194. Rossi A, Cicoira M, Zanolla L, Sandrini R, Golia G, Zardini P, et al. Determinants and prognostic value of left atrial volume in patients with dilated cardiomyopathy. J Am Coll Cardiol. (2002) 40:1425–30. doi: 10.1016/S0735-1097(02)02305-7
195. Merlo M, Stolfo D, Gobbo M, Gabassi G, Barbati G, Naso P, et al. Prognostic impact of short-term changes of E/E' ratio and left atrial size in dilated cardiomyopathy. Eur J Heart Fail. (2019) 21:1294–6. doi: 10.1002/ejhf.1543
196. Gulati A, Ismail TF, Jabbour A, Ismail NA, Morarji K, Ali A, et al. Clinical utility and prognostic value of left atrial volume assessment by cardiovascular magnetic resonance in non-ischaemic dilated cardiomyopathy. Eur J Heart Fail. (2013) 15:660–70. doi: 10.1093/eurjhf/hft019
197. Matsumoto K, Tanaka H, Imanishi J, Tatsumi K, Motoji Y, Miyoshi T, et al. Preliminary observations of prognostic value of left atrial functional reserve during dobutamine infusion in patients with dilated cardiomyopathy. J Am Soc Echocardiogr. (2014) 27:430–9. doi: 10.1016/j.echo.2013.12.016
198. Gopal D, Wang J, Han Y. Determinants of normal left atrial volume in heart failure with moderate-to-severely reduced ejection fraction. Cardiol Res Pract. (2018) 2018:7512758. doi: 10.1155/2018/7512758
199. Cameli M, Sciaccaluga C, Loiacono F, Simova I, Miglioranza MH, Nistor D, et al. The analysis of left atrial function predicts the severity of functional impairment in chronic heart failure: The FLASH multicenter study. Int J Cardiol. (2019) 286:87–91. doi: 10.1016/j.ijcard.2019.03.063
200. Malagoli A, Rossi L, Bursi F, Zanni A, Sticozzi C, Piepoli MF, et al. Left atrial function predicts cardiovascular events in patients with chronic heart failure with reduced ejection fraction. J Am Soc Echocardiogr. (2019) 32:248–56. doi: 10.1016/j.echo.2018.08.012
201. Guazzi M, Naeije R. Right heart phenotype in heart failure with preserved ejection fraction. Circ Hear Fail. (2021) 14. doi: 10.1161/CIRCHEARTFAILURE.120.007840
202. Melenovsky V, Hwang SJ, Redfield MM, Zakeri R, Lin G, Borlaug BA. Left atrial remodeling and function in advanced heart failure with preserved or reduced ejection fraction. Circ Hear Fail. (2015) 8:295–303. doi: 10.1161/CIRCHEARTFAILURE.114.001667
203. Putko BN, Savu A, Kaul P, Ezekowitz J, Dyck JR, Anderson TJ, et al. Left atrial remodelling, mid-regional pro-atrial natriuretic peptide, and prognosis across a range of ejection fractions in heart failure. Eur Heart J Cardiovasc Imaging. (2021) 22:220–8. doi: 10.1093/ehjci/jeaa041
204. Guazzi M, Villani S, Generati G, Ferraro OE, Pellegrino M, Alfonzetti E, et al. Right ventricular contractile reserve and pulmonary circulation uncoupling during exercise challenge in heart failure: pathophysiology and clinical phenotypes. JACC Hear Fail. (2016) 4:625–35. doi: 10.1016/j.jchf.2016.03.007
205. Guazzi M, Dixon D, Labate V, Beussink-Nelson L, Bandera F, Cuttica MJ, et al. Contractile function and its coupling to pulmonary circulation in heart failure with preserved ejection fraction: stratification of clinical phenotypes and outcomes. JACC Cardiovasc Imaging. (2017) 10:1211–21. doi: 10.1016/j.jcmg.2016.12.024
206. Bytyçi I, Dini FL, Bajraktari A, Pugliese NR, D'Agostino A, Bajraktari G, et al. Speckle tracking-derived left atrial stiffness predicts clinical outcome in heart failure patients with reduced to mid-range ejection fraction. J Clin Med. (2020) 9:1244. doi: 10.3390/jcm9051244
207. Modin D, Sengeløv M, Jørgensen PG, Olsen FJ, Bruun NE, Fritz-Hansen T, et al. Prognostic value of left atrial functional measures in heart failure with reduced ejection fraction. J Card Fail. (2019) 25:87–96. doi: 10.1016/j.cardfail.2018.11.016
208. Rossi A, Dini FL, Agricola E, Faggiano P, Benfari G, Temporelli PL, et al. Left atrial dilatation in systolic heart failure: a marker of poor prognosis, not just a buffer between the left ventricle and pulmonary circulation. J Echocardiogr. (2018) 16:155–61. doi: 10.1007/s12574-018-0373-9
209. Ersbøll M, Møller JE. Left atrial function in heart failure with reduced ejection fraction. Circ Cardiovasc Imaging. (2018) 11:e008427. doi: 10.1161/CIRCIMAGING.118.008427
210. Santos ABS, Roca GQ, Claggett B, Sweitzer NK, Shah SJ, Anand IS, et al. Prognostic relevance of left atrial dysfunction in heart failure with preserved ejection fraction. Circ Hear Fail. (2016) 9:1–11. doi: 10.1161/CIRCHEARTFAILURE.115.002763
211. Freed BH, Daruwalla V, Cheng JY, Aguilar FG, Beussink L, Choi A, et al. Prognostic utility and clinical significance of cardiac mechanics in heart failure with preserved ejection fraction: importance of left atrial strain. Circ Cardiovasc Imaging. (2016) 9:1–10. doi: 10.1161/CIRCIMAGING.115.003754
212. Abbasi SA, Shah R V, McNulty SE, Hernandez AF, Semigran MJ, Lewis GD, et al. Left atrial structure and function in heart failure with preserved ejection fraction: A RELAX substudy. PLoS ONE. (2016) 11:1–9. doi: 10.1371/journal.pone.0164914
213. Ye Z, Miranda WR, Yeung DF, Kane GC, Oh JK. Left atrial strain in evaluation of heart failure with preserved ejection fraction. J Am Soc Echocardiogr. (2020) 33:1490–9. doi: 10.1016/j.echo.2020.07.020
214. Bytyçi I, Bajraktari G. Left atrial changes in early stages of heart failure with preserved ejection fraction. Echocardiography. (2016) 33:1479–87. doi: 10.1111/echo.13306
215. Phan TT, Abozguia K, Nallur Shivu G, Ahmed I, Leyva F, Patel K, et al. Increased atrial contribution to left ventricular filling compensates for impaired early filling during exercise in heart failure with preserved ejection fraction. J Card Fail. (2009) 15:890–7. doi: 10.1016/j.cardfail.2009.06.440
216. Maffeis C, Morris DA, Belyavskiy E, Kropf M, Radhakrishnan AK, Zach V, et al. Left atrial function and maximal exercise capacity in heart failure with preserved and mid-range ejection fraction. ESC Hear Fail. (2021) 8:116–28. doi: 10.1002/ehf2.13143
217. Obokata M, Negishi K, Kurosawa K, Arima H, Tateno R, Ui G, et al. Incremental diagnostic value of la strain with leg lifts in heart failure with preserved ejection fraction. JACC Cardiovasc Imaging. (2013) 6:749–58. doi: 10.1016/j.jcmg.2013.04.006
218. Sugimoto T, Barletta M, Bandera F, Generati G, Alfonzetti E, Rovida M, et al. Central role of left atrial dynamics in limiting exercise cardiac output increase and oxygen uptake in heart failure: insights by cardiopulmonary imaging. Eur J Heart Fail. (2020) 22:1186–98. doi: 10.1002/ejhf.1829
219. Sugimoto T, Bandera F, Generati G, Alfonzetti E, Bussadori C, Guazzi M. Left atrial function dynamics during exercise in heart failure: pathophysiological implications on the right heart and exercise ventilation inefficiency. JACC Cardiovasc Imaging. (2017) 10:1253–64. doi: 10.1016/j.jcmg.2016.09.021
Keywords: left atrial strain, heart failure stages, myocardial deformation, echocardiography, cardiac magnetic resonance, heart valve disease, cardiomyopathy, exercise echocardiography
Citation: Bandera F, Mollo A, Frigelli M, Guglielmi G, Ventrella N, Pastore MC, Cameli M and Guazzi M (2022) Cardiac Imaging for the Assessment of Left Atrial Mechanics Across Heart Failure Stages. Front. Cardiovasc. Med. 8:750139. doi: 10.3389/fcvm.2021.750139
Received: 30 July 2021; Accepted: 14 December 2021;
Published: 13 January 2022.
Edited by:
Leonid Goubergrits, Charité University Medicine Berlin, GermanyReviewed by:
Piet Claus, KU Leuven, BelgiumLuigi P. Badano, University of Milano Bicocca, Italy
Ciro Santoro, Federico II University Hospital, Italy
Daniel Morris, Charité University Medicine Berlin, Germany
Copyright © 2022 Bandera, Mollo, Frigelli, Guglielmi, Ventrella, Pastore, Cameli and Guazzi. This is an open-access article distributed under the terms of the Creative Commons Attribution License (CC BY). The use, distribution or reproduction in other forums is permitted, provided the original author(s) and the copyright owner(s) are credited and that the original publication in this journal is cited, in accordance with accepted academic practice. No use, distribution or reproduction is permitted which does not comply with these terms.
*Correspondence: Francesco Bandera, Francesco.bandera@unimi.it