- 1Department of Cardiology, Guangdong Provincial Hospital of Chinese Medicine, Guangzhou, China
- 2The Second Affiliated Hospital of Guangzhou University of Chinese Medicine, Guangzhou, China
- 3Division of Cardiovascular Diseases, Department of Internal Medicine, Mayo Clinic and Foundation, Rochester, MN, United States
- 4Healthy Systems and Policy, Department of Global Public Health, Karolinska Institutet, Stockholm, Sweden
- 5Key Unit of Methodology in Clinical Research, Guangdong Provincial Hospital of Chinese Medicine, Guangzhou, China
- 6Guangdong Provincial Key Laboratory of Chinese Medicine for Prevention and Treatment of Refractory Chronic Diseases, Guangzhou, China
Background: As demand for cardiopulmonary exercise test using a supine position has increased, so have the testing options. However, it remains uncertain whether the existing evaluation criteria for the upright position are suitable for the supine position. The purpose of this meta-analysis is to compare the differences in peak oxygen uptake (VO2peak) between upright and supine lower extremity bicycle exercise.
Methods: We searched PubMed, Web Of Science and Embase from inception to March 27, 2021. Self-control studies comparing VO2peak between upright and supine were included. The quality of the included studies was assessed using a checklist adapted from published papers in this field. The effect of posture on VO2peak was pooled using random/fixed effects model.
Results: This meta-analysis included 32 self-control studies, involving 546 participants (63% were male). 21 studies included only healthy people, 9 studies included patients with cardiopulmonary disease, and 2 studies included both the healthy and cardiopulmonary patients. In terms of study quality, most of the studies (n = 21, 66%) describe the exercise protocol, and we judged theVO2peak to be valid in 26 (81%) studies. Meta-analysis showed that the upright VO2peak exceeded the supine VO2peak [relative VO2peak: mean difference (MD) 2.63 ml/kg/min, 95% confidence interval (CI) 1.66-3.59, I2 = 56%, p < 0.05; absolute VO2peak: MD 0.18 L/min, 95% CI 0.10-0.26, I2 = 63%, p < 0.05). Moreover, subgroup analysis showed there was more pooled difference in healthy people (4.04 ml/kg/min or 0.22 L/min) than in cardiopulmonary patients (1.03 ml/kg/min or 0.12 L/min).
Conclusion: VO2peak in the upright position is higher than that in supine position. However, whether this difference has clinical significance needs further verification.
Systematic Review Registration: identifier, CRD42021233468.
Introduction
The cardiopulmonary exercise test (CPET) is a non-invasive and safe method for comprehensive evaluation of cardiopulmonary function during exercise. It has been used in a variety of settings including differential diagnosis, surgical risk assessment, and prognosis evaluation. For example, patients with heart failure and chronic obstructive pulmonary disease with low peak oxygen uptake (VO2peak) have low survival rates (1, 2).
The two most common modes of CPET are upright bicycle and treadmill, followed by supine bicycle. Because of less arm and torso movement during supine cycling compared to upright cycle or treadmill, there can be less artifact in collected metrics and greater ease in obtaining clear and stable cardiac imaging and circulatory measurements when patients are supine. Therefore, supine CPET combined with cardiac imaging is the most comprehensive and sensitive means to evaluate the state of cardiac chambers, cardiac hemodynamics, and valve function during exercise (3, 4). Due to this advantage, clinical demand for supine CPET has been on the rise. However, researchers have hypothesized that the two positions' CPET results may be different. This is because the change from the upright position to supine position will affect the venous return, cardiac output (CO), the lung ventilation/perfusion matching (V/Q), and skeletal muscle blood flow and perfusion (5–8).
Studies have compared the cardiovascular response between upright and supine cycle exercise tests, but the results have been inconsistent. For example, Kramer's study of 14 men with heart failure showed that the VO2peak in the upright position exceeded that in the supine (9). Conversely, Bonzheim's study found that VO2peak in the supine was slightly higher than that in upright in patients with coronary artery disease (10). Therefore, the objective of this study was to compare the VO2peak attained from upright and supine lower extremity bicycle exercise.
Methods
This systematic review was conducted and reported in accordance with the Preferred Reporting Items for Systematic Reviews and Meta-Analyses statement (Supplementary Materials S1: PRISMA 2020 Checklist) and the Cochrane Handbook for Interventional Reviews and registered in PROSPERO (CRD42021233468).
Data Sources and Search Strategy
We searched PubMed, Web Of Science and Embase on March 27, 2021 using relevant keywords and a Boolean search string (see Supplementary Materials S2 for the detailed search strategy): (((((“Exercise test”)) OR (“exercise test” OR “cardiopulmonary exercise test” OR “cycle exercise” OR “ergometer” OR “CPET” OR “CPX”)) AND (“position” OR “posture” OR “supine” OR “recumbent” OR “recline” OR “lean” OR “tilt” OR “clinostatism” OR “decubitus” OR “lie”)) AND (“erect” OR “upright” OR “orthostatic” OR “sit”)) AND (“VO2” OR “oxygen uptake”). The search string consisted of MeSH and general search terms. Searches were restricted to English.
Inclusion and Exclusion Criteria
We included self-control trials, and selection criteria conformed to the PICOS approach, as described hereinafter.
Populations
There were no restrictions regarding subjects, except for persons with disabilities.
Intervention
Subjects completed an incremental maximum exercise test using a leg bicycle ergometer in a supine position. However, we excluded any studies if other interventions had been applied, such as the use of drugs that may affect hemodynamics, or lower limb negative pressure.
Comparators
The same subjects completed the exercise using a leg bicycle ergometer in an upright position with the same exercise protocol.
Outcomes
The outcome measure was absolute and/or relative VO2peak.
Study Selection
After eliminating duplicate articles, XW and CL screened the titles and abstracts. Studies that did not mention VO2peak or a synonym in the study title and/or abstracts, but were likely to have included them as a secondary measure, were also included. In the second step, XW and CL read the full texts of articles considered relevant based on title and abstract. There was full agreement on the inclusion of the full-text articles.
Assessing Methodological Quality
XW and CL assessed the quality of the included studies with a modified version of the Downs and Black checklist (see Supplementary Materials S3) (11). This checklist has been employed in several reviews in the field of sports science, which also uses cross-sectional studies for data retrieval. In our modified version, we considered four domains, including seven items, to evaluate the included studies' quality: (1) are the interventions of interest clarified? (2) are the test positions clarified? (3) is the time period the participants have between tests similar? (4) was the test order randomized? (5) how was VO2peak defined? (6) was the VO2peak test valid? and (7) are the characteristics of the participants included in the study clarified? All items were rated as “Yes,” “No,” or “Not sure.”
Data Extraction
We extracted data on VO2peak in the respective positions and the characteristics of the participants (number of participants, sex, age, body mass, types of disease) as well as the starting workload, rotation rate, duration and workload increase of the increments used during the test protocols.
Statistical Analysis
We performed statistical analysis with RevMan version 5.3. The data are segmented or combined based on the angle between the upper body and the horizontal plane, or whether there is cardiopulmonary disease, and the calculation process is based on the formula in Cochrane 7.7. Continuous variables are expressed by means and standard deviation. We assessed heterogeneity across included studies using a Cochran chi-square test (with 0.1 as the cutoff for statistical significance) and an I2 statistic test. We employed a random effects model where there was evidence of statistical heterogeneity (I2 statistic > 50%). Otherwise, we used a fixed effects model. If there was heterogeneity, we first analyzed the source of heterogeneity, and then used subgroup analysis or other methods to deal with it. After excluding obvious clinical heterogeneity, we conducted the meta-analysis with a random response model. The meta-analysis test level was p = 0.05.
Results
Search Results
The detailed study search and selection process is outlined in Figure 1. In total, we retrieved 1,179 records from the database searches. After excluding duplicates, we screened 1,011 potentially relevant abstracts, and excluded 891 for failing to meet the inclusion criteria. We read the remaining 120 full texts, and deemed 32 self-control trials eligible for systematic review (9, 10, 12–41).
Methodological Quality of the Included Studies
The quality of the included studies is shown in Table 1. Only nine studies (28%) clarified the participants' inclusion and exclusion criteria. Most of the studies (n = 21, 66%) clarified the exercise protocol, but only about half (n = 14, 44%) clarified the body position during exercise. Twenty studies (62.5%) mentioned the use of randomization methods to determine the sequence of positions used in the CPET. In addition, the interval between the two tests was similar in 18 (56%) of the studies. Although the VO2peak was clarified in 14(44%) studies, VO2peak was judged to be valid in 26 (81%) studies where the criteria for verification of maximal effort were stated explicitly.
Characteristics of the Included Studies
Details of the participants' characteristics are listed in Table 2. 546 participants were included in the meta-analysis, of which about 62% were male. 21 studies included only healthy people, 9 studies included patients with cardiopulmonary disease, and 2 studies included both the healthy and the patients with cardiopulmonary disease. The ages of the included population ranged from 9 to 72 years old. There were four articles on children (13, 23, 25, 34), one on both adults and children (41), and the remaining 27 articles were on adults.
Details on the exercise protocols used in the included studies are summarized in Table 3. All of the included studies used the continuous incremental exercise program, except for Quinn's study (12). The angle between the upper body and the horizontal in the supine position ranged from −6 to 65°. In some studies, there was only one position and one test for supine exercise, while in other studies, supine exercise contained multiple positions and multiple tests. For example, Egaña's study showed that the angles between the upper body and the horizontal plane in the supine position included zero, 15 and 30° (21). The interval between the two tests was within 1 month in all studies, except for May's and Mizumi's (13, 14).
Comparison of VO2peak Between Positions
In terms of the relative VO2peak, our pooled results showed that the upright VO2peak was higher than the supine VO2peak (relative VO2peak: MD = 2.63 ml/kg/min, 95% CI: 1.66, 3.59, I2 = 56%, p < 0.05; Figure 2). In the healthy subgroup, the upright VO2peak remained higher than supine and the effect size was larger without heterogeneity (relative VO2peak: MD = 4.04 ml/kg/min, 95% CI: 3.25, 4.83, I2 = 0%; Figure 2). Similarly, in patients with cardiopulmonary disease, upright VO2peak was also higher than supine, however, the effect size was lower compared to the healthy subgroup (relative VO2peak: MD = 1.03 ml/kg/min, 95% CI: 0.29, 1.76, I2 = 47%; Figure 2).
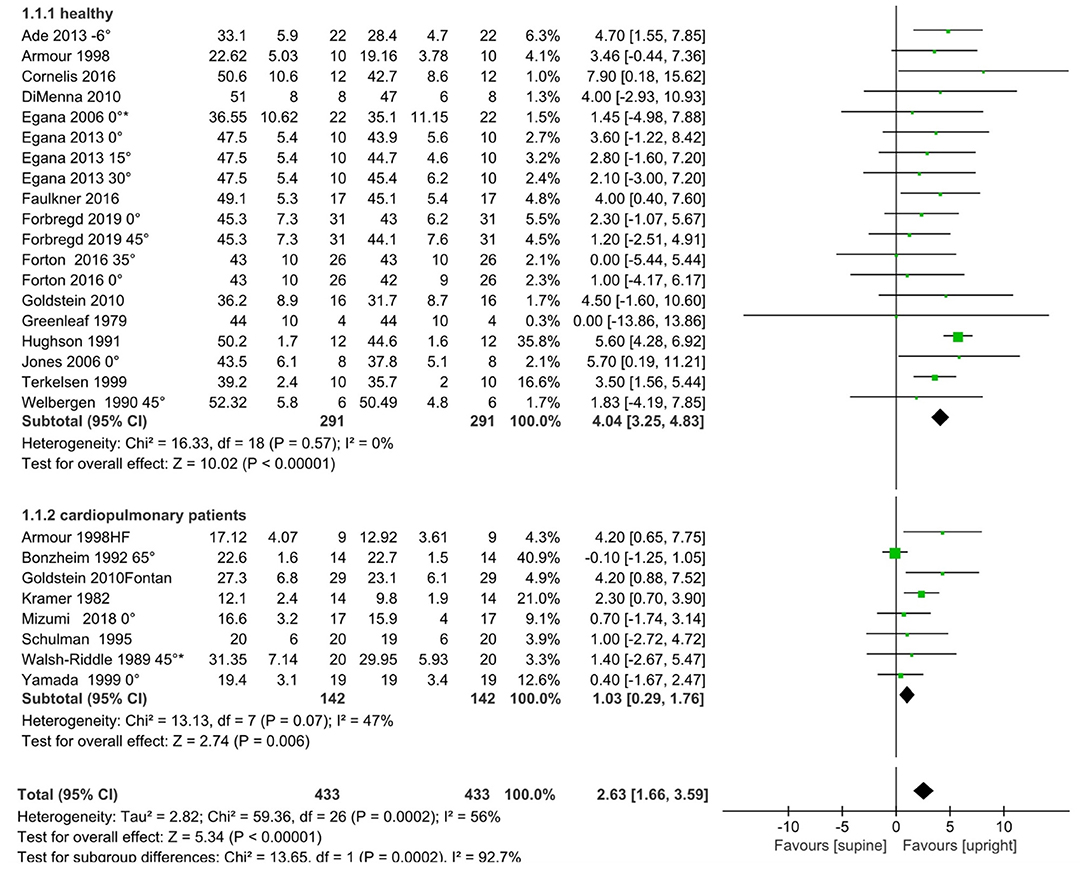
Figure 2. Meta-analysis results for relative VO2peak (ml/kg/min), including overall pooled effects and subgroup effects of the healthy and the cardiopulmonary disease patients. HF, heart failure; Fontan, Single Ventricle Receiving Fontan Palliation. A degree (°) symbol after the reference refers to the angle between upper body and horizontal plane in the supine position. A reference without a degree indicates that the details were unavailable in the original text. An asterisk means that the original text's grouping data are combined according to the formula in Cochrane 7.7, because the angle between the upper body and the horizontal plane is the same as in the supine position.
In terms of the absolute VO2peak, our pooled results also showed that the upright VO2peak was higher than the supine VO2peak (absolute VO2peak: MD = 0.18 L/min, 95% CI: 0.10, 0.26, I2 = 63%, p < 0.05; Figure 3). Again, the effect size was higher in the healthy subgroup (absolute VO2peak: MD = 0.22 L/min, 95% CI: 0.12, 0.32, I2 = 69%; Figure 3), but lower in the cardiopulmonary disease subgroup (absolute VO2peak: MD = 0.12 ml/kg/min, 95% CI: 0.02, 0.21 I2 = 31%; Figure 3).
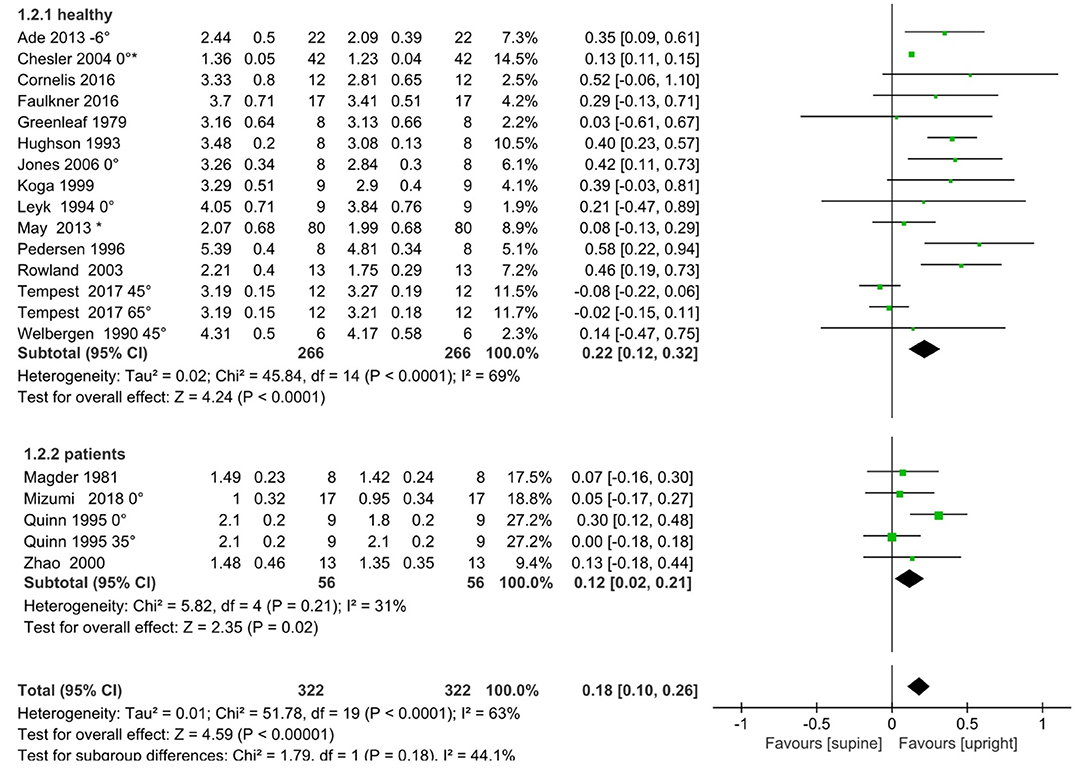
Figure 3. Meta-analysis results for absolute VO2peak (L/min), including overall pooled effects and subgroup effects of the healthy and the cardiopulmonary disease patients. A degree (°) marker after the reference refers to the angle between upper body and horizontal plane in the supine position. References without a degree symbol indicates that the details were unavailable in the original text. An asterisk means that the original text's grouping data are combined according to the formula in Cochrane 7.7, because the angle between upper body and horizontal plane is the same in the supine position.
Discussion
The purpose of this paper is to evaluate the effect of body position on VO2peak in the CPET via meta-analysis. The results indicate that the VO2peak measured by CPET in the upright position was higher than that of the supine position during an incremental cycling exercise test. Moreover, this difference exists in both healthy people and patients with cardiopulmonary disease.
Physiologic shifts in hemodynamic distribution occur with along with changes from supine to upright postures. This hemodynamic shift alters the preload to the right ventricle which subsequently affects preload to the left ventricle and the Frank-Starling mechanism associated with ventricular contraction and stroke volume. Thadani and Parker. have reported hemodynamic differences between the supine and upright positions in normal subjects. They found higher heart rates with lower left ventricular filling pressures and stroke volume index in the upright position, both at rest and during exercise. However, they noted no differences in cardiac index or peak work load between the two modes of exercise (42). Kramer's study of heart failure patients also showed significantly higher right atrial pressure in the supine position, but no differences in cardiac index or stroke index between the two positions. Further, these authors found significantly lower VO2peak in the supine position (9). Moreover, in chronic thromboembolic pulmonary hypertension patients, Mizumi's study showed that CO at rest in the supine position was significantly higher than that in the upright. However, CO at peak exercise was comparable between the two positions, even though VO2peak in the supine position tends to be lower than that of the upright (14). These studies suggest that increased ventricular preload caused by postural changes does not necessarily lead to an increase in stroke volume or CO as a key mechanism to facilitate increased oxygen uptake in the upright posture. In contrast, these data suggest that VO2peak in the supine position may be limited by factors other than the effect of central hemodynamic shifts on CO.
According to the Fick principle, VO2 = CO * C(a-v) O2 (arteriovenous oxygen difference) (43). Therefore, the VO2 is influenced by both central and peripheral components, including CO and C(a-v) O2, during exercise. Studies have shown that VO2peak in the upright position is higher than that in the supine position, and accompanied by higher C(a-v) O2, while there is no difference in cardiac index between the two positions (9, 44). The change in C(a-v) O2 is influenced by both muscle's ability to consume oxygen and the amount of muscle mass involved in the activity. The oxygen uptake in the muscles is determined by factors such as the amount of muscle work, perfusion pressure, blood flow and mitochondrial density and activity. With regards to muscle mass, the upright position requires greater muscle mass involvement, and thus more physiologic work. Research by Bouillon et al. has demonstrated that during upright cycling, the maximum voluntary isometric contraction of the gluteus maximus, gluteus medius, biceps femoris, lateral head of gastrocnemius, anterior tibialis, rectus femoris, lumbar erector spinalis and rectus abdominis exceed those measured in the semi-reclining position (45). Furthermore, when standing upright, the distribution of blood in the lower extremity vein increases due to increased orthostatic tension. Additionally, the arteries' diameter is wider than in the supine position, and the blood flow velocity is slower. This is conducive to the diffusion of more oxygen from hemoglobin to myoglobin (46). Furthermore, Eiken found that exposing working legs to sub-atmospheric pressure can increase perfusion pressure. This can improve motor ability in the supine position, and simulate upright exercise in normal gravity (47, 48). Further, several studies have used near-infrared spectroscopy illuminate the oxyhemoglobin relationship in the periphery during exercise. These studies suggest that muscle oxygen absorption capacity is higher in the upright position (49–51). Therefore, the C(a-v) O2 during peak exercise is higher in the upright position, and this may contribute to the increased VO2peak noted in the upright position.
In addition, studies have shown that the increased venous return in the supine position is conducive to increasing the fluid shift to the pulmonary circulation and interstitium. This may compress small airways and/or blood vessels, unbalancing the overall pulmonary V/Q ratio and reducing ventilatory efficiency (52). Bryan et al. (5) demonstrated that the change from an upright position to the supine position in healthy individuals results in a decrease in the V/Q of the entire lung from 0.83 to 0.76. Further, Sandoval et al. have shown that even in the resting state, the change in body position causes significant changes in alveolar-arterial oxygen partial pressure difference in patients with Eisenmenger syndrome. This decreases oxygen saturation in the supine position (53). In contrast, other studies have shown that the change from an upright position to a supine does not cause significant reduction in ventilatory efficiency in healthy people, or even in patients with stable heart failure (16, 18, 24, 37). As such, the extent to which these components affect peak oxygen uptake in the supine position, and contribute to the lower values when compared to those measured in the upright position, remains unclear.
Limitations
It is important to acknowledge limitations associated with this study. First, the sample sizes of most studies in this meta-analysis were very small. Second, since this research is a self-control study, it is difficult to blind participants to the exercise condition, and therefore difficult to prevent unintentional bias. Third, the difference in VO2peak between the upright position and the supine position may vary among patient groups, and the data for patients with cardiopulmonary disease was insufficient. Therefore, we could not conduct subgroup analysis according to specific disease types. In the future, with the increase in research data from patients with cardiopulmonary disease, subgroup analysis could be conducted according to specific disease types. Lastly, this analysis was unable to account for different supine position body angles. The body position of research participants should be clarified in future clinical studies to facilitate subgroup analysis.
Conclusion
VO2peak in the upright position is higher than that measured in the supine position, in both healthy subjects and patients with cardiopulmonary disease. However, additional verification is needed to determine whether this difference has clinical significance. Like bicycle and treadmill exercise modalities, both supine and upright exercise modalities can be applied for differential diagnosis, surgical risk assessment, prognosis, and to evaluate overall exercise capacity and therapeutic effects of a variety of clinical interventions. Researchers should consider these differences across exercise modalities, and choose the form of exercise which best suits their clinical requirements.
Data Availability Statement
The raw data supporting the conclusions of this article will be made available by the authors, without undue reservation.
Author Contributions
XW and WJ contributed to the conception and design of the study. XW and CL contributed to the data acquisition, analysis, and interpretation. XW drafted the first version of the manuscript and revised it based on other authors' contributions. All authors contributed important intellectual content to the manuscript's critical revision, read, and approved the final manuscript.
Funding
This work was supported by the Guangdong Provincial Key Laboratory of Chinese Medicine for Prevention and Treatment of Refractory Chronic Diseases (grant number: 2018B030322012); the General Research Fund of Traditional Chinese Medicine Science and Technology from Guangdong Provincial Hospital of Chinese Medicine (YN2018ML02); and the Clinical Research Funding of Traditional Chinese Medicine Science and Technology (Project 1010) from Guangdong Provincial Hospital of Chinese Medicine (YN10101910).
Conflict of Interest
The authors declare that the research was conducted in the absence of any commercial or financial relationships that could be construed as a potential conflict of interest.
Publisher's Note
All claims expressed in this article are solely those of the authors and do not necessarily represent those of their affiliated organizations, or those of the publisher, the editors and the reviewers. Any product that may be evaluated in this article, or claim that may be made by its manufacturer, is not guaranteed or endorsed by the publisher.
Acknowledgments
The authors wish to thank Dr. Xiaoguang Chen from the Department of Cardiology at GPHCM for his guidance in the use of RevMan, as well as research assistants from the GPHCM for their assistance with data collection.
Supplementary Material
The Supplementary Material for this article can be found online at: https://www.frontiersin.org/articles/10.3389/fcvm.2021.734687/full#supplementary-material
Abbreviations
C(a-v) O2, arteriovenous oxygen difference; CO, cardiac output; CPET, cardiopulmonary exercise test; SD, standard deviation; MD, mean difference; VO2peak, peak oxygen uptake; V/Q, Ventilation/perfusion ratio.
References
1. Mancini DM, Eisen H, Kussmaul W, Mull R, Edmunds LH Jr, Wilson JR. Value of peak exercise oxygen consumption for optimal timing of cardiac transplantation in ambulatory patients with heart failure. Circulation. (1991) 83:778-86. doi: 10.1161/01.CIR.83.3.778
2. Oga T, Nishimura K, Tsukino M, Sato S, Hajiro T. Analysis of the factors related to mortality in chronic obstructive pulmonary disease: role of exercise capacity and health status. Am J Respir Crit Care Med. (2003) 167:544-9. doi: 10.1164/rccm.200206-583OC
3. Badruddin SM, Ahmad A, Mickelson J, Abukhalil J, Winters WL, Nagueh SF, et al. Supine bicycle versus post-treadmill exercise echocardiography in the detection of myocardial ischemia: a randomized single-blind crossover trial [published correction appears in J Am Coll Cardiol. (1999) Aug;34:613]. J Am Coll Cardiol. (1999) 33:1485-90. doi: 10.1016/S0735-1097(99)00043-1
4. Skulstad H, Aakhus S. Stress echocardiography with semi-supine bicycle. Tidsskrift for Den Norske Lgeforening Tidsskrift for Praktisk Medicin Ny Rkke. (2012) 132:2175-8. doi: 10.4045/tidsskr.11.1538
5. Bryan AC, Bentivoglio LG, Beerel F, Macleish H, Zidulka A, Bates DV. Factors Affecting Regional Distribution of Ventilation and Perfusion in the Lung. J Appl Physiol. (1964) 19:395-402. doi: 10.1152/jappl.1964.19.3.395
6. Stanley A. Rubin, Harvey V. Brown. Ventilation and gas exchange during exercise in severe chronic heart failure. Am Rev Respir Dis. (1984) 129:63-4. doi: 10.1164/arrd.1984.129.2P2.S63
7. Sullivan MJ, Higginbotham MB. Increased exercise ventilation in patients with chronic heart failure: intact ventilatory control despite hemodynamic and pulmonary abnormalities. Circulation. (1988) 77:552-9. doi: 10.1161/01.CIR.77.3.552
8. Bønnelykke Sørensen V, Wroblewski H, Galatius S, Haunsø S, Kastrup J. Assessment of continuous skeletal muscle blood flow during exercise in humans. Microvasc Res. (2000) 59:301-9. doi: 10.1006/mvre.1999.2219
9. Kramer B, Massie B, Topic N. Hemodynamic differences between supine and upright exercise in patients with congestive heart failure. Circulation. (1982) 66:820-5. doi: 10.1161/01.CIR.66.4.820
10. Bonzheim SC, Franklin BA, DeWitt C, Marks C, Goslin B, Jarski R, et al. Physiologic responses to recumbent versus upright cycle ergometry, and implications for exercise prescription in patients with coronary artery disease. Am J Cardiol. (1992) 69:40-44. doi: 10.1016/0002-9149(92)90673-M
11. Downs SH, Black N. The feasibility of creating a checklist for the assessment of the methodological quality both of randomised and non-randomised studies of health care interventions. J Epidemiol Community Health. (1998) 52:377-84. doi: 10.1136/jech.52.6.377
12. Quinn TJ, Smith SW, Vroman NB, Kertzer R, Olney WB. Physiologic responses of cardiac patients to supine, recumbent, and upright cycle ergometry. Arch Phys Med Rehabil. (1995) 76:257-61. doi: 10.1016/S0003-9993(95)80612-1
13. May LJ, Punn R, Olson I, Kazmucha JA, Liu MY, Chin C. Supine cycling in pediatric exercise testing: disparity in performance measures. Pediatr Cardiol. (2014) 35:705-10. doi: 10.1007/s00246-013-0841-y
14. Mizumi S, Goda A, Takeuchi K, Kikuchi H, Inami T, Soejima K, et al. Effects of body position during cardiopulmonary exercise testing with right heart catheterization. Physiol Rep. (2018) 6:e13945. doi: 10.14814/phy2.13945
15. Ade CJ, Broxterman RM, Barstow TJ. Effects of body posture and exercise training on cardiorespiratory responses to exercise. Respir Physiol Neurobiol. (2013) 188:39-48. doi: 10.1016/j.resp.2013.04.023
16. Armour W, Clark AL, McCann GP, Hillis WS. Effects of exercise position on the ventilatory responses to exercise in chronic heart failure. Int J Cardiol. (1998) 66:59-63. doi: 10.1016/S0167-5273(98)00193-4
17. Chesler RM, Stein RA. The clinical use of supine left lateral leg cycle ergometry for exercise electrocardiographic testing in women, as used in exercise echocardiography [published correction appears in J Am Soc Echocardiogr. (2004) Jun;17:643]. J Am Soc Echocardiogr. (2004) 17:361-6. doi: 10.1016/j.echo.2003.12.002
18. Cornelis N, Buys R. The effects of exercise modality on maximal and submaximal exercise parameters obtained by graded maximal exercise testing. Int J Cardiol. (2016) 222:538-47. doi: 10.1016/j.ijcard.2016.07.190
19. DiMenna FJ, Wilkerson DP, Burnley M, Bailey SJ, Jones AM. Priming exercise speeds pulmonary O2 uptake kinetics during supine “work-to-work” high-intensity cycle exercise. J Appl Physiol (1985). (2010) 108:283-92. doi: 10.1152/japplphysiol.01047.2009
20. Egaña M, Green S, Garrigan EJ, Warmington S. Effect of posture on high-intensity constant-load cycling performance in men and women. Eur J Appl Physiol. (2006) 96:1-9. doi: 10.1007/s00421-005-0057-9
21. Egaña M, Columb D, O'Donnell S. Effect of low recumbent angle on cycling performance, fatigue, and V·O(2) kinetics. Med Sci Sports Exerc. (2013) 45:663-73. doi: 10.1249/MSS.0b013e318279a9f2
22. Faulkner J, Lambrick D, Kaufmann S, Stoner L. Effects of upright and recumbent cycling on executive function and prefrontal cortex oxygenation in young healthy men. J Phys Act Health. (2016) 13:882-7. doi: 10.1123/jpah.2015-0454
23. Forbregd TR, Aloyseus MA, Berg A, Greve G. Cardiopulmonary capacity in children during exercise testing: the differences between treadmill and upright and supine cycle ergometry. Front Physiol. (2019) 10:1440. doi: 10.3389/fphys.2019.01440
24. Forton K, Motoji Y, Deboeck G, Faoro V, Naeije R. Effects of body position on exercise capacity and pulmonary vascular pressure-flow relationships. J Appl Physiol (1985). (2016) 121:1145-50. doi: 10.1152/japplphysiol.00372.2016
25. Goldstein BH, Connor CE, Gooding L, Rocchini AP. Relation of systemic venous return, pulmonary vascular resistance, and diastolic dysfunction to exercise capacity in patients with single ventricle receiving fontan palliation. Am J Cardiol. (2010) 105:1169-75. doi: 10.1016/j.amjcard.2009.12.020
26. Greenleaf JE, Van Beaumont W, Brock PJ, Morse JT, Mangseth GR. Plasma volume and electrolyte shifts with heavy exercise in sitting and supine positions. Am J Physiol. (1979) 236:R206-14. doi: 10.1152/ajpregu.1979.236.3.R206
27. Hughson RL, Cochrane JE, Butler GC. Faster O2 uptake kinetics at onset of supine exercise with than without lower body negative pressure. J Appl Physiol (1985). (1993) 75:1962-7. doi: 10.1152/jappl.1993.75.5.1962
28. Hughson RL, Xing HC, Borkhoff C, Butler GC. Kinetics of ventilation and gas exchange during supine and upright cycle exercise. Eur J Appl Physiol Occup Physiol. (1991) 63:300-7. doi: 10.1007/BF00233866
29. Jones AM, Berger NJ, Wilkerson DP, Roberts CL. Effects of “priming” exercise on pulmonary O2 uptake and muscle deoxygenation kinetics during heavy-intensity cycle exercise in the supine and upright positions. J Appl Physiol (1985). (2006) 101:1432-41. doi: 10.1152/japplphysiol.00436.2006
30. Koga S, Shiojiri T, Shibasaki M, Kondo N, Fukuba Y, Barstow TJ. Kinetics of oxygen uptake during supine and upright heavy exercise. J Appl Physiol (1985). (1999) 87:253-60. doi: 10.1152/jappl.1999.87.1.253
31. Leyk D, Essfeld D, Hoffmann U, Wunderlich HG, Baum K, Stegemann J. Postural effect on cardiac output, oxygen uptake and lactate during cycle exercise of varying intensity. Eur J Appl Physiol Occup Physiol. (1994) 68:30-5. doi: 10.1007/BF00599238
32. Magder S, Linnarsson D, Gullstrand L. The effect of swimming on patients with ischemic heart disease. Circulation. (1981) 63:979-86. doi: 10.1161/01.CIR.63.5.979
33. Pedersen PK, Mandøe H, Jensen K, Andersen C, Madsen K. Reduced arterial O2 saturation during supine exercise in highly trained cyclists. Acta Physiol Scand. (1996) 158:325-31. doi: 10.1046/j.1365-201X.1996.575323000.x
34. Rowland T, Garrison A, Delulio A. Circulatory responses to progressive exercise: insights from positional dif ferences. Int J Sports Med. (2003) 24:512-7. doi: 10.1055/s-2003-42016
35. Schulman DS, Tugoen JF, Flores AR, Dianzumba S, Reichek N. Left ventricular ejection fraction during supine and upright exercise in patients with systemic hypertension and its relation to peak filling rale. Am J Cardiol. (1995) 76:61-5. doi: 10.1016/S0002-9149(99)80802-9
36. Tempest GD, Eston RG, Parfitt G. A comparison of head motion and prefrontal haemodynamics during upright and recumbent cycling exercise. Clin Physiol Funct Imaging. (2017) 37:723-9. doi: 10.1111/cpf.12365
37. Terkelsen KE, Clark AL, Hillis WS. Ventilatory response to erect and supine exercise. Med Sci Sports Exer. (1999) 31:1429-32. doi: 10.1097/00005768-199910000-00011
38. Walsh-Riddle M, Blumenthal JA. Cardiovascular responses during upright and semi-recumbent cycle ergometry testing. Med Sci Sports Exer. (1989) 21:581-5. doi: 10.1249/00005768-198910000-00014
39. Welbergen E, Clijsen LP. The influence of body position on maximal performance in cycling. Eur J Appl Physiol Occup Physiol. (1990) 61:138-42. doi: 10.1007/BF00236708
40. Yamada Sumio. Mechanism of increase in anaerobic threshold during recovery phase in patients with acute myocardial infarction. Jpn Circ J. (1999) 63:261-6. doi: 10.1253/jcj.63.261
41. Zhao L, Feinberg MS, Gaides M, Ben-Dov I. Why is exercise capacity reduced in subjects with pectus excavatum?. J Pediatr. (2000) 136:163-7. doi: 10.1016/S0022-3476(00)70096-5
42. Thadani U, Parker JO. Hemodynamics at rest and during supine and sitting bicycle exercise in normal subjects. Am J Cardiol. (1978) 41:52. doi: 10.1016/0002-9149(78)90131-5
43. Hamilton WF, Riley RL. Comparison of the Fick and dye injection methods of measuring the cardiac output in man. Am J Physiol. (1948) 153:309-21. doi: 10.1152/ajplegacy.1948.153.2.309
44. Takata T, Yamabe H, Fukuzaki H. The hemodynamic and metabolic basis for decreased aerobic capacity in the supine exercise compared with the upright exercise in coronary artery disease. Jpn Circ J. (1988) 52:495-502. doi: 10.1253/jcj.52.495
45. Bouillon L, Baker R, Gibson C, Kearney A, Busemeyer T. Comparison of trunk and lower extremity muscle activity among four stationary equipment devices: upright bike, recumbent bike, treadmill, and Elliptigo®. Int J Sports Phys Ther. (2016) 11:190-200.
46. MacDonald MJ, Shoemaker JK, Tschakovsky ME, Hughson RL. Alveolar oxygen uptake and femoral artery blood flow dynamics in upright and supine leg exercise in humans. J Appl Physiol (1985). (1998) 85:1622-8. doi: 10.1152/jappl.1998.85.5.1622
47. Eiken O. Effects of increased muscle perfusion pressure on responses to dynamic leg exercise in man. Eur J Appl Physiol Occup Physiol. (1988) 57:772-6. doi: 10.1007/BF01076002
48. Murthy G, Watenpaugh DE, Ballard RE, Hargens AR. Supine exercise during lower body negative pressure effectively simulates upright exercise in normal gravity. J Appl Physiol. (1994) 76:2742-8. doi: 10.1152/jappl.1994.76.6.2742
49. DeLorey DS, Kowalchuk JM, Paterson DH. Relationship between pulmonary O2 uptake kinetics and muscle deoxygenation during moderate-intensity exercise. J Appl Physiol. (1985) (2003) 95:113-20. doi: 10.1152/japplphysiol.00956.2002
50. DiMenna FJ, Bailey SJ, Jones AM. Influence of body position on muscle deoxy [Hb+Mb] during ramp cycle exercise. Respir Physiol Neurobiol. (2010) 173:138-45. doi: 10.1016/j.resp.2010.07.005
51. Inglis EC, Iannetta D, Murias JM. Evaluating the NIRS-derived microvascular O2 extraction “reserve” in groups varying in sex and training status using leg blood flow occlusions. PLoS ONE. (2019) 14:e0220192. doi: 10.1371/journal.pone.0220192
52. Tedjasaputra V, Sá RC, Anderson KM, Kim Prisk G, Hopkins SR. Heavy upright exercise increases ventilation-perfusion mismatch in the basal lung: indirect evidence for interstitial pulmonary edema. J Appl Physiol (1985). (2019) 127:473-81. doi: 10.1152/japplphysiol.00056.2019
Keywords: cardiopulmonary exercise test, peak oxygen uptake, bicycle, posture difference, meta-analysis
Citation: Wan X, Liu C, Olson TP, Chen X, Lu W and Jiang W (2021) Differences in Peak Oxygen Uptake in Bicycle Exercise Test Caused by Body Positions: A Meta-Analysis. Front. Cardiovasc. Med. 8:734687. doi: 10.3389/fcvm.2021.734687
Received: 01 July 2021; Accepted: 13 September 2021;
Published: 11 October 2021.
Edited by:
Jian Yang, Fudan University, ChinaReviewed by:
Candela Diaz Canestro, The University of Hong Kong, Hong Kong, SAR ChinaYuQin Shen, Tongji Hospital Affiliated to Tongji University, China
Copyright © 2021 Wan, Liu, Olson, Chen, Lu and Jiang. This is an open-access article distributed under the terms of the Creative Commons Attribution License (CC BY). The use, distribution or reproduction in other forums is permitted, provided the original author(s) and the copyright owner(s) are credited and that the original publication in this journal is cited, in accordance with accepted academic practice. No use, distribution or reproduction is permitted which does not comply with these terms.
*Correspondence: Weihui Lu, d2VpaHVpLmx1JiN4MDAwNDA7MTYzLmNvbQ==; Wei Jiang, ZHJqaWFuZ3dlaSYjeDAwMDQwOzEyNi5jb20=