Corrigendum: Facts and Gaps in Exercise Influence on Arrhythmogenic Cardiomyopathy: New Insights From a Meta-Analysis Approach
- 1Cardiology Department, Hospital Universitario y Politécnico La Fe, Valencia, Spain
- 2Laboratorio de Cardiogenética, Unidad de Cardiopatías Familiares, Instituto Murciano de Investigación Biosanitaria (IMIB-Arrixaca), Murcia, Spain
- 3Unidad CSUR (Centros, Servicios y Unidades de Referencia) en Cardiopatías Familiares, Hospital Universitario Virgen de la Arrixaca, Murcia, Spain
- 4CIBERCV, Center for Biomedical Network Research on Cardiovascular Diseases, Madrid, Spain
- 5Unidad de Cardiopatías Familiares, Muerte Súbita y Mecanismos de Enfermedad (CaFaMuSMe), Instituto de Investigación Sanitaria La Fe, Valencia, Spain
- 6Cardiology Department, Hospital Universitario Virgen de la Arrixaca, Murcia, Spain
- 7Instituto de Medicina Legal y Ciencias Forenses de Valencia, Histology Unit, Universitat de València, Valencia, Spain
Arrhythmogenic cardiomyopathy (ACM) is a genetic cardiac condition characterized by fibrofatty myocardial replacement, either at the right ventricle, at the left ventricle, or with biventricular involvement. Ventricular arrhythmias and heart failure represent its main clinical features. Exercise benefits on mental and physical health are worldwide recognized. However, patients with ACM appear to be an exception. A thorough review of the literature was performed in PubMed searching for original papers with the terms “ARVC AND sports/exercise” and “sudden cardiac death AND sports/exercise.” Additional papers were then identified through other sources and incorporated to the list. All of them had to be based on animal models or clinical series. Information was structured in a regular format, although some data were not available in some papers. A total of 34 papers were selected and processed regarding sports-related sudden cardiac death, pre-clinical models of ACM and sport, and clinical series of ACM patients engaged in sports activities. Eligible papers were identified to obtain pooled data in order to build representative figures showing the global incidence of the most important causes of sudden cardiac death in sports and the global estimates of life-threatening arrhythmic events in ACM patients engaged in sports. Tables and figures illustrate their major characteristics. The scarce points of controversy were discussed in the text. Fundamental concepts were summarized in three main issues: sports may accelerate ACM phenotype with either structural and/or arrhythmic features, restriction may soften the progression, and these rules also apply to phenotype-negative mutation carriers. Additionally, remaining gaps in the current knowledge were also highlighted, namely, the applicability of those fundamental concepts to non-classical ACM phenotypes since left dominant ACM or non-plakophillin-2 genotypes were absent or very poorly represented in the available studies. Hopefully, future research endeavors will provide solid evidence about the safest exercise dose for each patient from a personalized medicine perspective, taking into account a big batch of genetic, epigenetic, and epidemiological variables, for instance, in order to assist clinicians to provide a final tailored recommendation.
Introduction
The classical definition of arrhythmogenic cardiomyopathy (ACM) refers to a rare genetic disease resulting in myocardial loss and fibrofatty substitution of the ventricular myocardium, involving either right, left, or both ventricles (1, 2) and often presenting inflammatory infiltrates (1) (Figure 1). However, in the last years a broader definition of the disease has been proposed to include under this umbrella term also other acquired and genetic pathological entities which share a primary myocardial involvement and a clinical presentation with arrhythmias such as myocarditis, sarcoidosis, amyloidosis, sarcomeric, and mitochondrial defects (3). From now onward, this review is focused only on the original definition of ACM whose reported incidence is 1:5,000 in general population (3).
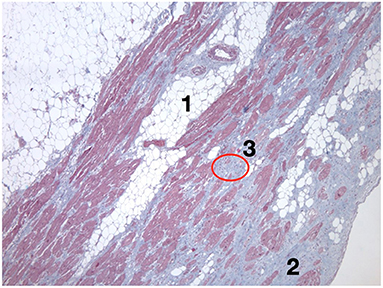
Figure 1. Histological view of the left ventricle of a heart with biventricular arrhythmogenic cardiomyopathy due to the TMEM43 S358L mutation. This patient suffered a sudden cardiac death while practicing sports. The histological hallmarks that define the disease are shown, such as myocardial loss due to fatty infiltration (1) and fibrosis (2); additionally, lymphocytic infiltrates can be observed (3). TMEM43, transmembrane protein 43.
The genetic basis of ACM has been widely expanded since the initial identification of mutations in the genes encoding desmosomal proteins, and currently other disease-causing non-desmosomal genes have also been recognized (1). Mutations in some genes are prone to present with classical forms of the disease and profound structural and electrical alterations arising from the right ventricle while mutations in other genes tend to involve the left ventricle either in isolation or as the main feature of the disease (Table 1) (1, 3–7). Mutations can be identified in 50% of the probands, and the family screening often confirms an incomplete penetrance and a variable expression of the disease. Thus, other factors are thought to play a relevant role as modulators to explain these clinical findings, including epigenetics, virus, sexual hormones, and sports (3). Importantly, gene elusive patients should not be reassessed as having a non-genetic disease since not all ACM genes are known so far and/or certain types of mutation might not be detected with the technology routinely employed (i.e., big rearrangements could be missed by conventional NGS sequencing without copy number variation analyses).
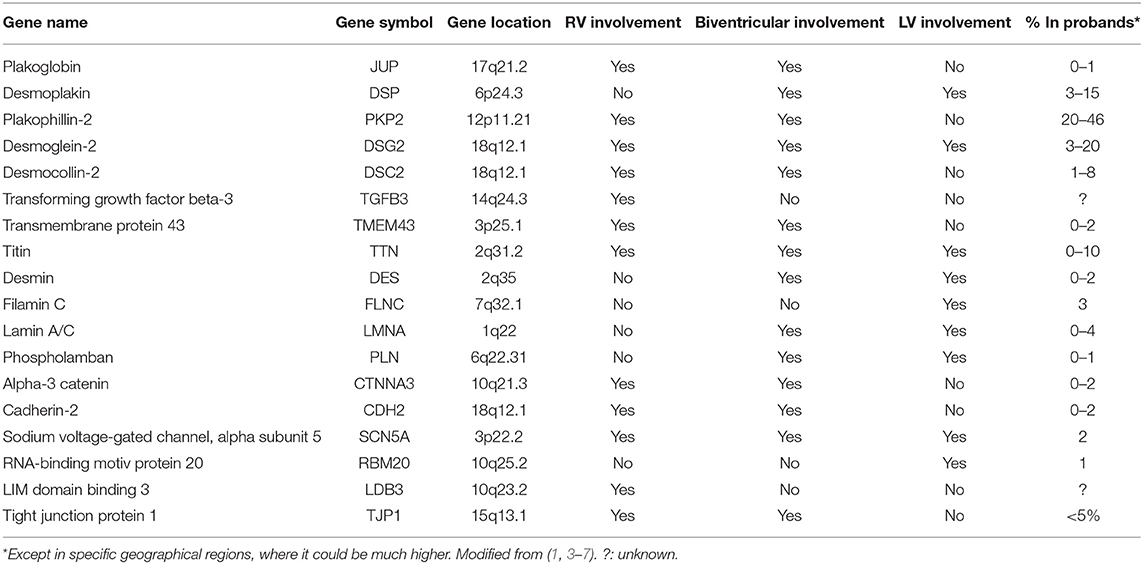
Table 1. List of arrhythmogenic cardiomyopathy-causing genes and the main characteristics of their phenotype.
Mutations in ACM mostly involve desmosomal genes and affect the composition of the intercalated disk. Structural remodeling at the intercalated disks yields subsequent electrical remodeling at the neighboring gap junctions and sodium channels and, furthermore, modifications in nuclear signaling and transcriptional activity mediated by Wnt and Hippo pathways (8, 9). The final myocardial substitution by fibrofatty tissue (Figure 1) provides the macroscopic anatomical substrate for the ventricular arrhythmias that characterize the disease, but also, at a subcellular scenario, the abovementioned gap junction and sodium current remodeling promote patchy slow conduction areas and re-entrant circuits for ventricular arrhythmias (3).
Exercise and sports practice confer beneficial effects on such a wide variety of organs that their recommendation in general population remains out of debate (10). Despite this compelling evidence, in the last years extensive data have been published to support an adverse influence on patients with ACM. Thus, clinical guidelines have accordingly been released to restrict sports recommendations in this population (Table 2) (11), yet a low-intensity exercise is recommended to these patients. A practical advice could be to avoid exercise levels that hamper maintaining a conversation (13).
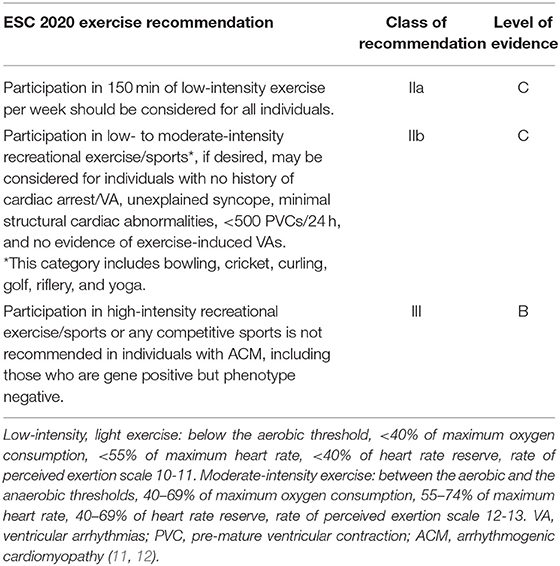
Table 2. Modified exercise recommendations for patients with arrhythmogenic cardiomyopathy included in the European Society of Cardiology guidelines.
Exercise testing is usually included in the routine study protocol triggered by the suspicion of ACM ever since it was recommended in the original Task Force criteria for probands and family members. These consensus documents considered left bundle branch ventricular arrhythmias recorded at different tests (including exercise testing) as a minor criterion for probands and as one of the four additional criteria besides being a first-degree relative for family members (14). A challenging paper demonstrated that exercise testing could unmask depolarization and repolarization abnormalities as well as ventricular arrhythmia in mutation carriers, irrespective of the fulfillment of Task Force criteria and the symptomatic state, suggesting a potential use of this test to prescribe exercise in case it could be validated in that scenario (15). Against this hypothesis, another interesting work compared the arrhythmogenicity response during the isoproterenol and the exercise test in 37 ACM patients and underlined the frequent and falsely reassuring reduction or abolishment of baseline ventricular arrhythmia during the exercise testing (16). Only one paper has assessed the safety and usefulness of cardiopulmonary exercise testing in 38 ACM patients, concluding that it is safe and that the ventilatory efficiency may predict heart transplantation-free survival (17). However, no studies have been carried out focused on the role of exercise and cardiopulmonary exercise testing to prescribe exercise in ACM patients engaged in sports and followed during a suitable period of time. Additionally, one may acknowledge that it is hard to extrapolate the ideal timely situation of these tests performed in a hospital setting with a random training session in which hydration, blood volume, electrolytes, acid–base balance, and catecholamine levels can widely vary and transiently increase the baseline electrical instability in ACM patients necessary to trigger ventricular arrhythmias during sports. Thus, no specific recommendation can be given regarding this issue and the authors strongly advise to adhere the current guidelines (Table 2) designed on the exercise dose without considering the result of any type of exercise testing and based on the body of evidence discussed in the following sections.
From a mechanistic point of view, the pressure overload produced by physical exertion may cause a stronger wall stress and more severe myocardial damage at the right ventricle than at the left ventricle (3, 13, 18). Thus, as long as no other factor played any other role, a more deleterious effect on ACM with right ventricular involvement might be expected than in left dominant forms. Damage may include abnormal signaling promoting apoptosis, fibrosis, and adipogenic and inflammatory cascades (13). The last one might be exaggerated in trained patients with ACM or mutation carriers since prolonged periods of intensive physical training can additionally depress immunity and promote inflammation as well (19).
Herein we present a review of the published evidence regarding exercise and sports practice in ACM patients, highlighting the remaining gaps to be addressed in the future.
Methods
A thorough review of the literature was performed in PubMed by searching papers with the terms “sudden cardiac death (SCD) AND sports/exercise” and “ARVC AND sports/exercise” between 2002 and February 2021 and in English language (Figure 2). This search yielded 1,366 papers, and 15 additional papers were then identified through other searches and/or cited by reviews. Duplicates were eliminated and records screened yielding 36 original papers assessed for eligibility. The information given in these papers was structured in a regular format and presented in Tables, although some data were not available in some papers. We next excluded two papers from the search “SCD AND sports/exercise” because data from SCD cases could not be differentiated from those from cases surviving from sudden cardiac arrests (n = 1) and because the same research group had published another selected paper with a bigger sample size which included the excluded paper (n = 1). At this point of the PRISMA flowchart, some other studies were excluded for quantitative analyses because relevant numerical data were missing but nonetheless were considered suitable for full-text qualitative synthesis and structured in descriptive Tables dealing with preclinical models on ACM and exercise (n = 9) and “ARVC AND sports/exercise” (n = 7). The final quantitative synthesis to analyze the global prevalence of the different causes of sports-related SCD and the quantitative synthesis to estimate the life-threatening arrhythmic events (LAEs) in ACM athletes were performed as follows. Proportions for each diagnosis as the cause of death in sports-related SCD series were extracted from each of the 10 selected studies, and their 95% confidence intervals (CIs) were calculated. Annual LAE rates of ACM athletes were calculated for each of the eight selected studies, and CIs were calculated by using the Poisson distribution. Standardized (5-year) rates of LAE were presented in forest plot graphs, and odds ratio calculations were obtained with a meta-analysis dedicated software [Review Manager (RevMan) 5.4. Copenhagen: The Nordic Cochrane Center, The Cochrane Collaboration, 2020]. Three main sections were prepared, namely, observational studies regarding ACM in sports-triggered sudden cardiac death, preclinical ACM models, and exercise and clinical series analyzing the effect of exercise on ACM. Finally, an additional fourth section supporting the possibility of strenuous exercise as stand-alone cause of ACM was added to the present manuscript.
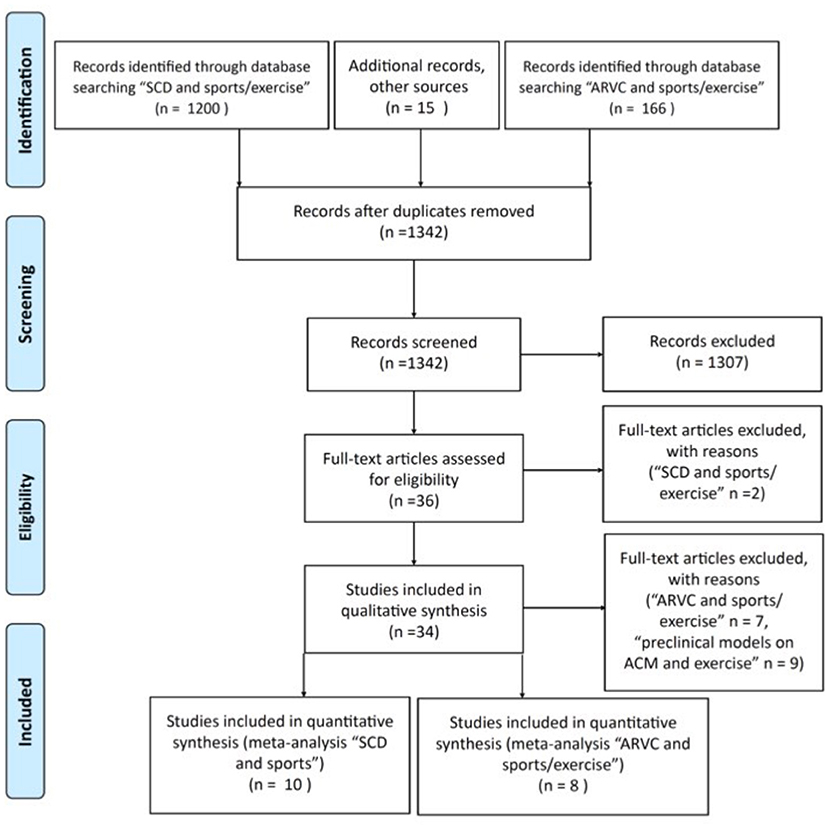
Figure 2. PRISMA flowchart for the identification of papers to obtained pooled data on the causes of sports-related sudden cardiac deaths and on the estimates of life-threatening arrhythmic events in ACM patients engaged in sports. SCD, sudden cardiac death; ARVC, arrhythmogenic cardiomyopathy.
Observational Studies: ACM in Sports-Triggered Sudden Cardiac Death
The causes underlying a heart arrest or an SCD of someone practicing sports have focused the attention of researchers. Published papers have tried to shed some light on the causes of sports-related SCD from a wide range of settings and based on a variable percentage of forensic studies. They have underlined that ACM represents a not negligible cause of death in this scenario, accounting for roughly 0–28% of the autopsied cases (20–29). The fact that in non-autopsy-based studies ACM is sometimes not even mentioned highlights the difficulties in getting a firm diagnosis when cardiac pathology is missing, as it often happens both in SCD and in sudden cardiac arrest reports (29, 30). Moreover, the pathological overlap of ACM, myocarditis, and idiopathic left ventricular fibrosis allows to speculate that some of the cases with those diagnoses should further increase the real percentage of ACM as the cause of death in case a molecular autopsy confirmed this hypothesis. Supplementary Table 1 summarizes the main papers reporting the cause of death in sports-related SCD series. As expected, the percentage of SCD attributable to coronary artery disease (CAD) increases paralleling the age of the recruited victims, with a maximal prevalence (63%) in the Spanish study by Morentin et al. (n = 288) which also reported male predominance and the highest mean age (44 ± 14 years old) of the reviewed series (28). For unknown reasons, even though Spain is considered a country with low prevalence of CAD, its 63% CAD prevalence (28) is double as much as that observed in the Danish series reported by Risgaard et al., with a similar age (41 ± 10 years old) and also with male predominance but with a remarkably smaller sample size (N = 44).
Pooled data obtained from papers in Supplementary Table 1 are shown in Figure 3 with the global estimates of the prevalence of CAD, hypertrophic cardiomyopathy, ACM, and sudden arrhythmic death syndrome in these series. Our data show that CAD and hypertrophic cardiomyopathy should be considered the leader causes of sports-related SCD, although in the youngest subsets of victims inherited cardiac conditions such as hypertrophic cardiomyopathy, ACM, and sudden arrhythmic death syndrome clearly prevail (20, 21, 24, 27). Right after CAD and hypertrophic cardiomyopathy, sudden arrhythmic death syndrome represents the third global cause of death in sports-related SCD. As cardiogenetic knowledge and resources continue to grow, more molecular autopsies will be hopefully performed and thus specifically ascribe these deaths to certain syndromes such as long QT syndrome, Brugada syndrome, and catecholaminergic polymorphic ventricular tachycardia. Taking into account the prevalence in general population of hypertrophic cardiomyopathy and ACM (1:500 vs. 1:5,000, respectively) and our global estimates for them in sports-related SCD series (roughly 20 and 10%, respectively), another important remark is that the risk for developing a sports-related SCD in ACM patients is five-fold that of patients with hypertrophic cardiomyopathy. In keeping with these data, in 72% of 66 ACM cases recruited after a sudden cardiac death or a sudden cardiac arrest, events occurred on exertion (31). Although the genetic background of the geographical area in which the studies were performed and the implementation of pre-participation screening may have influenced the final outcomes, it appears clear that ACM is overrepresented in sports-triggered sudden cardiac death.
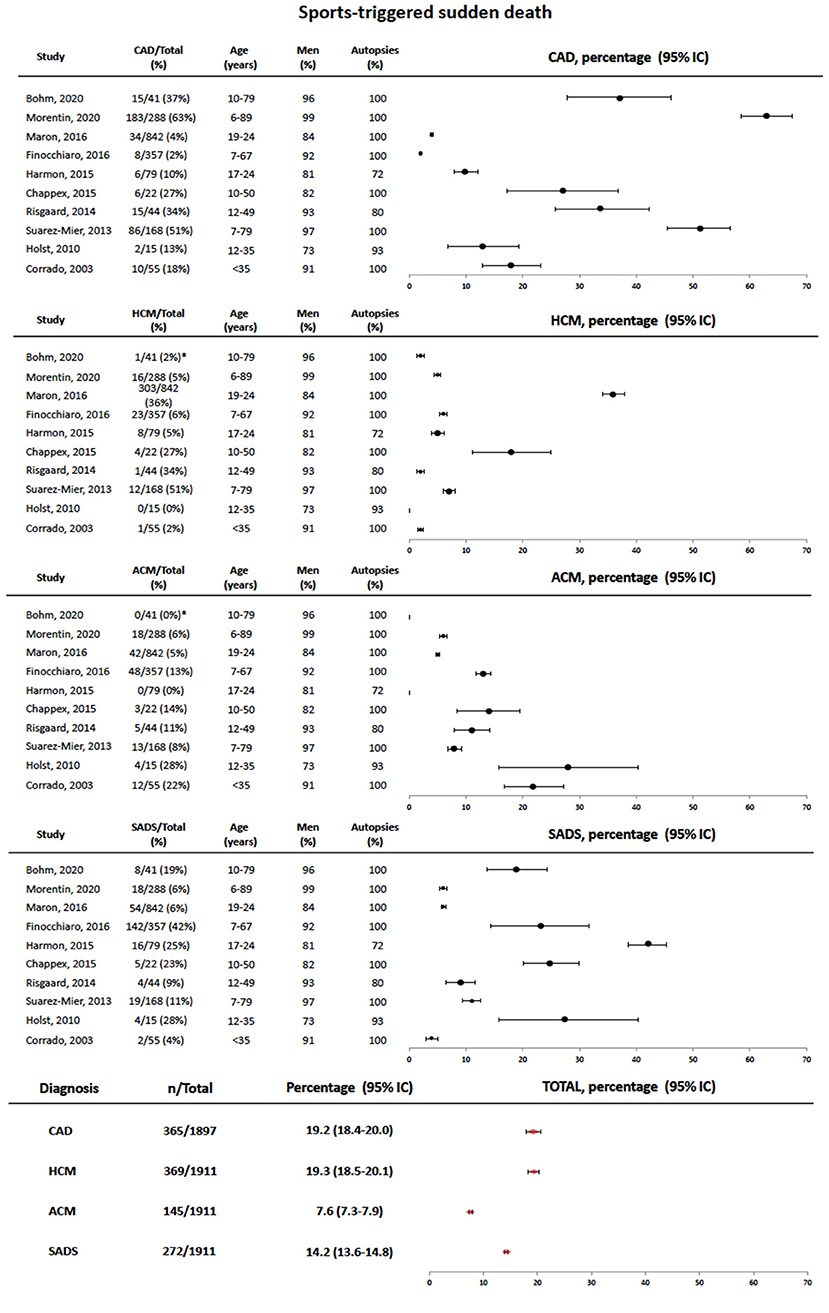
Figure 3. Forest plot showing pooled data of the causes of sports-triggered sudden cardiac death in different series referenced in the text. At the bottom, total estimates are provided for each diagnosis. SCD, sudden cardiac death; CAD, coronary artery disease; SADS, sudden arrhythmic death syndrome; HCM, hypertrophic cardiomyopathy; ACM, arrhythmogenic cardiomyopathy. *The causes of death can be only retrieved form the 41 cases of sports-related sudden death cases with autopsy.
Preclinical ACM Models and Exercise
Animal models provide a useful setting to test hypotheses that cannot easily be tested in in vitro cultures, such as the global effect of exercise in an organism in terms of development of both arrhythmic and structural burden. Table 3 updates the major findings of the effect of exercise in several animal models of ACM. Additionally, a paper based on cell cultures assuming stress shear as a subrogate of exercise has been incorporated.
In summary, trained heterozygous transgenic mice suffered adverse cardiac remodeling. All in all, experiments with plakoglobin, plakophillin-2, and desmoplakin transgenic animals revealed a pro-arrhythmic remodeling with an impaired cardiac electrical conduction and an altered expression of Ca2+-handling-related proteins, with controversial results with respect to development of structural abnormalities in this scenario, as summarized in Table 3. The great differences in the selected genetic background, the study protocols, and their endpoints foreclose precise comparisons between these studies and/or data processing to obtain pooled data with meta-analysis strategies. Slowed conduction was probably the consequence of the C×43 dyslocalization and reduced sodium current (33, 36) and the substrate for ventricular arrhythmias and increased inducibility (32–34, 39). Additionally, exercise-triggered development of fibrosis, apoptosis, chamber enlargement, and systolic dysfunction was reported by some authors (32, 33, 36–38) but not by others (39). Interestingly, pretreatment with preload-reducing therapy (furosemide and nitrates) softened the ACM phenotype after exercise, in terms of both structural and electrical abnormalities (33). Moreover, similar results to these were observed with intervention to downregulate the canonical Wnt pathway by inhibiting GSK3b with SB216763, either in sedentary and exercised mutant mice (38) or in cell cultures exposed to shear stress (35). Thus, in keeping with the current pathophysiology of ACM, down-expression of the Wnt pathway does play a key role in ACM development under exercise and wall stress conditions, which challenges the desmosome integrity and the electrical stability. In contrast, only one paper supported a somehow beneficial effect of exercise on ACM. Indeed, it showed a restoration of the abnormal baseline expression pattern in the left ventricular myocardium of DSP-mutant mice upon exercise, so that it reduced myocardial apoptosis and induced eccentric cardiac hypertrophy without affecting cardiac function or arrhythmia susceptibility (40) with a similar protocol to that used in another study also on DSP-mutant mice which yielded opposite results (37). Maybe the slight differences in the age of the animals, the details of the exercise treadmill protocol, or the different DSP mutations (deletion vs. missense) could account for part of these discrepancies which, anyhow, lay out of the scope of this review and could be clarified with future studies assessing this issue.
Clinical Series Analyzing the Effect of Exercise on ACM
In line with the previous sections of this manuscript regarding the causes of sports-associated SCD and the results on exercised ACM animal models, also clinicians have gathered a valuable piece of evidence by analyzing clinical series of ACM patients engaged in sports. Shown in Supplementary Table 2 is a list of observational studies which have reported outcomes in clinical series.
Taken all together, a definite deleterious effect of exercise on the structural and the arrhythmic phenotype of ACM has been proved. Some studies focus their interest on sports training defined in terms of intensity and duration (either competitive or recreational), but others broaden the analysis to physical activity in general. Some interesting series have helped to define the specific risk of physical activity in mutation carriers not exhibiting an overt ACM phenotype, concluding that exercise also promotes disease progression in this scenario (41, 42). Others have assessed the effect of exercise restriction to find out an improvement in outcomes (43, 44) regardless of the previous level of training (45). Although more exercise causes more electrical and/or structural progression (41, 43, 46–48), the intensity rather than the duration of the exercise performed seems to play a more determinant role. Indeed, intensity rather than duration is strongly linked to adverse prognosis (49, 50) and, furthermore, its reduction more effectively improves the arrhythmic burden (44). Also in the scenario of ACM patients with ablation for ventricular tachycardia, exercise activity was an independent risk factor for future LAEs (51, 52). Aiming to give a quantitative recommendation to patients, several authors suggested that <2.5 h per week and <6 METS both in probands and relatives (50) or <650 MET-Hr/years (metabolic equivalent hours obtained from multiplying METs by duration) in relatives (49) could be safe and healthy. Accordingly, a precise recommendation has been introduced in current guidelines (Table 2). From an ambiguous qualitative perspective, one paper highlighted that recreational but not competitive sports may be safe in ACM patients since their practice does not aggravate prognosis in comparison to inactive patients (53). However, reports on LAEs triggered by recreational sports in ACM patients (54) and the wide range of the intensity of recreational sports hamper the extrapolation of this result to everyday practice so that sports quantification in terms of intensity and duration is preferred over a recreational vs. competitive sports classification. Finally, the hypothesis of the interaction of environmental and genetic factors on the final ACM phenotype remarkably gathers strength in this scenario. Indeed, the more weight of the environment (sports), the least influence of genetics and, vice versa; highly lethal mutations entail such a poor prognosis that there is little room left for environmental influence on the final phenotype. Thus, patients with a negative genetic result appear to have the greatest influence of sports on their structural and electric ACM phenotype (42), whereas in carriers of the dangerous TMEM43 S358L mutation, the impact of exercise on prognosis fades out by increasing the threshold up to 9 MET-Hr/day (3,285 MET-Hr/year) in order to find significant differences in LAEs (55).
Suitable papers from Supplementary Table 2 were further processed to integrate all those series in a meta-analysis with stronger evidence. First, we acknowledge certain limitations in data collection derived from the great differences observed in the design of the studies. There were also differences in assessing the sport performed, sometimes before recruitment, others before and after, and occasionally only the sport performed after enrollment was used to classify patients. As observed in Figure 4, our pooled data confirm that ACM patients engaged in high-intensity sports activities have a significant two-fold increase in the risk of developing LAE when compared to ACM patients who report lower physical activity habits (OR 2.1, 95% CI 1.43–3.8). Unfortunately, we have not been able to compare the age of the LAEs in both groups, but, as observed in some of the reviewed papers, it was significantly younger in the sports-engaged ACM patients. Our meta-analysis approach to evaluate the risk of LAE in ACM patients depending on their sports practice is brand new and provides strong and compelling evidence to reassure the need of sports restriction in this scenario. Furthermore, our systematic review of all these papers has allowed us to highlight that the vast majority of patients exhibited a classical phenotype (definite Task Force Criteria 2010), that the representation of patients harboring non-PKP2 mutations was really small, and that the percentage of patients with left ventricular involvement remains widely unknown. Thus, clinicians should be aware that little evidence now support any exercise recommendation out of the clinical profile of the papers herein reviewed.
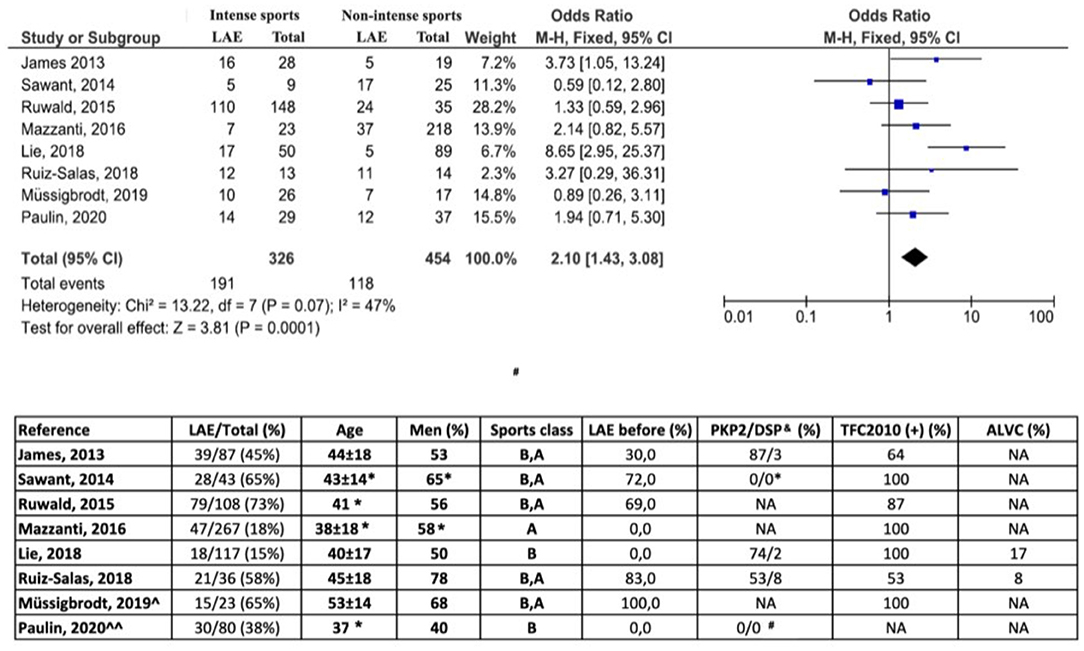
Figure 4. Forest plot represents standardized (5-year) rate of life-threatening arrhythmic events (LAE) and 95% CI from eight selected studies referenced in the text. Odds ratios for intense vs. non-intense exercise were calculated per study. Pooled data represented in the bottom black diamond (Review Manager 5.4). Bottom table summarizes relevant extracted demographic and clinical data from the eight selected articles. N, number; Y, years; FU, follow-up; TFC2010, Definite Task Force Criteria for arrhythmogenic right ventricular cardiomyopathy; ALVC, arrhythmogenic left ventricular cardiomyopathy; ∧VT ablation series, ∧∧ICD series, primary prophylaxis. Sports class: sports classification depending on physical activity before (B)/after (A) recruitment. &Data referred to the total sample size referred at the second column, even though some patients may not have had genetic studies performed and, if done, others may not have mutation identified. *Figure approximated from the data given in the paper. #All carriers of the mutation TMEM43 S358L. NA, not available.
Strenuous Exercise as Stand-Alone Cause of ACM
Strenuous exercise may induce cardiac adaptation in the so-called athlete heart. Further maladaptive remodeling may lead to develop features resembling ACM at one or both ventricles.
Endurance athletes are exposed to myocardial damage as a direct consequence of their high-level training with a subsequent rise in blood BNP, CK-MB, troponin-T, and troponin-I (56). Additionally, athletes exhibit an increased risk of right ventricular remodeling and ventricular arrhythmias typically arising from the right ventricle, yielding a variable percentage of individuals fulfilling ACM Task Force Criteria 2010, up to 59% definite and 30% borderline/possible by some authors (57) or 57% borderline/possible in other series (58). However, these athletes have been proved to have a lower than expected rate of mutation-positive studies (13% in athletes vs. 50% reported in papers concerning ACM in general) (59). Thus, the so-called exercise-induced ACM was proposed to explain the occasional development of a phenotype identical to classical ACM in endurance athletes (mostly cyclists) (56), sometimes with subepicardial right ventricular outflow tract scar as substrate for fast ventricular tachycardia (60), which was then reproduced in a mouse model (61). The incidence of such a cardiac behavior is rare, around 1/1,000 at risk individuals but suspected to be as high as 1/100 in top elite athletes (56). Remarkably, the continuum between sports-triggered right ventricular remodeling and an ACM phenotype, the low incidence of exercise-induced ACM, and the limitation of the yield of the genetic studies (see introduction) preclude an accurate definition of the outcomes of this entity. Thus, current guidelines on sports recommendations (11) do not make any distinction between genetic and suspected exercise-induced ACM in terms of exercise restriction once an overt ACM phenotype is present. Among the clinical series reviewed on the topic “ACM and sports/exercise,” two of them specifically focused on outcomes in gene elusive individuals (41, 46). As previously commented, this group might include both genetic ACM with negative genetic results and sports-induced ACM. Supplementary Table 2 includes a brief summary of these papers showing that, in comparison to gene-positive individuals, gene-negative patients need to train harder to develop the ACM phenotype (41) and that sports restriction reduced ventricular arrhythmias in them more often than in gene-positive patients (46).
Finally, also the left ventricle can suffer some sort of exercise-triggered damage identified as non-ischemic subepicardial scars mimicking those observed in left dominant ACM patients, healed myocarditis, or even Fabry disease. Indeed, myocardial fibrosis detected by late gadolinium enhancement resonance imaging has been reported to occur in up to 50% of asymptomatic athletes and veteran triathletes, mostly at meso/epicardial inferolateral left ventricular walls, mostly in men, and associated with higher blood pressure, myocardial mass, and longer cumulative distances (62–65). A cycling race distance of >1,880 km completed during competition had the highest accuracy to predict late gadolinium enhancement (63). On the contrary, other clinical series did not find any late gadolinium enhancement in endurance athletes (58) so that the real prevalence of this feature unfortunately remains widely unknown.
Conclusions
Arrhythmogenic cardiomyopathy accounts for roughly 10% of all sports-related SCDs which implies a five-fold risk of suffering an SCD in comparison to that of patients with hypertrophic cardiomyopathy based on sports-related SCD series. The young age of the athletes and certain specific geographical regions may profoundly increase these estimates. Despite the beneficial effect of exercise in general population, physical activity promotes the onset and aggravates the structural and electrical features of ACM both in preclinical models and in clinical series. The very few exceptions of papers reporting conflicting results may account for differences in the design of the studies. Our pooled data based on previously published studies confirm that high-intensity sport is associated with a two-fold increase in the risk of LAE. The promising preclinical data which support a beneficial effect of drug intervention to lower preload may open avenues in the future to partially mitigate the negative impact of sports on ACM patients who, despite international recommendations, decide to maintain their high-intensity physical activities. Already reported evidence shows that the intensity rather than duration of exercise is responsible for this negative effect. Moreover, sports restriction seems to partially improve the phenotype but does not completely blur the risk of structural progression and arrhythmic events in previously trained athletes, so that the decision to implant an ICD should remain independent of their degree of sport engagement. Physical exercise at <6 METS and <2.5 h (150 min) per week could be safe for both ACM patients and mutation carriers.
Remarkably, the knowledge herein reviewed suffer from some challenging limitations (knowledge and gaps are showed in Figure 5).
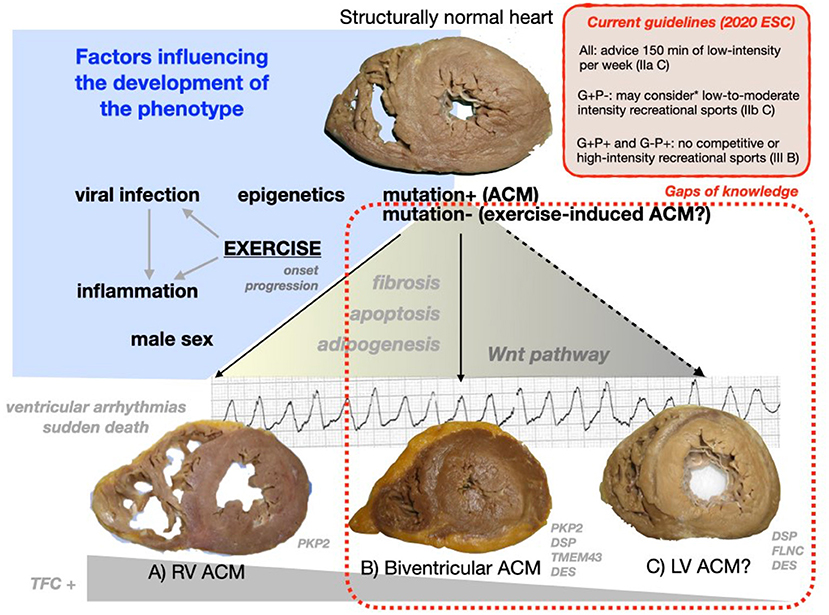
Figure 5. Central figure. The progression of a structurally normal heart harboring a mutation or without any genetic hit to an ACM phenotype involving just the right ventricle (A), both ventricles (B) or the left ventricle in isolation (C). (A–C) Were obtained at autopsies of patients with ACM and sports-triggered sudden cardiac death. Different factors may modulate this transition yielding structural and arrhythmic features through the Wnt pathway dysregulation (as presented in Table 3). The rate of patients fulfilling TFC 2010 and the specific mutated genes underlying may widely differ among ACM phenotypes A–C. The most important genotype–phenotype associations based on the authors clinical and forensic experience are shown at the bottom of this figure (further information on this topic is provided in Table 1). *Only applicable to low risk patients with no history of cardiac arrest/ventricular arrhythmias, unexplained syncope, minimal structural cardiac abnormalities, <500 PVCs/24 h, and no evidence of exercise-induced complex ventricular arrhythmias (11). ACM, arrhythmogenic cardiomyopathy; ESC, European Society of Cardiology; G, genotype; P, phenotype; TFC, Task Force Criteria 2010; LV, left ventricular; RV, right ventricular; PKP2, plakophillin-2; DSP, desmoplakin; DSG2, desmoglein-2; DES, desmin; TMEM43, transmembrane protein 43; FLNC, filamin C; PVCs, premature ventricular complexes.
First, evidence has been gathered in preclinical and clinical series with classical ACM phenotypes often requiring a definite Task Force Criteria 2010 at recruitment and with a high percentage of plakophillin-2 mutations. The clinical characterization of left ventricular involvement was incomplete in most of the available papers and the presence of mutations in genes typically associated with left ventricular phenotype is rare (Supplementary Table 2). Therefore, the global effect of physical activity and the threshold for a safe exercise recommendation in left ventricular ACM and in carriers of non-plakophillin-2 mutations (such as desmoplakin or desmin) have not been yet specifically addressed.
Second, although it has been suggested that strength exercise (predominantly static) might be safer for ACM patients than sports with a high dynamic demand, it has not been specifically studied and proved so far. Sports with a low dynamic and static component include bowling, cricket, curling, golf, riflery, and yoga.
Furthermore, to what extent endurance exercise may induce a right ventricular or left ventricular ACM phenotypes itself or if some sort of genetic background needs to be present remains unknown.
Future research endeavors may hopefully fill in these gaps soon (Figure 2). Personalized medicine will probably take into account a wide range of genetic, epigenetic, and epidemiological variables, to accurately assist clinicians willing to recommend a safe exercise-practice to ACM patients.
Data Availability Statement
Publicly available datasets were analyzed in this study. This data can be found here: we have reviewed the available papers on sports and arrhythmogenic cardiomyopathy to show the evidence in a systematic approach to the readers. No original dataset have been used for this article.
Author Contributions
JM-S and JS-M reviewed the papers regarding the effect of sport on ACM in clinical series, prepared Supplementary Table 2, and edited the manuscript. PM and EZ performed the Pubmed search, reviewed the papers regarding the sports-triggered SCD, prepared Supplementary Table 1, selected the pictures included in Figures 1, 5 from her personal archive, and edited the manuscript. JG, MS-M, and AB-B reviewed the papers regarding the pre-clinical evidence of sports on ACM, prepared the Table 3, and edited the manuscript. LM-D reviewed the current knowledge about the genetic background of ACM, prepared Tables 1, 2, and edited the manuscript. JG and EZ prepared the data from the papers to perform the meta-analyses. JG and MS-M performed the statistical analyses. MS-M built the Forest plots and draw the Figures 3, 4. EZ conceived the review manuscript, supervised the tables, figures, wrote, and edited the manuscript. All authors contributed to the article and approved the submitted version.
Funding
This work was in part supported by grants from Instituto de Salud Carlos III and FEDER Union Europea, Una forma de hacer Europa (PI18/01582, PI18/01231).
Conflict of Interest
The authors declare that the research was conducted in the absence of any commercial or financial relationships that could be construed as a potential conflict of interest.
Publisher's Note
All claims expressed in this article are solely those of the authors and do not necessarily represent those of their affiliated organizations, or those of the publisher, the editors and the reviewers. Any product that may be evaluated in this article, or claim that may be made by its manufacturer, is not guaranteed or endorsed by the publisher.
Supplementary Material
The Supplementary Material for this article can be found online at: https://www.frontiersin.org/articles/10.3389/fcvm.2021.702560/full#supplementary-material
References
1. Elliott PM, Anastasakis A, Asimaki A, Basso C, Bauce B, Brooke MA, et al. Definition and treatment of arrhythmogenic cardiomyopathy: an updated expert panel report. Eur J Heart Fail. (2019) 21:955–64. doi: 10.1002/ejhf.1534
2. Corrado D, Marra MP, Zorzi A, Beffagna G, Cipriani A, De Lazzari M, et al. Diagnosis of arrhythmogenic cardiomyopathy: the Padua criteria. Int J Cardiol. (2020) 319:106–14. doi: 10.1016/j.ijcard.2020.06.005
3. Bennett RG, Haqqani HM, Berruezo A, Della Bella P, Marchlinski FE, Hsu CJ, et al. Arrhythmogenic cardiomyopathy in 2018-2019: ARVC/ALVC or both? Heart Lung Circ. (2019) 28:164–77. doi: 10.1016/j.hlc.2018.10.013
4. Towbin JA, McKenna WJ, Abrams DJ, Ackerman MJ, Calkins H, Darrieux FC, et al. (2019). 2019 HRS expert consensus statement on evaluation, risk stratification, and management of arrhythmogenic cardiomyopathy. Heart Rhythm. 16:e301–72. doi: 10.1016/j.hrthm.2019.05.007
5. Lopez-Ayala JM, Ortiz-Genga M, Gomez-Milanes I, Lopez-Cuenca D, Ruiz-Espejo F, Sanchez-Munoz JJ, et al. A mutation in the Z-line Cypher/ZASP protein is associated with arrhythmogenic right ventricular cardiomyopathy. Clin Gen. (2015) 88:172–6. doi: 10.1111/cge.12458
6. Fedida J, Fressart V, Charron P, Surget E, Hery T, Richard P, et al. Contribution of exome sequencing for genetic diagnostic in arrhythmogenic right ventricular cardiomyopathy/dysplasia. PLoS ONE. (2017) 12:e0181840. doi: 10.1371/journal.pone.0181840
7. De Bortoli M, Postma AV, Poloni G, Calore M, Minervini G, Mazzotti E, et al. Whole-exome sequencing identifies pathogenic variants in TJP1 gene associated with arrhythmogenic cardiomyopathy. Circ Genom Precision Med. (2018) 11:e002123. doi: 10.1161/CIRCGEN.118.002123
8. Chen SN, Gurha P, Lombardi R, Ruggiero A, Willerson JT, Marian AJ. The hippo pathway is activated and is a causal mechanism for adipogenesis in arrhythmogenic cardiomyopathy. Circ Res. (2014) 114:454–68. doi: 10.1161/CIRCRESAHA.114.302810
9. Garcia-Gras E, Lombardi R, Giocondo MJ, Willerson JT, Schneider MD, Khoury DS, et al. Suppression of canonical Wnt/β-catenin signaling by nuclear plakoglobin recapitulates phenotype of arrhythmogenic right ventricular cardiomyopathy. J Clin Investig. (2006) 116:2012–21. doi: 10.1172/JCI27751
10. Sharma S, Merghani A, Mont L. Exercise and the heart: the good, the bad, the ugly. Eur Heart J. (2015) 36:1445–53. doi: 10.1093/eurheartj/ehv090
11. Pelliccia A, Sharma S, Gati S, Bäck M, Börjesson M, Caselli S, et al. 2020 ESC Guidelines on sports cardiology and exercise in patients with cardiovascular disease: the Task Force on sports cardiology and exercise in patients with cardiovascular disease of the European Society of Cardiology (ESC). Eur Heart J. (2021) 42:17–96. doi: 10.1093/eurheartj/ehaa605
12. De Innocentiis C, Ricci F, Khanji MY, Aung N, Tana C, Verrengia E, et al. Athlete's heart: diagnostic challenges and future perspectives sports medicine. Sports Med. (2018) 48:2463–77. doi: 10.1007/s40279-018-0985-2
13. Prior D, La Gerche A. Exercise and arrhythmogenic right ventricular cardiomyopathy. Heart Lung Circ. (2020) 29:547–55. doi: 10.1016/j.hlc.2019.12.007
14. Marcus FI, McKenna WJ, Sherrill D, Basso C, Bauce B, Bluemke DA, et al. Diagnosis of arrhythmogenic right ventricular cardiomyopathy/dysplasia: proposed modification of the Task Force Criteria. Eur Heart J. (2010) 31:806–14. doi: 10.1093/eurheartj/ehq025
15. Perrin MJ, Angaran P, Laksman Z, Zhang H, Porepa LF, Rutberg J, et al. Exercise testing in asymptomatic gene carriers exposes a latent electrical substrate of arrhythmogenic right ventricular cardiomyopathy. J Am Coll Cardiol. (2013) 62:1772–9. doi: 10.1016/j.jacc.2013.04.084
16. Denis A, Sacher F, Derval N, Martin R, Lim HS, Pambrun T, et al. Arrhythmogenic response to isoproterenol testing vs. exercise testing in arrhythmogenic right ventricular cardiomyopathy patients. Europace. (2018) 20:f30–6. doi: 10.1093/europace/euy007
17. Scheel PJ III, Florido R, Hsu S, Murray B, Tichnell C, James CA. Safety NA, and utility of cardiopulmonary exercise testing in arrhythmogenic right ventricular cardiomyopathy/dysplasia. J Am Heart Assoc. (2020) 9:e013695. doi: 10.1161/JAHA.119.013695
18. La Gerche A, Rakhit DJ, Claessen G. Exercise and the right ventricle: a potential Achilles' heel. Cardiovasc Res. (2017) 113:1499–508. doi: 10.1093/cvr/cvx156
19. Simpson RJ, Kunz H, Agha N, Graff R. Exercise and the regulation of immune functions. Prog Mol Biol Transl Sci. (2015) 135:355–80. doi: 10.1016/bs.pmbts.2015.08.001
20. Corrado D, Basso C, Rizzoli G, Schiavon M, Thiene G. Does sports activity enhance the risk of sudden death in adolescents and young adults? J Am Coll Cardiol. (2003) 42:1959–63. doi: 10.1016/j.jacc.2003.03.002
21. Holst AG, Winkel BG, Theilade J, Kristensen IB, Thomsen JL, Ottesen GL, et al. Incidence and etiology of sports-related sudden cardiac death in Denmark-implications for preparticipation screening. Heart Rhythm. (2010) 7:1365–71. doi: 10.1016/j.hrthm.2010.05.021
22. Suárez-Mier MP, Aguilera B, Mosquera RM, Sánchez-de-León MS. Pathology of sudden death during recreational sports in Spain. Foren Sci Int. (2013) 226:188–96. doi: 10.1016/j.forsciint.2013.01.016
23. Risgaard B, Winkel BG, Jabbari R, Glinge C, Ingemann-Hansen O, Thomsen JL, et al. Sports-related sudden cardiac death in a competitive and a noncompetitive athlete population aged 12 to 49 years: data from an unselected nationwide study in Denmark. Heart Rhythm. (2014) 11:1673–81. doi: 10.1016/j.hrthm.2014.05.026
24. Harmon KG, Asif IM, Maleszewski JJ, Owens DS, Prutkin JM, Salerno JC, et al. Incidence, cause, and comparative frequency of sudden cardiac death in national collegiate athletic association athletes: a decade in review. Circulation. (2015) 132:10–9. doi: 10.1161/CIRCULATIONAHA.115.015431
25. Chappex N, Schlaepfer J, Fellmann F, Bhuiyan ZA, Wilhelm M, Michaud K. Sudden cardiac death among general population and sport related population in forensic experience. J Foren Legal Med. (2015) 35:62–8. doi: 10.1016/j.jflm.2015.07.004
26. Finocchiaro G, Papadakis M, Robertus JL, Dhutia H, Steriotis AK, Tome M, et al. Etiology of sudden death in sports: insights from a united kingdom regional registry. J Am Coll Cardiol. (2016) 67:2108–15. doi: 10.1016/j.jacc.2016.02.062
27. Maron BJ, Haas TS, Ahluwalia A, Murphy CJ, Garberich RF. Demographics and epidemiology of sudden deaths in young competitive athletes: from the United States National Registry. Am J Med. (2016) 129:1170–7. doi: 10.1016/j.amjmed.2016.02.031
28. Morentin B, Suárez-Mier MP, Monzó A, Ballesteros J, Molina P, Lucena J. Sports-related sudden cardiac death in Spain. A multicenter, population-based, forensic study of 288 cases. Revista Española Cardiol. (2021) 74:225–32. doi: 10.1016/j.rec.2020.05.044
29. Bohm P, Scharhag J, Egger F, Tischer KH, Niederseer D, Schmied C, et al. Sports-Related sudden cardiac arrest in Germany. Can J Cardiol. (2021) 37:105–12. doi: 10.1016/j.cjca.2020.03.021
30. Landry CH, Allan KS, Connelly KA, Cunningham K, Morrison LJ, Dorian P. Sudden cardiac arrest during participation in competitive sports. N Engl J Med. (2017) 377:1943–53. doi: 10.1056/NEJMoa1615710
31. Gupta R, Tichnell C, Murray B, Rizzo S, Te Riele A, Tandri H, et al. Comparison of features of fatal versus nonfatal cardiac arrest in patients with arrhythmogenic right ventricular dysplasia/cardiomyopathy. Am J Cardiol. (2017) 120:111–7. doi: 10.1016/j.amjcard.2017.03.251
32. Kirchhof P, Fabritz L, Zwiener M, Witt H, Schäfers M, Zellerhoff S, et al. Age- and training-dependent development of arrhythmogenic right ventricular cardiomyopathy in heterozygous plakoglobin-deficient mice. Circulation. (2006) 114:1799–806. doi: 10.1161/CIRCULATIONAHA.106.624502
33. Fabritz L, Hoogendijk MG, Scicluna BP, Van Amersfoorth SC, Fortmueller L, Wolf S, et al. Load-reducing therapy prevents development of arrhythmogenic right ventricular cardiomyopathy in plakoglobin-deficient mice. J Am Coll Cardiol. (2011) 57:740–50. doi: 10.1016/j.jacc.2010.09.046
34. Lyon RC, Mezzano V, Wright AT, Pfeiffer E, Chuang J, Banares K, et al. Connexin defects underlie arrhythmogenic right ventricular cardiomyopathy in a novel mouse model. Hum Mol Genet. (2014) 23:1134–50. doi: 10.1093/hmg/ddt508
35. Hariharan V, Asimaki A, Michaelson JE, Plovie E, MacRae CA, Saffitz JE, et al. Arrhythmogenic right ventricular cardiomyopathy mutations alter shear response without changes in cell-cell adhesion. Cardiovasc Res. (2014) 104:280–9. doi: 10.1093/cvr/cvu212
36. Cruz FM, Sanz-Rosa D, Roche-Molina M, García-Prieto J, García-Ruiz JM, Pizarro G, et al. Exercise triggers ARVC phenotype in mice expressing a disease-causing mutated version of human plakophilin-2. J Am Coll Cardiol. (2015) 65:1438–50. doi: 10.1016/j.jacc.2015.01.045
37. Martherus R, Jain R, Takagi K, Mendsaikhan U, Turdi S, Osinska H, et al. Accelerated cardiac remodeling in desmoplakin transgenic mice in response to endurance exercise is associated with perturbed Wnt/β-catenin signaling. Am J Physiol Heart Circ Physiol. (2016) 310:H174–87. doi: 10.1152/ajpheart.00295.2015
38. Chelko SP, Asimaki A, Andersen P, Bedja D, Amat-Alarcon N, DeMazumder D, et al. Central role for GSK3β in the pathogenesis of arrhythmogenic cardiomyopathy. JCI Insight. (2016) 1:85923. doi: 10.1172/jci.insight.85923
39. van Opbergen CJ, Noorman M, Pfenniger A, Copier JS, Vermij SH, Li Z, et al. Plakophilin-2 haploinsufficiency causes calcium handling deficits and modulates the cardiac response towards stress. Int J Mol Sci. (2019) 20:4076. doi: 10.3390/ijms20174076
40. Cheedipudi SM, Hu J, Fan S, Yuan P, Karmouch J, Czernuszewicz G, et al. Exercise restores dysregulated gene expression in a mouse model of arrhythmogenic cardiomyopathy. Cardiovasc Res. (2020) 116:1199–213. doi: 10.1093/cvr/cvz199
41. Saberniak J, Hasselberg NE, Borgquist R, Platonov PG, Sarvari SI, Smith HJ, et al. Vigorous physical activity impairs myocardial function in patients with arrhythmogenic right ventricular cardiomyopathy and in mutation positive family members. Eur J Heart Fail. (2014) 16:1337–44. doi: 10.1002/ejhf.181
42. Sawant AC, Bhonsale A, te Riele AS, Tichnell C, Murray B, Russell SD, et al. Exercise has a disproportionate role in the pathogenesis of arrhythmogenic right ventricular dysplasia/cardiomyopathy in patients without desmosomal mutations. J Am Heart Assoc. (2014) 3:e001471. doi: 10.1161/JAHA.114.001471
43. James CA, Bhonsale A, Tichnell C, Murray B, Russell SD, Tandri H, et al. Exercise increases age-related penetrance and arrhythmic risk in arrhythmogenic right ventricular dysplasia/cardiomyopathy-associated desmosomal mutation carriers. J Am Coll Cardiol. (2013) 62:1290–7. doi: 10.1016/j.jacc.2013.06.033
44. Wang W, Orgeron G, Tichnell C, Murray B, Crosson J, Monfredi O, et al. Impact of exercise restriction on arrhythmic risk among patients with arrhythmogenic right ventricular cardiomyopathy. J Am Heart Assoc. (2018) 7:e008843. doi: 10.1161/JAHA.118.008843
45. Müssigbrodt A, Czimbalmos C, Stauber A, Bertagnolli L, Bode K, Dagres N, et al. Effect of exercise on outcome after ventricular tachycardia ablation in arrhythmogenic right ventricular dysplasia/cardiomyopathy. Int J Sports Med. (2019) 40:657–62. doi: 10.1055/a-0962-1325
46. Ruiz Salas A, Barrera Cordero A, Navarro-Arce I, Jiménez Navarro M, García Pinilla JM, Cabrera Bueno F, et al. Impact of dynamic physical exercise on high-risk definite arrhythmogenic right ventricular cardiomyopathy. J Cardiovasc Electrophysiol. (2018) 29:1523–9. doi: 10.1111/jce.13704
47. Lie ØH Rootwelt-Norberg C, Dejgaard LA, Leren IS, Stokke MK, Edvardsen T. Prediction of life-threatening ventricular arrhythmia in patients with arrhythmogenic cardiomyopathy: a primary prevention cohort study. JACC Cardiovasc Imag. (2018) 11:1377–86. doi: 10.1016/j.jcmg.2018.05.017
48. Costa S, Gasperetti A, Medeiros-Domingo A, Akdis D, Brunckhorst C, Saguner AM, et al. Familial arrhythmogenic cardiomyopathy: clinical determinants of phenotype discordance and the impact of endurance sports. J Clin Med. (2020) 9:3781. doi: 10.3390/jcm9113781
49. Sawant AC, Te Riele AS, Tichnell C, Murray B, Bhonsale A, Tandri H, et al. Safety of American Heart Association-recommended minimum exercise for desmosomal mutation carriers. Heart Rhythm. (2016) 13:199–207. doi: 10.1016/j.hrthm.2015.08.035
50. Lie ØH, Dejgaard LA, Saberniak J, Rootwelt C, Stokke MK, Edvardsen T, et al. Harmful effects of exercise intensity and exercise duration in patients with arrhythmogenic cardiomyopathy. JACC Clin Electrophysiol. (2018) 4:744–53. doi: 10.1016/j.jacep.2018.01.010
51. Mazzanti A, Ng K, Faragli A, Maragna R, Chiodaroli E, Orphanou N, et al. Arrhythmogenic right ventricular cardiomyopathy: clinical course and predictors of arrhythmic risk. J Am Coll Cardiol. (2016) 68:2540–50. doi: 10.1016/j.jacc.2016.09.951
52. Lin CY, Chung FP, Kuo L, Lin YJ, Chang SL, Lo LW, et al. Characteristics of recurrent ventricular tachyarrhythmia after catheter ablation in patients with arrhythmogenic right ventricular cardiomyopathy. J Cardiovasc Electrophysiol. (2019) 30:582–92. doi: 10.1111/jce.13853
53. Ruwald AC, Marcus F, Estes NM III, Link M, McNitt S, Polonsky B, et al. Association of competitive and recreational sport participation with cardiac events in patients with arrhythmogenic right ventricular cardiomyopathy: results from the North American multidisciplinary study of arrhythmogenic right ventricular cardiomyopathy. Eur Heart J. (2015) 36:1735–43. doi: 10.1093/eurheartj/ehv110
54. Catto V, Dessanai MA, Sommariva E, Tondo C, Dello Russo A. S-ICD is effective in preventing sudden death in arrhythmogenic cardiomyopathy athletes during exercise. Pacing Clin Electrophysiol. (2019) 42:1269–72. doi: 10.1111/pace.13702
55. Paulin FL, Hodgkinson KA, MacLaughlan S, Stuckless SN, Templeton C, Shah S, et al. Exercise and arrhythmic risk in TMEM43 p. S358L arrhythmogenic right ventricular cardiomyopathy. Heart Rhythm. (2020) 17:1159–66. doi: 10.1016/j.hrthm.2020.02.028
56. Heidbuchel H, Prior DL, La Gerche A. Ventricular arrhythmias associated with long-term endurance sports: what is the evidence? Br J Sports Med. (2012) 46(Suppl. 1):i44–50. doi: 10.1136/bjsports-2012-091162
57. Heidbüchel H, Hoogsteen J, Fagard R, Vanhees L, Ector MJ, Ector H, et al. High prevalence of right ventricular involvementin endurance athletes with ventricular arrhythmias: role of an electrophysiologic study in risk stratification. Eur Heart J. (2003) 24:1473–80. doi: 10.1016/S0195-668X(03)00282-3
58. Zaidi A, Sheikh N, Jongman JK, Gati S, Panoulas VF, Carr-White G, et al. Clinical differentiation between physiological remodeling and arrhythmogenic right ventricular cardiomyopathy in athletes with marked electrocardiographic repolarization anomalies. J Am Coll Cardiol. (2015) 65:2702–11. doi: 10.1016/j.jacc.2015.04.035
59. La Gerche A, Robberecht C, Kuiperi C, Nuyens D, Willems R, De Ravel T, et al. Lower than expected desmosomal gene mutation prevalence in endurance athletes with complex ventricular arrhythmias of right ventricular origin. Heart. (2010) 96:1268–74. doi: 10.1136/hrt.2009.189621
60. Venlet J, Piers SR, Jongbloed JD, Androulakis AF, Naruse Y, den Uijl DW, et al. Isolated subepicardial right ventricular outflow tract scar in athletes with ventricular tachycardia. J Am Coll Cardiol. (2017) 69:497–507. doi: 10.1016/j.jacc.2016.11.041
61. Benito B, Gay-Jordi G, Serrano-Mollar A, Guasch E, Shi Y, Tardif JC, et al. Cardiac arrhythmogenic remodeling in a rat model of long-term intensive exercise training. Circulation. (2011) 123:13–22. doi: 10.1161/CIRCULATIONAHA.110.938282
62. Pujadas S, Doñate M, Li CH, Merchan S, Cabanillas A, Alomar X, et al. Myocardial remodelling and tissue characterisation by cardiovascular magnetic resonance (CMR) in endurance athletes. BMJ Open Sport Exerc Med. (2018) 4:e000422. doi: 10.1136/bmjsem-2018-000422
63. Tahir E, Starekova J, Muellerleile K, von Stritzky A, Münch J, Avanesov M, et al. Myocardial fibrosis in competitive triathletes detected by contrast-enhanced CMR correlates with exercise-induced hypertension and competition history. JACC Cardiovasc Imag. (2018) 11:1260–70. doi: 10.1016/j.jcmg.2017.09.016
64. Wilson M, O'Hanlon R, Prasad S, Deighan A, Macmillan P, Oxborough D, et al. Diverse patterns of myocardial fibrosis in lifelong, veteran endurance athletes. J Appl Physiol. (2011) 110:1622–6. doi: 10.1152/japplphysiol.01280.2010
Keywords: sports, exercise, arrhythmogenic cardiomyopathy, disease progression, risk factors
Citation: Martínez-Solé J, Sabater-Molina M, Braza-Boïls A, Santos-Mateo JJ, Molina P, Martínez-Dolz L, Gimeno JR and Zorio E (2021) Facts and Gaps in Exercise Influence on Arrhythmogenic Cardiomyopathy: New Insights From a Meta-Analysis Approach. Front. Cardiovasc. Med. 8:702560. doi: 10.3389/fcvm.2021.702560
Received: 29 April 2021; Accepted: 09 September 2021;
Published: 18 October 2021.
Edited by:
Fabrizio Ricci, University of Studies G. D'Annunzio Chieti and Pescara, ItalyReviewed by:
Franz Gerald Greil, University of Texas Southwestern Medical Center, United StatesMaria Aurora Morales, National Research Council (CNR), Italy
Copyright © 2021 Martínez-Solé, Sabater-Molina, Braza-Boïls, Santos-Mateo, Molina, Martínez-Dolz, Gimeno and Zorio. This is an open-access article distributed under the terms of the Creative Commons Attribution License (CC BY). The use, distribution or reproduction in other forums is permitted, provided the original author(s) and the copyright owner(s) are credited and that the original publication in this journal is cited, in accordance with accepted academic practice. No use, distribution or reproduction is permitted which does not comply with these terms.
*Correspondence: Esther Zorio, em9yaW9fZXN0JiN4MDAwNDA7Z3ZhLmVz
†These authors share first authorship
‡These authors share second authorship