- 1Department for Internal Medicine II, University of Ulm, Ulm, Germany
- 2Department for Nuclear Medicine, University of Ulm, Ulm, Germany
Chronic total occlusion (CTO) of coronary arteries is a common finding in patients with known or suspected coronary artery disease (CAD). Although tremendous advances have been made in the interventional treatment of CTOs over the past decade, correct patient selection remains an important parameter for achieving optimal results. Non-invasive imaging can make a valuable contribution. Ischemia and viability, two major factors in this regard, can be displayed using echocardiography, single-photon emission tomography, positron emission tomography, computed tomography, and cardiac magnetic resonance imaging. Each has its own strengths and weaknesses. Although most have been studied in patients with CAD in general, there is an increasing number of studies with positive preselectional factors for patients with CTOs. The aim of this review is to provide a structured overview of the current state of pre-interventional imaging for CTOs.
Introduction
Chronic total occlusion (CTO) of coronary arteries is a common finding in coronary angiograms of patients with known or suspected coronary artery disease (CAD). Despite their frequency, CTOs are the most reliable predictor of an incomplete revascularization. This is the result of two major factors: (a) a lack of data from randomized controlled trials regarding a benefit on mortality and (b) the lower success rate accompanied by a higher complication rate of an interventional revascularization. Large registry studies have shown that CTO percutaneous coronary interventions (PCI) can reduce mortality (1–4). The EUROCTO trial, the first randomized controlled trial on CTO, showed a benefit in terms of ischemic symptom burden rather than other hard clinical outcomes (5). In another randomized controlled trial (REVASC Trial), CTO PCI did not show an improvement in analyzed cardiovascular magnetic resonance (CMR) parameters but a reduction in major adverse cardiovascular events (6).
Current guidelines and position papers therefore recommend CTO PCI in the case of ongoing symptoms and viable myocardia in the CTO territory (7–10). However, imaging of ischemia remains controversial. On the one hand, revascularization is recommended for CAD patients with more than 10% myocardial ischemia (7, 8). However, the trial underlying this recommendation focused on patients with CAD in general and not on CTO patients in particular (11). On the other hand, ischemia is always seen in viable CTO-related myocardia regardless of the grade of collaterals, and in most CTOs of major vessels, the degree of myocardial ischemia is above 10% (12, 13). Even though the ISCHEMIA trial found no benefit of an ischemia-driven revascularization approach, its results are not generally applicable to patients with CTOs (14, 15).
Viability is by definition present in segments with preserved systolic function. In contrast, dysfunctional segments are not a priori avital. The term “hibernation” was introduced to explain a potentially dysfunctional myocardium resulting in ischemia. Information is gained from an initial coronary angiogram where well-developed collaterals correlate with less extensive scarring, indicating a more viable myocardium (16, 17). A normal wall motion in CTO-related areas is a common finding, and extensive, transmural scarring is only observed in about 5% of CTO segments (16). Otherwise, the presence or extent of a Q wave or QS complexes in an electrocardiogram (ECG) is no proof of a vitality (18–20).
For the detection of a hibernating myocardium, a broad range of non-invasive imaging modalities is available. An overview is presented in Table 1 with an example of their use in Figure 1. The selection mainly depends on local availability and expertise. This review aims to discuss the possibilities and limits of the available non-invasive imaging modalities and future trends. The review focuses on studies aiming to optimize patient selection for CTO reperfusion and its outcomes, although most findings are applicable to CAD patients in general.
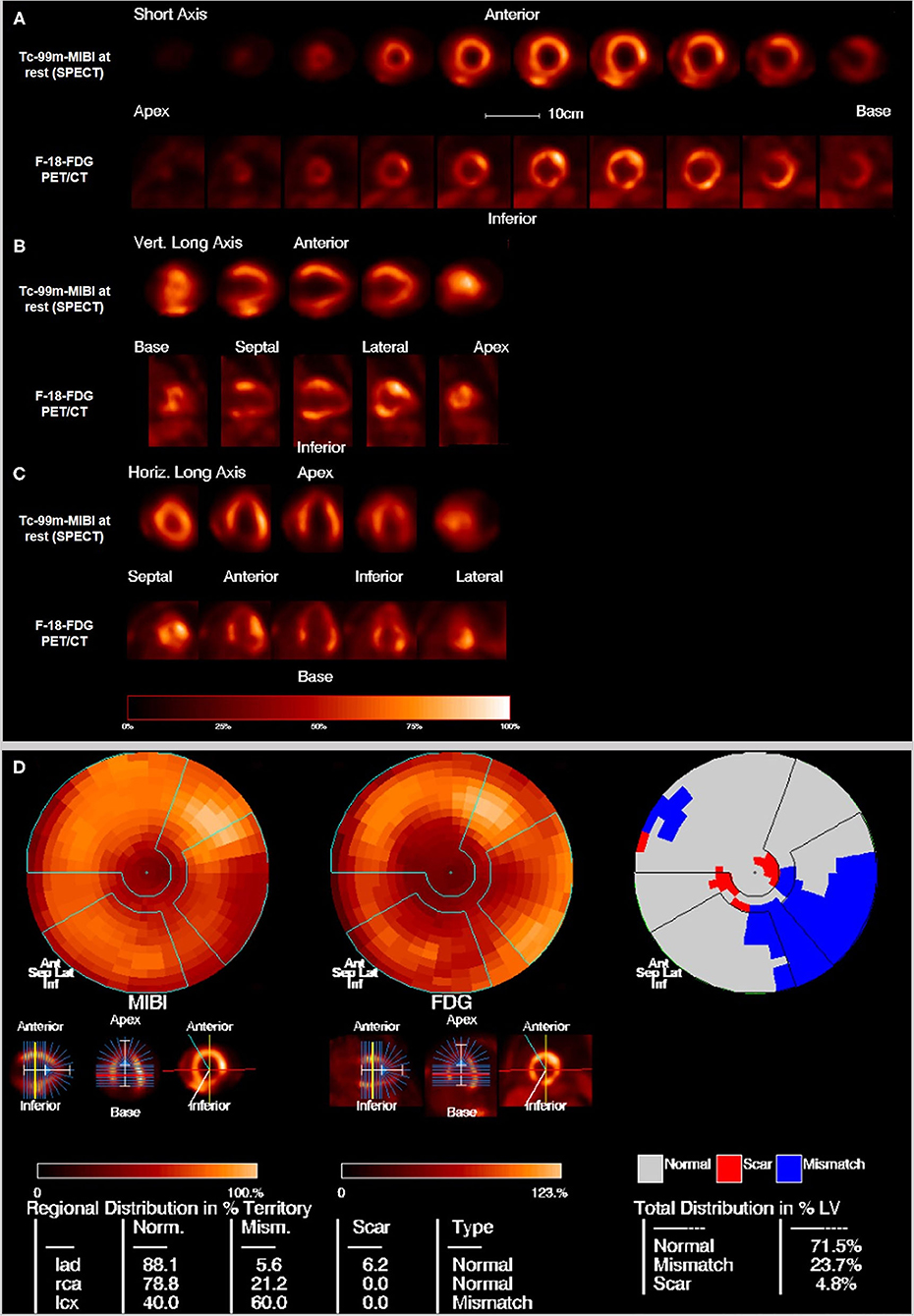
Figure 1. Example of hibernating myocardium: 76 years old male with a perfusion defect on the posterolateral wall in the Tc-99m-MIBI rest scan that shows FDG uptake in the viability F-18-FDG-PET/CT scan resulting in the diagnosis of hibernating myocardium. (A) Short axis, (B) vertical long axis, and (C) horizontal long axis. The upper rows show the perfusion scan at rest with Tc-99m-MIBI (SPECT), the lower rows show the viability scan with F-18-FDG (PET). (D) Polar maps and subtraction image to visualize the mismatch between the perfusion defect on SPECT and the viability on FDG-PET/CT. 23.7% of the myocardium in the left ventricle is in hibernating status.
Echocardiography
Echocardiography is inexpensive and available nearly everywhere. It is the standard modality for the evaluation of global and regional cardiac function. Besides pre-interventional examinations, the bedside use of echocardiography in the catheter laboratory provides the opportunity to detect complications during interventions, such as pericardial effusions or intramural hematomas.
However, the value and validity of echocardiography depend on the investigator's experience. In CAD patients, an increase in regional contractility under dobutamine stress (“contractile reserve”) can show viability and improvement of regional function after revascularization (27–29). The mostly older studies on dobutamine stress echocardiography (DSE) included small patient samples with a clear underrepresentation of women. The sensitivity of DSE in predicting an improvement of regional function ranges from 74 to 94% (30). In a relatively large trial involving 318 patients, revascularization in segments with viability on DSE was associated with reduced mortality (31). In another trial, viability assessed by DSE was associated with a relative reduction of mortality by 19.5% in patients with a severe left ventricular dysfunction (LVEF <35%) (32). Because of its wide distribution and cost-effectiveness and the accumulated experience in clinical practice, DSE appears to be a good tool for examining patients with CTOs, provided that the image quality is good. Another advantage is the low rate of side effects of dobutamine stress (33). Other forms of myocardial stress are also possible as an alternative to dobutamine, such as dipyridamole and treadmill exercise (34).
In addition to the examination under dobutamine stress, echocardiography at rest can predict viability under certain circumstances. An end-diastolic wall thickness (EDWT) of >0.6 cm in dysfunctional segments is a marker of hibernation. In a trial involving 45 patients undergoing 2D echocardiography, DSE, and rest-redistribution thallium-201 (Tl-201) tomography before revascularization, an EDWT of >0.6 cm had 94% sensitivity and 50% specificity with a ROC curve similar to the maximum Tl-201 uptake, while DSE increased specificity to 88% (33). More recent studies focusing on strain echocardiography have reported promising results (35, 36). However, the interpretation of deformation parameters depends heavily on image quality and the examiner's experience. For wider use, validation in a prospective cohort is warranted.
Myocardial Perfusion Scintigraphy
Myocardial perfusion scintigraphy (MPS) is a nuclear medicine technique that is used for around 50 years to assess regional left ventricular myocardial perfusion, diastolic and systolic function as well as to differentiate hibernating myocardium from transmural or non-transmural infarct. Three dimension images of myocardium are acquired after injecting radiopharmaceuticals with high first pass extraction by the myocardium using the single emission computed tomography (SPECT) technique. In majority of the centers the images are additionally corrected for attenuation correction using low dose CT images acquired simultaneously with SPECT images. As radioisotopes preferentially Technetium-99m (Tc-99m), specially in Europe and rarely Thallium-201 (Tl-201) are used (21). Tl-201 as a cyclotron product is injected as Thallium-201-Chloride. The Tc-99m is eluted from a generator and is either labeled with Sestamibi (CardioliteTM) or Tetrofosmin (MyoviewTM). Nowadays Tc-99m-labeled tracers are the tracer of choice because of more favorable imaging characteristics because of higher photon energy (140 keV compared to 69–83 keV) with less attenuation by the tissues around the heart, improved image quality and less radiation exposure (6 mSv vs. 17 mSv) compared to Tl-201 because of a shorter half-life (Tc-99m = 6 h, Tl-201 = 72 h). ECG-gated perfusion images allow the simultaneous assessment of perfusion as well as global and regional function of the left ventricle and are therefore an important tool in the diagnosis and management for CAD. To assess perfusion imaging a comparison between images at rest and images after stress is required. To replicate the normal physiological effect of strenuous exercise on perfusion, radiopharmaceuticals are injected after the patients underwent either physical stress (e.g., by a treadmill) or pharmacological stress (adenosine, regadenoson or dobutamine) tests. Thereafter the resting state images are acquired at a later time point. In patients without hemodynamically relevant myocardial ischemia, both stress and rest scans show a homogeneous distribution of the perfusion tracers. In comparison, patients with hemodynamically relevant stenosis of the coronary vessels, decreased segmental or subsegmental uptake of the tracer is seen in stress which however normalizes itself in the resting phase. A scar of the myocardium shows reduced or no uptake in the scar area both during stress and in rest conditions as a fixed defect.
The rarely used Tl-201 is a potassium analog and uses the Na/K ATPase system of viable myocardial cells. Its initial myocardial uptake is proportional to blood flow and it is rapidly cleared from the blood. After that up to 4 h a re-equilibration takes place when Tl-201 concentration levels are lower in the blood. This is also called redistribution and the process is directly proportional to blood flow to the area and viable myocardial cells. If the stress and rest images show matched homogeneous normal tracer uptake there is no sign of ischemia or infarction. If the tracer uptake during stress is abnormal but with normal uptake during rest / redistribution that is a sign for ischemia. Perfusion defects on both stress and rest images usually means there is a scar. To check that area for hibernating myocardium a reinjection of Tl-201 and another image acquisition after 18–24 h can be carried out. If there is Tl-201 uptake, there is hibernating myocardium in this area. If there is a scar no tracer uptake can be detected (37, 38).
The Tc-99m-labeled radiotracers are monovalent cations that enter cells through their lipophilic characteristics. Their uptake is also dependent on blood flow but as well as on electrochemical gradients of the plasma- and mitochondrial-membranes, the cellular pH and intact energy pathways. In the myocytes they are trapped mainly in the mitochondria with minimal washout and no redistribution in the blood. After the intravenous injection these tracers are first cleared by the liver and excreted through the bile. This makes it impossible to assess the myocardial uptake of the inferior wall right after injection and leads to a delay of 15–45 min before the start of the imaging acquisition, especially for Sestamibi. Tetrofosmin allows earlier imaging acquisition because of lower hepatic uptake. Stress and rest images can be acquired in 2-day and 1-day protocols that show no significant differences (21). For the 1-day protocol the stress images should be acquired first. If there is a homogenous tracer uptake the rest images can be avoided. In the 1-day protocols the injection for the second imaging (rest) higher amount of radioactivity is injected to overcome the shine through from the remaining radioactivity after stress. With the Tc-99m-labeled radiotracers perfusion and ischemia as well as viability can be examined but not hibernating myocardium (39). As Tl-201 is associated with higher radiation exposure, hibernating myocardium are best depicted by using a combination of Tc-99m Tetrofosmin or Sestamibi perfusion scan and F-18-FDG-PET.
The accurate interpretation of the myocardial perfusion imaging with SPECT is crucial. Absolute quantification of perfusion is more common with PET than with SPECT. According to the literature the sensitivity and the specificity of gated myocardial SPECT studies to diagnose clinically significant CAD with Tc-99m tracers is 88.3 and 75.8%, respectively (40). Cutoff values for the uptake of the tracers of > 50% of Tc-99m and > 60% of Tl-201 are commonly associated with regional functional recovery in CAD. However, in the detection of a viable hibernating myocardium, specificity is moderate (49–69%) and can lead to an overestimation of potential functional recovery (22). The presence of an ischemic area on a SPECT scan has shown a negative predictive value for cardiac events (41). In a trial involving patients with CTO of the left anterior descending artery, perfusion defects on SPECT scans before a PCI were associated with an improvement of clinical and functional markers, such as the 6-min walk test, left ventricular volumes and ejection fraction (42). The effect was stronger in patients with reversible perfusion defects than in patients with irreversible defects.
Visual interpretation of three vessels CAD is a challenge for nuclear cardiology field. However, with the development and standardization of protocols as well as advances in the scanner types (e.g., digital scanners or the even newer C-shaped heart-specific gamma cameras) there is a remarkable improvement in the quantification of myocardial wall thickening, contraction, and dilatation as well as in measurement of ejection fraction. Left ventricular dilatation in stress in comparison to rest can be quantified as transient ischemic dilatation (TID). A TID value above 1.3 is generally considered to be significant and can be used for assessment of three vessels disease (43).
However, the comparatively low spatial resolution of SPECT images compared to that of other imaging modalities is a potential disadvantage, as it can lead to overdiagnosis of subendocardial infarctions. Nevertheless, this is not a real disadvantage in the examination of patients with CTOs since transmurality is the main question in this case. There are new predefined criteria for differentiating between transmural and non-transmural infarction in SPECT images (44).
Overall, SPECT appears to be a useful examination modality for patients with CTOs. A radiation dose of 6–24 mSv, depending on the protocol and tracer used (lower radiation doses for Tc-99m and higher radiation doses for Tl-201) (21), as well as expertise, should be taken into consideration.
Positron Emission Tomography
Positron emission tomography (PET) is another nuclear medicine imaging technique that is in use for around 40 years in some specialized centers with a broader use during the last years. The main advantages of PET over SPECT are higher-quality images because of high-energy emitted photons (511 keV) with improved spatial and temporal resolution and shorter half-lives of the radioisotopes (mostly some minutes) as well as less radiation exposure (45, 46). By the use of F-18-FDG it is also possible to make the myocyte glucose metabolism visible. A combination of MPS and FDG-PET is important in the detection and risk stratification of CAD. Two examples for the detection of hibernating myocardium and the differentiation to scar are seen in Figures 1, 2. Additionally, perfusion PET also allows an improved functional evaluation of CAD because of a higher diagnostic accuracy and the possibility of measurements of myocardial blood flow (MBF) in absolute terms (milliliters per gram per minute). That makes it also possible to detect microvascular disease and balanced ischemia.
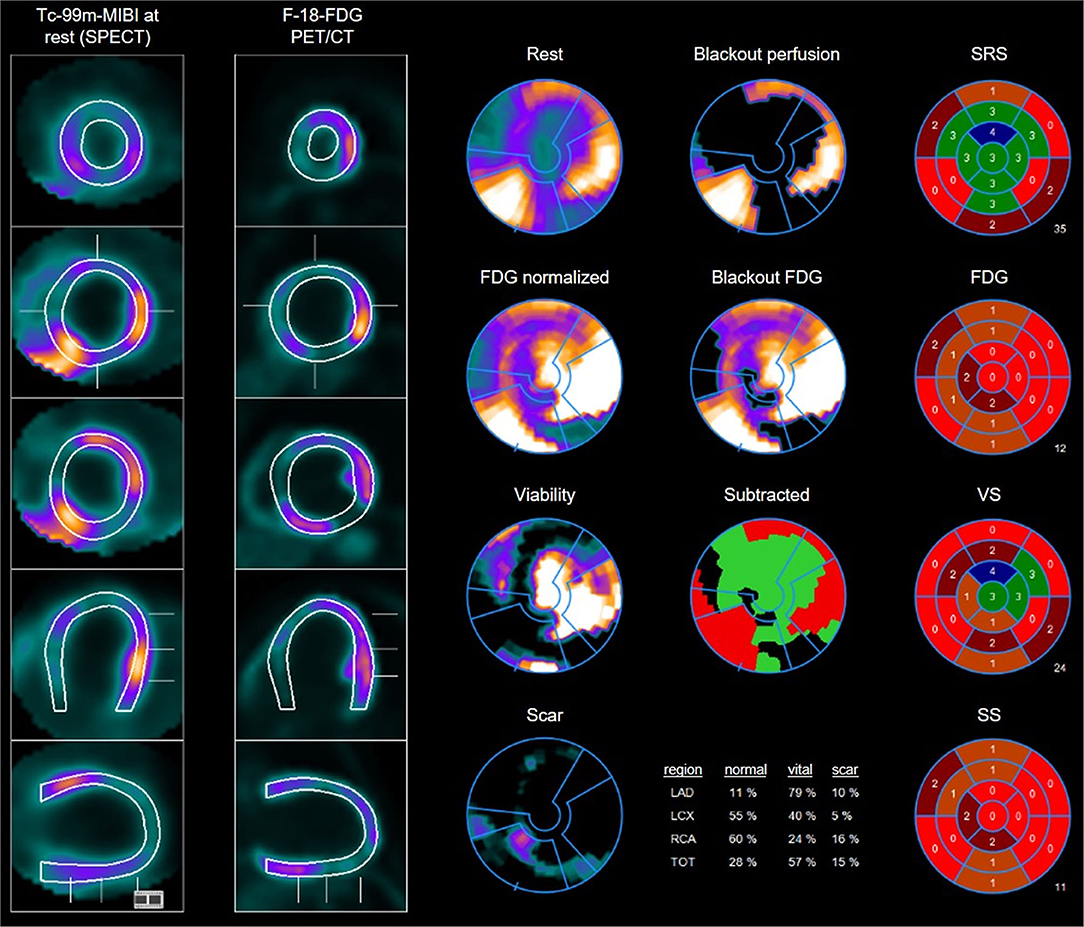
Figure 2. Example of multiple perfusion defect with hibernating myocardium as well as areas of scars: 45 years old male with perfusion defects in the anteroseptal wall as well as on the anterolateral and posterolateral wall in the Tc-99m-MIBI rest scan. FDG uptake in the viability F-18-FDG-PET/CT scan shows some uptake in the areas with perfusion defects resulting in the diagnosis of hibernating myocardium but also some areas with no FDG uptake especially septal and posterior resulting in the diagnosis of scars. Overview and polar maps with SRS, summed rest score; VS, vitality score; SS, scar score.
For the imaging with PET positron emitting radionuclides like Rubidium-82 (Rb-82), Oxygen-15 (O-15), Nitrogen-13 (N-13), and Flouride-18 (F-18) are used and incorporated into biochemical molecules. Rb-82 is a generator product, the other named radioisotopes are cyclotron products and for O-15 and N-13 with very short half-lives of 2 and 10 min, respectively, making an onsite cyclotron necessary. For perfusion imaging O-15 water, N-13 Ammonia, Rb-82 and F-18 Flurpiridaz are used with stress and rest imaging as in MPS. O-15 water is ideal for quantification of MBF in absolute terms. It can be seen as a one-compartment tracer kinetic model as it has no barrier effect form cellular membranes. Because of its very short half-life and poor contrast images between blood pool and the myocardium its use is limited. N-13 Ammonia is cationic and its first-pass extraction is related to blood flow for low flow rates. It reaches the cytosols via passive diffusion or active transport and is incorporated into the amino acid pool or diffuses back into the blood. Rb-82 is a potassium analog with a very short half-life of 75 s. During first-pass its extraction is not very high and builds a plateau with higher blood flow rates. F-18 Flurpiridaz is a novel PET mitochondrial complex-1 inhibitor in preparation with a half-life of 120 min. It has a high extraction rate with high flow and therefore makes absolute quantification of blood flow possible (47). As for SPECT tracer uptake between stress and rest images are compared to detect ischemia and scars.
For the differentiation of a perfusion defect in both stress and rest between a scar region and ischemic but viable region F-18-Fluorodeoxyglucose (FDG) is used. F-18-FDG competes with glucose for transport as well as phosphorylation by hexokinase. Once it is in the cytosol and phosphorylated it cannot be further processes by glycogen synthesis and is therefore trapped in the cell (45, 48). Its half-life of 110 min makes it easy to handle. To assess myocardial viability of a perfusion defect in stress and rest or in rest only (preferably from PET scans) an additional PET scan with F-18-FDG according to a special protocol can be performed. For non-diabetic and diabetic patients fasting for at least 6 h is required. After measurement of the serum glucose a protocol for glucose loading and insulin is required before F-18-FDG is administered intravenously (glucose <100 mg/dl 25–100 g oral load or dextrose infusion, later insulin is administered according to the glucose level, glucose <250 mg/dl only insulin is administered without an additional glucose load). In a viability study areas with highly reduced or absent perfusion but preserved or even enhanced metabolic activity Perfusion/metabolism mismatch, are hibernating but viable and have a high probability of regaining function after revascularization. Areas that show no perfusion during rest but do have preserved glucose metabolism are viable whereas the absence of glucose metabolism indicates non-viable myocardium. There are three different patterns which can be seen combining myocardial perfusion imaging at rest with F-18-FDG: (1) hibernating myocardium showing reduced perfusion with preserved FDG-uptake, (2) transmural scar without perfusion and without FDG-uptake, and (3) non-transmural scar with partially reduced perfusion of the myocardium with concordant FDG-uptake.
In CAD patients in general, an ischemia-driven revascularization approach guided by PET could result in a reduction in angina severity and a slight improvement in the left ventricular ejection fraction (49). Stulijfzand et al. who examined 69 CTO patients with O-15 water PET, found no correlation between the degree of ischemia and volumetric or functional improvements after CTO PCI but observed a significant ischemic burden reduction (13). In another study, this improvement in myocardial blood flow correlated to the defect size in a baseline PET examination (50). To summarize, PET imaging is a useful tool for evaluating ischemia and viability, with excellent spatial and temporal resolutions.
Computer Tomography
Computed tomography (CT) angiography has traditionally been used for anatomical evaluations of the coronary tree. With the advent of new-generation scanners with dual-source imaging, 64-row scanners, and modern software techniques, plaque morphology and regional calcium burden evaluations and functional assessments have also become possible (51, 52).
Since CTOs are found in many patients with known or suspected CAD, CT examinations can also contribute to their diagnosis. However, the distinction between a “true” CTO and subtotal stenosis constitutes a diagnostic difficulty. The distal lumen is often contrasted via collaterals, and the spatial resolution of CT is relatively low in comparison to classical angiography. Diagnostic markers such as a lesion length over 9 mm and the so-called reverse attenuation gradient sign have proven to be helpful, enabling a clear diagnosis in most cases (53–55). Apart from pure diagnostics, CT angiography is increasingly used for prognostic assessments and pre-procedural planning of coronary interventions and offers the possibility of follow-up restenosis assessments (56). Blooming artifacts are a limitation caused by severe calcification or stent struts, leading to a potential overestimation of lumen narrowing. This affects diagnosis of CTO as well as follow-up evaluation of the stent patency. Novel techniques and algorithms sought to overcome this limitation (57).
A key task of the pre-interventional examination of CTO patients is the abovementioned viability test. While cardiac CT was previously used for purely anatomical assessments of the coronary tree, it can also be used for hibernation assessments. These are based on classic measures, such as global and regional function, wall thickness, and wall thickening in systole, while assessment methods derived from CMR have not yet found their way into clinical routine. Reduced perfusion can be indicated by reduced contrast during the arterial phase. Similar to the flooding behavior of gadolinium-containing magnetic resonance imaging (MRI) contrast agents, delayed (late) enhancement with iodine-containing contrast media indicates myocardial scarring. A mismatch between underperfusion (arterial phase) and late enhancement indicates a potential intervention target (58). An additional late CT examination (5–15 min after the administration of the contrast medium) is associated with considerable radiation exposure. Currently, only data from experimental and animal studies showing good agreement with CMR scans are available (23, 52, 59, 60).
For the prediction of interventional recanalization success, the Computed Tomography Registry of Chronic Total Occlusion Revascularization (CT-RECTOR) score was established by analogy with angiography-based scores, such as the Japanese Multicenter CTO Registry (J-CTO) and Prospective Global Registry for the Study of Chronic Total Occlusion Intervention (PROGRESS-CTO) scores (61). It is calculated based on the presence of multiple occlusions, a blunt stump, severe calcification in the cross-sectional area of the occluded vessel, tortuosity, anamnestic information from a second attempt, and the presumed duration of the CTO. However, the J-CTO score has also shown applicability to CT angiographies (62).
The actual procedure can also be planned using CT angiography. Depending on factors such as good collateralization or the presence of an intensively calcified proximal cap, the access route (antegrade or retrograde) can be determined, or in the case of severe calcifications over short distances, an early switch to a stiffer wire can be decided. The anticipation of a need for rotablation or debulking devices is a potentially time-saving and patient-friendly factor (63). In a single-center study involving one interventionalist and 73 patients with CTOs, the use of pre-procedural CT angiography for planning significantly increased the recanalization rate in the matched analysis from 64 to 88% (64). In another study with 15 patients with pre-procedural CT planning and 59 patients in a purely classical angiographically controlled group, a stiff wire was chosen significantly more often as the initial wire, and there was less contrast exposure (65). An option after a CT scan that should not be neglected is referral to another center if there is no experience in the use of retrograde maneuvers or foreseeably necessary material is not available.
CT angiography can also be used to detect in-stent restenosis in follow-up investigations. However, as it has shown a high negative predictive value but a low positive predictive value (66–68), its clinical benefit in the case of frequent inconclusive findings, and thus the necessity of an invasive examination with double exposure to radiation and contrast media, must be further investigated in clinical trials. A combination with CT perfusion imaging or fractional flow reserve CT could further increase its value (69, 70).
In summary, pre-interventional multi-slice CT is a useful additional pre-interventional diagnostic tool. If necessary, it should be combined with other examination modalities to detect hibernation. Among all diagnostic modalities, CT is the closest to integrating diagnosis, risk calculation, intervention planning, and follow-up care in one modality.
Cardiovascular Magnetic Resonance
Cardiovascular magnetic resonance (CMR) is the gold standard for the evaluation of the left and right ventricular volumes and function, as well as ischemia and viability. A major advantage of CMR over nuclear and CT imaging is the lack of a need for ionizing radiation and the better tolerance of gadolinium-containing contrast media compared to iodine-containing contrast media. Its spatial and temporal resolutions are good thanks to modern sequences, such as balanced steady-state free precision imaging, ECG-gating, and breath holding techniques (71). Image quality is getting worse only in patients with severe arrhythmia or non-compliant patients. Real-time imaging without the need for breath holding is evolving to overcome these disadvantages (72).
Ischemia can be visualized using adenosine perfusion CMR. Less contrasted myocardial areas under vasodilator stress indicate a myocardium at risk (73). In CAD patients in general, adenosine perfusion imaging has shown potential for diagnosis and risk prediction (74, 75). Dobutamine stress can be used as an alternative to vasodilatory stress for risk assessment with comparable results (76, 77). However, the role of stress imaging in evaluating CTOs is controversial, as a study found a perfusion deficit in every CTO patient (78). Nevertheless, it can be argued that it may have its place in myocardium risk assessment. Perhaps the ongoing CARISMA_CTO study will provide more clarity on the matter (79).
Two modalities have been developed for viability assessment: low-dose dobutamine CMR (LDD-CMR) and LGE. LDD-CMR is the older method, but it has shown excellent results in predicting functional recovery after revascularization, even in direct comparison with other imaging techniques (80, 81). In low doses, dobutamine increases the regional function of an ischemic or hibernating myocardium (“contractile reserve”). Therefore, an increase in systolic wall thickness with low dobutamine doses is a predictor of functional recovery after revascularization in CAD patients in general. Conversely, in higher doses, the systolic function of an ischemic myocardium deteriorates. A combination with strain imaging is a promising method for improving diagnostic accuracy (82). To date, no studies have evaluated the utility of LDD-CMR prior to CTO revascularization.
LGE imaging is a valuable tool for distinguishing a hibernating myocardium from post-infarction scarring. Five to 15 min after the administration of a gadolinium-based contrast agent, focal deposits of gadolinium in the myocardium show a widening of the extracellular volume, indicating fibrosis and scarring, because of a delayed washout. LGE has shown a good correlation with PET examinations and excellent inter- and intra-observer agreement (83). In stable CAD, the combination of adenosine perfusion imaging and LGE has been shown to improve risk stratification and to help identify patients who will benefit from revascularization (74, 75, 84).
In a trial involving 59 patients with successful CTO PCIs, a pre-interventional LGE extent of <50% was associated with functional recovery of the related segment (85). In another study, CTO PCI was associated with an improvement of myocardial blood flow assessed using CMR and a reduction in the ischemic symptom burden (86). In that study, patients with a transmural LGE extent of more than 75% in most segments in the CTO territory were excluded. Although this approach seems obvious, it should not be seen as absolute, as the perfusion areas of the individual coronaries show significant variations between patients (87). Nevertheless, the two aforementioned studies suggest that a CMR-guided approach to CTO revascularization significantly improves regional blood flow and systolic function and sufficiently reduces patient symptoms. Accordingly, CMR imaging offers great benefits in the pre-interventional diagnostics of CTO patients, with a high prognostic value. An example of the combined use of CT and CMR in a patient with CTO is seen in Figure 3.
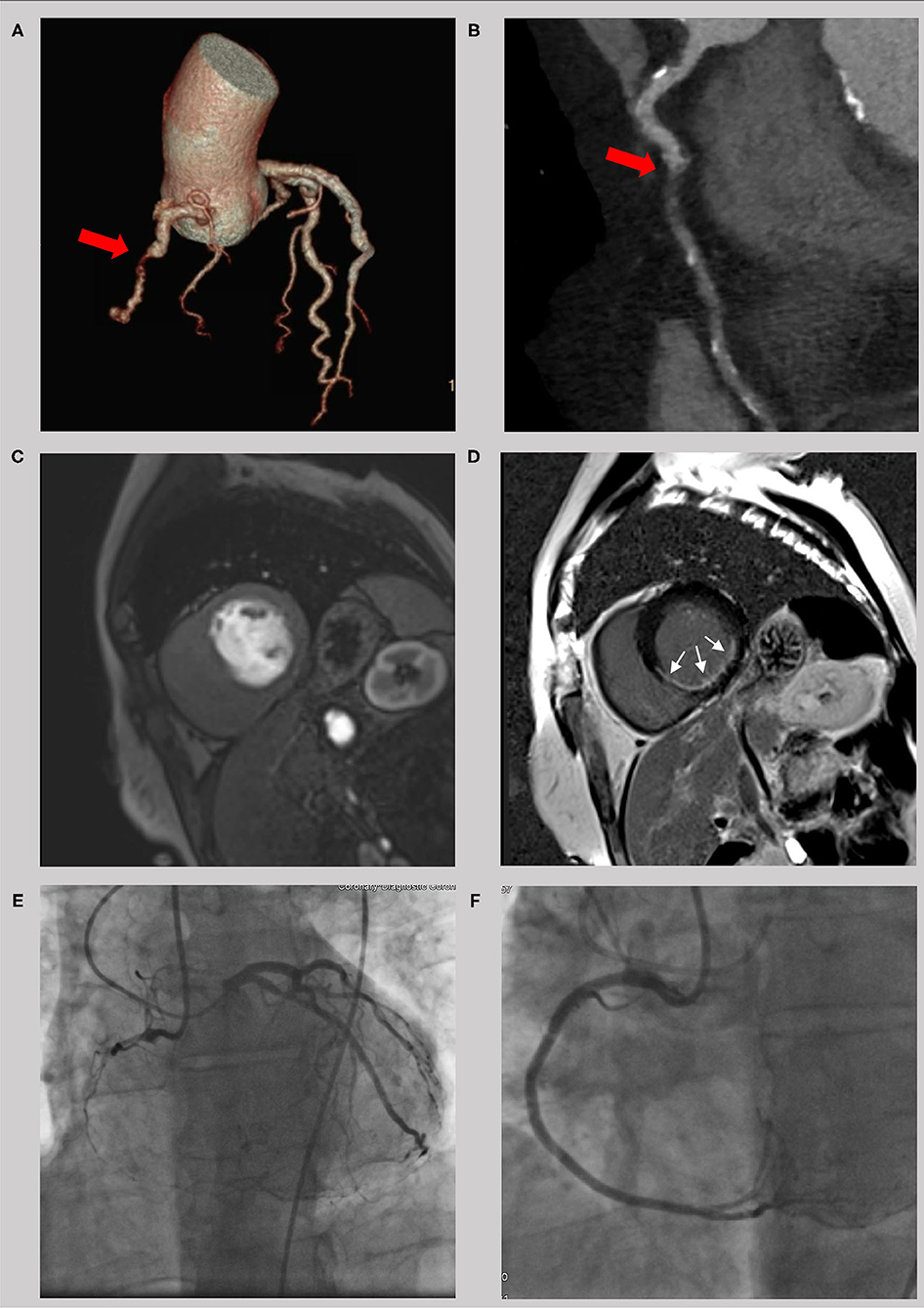
Figure 3. Example of a 56 year old male with a chronic total occlusion of the right coronary artery. The occlusion is seen in the computer tomography coronary angiogram (A and B, red cross) with a short occlusion length and a small amount of calcification. Because of an impaired regional function with thinning of the left ventricular wall, imaging of ischemia (C, first-pass perfusion) and scarring (D, late gadolinium enhancement) was done using cardiac magnetic resonance. With a LGE of <50% transmurality (white crosses) and a high symptom burden, interventional revascularization was successfully performed (E,F) with significant clinical improvement.
For even better workups, new techniques, such as parametric mapping, are currently under investigation. In a first study, the extracellular volume was found to be superior to LGE for functional recovery assessments (88).
Using CMR, patients have already been examined after complex PCI in order to investigate the extent and clinical impact of a peri-interventional infarction. This revealed a relevant proportion of patients with new LGE after PCI, which in turn had a negative impact on the clinical outcome (89). Techniques that are particularly prone to infarction, such as rotablation, could therefore have a negative impact on endpoints in CTO studies (90). Here CMR offers the potential for further investigations.
Outlook
A central point of future developments, besides a further increase in diagnostic accuracy, will be a reduction in patient exposure to contrast media or radiation. Since CMR typically works without radiation, a further improvement of CMR coronary angiography would be an obvious direction in the quest to overcome the disadvantages of CT in CTO intervention planning. CMR coronary angiography is already feasible for the evaluation of plaque morphology and composition (91–93). At the same time, radiation exposure in SPECT, PET, and CT imaging is now only a fraction of the historically required doses thanks to the constant development of new detectors, collimators, techniques, and software.
Another goal for further improvement of interventional outcomes is the integration of imaging modalities into the actual intervention. Opolski et al. demonstrated the feasibility and safety of an augmented-reality glass that provides the interventional cardiologist with additional information from coronary CT angiography (65). This is yet another indication that CT is the closest to integrating diagnostics, prognostic assessment, planning, and follow-up care.
As already discussed, each modality has its own strengths and weaknesses. Therefore, fusion imaging, such as PET/CT or PET/MRI, could improve the pre-interventional workup of CTO patients. For example, a combination of morphological assessments using CT with metabolic assessments using PET could improve patient selection (94). In a first human study using PET/MRI fusion scans prior to CTO PCI in 49 patients, the combined images predicted functional improvement more accurately than PET or CMR alone (95). However, their high costs and limited access in daily care are clear limitations of these techniques.
In summary, the use of non-invasive imaging for ischemia and viability assessments before interventional recanalization of a CTO is desirable. Although some imaging techniques have clear advantages over others, the selection depends mainly on regional availability and expertise. Better patient selection and prediction of interventional success should be the target of future prospective studies.
Author Contributions
JK and DB: concept and writing of the manuscript. NE and VP: writing of the SPECT and PET section, proofreading, and image examples. MK, SM, JM, NN, TS, MT, and TT: interpretation of the sources, proofreading, and image example. WR: supervision, concept, and proofreading. All authors contributed to the article and approved the submitted version.
Conflict of Interest
The authors declare that the research was conducted in the absence of any commercial or financial relationships that could be construed as a potential conflict of interest.
Publisher's Note
All claims expressed in this article are solely those of the authors and do not necessarily represent those of their affiliated organizations, or those of the publisher, the editors and the reviewers. Any product that may be evaluated in this article, or claim that may be made by its manufacturer, is not guaranteed or endorsed by the publisher.
References
1. Suero JA, Marso SP, Jones PG, Laster SB, Huber KC, Giorgi LV, et al. Procedural outcomes and long-term survival among patients undergoing percutaneous coronary intervention of a chronic total occlusion in native coronary arteries: a 20-year experience. J Am College Cardiol. (2001) 38:409–14. doi: 10.1016/s0735-1097(01)01349-3
2. Teng H-I, Sung S-H, Huang S-S, Pan J-P, Lin S-J, Chan W-L, et al. The impact of successful revascularization of coronary chronic total occlusions on long-term clinical outcomes in patients with non-ST-segment elevation myocardial infarction. J Interv Cardiol. (2018) 31:302–9. doi: 10.1111/joic.12501
3. Khan MF, Wendel CS, Thai HM, Movahed MR. Effects of percutaneous revascularization of chronic total occlusions on clinical outcomes: a meta-analysis comparing successful versus failed percutaneous intervention for chronic total occlusion. Catheter Cardiovasc Interv. (2013) 82:95–107. doi: 10.1002/ccd.24863
4. Werner GS, Gitt AK, Zeymer U, Juenger C, Towae F, Wienbergen H, et al. Chronic total coronary occlusions in patients with stable angina pectoris: impact on therapy and outcome in present day clinical practice. Clin Res Cardiol. (2009) 98:435–41. doi: 10.1007/s00392-009-0013-5
5. Werner GS, Martin-Yuste V, Hildick-Smith D, Boudou N, Sianos G, Gelev V, et al. A randomized multicentre trial to compare revascularization with optimal medical therapy for the treatment of chronic total coronary occlusions. Eur Heart J. (2018) 39:2484–93. doi: 10.1093/eurheartj/ehy220
6. Mashayekhi K, Nührenberg TG, Toma A, Gick M, Ferenc M, Hochholzer W, et al. A randomized trial to assess regional left ventricular function after stent implantation in chronic total occlusion: the REVASC Trial. JACC Cardiovasc Interv. (2018) 11:1982–91. doi: 10.1016/j.jcin.2018.05.041
7. Brilakis ES, Mashayekhi K, Tsuchikane E, Abi Rafeh N, Alaswad K, Araya M, et al. Guiding principles for chronic total occlusion percutaneous coronary intervention. Circulation. (2019) 140:420–33. doi: 10.1161/CIRCULATIONAHA.119.039797
8. Neumann F-J, Sousa-Uva M, Ahlsson A, Alfonso F, Banning AP, Benedetto U, et al. 2018 ESC/EACTS Guidelines on myocardial revascularization. Eur Heart J. (2019) 40:87–165. doi: 10.1093/eurheartj/ehy394
9. Patel MR, Calhoon JH, Dehmer GJ, Grantham JA, Maddox TM, Maron DJ, et al. ACC/AATS/AHA/ASE/ASNC/SCAI/SCCT/STS 2017 appropriate use criteria for coronary revascularization in patients with stable ischemic heart disease. J Am College Cardiol. (2017) 69:2212–41. doi: 10.1016/j.jacc.2017.02.001
10. Levine GN, Bates ER, Blankenship JC, Bailey SR, Bittl JA, Cercek B, et al. 2011 ACCF/AHA/SCAI Guideline for percutaneous coronary intervention: a report of the American College of Cardiology Foundation/American Heart Association Task Force on Practice Guidelines and the Society for Cardiovascular Angiography and Interventions. Circulation. (2011) 124:e574–651. doi: 10.1161/CIR.0b013e31823ba622
11. Shaw LJ, Berman DS, Maron DJ, Mancini GB, Hayes SW, Hartigan PM, et al. Optimal medical therapy with or without percutaneous coronary intervention to reduce ischemic burden: results from the clinical outcomes utilizing revascularization and aggressive drug evaluation (COURAGE) trial nuclear substudy. Circulation. (2008) 117:1283–91. doi: 10.1161/CIRCULATIONAHA.107.743963
12. Sachdeva R, Agrawal M, Flynn SE, Werner GS, Uretsky BF. The myocardium supplied by a chronic total occlusion is a persistently ischemic zone. Catheter Cardiovasc Interv. (2014) 83:9–16. doi: 10.1002/ccd.25001
13. Stuijfzand WJ, Biesbroek PS, Raijmakers PG, Driessen RS, Schumacher SP, van Diemen P, et al. Effects of successful percutaneous coronary intervention of chronic total occlusions on myocardial perfusion and left ventricular function. EuroIntervention. (2017) 13:345–54. doi: 10.4244/EIJ-D-16-01014
14. Maron DJ, Hochman JS, Reynolds HR, Bangalore S, O'Brien SM, Boden WE, et al. Initial invasive or conservative strategy for stable coronary disease. N Engl J Med. (2020) 382:1395–407. doi: 10.1056/NEJMoa1915922
15. Luca L, Uguccioni M, Meessen J, Temporelli PL, Tomai F, Rosa FM, et al. External applicability of the ISCHEMIA trial: an analysis of a prospective, nationwide registry of patients with stable coronary artery disease. EuroIntervention. (2020) 16:e966–73. doi: 10.4244/EIJ-D-20-00610
16. Schumacher SP, Everaars H, Stuijfzand WJ, Huynh JW, van Diemen PA, Bom MJ, et al. Coronary collaterals and myocardial viability in patients with chronic total occlusions. EuroIntervention. (2020) 16:e453–61. doi: 10.4244/EIJ-D-19-01006
17. Dong W, Li J, Mi H, Song X, Jiao J, Li Q. Relationship between collateral circulation and myocardial viability of 18F-FDG PET/CT subtended by chronic total occluded coronary arteries. Ann Nucl Med. (2018) 32:197–205. doi: 10.1007/s12149-018-1234-3
18. Al-Mohammad A, Norton MY, Mahy IR, Patel JC, Welch AE, Mikecz P, et al. Can the surface electrocardiogram be used to predict myocardial viability? Heart. (1999) 82:663–7. doi: 10.1136/hrt.82.6.663
19. Haft JI, Hammoudeh AJ, Conte PJ. Assessing myocardial viability: Correlation of myocardial wall motion abnormalities and pathologic Q waves with technetium 99m sestamibi single photon emission computed tomography. Am Heart J. (1995) 130:994–8. doi: 10.1016/0002-8703(95)90199-X
20. Schinkel AFL, Bax JJ, Elhendy A, Boersma E, Vourvouri EC, Sozzi FB, et al. Assessment of viable tissue in Q-wave regions by metabolic imaging using single-photon emission computed tomography in ischemic cardiomyopathy. Am J Cardiol. (2002) 89:1171–5. doi: 10.1016/S0002-9149(02)02299-3
21. Henzlova MJ, Duvall WL, Einstein AJ, Travin MI, Verberne HJ. ASNC imaging guidelines for SPECT nuclear cardiology procedures: stress, protocols, and tracers. J Nucl Cardiol. (2016) 23:606–39. doi: 10.1007/s12350-015-0387-x
22. Bax JJ, Wijns W, Cornel JH, Visser FC, Boersma E, Fioretti PM. Accuracy of currently available techniques for prediction of functional recovery after revascularization in patients with left ventricular dysfunction due to chronic coronary artery disease: comparison of pooled data. J Am College Cardiol. (1997) 30:1451–60. doi: 10.1016/s0735-1097(97)00352-5
23. Matsuda T, Kido T, Itoh T, Saeki H, Shigemi S, Watanabe K, et al. Diagnostic accuracy of late iodine enhancement on cardiac computed tomography with a denoise filter for the evaluation of myocardial infarction. Int J Cardiovasc Imaging. (2015) 31(Suppl. 2):177–85. doi: 10.1007/s10554-015-0716-9
24. Case JA, deKemp RA, Slomka PJ, Smith MF, Heller GV, Cerqueira MD. Status of cardiovascular PET radiation exposure and strategies for reduction: an information statement from the cardiovascular PET task force. J Nucl Cardiol. (2017) 24:1427–39. doi: 10.1007/s12350-017-0897-9
25. Romero J, Xue X, Gonzalez W, Garcia MJ. CMR imaging assessing viability in patients with chronic ventricular dysfunction due to coronary artery disease: a meta-analysis of prospective trials. JACC Cardiovasc Imaging. (2012) 5:494–508. doi: 10.1016/j.jcmg.2012.02.009
26. Schinkel AF, Bax JJ, Poldermans D, Elhendy A, Ferrari R, Rahimtoola SH. Hibernating myocardium: diagnosis and patient outcomes. Curr Probl Cardiol. (2007) 32:375–410. doi: 10.1016/j.cpcardiol.2007.04.001
27. Cigarroa CG, deFilippi CR, Brickner ME, Alvarez LG, Wait MA, Grayburn PA. Dobutamine stress echocardiography identifies hibernating myocardium and predicts recovery of left ventricular function after coronary revascularization. Circulation. (1993) 88:430–6. doi: 10.1161/01.cir.88.2.430
28. Krahwinkel W, Ketteler T, Wolfertz J, Gödke J, Krakau I, Ulbricht LJ, et al. Detection of myocardial viability using stress echocardiography. Eur Heart J. (1997) 18(Suppl. D):D111–6. doi: 10.1093/eurheartj/18.suppl_D.111
29. La Canna G, Alfieri O, Giubbini R, Gargano M, Ferrari R, Visioli O. Echocardiography during infusion of dobutamine for identification of reversible dyfunction in patients with chronic coronary artery disease. J Am College Cardiol. (1994) 23:617–26. doi: 10.1016/0735-1097(94)90745-5
30. Galatro K, Chaudhry FA. Prognostic implications of myocardial contractile reserve in patients with ischemic cardiomyopathy. Echocardiography. (2000) 17:61–7. doi: 10.1111/j.1540-8175.2000.tb00996.x
31. Afridi I, Grayburn PA, Panza JA, Oh JK, Zoghbi WA, Marwick TH. Myocardial viability during dobutamine echocardiography predicts survival in patients with coronary artery disease and severe left ventricular systolic dysfunction. J Am College Cardiol. (1998) 32:921–6. doi: 10.1016/S0735-1097(98)00321-0
32. Sicari R, Picano E, Cortigiani L, Borges AC, Varga A, Palagi C, et al. Prognostic value of myocardial viability recognized by low-dose dobutamine echocardiography in chronic ischemic left ventricular dysfunction. Am J Cardiol. (2003) 92:1263–6. doi: 10.1016/j.amjcard.2003.08.004
33. Mertes H, Sawada SG, Ryan T, Segar DS, Kovacs R, Foltz J, et al. Symptoms, adverse effects, and complications associated with dobutamine stress echocardiography. Experience in 1118 patients. Circulation. (1993) 88:15–9. doi: 10.1161/01.cir.88.1.15
34. Sicari R, Nihoyannopoulos P, Evangelista A, Kasprzak J, Lancellotti P, Poldermans D, et al. Stress echocardiography expert consensus statement–executive summary: European association of echocardiography (EAE) (a registered branch of the ESC). Eur Heart J. (2009) 30:278–89. doi: 10.1093/eurheartj/ehn492
35. Rösner A, Avenarius D, Malm S, Iqbal A, Bijnens B, Schirmer H. Severe regional myocardial dysfunction by stress echocardiography does not predict the presence of transmural scarring in chronic coronary artery disease. Eur Heart J Cardiovasc Imaging. (2015) 16:1074–81. doi: 10.1093/ehjci/jev096
36. Vitarelli A, Montesano T, Gaudio C, Conde Y, Cimino E, D'angeli I, et al. Strain rate dobutamine echocardiography for prediction of recovery after revascularization in patients with ischemic left ventricular dysfunction. J Card Fail. (2006) 12:268–75. doi: 10.1016/j.cardfail.2006.02.003
37. Kitsiou AN, Srinivasan G, Quyyumi AA, Summers RM, Bacharach SL, Dilsizian V. Stress-induced reversible and mild-to-moderate irreversible thallium defects: are they equally accurate for predicting recovery of regional left ventricular function after revascularization? Circulation. (1998) 98:501–8. doi: 10.1161/01.cir.98.6.501
38. Zimmermann R, Mall G, Rauch B, Zimmer G, Gabel M, Zehelein J, et al. Residual 201Tl activity in irreversible defects as a marker of myocardial viability. Clinicopathological study. Circulation. (1995) 91:1016–21. doi: 10.1161/01.cir.91.4.1016
39. Maes AF, Borgers PM, Flameng W, Nuyts JL, van de Werf F, Ausma JJ, et al. Assessment of myocardial viability in chronic coronary artery disease using technetium-99m Sestamibi SPECT. J Am College Cardiol. (1997) 29:62–8. doi: 10.1016/S0735-1097(96)00442-1
40. Parker MW, Iskandar A, Limone B, Perugini A, Kim H, Jones C, et al. Diagnostic accuracy of cardiac positron emission tomography versus single photon emission computed tomography for coronary artery disease: a bivariate meta-analysis. Circ Cardiovasc Imaging. (2012) 5:700–7. doi: 10.1161/CIRCIMAGING.112.978270
41. Galassi AR, Werner GS, Tomasello SD, Azzarelli S, Capodanno D, Barrano G, et al. Prognostic value of exercise myocardial scintigraphy in patients with coronary chronic total occlusions. J Interv Cardiol. (2010) 23:139–48. doi: 10.1111/j.1540-8183.2010.00527.x
42. Sun D, Wang J, Tian Y, Narsinh K, Wang H, Li C, et al. Multimodality imaging evaluation of functional and clinical benefits of percutaneous coronary intervention in patients with chronic total occlusion lesion. Theranostics. (2012) 2:788–800. doi: 10.7150/thno.4717
43. Katz JS, Ruisi M, Giedd KN, Rachko M. Assessment of transient ischemic dilation (TID) ratio in gated SPECT myocardial perfusion imaging (MPI) using regadenoson, a new agent for pharmacologic stress testing. J Nucl Cardiol. (2012) 19:727–34. doi: 10.1007/s12350-012-9559-0
44. Dilsizian V. Interpretation and clinical management of patients with “Fixed” myocardial perfusion defects A call for quantifying endocardial-to-epicardial distribution of blood flow. J Nucl Cardiol. (2021) 28:723–8. doi: 10.1007/s12350-020-02492-8
45. Dilsizian V, Bacharach SL, Beanlands RS, Bergmann SR, Delbeke D, Dorbala S, et al. ASNC imaging guidelines/SNMMI procedure standard for positron emission tomography (PET) nuclear cardiology procedures. J Nucl Cardiol. (2016) 23:1187–226. doi: 10.1007/s12350-016-0522-3
46. Bateman TM, Heller GV, McGhie AI, Friedman JD, Case JA, Bryngelson JR, et al. Diagnostic accuracy of rest/stress ECG-gated Rb-82 myocardial perfusion PET: comparison with ECG-gated Tc-99m sestamibi SPECT. J Nucl Cardiol. (2006) 13:24–33. doi: 10.1016/j.nuclcard.2005.12.004
47. Maddahi J, Lazewatsky J, Udelson JE, Berman DS, Beanlands RS, Heller GV, et al. Phase-III clinical trial of fluorine-18 flurpiridaz positron emission tomography for evaluation of coronary artery disease. J Am College Cardiol. (2020) 76:391–401. doi: 10.1016/j.jacc.2020.05.063
48. Underwood SR, Bax JJ, vom Dahl J, Henein MY, Knuuti J, van Rossum AC, et al. Imaging techniques for the assessment of myocardial hibernation. Report of a Study Group of the European Society of Cardiology. Eur Heart J. (2004) 25:815–36. doi: 10.1016/j.ehj.2004.03.012
49. Eitzman D, al-Aouar Z, Kanter HL, vom Dahl J, Kirsh M, Deeb GM, et al. Clinical outcome of patients with advanced coronary artery disease after viability studies with positron emission tomography. J Am College Cardiol. (1992) 20:559–65. doi: 10.1016/0735-1097(92)90008-b
50. Schumacher SP, Kockx M, Stuijfzand WJ, Driessen RS, van Diemen PA, Bom MJ, et al. Ischaemic burden and changes in absolute myocardial perfusion after chronic total occlusion percutaneous coronary intervention. EuroIntervention. (2020) 2020:e462–71. doi: 10.4244/EIJ-D-19-00631
51. Abdelmoneim I, Sadek A, Mosaad MA, Yassin I, Radwan Y, Shokry K, et al. Diagnostic accuracy of multi-slice computed tomography in identifying lesion characteristics in coronary total occlusion. Int J Cardiovasc Imaging. (2018) 34:1813–8. doi: 10.1007/s10554-018-1392-3
52. Hoe J. CT coronary angiography of chronic total occlusions of the coronary arteries: how to recognize and evaluate and usefulness for planning percutaneous coronary interventions. Int J Cardiovasc Imaging. (2009) 25(Suppl. 1):43–54. doi: 10.1007/s10554-009-9424-7
53. von Erffa J, Ropers D, Pflederer T, Schmid M, Marwan M, Daniel WG, et al. Differentiation of total occlusion and high-grade stenosis in coronary CT angiography. Eur Radiol. (2008) 18:2770–5. doi: 10.1007/s00330-008-1068-9
54. Li M, Zhang J, Pan J, Lu Z. Obstructive coronary artery disease: reverse attenuation gradient sign at CT indicates distal retrograde flow–a useful sign for differentiating chronic total occlusion from subtotal occlusion. Radiology. (2013) 266:766–72. doi: 10.1148/radiol.12121294
55. Choi J-H, Kim E-K, Kim SM, Kim H, Song YB, Hahn J-Y, et al. Noninvasive discrimination of coronary chronic total occlusion and subtotal occlusion by coronary computed tomography angiography. JACC Cardiovasc Interv. (2015) 8:1143–53. doi: 10.1016/j.jcin.2015.03.042
56. Opolski MP, Achenbach S. CT angiography for revascularization of CTO: crossing the borders of diagnosis and treatment. JACC Cardiovasc Imaging. (2015) 8:846–58. doi: 10.1016/j.jcmg.2015.05.001
57. Li P, Xu L, Yang L, Wang R, Hsieh J, Sun Z, et al. Blooming artifact reduction in coronary artery calcification by a new de-blooming algorithm: initial study. Sci Rep. (2018) 8:6945. doi: 10.1038/s41598-018-25352-5
58. Mahnken AH, Mühlenbruch G, Günther RW, Wildberger JE. CT imaging of myocardial viability: experimental and clinical evidence. Cardiovasc J Afr. (2007) 18:169–74.
59. Mahnken AH, Bruners P, Kinzel S, Katoh M, Mühlenbruch G, Günther RW, et al. Late-phase MSCT in the different stages of myocardial infarction: animal experiments. Eur Radiol. (2007) 17:2310–7. doi: 10.1007/s00330-006-0569-7
60. Buecker A, Katoh M, Krombach GA, Spuentrup E, Bruners P, Günther RW, et al. A feasibility study of contrast enhancement of acute myocardial infarction in multislice computed tomography: comparison with magnetic resonance imaging and gross morphology in pigs. Invest Radiol. (2005) 40:700–4. doi: 10.1097/01.rli.0000179524.58411.a2
61. Opolski MP, Achenbach S, Schuhbäck A, Rolf A, Möllmann H, Nef H, et al. Coronary computed tomographic prediction rule for time-efficient guidewire crossing through chronic total occlusion: insights from the CT-RECTOR multicenter registry (Computed Tomography Registry of Chronic Total Occlusion Revascularization). JACC Cardiovasc Interv. (2015) 8:257–67. doi: 10.1016/j.jcin.2014.07.031
62. Fujino A, Otsuji S, Hasegawa K, Arita T, Takiuchi S, Fujii K, et al. Accuracy of J-CTO score derived from computed tomography versus angiography to predict successful percutaneous coronary intervention. JACC Cardiovasc Imaging. (2018) 11:209–17. doi: 10.1016/j.jcmg.2017.01.028
63. Magro M, Schultz C, Simsek C, Garcia-Garcia HM, Regar E, Nieman K, et al. Computed tomography as a tool for percutaneous coronary intervention of chronic total occlusions. EuroIntervention. (2010) 6(Suppl. G):G123–31.
64. Rolf A, Werner GS, Schuhbäck A, Rixe J, Möllmann H, Nef HM, et al. Preprocedural coronary CT angiography significantly improves success rates of PCI for chronic total occlusion. Int J Cardiovasc Imaging. (2013) 29:1819–27. doi: 10.1007/s10554-013-0258-y
65. Opolski MP, Debski A, Borucki BA, Staruch AD, Kepka C, Rokicki JK, et al. Feasibility and safety of augmented-reality glass for computed tomography-assisted percutaneous revascularization of coronary chronic total occlusion: A single center prospective pilot study. J Cardiovasc Comput Tomogr. (2017) 11:489–96. doi: 10.1016/j.jcct.2017.09.013
66. Kumbhani DJ, Ingelmo CP, Schoenhagen P, Curtin RJ, Flamm SD, Desai MY. Meta-analysis of diagnostic efficacy of 64-slice computed tomography in the evaluation of coronary in-stent restenosis. Am J Cardiol. (2009) 103:1675–81. doi: 10.1016/j.amjcard.2009.02.024
67. Wan Y-L, Tsay P-K, Chen C-C, Juan Y-H, Huang Y-C, Chan W-H, et al. Coronary in-stent restenosis: predisposing clinical and stent-related factors, diagnostic performance and analyses of inaccuracies in 320-row computed tomography angiography. Int J Cardiovasc Imaging. (2016) 32(Suppl. 1):105–15. doi: 10.1007/s10554-016-0872-6
68. Eckert J, Renczes-Janetzko P, Schmidt M, Magedanz A, Voigtländer T, Schmermund A. Coronary CT angiography (CCTA) using third-generation dual-source CT for ruling out in-stent restenosis. Clin Res Cardiol. (2019) 108:402–10. doi: 10.1007/s00392-018-1369-1
69. Andreini D, Mushtaq S, Pontone G, Conte E, Collet C, Sonck J, et al. CT perfusion versus coronary CT angiography in patients with suspected in-stent restenosis or CAD progression. JACC Cardiovasc Imaging. (2020) 13:732–42. doi: 10.1016/j.jcmg.2019.05.031
70. Andreini D, Mushtaq S, Pontone G, Rogers C, Pepi M, Bartorelli AL. Severe in-stent restenosis missed by coronary CT angiography and accurately detected with FFRCT. Int J Cardiovasc Imaging. (2017) 33:119–20. doi: 10.1007/s10554-016-0971-4
71. Attili AK, Schuster A, Nagel E, Reiber JH, van der Geest RJ. Quantification in cardiac MRI: advances in image acquisition and processing. Int J Cardiovasc Imaging. (2010) 26(Suppl. 1):27–40. doi: 10.1007/s10554-009-9571-x
72. Li H, Metze P, Abaei A, Rottbauer W, Just S, Lu Q, et al. Feasibility of real-time cardiac MRI in mice using tiny golden angle radial sparse. NMR Biomed. (2020) 33:e4300. doi: 10.1002/nbm.4300
73. Nagel E, Klein C, Paetsch I, Hettwer S, Schnackenburg B, Wegscheider K, et al. Magnetic resonance perfusion measurements for the noninvasive detection of coronary artery disease. Circulation. (2003) 108:432–7. doi: 10.1161/01.CIR.0000080915.35024.A9
74. Buckert D, Dewes P, Walcher T, Rottbauer W, Bernhardt P. Intermediate-term prognostic value of reversible perfusion deficit diagnosed by adenosine CMR: a prospective follow-up study in a consecutive patient population. JACC Cardiovasc Imaging. (2013) 6:56–63. doi: 10.1016/j.jcmg.2012.08.011
75. Buckert D, Witzel S, Steinacker JM, Rottbauer W, Bernhardt P. Comparing cardiac magnetic resonance-guided versus angiography-guided treatment of patients with stable coronary artery disease: results from a prospective randomized controlled trial. JACC Cardiovasc Imaging. (2018) 11:987–96. doi: 10.1016/j.jcmg.2018.05.007
76. Jahnke C, Nagel E, Gebker R, Kokocinski T, Kelle S, Manka R, et al. Prognostic value of cardiac magnetic resonance stress tests: adenosine stress perfusion and dobutamine stress wall motion imaging. Circulation. (2007) 115:1769–76. doi: 10.1161/CIRCULATIONAHA.106.652016
77. Stacey RB, Vera T, Morgan TM, Jordan JH, Whitlock MC, Hall ME, et al. Asymptomatic myocardial ischemia forecasts adverse events in cardiovascular magnetic resonance dobutamine stress testing of high-risk middle-aged and elderly individuals. J Cardiovasc Magn Reson. (2018) 20:75. doi: 10.1186/s12968-018-0492-5
78. Monti L, Di Giovine G, Scardino C, Nardi B, Balzarini L, Gasparini G. Role of adenosine stress CMR before chronic total occlusion reopening. J Cardiovasc Magn Reson. (2016) 18:80. doi: 10.1186/1532-429X-18-S1-P80
79. Pica S, Di Giovine G, Bollati M, Testa L, Bedogni F, Camporeale A, et al. Cardiac magnetic resonance for ischaemia and viability detection. Guiding patient selection to revascularization in coronary chronic total occlusions: The CARISMA_CTO study design. Int J Cardiol. (2018) 272:356–62. doi: 10.1016/j.ijcard.2018.08.061
80. Baer FM, Voth E, LaRosée K, Schneider CA, Theissen P, Deutsch HJ, et al. Comparison of dobutamine transesophageal echocardiography and dobutamine magnetic resonance imaging for detection of residual myocardial viability. Am J Cardiol. (1996) 78:415–9. doi: 10.1016/s0002-9149(96)00329-3
81. Baer FM, Theissen P, Schneider CA, Voth E, Sechtem U, Schicha H, et al. Dobutamine magnetic resonance imaging predicts contractile recovery of chronically dysfunctional myocardium after successful revascularization. J Am College Cardiol. (1998) 31:1040–8. doi: 10.1016/s0735-1097(98)00032-1
82. Schuster A, Kutty S, Padiyath A, Parish V, Gribben P, Danford DA, et al. Cardiovascular magnetic resonance myocardial feature tracking detects quantitative wall motion during dobutamine stress. J Cardiovasc Magn Reson. (2011) 13:58. doi: 10.1186/1532-429X-13-58
83. Zhang LJ, Dong W, Li JN, Mi HZ, Jiao J, Dou RY, et al. Quantification of late gadolinium enhancement cardiovascular MRI in patients with coronary artery chronic total occlusion. Clin Radiol. (2020) 75:643.e19-643.e26. doi: 10.1016/j.crad.2020.03.032
84. Bernhardt P, Spiess J, Levenson B, Pilz G, Höfling B, Hombach V, et al. Combined assessment of myocardial perfusion and late gadolinium enhancement in patients after percutaneous coronary intervention or bypass grafts: a multicenter study of an integrated cardiovascular magnetic resonance protocol. JACC Cardiovasc Imaging. (2009) 2:1292–300. doi: 10.1016/j.jcmg.2009.05.011
85. Nakachi T, Kato S, Kirigaya H, Iinuma N, Fukui K, Saito N, et al. Prediction of functional recovery after percutaneous coronary revascularization for chronic total occlusion using late gadolinium enhanced magnetic resonance imaging. J Cardiol. (2017) 69:836–42. doi: 10.1016/j.jjcc.2017.01.002
86. Bucciarelli-Ducci C, Auger D, Di Mario C, Locca D, Petryka J, O'Hanlon R, et al. CMR guidance for recanalization of coronary chronic total occlusion. JACC Cardiovasc Imaging. (2016) 9:547–56. doi: 10.1016/j.jcmg.2015.10.025
87. Ortiz-Pérez JT, Rodríguez J, Meyers SN, Lee DC, Davidson C, Wu E. Correspondence between the 17-segment model and coronary arterial anatomy using contrast-enhanced cardiac magnetic resonance imaging. JACC Cardiovasc Imaging. (2008) 1:282–93. doi: 10.1016/j.jcmg.2008.01.014
88. Chen Y, Zheng X, Jin H, Deng S, Ren D, Greiser A, et al. Role of myocardial extracellular volume fraction measured with magnetic resonance imaging in the prediction of left ventricular functional outcome after revascularization of chronic total occlusion of coronary arteries. Korean J Radiol. (2019) 20:83–93. doi: 10.3348/kjr.2018.0069
89. Rahimi K, Banning AP, Cheng AS, Pegg TJ, Karamitsos TD, Channon KM, et al. Prognostic value of coronary revascularisation-related myocardial injury: a cardiac magnetic resonance imaging study. Heart. (2009) 95:1937–43. doi: 10.1136/hrt.2009.173302
90. McEntegart M, Corcoran D, Carrick D, Clerfond G, Sidik N, Collison D, et al. Incidence of procedural myocardial infarction and cardiac magnetic resonance imaging-detected myocardial injury following percutaneous coronary intervention with rotational atherectomy. EuroIntervention. (2018) 14:819–23. doi: 10.4244/EIJ-D-17-01077
91. Kato S, Kitagawa K, Ishida N, Ishida M, Nagata M, Ichikawa Y, et al. Assessment of coronary artery disease using magnetic resonance coronary angiography: a national multicenter trial. J Am College Cardiol. (2010) 56:983–91. doi: 10.1016/j.jacc.2010.01.071
92. Schuetz GM, Zacharopoulou NM, Schlattmann P, Dewey M. Meta-analysis: noninvasive coronary angiography using computed tomography versus magnetic resonance imaging. Ann Intern Med. (2010) 152:167–77. doi: 10.7326/0003-4819-152-3-201002020-00008
93. Hosoda H, Asaumi Y, Noguchi T, Morita Y, Kataoka Y, Otsuka F, et al. Correction to: Three-dimensional assessment of coronary high-intensity plaques with T1-weighted cardiovascular magnetic resonance imaging to predict periprocedural myocardial injury after elective percutaneous coronary intervention. J Cardiovasc Magn Reson. (2020) 22:27. doi: 10.1186/s12968-020-00620-4
94. Stuijfzand WJ, Raijmakers PG, Driessen RS, van Royen N, Nap A, van Rossum AC, et al. Value of hybrid imaging with PET/CT to guide percutaneous revascularization of chronic total coronary occlusion. Curr Cardiovasc Imaging Rep. (2015) 8:26. doi: 10.1007/s12410-015-9340-2
95. Vitadello T, Kunze KP, Nekolla SG, Langwieser N, Bradaric C, Weis F, et al. Hybrid PET/MR imaging for the prediction of left ventricular recovery after percutaneous revascularisation of coronary chronic total occlusions. Eur J Nucl Med Mol Imaging. (2020) 47:3074–83. doi: 10.1007/s00259-020-04877-w
Keywords: chronic total occlusion, revascularization, non-invasive imaging, hibernation, ischemia, viability
Citation: Kersten J, Eberhardt N, Prasad V, Keßler M, Markovic S, Mörike J, Nita N, Stephan T, Tadic M, Tesfay T, Rottbauer W and Buckert D (2021) Non-invasive Imaging in Patients With Chronic Total Occlusions of the Coronary Arteries—What Does the Interventionalist Need for Success? Front. Cardiovasc. Med. 8:713625. doi: 10.3389/fcvm.2021.713625
Received: 23 May 2021; Accepted: 06 August 2021;
Published: 30 August 2021.
Edited by:
Steffen Erhard Petersen, Queen Mary University of London, United KingdomReviewed by:
Alessia Gimelli, Gabriele Monasterio Tuscany Foundation (CNR), ItalyMichael Jerosch-Herold, Brigham and Women's Hospital and Harvard Medical School, United States
Copyright © 2021 Kersten, Eberhardt, Prasad, Keßler, Markovic, Mörike, Nita, Stephan, Tadic, Tesfay, Rottbauer and Buckert. This is an open-access article distributed under the terms of the Creative Commons Attribution License (CC BY). The use, distribution or reproduction in other forums is permitted, provided the original author(s) and the copyright owner(s) are credited and that the original publication in this journal is cited, in accordance with accepted academic practice. No use, distribution or reproduction is permitted which does not comply with these terms.
*Correspondence: Johannes Kersten, johannes.kersten@uni-ulm.de