- 1Department of Cardiology, Shanghai Ninth People's Hospital, Shanghai Jiaotong University School of Medicine, Shanghai, China
- 2Department of Vascular and Cardiology, Ruijin Hospital, Shanghai Jiaotong University School of Medicine, Shanghai, China
- 3Department of Endocrinology, The Second People's Hospital of Yunnan Province, Kunming, China
Objectives: This study explores the concentration and role of glucagon-like peptide-1 (GLP-1) in calcific aortic valve disease (CAVD).
Background: Calcific aortic valve disease is a chronic disease presenting with aortic valve degeneration and mineralization. We hypothesized that the level of GLP-1 is associated with CAVD and that it participates in the calcification of aortic valve interstitial cells (AVICs).
Methods: We compared the concentration of GLP-1 between 11 calcific and 12 normal aortic valve tissues by immunohistochemical (IHC) analysis. ELISA was used to measure GLP-1 in serum of the Control (n = 197) and CAVD groups (n = 200). The effect of GLP-1 on the calcification of AVICs and the regulation of calcific gene expression were also characterized.
Results: The GLP-1 concentration in the calcific aortic valves was 39% less than that in the control non-calcified aortic valves. Its concentration in serum was 19.3% lower in CAVD patients. Multivariable regression analysis demonstrated that GLP-1 level was independently associated with CAVD risk. In vitro, GLP-1 antagonized AVIC calcification in a dose- and time-dependent manner and it down-regulated RUNX2, MSX2, BMP2, and BMP4 expression but up-regulated SOX9 expression.
Conclusions: A reduction in GLP-1 was associated with CAVD, and GLP-1 participated in the mineralization of AVICs by regulating specific calcific genes. GLP-1 warrants consideration as a novel treatment target for CAVD.
Introduction
Glucagon-like peptide-1 (GLP-1), a hormone of 30 amino acids, is derived from proglucagon (1). It can increase insulin's sensitivity in regulating blood glucose (2), which is an effect that partially reverses aging-related degenerative disease (3). Calcific aortic valve disease (CAVD) is a common and chronic heart valve disease (4) that exhibits aortic valve thickening and calcification (5). Calcific aortic valve disease involves chronic inflammatory responses (6), lipid accumulation (7, 8), extracellular matrix rebuilding (9, 10), and osteogenic-related gene activation (11, 12). Glucagon-like peptide-1 has shown benefits on cardiovascular function in both preclinical and clinical studies (13–15). Furthermore, a 5-week infusion of GLP-1 (2.5 pmol/kg/min) added to standard therapy in patients with heart function of New York Heart Association class III/IV improved the LV ejection fraction in both diabetic and non-diabetic patients (16). However, the relationship between GLP-1 and CAVD has not been elucidated.
Valve interstitial cells (VICs) are heterogeneous cells that present various phenotypes (myofibroblasts, fibroblasts, and smooth muscle-like cells) and they participate in the physiological function of the aortic valve (17). For instance, the risk factors for CAVD evoke osteogenic signaling, which turn innate quiescent valve interstitial cells (qVICs) into activated valve interstitial cells (aVICs) and facilitate them to differentiate into osteoblastic valve interstitial cells (obVICs) (4, 18, 19). Glucagon-like peptide-1 suppresses vascular smooth muscle cell (VSMC) mineralization by reducing osteogenic gene expression and activating arterial calcification (20). The phenotypic changes in VICs are the main cytological events leading to aortic valve calcification; however, whether GLP-1 regulates the phenotype of VICs is unknown.
In this work, we hypothesized that GLP-1 is differentially regulated in the serum and tissue of CAVD patients and that its concentration is associated with aortic valve calcification.
Materials and Methods
Patients
We performed a retrospective study on 200 aortic valves with calcific degeneration (CAVD) and 197 without calcific degeneration between January 2013 and August 2014 in patients recruited from the database of Shanghai Rui Jin Hospital who underwent echocardiographic screening. According to recommendations of the American Society of Echocardiography during hospitalization and patients entered into the screening procedure (21), and underwent standard transthoracic echocardiography and Doppler flow imaging. Calcific aortic valve disease was defined as opaque leaflets with focal areas of mild thickening and increased stiffness with or without an elevated peak trans-aortic valve flow velocity (≥2.0 m/s) (22) (Figure 1). Patients with a history of rheumatic disease, endocarditis, or an inflammatory disease were excluded. Detailed medical and family histories were recorded, and fasting blood samples were collected during physical check-up. The diagnosis of type 2 diabetes, hypertension, and coronary artery diseases (CAD)was made according to corresponding criteria of the American Diabetes Association (23), hypertension (24), and CAD (25) guidelines.
Human aortic valves with calcification were obtained from 11 patients who underwent valve replacement. Aortic valve leaflets were collected from the explanted hearts of 12 patients undergoing heart transplantation as normal aortic valves. The study protocol was approved by the Ethics Committee of Ruijin Hospital, Shanghai Jiaotong University School of Medicine, and written informed consent was obtained from all patients.
Reagents and Antibodies
A High Sensitivity GLP-1 Active ELISA Kit (Cat# EZGLPHS-35K, Millipore, MA, USA) was used to measure GLP-1 in serum. Recombinant GLP-1 peptide (Human, Cat #SCP0153), a 3,3′-Diaminobenzidine Liquid Substrate System (Cat# D3939), an Alkaline Phosphatase Diethanolamine (ALP) Activity Kit (Cat# AP0100), Alizarin Red S (Cat# A5533), and a Masson Stain Kit (Cat# HT15) were purchased from Sigma-Aldrich (MO, USA). Primary antibodies were used to detect GLP-1 (Cat# ab22625, Abcam, MA, USA), RUNX2 (Cat# 12556, Cell Signaling Technology, MA, USA), MSX2 (Cat# ab69058, Abcam, MA, USA), SOX9 (Cat# 82630, Cell Signaling Technology, MA, USA), BMP2 (Cat# ab14933, Abcam, MA, USA), BMP4 (Cat# ab39973Abcam, MA, USA), and β-actin (Cat# 4970, Cell Signaling Technology, MA, USA) in immunohistochemical (IHC) or immunoblot assays. The secondary antibodies were horseradish peroxidase (HRP)-conjugated anti-rabbit antibodies (Cat# 7074, Cell Signaling Technology, MA, USA) or Alexa Fluor 594- or Alexa Fluor 488-conjugated anti-rabbit antibodies (Cat# R37119 or Cat# A27034, Thermo Fisher Scientific, NY, USA). Fetal bovine serum (FBS, Cat# 16000044), DMEM:F12 culture medium, penicillin, and streptomycin were from Gibco BRL (NY, USA).
Primary Aortic Valve Interstitial Cell Culture
Human aortic valve leaflets from the explanted hearts were gathered to culture the primary aortic valve interstitial cells (AVICs) (26). Briefly, valve leaflets were subjected to collagenase digestion and gently scraped to expose the endothelial layer. The leaflets were then cut into microscopic pieces (1–2 mm2) and cultured in DMEM:F12 (1:1) supplemented with 20% FBS, L-glutamine (2 mmol/L), penicillin (100 U/ml), and streptomycin (100 μg/ml). Cells were grown with 5% CO2 at 37°C. Upon reaching 80% confluency, the AVICs were passaged using trypsin-EDTA. AVICs between passage 3 and 8 were used for experiments.
Immunohistochemistry
Human calcific (n = 11) and non-calcific aortic valves (n = 12) were used for histological and immunochemical analysis. Samples were fixed in 4% paraformaldehyde overnight and cut into serial cryosections (5 μm thickness). Sections were used for hematoxylin and eosin (H&E) staining, Alizarin Red S staining, and Masson trichrome staining. Glucagon-like peptide-1 in the valves was detected by IHC using anti-GLP-1 antibody (1:50). After incubation with HRP-conjugated secondary antibodies (1:100), sections were incubated with 3,3′-diaminobenzidine.
The sections of primary AVICs were used for immunofluorescence analysis. The cells were immune-stained with anti-RUNX2 (1:50), anti-MSX2 (1:50), anti-SOX9 (1:50), anti-BMP2 (1:50), or anti-BMP4 (1:50) for 12 h at 4°C and incubated with Alexa Fluor 549- or Alexa Fluor 488-conjugated secondary antibody (1:1,000).
In vitro Calcification of AVICs
Primary AVICs were isolated from the human aortic valve. Aortic valve interstitial cell calcification was induced in osteogenic medium containing DMEM and supplemented with 15% FBS, 50 mg/ml ascorbate-2-phosphate, 10 nM dexamethasone, and 10 mM β-glycerol phosphate (27). The culture medium was changed every 48–72 h, and the cells were harvested for 3 weeks. Aortic valve interstitial cell calcification was determined by Alizarin Red S staining. The cells were washed in distilled water and then exposed to freshly prepared 2% Alizarin Red S (pH to 4.1–4.3) for 5 min (red/orange as positive staining). For quantitative analysis of Alizarin Red S staining, the dye was released from the cell matrix by incubating with cetylpyridinium chloride for 15 min. The released dye was quantified by spectrophotometry at 540 nm. Alkaline Phosphatase Diethanolamine activity was determined using the spectrophotometric measurement of the p-nitrophenol level in the AVICs (28). The amount of Alizarin Red S staining and ALP activity were normalized to the total amount of cellular protein.
Quantitative Real-Time PCR
Total RNA was extracted as described above. Briefly, 5 μg of total RNA was reverse-transcribed into cDNA using a reverse transcription system (Promega, WI, USA). PCR amplification was performed with Power SYBR Green PCR Master Mix (Applied Biosystems, CA, USA) in a StepOne system (Applied Biosystems). The oligonucleotides used in quantitative real-time PCR analysis are listed in Supplementary Table 1. The gene expression levels were normalized to beta-actin, and the data were analyzed with StepOne software v2.1 (Applied Biosystems).
Western Blot
Cells were lysed with the ProteoJET Mammalian Cell Lysis Reagent (Fermentas, MD, USA) to extract cytoplasmic proteins. Equal amounts of protein extracts were subjected to 10% SDS/PAGE and blotted onto a poly (vinylidene difluoride) membrane. The membrane was blocked and probed overnight at 4°C with antibodies against RUNX2 (1:1,000), MSX2 (1:1,000), SOX9 (1:100), BMP2 (1:500), BMP4 (1:500), and β-actin (1:2,000), followed by incubation with HRP-conjugated secondary antibodies (1:5,000) for 1 h at room temperature. Blots were developed using an ECL detection system (Millipore, MA, USA). Each image was captured and the intensity of each band was analyzed with Quantity One (Bio-Rad).
Statistical Analysis
We performed statistical analyses with SPSS software (version 20). All tests were two-tailed, and a p-value of < 0.05 was regarded as statistically significant. Demographic and clinical characteristics were compared between the CAVD and non-CAVD groups. Fisher's exact test was employed for categorical variables, and a two-independent-samples t-test or Wilcoxon rank-sum test was employed for numerical variables to evaluate between-group differences. To analyze risk factors for CAVD, candidate variables were decided a priori by referral to previous reports. Using these variables, we performed multivariable logistic regression analyses with a backward stepwise selection procedure. Odds ratios (ORs) and 95% CIs were calculated. For ALP and ALZ, repeated-measures ANOVA with the group as a fixed factor was employed to compare the differences between two groups.
Results
Distribution of GLP-1 in Calcific Aortic Valve
Based on the histological analysis, the calcific aortic valve exhibited structural thickening, mineralization and ECM remodeling (Figure 2A) and the demographic and clinical characteristics of the study participants were summarized in Table 1. The IHC analysis showed that GLP-1 was mainly evenly distributed in the rich region of VICs of control aortic valves, but GLP-1 was prominently distributed in the non-mineralized areas of calcific aortic valves (Figure 2A). The integrated optical density (IOD) of the GLP-1 level was calculated in 12 control valves and 11 calcific aortic valves. Compared with the control valves, the concentration of GLP-1 decreased in the calcific aortic valves by 39% (CAVD: 5,606 ± 750.4; vs. Control valves: 9,170 ± 695.9; P = 0.0042) (Figures 2A,B).
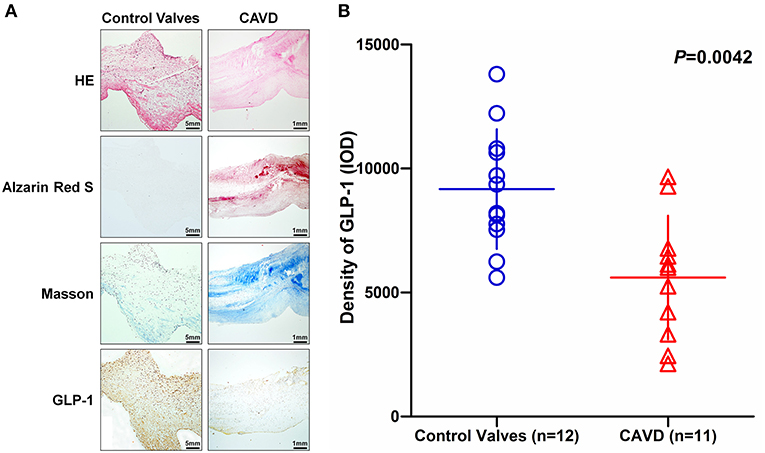
Figure 2. The distribution of GLP-1 in aortic valves with or without calcification. Human aortic valves with calcification (n = 11) that underwent valve replacement operation and without calcification (n = 12) undergoing heart transplantation were assessed by histological and immunochemical analysis. (A) Sections were stained with hematoxylin and eosin, Alizarin Red S, and Masson trichrome staining. IHC stains of GLP-1 and counterstained with hematoxylin. The results of the Non-CAVD valves (left line) are shown at 100 × magnification, and the results of CAVD (right line) are shown at 20 × magnification. (B) The concentration of GLP-1 detected by IHC was determined by assessing its staining with Image-Pro Plus 6.0. The results are shown as the integrated optical density (IOD)/area. The Non-CAVD (n = 12) and CAVD valves (n = 11) are representative of three independent experiments, and five different fields in each section were detected (the P-value was control valves compared with CAVD).
GLP-1 Regulates Calcification of AVICs
Based on the GLP-1 concentration of patients' serum in this study (Table 2), we treated AVICs with different doses of GLP-1 to identify the effect of GLP-1 on calcification in vitro. Alizarin Red S staining showed that the osteogenic medium (used in the Controls) induced AVIC mineralization, but a higher dose (25–100 pmol/L) of GLP-1 reversed the calcification of AVICs (Figure 3A). The Alizarin Red S dilution results [Control = 213.5 ± 9.248 μg/mg; GLP-1 (12.5 pmol/L) = 203.7 ± 7.535 μg/mg vs. Control, P = 0.318; GLP-1 (25 pmol/L) = 176.3 ± 5.754 μg/mg vs. Control, P = 0.033; GLP-1 (50 pmol/L) = 149.7 ± 7.632 μg/mg vs. Control, P = 0.007; GLP-1 (100 pmol/L) = 101.7 ± 8.950 μg/mg vs. Control, P = 0.001] and the activation of ALP [Control=605.5 ± 20.53 U/mg; GLP-1 (12.5 pmol/L) = 595.7 ± 18.26 U/mg vs. Control, P = 0.513; GLP-1 (25 pmol/L) = 519.5 ± 12.73 U/mg vs. Control, P = 0.002; GLP-1 (50 pmol/L) = 358.7 ± 17.94 U/mg vs. Control, P = 0.001; GLP-1 (100 pmol/L) = 218.3 ± 12.41 U/mg vs. Control, P < 0.001] also showed that GLP-1 reduced AVIC calcification in a dose-dependent manner (Figures 3B,C).
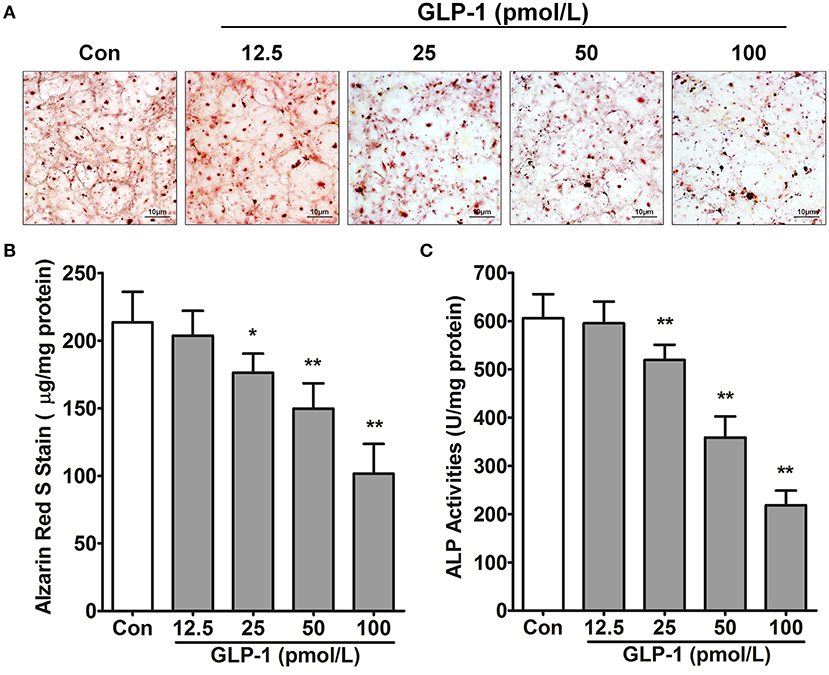
Figure 3. GLP-1 inhibits AVIC calcification. In osteogenic medium, the primary AVICs were incubated with increasing doses (12.5, 25, 50, and 100 pmol/L for 21 days) of GLP-1. The group without the GLP-1 treatment was used as a control (Con). (A) Alizarin Red S was used to stain the calcification cells. The pictures were taken using Image-Pro Plus 6.0. Alizarin Red S staining is shown at 400 × magnification. (B) Quantification of Alizarin Red S staining. (C) Quantification of ALP activation in AVICs. The dye was extracted and quantified as described in the Methods section (n = 6, mean ± SD, *P < 0.05, **P < 0.01 compared with Con).
In vitro, the AVICs were calcified over various periods from 7 to 21 days to mimic the process of aged-induced calcification. For further affirming GLP-1 regulated calcification of AVICs with time, we also treated AVICs with 100 pmol/L GLP-1 to identify the time-dependent effect of GLP-1 on calcification from 7 to 21 days. Alizarin Red S staining showed that the extent of calcification rapidly and significantly increased with the time extension (7 days = 129.0 ± 9.2 μg/mg, 14 days = 161.0 ± 6.4 μg/mg, and 21 days = 218.5 ± 7.6 μg/mg compared with 7 days: P < 0.01); however, GLP-1 significantly weakened this tendency (respectively, 79.0 ± 7.1, 91.3 ± 10.8, and 108.3 ± 10.5 μg/mg compared with the same groups without GLP-1 treatment: P < 0.01) (Figures 4A,B). Compared with the Control groups (7 days = 350.8 ± 28.2 U/mg, 14 days = 495.8 ± 37.2 U/mg, 21 days = 624.8 ± 40.9 U/mg, compared with 7 days: P < 0.01), a similar effect of GLP-1 with age (GLP-1: 7 days = 147.2 ± 21.3 U/mg, 14 days = 174.3 ± 19.1 U/mg, and 21 days = 212.8 ± 26.5 U/mg compared with same groups without GLP-1 treatment: P < 0.01) was demonstrated in the ALP activation test (Figure 4C).
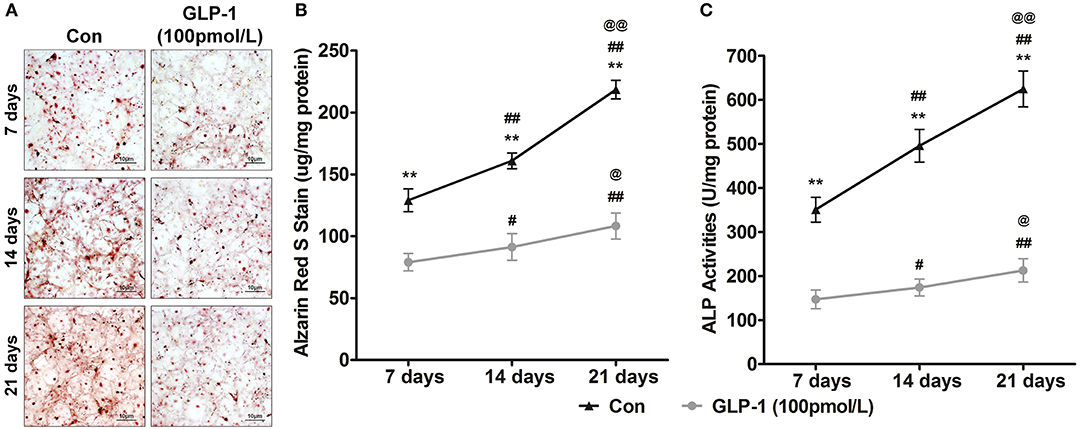
Figure 4. GLP-1 postpones the age-induced mineralization of AVICs. To determine the effect of GLP-1 on age-induced mineralization in AVICs, primary AVICs were cultured for 7, 14, and 21 days in osteogenic medium plus GLP-1 (100 pmol/L) or not. The group without the GLP-1 treatment was used as a control (Con). (A) Alizarin Red S stained the calcification cells, and the pictures were taken using Image-Pro Plus 6.0. Alizarin Red S staining is shown at 400 × magnification. (B) Quantification of Alizarin Red S staining. (C) Quantification of ALP activation in AVICs. The dye was extracted and quantified as described in the Methods section (n = 6, mean ± SD, **P < 0.01 compared with Con; #P < 0.05, ##P < 0.01 compared with 7-day culture; @P < 0.05, @@P < 0.01 compared with 14-day culture).
GLP-1 Regulated Calcification-Related Gene Expression
Many genes participated in AVIC mineralization, RUNX2 (29), MSX2 (30), and SOX9 (31) act as nuclear transcription factors to regulate downstream gene transcription. For example, the target genes BMP2 and BMP4 (32) promoted calcification of AVICs. Thus, we tested whether GLP-1 inhibited AVIC calcification by regulating the expression of these genes. First, the mRNA levels of RUNX2, MSX2, SOX9, BMP2, and BMP4 were detected by real-time PCR. Glucagon-like peptide-1 decreased the transcription of RUNX2 by 62% (Control = 1.01 ± 0.02 vs. GLP-1 = 0.38 ± 0.04, P < 0.01), MSX2 by 54% (Control = 1.00 ± 0.02 vs. GLP-1 = 0.46 ± 0.06, P < 0.01), BMP2 by 46% (Control = 1.00 ± 0.01 vs. GLP-1 = 0.54 ± 0.06, P < 0.01), and BMP4 by 59% (Control = 1.01 ± 0.03 vs. GLP-1 = 0.41 ± 0.02, P < 0.01) but increased SOX9 2.01-fold (Control = 1.00 ± 0.02 vs. GLP-1 = 2.01 ± 0.14, P < 0.01) (Figure 5A). Second, the distributions of RUNX2, MSX2, SOX9, BMP2, and BMP4 were identified by immunofluorescence in AVICs. RUNX2, MSX2, and SOX9 were located in the nucleus; BMP2 and BMP4 were expressed throughout the cells. GLP-1 also reduced the levels of RUNX2, MSX2, BMP2, and BMP4 but induced SOX9 (Figure 5B). Finally, the concentrations of these proteins were measured by western blot and analyzed by the IOD value (ratio of proteins/β-actin). GLP-1 decreased the expression of RUNX2 by 49% (Control = 2.21 ± 0.09 vs. GLP-1 = 1.12 ± 0.17, P < 0.01), MSX2 by 53% (Control = 1.75 ± 0.08 vs. GLP-1 = 0.83 ± 0.07, P < 0.01), BMP2 by 57% (Control = 1.38 ± 0.13 vs. GLP-1 = 0.60 ± 0.10, P < 0.01), and BMP4 by 48% (Control = 1.70 ± 0.09 vs. GLP-1 = 0.89 ± 0.16, P < 0.01) but increased SOX9 1.98-fold (Control = 0.90 ± 0.13 vs. GLP-1 = 1.78 ± 0.13, P < 0.01) (Figure 5C).
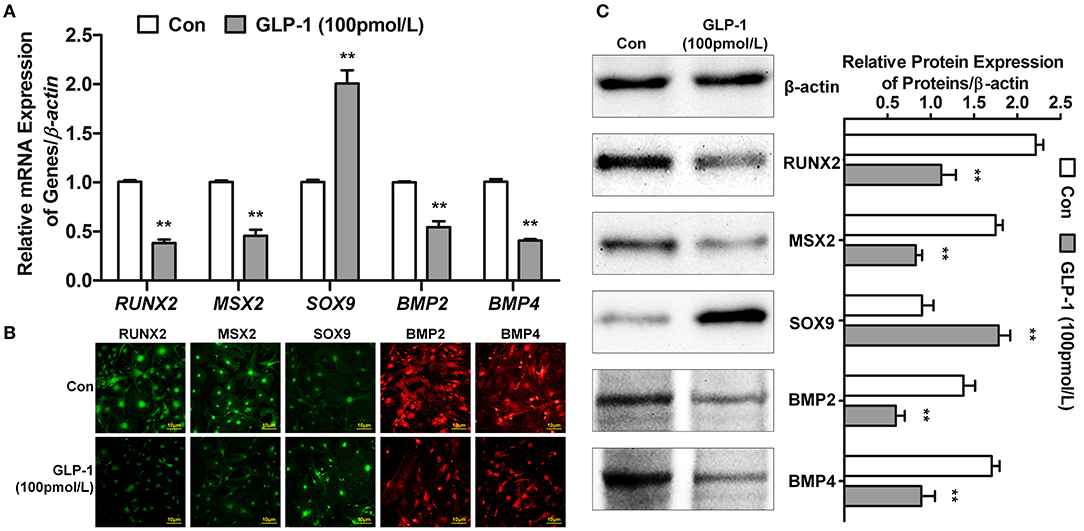
Figure 5. GLP-1 regulates the expression of calcification-related genes. The primary AVICs were stimulated with or without GLP-1 (100 pmol/L) for 21 days in osteogenic medium. The group without the GLP-1 treatment was used as a control (Con). (A) RUNX2, MSX2, SOX9, BMP2, and BMP4 were detected by real-time PCR. (B) The protein expression and location of RUNX2, MSX2, SOX9, BMP2, and BMP4 were determined by immunofluorescence analysis. RUNX2, MSX2, and SOX9 were assessed using Alexa Fluor 488-conjugated secondary antibody (Green); BMP2 and BMP4 were assessed using Alexa Fluor 549-conjugated secondary antibody (Red). (C) RUNX2, MSX2, SOX9, BMP2, and BMP4 were detected by western blot. The results are representative of three independent experiments. Real-time PCR (A) and western blot (C) quantified as relative units (genes or proteins/β-actin) (n = 3, mean ± SD, **P < 0.01 compared with Con).
Clinical Characteristics and GLP-1 Level in Serum of Non-CAVD and CAVD Groups
Previous results showed the concentration of GLP-1 decreased in valves of CAVD patients. In vitro, GLP-1 reversed mmineralization of AVICs with dose- and time-dependent manner, and GLP-1 downregulated pro-calcification genes expression but upregulated anti-calcification genes expression. The serum of CAVD (n = 200) and non-CAVD (n = 197) were collected to analysis the relationship of GLP-1 concentration and CAVD.
The demographic and clinical characteristics of the study participants were summarized in Table 2. Compared with the non-CAVD group, the patients in the CAVD group had a higher age range (non-CAVD: 59.02 ± 8.59 years vs. CAVD: 74.14 ± 7.67 years; P < 0.001), percentage of hypertension (non-CAVD: 64.5% vs. CAVD: 77.0%; P = 0.008), and rate of coronary heart disease (non-CAVD: 68.0% vs. CAVD: 88.5%; P < 0.001). There were no significant difference in sex, body mass index (BMI), active smoking, alcohol, percentage of diabetes, fasting glucose, HbA1c or γ-glutamyl transpeptidase between the two groups. The diastolic blood pressure was significantly higher in the CAVD group (Non-CAVD: 131.49 ± 17.73 vs. CAVD: 139.78 ± 20.68; P < 0.001), but the systolic blood pressure was significantly lower (Non-CAVD: 77.07 ± 11.70 vs. CAVD: 74.42 ± 11.43; P = 0.023). Calcific aortic valve disease patients had higher lipoprotein (A) [Non-CAVD: 0.12 (0.20) vs. CAVD: 0.14 (0.30); P = 0.026] and lower triglycerides [Non-CAVD: 1.78 ± 1.13 vs. CAVD: 1.56 ± 0.86; P = 0.025] and LDL (Non-CAVD: 1.13 ± 0.26 vs. CAVD: 1.07 ± 0.29; P = 0.044), but not total cholesterol or HDL. Renal and blood urea nitrogen (Non-CAVD: 5.06 ± 1.29 mmol/L vs. CAVD: 6.03 ± 2.40 mmol/L; P < 0.001), creatinine (Non-CAVD: 74.07 ± 16.07 mmol/L vs. CAVD: 86.38 ± 24.25 mmol/L; P < 0.001), and eGFR (CKD-EPI) (Non-CAVD: 90.39 ± 17.62 ml/min vs. CAVD: 72.29 ± 18.23 ml/min; P < 0.001) exhibited significant pathogenic propensity changes. No significant difference was observed in terms of the medication treatment between the Non-CAVD and CAVD groups.
Serum Similar to the IHC result for calcific aortic valves, serum GLP-1 was significantly reduced in the CAVD group [Non-CAVD: median = 14.00 pmol/L (25th−75th percentile 9.34–19.02 pmol/L; range 0.38–39.37 pmol/L) vs. CAVD: median = 11.29 pmol/L (25th−75th percentile 8.17–14.92 pmol/L; range 0.05–31.93 pmol/L), P < 0.001] (Table 2).
GLP-1 as an Independent Factor for CAVD
Based on the multivariable regression analysis, age (OR = 1.255; 95% CI 1.199–1.313), fasting glucose (OR = 0.824; 95% CI, 0.702–0.968), HbA1c (OR = 1.542; 95% CI, 1.171–2.031), HDL (OR = 0.142; 95% CI, 0.045–0.443), BUN (OR = 1.270; 95% CI, 1.032–1.563), and GLP-1 (OR = 0.889; 95% CI, 0.844–0.936) were independently associated with CAVD risk (Model 1 in Table 3). When the GLP-1 quartile (median = 12.31 pmol/L, 25th−75th percentiles 8.68–16.73 pmol/L; range 0.05–39.37 pmol/L) in the Non-CAVD and CAVD groups was included in Model 2 (baseline characteristics of the Non-CAVD and CAVD groups according to GLP-1 level, see Supplementary Table 2), the association between age, fasting glucose, HbA1c, HDL, BUN, and CAVD remained significant. Compared with the lowest quartile, the OR-values of the other quartiles decreased from 0.818 to 0.115. However, only the highest quartile (OR = 0.115; 95% CI, 0.045–0.291) was significantly associated with lower risk of CAVD (Model 2 in Table 3).
Age represented a remarkable risk factor for CAVD and it presented the largest difference from the Non-CAVD group in this study. Therefore, the interaction between GLP-1 quartile and age as covariates was analyzed by multivariable regression analysis. In this analysis, age, fasting glucose, HbA1c, HDL, and BUN remained independent risk factors associated with CAVD. Compared with the lowest quartile, higher GLP-1 reduced the aged-induced risk of pathogenesis from 0.996 to 0.966 (Table 4). Only the fourth quartile (OR = 0.966; 95% CI, 0.953–0.980) was significantly associated with CAVD (Table 4).
Discussion
Although some clinical, genetic, and animal studies have led to a partial understanding of CAVD, truly important advancements in the disease management (such as optimal diagnosis and treatment strategies) remain out of reach. This area of study thus requires further investigations, especially regarding endogenous protective factors. In this study, we found that GLP-1, a negative independent risk factor, was decreased in aortic valve and serum of CAVD patients. Glucagon-like peptide-1 is also associated with reduced the odds of CAVD and inhibited AVIC mineralization by regulating calcification related-genes. Thus, GLP-1 exhibited protective characteristics to antagonize CAVD.
The present study found that age, hypertension, systolic blood pressure, diastolic blood pressure, CAVD, triglycerides, LDL, lipoprotein (A), blood urea nitrogen, creatinine, and eGFR (CKD-EPI) (as pathogenic factors) significantly differed between the Non-CAVD and CAVD groups (Table 2). Among these indicators, age (33), hypertension (4), blood pressure (4), CAD (34), lipoprotein (A) (35), and renal function (36) have been reported to participate in and show pathogenicity associated with CAVD. However, there has been little research regarding the protective factors of CAVD. For these reasons, we focused on GLP-1, which has beneficial effects and is associated with LV diastolic function (37), heart rate (38), cardiac remodeling (39), blood pressure (38), lipid profile (40), and cardiovascular disease independent of adiposity or diabetes (41). Notably, the role of GLP-1 had not been reported in CAVD. We found that the level of GLP-1 was not only decreased in the serum of the CAVD group (Table 2) but was also reduced in calcified aortic valves (Figure 2). Thus, GLP-1 may be associated with CAVD; as expected, multivariable regression analysis found that GLP-1 was a negative independent factor for CAVD (OR = 0.922; 95% CI, 0.887–0.958) (Table 3) and significantly weakened the odds risk of CAVD. These results indicate that the variation in GLP-1 concentration affects CAVD. To determine the influence of GLP-1 concentration on CAVD, GLP-1 concentration was divided into quartiles. The patients with the highest quartile of GLP-1 showed the lowest rate of CAVD (31.3%, P < 0.001; Supplementary Table 2), and the highest quartile showed a significantly strong negative correlation with CAVD risk (OR = 0.115; 95% CI, 0.045–0.291), which demonstrated that a high dose of GLP-1 exerted an antagonistic effect on the odds risk of CAVD (Table 3). Thus, GLP-1 is a novel protective factor negatively associated with CAVD, and decreases in GLP-1 lead to the progressive calcification of the aortic valve.
Glucagon-like peptide-1 is an incretin hormone that is secreted into the serum by enteroendocrine L-cells (distal ileum and colon) and K-cells (duodenum and jejunum) (42); however, we found GLP-1 in non-mineralized aortic valve regions with or without calcified lesions, which indicates that GLP-1 was secreted from intestinal cells and recruited to the aortic valve to influence the function of AVICs. Glucagon-like peptide-1 localizes to interstitial spaces and tissues to regulate metabolic diseases, such as diabetes and obesity (43, 44); GLP-1 also regulates cell functions to protect against cardiovascular disease. In vitro and in vivo atherosclerosis studies demonstrate that GLP-1 promotes vasodilatation and suppresses the inflammatory response in endothelial cells, inhibits lipid uptake and inflammatory activity in macrophages, and represses the proliferation of smooth muscle cell (SMCs) to prevent atherosclerosis progression (45). In arterial calcification, similar to bone formation, VSMCs differentiate to the osteoblastic phenotype to play a key role in arterial calcification; however, GLP-1 inhibits osteoblastic differentiation and calcification in human VSMCs (20). There are several similarities between CAVD and arterial calcification; however, AVIC heterogenization and mineralization are key components of the cytopathology in CAVD, and these components differ from those in arterial calcification. In this study, the level of GLP-1 decreased by 19.3% in CAVD serum (Table 2) and 39% in calcified aortic valve (Figure 2), which indicated that a reduction in GLP-1 in the aortic valve caused AVIC calcification. However, whether GLP-1 can reverse CAVD by regulating AVIC osteoblastic differentiation and calcification was not known. To investigate this, we added various doses of GLP-1 to AVICs during the standard process of calcification. Glucagon-like peptide-1 significantly attenuated the density of Alizarin Red S and the activation of ALP at higher doses (Figure 3), which demonstrated that GLP-1 could attenuate CAVD by preventing the mineralization of AVICs. Considering this plus the results of Table 3, we hypothesized that high GLP-1 weakens the risk of CAVD. Our results indicate that GLP-1 inhibits the calcification of AVICs to exert its protective function in CAVD.
Calcific aortic valve disease is a chronic degenerative disease that has multiple risk factors, including diabetes (46), hypertension (4), dyslipidemia (36), and kidney disease (47). The Multi-Ethnic Study of Atherosclerosis (MESA) found that non-Hispanic whites had the highest frequency of CAVD, followed by Hispanics and blacks, which indicates that CAVD presents racial differences (48). Our study in a Chinese population found that age, fasting glucose, HbA1c, HDL, BUN, and GLP-1 were independent risk factors for CAVD, which indicates that age, diabetes, dyslipidemia and renal insufficiency were associated with CAVD in a Chinese population.
Although multiple pathogenic factors take part in CAVD, age is an important and irreversible risk factor and has the strongest correlation with CAVD (48). A previous study showed that more than 50% of patients with aortic valve calcification were older than 75 years, whereas severe stenosis was found in 2–3% of this elderly population (49). And some reports shown with aged, AVICs can form calcium node (50). In our experiments, we found in extended-duration in vitro calcification culture, the mineralization level of AVICs increased (Figure 4). These results illustrate that age plays a key role in CAVD mineralization. Moreover, we previously found an effect of GLP-1 on neuroprotection via its reversal of age-induced neurodegeneration, such as that in Alzheimer's and Parkinson's diseases (51).We observed that GLP-1 not only inhibited mineralization but also reduced time-dependent calcification in AVICs from 7 to 21 days (Figure 4). These results demonstrate that the leading risk factor of CAVD may can be attenuated by GLP-1.
RUNX2, MSX2, SOX9, BMP2, and BMP4 are important proteins related to calcification. RUNX2 is an osteogenic and chondrogenic transcription factor that is regulated in multiple manners (52). RUNX2 is upregulated in atherosclerotic calcification and endochondral mineralization programs (29). Hydrogen peroxide activates osteogenic Cbfa1/RUNX2 (53) and MSX2/Wnt signaling (30), thereby enhancing mineralization. Miller et al. also found that both of these regulatory cascades were activated in calcifying human aortic valves (54). Acharya et al. (31) demonstrated that Notch1 maintains SOX9 expression to inhibit osteogenic mineralization in AVICs. BMP2 and BMP4 increase the secretion of OPN by upregulating ALP, resulting in the degradation of tissue pyrophosphate (32). Glucagon-like peptide-1 attenuates osteoblastic differentiation and calcification by inhibiting ALP, osteocalcin (OC), and RUNX2 in human VSMCs (20), but whether GLP-1 regulates the expression of MSX2, SOX9, BMP2, and BMP4 remains unknown. As we observed in this study, GLP-1 decreased the expression of RUNX2, MSX2, BMP2, and BMP4 but increased the expression of SOX9 in AVICs (Figure 5), which were first suggested a relationship between GLP-1 and these genes in the AVIC calcification process. These results indicate that GLP-1 reversed mineralization in AVICs via two pathways, first by inhibiting the expression of osteogenic genes and second by promoting the expression of anti-osteogenic genes. These results indicate that GLP-1 reversed mineralization in AVICs via two pathways, first by inhibiting the expression of osteogenic genes and second by promoting the expression of anti-osteogenic genes.
This study showed that the level of GLP-1 decreased in both the local calcific aortic valve and in the serum of CAVD patients and that this decrease was associated with age. This indicated that GLP-1 could have value in predicting the occurrence and development of CAVD.
Study Limitations
In this study, we examined the role of GLP-1 in CAVD; however, some in vivo experiments and details of the molecular mechanism were lacking. We found HDL and fasting glucose to be negative independent risk factors associated with CAVD. HDL has anti-oxidative and anti-inflammatory properties, but the role of HDL in CAVD is not clear. Interestingly, our study found that fasting glucose was associated with a reduced risk of CAVD and that high glucose reversed AVIC calcification (data not shown); however, the mechanisms of these effects remain unknown. HDL and fasting glucose in CAVD will be investigated in further studies. Moreover, as a single center cross-sectional study with retrospective characteristic, it might be susceptible to center biases or recall bias. Also, as a retrospective study, the study only indicated associations but not formulate causal relationships. Although we took multiple clinical important cofounders into consideration, it might be possible that unknown potential factors may be missed and it might interfere in our findings. Finally, CAVD was only assessed by echocardiography. Cardiac computed tomography is also another useful approach to quantify aortic valve calcium burden. Finally, although we carefully controlled for the major known confounders, unknown factors may still have interfered in our findings.
Therefore, the results remained to be further confirmed in larger sample size study with prospective randomized controlled designs.
Conclusion
Valve tissue and serum from CAVD patients were characterized with lower level of GLP-1. Clinical and cellular evidence suggests that GLP-1 participates in the pathological calcification of the aortic valve. Calcific aortic valve disease is highly prevalent in the elderly, and there are currently no absolute effective treatments to reverse its progression. This study reveals some novel characteristics of GLP-1 and its potential therapeutic value for CAVD.
Data Availability Statement
The raw data supporting the conclusions of this article will be made available by the authors, without undue reservation.
Ethics Statement
The studies involving human participants were reviewed and approved by Shanghai Ninth People's Hospital+Shanghai Jiaotong University School of Medicine. The patients/participants provided their written informed consent to participate in this study. The animal study was reviewed and approved by Shanghai Ninth People's Hospital+Shanghai Jiaotong University School of Medicine.
Author Contributions
YL and KY designed the study, performed data analysis and interpretation, and drafted the manuscript. FX, QZha, and QZhang performed data collection and analysis. FX, QW, and YY contributed to interpretation, drafting, and editing the manuscript. YL and KY provided study design, interpretation, wrote and edited manuscript, are the guarantors of this work and takes full responsibility for the work as a whole, including the study design, access to data, and the decision to submit and publish the manuscript. All authors contributed to conception and design, acquisition of data or analysis and interpretation of data, drafting the article or revising it critically for important intellectual content, and gave final approval of the version to be published.
Funding
This work was supported by grants from the Natural Science Foundation of China (81770384, 82070401, 81760734, and 81800339), the Natural Science Foundation of Yunnan Province (No. 2017FA048), the fund of Diabetic Innovation Team (2019HC002), and Endocrine Clinical Medical Center of Yunnan Province (No. ZX2019-02-02). The fund of medical leader in Yunnan Province (No. L-201609). Fundamental research program funding of Ninth People's Hospital affiliated to Shanghai Jiao Tong University School of Medicine (No. JYZZ100).
Conflict of Interest
The authors declare that the research was conducted in the absence of any commercial or financial relationships that could be construed as a potential conflict of interest.
Publisher's Note
All claims expressed in this article are solely those of the authors and do not necessarily represent those of their affiliated organizations, or those of the publisher, the editors and the reviewers. Any product that may be evaluated in this article, or claim that may be made by its manufacturer, is not guaranteed or endorsed by the publisher.
Acknowledgments
We would like to thank Dr. Ying Huang and her associates in the core facility unit (School of Medicine, Shanghai Jiao Tong University) for their professional support in imaging capture and processing.
Supplementary Material
The Supplementary Material for this article can be found online at: https://www.frontiersin.org/articles/10.3389/fcvm.2021.709741/full#supplementary-material
References
1. Baggio LL, Drucker DJ. Biology of incretins: GLP-1 and GIP. Gastroenterology. (2007). 132:2131–57. doi: 10.1053/j.gastro.2007.03.054
2. Tahrani AA, Bailey CJ, Del Prato S, Barnett AH. Management of type 2 diabetes: new and future developments in treatment. Lancet. (2011). 378:182–97. doi: 10.1016/S0140-6736(11)60207-9
3. Li Y, Perry T, Kindy MS, Harvey BK, Tweedie D, Holloway HW, et al. GLP-1 receptor stimulation preserves primary cortical and dopaminergic neurons in cellular and rodent models of stroke and Parkinsonism. Proc Natl Acad Sci USA. (2009). 106:1285–90. doi: 10.1073/pnas.0806720106
4. Rajamannan NM, Evans FJ, Aikawa E, Grande-Allen KJ, Demer LL, Heistad DD, et al. Calcific aortic valve disease: not simply a degenerative process: A review and agenda for research from the National Heart and Lung and Blood Institute Aortic Stenosis Working Group. Executive summary: Calcific aortic valve disease-2011 update. Circulation. (2011). 124:1783–91. doi: 10.1161/CIRCULATIONAHA.110.006767
5. Otto CM, Kuusisto J, Reichenbach DD, Gown AM, O'Brien KD. Characterization of the early lesion of 'degenerative' valvular aortic stenosis. Histological and immunohistochemical studies. Circulation. (1994) 90:844–53. doi: 10.1161/01.CIR.90.2.844
6. Zeng Q, Jin C, Ao L, Cleveland JC Jr, Song R, Xu D, et al. Cross-talk between the Toll-like receptor 4 and Notch1 pathways augments the inflammatory response in the interstitial cells of stenotic human aortic valves. Circulation. (2012). 126:S222–30. doi: 10.1161/CIRCULATIONAHA.111.083675
7. Shavelle DM, Takasu J, Budoff MJ, Mao S, Zhao XQ, O'Brien KD. HMG CoA reductase inhibitor (statin) and aortic valve calcium. Lancet. (2002). 359:1125–6. doi: 10.1016/S0140-6736(02)08161-8
8. Bouchareb R, Mahmut A, Nsaibia MJ, Boulanger MC, Dahou A, Lepine JL, et al. Autotaxin derived from lipoprotein (A) and valve interstitial cells promotes inflammation and mineralization of the aortic valve. Circulation. (2015). 132:677–90. doi: 10.1161/CIRCULATIONAHA.115.016757
9. Freeman RV, Otto CM. Spectrum of calcific aortic valve disease: pathogenesis, disease progression, and treatment strategies. Circulation. (2005). 111:3316–26. doi: 10.1161/CIRCULATIONAHA.104.486738
10. Merryman WD. Insights into (the interstitium of) degenerative aortic valve disease. J Am Coll Cardiol. (2008). 51:1415; author reply 1416. doi: 10.1016/j.jacc.2007.11.068
11. Wirrig EE, Hinton RB, Yutzey KE. Differential expression of cartilage and bone-related proteins in pediatric and adult diseased aortic valves. J Mol Cell Cardiol. (2011). 50:561–9. doi: 10.1016/j.yjmcc.2010.12.005
12. Yetkin E, Waltenberger J. Molecular and cellular mechanisms of aortic stenosis. Int J Cardiol. (2009). 135:4–13. doi: 10.1016/j.ijcard.2009.03.108
13. Munaf M, Pellicori P, Allgar V, Wong K. A meta-analysis of the therapeutic effects of glucagon-like peptide-1 agonist in heart failure. Int J Pept. (2012). 2012:249827. doi: 10.1155/2012/249827
14. Hausenloy DJ, Whittington HJ, Wynne AM, Begum SS, Theodorou L, Riksen N, et al. Dipeptidyl peptidase-4 inhibitors and GLP-1 reduce myocardial infarct size in a glucose-dependent manner. Cardiovasc Diabetol. (2013). 12:154. doi: 10.1186/1475-2840-12-154
15. Woo JS, Kim W, Ha SJ, Kim JB, Kim SJ, Kim WS, et al. Cardioprotective effects of exenatide in patients with ST-segment-elevation myocardial infarction undergoing primary percutaneous coronary intervention: results of exenatide myocardial protection in revascularization study. Arterioscler Thromb Vasc Biol. (2013). 33:2252–60. doi: 10.1161/ATVBAHA.113.301586
16. Sokos GG, Nikolaidis LA, Mankad S, Elahi D, Shannon RP. Glucagon-like peptide-1 infusion improves left ventricular ejection fraction and functional status in patients with chronic heart failure. J Card Fail. (2006). 12:694–9. doi: 10.1016/j.cardfail.2006.08.211
17. Liu AC, Joag VR, Gotlieb AI. The emerging role of valve interstitial cell phenotypes in regulating heart valve pathobiology. Am J Pathol. (2007). 171:1407–18. doi: 10.2353/ajpath.2007.070251
18. Jian B, Narula N, Li QY, Mohler ER III, Levy RJ. Progression of aortic valve stenosis: TGF-beta1 is present in calcified aortic valve cusps and promotes aortic valve interstitial cell calcification via apoptosis. Ann Thorac Surg. (2003). 75:457–65; discussion 465–6. doi: 10.1016/S0003-4975(02)04312-6
19. Galeone A, Brunetti G, Oranger A, Greco G, Di Benedetto A, Mori G, et al. Aortic valvular interstitial cells apoptosis and calcification are mediated by TNF-related apoptosis-inducing ligand. Int J Cardiol. (2013). 169:296–304. doi: 10.1016/j.ijcard.2013.09.012
20. Zhan JK, Wang YJ, Wang Y, Tang ZY, Tan P, Huang W, et al. The protective effect of GLP-1 analogue in arterial calcification through attenuating osteoblastic differentiation of human VSMCs. Int J Cardiol. (2015). 189:188–93. doi: 10.1016/j.ijcard.2015.04.086
21. Baumgartner H, Hung J, Bermejo J, Chambers JB, Edvardsen T, Goldstein S, et al. Recommendations on the echocardiographic assessment of aortic valve stenosis: a focused update from the European Association of Cardiovascular Imaging and the American Society of Echocardiography. J Am Soc Echocardiogr. (2017). 30:372–92. doi: 10.1016/j.echo.2017.02.009
22. American College of Cardiology/American Heart Association Task Force on Practice Guidelines; Society of Cardiovascular Anesthesiologists; Society for Cardiovascular Angiography Interventions; Society of Thoracic Surgeons; Bonow RO Carabello BA Kanu C . ACC/AHA 2006 guidelines for the management of patients with valvular heart disease: a report of the American College of Cardiology/American Heart Association Task Force on Practice Guidelines (writing Committee to Revise the 1998 guidelines for the management of patients with valvular heart disease) developed in collaboration with the Society of Cardiovascular Anesthesiologists endorsed by the Society for Cardiovascular Angiography and Interventions and the Society of Thoracic Surgeons. J Am Coll Cardiol. (2006). 48:e1–148. doi: 10.1161/CIRCULATIONAHA.106.176857
23. Puavilai G, Chanprasertyotin S, Sriphrapradaeng A. Diagnostic criteria for diabetes mellitus and other categories of glucose intolerance: 1997 criteria by the Expert Committee on the Diagnosis and Classification of Diabetes Mellitus (ADA), 1998 WHO consultation criteria, and 1985 WHO criteria. World Health Organization. Diabetes Res Clin Pract. (1999). 44:2–6. doi: 10.1016/S0168-8227(99)00008-X
24. Chobanian AV, Bakris GL, Black HR, Cushman WC, Green LA, Izzo JL, et al. Seventh report of the joint national committee on prevention, detection, evaluation, and treatment of high blood pressure. Hypertension. (2003). 42:1206–52. doi: 10.1161/01.HYP.0000107251.49515.c2
25. Taylor AJ, Cerqueira M, Hodgson JM, Mark D, Min J, O'Gara P, et al. ACCF/SCCT/ACR/AHA/ASE/ASNC/NASCI/SCAI/SCMR 2010 appropriate use criteria for cardiac computed tomography. A report of the American College of Cardiology Foundation Appropriate Use Criteria Task Force, the Society of Cardiovascular Computed Tomography, the American College of Radiology, the American Heart Association, the American Society of Echocardiography, the American Society of Nuclear Cardiology, the North American Society for Cardiovascular Imaging, the Society for Cardiovascular Angiography and Interventions, and the Society for Cardiovascular Magnetic Resonance. J Am Coll Cardiol. (2010). 56:1864–94. doi: 10.1016/j.jacc.2010.07.005
26. Mohler ER III, Chawla MK, Chang AW, Vyavahare N, Levy RJ, et al. Identification and characterization of calcifying valve cells from human and canine aortic valves. J Heart Valve Dis. (1999). 8:254–60.
27. Mathieu P, Voisine P, Pepin A, Shetty R, Savard N, Dagenais F. Calcification of human valve interstitial cells is dependent on alkaline phosphatase activity. J Heart Valve Dis. (2005). 14:353–7.
28. Osman L, Yacoub MH, Latif N, Amrani M, Chester AH. Role of human valve interstitial cells in valve calcification and their response to atorvastatin. Circulation. (2006). 114:I547–52. doi: 10.1161/CIRCULATIONAHA.105.001115
29. Tyson KL, Reynolds JL, McNair R, Zhang Q, Weissberg PL, Shanahan CM. Osteo/chondrocytic transcription factors and their target genes exhibit distinct patterns of expression in human arterial calcification. Arterioscler Thromb Vasc Biol. (2003). 23:489–94. doi: 10.1161/01.ATV.0000059406.92165.31
30. Lai CF, Shao JS, Behrmann A, Krchma K, Cheng SL, Towler DA. TNFR1-activated reactive oxidative species signals up-regulate osteogenic Msx2 programs in aortic myofibroblasts. Endocrinology. (2012). 153:3897–910. doi: 10.1210/en.2012-1216
31. Acharya A, Hans CP, Koenig SN, Nichols HA, Galindo CL, Garner HR, et al. Inhibitory role of Notch1 in calcific aortic valve disease. PLoS ONE. (2011). 6:e27743. doi: 10.1371/journal.pone.0027743
32. Steitz SA, Speer MY, McKee MD, Liaw L, Almeida M, Yang H, et al. Osteopontin inhibits mineral deposition and promotes regression of ectopic calcification. Amer J Pathol. (2002). 161:2035–46. doi: 10.1016/S0002-9440(10)64482-3
33. Nkomo VT, Gardin JM, Skelton TN, Gottdiener JS, Scott CG, Enriquez-Sarano M. Burden of valvular heart diseases: a population-based study. Lancet. (2006). 368:1005–11. doi: 10.1016/S0140-6736(06)69208-8
34. Pohle K, Maffert R, Ropers D, Moshage W, Stilianakis N, Daniel WG, et al. Progression of aortic valve calcification: association with coronary atherosclerosis and cardiovascular risk factors. Circulation. (2001). 104:1927–32. doi: 10.1161/hc4101.097527
35. Hung MY, Witztum JL, Tsimikas S. New therapeutic targets for calcific aortic valve stenosis: the lipoprotein(a)-lipoprotein-associated phospholipase A2-oxidized phospholipid axis. J Am Coll Cardiol. (2014). 63:478–80. doi: 10.1016/j.jacc.2013.08.1639
36. Stewart BF, Siscovick D, Lind BK, Gardin JM, Gottdiener JS, Smith VE, et al. Clinical factors associated with calcific aortic valve disease. Cardiovascular Health Study. J Am Coll Cardiol. (1997). 29:630–4. doi: 10.1016/S0735-1097(96)00563-3
37. Nathanson D, Zethelius B, Berne C, Lind L, Andren B, Ingelsson E, et al. Plasma levels of glucagon like peptide-1 associate with diastolic function in elderly men. Diabet Med. (2011). 28:301–5. doi: 10.1111/j.1464-5491.2010.03207.x
38. Trahair LG, Horowitz M, Stevens JE, Feinle-Bisset C, Standfield S, Piscitelli D, et al. Effects of exogenous glucagon-like peptide-1 on blood pressure, heart rate, gastric emptying, mesenteric blood flow and glycaemic responses to oral glucose in older individuals with normal glucose tolerance or type 2 diabetes. Diabetologia. (2015). 58:1769–78. doi: 10.1007/s00125-015-3638-0
39. DeNicola M, Du J, Wang Z, Yano N, Zhang L, Wang Y, et al. Stimulation of glucagon-like peptide-1 receptor through exendin-4 preserves myocardial performance and prevents cardiac remodeling in infarcted myocardium. Am J Physiol Endocrinol Metab. (2014). 307:E630–43. doi: 10.1152/ajpendo.00109.2014
40. Fisher M. Glucagon-like peptide 1 receptor agonists and cardiovascular risk in type 2 diabetes: a clinical perspective. Diabetes Obes Metab. (2015). 17:335–42. doi: 10.1111/dom.12380
41. Yamaoka-Tojo M, Tojo T, Takahira N, Matsunaga A, Aoyama N, Masuda T, et al. Elevated circulating levels of an incretin hormone, glucagon-like peptide-1, are associated with metabolic components in high-risk patients with cardiovascular disease. Cardiovasc Diabetol. (2010). 9:17. doi: 10.1186/1475-2840-9-17
42. Cabou C, Burcelin R. GLP-1, the gut-brain, and brain-periphery axes. Rev Diabet Stud. (2011). 8:418–31. doi: 10.1900/RDS.2011.8.418
43. Moran-Ramos S, Tovar AR, Torres N. Diet: friend or foe of enteroendocrine cells–how it interacts with enteroendocrine cells. Adv Nutr. (2012). 3:8–20. doi: 10.3945/an.111.000976
44. Moss C, Dhillo WS, Frost G, Hickson M. Gastrointestinal hormones: the regulation of appetite and the anorexia of ageing. J Hum Nutr Diet. (2012). 25:3–15. doi: 10.1111/j.1365-277X.2011.01211.x
45. Mita T, Watada H. Glucagon like peptide-1 and atherosclerosis. Cardiovasc Hematol Agents Med Chem. (2012). 10:309–18. doi: 10.2174/187152512803530388
46. Katz R, Wong ND, Kronmal R, Takasu J, Shavelle DM, Probstfield JL, et al. Features of the metabolic syndrome and diabetes mellitus as predictors of aortic valve calcification in the Multi-Ethnic Study of Atherosclerosis. Circulation. (2006). 113:2113–9. doi: 10.1161/CIRCULATIONAHA.105.598086
47. Ix JH, Shlipak MG, Katz R, Budoff MJ, Shavelle DM, Probstfield JL, et al. Kidney function and aortic valve and mitral annular calcification in the Multi-Ethnic Study of Atherosclerosis (MESA). Am J Kidney Dis. (2007). 50:412–20. doi: 10.1053/j.ajkd.2007.05.020
48. Nasir K, Katz R, Takasu J, Shavelle DM, Detrano R, Lima JA, et al. Ethnic differences between extra-coronary measures on cardiac computed tomography: Multi-Ethnic Study of Atherosclerosis (MESA). Atherosclerosis. (2008). 198:104–14. doi: 10.1016/j.atherosclerosis.2007.09.008
49. Lindroos M, Kupari M, Heikkila J, Tilvis R. Prevalence of aortic valve abnormalities in the elderly: an echocardiographic study of a random population sample. J Am Coll Cardiol. (1993). 21:1220–5. doi: 10.1016/0735-1097(93)90249-Z
50. Schnitzler JG, Ali L, Groenen AG, Kaiser Y, Kroon J. Lipoprotein(a) as orchestrator of calcific aortic valve stenosis. Biomolecules. (2019). 9:760. doi: 10.3390/biom9120760
51. Holscher C. Central effects of GLP-1: new opportunities for treatments of neurodegenerative diseases. J Endocrinol. (2014). 221:T31–41. doi: 10.1530/JOE-13-0221
52. Steitz SA, Speer MY, Curinga G, Yang HY, Haynes P, Aebersold R, et al. Smooth muscle cell phenotypic transition associated with calcification: upregulation of Cbfa1 and downregulation of smooth muscle lineage markers. Circ Res. (2001). 89:1147–54. doi: 10.1161/hh2401.101070
53. Byon CH, Javed A, Dai Q, Kappes JC, Clemens TL, Darley-Usmar VM, et al. Oxidative stress induces vascular calcification through modulation of the osteogenic transcription factor Runx2 by AKT signaling. J Biol Chem. (2008). 283:15319–27. doi: 10.1074/jbc.M800021200
Keywords: glucagon-like peptide-1, calcific aortic valve disease, calcification, aortic valve interstitial cells, age
Citation: Xiao F, Zha Q, Zhang Q, Wu Q, Chen Z, Yang Y, Yang K and Liu Y (2021) Decreased Glucagon-Like Peptide-1 Is Associated With Calcific Aortic Valve Disease: GLP-1 Suppresses the Calcification of Aortic Valve Interstitial Cells. Front. Cardiovasc. Med. 8:709741. doi: 10.3389/fcvm.2021.709741
Received: 14 May 2021; Accepted: 23 July 2021;
Published: 26 August 2021.
Edited by:
Joy Lincoln, Medical College of Wisconsin, United StatesReviewed by:
Kristyn Simcha Masters, University of Wisconsin-Madison, United StatesErnesto Greco, Sapienza University of Rome, Italy
Copyright © 2021 Xiao, Zha, Zhang, Wu, Chen, Yang, Yang and Liu. This is an open-access article distributed under the terms of the Creative Commons Attribution License (CC BY). The use, distribution or reproduction in other forums is permitted, provided the original author(s) and the copyright owner(s) are credited and that the original publication in this journal is cited, in accordance with accepted academic practice. No use, distribution or reproduction is permitted which does not comply with these terms.
*Correspondence: Yan Liu, liuyan_ivy@126.com; Ke Yang, ykk_ykkk@126.com
†These authors have contributed equally to this work