- 1Department of Ophthalmology, Guangdong Eye Institute, Guangdong Provincial People's Hospital, Guangdong Academy of Medical Sciences, Guangzhou, China
- 2School of Medicine, South China University of Technology, Guangzhou, China
- 3Guangdong Provincial Key Laboratory of South China Structural Heart Disease, Department of Cardiovascular Surgery, Guangdong Cardiovascular Institute, Guangdong Provincial People's Hospital, Guangdong Academy of Medical Sciences, Guangzhou, China
- 4Medical College, Shantou University, Shantou, China
- 5The Second School of Clinical Medicine, Southern Medical University, Guangzhou, China
Background: Microcirculatory changes in congenital heart disease (CHD) patients undergoing cardiac surgery are not fully understood. We aimed to investigate the changes of retinal microcirculation in CHD patients after cardiac surgery by optical coherence tomography angiography (OCTA) and explore the association between retinal microcirculation and surgical outcome.
Methods: This prospective observational study consisted of 71 CHD patients aged ≥6 years undergoing cardiac surgery including 19 cyanotic CHD (CCHD) and 52 acyanotic CHD (ACHD). Optical coherence tomography angiography (OCTA) was used to measure vessel density (VD) and capillary density (CD) of radial peripapillary capillary (RPC) and peripapillary, VD of superficial capillary plexus (SCP) and deep capillary plexus (DCP), thickness of retinal nerve fiber layer (RNFL) and ganglion cell complex (GCC) preoperatively and 1 month postoperatively. Transthoracic echocardiography was conducted to measure macrocirculation.
Results: In CCHD patients, VD and CD of RPC and peripapillary increased postoperatively (all P < 0.05). In ACHD patients, VD of peripapillary, CD of RPC and peripapillary, and RNFL thickness increased postoperatively (all P < 0.05). VD of SCP and DCP, and GCC thickness did not change significantly in CHD patients after surgery. Lower preoperative retinal microvascular density was associated with longer cardiopulmonary bypass (CPB) time and postoperative length of stay (PLOS). No correlation was found between microcirculatory and macrohemodynamic parameters (all P > 0.05).
Conclusions: Improved retinal microcirculation was observed after congenital cardiac surgery and impaired preoperative retinal microvasculature was associated with prolonged CPB time and PLOS, which might provide potential information about the outcome of congenital cardiac surgery.
Introduction
Although advances in the surgical treatment of congenital heart disease (CHD) have substantially improved the overall survival of patients, the perioperative management and prediction of the surgical outcome remain challenging (1, 2). It has been documented that microcirculation dysfunction was independently associated with adverse outcome in patients with valvular or coronary cardiac surgery using cardiopulmonary bypass (CPB), implying microcirculatory monitoring could provide the predictive value of surgical outcome (3, 4). Common techniques have been used to explore the changes of microcirculation including sublingual microscopy (5), laser Doppler perfusion monitoring (6), and near-infrared reflectance spectroscopy (7). Researches exploring new methods for accurately evaluating microcirculation in patients undergoing congenital cardiac surgery are of continual interest and need.
The retina and other end organs (such as the brain and kidney) share similar anatomical features and physiological properties, which allows direct non-invasive visualization of the body's microvasculature, offering a unique and easily accessible window to systemic microvascular pathology in patients undergoing cardiac surgery (8, 9). Optical coherence tomography angiography (OCTA) is a non-invasive, rapid imaging technique used to obtain high-resolution, three-dimensional images of the retinal microvasculature, and can be used to automatically quantify retinal microcirculatory perfusion at different layers (10). Given its excellent repeatability and reproducibility, OCTA has been widely used in clinical practice for monitoring microvascular function in hypertension (11), diabetes (12), and so on (13). In our previous study, a significant impairment of retinal microvasculature was found in the OCTA imaging of CHD patients (14). As for the alteration of postoperative retinal microvasculature, although it has been investigated in patients undergoing coronary artery bypass or valve replacement with CPB (15–18), there is little evidence regarding patients undergoing congenital cardiac surgery.
In this prospective observational study, we aimed to reveal the changes of postoperative retinal microcirculation in CHD patients and explore the association between preoperative retinal microcirculation and adverse outcome of congenital cardiac surgery.
Materials and Methods
Study Design and Patient Population
In this study, all CHD patients (aged ≥ 6 years) were scheduled for cardiac surgery with CPB between May 2017 and October 2020 in Guangdong Provincial People's Hospital. CHD patients were classified into acyanotic (ACHD, SaO2 > 90%) and cyanotic (CCHD, SaO2 ≤ 90%) based on the value of their preoperative resting oxygen saturation (SaO2) (19). All patients went through comprehensive ophthalmologic and cardiac examination prior to the surgery and 1 month after surgery. The examination included refraction measurement, best-corrected visual acuity (BCVA) test, intraocular pressure (IOP) measurement using a non-contact tonometer, OCTA, and transthoracic echocardiography.
The exclusion criteria were as follows: (1) did not perform CPB during surgery; (2) a history of glaucoma, uveitis, or retinal diseases; (3) IOP > 21 mmHg; (4) a history of intraocular surgery; (5) media opacities preventing high-quality imaging; (6) patients with preoperative comorbidities such as hypertension, diabetes, renal disease, and severe cardiovascular diseases.
All study procedures were conducted in accordance with the principles of the Declaration of Helsinki, and ethics approval was obtained from the Research Ethics Committee of Guangdong Provincial People's Hospital [No.GDREC2018148H(R1)]. The study protocol was explained in detail to all participants and written informed consent was obtained from participants (or their parent or legal guardian in the case of children under 16 years) prior to the study.
Optical Coherence Tomography Angiography
Patients underwent an OCTA examination using an AngioVue OCTA system (Version 2017.1.0.151; RTVue-XR Avanti; Optovue, Fremont, CA, USA) which included a 6 × 6 mm2 high-definition (HD) macular scan and a 4.5 × 4.5 mm2 HD optic disc scan. The system uses a light source with peak wavelength at 840 nm and a bandwidth of 50 nm with a scanning speed at 70 kHz and can provide an axial tissue resolution of 5 μm.
The software of the RTVue-XR Avanti utilizes a projection-resolved OCTA algorithm to automatically segment the superficial capillary plexus (SCP), the deep capillary plexus (DCP), and the radial peripapillary capillary (RPC). A split-spectrum amplitude-decorrelation angiography (SSADA) software algorithm was used to automatically calculate retinal vessel density (RVD) defined as the percentage of the measured area occupied by flowing blood vessels (20). An orthogonal registration algorithm for correction of motion artifacts was employed to merge three-dimensional OCT angiograms.
In the optic disc, RPC is defined as the layer between the outer limit of the retinal nerve fiber layer (RNFL) and the internal limiting membrane (ILM). The software automatically fits a 2 mm diameter circle centered around the optic disc; the peripapillary region is defined as the area extending between the 2- and 4-mm-diameter elliptical contour lines around the optic disc boundary. Capillary density (CD) was measured using the built-in software that automatically removes larger vessels of diameter ≥ 3 pixels (~≥33 μm) during the 4.5 × 4.5 mm2 HD optic disc scan (11). The following parameters were measured at the optic disc: the VD and CD of RPC and peripapillary, and RNFL thickness.
In the macular region, SCP is defined as the ILM to 10 μm above the inner plexiform layer (IPL) and DCP is defined as 10 μm above the IPL to 10 μm below the outer plexiform layer (OPL). The ganglion cell complex (GCC) layer is defined as the layer extending from RNFL to IPL. The following parameters were measured at the macula: the VD of SCP and DCP, and GCC thickness.
For each participant, one eye was selected randomly, and the same eye was measured preoperatively and postoperatively. Poor quality images were excluded from the analysis. More specifically, an image was excluded if one of the following criteria were met: (1) quality index (QI) <6; (2) residual motion artifacts; (3) segmentation error; and (4) local weak signal caused by artifacts such as floaters.
Surgical Procedures
Before the initiation of CPB, anticoagulation was achieved using heparin to maintain an activated clotting time ≥ 480 s. During CPB, diluted hematocrit was generally maintained at 25–30%. CPB was generally conducted under mild hypothermia (30–34°C) according to the surgical requirements. Standard ultrafiltration was performed routinely. Myocardial protection was achieved by intermittent antegrade modified St. Thomas cardioplegia. All surgical procedures were done by the same team, and then CHD patients were rewarmed to a bladder temperature >35°C before separation from CPB. After separation, heparin was neutralized by the administration of protamine. Patients were transferred to the intensive care unit (ICU) after surgery.
Data Collection
Clinical, demographic, procedural, and outcome data were extracted and recorded from electronic patient files and ICU charts. Patient preoperative characteristics including age, sex, body mass index, blood pressure, hemoglobin, hematocrit, SaO2, and anatomic diagnosis were recorded. Intraoperative and postoperative data were collected including operation time, CPB time, aortic cross-clamp time, details on the procedure performed, mechanical ventilation time, length of ICU stay, and postoperative length of stay (PLOS). All participants underwent transthoracic echocardiography performed by trained sonographers before and 1 month after surgery, and measurements and analyses were performed by expert technicians and cross-checked by certified echocardiographers. Echocardiographic parameters collected included left ventricular ejection fraction (LVEF), left ventricular end-diastolic dimension (LVEDd), left ventricular end-systolic dimension (LVEDs), early mitral annular velocity (e'), and early transmitral inflow to early mitral relaxation velocity ratio (E/e').
Statistical Analysis
Continuous variables are presented as mean and standard deviation (SD) or median and interquartile range (IQR) according to data distribution. Categorical variables are presented as a number (percentage). The normality of the data was tested using the Shapiro-Wilk test. Paired t-test was used to examine the change of postoperative RVD in the ACHD and CCHD patients. The change of RVD was compared between ACHD and CCHD patients using the student t-test. The 50th percentile of CPB time and PLOS were used to establish the cutoff value for defining prolonged CPB time and PLOS. Prolonged CPB time was used as an essential perioperative risk factor which has been confirmed to be associated with increased mortality and morbidity, and prolonged PLOS was used as an indicator for the surgical outcome. Student t-test was performed to compare the differences of RVD in patients with and without prolonged CPB time and PLOS. Receiver operating characteristic (ROC) analysis was carried out to determine the performance of OCTA parameters to identify patients with prolonged CPB time and PLOS. Spearman's correlation analysis was used to investigate the relationship between RVD and echocardiographic parameters. Statistical analysis was performed with SPSS (IBM SPSS, Version 25.0, IBM Corporation, Armonk, NY, USA). All P-values were from 2-sided tests and results were deemed statistically significant at P < 0.05.
Results
Patient Characteristics
A total of 82 CHD patients were consecutively enrolled in our study. Eight patients were excluded because CPB was not used and three patients were excluded because of poor OCTA image quality in the follow-up examination. Finally, a total of 71 patients with CHD (age range, 6–53 years; 41% male) were enrolled including 52 ACHD patients and 19 CCHD patients. The baseline demographic and clinical characteristics are summarized in Table 1. There was no significant change in BCVA and IOP after surgery (BCVA: 0.02 ± 0.05 logMAR vs. 0.02 ± 0.07 logMAR, P = 0.306; IOP: 14.83 ± 2.56 mmHg vs. 14.47 ± 3.10 mmHg, P = 0.369). The distribution of congenital cardiac lesions is shown in Table 2.
Comparison of Preoperative and Postoperative RVD
The VD and CD of RPC and peripapillary, and RNFL thickness were significantly increased in CHD patients after cardiac surgery (all P < 0.05). However, there were no significant differences in VD of SCP and DCP, and GCC thickness (all P > 0.05) (Table 3). In CCHD patients, a significant increase was observed in postoperative VD and CD of RPC and peripapillary (all P < 0.05) (Figure 1). However, no significant differences were found in VD of SCP and DCP, and thickness of RNFL and GCC (all P > 0.05). In ACHD patients, VD of peripapillary, CD of RPC and peripapillary, and RNFL thickness increased significantly after surgery (all P < 0.05). No significant differences were observed in VD of RPC, SCP and DCP, and GCC thickness (all P > 0.05) (Table 4). The changes in VD of RPC, and CD of RPC and peripapillary were found to be more significant in CCHD patients when compared with ACHD patients (all P < 0.05) (Table 5). Besides, the comparison of preoperative and postoperative RVD was also conducted in different age groups divided by median age (Table 6). The postoperative CD of RPC and peripapillary were significantly increased in younger patients (all P < 0.05), and the postoperative VD and CD of RPC and peripapillary were also significantly increased in older patients (all P < 0.05).

Figure 1. Typical 4.5 × 4.5 mm2 HD OCTA color-coded vessel density map of a cyanotic congenital heart disease eye preoperatively (A) and postoperatively (B).
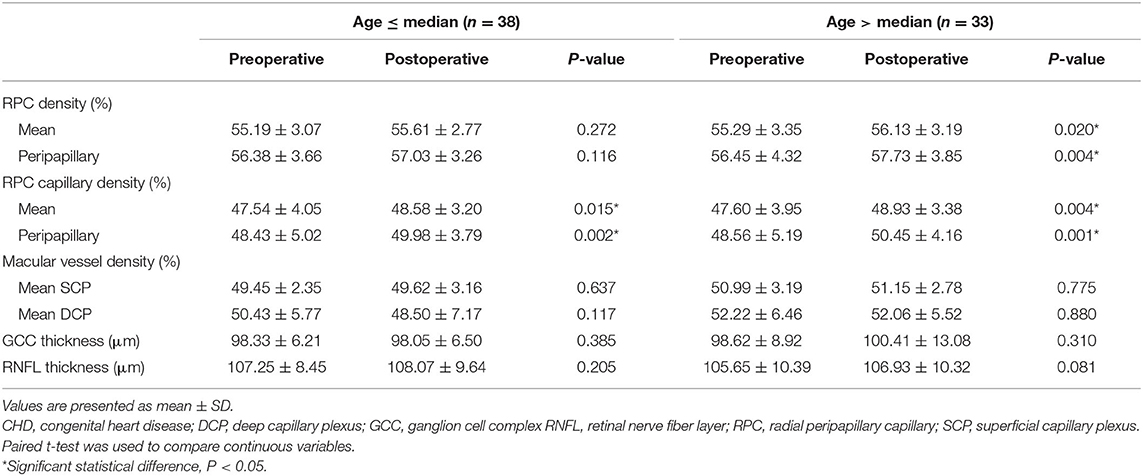
Table 5. Comparison of retinal vessel density before and after surgery in CHD patients of different age groups (n = 71).
Comparison of RVD Between Patients With and Without Prolonged CPB Time and PLOS
Compared with patients without prolonged CPB time, the CD of RPC and peripapillary were significantly lower in patients with prolonged CPB time (both P < 0.05). No significant differences were found in VD of RPC and peripapillary (both P > 0.05). In patients with prolonged PLOS, VD of peripapillary and CD of RPC and peripapillary were significantly lower compared with patients without prolonged PLOS (all P < 0.05). No significant difference was found in VD of RPC (P > 0.05) (Table 7). The ROC showed the ability of RVD to distinguish patients with prolonged CPB time and PLOS (Supplementary Figure 1). The area under the receiver operating characteristic curve (AUC) of the VD of mean RPC, RPC capillary, peripapillary RPC and peripapillary RPC capillary to identify patients with prolonged CPB time is 0.679, 0.695, 0.670, and 0.702, respectively. The AUC of the VD of mean RPC, RPC capillary, peripapillary RPC and peripapillary RPC capillary to identify patients with prolonged PLOS is 0.611, 0.650, 0.626, and 0.676, respectively.
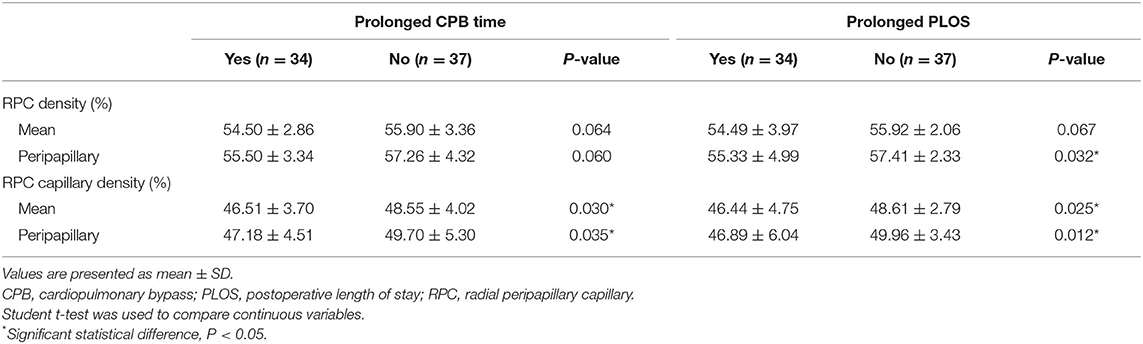
Table 7. Comparison of preoperative retinal vessel density in patients with and without prolonged CPB time and PLOS.
Correlation Between Microcirculatory and Echocardiographic Parameters
Regarding the relationship between microcirculatory and macrohemodynamic characteristics, no significant correlation was observed between RVD and LVEF, LVEDs, LVEDd, e', and E/e' ratio preoperatively and postoperatively (all P > 0.05) (Table 8).
Discussion
Results of our prospective study showed that RVD was improved after congenital cardiac surgery with CPB and revealed the association between preoperative impairment in RVD and prolonged CPB time and PLOS, which indicated the potential of retinal microcirculation in the assessment of outcome for patients with congenital cardiac surgery. Besides, no correlation was found between microcirculatory variables and macrohemodynamic parameters in CHD patients undergoing cardiac surgery.
Retinal perfusion was significantly increased in CCHD patients after cardiac surgery with CPB in our study. Similarly, previous studies have found that the retinal vascular morphology, such as dilatation and tortuosity, would return to normal after cardiac surgery in CCHD patients (21, 22). On the one hand, postoperative higher oxy-hemoglobin concentrations could enhance oxygen delivery to microcirculation (23, 24). On the other hand, reduced blood viscosity could change the shear stress and increase nitric oxide (NO) production and result in vascular smooth muscle cell relaxation and vasodilation after surgery (25, 26). A previous study examined the microcirculatory changes in CHD patients undergoing cardiac surgery with CPB and reported an increased sublingual microcirculatory perfusion in CCHD patients during ICU stay (27). However, preoperative and late postoperative microcirculatory parameters could not be studied due to the difficulty to use sublingual microscopy in extubated and awake patients.
In the present study, a large-vessel masking approach was selected to separate larger vessels from capillaries. The results showed that the increase of RVD was more notable in capillaries. This might result from variations in blood viscosity that could exert a more obvious effect at a capillary level compared to large vessels (28). In addition, large vessels make up a larger proportion of VD and have a substantial contribution to the total peripapillary perfusion, which might mask a change in VD (29). Several studies have also shown that subtraction of the peripapillary large vessels was associated with enhanced diagnostic accuracy of peripapillary perfusion for glaucoma (30) and diabetic retinopathy (31).
Our results also revealed that preoperative impairment in RVD was associated with longer CPB time and PLOS. Furthermore, the ROC analysis showed that preoperative RVD could provide reasonable classification of patients with prolonged CPB time and PLOS. It has been proved that prolonged CPB time and PLOS could reflect the adverse outcome in cardiac surgical patients (32–34). To some extent, microcirculatory impairment might play an important role in the outcome of cardiac surgery. Firstly, microcirculation is essential to provide oxygen and nutrients to tissues and is the main site of immunological response, regulation of homeostasis, and vasomotor tone control (35). Secondly, it has been stated that microcirculatory impairment was an independent predictor of morbidity and mortality in several acute pathological conditions, such as cardiac surgery and septic shock (36, 37). However, the association between retinal microcirculation and clinical outcome of CHD patients undergoing cardiac surgery remains unknown. Our results might provide valuable insights into the predictive value of retinal microcirculation in the outcome of congenital cardiac surgery.
As previously documented, impairment of retinal microvasculature was associated with cardiac structure and function (38). Hence, we speculated the association between impaired retinal microcirculation and adverse surgical outcome might reflect sicker patients with lower cardiac output. However, our results indicated that microcirculatory variables were not associated with macrohemodynamic parameters in CHD patients undergoing cardiac surgery. Also, previous studies found that microcirculatory impairment could be observed even when systemic hemodynamics were within satisfactory limits in patients undergoing cardiac surgery (27, 39). This could be explained by the different anatomical structures and physiological regulation mechanisms of macro- and micro-circulation. On one hand, macro- and micro-vessels respond differently to therapeutic interventions and inflammatory molecules (40). On the other hand, cellular sensing mechanisms needed to maintain the microvascular autoregulation were damaged in patients with cardiac surgery (41). Notably, microvascular dysfunction might play an important role in the development of postoperative organ dysfunction but the change in microvascular perfusion was independent of the change in systemic hemodynamics. Therefore, it is essential to monitor the microcirculation of patients with cardiac surgery.
In the current context, more and more clinical trials have sought to evaluate the microcirculatory changes in patients undergoing cardiac surgery. Technological advancements made it possible to have real-time imaging of microcirculation. Several methods have been used to visualize microcirculation, such as video microscopy, near-infrared spectroscopy, and laser Doppler flowmetry. OCTA was a newly developed, reproducible and reliable microcirculation monitoring tool as shown by recent studies (42, 43) and the time required for a single blood flow imaging scan of OCTA is around 2 to 3 seconds, providing a non-invasive and rapid tool for assessment of the microcirculation in patients undergoing cardiac surgery. As previously reported, assessment of RVD using OCTA could offer a window to evaluate the systemic microvasculature (44, 45). For this reason, the implementation of OCTA might provide potential information into the outcome of patients undergoing cardiac surgery, assisting physicians to frame more customized and precise therapeutic decisions for the patients. Studies comparing the current methods and OCTA in the quantification of microcirculation are needed to investigate the value of adopting OCTA as a clinical tool for the CHD patients.
The main strength of our prospective study is the use of a state-of-the-art technique, namely OCTA, to observe the changes of retinal microcirculation in CHD patients undergoing cardiac surgery with CPB. Nonetheless, we acknowledge several limitations in our study. Firstly, we did not evaluate RVD during and immediately after surgery due to technical issues. In the future, a portable handheld OCTA instrument could be used to explore the application of OCTA during and soon after surgery (46, 47). Secondly, a relatively small sample of CCHD patients was studied, which might have limited power for non-significant associations. Thirdly, the rate of the outcome events like mortality, neurological injury and end-organ damage was relatively low in the present study, limiting the power of our statistical analyses to assess the association between retinal microcirculation and these outcome events. In addition, we did not compare the differences in RVD changes for patients with different types of cardiac lesions and different severity due to the small sample size. Also, the study population was mostly elderly pediatric congenital heart disease patients, which could not represent for the whole perspective of congenital heart disease. Future studies with larger and multi-center sample sizes are needed.
Conclusions
Our study results showed that RVD was improved after congenital cardiac surgery and impaired preoperative retinal microcirculation was associated with prolonged CPB time and PLOS, indicating OCTA could be used to identify potential adverse outcome due to prolonged CPB or PLOS and providing a novel non-invasive and rapid microcirculation monitoring tool for patients undergoing cardiac surgery.
Data Availability Statement
The original contributions presented in the study are included in the article/Supplementary Material, further inquiries can be directed to the corresponding author/s.
Ethics Statement
The studies involving human participants were reviewed and approved by the Research Ethics Committee of Guangdong Provincial People's Hospital [No.GDREC2018148H(R1)]. The patients/participants (or their parent or legal guardian in the case of children under 16 years) provided their written informed consent to participate in this study.
Author Contributions
XY, HYu, CL, ZZ, and HYua: conception, design, drafting the article or revising it, and providing intellectual content of critical importance to the work described. CL, ZZ, HYua, PZ, QP, XD, MH, BL, YR, YK, XZ, HYu, and XY: analysis and interpretation of data. All authors: final approval of the version to be published.
Funding
This work was supported by Science and Technology Program of Guangzhou, China (202002020049) (XY); Project of Investigation on Health Status of Employees in Financial Industry in Guangzhou (Z012014075) (XY); The National Natural Science Foundation of China (81870663) (HYu); The Talent Introduction Fund of Guangdong Provincial People's Hospital (KJ012019087, Y012018145) (HYu); Medical Scientific Research Foundation of Guangdong Province of China (C2019044) (MH); and Project of Special Research on Cardiovascular Diseases (2020XXG007) (XY).
Conflict of Interest
The authors declare that the research was conducted in the absence of any commercial or financial relationships that could be construed as a potential conflict of interest.
Publisher's Note
All claims expressed in this article are solely those of the authors and do not necessarily represent those of their affiliated organizations, or those of the publisher, the editors and the reviewers. Any product that may be evaluated in this article, or claim that may be made by its manufacturer, is not guaranteed or endorsed by the publisher.
Acknowledgments
We would like to thank HYu and XY for their conception, design, administrative support, critical revision, and supervision; they are considered co-corresponding authors. The authors would also like to thank the staff of the department of cardiovascular surgery in Guangdong Provincial People's Hospital, for their assistance with the recruitment of the participants.
Supplementary Material
The Supplementary Material for this article can be found online at: https://www.frontiersin.org/articles/10.3389/fcvm.2021.712308/full#supplementary-material
References
1. Ringle ML, Wernovsky G. Functional, quality of life, and neurodevelopmental outcomes after congenital cardiac surgery. Semin Perinatol. (2016) 40:556–70. doi: 10.1053/j.semperi.2016.09.008
2. Namachivayam SP, D'udekem Y, Millar J, Cheung MM, Butt W. Survival status and functional outcome of children who required prolonged intensive care after cardiac surgery. J Thorac Cardiovasc Surg. (2016) 152:6. doi: 10.1016/j.jtcvs.2016.05.006
3. Kim TK, Cho YJ, Min JJ, Murkin JM, Bahk J-H, Hong DM, et al. Microvascular reactivity and clinical outcomes in cardiac surgery. Crit Care. (2015) 19:316. doi: 10.1186/s13054-015-1025-3
4. Kim TK, Cho YJ, Min JJ, Murkin JM, Bahk J-H, Hong DM, et al. Preoperative endothelial dysfunction in cutaneous microcirculation is associated with postoperative organ injury after cardiac surgery using extracorporeal circulation: a prospective cohort study. Ann Intensive Care. (2021) 11:4. doi: 10.1186/s13613-020-00789-y
5. Flick M, Duranteau J, Scheeren TWL, Saugel B. Monitoring of the sublingual microcirculation during cardiac surgery: current knowledge and future directions. J Cardiothorac Vasc Anesth. (2020) 34:2754–65. doi: 10.1053/j.jvca.2019.10.038
6. Gomes V, Gomes MB, Tibirica E, Lessa MA. Post-operative endothelial dysfunction assessment using laser Doppler perfusion measurement in cardiac surgery patients. Acta Anaesthesiol Scand. (2014) 58:468–77. doi: 10.1111/aas.12286
7. Mukaida H, Matsushita S, Inotani T, Futaki S, Takano A, Watanabe M, et al. Peripheral circulation evaluation with near-infrared spectroscopy in skeletal muscle during cardiopulmonary bypass. Perfusion. (2015) 30:653–59. doi: 10.1177/0267659115575419
8. Cheung CY-L, Ong YT, Ikram MK, Ong SY, Li X, Hilal S, et al. Microvascular network alterations in the retina of patients with Alzheimer's disease. Alzheimers Dement. (2014) 10:135–42. doi: 10.1016/j.jalz.2013.06.009
9. Cheung CY-L, Ikram MK, Sabanayagam C, Wong TY. Retinal microvasculature as a model to study the manifestations of hypertension. Hypertension. (2012) 60:1094–103. doi: 10.1161/HYPERTENSIONAHA.111.189142
10. Kashani AH, Chen C-L, Gahm JK, Zheng F, Richter GM, Rosenfeld PJ, et al. Optical coherence tomography angiography: a comprehensive review of current methods and clinical applications. Prog Retin Eye Res. (2017) 60:66–100. doi: 10.1016/j.preteyeres.2017.07.002
11. Peng QS, Hu YJ, Huang MQ, Wu Y, Zhong PT, Dong XR, et al. Retinal neurovascular impairment in patients with essential hypertension: an optical coherence tomography angiography study. Invest Ophthalmol Vis Sci. (2020) 61:42. doi: 10.1167/iovs.61.8.42
12. Ting DSW, Tan GSW, Agrawal R, Yanagi Y, Sie NM, Wong CW, et al. Optical coherence tomographic angiography in type 2 diabetes and diabetic retinopathy. J Am Med Assoc Ophthalmol. (2017) 135:306–12. doi: 10.1001/jamaophthalmol.2016.5877
13. Farrah TE, Dhillon B, Keane PA, Webb DJ, Dhaun N. The eye, the kidney, and cardiovascular disease: old concepts, better tools, and new horizons. Kidney Int. (2020) 98:323–42. doi: 10.1016/j.kint.2020.01.039
14. Li C, Zhong PT, Yuan HY, Dong XR, Peng QS, Huang MQ, et al. Retinal microvasculature impairment in patients with congenital heart disease investigated by optical coherence tomography angiography. Clin Exp Ophthalmol. (2020) 48:1219–28. doi: 10.1111/ceo.13846
15. Pekel G, Alur I, Alihanoglu YI, Yagci R, Emrecan B. Choroidal changes after cardiopulmonary bypass. Perfusion. (2014) 29:560–66. doi: 10.1177/0267659114529324
16. Hayashi H, Kawaguchi M, Hasuwa K, Inoue S, Okamoto M, Matsuura T, et al. Changes in intraocular pressure during cardiac surgery with and without cardiopulmonary bypass. J Anesth. (2010) 24:663–68. doi: 10.1007/s00540-010-0983-5
17. Deutch D, Lewis RA. Intraocular pressure after cardiopulmonary bypass surgery. Am J Ophthalmol. (1989) 107:18–22. doi: 10.1016/0002-9394(89)90808-8
18. Nenekidis I, Geiser M, Riva C, Pournaras C, Tsironi E, Vretzakis G, et al. Blood flow measurements within optic nerve head during on-pump cardiovascular operations. A window to the brain? Interact Cardiovasc Thorac Surg. (2011) 12:718–22. doi: 10.1510/icvts.2010.260950
19. Stout KK, Daniels CJ, Aboulhosn JA, Bozkurt B, Broberg CS, Colman JM, et al. 2018 AHA/ACC guideline for the management of adults with congenital heart disease: a report of the American College of Cardiology/American Heart Association Task Force on Clinical Practice Guidelines. Circulation. (2019) 139: e698–800. doi: 10.1161/CIR.0000000000000603
20. Tokayer J, Jia Y, Dhalla A-H, Huang D. Blood flow velocity quantification using split-spectrum amplitude-decorrelation angiography with optical coherence tomography. Biomed Opt Express. (2013) 4:1909–24. doi: 10.1364/BOE.4.001909
21. Petersen RA, Rosenthal A. Retinopathy and papilledema in cyanotic congenital heart disease. Pediatrics. (1972) 49:243–49.
22. Kohner EM, Allen EM, Saunders KB, Emery VM, Pallis C. Electroencephalogram and retinal vessels in congenital cyanotic heart disease before and after surgery. Br Med J. (1967) 4:207–10. doi: 10.1136/bmj.4.5573.207
23. Li J. Evolution of the concept of oxygen transport in the critically ill, with a focus on children after cardiopulmonary bypass. Cardiol Young. (2018) 28:186–91. doi: 10.1017/S1047951117001706
24. Chakravarti SB, Mittnacht AJC, Katz JC, Nguyen K, Joashi U, Srivastava S. Multisite near-infrared spectroscopy predicts elevated blood lactate level in children after cardiac surgery. J Cardiothorac Vasc Anesth. (2009) 23:663–67. doi: 10.1053/j.jvca.2009.03.014
25. Toporsian M, Govindaraju K, Nagi M, Eidelman D, Thibault G, Ward ME. Downregulation of endothelial nitric oxide synthase in rat aorta after prolonged hypoxia in vivo. Circ Res. (2000) 86:671–75. doi: 10.1161/01.RES.86.6.671
26. Ferreiro CR, Chagas AC, Carvalho MH, Dantas AP, Jatene MB, Souza LCBD, et al. Influence of hypoxia on nitric oxide synthase activity and gene expression in children with congenital heart disease: a novel pathophysiological adaptive mechanism. Circulation. (2001) 103:2272–76. doi: 10.1161/01.CIR.103.18.2272
27. Scolletta S, Marianello D, Isgrò G, Dapoto A, Terranova V, Franchi F, et al. Microcirculatory changes in children undergoing cardiac surgery: a prospective observational study. Br J Anaesth. (2016) 117:206–13. doi: 10.1093/bja/aew187
28. Pries AR, Secomb TW, Gessner T, Sperandio MB, Gross JF, Gaehtgens P. Resistance to blood flow in microvessels in vivo. Circ Res. (1994) 75:904–15. doi: 10.1161/01.RES.75.5.904
29. Ghahari E, Bowd C, Zangwill LM, Proudfoot J, Hasenstab KA, Hou H, et al. Association of macular and circumpapillary microvasculature with visual field sensitivity in advanced glaucoma. Am J Ophthalmol. (2019) 204:51–61. doi: 10.1016/j.ajo.2019.03.004
30. Geyman LS, Garg RA, Suwan Y, Trivedi V, Krawitz BD, Mo S, et al. Peripapillary perfused capillary density in primary openangle glaucoma across disease stage an optical coherence tomography angiography study. Br J Ophthalmol. (2017) 101:1261–68. doi: 10.1136/bjophthalmol-2016-309642
31. Tan B, Chua J, Lin E, Cheng J, Gan A, Yao X, et al. Quantitative microvascular analysis with wide-field optical coherence tomography angiography in eyes with diabetic retinopathy. J Am Med Assoc Netw Open. (2020) 3:e1919469. doi: 10.1001/jamanetworkopen.2019.19469
32. Green MD, Parker DM, Everett AD, Vricella L, Jacobs ML, Jacobs JP, et al. Cardiac biomarkers associated with hospital length of stay after pediatric congenital heart surgery. Ann Thorac Surg. (2020) 112:632–7. doi: 10.1016/j.athoracsur.2020.06.059
33. Shi GC, Zhu ZQ, Chen J, Ou YQ, Hong HF, Nie ZQ, et al. Total anomalous pulmonary venous connection: the current management strategies in a pediatric cohort of 768 patients. Circulation. (2017) 135:48–58. doi: 10.1161/CIRCULATIONAHA.116.023889
34. Vedovelli L, Padalino M, Simonato M, D'aronco S, Bertini D, Stellin G, et al. Cardiopulmonary bypass increases plasma glial fibrillary acidic protein only in first stage palliation of hypoplastic left heart syndrome. Can J Cardiol. (2016) 32:355–61. doi: 10.1016/j.cjca.2015.06.023
35. Abrard S, Lasocki S, Henni S. Pre-operative evaluation of microcirculation for the prediction of complications after cardiac surgery under extracorporeal circulation: study protocol. Eur J Anaesthesiol. (2019) 36:613–15. doi: 10.1097/EJA.0000000000000968
36. Sakr Y, Dubois M-J, Backer DD, Creteur J, Vincent J-L. Persistent microcirculatory alterations are associated with organ failure and death in patients with septic shock. Crit Care Med. (2004) 32:1825–31. doi: 10.1097/01.CCM.0000138558.16257.3F
37. Backer DD, Creteur J, Dubois M-J, Sakr Y, Vincent J-L. Microvascular alterations in patients with acute severe heart failure and cardiogenic shock. Am Heart J. (2004) 147:91–9. doi: 10.1016/j.ahj.2003.07.006
38. Chandra A, Seidelmann SB, Claggett BL, Klein BE, Klein R, Shah AM, et al. The association of retinal vessel calibres with heart failure and long-term alterations in cardiac structure and function: the Atherosclerosis Risk in Communities (ARIC) Study. Eur J Heart Fail. (2019) 21:1207–15. doi: 10.1002/ejhf.1564
39. Backer DD, Ortiz JA, Salgado D. Coupling microcirculation to systemic hemodynamics. Curr Opin Crit Care. (2010) 16:250–54. doi: 10.1097/MCC.0b013e3283383621
40. Dubin A, Pozo MO, Casabella CA FP Jr, Murias G, Moseinco MC, et al. Increasing arterial blood pressure with norepinephrine does not improve microcirculatory blood flow: a prospective study. Crit Care. (2009) 13:R92. doi: 10.1186/cc7922
41. Ince C. Hemodynamic coherence and the rationale for monitoring the microcirculation. Crit Care. (2015) 19(Suppl.3):S8. doi: 10.1186/cc14726
42. Lei J, Pei C, Wen C, Abdelfattah NS. Repeatability and reproducibility of quantification of superficial peri-papillary capillaries by four different optical coherence tomography angiography devices. Sci Rep. (2018) 8:17866. doi: 10.1038/s41598-018-36279-2
43. You Q, Freeman WR, Weinreb RN, Zangwill L, Manalastas PIC, Saunders LJ, et al. Reproducibility of vessel density measurement with optical coherence tomography angiography in eyes with and without retinopathy. Retina. (2017) 37:1475–82. doi: 10.1097/IAE.0000000000001407
44. Wei X, Balne PK, Meissner KE, Barathi VA, Schmetterer L, Agrawal R. Assessment of flow dynamics in retinal and choroidal microcirculation. Surv Ophthalmol. (2018) 63:646–64. doi: 10.1016/j.survophthal.2018.03.003
45. Serre K, Sasongko MB. Modifiable lifestyle and environmental risk factors affecting the retinal microcirculation. Microcirculation. (2012) 19:29–36. doi: 10.1111/j.1549-8719.2011.00121.x
46. Yang J, Liu L, Campbell JP, Huang D, Liu G. Handheld optical coherence tomography angiography. Biomed Opt Express. (2017) 8:2287–300. doi: 10.1364/BOE.8.002287
Keywords: congenital heart disease, cardiac surgery, retinal vessel density, optical coherence tomography angiography, microcirculation
Citation: Li C, Zhu Z, Yuan H, Zhong P, Peng Q, Dong X, Huang M, Liu B, Ren Y, Kuang Y, Zeng X, Yu H and Yang X (2021) Improved Retinal Microcirculation After Cardiac Surgery in Patients With Congenital Heart Disease. Front. Cardiovasc. Med. 8:712308. doi: 10.3389/fcvm.2021.712308
Received: 20 May 2021; Accepted: 06 August 2021;
Published: 31 August 2021.
Edited by:
Antonio Miceli, Istituto Clinico Sant'Ambrogio, ItalyReviewed by:
Alexandra White, University Hospital Galway, IrelandOkan Yurdakok, Dr. Siyami Ersek Chest Cardiovascular Surgery Training and Research Hospital, Turkey
Copyright © 2021 Li, Zhu, Yuan, Zhong, Peng, Dong, Huang, Liu, Ren, Kuang, Zeng, Yu and Yang. This is an open-access article distributed under the terms of the Creative Commons Attribution License (CC BY). The use, distribution or reproduction in other forums is permitted, provided the original author(s) and the copyright owner(s) are credited and that the original publication in this journal is cited, in accordance with accepted academic practice. No use, distribution or reproduction is permitted which does not comply with these terms.
*Correspondence: Xiaohong Yang, c3l5YW5neGhAc2N1dC5lZHUuY24=; Honghua Yu, eXVob25naHVhQGdkcGgub3JnLmNu
†These authors have contributed equally to this work and share first authorship